- 1Department of Microbiology, Radboud University, Nijmegen, Netherlands
- 2Institute for Biological Interfaces 5, Karlsruhe Institute of Technology, Eggenstein-Leopoldshafen, Germany
- 3Leibniz Institute DSMZ, Braunschweig, Germany
- 4Faculty for Energy-, Process- and Bioengineering, University of Stuttgart, Stuttgart, Germany
- 5Institute of Biomaterials and Biomolecular Systems, University of Stuttgart, Stuttgart, Germany
- 6Central Facility for Microscopy, Helmholtz Centre for Infection Research, Braunschweig, Germany
- 7Department of Microbial Interactions, Institute of Microbiology, Friedrich Schiller University, Jena, Germany
Marine ecosystems serve as global carbon sinks and nutrient source or breeding ground for aquatic animals. Sponges are ancient parts of these important ecosystems and can be found in caves, the deep-sea, clear waters, or more turbid environments. Here, we studied the bacterial community composition of the calcareous sponge Clathrina clathrus sampled close to the island Corsica in the Mediterranean Sea with an emphasis on planctomycetes. We show that the phylum Planctomycetes accounts for 9% of the C. clathrus-associated bacterial community, a 5-fold enrichment compared to the surrounding seawater. Indeed, the use of C. clathrus as a yet untapped source of novel planctomycetal strains led to the isolation of strain KS4T. The strain represents a novel genus and species within the class Phycisphaerae in the phylum Planctomycetes and displays interesting cell biological features, such as formation of outer membrane vesicles and an unexpected mode of cell division.
Introduction
Aquatic ecosystems comprise biological communities of organisms, which together shape a local habitat. Such ecosystems include kelp forests, seagrass meadows, salt marshes, and coral reefs, which are occupied by photosynthetic primary producers, animals, and microbial communities that live in interaction with each other (Foster and Schiel, 1985; Häder et al., 1998). Sponges are ubiquitous, ancient and environmentally important members of such marine ecosystems. They are common in clear waters of tropic reefs, such as the Caribbean, but can also be found in caves, the deep-sea, and more turbid environments (Hooper and van Soest, 2002). Approximately 5% of the over 8,000 known species of the phylum Porifera are calcareous sponges (class Calcarea), which prefer shallow water and are often hidden in cave areas. Species of this class have a calcareous skeletal network (instead of a siliceous skeleton) and lack chitin (Ruys, 2013; Żółtowska, 2019). With a special interest in the relevance of the bacterial phylum Planctomycetes, here we analyzed the bacterial community composition on the surface of the calcareous sponge Clathrina clathrus Schmidt, 1864, which has a yellow color, reaches sizes of up to 10 cm, and inhabits the Atlantic coast of Western Europe and coasts in the Mediterranean Sea (Burton, 1963; Hooper and van Soest, 2002). Previous cultivation-dependent analyses of bacterial communities associated with C. clathrus showed that α- and γ-proteobacteria may account for up to 75–90% of the phylogenetic bacterial diversity (Muscholl-Silberhorn et al., 2008; Roué et al., 2012). The residual 10–25% were attributed to Firmicutes and Actinobacteria. However, although not previously found as dominant members in C. clathrus-associated bacterial communities, strains belonging to the phylum Planctomycetes are frequently isolated from marine and freshwater sponges (Pimentel-Elardo et al., 2003; Gade et al., 2004; Izumi et al., 2013; Kohn et al., 2020b). Members of Planctomycetia, the class within the phylum Planctomycetes with the currently highest number of described species, are often found attached to biotic and abiotic marine surfaces, on which they can be highly abundant (Lage and Bondoso, 2014; Wiegand et al., 2018). Once attached to a biotic surface, e.g., a macroscopic phototroph, planctomycetes are thought to use released polysaccharides as carbon and energy source probably using a specialized system for uptake of entire high-molecular weight sugar molecules and subsequent intracellular degradation (Boedeker et al., 2017). Employing such a system represents a decisive advantage compared to other, often faster growing bacteria inhabiting the same ecological niche, e.g., members of the “Roseobacter group” (Frank et al., 2015). Many of such competitors use catabolic exoenzymes for an extracellular cleavage of polysaccharides, a strategy which provides easily available carbon sources also to other members of the bacterial community. Other planctomycetal characteristics, such as the potential for production of secondary metabolites or the observed resistance to several antibiotics, might also contribute to the compensation of lower growth rates of planctomycetes (Jeske et al., 2013; Godinho et al., 2019) and are worth to be investigated in more detail.
Well-established methods, however, can often not be applied for planctomycetes without extensive optimization. Members of this phylum likely escaped detection due to the use of mismatching 16S rRNA gene primers and due to cultivation bias discriminating against slow-growing bacteria (for review see Wiegand et al., 2018). This can provide an explanation why they have not been captured in previous cultivation-dependent studies. A similar argumentation might be applicable for a previous analysis of a C. clathrus-associated bacterial community, in which γ- and α-Proteobacteria turned out to be the bacterial classes with the highest relative abundances of 83 and 14%, respectively (Quévrain et al., 2014). Similar observations were made for the related calcareous sponge Leuconia johnstoni, for which the relative abundance of γ-Proteobacteria was slightly lower (61%), but instead a higher abundance of Bacteroidetes (12%) and Firmicutes (9%) was determined (Quévrain et al., 2014). In the above-mentioned study, the authors followed a cultivation-dependent approach and incubated enriched bacterial cell suspensions extracted from calcareous sponges on nutrient-rich marine agar plates. Plates were cultivated for only 2 days at a relatively low temperature of 18°C. These conditions are not suitable for the isolation of planctomycetes, which have generation times ranging from 8 h to several days and typically show optimal growth at temperatures of 26–30°C (Wiegand et al., 2018). In addition to different optimal conditions of bacterial species inhabiting such communities, e.g., with regard to temperature, pH, and NaCl concentration, differences in growth rates inevitably lead to biased abundance of different bacterial phyla, favoring fast-growing species well-adapted to the used cultivation medium. A related study showed that members of the phylum Spirochaetes can be present in considerable numbers in C. clathrus-associated communities, but escaped previously performed analyses (Neulinger et al., 2010). The recent development of novel tools and laboratory techniques motivated us to reinvestigate calcareous sponges for the occurrence of planctomycetes (Jogler and Jogler, 2013; Wiegand et al., 2020). For this purpose, we included a cultivation-independent analysis of the bacterial community of C. clathrus sampled near the coast of the island Corsica in the Mediterranean Sea (Figures 1A–C). By using an optimized medium formulation, we were also able to isolate planctomycete strain KS4T, a novel member of the class Phycisphaerae, from C. clathrus and analyzed its phylogenetic position, as well as phenotypic, genomic and genome-encoded features. The description of strain KS4T and access to an axenic culture are prerequisite to further investigate the barely studied class Phycisphaerae in the phylum Planctomycetes. In the long run, the large observed differences in the natural abundance of planctomycetes on different marine biotic surfaces (ranging from <1 to over 80%) can be analyzed based on presented sequencing data in this and closely related studies.
