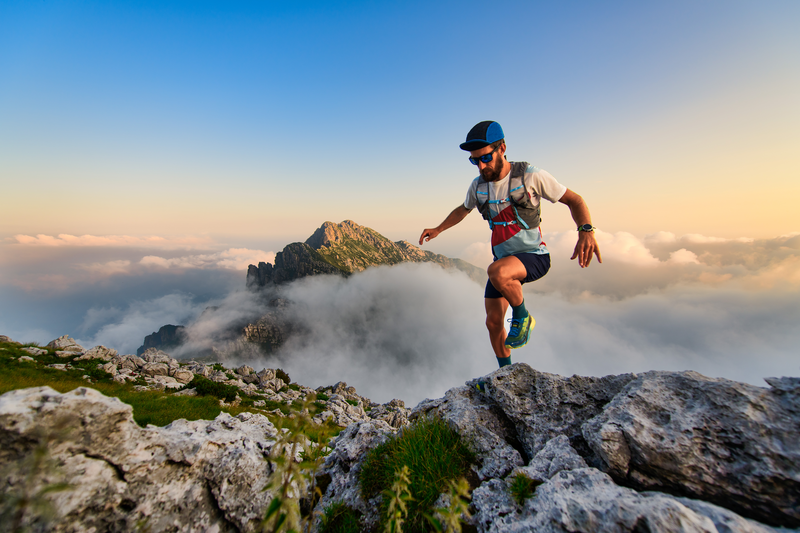
94% of researchers rate our articles as excellent or good
Learn more about the work of our research integrity team to safeguard the quality of each article we publish.
Find out more
ORIGINAL RESEARCH article
Front. Microbiol. , 11 December 2020
Sec. Food Microbiology
Volume 11 - 2020 | https://doi.org/10.3389/fmicb.2020.608314
This article is part of the Research Topic Bacteriocin-Producing Probiotic Bacteria: A Natural Solution for Increasing Efficiency and Safety of Livestock Food Production View all 5 articles
An Enterococcus durans strain, designated OSY-EGY, was previously isolated from artisanal cheese. In this work, comparative genomic and phenotypic analyses were utilized to assess the safety characteristics and probiotic traits of the bacterium. The comparative genomic analysis revealed that the strain is distantly related to potentially pathogenic Enterococcus spp. The genome was devoid of genes encoding acquired antibiotic resistance or marker virulence factors associated with Enterococcus spp. Phenotypically, the bacterium is susceptible to vancomycin, ampicillin, tetracycline, chloramphenicol, and aminoglycosides and does not have any hemolytic or gelatinase activity, or cytotoxic effect on Caco-2 cells. Altogether, these findings confirm the lack of hazardous traits in E. durans OSY-EGY. Mining E. durans OSY-EGY genome, for probiotic-related sequences, revealed genes associated with acid and bile salts tolerance, adhesion, competitiveness, antioxidant activitiy, antimicrobial activity, essential amino acids production, and vitamins biosynthesis. Phenotypically, E. durans OSY-EGY was tolerant to acidic pH (3.0), and presence of 0.3% bile salts. The bacterium showed adhesion capability to Caco-2 cells, cholesterol-lowering effect, DPPH scavenging activity, and antimicrobial activity against several Gram-positive pathogenic bacteria. Based on the current work, we propose that E. durans OSY-EGY is a potentially safe strain with desirable probiotic and antimicrobial traits. Thus, the investigated strain could be a promising candidate for several industrial applications.
Enterococci are non-spore forming bacteria with Gram-positive cell wall and fermentative metabolism (Ludwig et al., 2009). They are characterized by their tolerance to heat, salinity and extreme pH (Hanchi et al., 2018). Enterococci could be found in fermented food products such as cheeses, sausages, olives and vegetables (Franz et al., 1999; Giraffa, 2002). The occurrence of enterococci in food has been viewed for long time as an indicator of poor manufacturing practice and product contamination (Thompson and Marth, 1986; Halkman and Halkman, 2014); however, other researchers consider enterococci to have a minimum role as a hygienic index in the food industry (Birollo et al., 2001). Presence of enterococci in raw-milk-cheeses at populations up to 108 CFU/g suggests their commensal nature or their role as natural starter culture (Giraffa et al., 1997). These bacteria may have a role in ripening, aroma development and shelf-life improvement of fermented foods (Foulquié Moreno et al., 2006). Some strains produce enterocins, which are antimicrobial peptides active against some foodborne pathogens and spoilage bacteria (Khan et al., 2010).
Desirable probiotic characteristics have been reported in some enterococcal strains; these include their ability to inhabit human and animals gastrointestinal tract (GIT) and effectively participate in developing gut microbiome (Dominguez-Bello et al., 2010). Enterococcal strains also may exert their probiotic functions throughout immune system regulation, antioxidant activity, maintaining normal intestinal microflora, and lowering cholesterol levels (Franz et al., 2011). When ingested in high numbers, some enterococci may improve remission of irritable bowel syndrome or antibiotic-associated diarrhea (Gaggìa et al., 2010; Kreuzer et al., 2012). Enterococcus faecalis and E. faecium were used as commercial probiotics or feed additives (Franz et al., 2011) whereas other species such as E. durans and E. hirae were proposed for such use (Nami et al., 2014, 2019; Pieniz et al., 2014). Examples of commercial enterococci probiotic preparations are E. faecium NCIMB 10415(SF68), used in Cernivet® (Cerbios-Pharma SA, Switzerland) and Cylactin® (Hoffmann-La Roche, Basel, Switzerland), and E. faecalis Symbioflor® 1 used in Symbioflor 1 (Symbiopharm, Herborn, Germany) (Serio et al., 2010; Baccouri et al., 2019).
Despite these beneficial uses, some enterococcal strains have been associated with human diseases and nosocomial infections. These strains can cause bacteremia, urinary tract infection, endocarditis, surgical site infection, and diarrhea (Murray and Weinstock, 1999; Kayser, 2003; Klare et al., 2003; Werner et al., 2011). Potentially pathogenic enterococci have been associated with hospital infections, but these are rarely associated with infections outside healthcare settings (Sanders et al., 2010). It has been proposed that the use of broad spectrum antibiotics, catheters, immune-suppressors or chemotherapy affects host-microbe balance and facilitates infection with some enterococci, mostly E. faecalis and E. faecium (Murray, 2000; Ubeda et al., 2010). Potentially pathogenic enterococci usually carry clinically relevant antibiotic resistances and marker virulence factors (Ike, 2017). Considering these findings, Enterococcus spp. are neither recommended for the “qualified presumption of safety” list of biological agents by the European Food Safety Authority (EFSA) nor have the generally recognized as safe (GRAS) status from the US Food and Drug Administration (Hanchi et al., 2018). For distinction between commensal/beneficial and potentially pathogenic enterococcal strains, comprehensive assessment of their safety characteristics is necessary (EFSA, 2012a,b).
The rise of antimicrobial resistance in foodborne pathogens and the need for natural food bio-preservatives prompted the search for new strains capable of producing potent antimicrobial agents. E. durans OSY-EGY, used in this study was isolated from artisanal cheese and showed a promising antimicrobial activity against selected pathogenic species (Hussein et al., 2019). The current study was initiated to thoroughly explore the safety, antimicrobial and probiotic traits of E. durans OSY-EGY and its potential usefulness to the industry.
E. durans OSY-EGY was streaked on de Man, Rogosa and Sharpe (MRS) agar (Oxoid, Thermo scientific, Waltham, MA, United States), and its overnight subculture in MRS broth was mixed with 80% sterile glycerol in a 1:1 ratio and stocked at −80°C. In preparation for experiments, frozen stock culture was streaked on MRS agar and inoculated plates were incubated at 30°C for 48 h followed by cultivation in MRS broth at 30°C for 18 h. E. faecalis Symbioflor 1 strain was isolated from Symbioflor® 1 commercial preparation (SymbioPharm, Herborn, Germany), by streaking on MRS agar and incubation at 30°C for 48 h followed by cultivation in MRS broth at 30°C for 18 h. The control strains, Bacillus cereus ATCC 14579, Escherichia coli K12, Staphylococcus aureus ATCC 25923, E. faecalis ATCC 29212 and E. coli BL21-pET22 (+) (Invitrogen, Carlsbad, CA, United States), Listeria monocytogenes Scott A, and S. aureus ATCC 29213 were obtained from the culture collection of the food microbiology laboratory, at the Ohio State University (Columbus, OH, United States).
For safety assessment, E. durans OSY-EGY genome (Hussein et al., 2019) was screened using the Comprehensive Antibiotic Resistance Database (CARD) (Jia et al., 2017) and Virulence Factor of Bacterial Pathogens Database (VFDB)1 (Chen et al., 2005). Additionally, E. durans OSY-EGY genome was mined for genes associated with biosynthetic pathways of biogenic amines using KAAS-KEGG Automatic Annotation Server2 (Moriya et al., 2007) and KEGG Mapper3 (Kanehisa et al., 2019). The genome was also screened for the presence of Clustered Regularly Interspersed Short Palindromic Repeats (CRISPR) using CRISPR Finder4 (Grissa et al., 2007). For beneficial traits, E. durans OSY-EGY draft genome was mined for genes associated with biosynthetic pathways of essential amino acids and vitamins using KAAS-KEGG Automatic Annotation Server (see text footnote 2) (Moriya et al., 2007) and KEGG Mapper (see text footnote 3) (Kanehisa et al., 2019).
