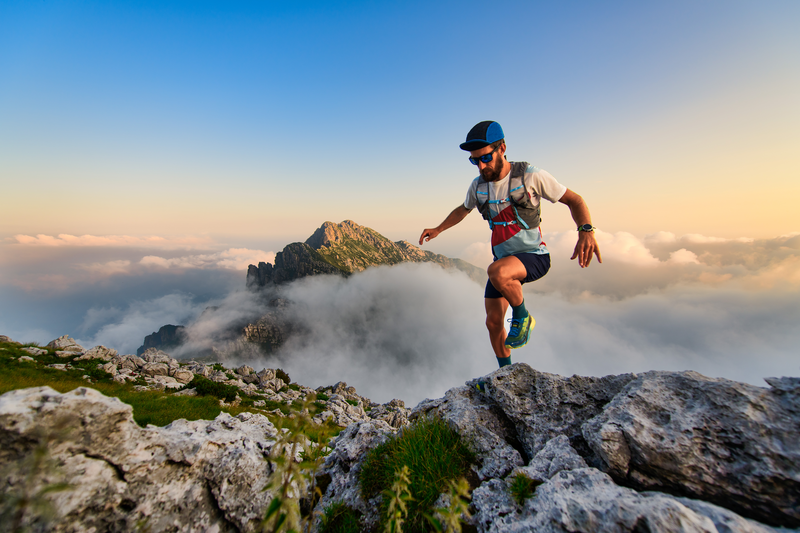
94% of researchers rate our articles as excellent or good
Learn more about the work of our research integrity team to safeguard the quality of each article we publish.
Find out more
ORIGINAL RESEARCH article
Front. Microbiol. , 15 January 2021
Sec. Antimicrobials, Resistance and Chemotherapy
Volume 11 - 2020 | https://doi.org/10.3389/fmicb.2020.604278
This study aimed to characterize 16S rRNA methylase genes among Salmonella and to elucidate the structure and evolution of rmtB-carrying plasmids. One hundred fifty-eight Salmonella isolates from one pig slaughterhouse were detected as containing 16S rRNA methylase genes; two (1.27%) Salmonella London isolates from slaughtered pigs were identified to carry rmtB. They were resistant to gentamicin, amikacin, streptomycin, ampicillin, tetracycline, florfenicol, ciprofloxacin, and sulfamethoxazole/trimethoprim. The complete sequences of RmtB-producing isolates were obtained by PacBio single-molecule real-time sequencing. The isolate HA1-SP5 harbored plasmids pYUHAP5-1 and pYUHAP5-2. pYUHAP5-1 belonged to the IncFIBK plasmid and showed high similarity to multiple IncFIBK plasmids from Salmonella London in China. The rmtB-carrying plasmid pYUHAP5-2 contained a typical IncN-type backbone; the variable region comprising several resistance genes and an IncX1 plasmid segment was inserted in the resolvase gene resP and bounded by IS26. The sole plasmid in HA3-IN1 designated as pYUHAP1 was a cointegrate of plasmids from pYUHAP5-1-like and pYUHAP5-2-like, possibly mediated by IS26 via homologous recombination or conservative transposition. The structure differences between pYUHAP1 and its corresponding part of pYUHAP5-1 and pYUHAP5-2 may result from insertion, deletion, or recombination events mediated by mobile elements (IS26, ISCR1, and ISKpn43). This is the first report of rmtB in Salmonella London. IncN plasmids are efficient vectors for rmtB distribution and are capable of evolving by reorganization and cointegration. Our results further highlight the important role of mobile elements, particularly IS26, in the dissemination of resistance genes and plasmid evolution.