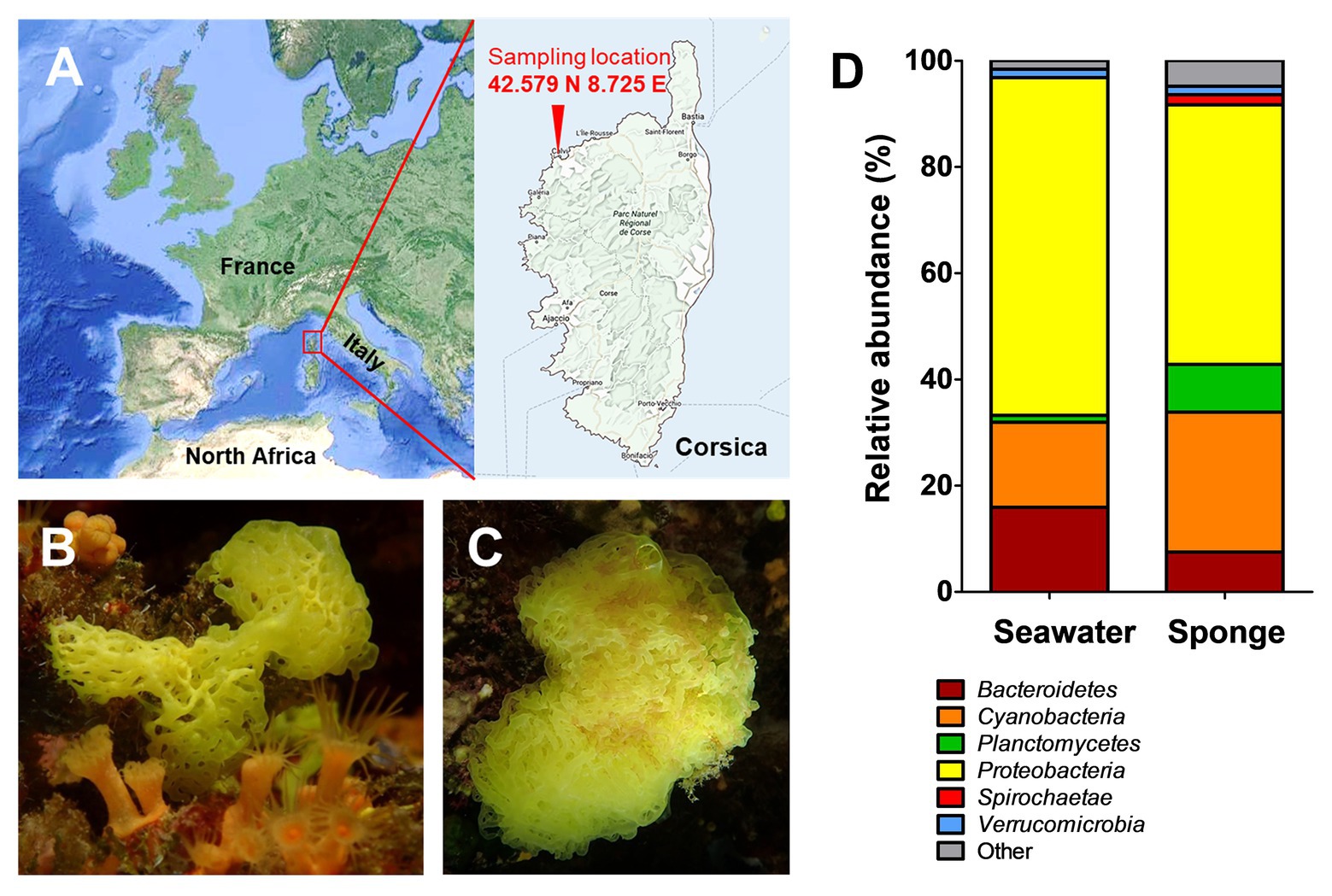
Figure 1. Sampling location and results of the cultivation-independent analysis of the Clathrina clathrus-associated bacterial community. Samples were taken close to Calvi on the Northern coast of the island Corsica, France (A; exact coordinates are given in the picture). Picture of the investigated calcareous sponge as side view (B) and top view (C). Determination of the bacterial community composition of C. clathrus on bacterial phylum level compared to surrounding seawater (D). Data for the surrounding seawater was taken from a previous study (Kohn et al., 2020a).
Materials and Methods
Cultivation-Independent Bacterial Community Analysis
The calcareous sponge C. clathrus was sampled in September 2015 near the coast of the island Corsica (exact location: 42.579° N 8.725° E; Figure 1A). One individual was sampled, and three pieces were used for DNA extraction and pooled for further processing. The sample for DNA extraction from the sponge surface was obtained by scraping off the sponge-associated biofilm from the three pieces using single-use scalpels and resuspension of the pooled material into sterile natural seawater. The surrounding seawater was sampled at three different locations in the immediate vicinity of the sponge and further processed within 8 h. In the laboratory, the triplicate water samples (approximately 300 ml each) were homogenized by gentle stirring and subsequently filtered through a single polycarbonate membrane filter (Ø 47 mm, Isopore, Merck) with a pore size of 0.22 μm to collect planktonic bacteria. Filters were frozen and stored at −20°C until DNA extraction. Isolation of DNA and all subsequent steps for amplification of DNA and amplicon sequencing were performed as previously described (Kohn et al., 2020a). The analysis of operational taxonomic units present in the samples was performed using the SILVAngs pipeline (Quast et al., 2012). The analysis was based on 22,364 sequences of the variable V3 region of 16S rRNA genes with an average length of 143 bp. Classification of the sequences was performed using SILVA reference version 132 and BLASTn version 2.2.30+ with a sequence similarity of 93%.
Isolation of Strain KS4T and Physiological Characterization
For the isolation of strain KS4T, a small piece of C. clathrus was washed with sterile-filtered seawater, additionally supplemented with 100 mg/L cycloheximide to prevent fungal growth. Afterward, the material was swabbed over three M1H NAG ASW plates solidified with 8 g/L gellan gum and supplemented with 1,000 mg/L streptomycin, 200 mg/L ampicillin, and 20 mg/L cycloheximide (Wiegand et al., 2020). Plates were subsequently incubated at 20°C for 8 weeks and regularly inspected for the presence of colonies. Slow-growing colonies with a white or pink color were subjected to colony-PCR to amplify the 16S rRNA gene, which was subsequently sequenced. Strains identified as members of the phylum Planctomycetes, but with a 16S rRNA gene similarity below 98% to already chracterized strains, were further cultivated. Strain KS4T, isolated using the above-mentioned strategy, showed good growth also in liquid M1H NAG ASW medium (Boersma et al., 2019) and was characterized in detail. Unless otherwise stated, growth experiments were performed at pH 7.5, and 27°C. For determination of the pH optimum for growth, different buffering compounds were used: 100 mM 2-(N-morpholino)ethanesulfonic acid (MES) for pH 5–6, 100 mM 4-(2-hydroxyethyl)-1-piperazineethanesulfonic acid (HEPES) for pH 7-8, or 100 mM N-cyclohexyl-2-aminoethanesulfonic acid (CHES) for pH 9–10. In the growth experiments without either NaCl (0% w/v NaCl) or artificial seawater (ASW) (0% ASW), the medium still contained all chemical elements required for growth (see medium recipe; Wiegand et al., 2020). Concentrated ASW contained 46.94 g/L NaCl, 7.84 g/L Na2SO4, 21.28 g/L MgCl2 x 6 H2O, 2.86 g/L CaCl2 x 2 H2O, 0.384 g/L NaHCO3, 1.384 g/L KCl, 0.192 g/L KBr, 0.052 g/L H3BO3, 0.08 g/L SrCl2 x 6 H2O, and 0.006 g/L NaF. 250 ml of concentrated ASW were used for the preparation of 1 L of M1H NAG ASW medium and was considered 100% ASW. A range of 0–200% ASW was tested in the cultivation experiments.