For comparative genomic analysis, the genomic sequences of eleven strains were selected to represent either probiotic or potentially pathogenic enterococci. Group 1, representing probiotic enterococci, included four commercial probiotic strains (E. faecium T110, E. faecium L3, E. faecium LX, and E. faecalis Symbioflor 1) and two potential probiotic strains (E. durans KLDS6.0930 and E. faecium 17OM39). Group 2, representing potentially pathogenic enterococci, included five strains (E. faecium DO, E. faecium 6E6, E. faecium Aus0085, E. faecium Aus0004, and E. faecium E39). The chromosomal genome sequences of these eleven strains were downloaded from the GenBank database using their GenBank accession numbers listed in Table 1. E. durans OSY-EGY draft genome was compared with these selected genomes based on the presence of antibiotic resistance, virulence genes, genes associated with acid tolerance (Ladero et al., 2013; Liu et al., 2016), bile salts tolerance (Budin-Verneuil et al., 2007; Hamon et al., 2011), cellular adhesion (Ladero et al., 2013; Hymes and Klaenhammer, 2016; Liu et al., 2016), competitiveness and persistence (Ghattargi et al., 2018), and antioxidant effects (Li et al., 2018a), retrieved from literature and analyzed using online NCBI BLAST tools; BLASTN for nucleotide sequences (Chen et al., 2013) or TBLASTN for amino acid sequences (Gertz et al., 2006). The presence and absence of genes, in the compared genomes, were visualized as heatmaps constructed using R5.
Table 1. Genomes of Enterococcus durans OSY-EGY and probiotic and potentially pathogenic members of the genus.
To decipher the genomic difference between E. durans OSY-EGY and five genomes of the potentially pathogenic group, BLAST atlas was generated to align the query genomes using E. faecium DO as a reference. The BLAST atlas was drawn using BLAST Ring Image Generator (BRIG) (Alikhan et al., 2011).
Genomes of E. durans OSY-EGY and six potential or commercial probiotic enterococci were additionally compared. BLAST atlas was generated to align the query genomes using E. durans OSY-EGY as a reference. Sequences encoding bacteriocins were searched in the query genomes using the antibiotics and secondary metabolite analysis shell, antiSMASH v4.1.0 (Blin et al., 2018) and the web-based bacteriocin genome mining tool, BAGEL v4.0 (Walsh et al., 2011).
PCR analysis was conducted on E. durans OSY-EGY and E. faecalis Symbioflor 1 to assess the presence of selected enterococcal virulence determinants and genes encoding biogenic amines. The analysis covered the following groups of genes; (i) genes associated with tissue damage that include cylA (cytolysin), gelE (gelatinase), and hyl (hyaluronidase); (ii) genes involved in adhesion and colonization, which include asa1 and agg (aggregation substances), esp (enterococcal surface protein), ace (collagen adhesion protein), and efaA (endocarditis antigen); and (iii) genes encoding biogenic amines, i.e., hdc (histidine decarboxylase), tdc (tyrosine decarboxylase) and odc (ornithine decarboxylase). For Genomic DNA (gDNA) extraction, E. durans OSY-EGY and E. faecalis Symbioflor 1 were grown in MRS broth at 37°C for 16 h. Aliquots (1 ml) of each culture were centrifuged at 10,000 × g for 10 min at 4°C (Model 5415R: Eppendorf, Hauppauge, NY, United States) and cell pellets were washed two times with sterile saline solution. The gDNA was isolated from the cell pellets using genomic DNA purification kit (Wizard® kit; Promega, Madison, WI, United States) following the manufacturer’s protocol. PCR amplification was performed in 30 μL reaction mixture containing 1 μg of gDNA, 1 × PCR buffer, 1.5 mM MgCl2, 0.2 mM of dNTP mix, forward primer, and reverse primer and 2 U Taq DNA polymerase (Platinum®; Thermo Fisher Scientific, Waltham, MA, United States). Genes, corresponding primers, primer concentration, product size and the PCR conditions applied are presented in Table 2. Amplifications were done by a thermal cycler (CFX96 Real-Time System; Bio-Rad, Hercules, CA, United States). Amplicon visualization was done with DNA gel stain (SYBER safe; Invitrogen) under UV light using gel documentation system (ChemiDoc; Bio-Rad).
Table 2. Use of PCR in the detection of virulence and biogenic amines production genes in Enterococcus durans OSY-EGY and E. faecalis Symbioflor 1.
E. durans OSY-EGY was screened for presence of plasmids. E. faecalis Symbioflor 1 was used as negative control (Domann et al., 2007) and Escherichia coli BL21-pET22(+) was used as positive control. E. durans OSY-EGY and E. faecalis Symbioflor 1 were grown in MRS broth at 37°C for 16 h. E. coli was grown in Luria-Bertani broth (Sigma-Aldrich, St. Louis, MO, United States) at 37°C with orbital shaking at 200 rpm for 16 h. Aliquots (1 ml) of each culture were centrifuged at 8,000 × g, for 10 min (Model 55415R; Eppendorf), the supernatants were discarded, and cell pellets were used for the subsequent plasmid isolation. Plasmid DNA extraction was performed using a plasmid extraction kit (PureYieldTM Plasmid Miniprep System; Promega), following the instructions of the manufacturer. DNA gel stain (SYBR Safe; Invitrogen) was used for plasmid visualization under UV light (ChemiDoc; Bio-Rad) for image analysis.
The minimum inhibitory concentration (MIC) of 9 antibiotics (ampicillin, kanamycin, vancomycin, clindamycin, tetracycline, erythromycin, gentamycin, streptomycin, and chloramphenicol) was determined against E. durans OSY-EGY strain using the broth microdilution method (Wikler et al., 2006). Briefly, two-fold serially diluted antibiotics, at final concentrations of 1, 2, 4, 8, 16, 32, 64, 128, 256, 512, and 1,024 μg/ml, were prepared before 50-μl aliquots of the antibiotics’ preparations were added into 96-well plates (Corning, Tewksbury, MA, United States). This was followed by adding equal volume of E. durans OSY-EGY culture suspension that was adjusted to OD600 of 0.001 in Muller Hinton broth (BD Diagnostic, Franklin Lakes, NJ, United States). The MIC of each antibiotic was determined as the lowest concentration at which no growth of the OSY-EGY strain was observed following incubation for 24 h at 37°C. Interpretations for susceptibility to each antibiotic was based on the guidelines adopted by EFSA (2012a). The test was performed in triplicate.
E. durans OSY-EGY was tested for gelatinase production using a method described previously (Su et al., 1991) with some modifications. Briefly, single colony of E. durans OSY-EGY was streaked on nutrient agar (BD Diagnostic) containing 3% gelatin (BD Diagnostic) and incubated at 37°C for 24 h. After incubation, saturated solution of ammonium sulfate (Fisher scientific, Pittsburgh, PA, United States) was poured on the surface of the medium to precipitate the non-hydrolyzed gelatin. Transparent haloes around the colonies were indicative of the gelatinase activity. B. cereus ATCC 14579 was used as a positive control and E. coli K12 was used as a negative control. The test was repeated three times and averages were reported.
Hemolysin activity (Eaton and Gasson, 2001) was tested after growing culture of E. durans OSY-EGY overnight at 37°C on tryptic soy agar supplemented with 5% sheep blood (Remel San Diego, CA, United States). S. aureus ATCC 25923 was used as positive control for β hemolysis and E. faecalis ATCC 29212 was used as negative control. The test was repeated three times and averages were reported.
Human colorectal adenocarcinoma cell line Caco-2 cells (HTB-37TM, American Type Culture Collection, Manassas, VA, United States) were cultured in Dulbecco’s modified Eagle’s medium (DMEM) (Thermo Fisher Scientific) containing 4.5 g/L glucose and supplemented with 15% heat-inactivated fetal bovine serum (FBS), 1% non-essential amino acids, 1% L-glutamine, and 1% penicillin-streptomycin (GibcoTM, Thermo Fisher Scientific). The cells were incubated at 37°C with 5% CO2. Caco-2 cells were grown in T-75 flasks (Corning®, Corning, NY, United States) for 4–6 days until reaching 70–90% confluence, then dispersed using 0.05% trypsin-0.5 mM EDTA (Gibco, Thermo Fisher Scientific). Cells were sub-cultured in the appropriate plates depending on the experiment to be performed. After reaching 100% confluency, the cells were incubated at 37°C for 11–14 days, a period at which they become highly differentiated. The medium was refreshed every second day with fresh DMEM medium. At 100% confluency, the content of the FBS was decreased to 7.5% (v/v).
Caco-2 cells were seeded in 96-well culture plate (Corning®), at a density of 2 × 103 cells/well and incubated as described earlier until post-confluence. DMEM medium was removed and Caco-2 cells were washed with calcium and magnesium-free Dulbecco’s phosphate buffered saline (DPBS) (Gibco, Thermo Fisher Scientific) followed by adding DMEM containing 1% (v/v) FBS.
E. durans OSY-EGY and E. faecalis Symbioflor 1 were cultured in MRS broth at 30°C for 18 h, the cultures were centrifuged at 16,000 × g for 2 min and the resulting cell pellets were washed two times in DPBS. Antibiotic-FBS-free DMEM was used to resuspend and dilute the cell pellets to final cell density of 108 CFU/ml. Caco-2 cells were then exposed to E. durans OSY-EGY or E. faecalis Symbioflor 1 cells and the plate was incubated at 37°C for 24 h. After incubation, the cytotoxicity was evaluated by measuring the activity of lactate dehydrogenase (LDH) released in the culture media by the damaged cells using cytotoxicity detection kit (Roche, Basel, Switzerland) following manufacturer’s recommendations. The final absorbance was measured at 490 nm and 680 nm (test and reference wavelengths, respectively) using a microplate reader (Multiskan GO, Thermo Scientific). The results were expressed as percent of the positive control (Caco-2 cells exposed to 1% of Triton X-100). The Caco-2 cells was also observed using inverted microscope after 3 h of exposure to E. durans OSY-EGY or E. faecalis Symbioflor 1 cells suspended in DMEM to final cell density of 106 CFU/ml, compared to untreated Caco-2 cells.
The acid tolerance of E. durans OSY-EGY was tested as previously described (Ramos et al., 2013) with some modifications. E. durans OSY-EGY was cultured in MRS broth at 30°C for 18 h followed by centrifugation at 16,000 × g for 10 min at 4°C (Model 55415R; Eppendorf). The cell pellets were resuspended in a freshly pH-adjusted MRS. The MRS was adjusted to pH 2.0 and pH 3.0 using 1 N HCl (Fisher Scientific) to mimic the gastric acidic conditions and to pH 7.0 using 1N NaOH (Fisher Scientific) as control. The final cell density was adjusted to approximately 108 CFU/ml. After incubation at 37°C for 2 h, 1-ml samples were taken immediately before and after the incubation, and tenfold serially diluted before spread-plating on MRS agar. After incubation at 30°C for 48 h, the colonies were counted and expressed as Log10 CFU/ml. The assay was performed in duplicate and repeated three times.