Aminoglycosides such as gentamicin and amikacin have been widely used to treat infections caused by Gram-negative bacteria in clinical settings (human and animal), and they also used for growth promotion in animal husbandry. Resistance to aminoglycosides is due to enzymatic modification/inactivation of aminoglycosides, mutation or modification of aminoglycoside-binding site (16S rRNA of 30S ribosomal subunits), decreased permeability, and augmented efflux (Doi et al., 2016). Among them, 16S rRNA methylases are of great concern for conferring high-level resistance to all aminoglycosides used to treat systemic infections. Since the identification of armA in 2003, 10 16S rRNA methylase genes (armA, rmtA, rmtB, rmtC, rmtD, rmtE, rmtF, rmtG, rmtH, and npmA) have been identified and globally disseminated in Enterobacteriaceae (Galimand et al., 2003; Doi et al., 2016). Although 16S rRNA methylase genes, especially armA and rmtB, are widely disseminated in Escherichia coli isolates from various sources, low prevalence is observed in Salmonella (Folster et al., 2009; Doi et al., 2016; Fang et al., 2019). Salmonella spp. are one of the leading causes of foodborne illness, and contaminated food, particularly animal-derived food products, are the main sources of Salmonella infections for humans (European Food Safety Authority and European Centre for Disease Prevention and Control [EFSA and ECDC], 2018; Marus et al., 2019). The dramatic increase of antibiotic resistance in Salmonella has been a global health concern.
Mobile genetic elements play a critically important role in the acquisition and dissemination of resistance genes in Gram-negative and Gram-positive bacteria (Partridge et al., 2018). Cointegration between plasmids via homologous recombination or IS26-mediated replicative or conservative transposition has been previously described, allowing plasmids to acquire more resistance or virulence genes (Sun et al., 2016; Wong et al., 2017; Hua et al., 2020).
In this study, we aimed to investigate the prevalence of 16S rRNA methylase genes among Salmonella isolates from one pig slaughterhouse in Jiangsu province, China, and to elucidate the structure and evolution of rmtB-carrying plasmids.
One hundred fifty-eight Salmonella enterica isolates including Salmonella Derby (n = 69), Salmonella Typhimurium (n = 33), Salmonella Rissen (n = 28), Salmonella London (n = 16), Salmonella Chester (n = 5), Salmonella Yoruba (n = 2), Salmonella Indiana (n = 2), Salmonella Pakistan (n = 2), and Salmonella Enteritidis (n = 1) were previously obtained from pig carcass swab samples, environmental samples, equipment samples, and intestinal content samples in one slaughterhouse in Huai’an, Jiangsu province, 2016 (Zhou et al., 2017). The presence of 16S rRNA methylase genes (rmtA, rmtB, rmtC, rmtD, rmtE, rmtF, rmtG, rmtH, and armA) was detected by PCR and sequencing (Supplementary Table S1).
The rmtB-positive isolates were determined for MICs of ampicillin, cefotaxime, meropenem, gentamicin, amikacin, streptomycin, tetracycline, florfenicol, ciprofloxacin, colistin, and sulfamethoxazole/trimethoprim using microbroth dilution method. The results were interpreted according to EUCAST1. The E. coli strain ATCC 25922 was used for quality control.
Conjugation experiments were performed using streptomycin-resistant E. coli C600 as the recipient strain as previously described (Chen et al., 2007). Transconjugants were selected on MacConkey agar plates containing 3,000 mg/L streptomycin and 32 mg/L amikacin (pYUHAP5-2 and pYUHAP1) or chloramphenicol (pYUHAP5-1). Conjugal transfer frequencies were calculated as the number of transconjugants per recipient; experiments were performed in triplicate.
The stability of rmtB-carrying plasmids pYUHAP5-2 and pYUHAP1 in the original strains was investigated by passage in daily refreshed (100-fold dilution) antibiotic-free LB broth for 7 days. On the last day, the cultures were streaked on LB agar plates. 100 colonies were replica-plated on LB agar plates with amikacin (32 mg/L) and were randomly selected to confirm the presence of rmtB by PCR.
The whole genome of rmtB-positive isolates was extracted and sequenced using PacBio single-molecule real-time sequencing (RSII platform) (Pacific Biosciences, Menlo Park, CA). Raw sequence data were introduced into the nonhybrid Hierarchical Genome Assembly Process (HGAP version 4). The plasmid sequences were analyzed and annotated by the RAST server2, ResFinder3, PlasmidFinder4, and BLAST5.
The whole genome sequences of strains HA3-IN1 and HA1-SP5 have been deposited in the GenBank database under accession number PRJNA648279.