Morphological Characterization
Phase contrast and field emission scanning electron microscopy were performed as previously described (Kohn et al., 2016).
Genome Information and Analysis of Genome-Encoded Features
The genome sequence of strain KS4T is available from GenBank under the accession number CP036425. The 16S rRNA gene sequence can be found under accession number MK559978. DNA isolation and genome sequencing are part of a previously published study (Wiegand et al., 2020). The number of carbohydrate-active enzymes was obtained from the CAZy database (Terrapon et al., 2017). Biosynthetic gene clusters associated to secondary metabolite production were determined using antiSMASH version 4.0 (Blin et al., 2017).
Phylogenetic Analysis
The 16S rRNA gene sequence-based phylogeny was computed for strain KS4T, the type strains of all described planctomycetal species (assessed in January 2020) and all isolates recently published and described (Boersma et al., 2019; Kallscheuer et al., 2019a,b,c, 2020; Dedysh et al., 2020; Kohn et al., 2020a; Peeters et al., 2020). The 16S rRNA gene sequences were aligned with SINA (Pruesse et al., 2012), and the phylogenetic inference was calculated with RAxML (Stamatakis, 2014) using a maximum likelihood approach with 1,000 bootstraps, nucleotide substitution model GTR, gamma distributed rate variation, and estimation of proportion of invariable sites (GTRGAMMAI option). The 16S rRNA genes of the following bacterial strains from the Planctomycetes-Verrucomicrobia-Chlamydiae (PVC) superphylum outside of the phylum Planctomycetes were used as the outgroup: Opitutus terrae (NCBI accession number: AJ229235), Kiritimatiella glycovorans (NCBI accession number: NR_146840), and Lentisphaera araneosa (NCBI accession number: NR_027571). For the multi-locus sequence analysis (MLSA), the unique single-copy core genome of the analyzed genomes was determined with proteinortho5 (Lechner et al., 2011) with the “selfblast” option enabled. The protein sequences of the resulting orthologous groups were aligned using MUSCLE v.3.8.31 (Edgar, 2004). After clipping, partially aligned C- and N-terminal regions and poorly aligned internal regions were filtered using Gblocks (Castresana, 2000). The final alignment was concatenated and clustered using the maximum likelihood method implemented by RAxML (Stamatakis, 2014) with the “rapid bootstrap” method and 500 bootstrap replicates. Two strains from the family Isosphaeraceae (Singulisphaera acidiphila, accession number CP003364.1, and Isosphaera pallida, accession number CP002353.1) were used as outgroup in the MLSA-based phylogenetic tree. Average nucleotide identities (ANI) were calculated using OrthoANI (Lee et al., 2016). The average amino acid identity (AAI) was calculated using the aai.rb script of the enveomics collection (Rodriguez-R and Konstantinidis, 2016) and the percentage of conserved proteins (POCP) was calculated as described (Qin et al., 2014).
Results and Discussion
Cultivation-Independent Analysis of Clathrina clathrus-Associated Bacteria
In order to get an unbiased impression of the bacterial diversity associated with C. clathrus, we followed a cultivation-independent approach based on 16S rRNA gene amplicon sequencing. The assignment of the 16S rRNA gene sequences (in this case: the variable V3 region of the 16S rRNA) to different bacterial phyla excludes most of the influence of growth characteristics and physiological properties and ensures that slower-growing or non-cultivable species are also included. Samples from the surrounding seawater published in a previous study were analyzed for comparison (Kohn et al., 2020a). During assessment of obtained data on phylum level (Figure 1D), we could confirm previous observations that Proteobacteria is the most abundant phylum, both in the C. clathrus-associated community (relative abundance of 49%) and in the surrounding seawater (relative abundance of 64%; Quévrain et al., 2014). Our cultivation-independent analysis points toward a larger diversity as suggested by the previous approach based on cultivated isolates (Quévrain et al., 2014). The phyla Cyanobacteria and Planctomycetes showed the second and third highest relative abundances in the C. clathrus-associated community of 26 and 9%, respectively (Figure 1D). While relative abundances of Cyanobacteria and Bacteroidetes in the sponge-associated community and seawater did not differ more than 2-fold, members of the phylum Planctomycetes were preferentially found attached to the sponge (9.0% relative abundance) compared to the surrounding seawater (1.3%). This finding is in line with the observed preference of planctomycetes to attach to diverse biotic and abiotic surfaces and the tendency to grow in larger aggregates (Lage and Bondoso, 2014). This often leads to the formation of flakes or stable biofilms during laboratory-scale cultivation of axenic cultures of isolated strains (Boersma et al., 2019; Kallscheuer et al., 2020). The observed presence of Spirochaetes in the sponge-associated bacterial community is in line with previously published results (Neulinger et al., 2010), while this phylum was not detected in the surrounding seawater in our dataset. Other phyla, such as Verrucomicrobia, were equally abundant in both samples (1.6% relative abundance). When comparing the ratios of abundance in the sponge-associated community and in the surrounding seawater, ratios in the range of 1.0–2.5 were observed (in case of Spirochaetes, no ratio could be calculated as the abundance in seawater was zero). Planctomycetes stood out with a ratio of 6.9, further underlining the preference of members of this phylum to live in an attached or a eukaryote-associated state. Although much higher abundances of Planctomycetes of 60–85% on biotic surfaces were observed before (Bengtsson and Øvreås, 2010; Wiegand et al., 2018), values of nearly 10% found in this study are still in sharp contrast to the results of the previously published cultivation-dependent analysis (Quévrain et al., 2014), which probably did not capture Planctomycetes due to unsuitable cultivation conditions for strains of this phylum (Kohn et al., 2020a). This said, we analyzed the sequences obtained for members of the phylum Planctomycetes in more detail and calculated relative abundances on the order/family and genus level (Figure 2). Of a total number of 22,364 obtained sequences, 2,015 sequences belong to members of the phylum Planctomycetes. For the relative abundances shown in Figure 2 and in the text below, this number was set to 100%. The majority of obtained sequences could be assigned to two planctomycetal families, namely Pirellulaceae (53%) and Planctomycetaceae (42%), together accounting for 95% of the total number of Planctomycetes-derived sequences. Abundant genera in the family Pirellulaceae include Blastopirellula (24%), Pirellula (12%), and Rhodopirellula (6%). Uncultivated Pirellulaceae accounted for 5% of the 2,015 sequences. In contrast, uncultivated members made up the majority of the family Planctomycetaceae (36%) in our dataset, while members of the genera Fuerstiella (4%) and Planctomicrobium (2%) were also found (Figure 2). Both families, Pirellulaceae and Planctomycetaceae, more specifically the respective orders, Pirellulales and Planctomycetales, belong to the class Planctomycetia, which additionally comprises the orders Gemmatales and Isosphaerales. Five (0.25%) and seven (0.35%) sequences, respectively, could be assigned to these two orders. Fifteen sequences (0.7%) belong to members of Phycisphaerae, another class within the phylum Planctomycetes.
