- 1Central European Institute of Technology, University of Veterinary and Pharmaceutical Sciences Brno, Brno, Czechia
- 2Department of Biology and Wildlife Diseases, Faculty of Veterinary Hygiene and Ecology, University of Veterinary and Pharmaceutical Sciences Brno, Brno, Czechia
- 3Department of Microbiology, Faculty of Medicine and University Hospital in Plzen, Charles University, Plzen, Czechia
- 4Faculty of Medicine, Biomedical Center, Charles University, Plzen, Czechia
- 5Department of Microbiology, University Hospital of Larissa, Larissa, Greece
- 6Department of Bacteriology, Veterinary Research Institute, Brno, Czechia
- 7Department of Experimental Biology, Faculty of Science, Masaryk University Brno, Brno, Czechia
The aim of our study was to determine complete nucleotide sequence of mcr-1-carrying plasmids from Enterobacterales isolates recovered from domestic and imported raw retailed meat and compare them with plasmids available at the GenBank sequence database. A set of 16 plasmids originating from Escherichia coli (n = 13), Klebsiella pneumoniae (n = 2), and Citrobacter braakii (n = 1) were analyzed. In our previous study, data from whole genome sequencing showed that mcr-1 gene was located on plasmids of different incompatibility groups (IncHI2, IncI2, and IncX4). The IncI2 (n = 3) and IncX4 (n = 8) plasmids harbored mcr-1.1 gene only, whereas IncHI2 sequence type 4 plasmids (n = 5) carried large multidrug resistance (MDR) regions. MDR regions of IncHI2 plasmids included additional antimicrobial resistance genes conferring resistance to β-lactams (blaTEM−1), aminoglycosides [aadA1, aadA2, and aph(6)-Id], macrolides [mef (B)], tetracycline (tetA, tetR), and sulphonamides (sul1, sul2, and sul3). Likewise, IncHI2 plasmids carried several insertion sequences including IS1, IS3, IS26, IS1326, and ISApl1. In conclusion, our findings confirmed the involvement of IncX4, IncI2, and IncHI2 plasmids in the dissemination of mcr-1.1 gene in several environmental niches, as in samples of retail meat originating from different geographical regions. In contrast to IncX4 and IncI2, IncHI2 plasmids were more diverse and carried additional genes for resistance to heavy metals and multiple antimicrobials.
Introduction
The emergence of new genetic elements encoding antimicrobial resistance in bacterial pathogens represents a threat to public health. Polymyxins are cationic polypeptide antimicrobials and include five different compounds (polymyxin A–E) of which only two compounds, polymyxin B and polymyxin E (colistin), are used clinically (Falagas et al., 2010). Colistin is considered as a last resort antimicrobial agent against multi-drug resistant Gram-negative bacteria including Enterobacterales. However, the occurrence and spread of colistin-resistant bacteria has rapidly increased worldwide (Li et al., 2017).
Resistance to colistin can be mediated via chromosomal mutations in genes involved in lipopolysaccharide synthesis (Olaitan et al., 2014). These mutations mediate modifications of the bacterial outer membrane through alteration of the lipopolysaccharide (Landman et al., 2008). In 2016, the first plasmid-mediated colistin resistance gene, mcr-1, was identified among Chinese Enterobacterales isolates (Liu et al., 2016). Since the first discovery of mcr-1 gene in 2016 in China 10 variants of mcr genes have been reported (Wang et al., 2020). These genes encode phosphoethanolamine transferases that catalyze the addition of phosphoethanolamine to the phosphate group of lipid A, reducing the negative charge of the bacterial outer membrane and attenuating its affinity for colistin, resulting in antimicrobial resistance (Poirel et al., 2017).
Antimicrobial resistance genes are mostly located on conjugative plasmids that allow their efficient dissemination among bacteria. Resistance plasmids belong to diverse incompatibility groups and they usually carry a wide variety of genes conferring resistance to β-lactams, aminoglycosides, co-trimoxazole, quinolones, and other antimicrobials (Carattoli et al., 2014; Rozwandowicz et al., 2018). In Enterobacterales several major plasmid families, mainly carrying C, HI2, I1, I2, M, N, X, F, and X replicons have been found in association with emerging antimicrobial resistance determinants (Carattoli, 2013; Matamoros et al., 2017). Plasmids carrying mcr-1 gene have been reported to include mainly IncHI2, IncI2, IncFII, IncP, and IncX4 families (Doumith et al., 2016; Xavier et al., 2016). A recent study, focused on mobile genetic elements (MGEs) carrying mcr-1 gene, reported the regional spread of IncHI2 plasmids in Europe and of IncI2 plasmids in Asia (Matamoros et al., 2017). Another study has highlighted chicken meat as an emerging reservoir of colistin-resistant E. coli strains, carrying mcr-1 on IncX4 plasmids, in South America (Monte et al., 2017). ISApl1 found upstream of mcr-1 gene has been proposed to be the key element mediating translocation of mcr-1 into various plasmid backbones (Sun et al., 2017).
In a previous study from our group, a high prevalence of Enterobacterales with mcr-mediated colistin resistance was observed in retail meat of different origins, including imported meat and meat from domestic production (Gelbíčová et al., 2019). Thus, the aim of the current study was to characterize the complete nucleotide sequence of mcr-1-carrying plasmids, which were assigned to different Inc groups, in order to examine the nature of the MGEs involved in the acquisition and spread of mcr-1 in foodborne Enterobacterales in the Czech Republic.
Materials and Methods
Bacterial Isolates and Plasmids
In a previous study, a total of 61 MCR-1-producing Enterobacterales isolates were identified from meat at retail markets in the Czech Republic (Gelbíčová et al., 2019). These isolates included E. coli (n = 54), Klebsiella pneumoniae (n = 6), and Citrobacter braakii (n = 1) and were assigned to 34 different sequence types (STs), of which E. coli isolates belonging to ST10, ST93, and ST744 were the most common. In our follow-up study, 16 mcr-1-positive plasmids being representatives of IncHI2 (n = 5), IncI2 (n = 3), and IncX4 (n = 8) groups from isolates of different country (Brazil, Czech Republic, China, Germany and Poland) and species origin (turkey and rabbit) were selected for characterization of complete nucleotide sequences (Table 1).
Plasmid Assembly and Analysis
For isolates, harboring mcr-1-carrying plasmids that belonged to IncX4 or IncI2 groups, plasmid sequences were extracted from Illumina data obtained previously (Gelbíčová et al., 2019). The sequence gaps were filled by a PCR-based strategy and Sanger sequencing.
IncHI2 plasmids carrying mcr-1 were sequenced using PacBio technology and assembled by the Hierarchical Genome Assembly Process (HGAP) v.4 (Chin et al., 2013) which provides long reads of single-molecule DNA and enables the closing of the whole plasmid sequences.