Among the 158 Salmonella isolates, two ST155 Salmonella London isolates from carcass swab samples (Zhou et al., 2017) were positive for rmtB. None of the other 16S rRNA methylase genes were detected in this study. Salmonella London was rarely reported before, but it has begun to emerge in various sources (patients, pigs, wild birds, meat products, and the environment) and spread worldwide over the past decades (Shipp and Dickson, 2011; Bonardi et al., 2016; Jurado-Tarifa et al., 2016; Trimoulinard et al., 2017; Chen et al., 2019b). To our knowledge, this is the first report of 16S rRNA methylase genes in Salmonella London.
As shown in Table 1, two rmtB-positive Salmonella London strains HA1-SP5 and HA3-IN1 exhibited high-level resistance to amikacin (MIC > 256 mg/L) and also showed resistance to ampicillin, gentamicin, streptomycin, tetracycline, florfenicol, ciprofloxacin, and sulfamethoxazole/trimethoprim, but susceptibility to cefotaxime, meropenem, and colistin.
To better understand the genetic features of rmtB, complete sequences of the two rmtB-bearing Salmonella London strains were obtained. The isolate HA1-SP5 consisted of a 4,747,268-bp chromosome and two plasmids pYUHAP5-1 and pYUHAP5-2 (Table 1). The isolate HA3-IN1 consisted of a 4,746,179-bp chromosome and a single plasmid pYUHAP1 (Table 1). Both of them carried the aminoglycoside resistance gene aac(6’)-Iaa in the chromosome and mutation in parC (T57S) which could partly explain the ciprofloxacin resistance (Table 1).
The largest plasmid in HA1-SP5, designated as pYUHAP5-1, had a size of 112,916 bp with GC content of 54.93%. It belonged to the IncFIBK type and carried 14 resistance genes (Table 1). pYUHAP5-1 was highly similar to plasmids pSa63-CIP (GenBank accession no. MG874043), pSa44-CIP (MH430882), pSa76-CIP (MG874044), and pSa128 (MG870194) carried by Salmonella London from meat products in Shenzhen, China (83–98% coverage and 99.9% identity) (Chen et al., 2018; Li et al., 2018; Chen et al., 2019a; Figure 1A). Similar IncFIBK plasmids were detected in Salmonella isolates, particularly Salmonella London (Chen et al., 2018). In line with previous studies (Chen et al., 2018; Chen et al., 2019a), pYUHAP5-1 was conjugative at a high frequency of 4.6 × 10–1 transconjugants per recipient.
Figure 1. (A) Comparison of IncFIBK plasmid pYUHAP5-1 in this study with other IncFIBK plasmids pSa44-CIP (Salmonella London, food, MH430882), pSa63-CIP (Salmonella London, meat, MG874043), pSa76-CIP (Salmonella London, meat, MG874044), and pSa128 (Salmonella London, pork, MG870194) in China. (B) Comparison of IncN plasmid pYUHAP5-2 in this study with other IncN plasmids R46 (Salmonella Typhimurium, AY046276), pRSB206 (uncultured bacterium, Germany, wastewater treatment plants effluents, JN102344), pVQS1 (Salmonella Virchow, Switzerland, human, JQ609357), pL2-43 (Escherichia coli, Switzerland, lamb, KJ484641), and p160070-CTXM (Klebsiella pneumoniae, China, food, MG288677). The outer circles in red with annotation are the reference plasmids pYUHAP5-1 and pYUHAP5-2, respectively.
The primary feature of these IncFIBK plasmids is the co-location of qnrB6-aac(6′)-Ib-cr within a complex class 1 integron (intI1-aac(6′)-Ib-cr-arr-3-dfrA27-aadA16-qacEΔ1-sul1-ISCR1-qnrB6-qacEΔ1-sul1). It was followed by the mph(A) region [mph(A)-mrx-mphR(A)] flanked by IRt-IS6100 and IS26 (Figure 2A). The unique difference between these IncFIBK plasmids and pYUHAP5-1 was the absence of ∼5.5 kb ISCR1-qnrB6 segment in pYUHAP5-1 (Figure 2A). ISCR1 can mobilize itself and adjacent DNA segments, including resistance genes, into the 3’-conserved segment (3’-CS) by rolling circle transposition (Toleman et al., 2006). The common IncFIBK plasmids in Salmonella London (e.g., pSa63-CIP) may evolve from pYUHAP5-1-like plasmids by acquiring qnrB6 through ISCR1-mediated transposition within class 1 integron. A circular intermediate containing ISCR1, adjacent region, and part of the 3′-CS is created, and it can be rescued via homologous recombination with the 3′-CS of class 1 integron, resulting in duplications of this circular intermediate (Toleman et al., 2006). The ISCR1-qnrB6 segment in tandem repeats has been previously described in IncFIBK plasmids (Li et al., 2018).