For testing the bile tolerance, the previously described method (Walker and Gilliland, 1993) was followed with some modifications. Fresh 18 h culture of E. durans OSY-EGY was inoculated, at final cell density of 108 CFU/ml, in MRS broth supplemented with 0.3% bile salts (Oxgall, Sigma-Aldrich). Cells grown in MRS broth without bile salt was used as control. Culture aliquots, 200 μl each, were distributed into 96-well microplate (Corning) before incubation at 37°C for 9 h in anaerobic jar to simulate human intestinal conditions. Bacterial growth was monitored by measuring the culture’s optical density at 600 nm (OD600) hourly using microplate reader (UV max; Molecular Devices). The measured absorbance values were plotted against the time of incubation and compared with the control. The assays were performed in triplicate and repeated three times.
Caco-2 cells were seeded in 12-well culture plate (Corning®), at a density of 1 × 105 cells/well, and incubated as described earlier until post-confluence. DMEM medium was removed, and the monolayer of Caco-2 cells were washed two times with antibiotic-FBS-free DMEM. E. durans OSY-EGY or E. faecalis Symbioflor 1 were cultured in MRS broth at 30°C for 18 h, cultures were centrifuged at 16,000 × g for 2 min and the cell pellets were washed two times in DPBS. Antibiotic-FBS-free DMEM was used to resuspend and dilute the cell pellets to a final cell density of 106 CFU/ml. The Caco-2 cell were co-cultured with 1 mL of bacterial cell suspension and incubated 37°C for 3 h. The medium was aspirated to remove the non-adhering bacterial cells, and Caco-2 cells were rinsed three times with 1 mL of DPBS. To release attached bacteria, each well was treated with 1 mL of 1% (v/v) Triton X-100 in DPBS and incubated at 4oC for 30 min. The cell suspension including bacterial and Caco-2 cells were recovered from each well, centrifuged at 8,000 × g for 10 min at 4°C and washed two times with 1 mL of DPBS. Finally, the pellet was resuspended in PBS and serial dilutions were used to inoculate MRS agar media followed by incubation at 37oC for 48 h. Bacterial cell adhesion was expressed as percent bacterial CFU attached to Caco-2 cells, relative to the initial bacterial population added per well. The results correspond to the means of two independent experiments, three replicates each.
Auto-aggregation test was performed as described previously (Del Re et al., 2000) with modifications. Overnight cultures of E. durans OSY-EGY and E. faecalis symbioflor 1 were harvested and cell pellets were washed twice with sterile phosphate buffer saline (PBS, pH 7.0) and resuspended in the same buffer to obtain a cell suspension of approximately 108 CFU/ml. Portions (4 ml each) of cell suspensions were incubated at 37°C for 24 h. One-milliliter aliquots of the supernatants at time 0 and 24 h of incubation without agitation were transferred to a spectrophotometer cuvette and absorbance (OD600) at these two points (A0 and A24h, respectively) was measured. The percentage of auto-aggregation was then calculated as follows:
The assay was performed in triplicate.
Hydrophobicity test was performed as initially described (Rosenberg et al., 1980) with some modifications. Overnight cultures of E. durans OSY-EGY and E. faecalis symbioflor 1 were harvested and cell pellets were washed twice with sterile phosphate buffer saline (PBS, pH 7.0) and resuspended in the same buffer to obtain a cell suspension of approximately 108 CFU/ml. One-milliliter of toluene (99.8%; Sigma-Aldrich) was added to 3 ml of each cell suspension, vortexed for 90 s and incubated at 37°C for 1h for phase separation. After incubation, OD600nm of aqueous phase was measured and hydrophobicity was calculated as follows:
where A0 and A are the measured absorbance values before and after mixing with toluene, respectively. The assay was performed in triplicate.
The radical scavenging ability of 2,2-diphenyl-1-picrylhydrazyl (DPPH) was used to evaluate the antioxidant effect (Kedare and Singh, 2011). The assay was performed on E. durans OSY-EGY as described previously (Brand-Williams et al., 1995) with some modifications. The DPPH was freshly prepared in methyl alcohol at a final concentration of 60 μM and transferred to a dark glass bottle. Aliquots (100 μl) of filter-sterilized cell-free supernatant of E. durans OSY-EGY strain were mixed with 3.9 ml of DPPH radical solution. Methyl alcohol was used as a blank. Aliquots (100 μl) of water mixed with 3.9 ml of DPPH solution were prepared as control. The absorbance of the resulting solutions was measured at 515 nm at 5-min intervals for 65 min. The assay was performed in triplicate.
Assimilation of cholesterol was determined as described previously (Choi and Chang, 2015) with slight modifications. Cholesterol (Sigma–Aldrich) was dissolved in 50% ethanol at final concentration of 5mg/ml, followed by filter sterilization using 0.45 μm micro-filter (Fisher Scientific). The soluble cholesterol was added to MRS broth supplemented with 0.3% bile salts, resulting in 0.1 g/l final concentration of cholesterol in MRS broth. Cholesterol-MRS broth was inoculated with E. durans OSY-EGY overnight culture at 108 CFU/ml final cell density before anaerobic incubation at 37°C for 24h. After incubation, aliquots of E. durans OSY-EGY culture was centrifuged at 16,000 × g at 4°C for 5min before the supernatant was transferred for measuring residual cholesterol concentration. Cholesterol-MRS broth without E. durans cells was used as untreated control. Residual cholesterol concentration was determined using o-phthalaldehyde-based method (Rudel and Morris, 1973). Briefly, 1 ml of the supernatant was added to 2 ml of KOH (33%, w/v) and 3mL of 95% ethanol followed by shaking for 1 min and heating at 60°C for 10 min. After cooling in cold water, 5 ml hexane were added, followed by vortexing for 1 min, and adding 1 ml distilled water. The tube was held at ∼22°C for 10 min to allow phase separation followed by transferring 3 ml of the hexane layer into a clean glass tube for evaporation using speed vacuum dryer (Savant AES 2010 Rotary Evaporation System; Savant Inc., Holbrook, NY). Four milliliters of O-phthalaldehyde solution (0.5 mg of O-phthalaldehyde/ml of acetic acid; Sigma–Aldrich) were added to the residual cholesterol, and the mixture was vortexed and held at ∼22°C for 10 min. Subsequently, 2 ml concentrated sulfuric acid were added before incubation for 10 min at 25°C. The absorbance was then measured at 550 nm using a spectrophotometer (Spectronic 20 Genesys; Spectronic Instruments, Inc., Rochester, NY). Cholesterol removal was calculated from the equation:
where As and Ac are the absorbance at 550 nm for E. durans OSY-EGY supernatant and control (un-inoculated Cholesterol-MRS broth), respectively.
The antimicrobial potential of E. durans OSY-EGY was assayed using the previously described spot-on-lawn method (He et al., 2007). Briefly, stock culture of E. durans OSY-EGY was streaked on MRS agar followed by incubation at 30°C for 48 h. Subsequently, a single colony was inoculated in MRS broth followed by incubation at 30°C for 48 h. The obtained fermentate was centrifuged at 7,710 × g for 15 min and the culture supernatant was filter-sterilized using 0.45-μm syringe filter (Millex HV Durapore PVDF; Merk Millipore, Ltd., Cork, IRL). The resulting cell-free supernatant (CFS) was assayed for antimicrobial efficacy against selected bacterial strains (Table 3) as follows. Aliquots (10 μl) of overnight cultures of tested bacteria were transferred into 10 ml sterile molten TSB soft agar (0.75% agar). The inoculated soft agar was overlayed onto basal TSA agar plates. After the soft agar solidified, 10 μl of the CFS were spotted on the indicator lawn. After overnight incubation at 37°C, the clear inhibitory areas, where the CFS was spotted, were observed.
Statistical analysis was performed using R (see text footnote 5). Data were expressed as mean ± standard deviation (SD). Statistical significance was determined using one-way analysis of variance (ANOVA) for comparisons among treatments. Control-treatment comparisons were performed by Student’s t-test and significance was considered at p < 0.05.
A new lactic acid bacterium strain, E. durans OSY-EGY, was isolated from an artisanal cheese and found to have a promising antimicrobial activity that could be beneficial in food applications (Hussein et al., 2019). Before proposing the use of the strain in further industrial applications, research is needed to assess its safety, antimicrobial, and probiotic traits. This study, therefore, was initiated to thoroughly assess these traits using genomic and phenotypic analyses.
The draft genome of E. durans OSY-EGY was mined for genetic determinants associated with safety and probiotic traits. The strain was found lacking genes encoding hyaluronidase (hyl), cytolysin (cylA), gelatinase (gelE) and genes involved in biogenic amines biosynthesis except tyramine decarboxylase (tdc) for tyramine production. For immunity against mobile genetic elements, E. durans OSY-EGY genome was found to contain one confirmed and four potential CRISPR arrays. The genome, however, was found to contain genes involved in biosynthesis of the essential amino acids tryptophan, phenylalanine, methionine, valine, leucine, isoleucine, histidine, arginine and lysine (Table 4) and the vitamins pantothenate, thiamin, riboflavin and folate (Table 5).
Table 4. Essential amino acid and associated biosynthetic proteins detected in Enterococcus durans OSY-EGY genome.
Genomic comparison was performed to reveal the differences between this strain or probiotic or pathogenic enterococci. When screened for the presence of antibiotic resistance genes (Figure 1A), E. durans OSY-EGY and E. durans KLDS6.0930 genomes were found to contain aac(6’)-Iid and LIU_RS08465 genes, which are associated with aminoglycosides and tetracycline resistance, respectively. The genome of the commercial probiotic T110 possessed genes involved in vancomycin, aminoglycosides and macrolides resistance. Genomes of the potentially pathogenic strains (DO, Aus0085, or 6E6) harbored genes conferring resistance to vancomycin, tetracycline, aminoglycosides, and macrolides. When screening for the presence of virulence genes (Figure 1B), E. durans OSY-EGY genome was void of the virulence factors IS16, acm, Scm, SgrA and esp. However, genes associated with adhesion (ebpA, ebpB, ebpC, srtC, EfaA and sagA), pilli formation (pilA, pilB, pilE and pilF) and biofilm formation (bopD) existed in all enterococcal genomes.