For sequence analysis and annotation, the BLAST algorithm (www.ncbi.nlm.nih.gov/BLAST), the ISfinder database (www-is.biotoul.fr/), and the ORF finder tool (www.bioinformatics.org/sms/) were utilized. The CGE online tools (https://cge.cbs.dtu.dk/) were used to identify antimicrobial resistance genes (ResFinder 4.1) (Zankari et al., 2012), plasmid replicons (PlasmidFinder 2.1), and plasmid STs (pMLST 2.0) (Carattoli et al., 2014). The Integrall integron database (http://integrall.bio.ua.pt) was used to analyze and assign integron sequences (Moura et al., 2009). Alignments with highly similar complete plasmid sequences available in NCBI were conducted using the BRIG tool (v0.95).
Nucleotide sequence Accession Numbers
The sequences of our reported plasmids have been deposited in GenBank under accession numbers MT929275, MT929276, MT929277, MT929278, MT929279, MT929280, MT929281, MT929282, MT929283, MT929284, MT929285, MT929286, MT929287, MT929288, MT929289, and MT929290.
Results
Characteristics of mcr-1-Carrying Plasmids
Sixteen mcr-1-positive plasmids, selected for this study, included three main groups: (i) two ~60 kb IncI2 plasmids originated from raw rabbit meat imported from China, (ii) five IncHI2 plasmids with size between ~250 and 290 kb originated from raw turkey meat imported from Poland, and (iii) IncX4 group (n = 8) which sized ~33 kb and predominated our collection (Table 1). The IncI2-X4 hybrid plasmid (pMCR_1138_A1) of ~95 kb was obtained from raw turkey meat imported from Germany. The mcr-1.1 genes from all wild strains were transferred to sodium azide-resistant E. coli J53 K12 laboratory strain by conjugation (Gelbíčová et al., 2019), confirming the ability of mcr-1.1-carrying plasmids to further disseminate the mcr-1.1 in other clones or species.
Fifteen of the plasmids carried a complete mcr-1.1 gene, whereas one IncHI2 plasmid (pMCR_1139_D1) carried a mcr-1.1 gene with a truncation of the 3′ end, which was bordered by two ISApl1 elements. Plasmids belonging to IncI2 and IncX4 groups (including the hybrid strain), did not carry additional antimicrobial resistance genes, whereas IncHI2 plasmids exhibited large MDR regions.
Structure of IncX4 mcr-1.1-Carrying Plasmids
IncX4 plasmids carrying mcr-1.1 originated from E. coli and K. pneumoniae isolates recovered from raw turkey meat or liver from Brazil, Germany, Poland and the Czech Republic (Table 1). They were all derivatives of the plasmid pHNSHP49 that was described in the E. coli strain SHP49 recovered from a pig in China (GenBank accession no. MF774188). Seven out of the eight sequenced IncX4 plasmids showed high degrees of similarity to each other and to pHNSHP49 (100% coverage, 99% identity) (Figure 1). The mcr-1.1 gene was bordered by two ORFs encoding a hypothetical protein and a PAP2 transmembrane protein. Plasmid pMCR_1525_C2, which was present in K. pneumoniae ST11 isolate originating from Brazil, differed from pHNSHP49 by the insertion of IS1A element, upstream of mcr-1.1 gene.
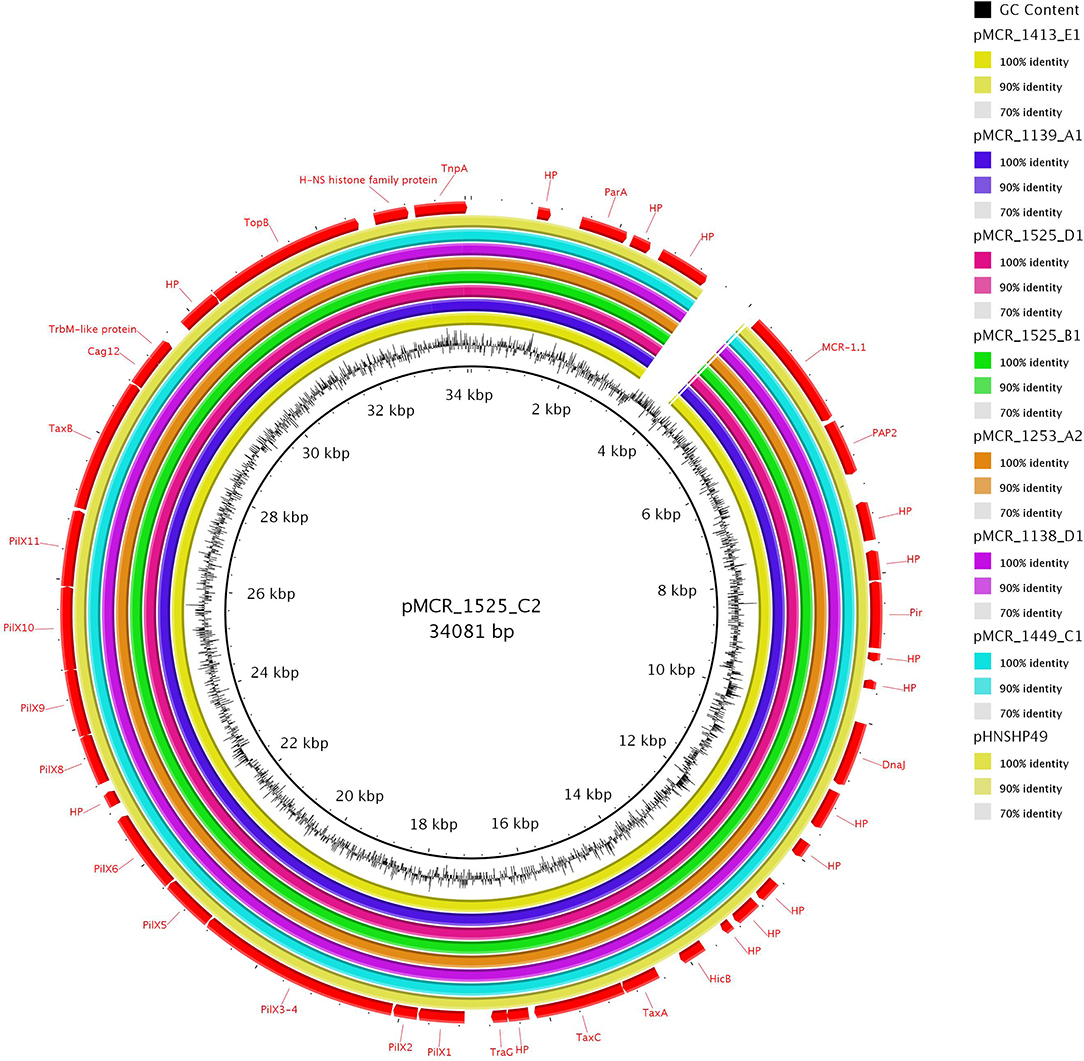
Figure 1. Sequence alignment of eight IncX4 and one hybrid IncX4-I2 mcr-1-encoding plasmids. The plasmid pMCR_1525_C2 (GenBank accession no. MT929281) was used as a reference for the comparison.