Figure 2. Genetic organization of the multiresistance region of (A) IncFIBK plasmids pYUHAP5-1 and pYUHAP1. Plasmid pSa128 harbors four copies of ISCR1-qnrB6 segment. Arrowheads show the relative directions of IR. The IRt was not included in the complex class 1 integron, it was the short remnants of the IRt end of tni402 flanking IS6100. (B) IncN plasmids pYUHAP5-2 and pYUHAP1 and structural comparison with plasmids pRSB206 (JN102344), pEC012 (KT282968), pHN7A8 (JN232517), pABC143-NDM (MH316136), pHN3A11 (JX997935), pHNZY32 (MG197502), pHNAH24 (MG197495), and pHNMCC14 (MG197498). The extents and directions of antibiotic resistance (thick arrows) and other genes are indicated. Δ indicates a truncated gene or mobile element. ISs are shown as boxes labeled with their name. Tall bars represent the inverted repeats (IR) of transposon.
The rmtB-carrying plasmid pYUHAP5-2 was 57,187-bp with a GC content of 49.76%, and belonged to IncN plasmid group. IncN plasmids have been described as vectors for rmtB dissemination in E. coli, but F2:A1:B1 plasmids were mainly associated with rmtB spread in Salmonella (Yao et al., 2011; Fang et al., 2019). pYUHAP5-2 contained the typical IncN backbone encoding functions for replication, conjugal transfer, maintenance, and stability, and showed high similarity (>99% identity) to other IncN plasmids such as the archetypal IncN1 plasmid R46 (AY046276) (Figure 1B). As observed in other IncN plasmids (e.g., R46, pRSB206, pVQS1), repetitive elements (CUP1-6, CUPA, CUPB, A1, and A2), which function as regulatory elements (Delver and Belogurov, 1997), and iteron regions I and II (with tandem repeats of 37 bp) for plasmid replication and copy number control (Krishnan and Iyer, 1990; Papp and Iyer, 1995) were also identified on plasmid pYUHAP5-2 (Figure 1B), but differed by the numbers of 37-bp repeats within iteron region I (Supplementary Table S2). pYUHAP5-2 was stably maintained within the original isolate for 7 days without selection, and could be transferred to E. coli C600 at a frequency of 1.9 × 10–4 transconjugants/recipient.
The variable region (∼23.5 kb) of pYUHAP5-2 was inserted in the resolvase gene resP and bounded at both ends by IS26, resulting in the deletion of 3,513-bp segment (ΔresP-ecoRII-dcm) compared to pRSB206 (JN102344, IncN1) (Figure 2B). The variable region consisted of four IS26 elements flanking three different parts. The first part (∼14.6 kb) contained resistance genes Δble (bleomycin resistance) and aph(3’)-IIa (aminoglycoside resistance); mobile elements ΔIS50, IS1294, and ΔIS26; and IncX1 plasmid region (stbD/E-pir-repX-bis-ddp3-parG/F) truncated by ΔIS1 (Figure 2B). This part was identical to those of plasmids pACN001-A (KC853434, E. coli), pCTXM-2271 (MF589339, E. coli), and pCFSA1007-2 (CP033386, Salmonella enterica), differed by two to six nucleotide changes. The IncX1 region in pYUHAP5-2 was 99.7% identical to the corresponding part of pOLA52 (EU370913, IncX1) with 89% coverage, whereas repX was absent in pOLA52. The 10,044-bp segment (IS26-Δble-aph(3’)-IIa-ΔIS50-IS1294-ΔIS26-stbD/E-pir-repX-bis-ddp3-314 bp) was previously detected with opposite orientation in multiple F33:A-:B- plasmids from E. coli of various origins in China, such as pHNZY32 (MG197502, patient), pHNAH24 (MG197495, chicken), and pHNMCC14 (MG197498, chicken meat) (Wang et al., 2018; Figure 2B). It highlights the ability of the IncX1 plasmid segment, along with resistance genes, to be captured by distinct plasmids through mobile elements. It is common to observe multiple replication regions within a single plasmid, which may increase its host range (Partridge et al., 2018).