Figure 1. Heatmap showing the clustering of the compared Enterococcus spp. genomes based on the presence of (A) antibiotic resistance genes, and (B) virulence genes.
The ability of probiotics to exert health benefits depends on their survival and persistence in the GIT. Genes encoding these traits such as acid and bile tolerance, competitiveness, persistence, and adherence were mined in the compared genomes. All studied genomes encoded acid tolerance, bile tolerance, competitiveness, and persistence genes (Figure 2A). Genes encoding cell adhesion-associated proteins existed in all genomes except those encoding S-layer protein and collagen binding proteins, which were found only in E. durans OSY-EGY and probiotic genomes. The strains OSY-EGY, KLDS6.0930, and T110 contained several genes involved in the biosynthesis of exopolysaccharides. The shortage of these genes in the potentially pathogenic strains may indicate the competitive advantage of the probiotic strains to colonize the GIT (Figure 2B). The E. durans OSY-EGY and KLDS6.0930 were found to share 14 genes associated with antioxidant activities, suggesting their potential ability to survive in the host environment. In comparison, only six genes associated with antioxidant activity were found in the potentially pathogenic genomes (Figure 2C). Detailed information about all genes used for comparison is listed in the Supplementary Tables 1–5. Genome of E. durans OSY-EGY was aligned with those of potentially pathogenic enterococci and results are shown in Figure 3A. This genomic comparison revealed how different and distantly removed is E. durans OSY-EGY from the potentially pathogenic strains. When aligning genomes of E. durans OSY-EGY with probiotic enterococci, it was found that durans KLDS6.0930, a well characterized potential probiotic (Li et al., 2018b), has the highest similarity to E. durans OSY-EGY. Compared to other genomes, E. durans OSY-EGY has the advantage of carrying genes encoding multiple bacteriocins; these are two novel sequences of lantibiotics A and B, enterocin N, and the two previously known enterocins, L50A and L50B (Figure 3B).
Figure 2. Heatmap showing the clustering of the compared Enterococcus spp. genomes based on the presence of (A) genes associated with survival in the GIT, (B) genes associated with adherence to the GIT, and (C) genes associated with antioxidant activity.
Figure 3. Blast Ring Image Generator (BRIG) diagram comparing Enterococcus durans OSY-EGY genome with genomes of (A) potentially pathogenic Enterococcus spp. using complete genome of E. faecium DO as a reference (B) probiotic Enterococcus spp. using draft genome of E. durans OSY-EGY as a reference.
For confirming the in silico analysis of selected virulence factors and biogenic amines production, PCR was conducted on the genomic DNA extracted from OSY-EGY and Symbioflor 1, using primers specific for the corresponding genes (Table 2). The results showed that GelE, cylA, asa1, esp, hdc, odc were absent in E. durans OSY-EGY and Symbioflor 1 genomes. Ace, agg and hyl genes were found only in Symbioflor 1 genome. The genes EfaA and tdc were found in the genomes of both E. durans OSY-EGY and Symbioflor 1 (Table 6), which confirmed the genomic analysis findings.
Table 6. Detection of selected virulence and biogenic amines production genes in the genomes of Enterococcus durans OSY-EGY and E. faecalis Symbioflor 1.
Plasmids include considerable portion of genomic information associated with antibiotic resistance and virulence factors. Plasmid DNA was extracted from E. durans OSY-EGY, Symbioflor 1 and E. coli Pet22b strains followed by visualization using gel electrophoresis. Unlike E. coli Pet22b, E. durans OSY-EGY and Symbioflor 1 were devoid of plasmids (Data are not shown).
The minimum inhibitory concentrations (MICs) for nine antibiotics were determined against E. durans OSY-EGY (Table 7). Results show that the strain is susceptible to all tested antibiotics. The absence of cytolysin and gelatinase genes, revealed from the genomic analysis, was confirmed using the phenotypic assays. Unlike B. cereus ATCC 14579, E. durans OSY-EGY did not exhibit any gelatin hydrolysis (Figure 4A). When E. durans OSY-EGY was grown on blood agar, it did not exhibit any hemolytic effects, compared to S. aureus ATCC 25923 which produced strong β hemolysis (Figure 4A).
Table 7. The minimum inhibitory concentrations of selected antibiotics tested against Eterococcus durans OSY-EGY.
Figure 4. Safety traits of Enterococcus durans OSY-EGY. (A) Gelatinase activity (Left panel) of E. durans OSY-EGY compared to Bacillus cereus ATCC 14579 (positive control) and Escherichia coli k12 (negative control) on nutrient agar containing 3% gelatin and flooded with ammonium sulfate saturated solution. Clear zone indicates gelatin hydrolysis due to gelatinase production. Hemolytic activities (right panel) of E. durans OSY-EGY on blood agar compared to S. aureus ATCC 25923 as positive control and E. faecalis ATCC 29212 as negative control. Clear zone indicates red blood cells lysis due to hemolysin production. (B) Cytotoxic effect of E. durans OSY-EGY or E. Faecalis (Symbioflor 1) strains on Caco-2 cells after 24 h treatments. Values were expressed as means ± SD of two independent repeats. Treatments that were significantly different at P ≤ 0.05 were indicated by different letters. (C) Microscopy observation of Caco-2 cells after 3 h of treatment with E. durans OSY-EGY or E. faecalis Symbioflor 1 compared to untreated Caco-2 cells.
After 24 h of treating Caco-2 cells with E. durans OSY-EGY or E. faecalis Symbioflor 1, the amount of LDH released was measured as an indicator of cytotoxic effect. The results showed that there was no significant difference (p > 0.05), in the LDH release (approximately 5%), between E. durans OSY-EGY, E. faecalis Symbioflor 1, or PBS-treated cells (Figure 4B). The microscopic observation did not show differences in the morphology of treated or untreated Caco-2 cells (Figure 4C).
When E. durans OSY-EGY was exposed to pH 3.0 for 2 h at 37°C, the strain population did not decrease significantly (P > 0.05) as shown in Figure 5A. However, there was a decrease in the population (P < 0.05) after holding E. durans OSY-EGY at pH 2.0 for 2 h. These results indicate that E. durans OSY-EGY can survive acidic conditions as low as pH 3.0. The strain OSY-EGY also grew uninhibited for 8 h in the presence of bile salt at a concentration of 0.3% (p > 0.05), as shown in Figure 5B.
Figure 5. (A) Tolerance of Enterococcus durans OSY-EGY to acidic pH after 2 h of incubation at 37°C. Values were expressed as means ± SD of three repeats and values that were statistically different were indicated by asterisks (∗) if p < 0.05. (B) Tolerance of E. durans OSY-EGY to 0.3% bile salts after 9 h of incubation at 37°C under anaerobic conditions. Values were expressed as means ± SD of three repeats and values that were statistically different were indicated by asterisks (*) if p < 0.05.
OSY-EGY and Symbioflor 1 strains showed similar capabilities to adhere effectively to Caco-2 cells (Figure 6A). For additional adhesion characteristics, OSY-EGY and Symbioflor 1 were evaluated for auto-aggregation capabilities and hydrophobicity. The auto-aggregation capability was not significantly higher in OSY-EGY than in Symbioflor 1 strain (p > 0.05) (Figure 6B). Likewise, after 1 h of incubation with toluene, OSY-EGY strain showed non-significant increase in hydrophobicity than did Symbioflor 1 strain (Figure 6C).
Figure 6. Adhesion capabilities of Enterococcus durans OSY-EGY and E. faecalis Symbioflor 1 strains. (A) Adherence to Caco-2 cells monolayer after 3 h of incubation. (B) Auto-aggregation capabilities after 24 h or incubation at 37°C, and (C) hydrophobicity measured as adhesion percentage to toluene after 1 h of incubation at 37°C. Values were expressed as means ± SD of three repeats.
When evaluating the antioxidant activity, E. durans OSY-EGY showed DPPH radicals scavenging ability of 9% after 65 min of incubation, compared to the initial DPPH concentration (Figure 7A). The strain also had a significantly higher cholesterol lowering potential (p < 0.05), compared to the uninoculated control medium (Figure 7B). The CFS of E. durans OSY-EGY showed antimicrobial activity when tested against selected bacterial stains (Table 3); these include the pathogenic B. cereus, L. monocytogenes, and S. aureus and the spoilage E. faecalis. However, E. durans OSY-EGY was not effective against the Gram-negative E. coli K12.
Figure 7. Antioxidant activity and cholesterol lowering effect of Enterococcus durans OSY-EGY. (A) Antioxidant activity measured by scavenging 2,2-diphenyl-1-picrylhydrazyl (DPPH) radicals. (B) Cholesterol lowering effect of Enterococcus durans OSY-EGY detected after incubation with cholesterol (0.1 g/L) and 0.3% (wt/vol) bile salt. Values were expressed as means ± SD of three repeats and values that were statistically different were indicated by asterisks (∗) if P < 0.05.
Enterococcus spp. are known for their diversity, ubiquitous distribution in the environment, and presence in fermented foods (Ghattargi et al., 2018). There has been an extensive research interest in enterococci as probiotic candidates (Foulquié Moreno et al., 2006; Franz et al., 2011; Nami et al., 2014; Li et al., 2018b; Baccouri et al., 2019). To assess the safety and the industrial application feasibility of a strain, it is important to conduct genomic and phenotypic analyses on traits of interest. Emerging technologies such multi-locus sequence typing (Homan et al., 2002; Leavis et al., 2006; Ruiz-Garbajosa et al., 2006) and whole-genome sequence analyses (van Schaik et al., 2010; Qin et al., 2012; Beukers et al., 2017) have provided evidences that commensal enterococcal strains are different from the nosocomial infectious strains. Some of the genomic variability in Enterococcus spp. is due to the horizontal gene transfer leading to acquisition of mobile and foreign genetic elements such as bacteriophages and insertion sequence (IS) elements (Paulsen et al., 2003; Foulquié Moreno et al., 2006). Considering the potential hazards associated with gene transfer, new Enterococcus strains should be selected for industrial applications based on their genomes characterizations and absence of antibiotic resistance or virulence genes (Domann et al., 2007). A case-by-case safety evaluation assessment is critical to determine strain suitability for use as bio-protective culture or in probiotic preparations (Araújo and Ferreira, 2013; Hanchi et al., 2018).