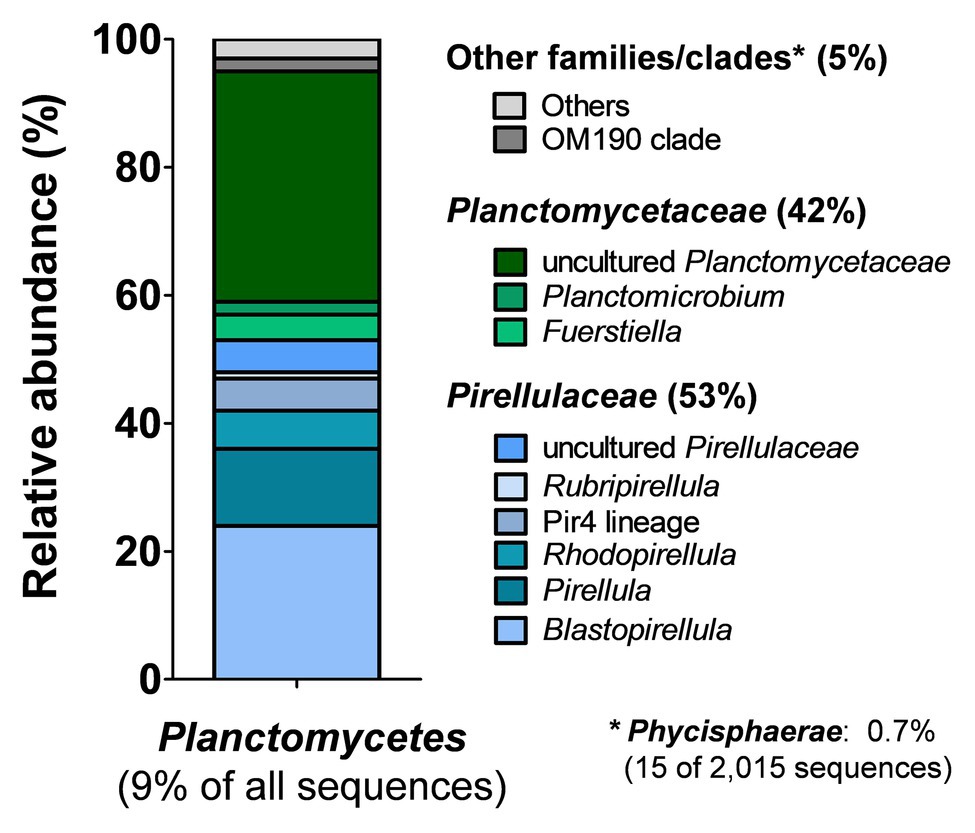
Figure 2. Analysis of amplicon sequences belonging to the phylum Planctomycetes. Of the total number of 22,364 partial 16S rRNA gene sequences, 2,015 (9.0%) belong to strains of the phylum Planctomycetes. Relative abundances with regard to the planctomycete-derived sequences (2,015 sequences = 100%) were further analyzed on the order/family or genus level.
Targeted Isolation of Novel Planctomycetes Associated to C. clathrus
Based on the results described above, we were eager to explore C. clathrus as a yet untapped source for novel planctomycetal strains. We used an established pipeline for processing the biological sampling material, isolation, and initial cultivation of planctomycetal strains (Wiegand et al., 2020). For isolation of novel planctomycetes, we typically exploit their inherent resistance against several antibiotics as the selection condition and prolong the incubation time to several weeks. Applying these two key factors, we were able to bring strain KS4T into axenic culture and here present a detailed strain characterization of the novel isolate. To the best of our knowledge, strain KS4T is the first planctomycete isolated from a calcareous sponge. The V3 region of the 16S rRNA gene sequence of strain KS4T was absent from the amplicon dataset. The highest sequence similarity of the V3 region of 88% was found to an uncultivated planctomycete strain designated GMD14H07 (GenBank accession no. AY162124). The near full length 16S rRNA gene sequence identity of 85% was even lower and, thus, probably excludes a relationship on the genus or species level (genus threshold 94.5%, species threshold 98.7%; Stackebrandt and Ebers, 2006; Yarza et al., 2014).
Phylogenetic Inference of Strain KS4T Isolated From C. clathrus
In phylogenetic trees based on 16S rRNA gene sequences and MLSA, strain KS4T clusters within the class Phycisphaerae in the phylum Planctomycetes (Fukunaga et al., 2009; Figure 3). Comparison on the level of 16S rRNA gene sequences suggests Algisphaera agarilytica 06SJR6-2T as the current closest relative (Yoon et al., 2014), since this strain showed the highest 16S rRNA gene sequence similarity of 88.7% with strain KS4T. The second closest relative is Phycisphaera mikurensis (84.6% 16S rRNA gene sequence similarity; Fukunaga et al., 2009). Based on these values, strain KS4T is likely a member of the family Phycisphaeraceae, order Phycisphaerales, class Phycisphaerae. 16S rRNA gene sequence similarities of strain KS4T with known members of the family are below the proposed genus threshold of 94.5% (Yarza et al., 2014), which delineates strain KS4T from the two described genera in the family (Figure 3A). As no genome sequence of A. agarilytica is available, the whole genome-based phylogenetic analysis only allowed comparison to P. mikurensis. The classification of strain KS4T as a member of a novel genus is supported by AAI values of 44.9% and POCP of 30.9%, both clearly below the genus thresholds of 60 and 50%, respectively (Konstantinidis and Tiedje, 2005; Qin et al., 2014). An ANI of 66.8% ensures that the novel strain does not belong to the species P. mikurensis (species threshold 95%; Kim et al., 2014). Taken together, all analyzed phylogenetic markers support the delineation of strain KS4T from the genus Phycisphaera. Due to the lack of genome information, delineation from the genus Algisphaera can only be performed based on the 16S rRNA gene comparison. However, as the observed 16S rRNA similarity of 88.0% is closer to the family threshold (86.5%) than to the genus threshold (94.5%; Yarza et al., 2014), it appears highly unlikely that strain KS4T is a member of the genus Algisphaera.