Structure of IncI2 mcr-1.1-Carrying Plasmids
Two IncI2 plasmids (pMCR_1884_B3 and pMCR_1884_C3) shared high sequence identity with mcr-1.1-positive plasmid pHNSHP45 (99% coverage, 99% identity) (GenBank accession number KP347127) which was isolated from swine in China (Liu et al., 2016) (Figure 2). The mcr-1.1 region was inserted downstream the nikB gene of IncI2 plasmid backbone, as found in pHNSHP45. Unlike pHNSHP45, an ISApl1 element was not found upstream mcr-1.1 gene.
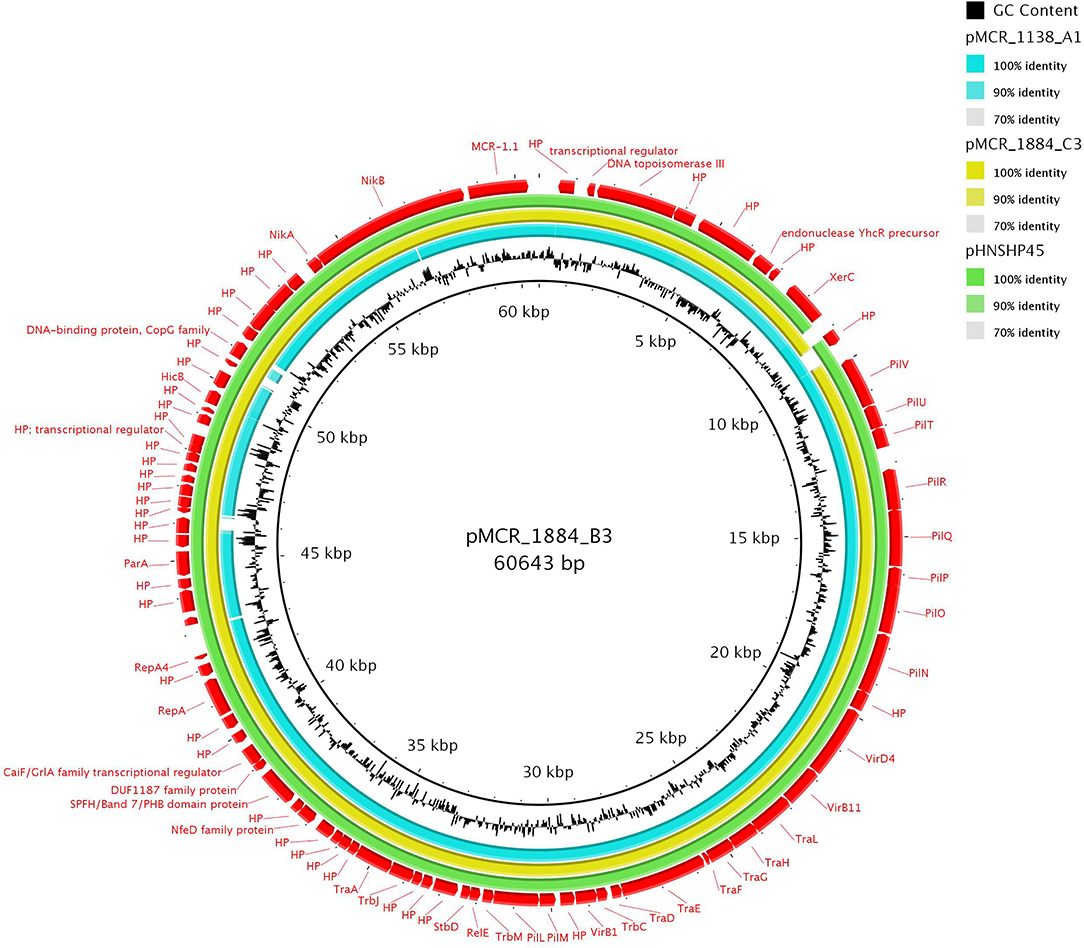
Figure 2. Sequence alignment of two IncI2 and one hybrid IncX4-I2 mcr-1-encoding plasmids. The plasmid pMCR_1884_B3 (GenBank accession no. MT929283) was used as reference to compare with other plasmids.
Furthermore, plasmid pMCR_1138_A1, being an IncI2-X4 hybrid, was characterized from an E. coli ST162 isolate originating from Germany. Sequencing data suggested that the progenitor of pMCR_1138_A1 is an IncX4 plasmid, carrying mcr-1.1. At a certain step of the evolution of pMCR_1138_A1, an IncX4 plasmid, carrying mcr-1.1, and an IncI2 plasmid may have formed a fusion structure. Fusion structure may have been formed via a recombination event between a homologous region (nt 29,159–31,608 in pMCR_1525_D1; Supplementary Figure 1) encoding a DNA topoisomerase III. Recombination event resulted in duplication of homologous region, in pMCR_1138_A1. Additionally, pMCR_1138_A1 harbored a Tn3-like transposon, which carried blaTEM−32 gene. The Tn3-like structure was found upstream IncX4 parA gene.
Structure of mcr-1-Encoding IncHI2 Plasmids
All five IncHI2 plasmids exhibited sequences closely related to other mcr-1-carrying IncHI2 plasmids, like pSE08-00436-1 from a Salmonella enterica strain recovered from a chicken (GenBank accession no. CP020493) in Germany (Figure 3) and belonged to ST4. IncHI2 plasmid backbones were composed of regions for replication (reHI2), conjugative transfer (tra genes), and plasmid maintenance (par gene). Additionally, IncHI2 plasmids (except pMCR_1139_D1) carried tellurium resistance genes (terZABCDEF), commonly associated with this plasmid family, in addition to terY1, terY2, and terW (Zingali et al., 2020). Also, IncHI2 plasmids (except pMCR_915_E1 and pMCR_1085_C1) carried the operons encoding sil and pco, conferring resistance to copper and silver. In all IncHI2 plasmids, mcr-1.1 gene was inserted downstream the terY2 gene, as observed in other IncHI2 plasmids like pSE08-00436-1 (GenBank accession no. CP020493) and pEGY1-MCR-1 (GenBank accession no. CP023143). In plasmids pMCR_170_D1, pMCR_915_E1, and pMCR_1085_C1, mcr-1.1 gene was bounded by an ISApl1 element and an ORF encoding PAP2 transmembrane protein while in pMCR_915_C1, an IS1 was found upstream mcr-1.1 gene. Deletion of the second copy of ISApl1 element, flanking the mcr-1.1 segment, was observed in the IncHI2 plasmids mentioned above. As mentioned above, in IncHI2 plasmid pMCR_1139_D1, mcr-1.1 gene was truncated at the 3′ end due to insertion of a second ISApl1 element. Insertion of ISApl1 also deleted the ORF encoding PAP2 transmembrane protein and the adjacent ter operon.