In the current study, we found that E. durans OSY-EGY has a potent antimicrobial activity against Gram-positive, but not Gram-negative, bacteria. The strain was effective against L. monocytogenes, S. aureus, E. faecalis, and B. cereus and its genome was found to contain multiple bacteriocin biosynthetic gene clusters. Considering its promising antimicrobial activity, E. durans OSY-EGY has a potential to be used as bio-protective culture in some food fermentations. The presence of desirable probiotic characteristics is an additional advantage that may help in qualifying the strain for industrial applications. In terms of fitness and efficacy as a probiotic, antimicrobial activity is traditionally considered an important trait. Some researchers suggested that production of antimicrobials helps probiotic strains in competing against GIT microbes including pathogens (Gillor et al., 2009; Bosák et al., 2018).
In the current study, a genome analysis was conducted to assess the safety and probiotic traits in E. durans OSY-EGY, in comparison with known probiotic and potentially pathogenic Enterococcus strains (Ghattargi et al., 2018). BLAST Atlas revealed that E. durans OSY-EGY is closely related to the potential probiotic strain, KLDS6.0930, and distantly related to the potentially pathogenic group.
The antibiotic resistance is not uncommon in lactic acid bacteria (LAB), including strains proposed as probiotic candidates (Birri et al., 2012; Jeronymo-Ceneviva et al., 2014; Casarotti et al., 2017). In the GIT, there is a high potential for horizontal transfer of acquired antibiotic resistance genes among intestinal microbiota, including human pathogens. However, the horizontal transfer of intrinsic antibiotic resistance genes has a minimum potential (Devirgiliis et al., 2011; van Reenen and Dicks, 2011). EFSA requires the absence of acquired antibiotic resistance genes in bacterial strains, intended to be used in animal feed, as a critical part of its safety qualification (EFSA, 2012a). In silico analysis showed that E. durans OSY-EGY genome encodes genes that could be associated only with tetracycline (LIU_RS08465) and aminoglycoside (aac(6′)-Iid) resistance. In the current study, we screened, in silico, twenty E. durans genomes found in NCBI database for the presence of LIU_RS08465 and aac(6’)-Iid genes in an attempt to determine if these genes are associated with acquired antibiotic resistance. The results showed that these two genes were detected in all analyzed genomes (Supplementary Table 6) which indicates these antibiotic resistance genes are conserved in the genomes of E. durans and are associated with intrinsic resistances, with a low risk for lateral transfer (EFSA, 2012a). Additionally, we investigated the stability of E. durans OSY-EGY genome by analyzing for the presence of plasmids and CRISPER systems. E. durans OSY-EGY was found to be devoid of plasmids and its genome carries one confirmed and three potential CRISPR arrays which confers a sequence-based immunity and genome stability against phage modification and horizontal genes transfer (Marraffini and Sontheimer, 2008). Importantly, the antibiotic susceptibility test revealed that E. durans OSY-EGY is susceptible to clinically relevant antibiotics including ampicillin, vancomycin, tetracycline, and aminoglycosides. Although the commercial probiotic, E. faecalis Symbioflor 1, was used for more than 50 years without reporting of any adverse effect (Domann et al., 2007), it was previously reported to be gentamycin and erythromycin resistant (Baccouri et al., 2019).
According to EFSA guidelines, when the strain is ampicillin sensitive and the virulence genes IS16, esp and hyl are absent, the strain is considered safe to be used as feed additive (EFSA, 2012b); E. durans OSY-EGY genome lacks these markers. Absence of selected virulence genes in E. durans OSY-EGY genome was also verified using PCR. However, the commercial probiotic strain Symbioflor 1 contains the virulence genes, ace and hyl, in its genome. These findings support that E. durans OSY-EGY does not carry any potential pathogenicity. On the other hand, genes associated with adhesion, pilli formation and biofilm formation existed in all enterococcal genomes used for comparison (Figure 1B). The term virulence factor not only refers to elements that confer bacterial pathogenicity and development of infection but also to elements associated with cell adhesion and protection against host defense (Li et al., 2018b). However, factors involved in host cells adhesion and colonization of the intestine are needed in probiotics (Pillar and Gilmore, 2004; Wassenaar et al., 2015; Li et al., 2018b). The European Union recommended that the presence of confirmed known virulence genes in Enterococcus or other LAB strains should exclude these strains from use.
Before selecting new beneficial Enterococcus strain, its hemolytic activity and cytotoxic effect should be evaluated (Salminen et al., 1998). According to current study, E. durans OSY-EGY does not produce gelatinase or hemolysin. LDH assays are widely used for assessing the cytotoxic effect of proposed probiotic strains (Sanders et al., 2010; Hanchi et al., 2018). Using Caco-2 cells, coupled with LDH assay, to assess the cytotoxic effect of E. durans OSY-EGY and E. faecalis Symbioflor 1 showed that both strains had no cytotoxicity to this intestinal cell line. Members of LAB can synthesize biogenic amines from their precursor amino acids by amino acid decarboxylases (Benkerroum, 2016). Biogenic amines, including histamine, tyramine, ornithine, phenylethylamine and cadaverine, may cause intoxication if consumed in amounts that exceed critical thresholds (EFSA, 2011). When the genomes of E. durans OSY-EGY and E. faecalis Symbioflor 1 were screened for the presence of genes associated with biogenic amines production, both strains were void of all investigated genes except that encoding tyrosine decarboxylase, which is involved in tyramine production. Compared to E. durans KLDS6.0930, as the closest strain to OSY-EGY, KLDS6.0930 genome was found to contain the genes for tyramine production. However, KLDS6.0930 strain produced tyramine in amount less than the critical threshold level (Li et al., 2018b). Additionally, presence of the gene does not necessarily mean the protein is expressed; hence, further phenotypic validation of tyramine production needs to be conducted in a further study.
According to the World Health Organization (WHO), probiotics are ‘live microorganisms which when administered in adequate amounts confer a health benefit on the host’ (Food and Agriculture Organization [FAO], and World Health Organization [WHO], 2001). In order to confer such benefits, the probiotic strain should have the capacity to persist and survive under GIT conditions. The strain tolerance to acidic environment and bile salts are two criteria for a candidate probiotic strain to survive the GIT (Nami et al., 2019). In our study, we performed genomic and phenotypic analyses to confirm such traits in E. durans OSY-EGY. Genomically, E. durans OSY-EGY and the potential probiotic strain, KLDS6.0930, contained genes that encode proteins associated with counteracting acid stress such as tyrosine-tRNA ligase (Ladero et al., 2013), ATPase V, Na+/H+ antiporter, H+/K+ uptake transporter (Liu et al., 2016) and Tyrosyl-tRNA synthetase. The expression of the latter increases under low pH, suggesting its potential role in acid stress response (Linares et al., 2012). All Enterococcus genomes tested contain genes associated with bile tolerance, namely, genes encoding choloylglycine hydrolase and cyclopropane-fatty-acyl-phospholipid synthase. Transcription of the latter increased in lactic acid bacteria in response to bile (Budin-Verneuil et al., 2007; Hamon et al., 2011). On the other hand, E. durans OSY-EGY showed acid tolerance when grown at pH 2 or 3 and did not encounter growth reduction in the presence of 0.3% bile salts. This augments the potential capability of the strain to survive similar harsh conditions found in the GIT.
Colonization in the GIT is a critical trait to be considered for a potential probiotic strain. All genomes studied in this work were found to encode for cell adhesion factors such as fibronectin-binding protein (Hymes and Klaenhammer, 2016), aggregation-promoting factor that could be contributing to gut milieu binding (Ladero et al., 2013), collagen-binding protein and proteins associated with extracellular polymeric substance production which enhance adhesion and persistence in the gut environment (Liu et al., 2016). Phenotypically, E. durans OSY-EGY showed adhesion capabilities to Caco-2 cells. Cell surface hydrophobicity is an additional characteristic associated with the adhesion capability of the probiotic strains. The higher ability of probiotics to adhere to epithelial cells is a result of higher cell surface hydrophobicity (Xu et al., 2009). In the current study, E. durans OSY-EGY and E. Faecalis (Symbioflor 1) showed worthy hydrophobicity characteristics (Figure 6C). The auto-aggregation is defined as same-species bacterial accumulation and the trait is important in aiding cellular adhesion (Collado et al., 2008; Campana et al., 2017). In current study, E. durans OSY-EGY and E. Faecalis Symbioflor 1 showed promising auto-aggregation abilities (Figure 6B). Altogether, these results suggest that E. durans OSY-EGY most probably would survive GIT harsh conditions.
Probiotic desirable traits may include production of antioxidants, vitamins, and essential amino acids, and expression of enteric pathogens antagonism. E. durans OSY-EGY and other six genomes were screened for genes known to be associated with antioxidant effect. E. durans OSY-EGY and KLDS6.0930 shared 14 genes coding for antioxidant traits and only 5 genes were found in the potentially pathogenic strains. Genomes of pathogenic strains may include genes associated with GIT survival and even some beneficial characteristics. However, the presence of known virulence factors and antibiotic resistance is what makes these strains pathogenic to the host (Ghattargi et al., 2018). Consistent with its antioxidant characteristic, E. durans OSY-EGY showed DPPH radical scavenging effect (9%). Compared to E. durans OSY-EGY, E. durans F3 showed better DPPH scavenging activity (more than 40%) (Alshammari et al., 2019). This could be attributed to strain-specific differences in their antioxidant activities or the types of the antioxidants produced (Wang et al., 2017). E. durans OSY-EGY genome was found to have genes involved in biosynthetic pathways of essential amino acids and vitamins. The synthesis pathways for vitamins and amino acid were reported previously to be absent in the genomes of the potentially pathogenic strains DO, Aus0085, and 6E6 of E. faecium (Ghattargi et al., 2018). Other health-promoting benefit such as cholesterol lowering effect was observed in E. durans OSY-EGY (Figure 7B). Removal of cholesterol and biosynthesis of vitamins and essential amino acids further support the potential applications of E. durans OSY-EGY in promoting health and possibly in developing nutrient-rich foods. The promising antimicrobial activity of E. durans OSY-EGY against Gram-positive pathogenic and spoilage bacteria, indicates its usefulness for several industrial applications.