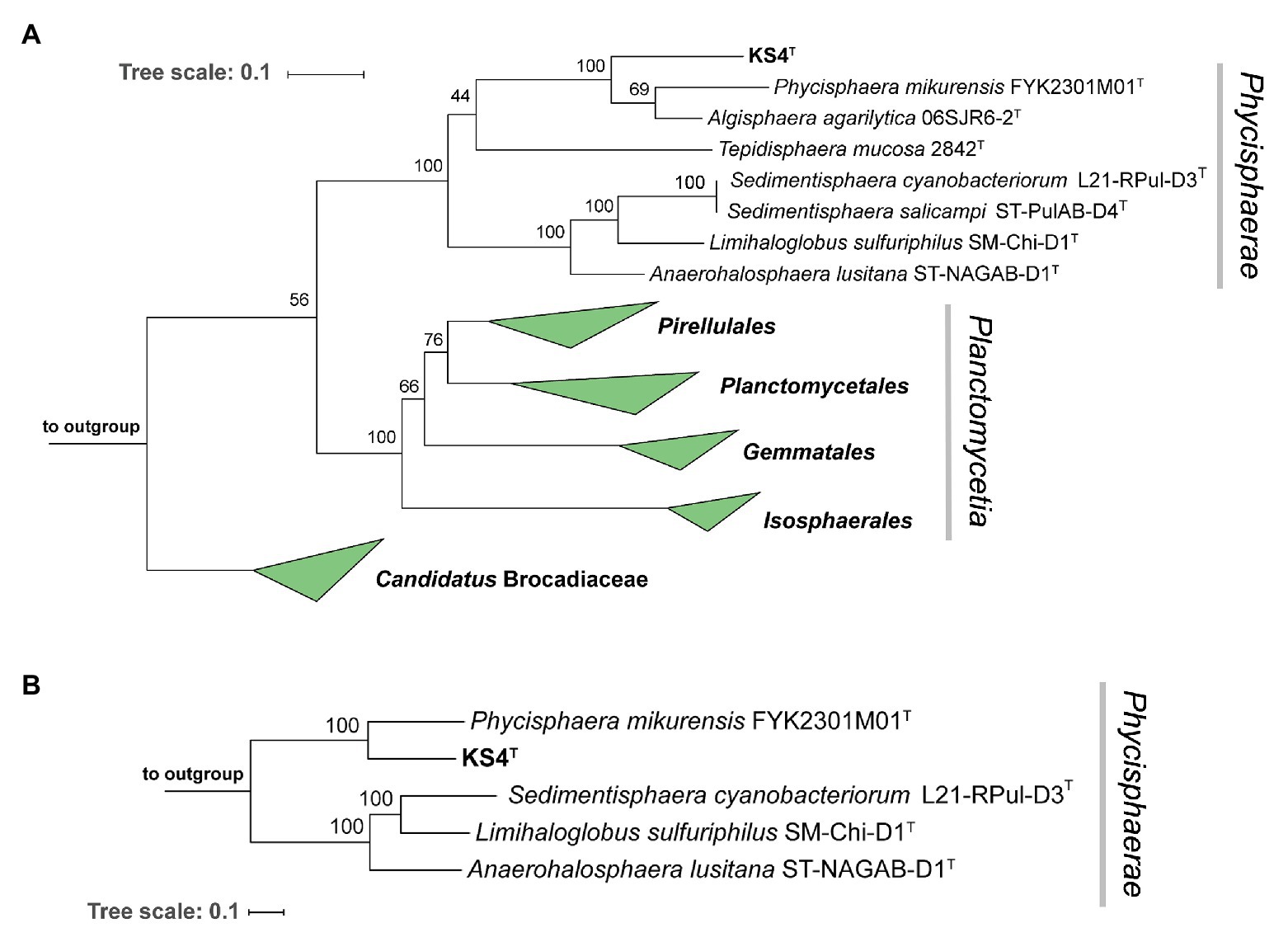
Figure 3. Maximum likelihood phylogenetic analysis. Phylogenetic trees showing the position of strain KS4T. 16S rRNA gene- (A) and multi-locus sequence analysis (MLSA)-based phylogenetic analysis (B) was performed as described in the Material and Methods section. Bootstrap values after 1,000 re-samplings (16S rRNA gene) or 500 re-samplings (MLSA) are given at the nodes (in %). The strains used as outgroup are provided in the Material and Methods section.
Morphological Features of Strain KS4T
For a morphological characterization, cells of strain KS4T harvested during the exponential growth phase were subjected to light and electron microscopy. KS4T cells are rather small and spherical (average diameter of 0.6 ± 0.2 μm) and grow in larger aggregates (Figures 4A–C). Cells are motile and form white colonies on solid medium. The cell surface is rough and typically covered with conspicuous outer membrane vesicles, which were not described for closely related species (Fukunaga et al., 2009; Yoon et al., 2014; Figures 4D,E). Typical characteristics of many planctomycetes, such as the crateriform structures, the stalk structure and the holdfast structure, were not observed for strain KS4T. This, however, is in line with its phylogenetic position since known members of the class Phycisphaerae also lack a stalk or holdfast structure (Fukunaga et al., 2009; Kovaleva et al., 2015; Wiegand et al., 2018). Light and electron microscopic images indicate that strain KS4T divides by budding (Figures 4A,B,E). At the final stage of cell division, in which the spherical mother and daughter cells have reached the same size, we were not able to differentiate between budding and binary fission. However, at the initial stage of cell division, large differences in the cell sizes of the mother and daughter cells are clearly observable during light microscopy (Figure 4B), which is a clear hint towards asymmetric division by budding. All known species of the class Planctomycetia divide by budding, however, our observation is surprising, given that strain KS4T is a member of the class Phycisphaerae, in which all characterized species divide by binary fission (Fukunaga et al., 2009; Yoon et al., 2014; Kovaleva et al., 2015; Pradel et al., 2019). Division by budding can thus be regarded as an uncommon feature in the class Phycisphaerae requiring further attention. This, however, exceeds the scope of this study, since we cannot exclude that strain KS4T might even be capable to switch between both modes of cell division as recently shown in the proposed phylum Saltatorellota (Wiegand et al., 2019). The spherical cell shape and suspected mode of division by budding resembles members of the orders Isosphaerales or Gemmatales, while phylogenetically strain KS4T is clearly a member of the class Phycisphaerae. Such findings might indicate that the mode of cell division is not necessarily a conserved feature in different classes within the phylum Planctomycetes. This is not entirely unlikely as the class Phycisphaerae is quite heterogeneous (see physiological and genomic comparison of strain KS4T in the following section and Table 1). At this stage, it should also be kept in mind that the current closest relative of strain KS4T has a 16S rRNA gene sequence similarity of only 88.7%, which is close to the family threshold of 86.5%.
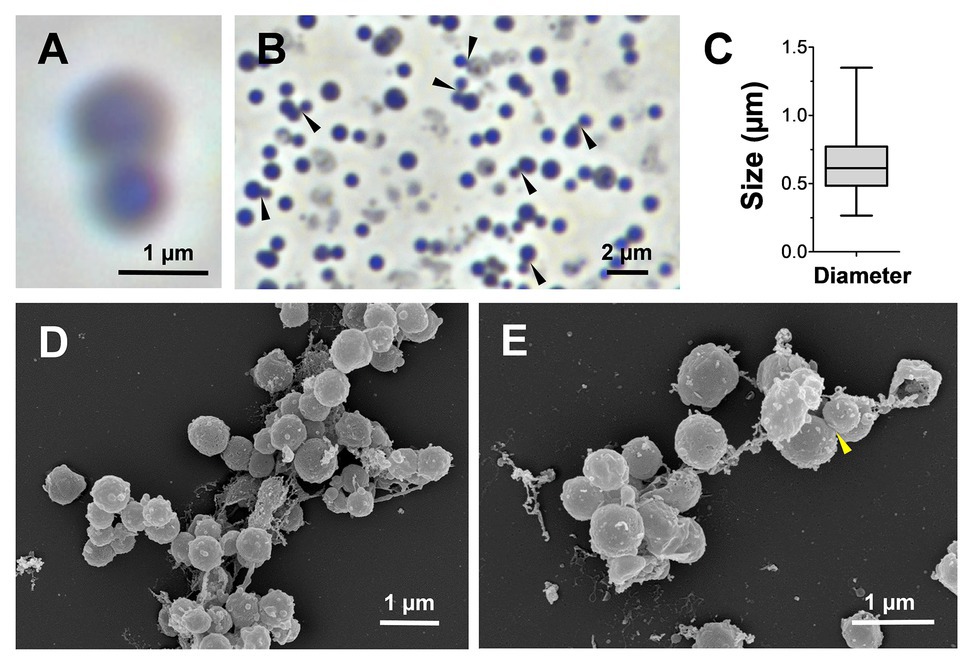
Figure 4. Microscopy images and cell size plot of strain KS4T. Phase contrast (A,B) and electron microscopic images (D,E) of strain KS4T are shown. For determination of the cell size (C), at least 100 representative cells were counted manually or by using a semi-automated object count tool. Cells, for which we observed budding as potential mode of cell division, are indicated by black or yellow arrows (B and E, respectively).