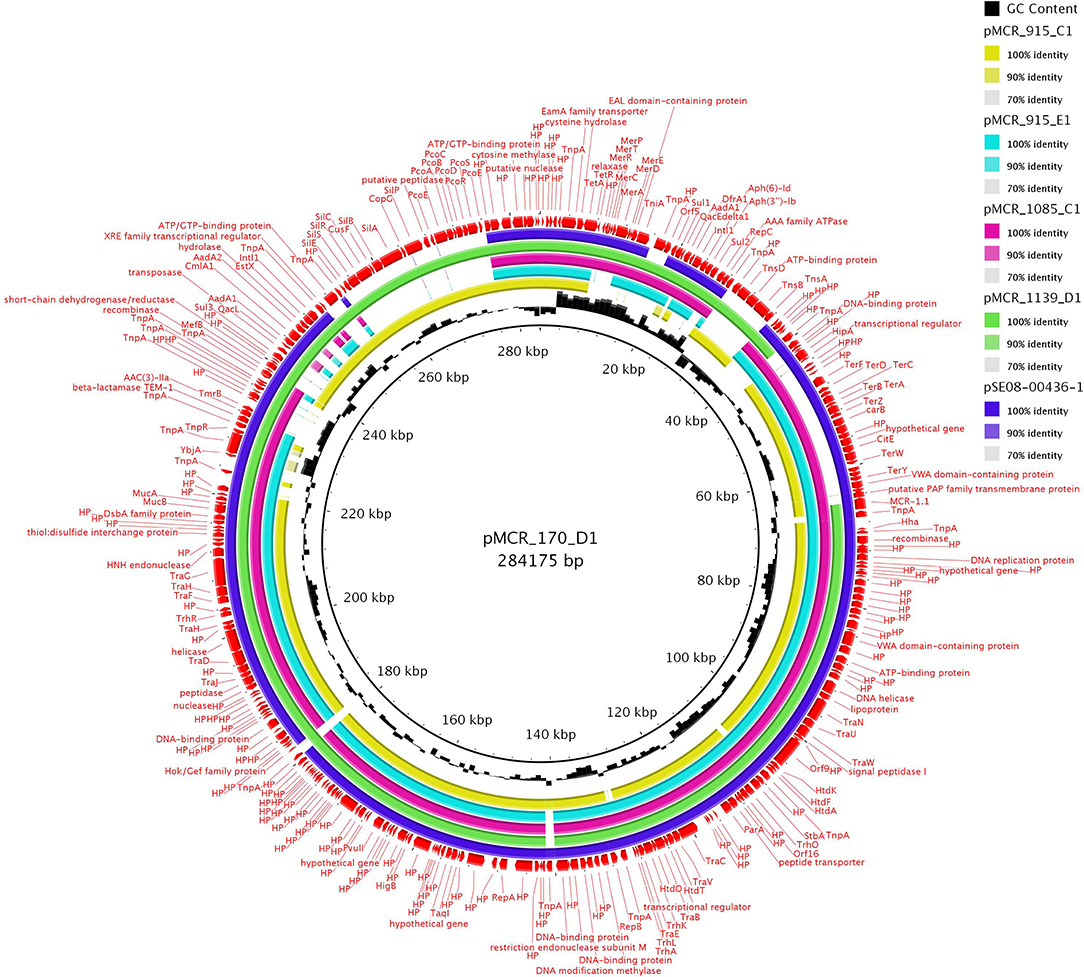
Figure 3. Circular representation of IncHI2 mcr-1-encoding plasmids. Plasmid pMCR_170_D1 (GenBank accession no. MT929288) was used as a reference for the comparison.
Additionally, at least one MDR region was identified in each IncHI2 plasmid. MDR regions ranged from 18,305 to 63,635 bp in size (Table 2). In all IncHI2 plasmids, the MDR regions were inserted in the same site, including a Tn1721-specific tetracycline module (Figure 4). The Tn21-specific mercury resistance module was found next to Tn1721-specific sequence in plasmids pMCR_170_D1, pMCR_1085_C1, and pMCR_1139_D1. The latter plasmids included also the class 1 integron In369, comprising dfrA1b and aadA1b cassettes, and a streptomycin resistance module, consisting of sul2, strA, and strB. The streptomycin resistance module has originated from plasmid RSF1010 (Yau et al., 2010), as indicated by IncQ-derived segment (nts 23,451–27,940 in pMCR_170_D1) containing repAC operon found next to it. Additionally, the sul3-associated class 1 integron, In641, carrying the estX-3, psp, aadA2, cmlA1, aadA1a, and qacH2 cassettes, was identified in plasmids pMCR_170_D1, pMCR_915_C1, and pMCR_1139_D1. In641 was followed by tnp440 transposase and a sul3-orfB-orfA-mefB module. The Tn21 transposition module, and the blaTEM−1 and aac(3)-IIa resistance genes were also found in the MDR regions of pMCR_170_D1 and pMCR_1139_D1.
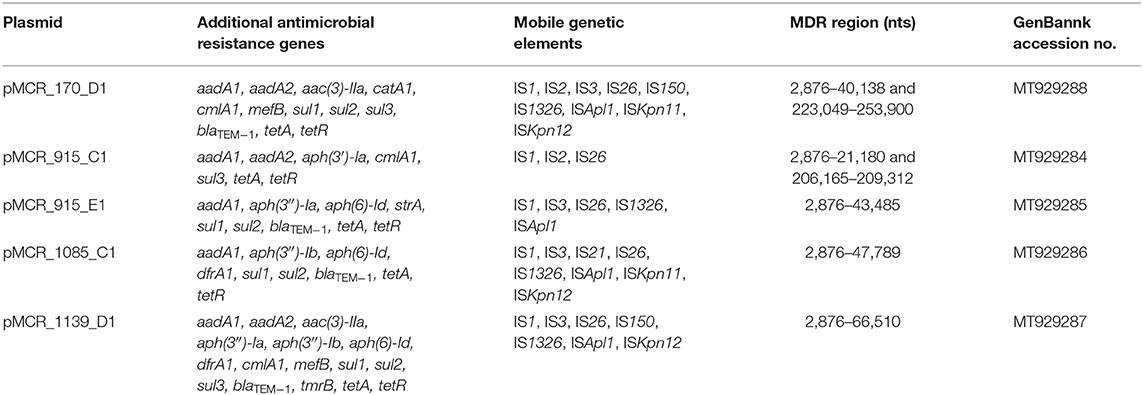
Table 2. The additional antimicrobial resistance genes and mobile genetic elements in IncHI2 plasmids.