The second part (2,828-bp) consisted of an incomplete Tn2 (containing β-lactam resistance gene blaTEM–1b) and rmtB and was found in numerous plasmids such as pABC143C-NDM (KY130431, F2:A-:B-) and pGDD25-21 (MH316136, F2:A1:B1) (Figure 2B). This segment was identical to those of F2:A-:B- plasmids or related plasmids, whereas slight difference was observed in other types of plasmids, e.g., pHN7A8 (JN232517, F33:A-:B-) and pEC012 (KT282968, IncI1) (Figure 2B). The last part (2,780-bp) was an incomplete class 1 integron with ΔintI1 and aadA22-lnu(F) cassette flanked by IS26, as observed in other plasmids with the same IS26/5’-CS and 3’-CS/IS26 boundary, such as p160070-CTXM (MG288677, K. pneumoniae) and pNDM33-1 (MN915011, E. coli). Our results further confirm that IS26 elements may play a vital role in the dissemination of resistance genes and formation of variable region of pYUHAP5-2.
The sole plasmid in HA3-IN1, namely pYUHAP1, was 176,767 bp with an average G+C content of 53.14%. It harbored three replicons, including IncN1, IncX1, and IncFIBK (Table 1). pYUHAP1 was a cointegrate plasmid comprised of sequence from pYUHAP5-1-like and pYUHAP5-2-like plasmids (Figure 3). The IncN1-X1 RmtB-producing plasmid region of pYUHAP1 was similar to pYUHAP5-2, except that the first and second parts of variable region in pYUHAP5-2 (Δble-aph(3’)-IIa-ΔIS50-IS1294-ΔIS26-stbD/E-pir-repX-bis-ddp3-ΔIS1-IS26-Tn2-rmtB) was in the opposite orientation with a shorter 157-bp IS1 in pYUHAP1 (Figure 2B). This could be readily explained by homologous recombination between IS26 located in inverse orientations. Additionally, the pYUHAP5-1-derived region in pYUHAP1 was 119,756 bp, the size difference was due to the acquisition of 5,466-bp ISCR1-qnrB6 segment within the class 1 integron, and the presence of one copy of ISKpn43 (1,374 bp) located between ΔIS4321 and IS903-like in pYUHAP1 (Figure 3A). The presence of additional plasmid-mediated quinolone resistance gene qnrB6 could easily explain the difference of MIC of ciprofloxacin between HA1-SP5 and HA3-IN1 (Table 1). The regions of pYUHAP1 derived from the different ancestor plasmids were separated by a pair of IS26, the cointegrate formation was likely generated by IS26 (Figure 3B). We did not observe additional copies of IS26 or 8-bp target-site duplication, suggesting that this cointegration may occur by IS26-mediate homologous recombination or conservative transposition rather than replicative transposition (He et al., 2015; Harmer and Hall, 2016). The insertion, deletion, or recombination events mediated by mobile elements (e.g. IS26, ISCR1, ISKpn43) have occurred to form plasmids as ancestors for pYUHAP1 or occurred after cointegration, but the precise series of events cannot be determined with the available data.
Figure 3. Proposed mechanism for the formation of cointegrate plasmid pYUHAP1. (A) Linear sequence comparison of pYUHAP1, pYUHAP5-1, pYUHAP5-2. Green arrows represent ORFs. Regions of homology are shaded in gray. (B) Proposed model for the plasmid cointegration by IS26. IS26 involved in the cointegration are shown as green arrows, the 8-bp sequences adjacent to each IS26 are indicated by black lines with red or blue letters. Colored arrows with black, red, and blue represent replicon genes of IncN, IncFIBK, and IncX plasmids. Orange segments represent the complex class 1 integron shown in Figure 2A.