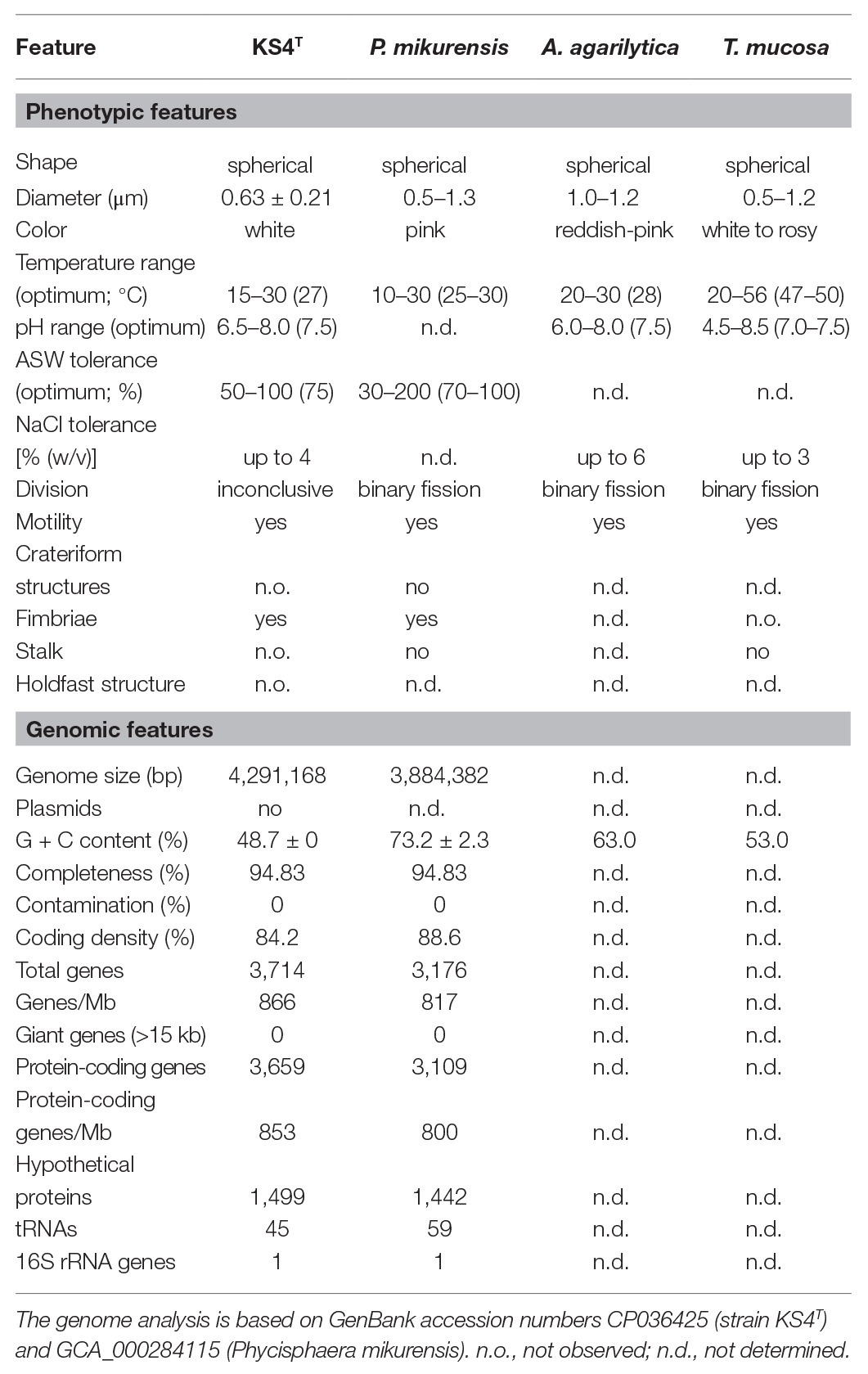
Table 1. Phenotypic and genotypic features of strain KS4T compared to closely related strains in the class Phycisphaerae.
Analysis of Phenotypic Characteristics of Strain KS4T
The physiology of strain KS4T was analyzed during laboratory-scale cultivations in M1H NAG ASW medium, which was also used for the initial cultivation of the strain after isolation. Growth of strain KS4T turned out to be planktonic during cultivation at the optimal temperature of 27°C, which allowed measurement of cell densities as the optical density at 600 nm (OD600). However, at temperatures below or above the optimal temperature, strain KS4T formed larger aggregates in form of white flakes, which settled down in the cultivation tube (Figure 5A). In such cases, resuspension of cells and OD600 measurement was not possible. For determination of the temperature optimum, the tubes were thus inspected visually. All other experiments were conducted at 27°C, which allowed to measure cell densities and to calculate maximal growth rates. Strain KS4T is able to grow over a temperature range of 15–30°C (optimum 27°C) and a pH range of 6.5–8.0 (optimum 7.5; Figures 5A,B). The maximal growth rate was calculated to be 0.029 h−1, which corresponds to a generation time of 24 h. Artificial seawater concentrations of 50–100% allowed for growth, with the highest growth rate observed at a concentration of 75% (Figure 5C). The strain tolerates a maximal NaCl concentration of 4% (w/v), but showed optimal growth in the absence of NaCl (Figure 5D). Strain KS4T is heterotrophic, aerobic, mesophilic, and neutrophilic. Physiological features observed for different species in the class Phycisphaerae are quite heterogeneous. Strain KS4T and the type strains of P. mikurensis, A. agarilytica, and Tepidisphaera mucosa cover a similar pH range and grow optimally at pH 7.5 (Table 1; Fukunaga et al., 2009; Yoon et al., 2014; Kovaleva et al., 2015). The first three strains have a similar temperature preference (25–28°C), while T. mucosa is thermophilic (growth optimum 47–50°C). Salt tolerance varies between 3 and 6% (w/v) NaCl and colony colors cover the entire range from white (unpigmented) to reddish-pink (Table 1).