Considering the antimicrobial effect of E. durans OSY-EGY, it was important to investigate its safety and to ensure its suitability for use in the industry as bio-protective or probiotic culture. The in silico comparative genomic analysis determined how distant is E. durans OSY-EGY from potentially pathogenic strains, as evident by the absence of antibiotic resistance and virulence genes. The analysis revealed genes associated with desirable probiotic traits such as acid tolerance, bile tolerance, competitiveness, persistence, adherence and health promoting effect. The functionality of the predicted genomic features was validated using a battery of in vitro analyses. From the findings of the current study, it can be concluded that E. durans OSY-EGY is potentially safe and beneficial strain that possesses desirable probiotic traits, in addition to its promising antimicrobial activity. This support the notion that the strain is potentially suitable for use in various applications.
The datasets presented in this study can be found in online repositories. The names of the repository/repositories and accession number(s) can be found in the article/Supplementary Material.
WH analyzed the strain phenotypically and genetically, drafted the manuscript. AA helped with genomic and bioinformatic analyses and contributed to the phenotypic and antibiotic susceptibility testing, and writing/revising the manuscript. DR-M carried out the experiments on cell-line. IG-C helped with the tissue culture work and completed the plasmid isolation experiment. AY oversaw the project and reviewed and revised the manuscript. All authors read and proved the manuscript.
This work was partially funded by the Ohio Agricultural Research and Development Center (OARDC) and by the Center for Advanced Processing and Packaging Studies (CAPPS). WH was supported by a graduate scholarship from the Egyptian Ministry of Higher Education.
The authors declare that the research was conducted in the absence of any commercial or financial relationships that could be construed as a potential conflict of interest.
We thank Dr. Rafael Jiménez-Flores for providing us access to his cell culture laboratory resources.
The Supplementary Material for this article can be found online at: https://www.frontiersin.org/articles/10.3389/fmicb.2020.608314/full#supplementary-material
Alikhan, N. F., Petty, N. K., Ben Zakour, N. L., and Beatson, S. A. (2011). BLAST ring image generator (BRIG): simple prokaryote genome comparisons. BMC Genom. 12:402. doi: 10.1186/1471-2164-12-402
Alshammari, E., Patel, M., Sachidanandan, M., Kumar, P., and Adnan, M. (2019). Potential evaluation and health fostering intrinsic traits of novel probiotic strain Enterococcus durans F3 isolated from the gut of fresh water fish catla catla. Food Sci. Anim. Resour. 39, 844–861. doi: 10.5851/kosfa.2019.e57
Araújo, T. F., and Ferreira, C. L. (2013). The genus Enterococcus as probiotic: safety concerns. Braz. Arch. Biol. Technol. 56, 457–466. doi: 10.1590/S1516-89132013000300014
Baccouri, O., Boukerb, A. M., Farhat, L., Ben, Z. A., Zimmermann, K., Domann, E., et al. (2019). Probiotic potential and safety evaluation of Enterococcus faecalis OB14 and OB15, isolated from traditional Tunisian testouri cheese and rigouta, using physiological and genomic analysis. Front. Microbiol. 10:881. doi: 10.3389/fmicb.2019.00881
Benkerroum, N. (2016). Biogenic amines in dairy products: origin, incidence, and control means. Compr. Rev. Food Sci. Food Saf. 15, 801–826. doi: 10.1111/1541-4337.12212
Beukers, A. G., Zaheer, R., Goji, N., Amoako, K. K., Chaves, A. V., Ward, M. P., et al. (2017). Comparative genomics of Enterococcus spp. isolated from bovine feces. BMC Microbiol. 17:52. doi: 10.1186/s12866-017-0962-1
Birollo, G. A., Reinheimer, J. A., and Vinderola, C. G. (2001). Enterococci vs non-lactic acid microflora as hygiene indicators for sweetened yoghurt. Food Microbiol. 18, 597–604. doi: 10.1006/fmic.2001.0435
Birri, D. J., Brede, D. A., and Nes, I. F. (2012). Salivaricin D, a novel intrinsically trypsin-resistant lantibiotic from Streptococcus salivarius 5M6c isolated from a healthy infant. Appl. Environ. Microbiol. 78, 402–410. doi: 10.1128/AEM.06588-11
Blin, K., Wolf, T., Chevrette, M. G., Lu, X., Schwalen, C. J., Kautsar, S. A., et al. (2018). antiSMASH 4.0-improvements in chemistry prediction and gene cluster boundary identification. Nucleic Acids Res. 45, W36–W41. doi: 10.1093/nar/gkx319
Bosák, J., Micenková, L., Hrala, M., Pomorská, K., Kunova Bosakova, M., Krejci, P., et al. (2018). Colicin FY inhibits pathogenic Yersinia enterocolitica in mice. Sci. Rep. 8:12242. doi: 10.1038/s41598-018-30729-7
Brand-Williams, W., Cuvelier, M. E., and Berset, C. (1995). Use of a free radical method to evaluate antioxidant activity. LWT Food Sci. Technol. 28, 25–30. doi: 10.1016/S0023-6438(95)80008-5
Budin-Verneuil, A., Pichereau, V., Auffray, Y., Ehrlich, D., and Maguin, E. (2007). Proteome phenotyping of acid stress-resistant mutants of Lactococcus lactis MG1363. Proteomics 7, 2038–2046. doi: 10.1002/pmic.200600773
Campana, R., van Hemert, S., and Baffone, W. (2017). Strain-specific probiotic properties of lactic acid bacteria and their interference with human intestinal pathogens invasion. Gut Pathog. 9:12. doi: 10.1186/s13099-017-0162-4
Casarotti, S. N., Carneiro, B. M., Todorov, S. D., Nero, L. A., Rahal, P., and Penna, A. L. B. (2017). In vitro assessment of safety and probiotic potential characteristics of Lactobacillus strains isolated from water buffalo mozzarella cheese. Ann. Microbiol. 67, 289–301. doi: 10.1007/s13213-017-1258-2
Chen, L., Yang, J., Yu, J., Yao, Z., Sun, L., Shen, Y., et al. (2005). VFDB: a reference database for bacterial virulence factors. Nucleic Acids Res. 33, D325–D328. doi: 10.1093/nar/gki008
Chen, Y., Schmidt, B., and Maskell, D. L. (2013). Reconfigurable accelerator for the word-matching stage of BLASTN. IEEE Trans. Very Larg. Scale Integr. Syst. 21, 659–669. doi: 10.1109/TVLSI.2012.2196060
Choi, E. A., and Chang, H. C. (2015). Cholesterol-lowering effects of a putative probiotic strain Lactobacillus plantarum EM isolated from kimchi. LWT Food Sci. Technol. 62, 210–217. doi: 10.1016/J.LWT.2015.01.019
Collado, M. C., Meriluoto, J., and Salminen, S. (2008). Adhesion and aggregation properties of probiotic and pathogen strains. Eur. Food Res. Technol. 226, 1065–1073. doi: 10.1007/s00217-007-0632-x
Del Re, B., Sgorbati, B., Miglioli, M., and Palenzona, D. (2000). Adhesion, autoaggregation and hydrophobicity of 13 strains of Bifidobacterium longum. Lett. Appl. Microbiol. 31, 438–442. doi: 10.1046/j.1365-2672.2000.00845.x
Devirgiliis, C., Barile, S., and Perozzi, G. (2011). Antibiotic resistance determinants in the interplay between food and gut microbiota. Genes Nutr. 6, 275–284. doi: 10.1007/s12263-011-0226-x
Domann, E., Hain, T., Ghai, R., Billion, A., Kuenne, C., Zimmermann, K., et al. (2007). Comparative genomic analysis for the presence of potential Enterococcal virulence factors in the probiotic Enterococcus faecalis strain Symbioflor 1. Int. J. Med. Microbiol. 297, 533–539. doi: 10.1016/J.IJMM.2007.02.008
Dominguez-Bello, M. G., Costello, E. K., Contreras, M., Magris, M., Hidalgo, G., Fierer, N., et al. (2010). Delivery mode shapes the acquisition and structure of the initial microbiota across multiple body habitats in newborns. Proc. Natl. Acad. Sci. U.S.A. 107, 11971–11975. doi: 10.1073/pnas.1002601107
Eaton, T. J., and Gasson, M. J. (2001). Molecular screening of Enterococcus virulence determinants and potential for genetic exchange between food and medical isolates. Appl. Environ. Microbiol. 67, 1628–1635. doi: 10.1128/AEM.67.4.1628-1635.2001
EFSA (2011). EFSA panel on biological hazards (BIOHAZ). Scientific opinion on risk based control of biogenic amine formation in fermented foods. EFSA J. 9:2393. doi: 10.2903/j.efsa.2011.2393
EFSA (2012a). EFSA panel on additives and products or substances used in animal feed (FEEDAP). Guidance on the assessment of bacterial susceptibility to antimicrobials of human and veterinary importance. EFSA J. 10, 2740–2749. doi: 10.2903/j.efsa.2012.2740
EFSA (2012b). EFSA panel on additives and products or substances used in animal feed (FEEDAP). Guidance on the safety assessment of Enterococcus faecium in animal. EFSA J. 10:2682. doi: 10.2903/j.efsa.2012.2682
Food and Agriculture Organization [FAO] and World Health Organization [WHO] (2001). Probiotics in food: health and nutritional properties and guidelines for evaluation. Food Nutr. Pap. 85:71.