Similar cointegration events mediated by IS26 have occurred between IncN1-F33:A-:B- plasmids and mcr-1-carrying phage-like plasmid in E. coli (He et al., 2019), IncN, F33:A-: B-, and rolling-circle plasmids in Proteus mirabilis (Hua et al., 2020), or virulence plasmid and IncHI2 resistance plasmid in Salmonella Enteritidis (Wong et al., 2017). IS26 elements play a vital role in the dissemination of resistance genes, formation of mosaic resistance regions, and reorganization of plasmids in Gram-negative bacteria (He et al., 2015; Partridge et al., 2018). They also indicate that cointegration formation between plasmids is an important but not rare way for plasmids to evolve and capture more resistance genes, virulence genes, or other beneficial genes for their dissemination and maintenance. In this study, pYUHAP1 acquired more resistance genes through cointegration between two resistance plasmids, but it remained stable (100%) and conjugative with frequency of 1.03 × 10–4 transconjugants/recipient.
This study revealed the emergence of rmtB in Salmonella London of swine origin in China. The fusion of rmtB-carrying IncN plasmid and IncFIBK multiresistance plasmid was mediated by IS26. IncN plasmids have become efficient vectors for rmtB transmission not only in E. coli, but also in Salmonella, and are able to evolve by reorganization and co-integration. Our results further confirm the critical role of mobile elements, particularly IS26, in the mobilization of resistance genes, formation of resistance regions, and diversity of plasmid structures.
The datasets presented in this study can be found in online repositories. The names of the repository/repositories and accession number(s) can be found in the article/Supplementary Material.
Z-MP, XJ, and JW conceived the study. YW, Z-YW, FS, HW, P-CS, and JW carried out the experiments. JW, Z-YW, and WL analyzed the data. JW wrote the manuscript. Z-MP and XJ revised the manuscript. All authors read and approved the final manuscript.
This work was supported by the National Natural Science Foundation of China (grant no. 31902319), Key Research and Development Program (Modern Agriculture) project of Jiangsu Province (grant no. BE2017341), and the Priority Academic Program Development of Jiangsu Higher Education Institutions (PAPD).
The authors declare that the research was conducted in the absence of any commercial or financial relationships that could be construed as a potential conflict of interest.
The Supplementary Material for this article can be found online at: https://www.frontiersin.org/articles/10.3389/fmicb.2020.604278/full#supplementary-material
Bonardi, S., Alpigiani, I., Bruini, I., Barilli, E., Brindani, F., Morganti, M., et al. (2016). Detection of Salmonella enterica in pigs at slaughter and comparison with human isolates in Italy. Int. J. Food Microbiol. 218, 44–50. doi: 10.1016/j.ijfoodmicro.2015.11.005
Chen, K. C., Chan, E. W. C., and Chen, S. (2019a). Evolution and transmission of a conjugative plasmid encoding both ciprofloxacin and ceftriaxone resistance in Salmonella. Emerg. Microb. Infect. 8, 396–403. doi: 10.1080/22221751.2019.1585965
Chen, T., Jiang, J., Ye, C., Xie, J., Chen, X., Xu, D., et al. (2019b). Genotypic characterization and antimicrobial resistance profile of Salmonella isolated from chicken, pork and the environment at abattoirs and supermarkets in Chongqing. China. BMC Vet. Res. 15:456. doi: 10.1186/s12917-019-2202-4
Chen, K. C., Dong, N., Zhao, S. H., Liu, L. Z., Li, R. C., Xie, M. M., et al. (2018). Identification and characterization of conjugative plasmids that encode ciprofloxacin resistance in Salmonella. Antimicr. Agents Chemother. 62, 575–e518. doi: 10.1128/AAC.005
Chen, L., Chen, Z. L., Liu, J. H., Zeng, Z. L., Ma, J. Y., and Jiang, H. X. (2007). Emergence of RmtB methylase-producing Escherichia coli and Enterobacter cloacae isolates from pigs in China. J. Antimicr. Chemother. 59, 880–885. doi: 10.1093/jac/dkm065
Delver, E. P., and Belogurov, A. A. (1997). Organization of the leading region of IncN plasmid pKM101 (R46): a regulation controlled by CUP sequence elements. J. Mol. Biol. 271, 13–30. doi: 10.1006/jmbi.1997.1124
Doi, Y., Wachino, J. I., and Arakawa, Y. (2016). Aminoglycoside Resistance: The Emergence of Acquired 16S Ribosomal RNA Methyltransferases. Infect. Dis. Clin. North Am. 30, 523–537. doi: 10.1016/j.idc.2016.02.011
European Food Safety Authority and European Centre for Disease Prevention and Control [EFSA and ECDC] (2018). The European Union summary report on trends and sources of zoonoses, zoonotic agents and food-borne outbreaks in 2017. EFSA J. 16:e5500.