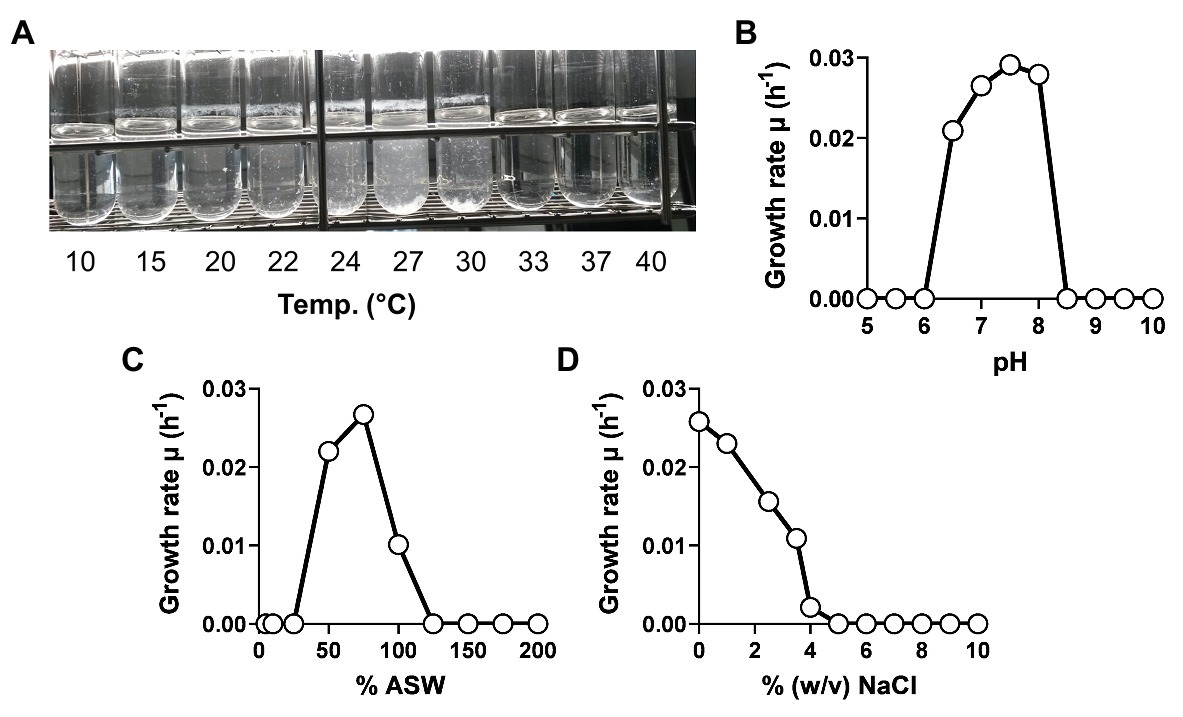
Figure 5. Physiological characterization of strain KS4T. Growth of strain KS4T under different cultivation conditions was tested. Temperature (A), pH (B), artificial seawater (C), and NaCl concentration (D) ranges enabling growth were analyzed by cultivation of the strain in M1H NAG ASW medium in biological triplicates. Due to the aggregation of cells at temperatures above and below the temperature optimum, no growth rates could be calculated for this experiment. The temperature optimum was determined by visual inspection of the cultivation tubes.
Analysis of Genome-Encoded Features of Strain KS4T
The comparison of genomic characteristics focused on P. mikurensis as the genomes of A. agarilytica and T. mucosa have not been sequenced. The genomes of P. mikurensis and strain KS4T are similar in size (3.9–4.3 Mb) and also show similar numbers of protein-coding genes and coding densities (Table 1). Both genomes harbor a single copy of the 16S rRNA gene. The two genomes are clearly distinguishable by a different G + C content. Strain KS4T has a moderate G + C content of 49%, whereas P. mikurensis features 73%, which is among the highest G + C contents found in bacteria (Hildebrand et al., 2010). We also analyzed the numbers of carbohydrate-active enzymes and genes/gene clusters putatively involved in secondary metabolite production based on the genomes of strain KS4T and P. mikurensis NBRC102666T (Table 2). A total number of 140–180 carbohydrate-active enzymes was found to be encoded in the genome of the two strains. The numbers do not reflect the genome size since strain KS4T has a slightly larger genome, but 36 carbohydrate-enzymes less than P. mikurensis. About 43–45% of the enzymes fall within the family of glycosyl hydrolases and a similar number within the family of glycosyl transferases. The residual 10% are distributed among polysaccharide lyases, carbohydrate esterases, carbohydrate-binding module proteins and auxiliary proteins.
The number of secondary metabolism-related gene clusters typically correlates with the genome size. Not surprisingly, given the relatively small genomes of around 4 Mb compared to most of the other known members of the phylum Planctomycetes, the numbers of predicted gene clusters involved in secondary metabolite production are rather small. One to two identified clusters are related to terpenoid production. These are probably involved in carotenoid production in pigmented P. mikurensis. Since strain KS4T is non-pigmented, the cluster may also be relevant for the synthesis of other terpenoids, e.g., hopanoids, as indicated by the presence of a squalene-hopene cyclase-encoding gene (locus KS4_02460) in strain KS4T. Except for a single putative type III polyketide synthase-encoding gene present in P. mikurensis, but absent in strain KS4T, both appear to lack PKSs and non-ribosomal peptide synthetases. Given that planctomycetes with larger genomes harbor up to 13 gene clusters associated with secondary metabolite production (Wiegand et al., 2020), such strains appear to be a more promising basis for discovery of bioactive molecules than the two here analyzed strains of the family Phycisphaeraceae.
Taken together, our analysis of physiological and genomic features of strain KS4T supports the results of the phylogenetic inference, which together delineate the novel strain from known species and genera within the class Phycisphaerae. We thus conclude that strain KS4T represents a novel species of a novel genus, for which we propose the name Poriferisphaera corsica gen. nov., sp. nov.
Genus and Species Description
Description of Poriferisphaera Gen. Nov.
Po.ri.fe.ri.sphae’ra. N.L. neut. pl. n. Porifera name of a phylum (sponges); L. fem. n. sphaera a ball, sphere; N.L. fem. n. Poriferisphaera a spherical bacterium isolated from Porifera.
Members of the genus form spherical and motile cells. Cells are aerobic heterotrophs with a mesophilic and neutrophilic growth profile and lack crateriform structures, stalk structure, and holdfast structure. The G + C content of the genome is around 49%. The genus belongs to the family Phycisphaeraceae, order Phycisphaerales, class Phycisphaerae, phylum Planctomycetes. The type species of this genus is Poriferisphaera corsica.
Description of Poriferisphaera corsica Sp. Nov.
cor’si.ca. L. fem. adj. corsica corsican; corresponding to the origin of the strain from the Mediterranean island Corsica.
In addition to the genus characteristics, cells have an average diameter of 0.63 ± 0.21 μm and form aggregates. The cell surface is covered with outer membrane vesicles. Cells are aerobic and show asymmetric cell division. Cells of the type strain grow over a range of 15–30°C (optimum 27°C), pH 6.5–8.0 (optimum 7.5) and up to NaCl concentrations of 4% (w/v). Optimal growth was observed at 75% ASW, while amounts higher than in standard M1H NAG ASW (>100% ASW) or below 50% abolished growth. Colonies are white.
The type strain is KS4T (=DSM 103958T = LMG 29824T), isolated from the calcareous sponge C. clathrus close to the island Corsica, France, in September 2015. The genome size of the type strain is 4.29 Mb with a DNA G + C content of 48.7%.