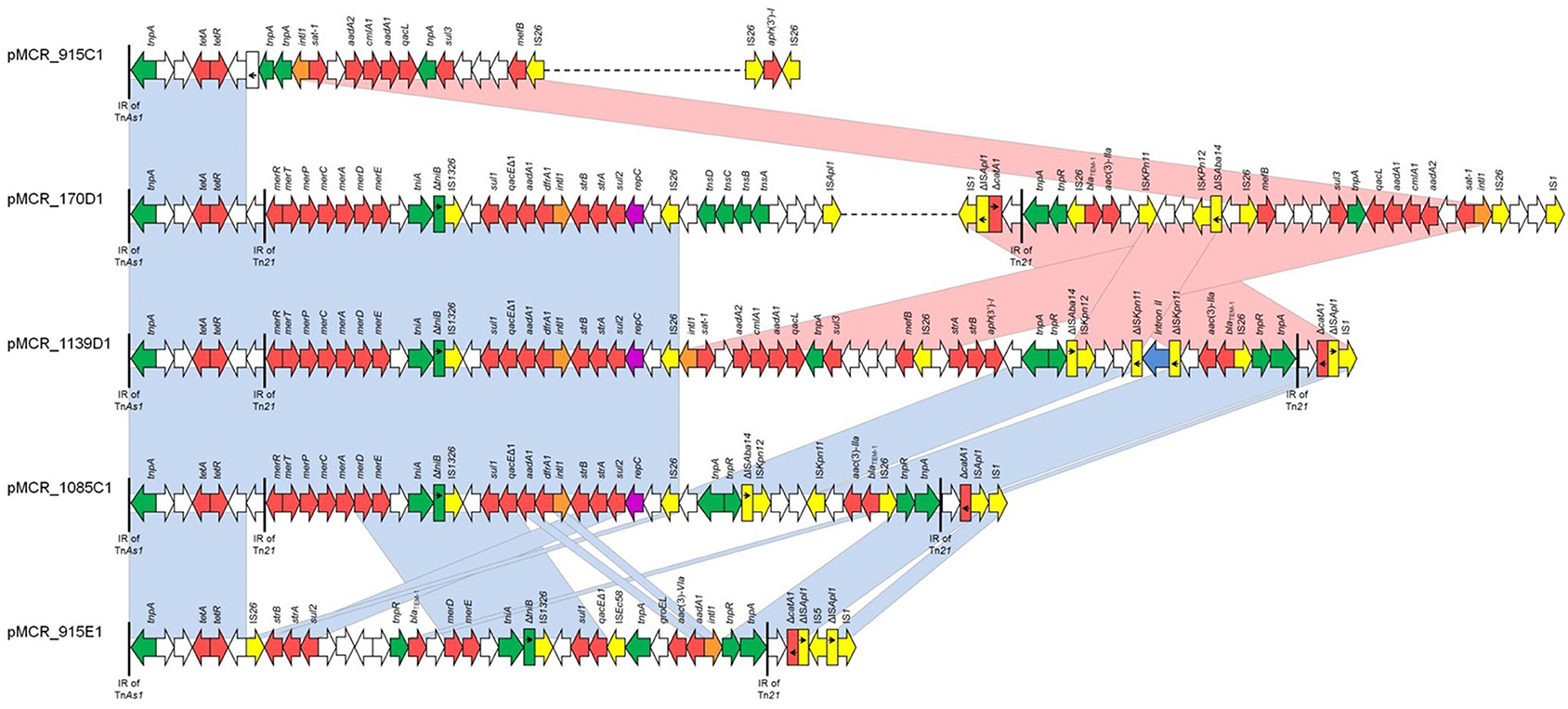
Figure 4. Linear maps of the multidrug resistance regions of IncHI2 plasmids, carrying mcr-1.1 genes. Arrows show the direction of transcription of open reading frames (ORFs), while truncated ORFs appear as rectangles (arrows within rectangles indicate the direction of transcription). Antimicrobial resistance genes are shown in red. IS elements and transposases are shown in yellow and green, respectively. intI1 genes are shaded orange. The remaining genes are shown in white. Homologous segments (representing ≥99% sequence identity) are indicated by light blue shading, while pink shading shows inverted homologous segments.
Furthermore, a composite transposon containing kanamycin-resistance gene aphA1 bounded by two IS26 elements in inverse orientation was identified in a distinct region of pMCR_915_C1.
On the other hand, in plasmid pMCR_915_E1, a streptomycin resistance module bounded by an IS26, and a module with the blaTEM−1b gene derived from a Tn2 transposon were found next to Tn1721-specific sequence. These modules have been previously described in Tn6029-like transposons (Chowdhury et al., 2015). Also, pMCR_915_C1 included the class 1 integron In2 inserted in the Tn21 backbone. Finally, in all IncHI2 plasmids, several intact and defective mobile elements that could be involved in further reorganization of MDR regions were identified (Figure 4).