Fang, L. X., Deng, G. H., Jiang, Q., Cen, D. J., Yang, R. S., Feng, Y. Y., et al. (2019). Clonal expansion and horizontal transmission of epidemic F2:Al:B1 plasmids involved in co-spread of rmtB with qepA and blaCTX–M–27 in extensively drug-resistant Salmonella enterica serovar Indiana isolates. J. Antimicr. Chemother. 74, 334–341. doi: 10.1093/jac/dky441
Folster, J. P., Rickert, R., Barzilay, E. J., and Whichard, J. M. (2009). Identification of the aminoglycoside resistance determinants armA and rmtC among non-Typhi Salmonella isolates from humans in the United States. Antimicr. Agents Chemother. 53, 4563–4564. doi: 10.1128/aac.00656-09
Galimand, M., Courvalin, P., and Lambert, T. (2003). Plasmid-mediated high-level resistance to aminoglycosides in Enterobacteriaceae due to 16S rRNA methylation. Antimicrob. Agents Chemother. 47, 2565–2571. doi: 10.1128/aac.47.8.2565-2571.2003
Harmer, C. J., and Hall, R. M. (2016). IS26-mediated formation of transposons carrying antibiotic resistance genes. Msphere 1:e0003 doi: 10.1128/mSphere.00038-16
He, D., Zhu, Y., Li, R., Pan, Y., Liu, J., Yuan, L., et al. (2019). Emergence of a hybrid plasmid derived from IncN1-F33:A-:B- and mcr-1-bearing plasmids mediated by IS26. J. Antimicrob. Chemother. 74, 3184–3189. doi: 10.1093/jac/dkz327
He, S. S., Hickman, A. B., Varani, A. M., Siguier, P., Chandler, M., Dekker, J. P., et al. (2015). Insertion sequence IS26 reorganizes plasmids in clinically isolated multidrug-resistant bacteria by replicative transposition. Mbio 6:e0076. doi: 10.1128/mBio.00762-15
Hua, X., Zhang, L., Moran, R. A., Xu, Q., Sun, L., Van Schaik, W., et al. (2020). Cointegration as a mechanism for the evolution of a KPC-producing multidrug resistance plasmid in Proteus mirabilis. Emerg. Microbes Infect. 9, 1206–1218. doi: 10.1080/22221751.2020.1773322
Jurado-Tarifa, E., Torralbo, A., Borge, C., Cerda-Cuellar, M., Ayats, T., Carbonero, A., et al. (2016). Genetic diversity and antimicrobial resistance of Campylobacter and Salmonella strains isolated from decoys and raptors. Comp. Immunol. Microbiol. Infect. Dis. 48, 14–21. doi: 10.1016/j.cimid.2016.07.003
Krishnan, B. R., and Iyer, V. N. (1990). IncN plasmid replicon. A deletion and subcloning analysis. J. Mol. Biol. 213, 777–788. doi: 10.1016/S0022-2836(05)80263-3
Li, R. C., Chen, K. C., Chan, E. W. C., and Chen, S. (2018). Resolution of dynamic MDR structures among the plasmidome of Salmonella using MinION single-molecule, long-read sequencing. J. Antimicr. Chemother. 73, 2691–2695. doi: 10.1093/jac/dky243
Marus, J. R., Magee, M. J., Manikonda, K., and Nichols, M. C. (2019). Outbreaks of Salmonella enterica infections linked to animal contact: Demographic and outbreak characteristics and comparison to foodborne outbreaks-United States, 2009-2014. Zoon. Publ. Health 66, 370–376. doi: 10.1111/zph.12569
Papp, P. P., and Iyer, V. N. (1995). Determination of the binding sites of RepA, a replication initiator protein of the basic replicon of the IncN group plasmid pCU1. J. Mol. Biol. 246, 595–608. doi: 10.1016/s0022-2836(05)80109-3
Partridge, S. R., Kwong, S. M., Firth, N., and Jensen, S. O. (2018). Mobile Genetic Elements Associated with Antimicrobial Resistance. Clin. Microbiol. Rev. 31, 17–88.e. doi: 10.1128/CMR.00088-17