Foulquié Moreno, M. R., Sarantinopoulos, P., Tsakalidou, E., and De Vuyst, L. (2006). The role and application of Enterococci in food and health. Int. J. Food Microbiol. 106, 1–24. doi: 10.1016/j.ijfoodmicro.2005.06.026
Franz, C. M. A. P., Holzapfel, W. H., and Stiles, M. E. (1999). Enterococci at the crossroads of food safety? Int. J. Food Microbiol. 47, 1–24. doi: 10.1016/S0168-1605(99)00007-0
Franz, C. M. A. P., Huch, M., Abriouel, H., Holzapfel, W., and Gálvez, A. (2011). Enterococci as probiotics and their implications in food safety. Int. J. Food Microbiol. 151, 125–140. doi: 10.1016/J.IJFOODMICRO.2011.08.014
Gaggìa, F., Mattarelli, P., and Biavati, B. (2010). Probiotics and prebiotics in animal feeding for safe food production. Int. J. Food Microbiol. 141, S15–S28. doi: 10.1016/j.ijfoodmicro.2010.02.031
Geldart, K., and Kaznessis, Y. N. (2017). Characterization of class IIa bacteriocin resistance in Enterococcus faecium. Antimicrob. Agents Chemother. 61:AAC.02033-16. doi: 10.1128/AAC.02033-16
Gertz, E. M., Yu, Y. K., Agarwala, R., Schäffer, A. A., and Altschul, S. F. (2006). Composition-based statistics and translated nucleotide searches: improving the TBLASTN module of BLAST. BMC Biol. 4:41. doi: 10.1186/1741-7007-4-41
Ghattargi, V. C., Gaikwad, M. A., Meti, B. S., Nimonkar, Y. S., Dixit, K., Prakash, O., et al. (2018). Comparative genome analysis reveals key genetic factors associated with probiotic property in Enterococcus faecium strains. BMC Genom. 19:652. doi: 10.1186/s12864-018-5043-9
Gillor, O., Giladi, I., and Riley, M. A. (2009). Persistence of colicinogenic Escherichia coli in the mouse gastrointestinal tract. BMC Microbiol. 9:165. doi: 10.1186/1471-2180-9-165
Giraffa, G. (2002). Enterococci from foods. FEMS Microbiol. Rev. 26, 163–171. doi: 10.1111/j.1574-6976.2002.tb00608.x
Giraffa, G., Carminati, D., and Neviani, E. (1997). Enterococci isolated from dairy products: a review of risks and potential technological use. J. Food Prot. 60, 732–738. doi: 10.4315/0362-028X-60.6.732
Grissa, I., Vergnaud, G., and Pourcel, C. (2007). CRISPRFinder: a web tool to identify clustered regularly interspaced short palindromic repeats. Nucleic Acids Res. 35, W52–W57. doi: 10.1093/nar/gkm360
Halkman, H. B. D., and Halkman, A. K. (2014). “Indicator organisms,” in Encyclopedia of Food Microbiology, 2nd Edn, eds C. A. Batt and M. L. Tortorello (Amsterdam: Elsevier Inc), 358–363. doi: 10.1016/B978-0-12-384730-0.00396-7
Hamon, E., Horvatovich, P., Izquierdo, E., Bringel, F., Marchioni, E., Aoudé-Werner, D., et al. (2011). Comparative proteomic analysis of Lactobacillus plantarum for the identification of key proteins in bile tolerance. BMC Microbiol. 11:63. doi: 10.1186/1471-2180-11-63
Hanchi, H., Mottawea, W., Sebei, K., and Hammami, R. (2018). The genus Enterococcus: between probiotic potential and safety concerns—an update. Front. Microbiol. 9:1791. doi: 10.3389/fmicb.2018.01791
He, Z., Kisla, D., Zhang, L., Yuan, C., Green-Church, K. B., and Yousef, A. E. (2007). Isolation and identification of a Paenibacillus polymyxa strain that coproduces a novel lantibiotic and polymyxin. Appl. Environ. Microbiol. 73, 168–178. doi: 10.1128/AEM.02023-2026
Homan, W. L., Tribe, D., Poznanski, S., Li, M., Hogg, G., Spalburg, E., et al. (2002). Multilocus sequence typing scheme for Enterococcus faecium. J. Clin. Microbiol. 40, 1963–1971. doi: 10.1128/jcm.40.6.1963-1971.2002
Hussein, W. E., Xiaoli, L., and Yousef, A. E. (2019). Draft genome sequence of Enterococcus durans OSY-EGY, a multiple-antimicrobial-peptide producer isolated from Egyptian hard cheese. Microbiol. Resour. Announc. 8:e0303-19.
Hymes, J. P., and Klaenhammer, T. R. (2016). Stuck in the middle: fibronectin-binding proteins in Gram-positive bacteria. Front. Microbiol. 7:1504. doi: 10.3389/fmicb.2016.01504
Ike, Y. (2017). Pathogenicity of Enterococci. Nippon Saikingaku Zasshi 72, 189–211. doi: 10.3412/jsb.72.189
Jeronymo-Ceneviva, A. B., de Paula, A. T., Silva, L. F., Todorov, S. D., Franco, B. D. G. M., and Penna, A. L. B. (2014). Probiotic properties of lactic acid bacteria isolated from water-buffalo mozzarella cheese. Probiot. Antimicrob. Proteins 6, 141–156. doi: 10.1007/s12602-014-9166-2
Jia, B., Raphenya, A. R., Alcock, B., Waglechner, N., Guo, P., Tsang, K. K., et al. (2017). CARD 2017: expansion and model-centric curation of the comprehensive antibiotic resistance database. Nucleic Acids Res. 45, D566–D573. doi: 10.1093/nar/gkw1004
Kanehisa, M., Sato, Y., Furumichi, M., Morishima, K., and Tanabe, M. (2019). New approach for understanding genome variations in KEGG. Nucleic Acids Res. 47, D590–D595. doi: 10.1093/nar/gky962
Kayser, F. H. (2003). Safety aspects of Enterococci from the medical point of view. Int. J. Food Microbiol. 88, 255–262. doi: 10.1016/S0168-1605(03)00188-0
Kedare, S. B., and Singh, R. P. (2011). Genesis and development of DPPH method of antioxidant assay. J. Food Sci. Technol. 48, 412–422. doi: 10.1007/s13197-011-0251-1
Khan, H., Flint, S., and Yu, P. L. (2010). Enterocins in food preservation. Int. J. Food Microbiol. 141, 1–10. doi: 10.1016/J.IJFOODMICRO.2010.03.005
Klare, I., Konstabel, C., Badstübner, D., Werner, G., and Witte, W. (2003). Occurrence and spread of antibiotic resistances in Enterococcus faecium. Int. J. Food Microbiol. 88, 269–290. doi: 10.1016/S0168-1605(03)00190-9
Kreuzer, S., Machnowska, P., Aßmus, J., Sieber, M., Pieper, R., Schmidt, M. F., et al. (2012). Feeding of the probiotic bacterium Enterococcus faecium NCIMB 10415 differentially affects shedding of enteric viruses in pigs. Vet. Res. 43:58. doi: 10.1186/1297-9716-43-58
Ladero, V., Linares, D. M., Del Rio, B., Fernandez, M., Martin, M. C., and Alvarez, M. A. (2013). Draft genome sequence of the tyramine producer Enterococcus durans strain IPLA 655. Genome Announc. 1:e0265-13. doi: 10.1128/genomeA.00265-13
Lam, M. M. C., Seemann, T., Bulach, D. M., Gladman, S. L., Chen, H., Haring, V., et al. (2012). Comparative analysis of the first complete Enterococcus faecium genome. J. Bacteriol. 194, 2334–2341. doi: 10.1128/JB.00259-12
Leavis, H. L., Bonten, M. J., and Willems, R. J. (2006). Identification of high-risk enterococcal clonal complexes: global dispersion and antibiotic resistance. Curr. Opin. Microbiol. 9, 454–460. doi: 10.1016/j.mib.2006.07.001
Li, B., Evivie, S. E., Jin, D., Meng, Y., Li, N., Yan, F., et al. (2018a). Complete genome sequence of Enterococcus durans KLDS6.0933, a potential probiotic strain with high cholesterol removal ability. Gut Pathog. 10:32. doi: 10.1186/s13099-018-0260-y
Li, B., Zhan, M., Evivie, S. E., Jin, D., Zhao, L., Chowdhury, S., et al. (2018b). Evaluating the safety of potential probiotic Enterococcus durans KLDS6.0930 using whole genome sequencing and oral toxicity study. Front. Microbiol. 9:1943. doi: 10.3389/fmicb.2018.01943
Linares, D. M., Fernández, M., Del-Río, B., Ladero, V., Martin, M., and Alvarez, M. A. (2012). The tyrosyl-tRNA synthetase like gene located in the tyramine biosynthesis cluster of Enterococcus durans is transcriptionally regulated by tyrosine concentration and extracellular pH. BMC Microbiol. 12:23. doi: 10.1186/1471-2180-12-23
Liu, F., Li, B., Du, J., Yu, S., Li, W., Evivie, S. E., et al. (2016). Complete genome sequence of Enterococcus durans KLDS6.0930, a strain with probiotic properties. J. Biotechnol. 217, 49–50. doi: 10.1016/J.JBIOTEC.2015.11.012
Ludwig, W., Schleifer, K.-H., and Whitman, W. B. (2009). “Family IV. Enterococcaceae fam. nov.,” in Bergey’s Manual of Systematic Bacteriology, The Firmicutes, 2nd Edn. Vol. 3, eds P. Vos, G. Garrity, D. Jones, N. R. Krieg, W. Ludwig, F. A. Rainey, et al., (New York, NY: Springer), 594.