Discussion
The plasmid-mediated colistin resistance in Enterobacterales has been reported worldwide (Monte et al., 2017; Tijet et al., 2017; Wang et al., 2018). In our study, 16 mcr-1.1-carrying plasmids, belonging to three different Inc groups (IncI2, IncX4, and IncHI2) and originating from different geographical areas were completely sequenced, closed and their comparative analysis was performed. IncI2, IncX4, and IncHI2 plasmid groups have been frequently reported in association with mcr-1 gene (Li et al., 2017; Matamoros et al., 2017; Tijet et al., 2017), highlighting their involvement in the dissemination of this important resistance determinant. IncI2 plasmids characterized from bacteria recovered from raw rabbit meat imported from China, exhibited high sequence homology to previously characterized plasmid pHNSHP45 from China. This finding, in agreement with the data of a previous study (Matamoros et al., 2017), confirmed the origin of IncI2 plasmid, carrying mcr-1.1 resistance gene, from China. The first described IncI2 plasmid carrying mcr-1 gene originated from E. coli from swine in China (Liu et al., 2016). On the other hand, IncX4 mcr-1.1-carrying plasmids, which were highly similar to each other, originated from raw turkey meat and liver of different geographical areas including Czech Republic, Brazil, Poland, and Germany. The occurrence of IncX4 plasmids carrying mcr-1 of poultry meat origin has been reported in Brazil (Moreno et al., 2019), China (Sun et al., 2017), South America (Monte et al., 2017), and Switzerland (Donà et al., 2017). These data confirmed the worldwide spread of IncX4 mcr-1.1-carrying plasmids, underlining the important role of IncX4 plasmid group in the dissemination of clinically significant resistance determinants. Interestingly, in most IncI2 and IncX4 plasmids carrying mcr-1.1, no insertion sequence (IS) that could be involved in the spread of the specific resistance gene was found. Previous reports have also described the absence of ISs in association with mcr genes (Caltagirone et al., 2017; Donà et al., 2017). Only, in IncX4 plasmid pMCR_1525_C2 isolated from K. pneumoniae ST11 originating from Brazil, an IS1A element was upstream of mcr-1.1. The presence of IS1-like elements upstream of mcr genes has been previously reported only in IncHI2 plasmids pASSD2-MCR1 (GenBank accession no. KX856065) and p2017.02.01CC (GenBank accession no. LC511657). This finding underlines the plethora of mobile genetic elements that could be involved in the spread of resistance determinants, as mcr genes.
Furthermore, IncHI2 plasmids were only found in raw turkey meat imported from Poland. A previous report revealed a regional spread of IncHI2 plasmids, carrying mcr-1 gene, in Europe (Matamoros et al., 2017). In addition, IncHI2 plasmids, sequenced during this study, exhibited closely related to mcr-1-carrying IncHI2 plasmid pSE08-00436-1 from a Salmonella enterica strain recovered from a chicken (GenBank accession no. CP020493) in Germany. This finding further highlighted the important role of IncHI2 plasmid family in the dissemination of mcr-1 resistance determinant in Europe. However, IncHI2 plasmids, carrying mcr-1 gene, showed higher diversity than other plasmid groups. As previously described in other IncHI2 plasmids (Wyrsch et al., 2015; Zingali et al., 2020), diversity of these molecules was mainly observed in MDR regions, and could be explained by acquisition and/or loss of transposons, insertion sequences, and antimicrobial resistance genes. In addition, mobile elements play a significant role in the formation of hybrid plasmids and instances where resistance plasmids have fused are known (Mangat et al., 2017). As such, the presence of several mobile elements in IncHI2 plasmids represents a potential hotspot for the introduction of new resistance gene cargo. Additionally, differences among IncHI2 plasmids were observed in the presence/absence of regions involved in resistance to tellurite, silver and copper. These results are in line with previous data (Gilmour et al., 2004), showing that IncHI2 plasmids are highly heterologous and evolve through acquisition/loss of mobile genetic elements. Additionally, previous studies have reported that IncHI2 plasmids are characterized by the presence of different operons conferring resistance to heavy metals (Gilmour et al., 2004; Wyrsch et al., 2015; Fang et al., 2016). Heavy metals are found in disinfectants, soil fertilizers and livestock feed and are recognized as environmental pollutants. Therefore, it is common to identify bacterial populations, with genetic determinants conferring resistance to heavy metals, in the gastrointestinal flora of intensively reared farm animal species. Previous studies have suggested that the presence of pco and sil operons in IncHI2 plasmids may have arisen due to co-selection pressures afforded by the use of heavy metals in feed additives (Wyrsch et al., 2015; Zingali et al., 2020). In IncHI2 plasmids, mcr-1.1 gene was found downstream of the mobile element ISApl1. ISApl1 element has been previously associated with the mobilization and/or stability of mcr-1 gene (Sun et al., 2016; Partridge et al., 2018), found in various plasmids belonging to different Inc groups. Although, plasmid pMCR_1139_D1 carried a truncated mcr-1.1 gene the E. coli 1139D1 strain was phenotypically resistant to colistin (MIC = 4 mg/L).
Our study described complete nucleotide sequences of IncX4, IncI2, and IncHI2 plasmids, carrying mcr-1.1, from Enterobacterales isolates originating from retail meat of different geographical origins. The presence of mcr-1.1 gene in Enterobacterales from retail meat has been previously reported in studies from different countries (Kluytmans-van den Bergh et al., 2016; Zajac et al., 2019; Hassen et al., 2020), pointing out the role of food-producing animals, and retail meat, as reservoirs of mcr-1-carrying bacteria. In the studies mentioned above, spread of mcr-1.1 gene was associated with IncX4, IncI2, and IncHI2 plasmid types. These findings confirmed the involvement of IncX4, IncI2, and IncHI2 plasmids in the dissemination of mcr-1.1 gene in several environmental niches, as in samples of retail meat originating from different geographical regions. In agreement with the previous data (Matamoros et al., 2017), our findings demonstrated the increased stability of IncX4 and IncI2 plasmids carrying mcr-1.1 gene. Furthermore, in contrast to IncX4 and IncI2, IncHI2 plasmids were more diverse due to acquisition of transposons and antimicrobial resistance genes, conferring multidrug resistance and thus posing a public health threat. Colistin is recommended for the treatment of gastrointestinal infections caused by non-invasive E. coli, mainly in pigs, chickens, turkey, calves, and sheep. Thus, extensive consumption of colistin by farm animals may indicate the reason for the dissemination of mcr-1-carrying plasmids in turkey and rabbit meat. Therefore, the animal and food market can contribute to the worldwide spread of colistin-resistant bacteria. However, extensive studies combining antimicrobial consumption and resistance are limited. In conclusion, the spread of mcr-1.1 gene in animal and food market is an alarming situation having public health, ecological and economical effects, justifying the need of surveillance programs on colistin-resistant bacteria in farm animals, especially in poultry, and in slaughterhouses.
Data Availability Statement
The datasets generated for this study can be found in online repositories. The names of the repository/repositories and accession number(s) can be found in the article/Supplementary Material.
Author Contributions
MZ performed the laboratory work, data analysis, and prepared the manuscript. CP performed the data analysis and prepared the manuscript. AV and MM performed the bioinformatic analysis of whole-genome sequencing data. IB and JH performed the PacBio sequencing. TG and RK provided the samples. AB helped with data analysis. IK helped with the laboratory work. MD supervised the project. All authors discussed the results.
Funding
This study was founded by Czech Research Health Council NV18-09-00605 and Ministry of Agriculture of the Czech Republic, institutional support MZE-RO0518.
Conflict of Interest
The authors declare that the research was conducted in the absence of any commercial or financial relationships that could be construed as a potential conflict of interest.
Acknowledgments
We thank to Ivana Jamborova and Petra Sismova for their assistance in the laboratory.