Shipp, G. M., and Dickson, J. S. (2011). The establishment of Enterobacteriaceae and Salmonella London in a new dairy farm environment. Foodbor. Pathog. Dis. 8, 411–420. doi: 10.1089/fpd.2010.0692
Sun, J., Yang, R. S., Zhang, Q., Feng, Y., Fang, L. X., Xia, J., et al. (2016). Co-transfer of blaNDM–5 and mcr-1 by an IncX3-X4 hybrid plasmid in Escherichia coli. Nat. Microbiol. 1:16176. doi: 10.1038/nmicrobiol.2016.176
Toleman, M. A., Bennett, P. M., and Walsh, T. R. (2006). ISCR elements: Novel gene-capturing systems of the 21st century? Microbiol. Mole. Biol. Rev. 70:296. doi: 10.1128/Mmbr.00048-05
Trimoulinard, A., Beral, M., Henry, I., Atiana, L., Porphyre, V., Tessier, C., et al. (2017). Contamination by Salmonella spp., Campylobacter spp. and Listeria spp. of most popular chicken- and pork-sausages sold in Reunion Island. Int. J. Food Microbiol. 250, 68–74. doi: 10.1016/j.ijfoodmicro.2017.03.017
Wang, J., Zeng, Z. L., Huang, X. Y., Ma, Z. B., Guo, Z. W., Lv, L. C., et al. (2018). Evolution and comparative genomics of F33:A-:B- plasmids carrying blaCTX–M–55 or blaCTX–M–65 in Escherichia coli and Klebsiella pneumoniae isolated from animals, food products, and humans in China. mSphere 3, e137–e118. doi: 10.1128/mSphere.00137-18
Wong, M. H. Y., Chan, E. W. C., and Chen, S. (2017). IS26-mediated formation of a virulence and resistance plasmid in Salmonella Enteritidis. J. Antimicr. Chemother. 72, 2750–2754. doi: 10.1093/jac/dkx238
Yao, Q. F., Zeng, Z. L., Hou, J. X., Deng, Y. T., He, L. Y., Tian, W., et al. (2011). Dissemination of the rmtB gene carried on IncF and IncN plasmids among Enterobacteriaceae in a pig farm and its environment. J. Antimicr. Chemother. 66, 2475–2479. doi: 10.1093/jac/dkr328
Keywords: cointegration, IncN, IS26, rmtB, Salmonella
Citation: Wang J, Wang Z-Y, Wang Y, Sun F, Li W, Wu H, Shen P-C, Pan Z-M and Jiao X (2021) Emergence of 16S rRNA Methylase Gene rmtB in Salmonella Enterica Serovar London and Evolution of RmtB-Producing Plasmid Mediated by IS26. Front. Microbiol. 11:604278. doi: 10.3389/fmicb.2020.604278
Received: 09 September 2020; Accepted: 08 December 2020;
Published: 15 January 2021.
Edited by:
Steven Lee Foley, National Center for Toxicological Research (FDA), United StatesReviewed by:
Patrice Courvalin, Institut Pasteur, FranceCopyright © 2021 Wang, Wang, Wang, Sun, Li, Wu, Shen, Pan and Jiao. This is an open-access article distributed under the terms of the Creative Commons Attribution License (CC BY). The use, distribution or reproduction in other forums is permitted, provided the original author(s) and the copyright owner(s) are credited and that the original publication in this journal is cited, in accordance with accepted academic practice. No use, distribution or reproduction is permitted which does not comply with these terms.
*Correspondence: Zhi-Ming Pan, em1wYW5AeXp1LmVkdS5jbg==; Xinan Jiao, amlhb0B5enUuZWR1LmNu
†These authors have contributed equally to this work
Disclaimer: All claims expressed in this article are solely those of the authors and do not necessarily represent those of their affiliated organizations, or those of the publisher, the editors and the reviewers. Any product that may be evaluated in this article or claim that may be made by its manufacturer is not guaranteed or endorsed by the publisher.
Research integrity at Frontiers
Learn more about the work of our research integrity team to safeguard the quality of each article we publish.