Marraffini, L. A., and Sontheimer, E. J. (2008). CRISPR interference limits horizontal gene transfer in Staphylococci by targeting DNA. Science 322, 1843–1845. doi: 10.1126/science.1165771
Moriya, Y., Itoh, M., Okuda, S., Yoshizawa, A. C., and Kanehisa, M. (2007). KAAS: an automatic genome annotation and pathway reconstruction server. Nucleic Acids Res. 35, W182–W185. doi: 10.1093/nar/gkm321
Murray, B. E. (2000). Vancomycin-resistant Enterococcal infections. N. Engl. J. Med. 342, 710–721. doi: 10.1056/NEJM200003093421007
Murray, B. E., and Weinstock, G. M. (1999). Enterococci: new aspects of an old organism. Proc. Assoc. Am. Phys. 111, 328–334. doi: 10.1046/j.1525-1381.1999.99241.x
Nami, Y., Abdullah, N., Haghshenas, B., Radiah, D., Rosli, R., and Khosroushahi, A. Y. (2014). Probiotic assessment of Enterococcus durans 6HL and Lactococcus lactis 2HL isolated from vaginal microflora. J. Med. Microbiol. 63, 1044–1051. doi: 10.1099/jmm.0.074161-0
Nami, Y., Vaseghi Bakhshayesh, R., Mohammadzadeh Jalaly, H., Lotfi, H., Eslami, S., and Hejazi, M. A. (2019). Probiotic properties of Enterococcus isolated from artisanal dairy products. Front. Microbiol. 10:300. doi: 10.3389/fmicb.2019.00300
Natarajan, P., and Parani, M. (2015). First complete genome sequence of a probiotic Enterococcus faecium strain T-110 and its comparative genome analysis with pathogenic and non-pathogenic Enterococcus faecium genomes. J. Genet. Genom. 42, 43–46. doi: 10.1016/j.jgg.2014.07.002
Paulsen, I. T., Banerjei, L., Myers, G. S. A., Nelson, K. E., Seshadri, R., Read, T. D., et al. (2003). Role of mobile DNA in the evolution of vancomycin-resistant Enterococcus faecalis. Science 299, 2071–2074. doi: 10.1126/SCIENCE.1080613
Pieniz, S., Andreazza, R., Anghinoni, T., Camargo, F., and Brandelli, A. (2014). Probiotic potential, antimicrobial and antioxidant activities of Enterococcus durans strain LAB18s. Food Control 37, 251–256. doi: 10.1016/j.foodcont.2013.09.055
Pillar, C. M., and Gilmore, M. S. (2004). Enterococcal virulence - pathogenicity island of Enterococcus faecalis. Front. Biosci. 9, 2335–2346. doi: 10.2741/1400
Qin, X., Galloway-Peña, J. R., Sillanpaa, J., Roh, J. H., Nallapareddy, S. R., Chowdhury, S., et al. (2012). Complete genome sequence of Enterococcus faecium strain TX16 and comparative genomic analysis of Enterococcus faecium genomes. BMC Microbiol. 12:135. doi: 10.1186/1471-2180-12-135
Ramos, C. L., Thorsen, L., Schwan, R. F., and Jespersen, L. (2013). Strain-specific probiotics properties of Lactobacillus fermentum, Lactobacillus plantarum and Lactobacillus brevis isolates from Brazilian food products. Food Microbiol. 36, 22–29. doi: 10.1016/J.FM.2013.03.010
Rosenberg, M., Gutnick, D., and Rosenberg, E. (1980). Adherence of bacteria to hydrocarbons: a simple method for measuring cell-surface hydrophobicity. FEMS Microbiol. Lett. 9, 29–33. doi: 10.1111/j.1574-6968.1980.tb05599.x
Rudel, L. L., and Morris, M. D. (1973). Determination of cholesterol using o-phthalaldehyde. J. Lipid Res. 14, 364–371.
Ruiz-Garbajosa, P., Bonten, M. J. M., Robinson, D. A., Top, J., Nallapareddy, S. R., Torres, C., et al. (2006). Multilocus sequence typing scheme for Enterococcus faecalis reveals hospital-adapted genetic complexes in a background of high rates of recombination. J. Clin. Microbiol. 44, 2220–2228. doi: 10.1128/JCM.02596-05
Salminen, S., Ouwehand, A. C., and Isolauri, E. (1998). Clinical applications of probiotic bacteria. Int. Dairy J. 8, 563–572. doi: 10.1016/S0958-6946(98)00077-6
Sanders, M. E., Akkermans, L. M. A., Haller, D., Hammerman, C., Heimbach, J., Hörmannsperger, G., et al. (2010). Safety assessment of probiotics for human use. Gut Microb. 1, 164–185. doi: 10.4161/gmic.1.3.12127
Serio, A., Chaves-López, C., Paparella, A., and Suzzi, G. (2010). Evaluation of metabolic activities of Enterococci isolated from pecorino abruzzese cheese. Int. Dairy J. 20, 459–464. doi: 10.1016/J.IDAIRYJ.2010.02.005
Su, Y. A., Sulavik, M. C., He, P., Makinen, K. K., Makinen, P. L., Fiedler, S., et al. (1991). Nucleotide sequence of the gelatinase gene (gelE) from Enterococcus faecalis subsp. liquefaciens. Infect. Immun. 59, 415–420. doi: 10.1128/iai.59.1.415-420.1991
Suvorov, A., Ermolenko, E., Alechina, G., Chernysh, A., Karaseva, A., Di Pierro, F., et al. (2019). Enterococcus as probiotics: what is the advantage? Nutrafoods 1, 17–25. doi: 10.17470/NF-019-0003
Thompson, T., and Marth, E. (1986). Changes in Parmesan cheese during ripening?: microflora - coliforms, Enterococci, anaerobes, Propionibacteria and Staphylococci. Milchwissenschaft 41, 201–205.
Ubeda, C., Taur, Y., Jenq, R. R., Equinda, M. J., Son, T., Samstein, M., et al. (2010). Vancomycin-resistant Enterococcus domination of intestinal microbiota is enabled by antibiotic treatment in mice and precedes bloodstream invasion in humans. J. Clin. Invest. 120, 4332–4341. doi: 10.1172/JCI43918
van Reenen, C. A., and Dicks, L. M. T. (2011). Horizontal gene transfer amongst probiotic lactic acid bacteria and other intestinal microbiota: what are the possibilities? A review. Arch. Microbiol. 193, 157–168. doi: 10.1007/s00203-010-0668-3
van Schaik, W., Top, J., Riley, D. R., Boekhorst, J., Vrijenhoek, J. E. P., Schapendonk, C. M. E., et al. (2010). Pyrosequencing-based comparative genome analysis of the nosocomial pathogen Enterococcus faecium and identification of a large transferable pathogenicity island. BMC Genomics 11:239. doi: 10.1186/1471-2164-11-239
Walker, D. K., and Gilliland, S. E. (1993). Relationship among bile tolerance, bile salt deconjugation, and assimilation of cholesterol by Lactobacillus acidophilus. J. Dairy Sci. 76, 956–961. doi: 10.3168/jds.S0022-0302(93)77422-6
Walsh, C. J., Guinane, C. M., Hill, C., Ross, R. P., O’toole, P. W., and Cotter, P. D. (2011). In silico identification of bacteriocin gene clusters in the gastrointestinal tract, based on the Human Microbiome Project’s reference genome database. BMC Microbiol. 15:183. doi: 10.1186/s12866-015-0515-4
Wang, Y., Wu, Y., Wang, Y., Xu, H., Mei, X., Yu, D., et al. (2017). Antioxidant properties of probiotic bacteria. Nutrients 9:521. doi: 10.3390/nu9050521
Wassenaar, T., Zschüttig, A., Beimfohr, C., Geske, T., Auerbach, C., Cook, H., et al. (2015). Virulence genes in a probiotic Escherichia coli product with a recorded long history of safe use. Eur. J. Microbiol. Immunol. 5, 81–93. doi: 10.1556/EuJMI-D-14-00039
Werner, G., Fleige, C., Geringer, U., van Schaik, W., Klare, I., and Witte, W. (2011). IS element IS16 as a molecular screening tool to identify hospital-associated strains of Enterococcus faecium. BMC Infect. Dis. 11:80. doi: 10.1186/1471-2334-11-80
Wikler, M. A., Low, D. E., Cockerill, F. R., Sheehan, D. J., Craig, W. A., Tenover, F. C., et al. (2006). Methods for Dilution Antimicrobial Susceptibility Tests for Bacteria that Grow Aerobically: Approved Standard. Wayne, PA: Clinical and Laboratory Standards Institute.
Xu, H., Jeong, H. S., Lee, H. Y., and Ahn, J. (2009). Assessment of cell surface properties and adhesion potential of selected probiotic strains. Lett. Appl. Microbiol. 49, 434–442. doi: 10.1111/j.1472-765X.2009.02684.x
Keywords: probiotics, Enterococcus durans, genome mining, safety, bioinformatics
Citation: Hussein WE, Abdelhamid AG, Rocha-Mendoza D, García-Cano I and Yousef AE (2020) Assessment of Safety and Probiotic Traits of Enterococcus durans OSY-EGY, Isolated From Egyptian Artisanal Cheese, Using Comparative Genomics and Phenotypic Analyses. Front. Microbiol. 11:608314. doi: 10.3389/fmicb.2020.608314
Received: 20 September 2020; Accepted: 13 November 2020;
Published: 11 December 2020.
Edited by:
Carla Sabia, University of Modena and Reggio Emilia, ItalyReviewed by:
Celia Costa Silva, University of the Azores, PortugalCopyright © 2020 Hussein, Abdelhamid, Rocha-Mendoza, García-Cano and Yousef. This is an open-access article distributed under the terms of the Creative Commons Attribution License (CC BY). The use, distribution or reproduction in other forums is permitted, provided the original author(s) and the copyright owner(s) are credited and that the original publication in this journal is cited, in accordance with accepted academic practice. No use, distribution or reproduction is permitted which does not comply with these terms.
*Correspondence: Ahmed E. Yousef, eW91c2VmLjFAb3N1LmVkdQ==
Disclaimer: All claims expressed in this article are solely those of the authors and do not necessarily represent those of their affiliated organizations, or those of the publisher, the editors and the reviewers. Any product that may be evaluated in this article or claim that may be made by its manufacturer is not guaranteed or endorsed by the publisher.
Research integrity at Frontiers
Learn more about the work of our research integrity team to safeguard the quality of each article we publish.