Supplementary Material
The Supplementary Material for this article can be found online at: https://www.frontiersin.org/articles/10.3389/fmicb.2020.604067/full#supplementary-material
References
Caltagirone, M., Nucleo, E., Spalla, M., Zara, F., Novazzi, F., Marchetti, V. M., et al. (2017). Occurrence of extended spectrum β-lactamases, KPC-type, and MCR-1.2-producing Enterobacteriaceae from wells, river water, and wastewater treatment plants in Oltrepò Pavese area, Northern Italy. Front. Microbiol. 8:2232. doi: 10.3389/fmicb.2017.02232
Carattoli, A. (2013). Plasmids and the spread of resistance. Int. J. Med. Microbiol. 303, 298–304. doi: 10.1016/j.ijmm.2013.02.001
Carattoli, A., Zankari, E., García-Fernández, A., Larsen, M. V., Lund, O., Villa, L. and Hasman, H. (2014). In silico detection and typing of plasmids using PlasmidFinder and plasmid multilocus sequence typing. Antimicrob. Agents Chemother. 58, 3895–3903. doi: 10.1128/AAC.02412-14
Chin, C.S., Alexander, D.H., Marks, P., Klammer, A.A., Drake, J., Heiner, C., et al. (2013). Nonhybrid, finished microbial genome assemblies from long-read SMRT sequencing data. Nat. Methods 10, 563–569. doi: 10.1038/nmeth.2474
Chowdhury, P. R., Charles, I. G., and Djordjevic, S. P. (2015). A role for Tn6029 in the evolution of the complex antibiotic resistance gene loci in genomic island 3 in enteroaggregative hemorrhagic Escherichia coli O104:H4. PLoS ONE 10:e0115781. doi: 10.1371/journal.pone.0115781
Donà, V., Bernasconi, O. J., Pires, J., Collaud, A., Overesch, G., Ramette, A., et al. (2017). Heterogeneous genetic location of mcr-1 in colistin-resistant Escherichia coli isolates from humans and retail chicken meat in Switzerland: emergence of mcr-1-carrying IncK2 plasmids. Antimicrob. Agents Chemother. 61:e01245-17. doi: 10.1128/AAC.01245-17
Doumith, M., Godbole, G., Ashton, P., Larkin, L., Dallman, T., Day, M. and Johnson, A. P. (2016). Detection of the plasmid-mediated mcr-1 gene conferring colistin resistance in human and food isolates of Salmonella enterica and Escherichia coli in England and Wales. J. Antimicrob. Chemother. 71, 2300–2305. doi: 10.1093/jac/dkw093
Falagas, M.E., Rafailidis, P.I., and Matthaiou, D.K. (2010). Resistance to polymyxins: mechanisms, frequency and treatment options. Drug Resist. Update 13, 132–138. doi: 10.1016/j.drup.2010.05.002
Fang, L., Li, X., Li, L., Li, S., Liao, X., Sun, J. and Liu, Y. (2016). Co-spread of metal and antibiotic resistance within ST3-IncHI2 plasmids from E. coli isolates of food-producing animals. Sci. Rep. 6:25312. doi: 10.1038/srep25312
Gelbíčová, T., Barakova, A., Florianová, M., Jamborova, I., Zelendova, M., Pospisilova, L. et al. (2019). Dissemination and comparison of genetic determinants of mcr-mediated colistin resistance in Enterobacteriaceae via retailed raw meat products. Front. Microbiol. 10:2824. doi: 10.3389/fmicb.2019.02824
Gilmour, M.W., Thomson, N.R., Sanders, M., Parkhill, J., and Taylor, D.E. (2004). The complete nucleotide sequence of the resistance plasmid R478: defining the backbone components of incompatibility group H conjugative plasmids through comparative genomics. Plasmid 52, 182–202. doi: 10.1016/j.plasmid.2004.06.006
Hassen, B., Abbassi, M.S., Ruiz-Ripa, L., Mama, O.M., Hassen, A., Torres, C., et al. (2020). High prevalence of mcr-1 encoding colistin resistance and first identification of blaCTX−M−55 in ESBL/CMY-2-producing Escherichia coli isolated from chicken faeces and retail meat in Tunisia. Int. J. Food Microbiol. 318:108478. doi: 10.1016/j.ijfoodmicro.2019.108478
Kluytmans-van den Bergh, M.F., Huizinga, P., Bonten, M.J., Bos, M., De Bruyne, K., Friedrich, A.W., et al. (2016). Presence of mcr-1-positive Enterobacteriaceae in retail chicken meat but not in humans in the Netherlands since 2009. Euro Surveill. 21:30149. doi: 10.2807/1560-7917.ES.2016.21.9.30149
Landman, D., Georgescu, C., Martin, D. A., and Quale, J. (2008). Polymyxins revisited. Clin. Microbiol. Rev. 21, 449–465. doi: 10.1128/CMR.00006-08
Li, R., Xie, M., Zhang, J., Yang, Z., Liu, L., Liu, X. and Chen, S. (2017). Genetic characterization of mcr-1-bearing plasmids to depict molecular mechanisms underlying dissemination of the colistin resistance determinant. J. Antimicrob. Chemother. 72, 393–401. doi: 10.1093/jac/dkw411
Liu, Y. Y., Wang, Y., Walsh, T. R., Yi, L. X., Zhang, R., Spencer, J. and Yu, L. F. (2016). Emergence of plasmid-mediated colistin resistance mechanism MCR-1 in animals and human beings in China: a microbiological and molecular biological study. Lancet Infect. Dis. 16, 161–168. doi: 10.1016/S1473-3099(15)00424-7
Mangat, C. S., Bekal, S., Irwin, R. J. and Mulvey, M. R. (2017). A novel hybrid plasmid carrying multiple antimicrobial resistance and virulence genes in Salmonella enterica serovar Dublin. Antimicrob. Agents Chemother. 61:e02601-16. doi: 10.1128/AAC.02601-16
Matamoros, S., Van Hattem, J. M., Arcilla, M. S., Willemse, N., Melles, D. C., Penders J., et al. (2017). Global phylogenetic analysis of Escherichia coli and plasmids carrying the mcr-1 gene indicates bacterial diversity but plasmid restriction. Sci. Rep. 7:15364. doi: 10.1038/s41598-017-15539-7
Monte, D. F., Fernandes, M. R., Cerdeira, L., Esposito, F., Galvão, J. A., Franco, B. D. and Landgraf, M. (2017). Chicken meat as a reservoir of colistin-resistant Escherichia coli strains carrying mcr-1 genes in South America. Antimicrob. Agents Chemother. 61:e02718-16. doi: 10.1128/AAC.02718-16
Moreno, L. Z., Gomes, V. T., Moreira, J., de Oliveira, C. H., Peres, B. P., Silva, A. P. S., et al. (2019). First report of mcr-1-harboring Salmonella enterica serovar Schwarzengrund isolated from poultry meat in Brazil. Diagn. Microbiol. Infect. Dis. 93, 376–379. doi: 10.1016/j.diagmicrobio.2018.10.016
Moura, A., Soares, M., Pereira, C., Leitão, N., Henriques, I., and Correia, A. (2009). INTEGRALL: a database and search engine for integrons, integrases and gene cassettes. Bioinformatics 25, 1096–1098. doi: 10.1093/bioinformatics/btp105
Olaitan, A. O., Morand, S., and Rolain, J. M. (2014). Mechanisms of polymyxin resistance: acquired and intrinsic resistance in bacteria. Front. Microbiol. 5:643. doi: 10.3389/fmicb.2014.00643
Partridge, S.R., Kwong, S.M., Firth, N. and Jensen, S.O. (2018). Mobile genetic elements associated with antimicrobial resistance. Clin. Microbiol. Rev. 31:e00088-17. doi: 10.1128/CMR.00088-17
Poirel, L., Jayol, A., and Nordmann, P. (2017). Polymyxins: antibacterial activity, susceptibility testing, and resistance mechanisms encoded by plasmids or chromosomes. Clin. Microbiol. Rev. 30, 557–596. doi: 10.1128/CMR.00064-16
Rozwandowicz, M., Brouwer, M.S.M., Fischer, J., Wagenaar, J.A., Gonzalez-Zorn, B., Guerra, B., et al. (2018). Plasmids carrying antimicrobial resistance genes in Enterobacteriaceae. J. Antimicrob. Chemother. 73, 1121–1137. doi: 10.1093/jac/dkx488
Sun, J., Fang, L. X., Wu, Z., Deng, H., Yang, R. S., Li, X. P., et al. (2017). Genetic analysis of the IncX4 plasmids: implications for a unique pattern in the mcr-1 acquisition. Sci. Rep. 7:424. doi: 10.1038/s41598-017-00095-x
Sun, J., Li, X. P., Yang, R. S., Fang, L. X., Huo, W., Li, S. M. and Liu, Y. H. (2016). Complete nucleotide sequence of an IncI2 plasmid coharboring blaCTX-M-55 and mcr-1. Antimicrob. Agents Chemother. 60, 5014–5017. doi: 10.1128/AAC.00774-16
Tijet, N., Faccone, D., Rapoport, M., Seah, C., Pasteran, F., Ceriana, P. and Melano, R. G. (2017). Molecular characteristics of mcr-1-carrying plasmids and new mcr-1 variant recovered from polyclonal clinical Escherichia coli from Argentina and Canada. PLoS ONE. 12:e0180347. doi: 10.1371/journal.pone.0180347
Wang, C., Feng, Y., Liu, L., Wei, L., Kang, M. and Zong, Z. (2020). Identification of novel mobile colistin resistance gene mcr-10. Emerg. Microbes Infect. 9, 508–516. doi: 10.1080/22221751.2020.1732231
Wang, R., van Dorp, L., Shaw, L. P., Bradley, P., Wang, Q., Wang, X. and Dorai-Schneiders, T. (2018). The global distribution and spread of the mobilized colistin resistance gene mcr-1. Nat. Commun. 9, 1–9. doi: 10.1038/s41467-018-03205-z
Wyrsch, E., Chowdhury, P. R., Abraham, S., Santos, J., Darling, A.E., Charles, I.G., et al. (2015). Comparative genomic analysis of a multiple antimicrobial resistant enterotoxigenic E. coli O157 lineage from Australian pigs. BMC Genomics. 16:165. doi: 10.1186/s12864-015-1382-y
Xavier, B. B., Lammens, C., Butaye, P., Goossens, H., and Malhotra-Kumar, S. (2016). Complete sequence of an IncFII plasmid harbouring the colistin resistance gene mcr-1 isolated from Belgian pig farms. J. Antimicrob. Chemother. 71, 2342–2344. doi: 10.1093/jac/dkw191
Yau, S., Liu, X., Djordjevic, S.P. and Hall, R.M. (2010). RSF1010-like plasmids in Australian Salmonella enterica serovar Typhimurium and origin of their sul2-strA-strB antibiotic resistance gene cluster. Microb. Drug. Resist. 16, 249–252. doi: 10.1089/mdr.2010.0033
Zajac, M., Sztromwasser, P., Bortolaia, V., Leekitcharoenphon, P., Cavaco, L.M., Ziȩtek-Barszcz, A., et al. (2019). Occurrence and characterization of mcr-1-positive Escherichia coli isolated from food-producing animals in Poland, 2011–2016. Front Microbiol. 10:1753. doi: 10.3389/fmicb.2019.01753
Zankari, E., Hasman, H., Cosentino, S., Vestergaard, M., Rasmussen, S., Lund, O. and Larsen, M. V. (2012). Identification of acquired antimicrobial resistance genes. J Antimicrob. Chemother. 67, 2640–2644. doi: 10.1093/jac/dks261
Keywords: antimicrobial resistance, colistin, IncHI2, IncI2, IncX4, meat
Citation: Zelendova M, Papagiannitsis CC, Valcek A, Medvecky M, Bitar I, Hrabak J, Gelbicova T, Barakova A, Kutilova I, Karpiskova R and Dolejska M (2021) Characterization of the Complete Nucleotide Sequences of mcr-1-Encoding Plasmids From Enterobacterales Isolates in Retailed Raw Meat Products From the Czech Republic. Front. Microbiol. 11:604067. doi: 10.3389/fmicb.2020.604067
Received: 08 September 2020; Accepted: 21 December 2020;
Published: 15 January 2021.
Edited by:
Jørgen J. Leisner, University of Copenhagen, DenmarkReviewed by:
Magdalena Popowska, University of Warsaw, PolandJoão Pedro Rueda Furlan, University of São Paulo, Brazil
Copyright © 2021 Zelendova, Papagiannitsis, Valcek, Medvecky, Bitar, Hrabak, Gelbicova, Barakova, Kutilova, Karpiskova and Dolejska. This is an open-access article distributed under the terms of the Creative Commons Attribution License (CC BY). The use, distribution or reproduction in other forums is permitted, provided the original author(s) and the copyright owner(s) are credited and that the original publication in this journal is cited, in accordance with accepted academic practice. No use, distribution or reproduction is permitted which does not comply with these terms.
*Correspondence: Monika Dolejska, bW9uaWthLmRvbGVqc2thQGdtYWlsLmNvbQ==