Data Availability Statement
The datasets presented in this study can be found in online repositories. The names of the repository/repositories and accession number(s) can be found at: https://www.ncbi.nlm.nih.gov/genbank/, CP036425, https://www.ncbi.nlm.nih.gov/genbank/, MK559978.
Author Contributions
NK analyzed the cultivation data and wrote the article. SW and MJo performed the genomic and phylogenetic analyses. TK performed the amplicon sequencing. CB performed the light microscopic analysis together with TK. R-WM and FB led the sampling expedition and performed initial experiments in Corsica. OJ, PR, R-WM, and FB contributed to data analysis and preparation of the figures. AH and PR isolated the strain and performed the strain cultivation and deposition. MJe contributed to text preparation and revised the article. MR performed the electron microscopic analysis. CJ was involved in sampling and supervised the study. All authors contributed to the article and approved the submitted version.
Funding
This work was supported by the Deutsche Forschungsgemein-schaft (DFG) grants KA 4967/1-1 and JO 893/4-1 and the Radboud Excellence fellowship.
Conflict of Interest
The authors declare that the research was conducted in the absence of any commercial or financial relationships that could be construed as a potential conflict of interest.
Acknowledgments
We thank Ina Schleicher for skillful technical assistance. We thank Brian Tindall and Regine Fähnrich from the DSMZ, as well as the staff from the BCCM/LMG Bacteria collection in Belgium, for their support during strain deposition. We also thank the staff of the STARESO (Station de Recherche Océanographiques et sous-marines) on Corsica for sampling support.
References
Bengtsson, M. M., and Øvreås, L. (2010). Planctomycetes dominate biofilms on surfaces of the kelp Laminaria hyperborea. BMC Microbiol. 10:261. doi: 10.1186/1471-2180-10-261
Blin, K., Wolf, T., Chevrette, M. G., Lu, X., Schwalen, C. J., Kautsar, S. A., et al. (2017). antiSMASH 4.0—improvements in chemistry prediction and gene cluster boundary identification. Nucleic Acids Res. 45, W36–W41. doi: 10.1093/nar/gkx319
Boedeker, C., Schuler, M., Reintjes, G., Jeske, O., van Teeseling, M. C., Jogler, M., et al. (2017). Determining the bacterial cell biology of Planctomycetes. Nat. Commun. 8:14853. doi: 10.1038/ncomms14853
Boersma, A. S., Kallscheuer, N., Wiegand, S., Rast, P., Peeters, S. H., Mesman, R. J., et al. (2019). Alienimonas californiensis gen. nov. sp. nov., a novel Planctomycete isolated from the kelp forest in Monterey Bay. Antonie Van Leeuwenhoek. 113, 1751–1766. doi: 10.1007/s10482-019-01367-4
Burton, M. (1963). A revision of the classification of the calcareous sponges: With a catalogue of the specimes in the British museum (natural history). London: Printed by order of the trustees of the British Museum (Natural History).
Castresana, J. (2000). Selection of conserved blocks from multiple alignments for their use in phylogenetic analysis. Mol. Biol. Evol. 17, 540–552. doi: 10.1093/oxfordjournals.molbev.a026334
Dedysh, S. N., Kulichevskaya, I. S., Beletsky, A. V., Ivanova, A. A., Rijpstra, W. I. C., Damsté, J. S. S., et al. (2020). Lacipirellula parvula gen. nov., sp. nov., representing a lineage of Planctomycetes widespread in low-oxygen habitats, description of the family Lacipirellulaceae fam. nov. and proposal of the orders Pirellulales ord. nov., Gemmatales ord. nov. and Isosphaerales ord. nov. Syst. Appl. Microbiol 43:126050. doi: 10.1016/j.syapm.2019.126050
Edgar, R. C. (2004). MUSCLE: multiple sequence alignment with high accuracy and high throughput. Nucleic Acids Res. 32, 1792–1797. doi: 10.1093/nar/gkh340
Foster, M. S., and Schiel, D. R. (1985). The ecology of giant kelp forests in California: A community profile. Washington, D.C.: US Fish and Wildlife Service.
Frank, O., Michael, V., Päuker, O., Boedeker, C., Jogler, C., Rohde, M., et al. (2015). Plasmid curing and the loss of grip–the 65-kb replicon of Phaeobacter inhibens DSM 17395 is required for biofilm formation, motility and the colonization of marine algae. Syst. Appl. Microbiol. 38, 120–127. doi: 10.1016/j.syapm.2014.12.001
Fukunaga, Y., Kurahashi, M., Sakiyama, Y., Ohuchi, M., Yokota, A., and Harayama, S. (2009). Phycisphaera mikurensis gen. nov., sp. nov., isolated from a marine alga, and proposal of Phycisphaeraceae fam. nov., Phycisphaerales ord. nov. and Phycisphaerae classis nov. in the phylum Planctomycetes. J. Gen. Appl. Microbiol. 55, 267–275. doi: 10.2323/jgam.55.267
Gade, D., Schlesner, H., Glöckner, F., Amann, R., Pfeiffer, S., and Thomm, M. (2004). Identification of Planctomycetes with order-, genus-, and strain-specific 16S rRNA-targeted probes. Microb. Ecol. 47, 243–251. doi: 10.1007/s00248-003-1016-9
Godinho, O., Calisto, R., Øvreås, L., Quinteira, S., and Lage, O. M. (2019). Antibiotic susceptibility of marine Planctomycetes. Antonie Van Leeuwenhoek 112, 1273–1280. doi: 10.1007/s10482-019-01259-7
Häder, D. -P., Kumar, H., Smith, R., and Worrest, R. (1998). Effects on aquatic ecosystems. J. Photochem. Photobiol. B Biol. 46, 53–68. doi: 10.1016/S1011-1344(98)00185-7
Hildebrand, F., Meyer, A., and Eyre-Walker, A. (2010). Evidence of selection upon genomic GC-content in bacteria. PLoS Genet. 6:e1001107. doi: 10.1371/journal.pgen.1001107
Hooper, J. N., and van Soest, R. W. (2002). Systema Porifera: A guide to the classification of sponges. New York: Springer Science+Business Media.
Izumi, H., Sagulenko, E., Webb, R. I., and Fuerst, J. A. (2013). Isolation and diversity of Planctomycetes from the sponge Niphates sp., seawater, and sediment of Moreton Bay, Australia. Antonie Van Leeuwenhoek 104, 533–546. doi: 10.1007/s10482-013-0003-5
Jeske, O., Jogler, M., Petersen, J., Sikorski, J., and Jogler, C. (2013). From genome mining to phenotypic microarrays: Planctomycetes as source for novel bioactive molecules. Antonie Van Leeuwenhoek 104, 551–567. doi: 10.1007/s10482-013-0007-1
Jogler, M., and Jogler, C. (2013). “Toward the development of genetic tools for Planctomycetes” in Planctomycetes: Cell structure, origins and biology, fuerst. ed.A. John (New York: Springer Science+Business Media), 141–164.
Kallscheuer, N., Jogler, M., Wiegand, S., Peeters, S. H., Heuer, A., Boedeker, C., et al. (2019a). Rubinisphaera italica sp. nov. isolated from a hydrothermal area in the Tyrrhenian Sea close to the volcanic island Panarea. Antonie Van Leeuwenhoek. 113, 1727–1736. doi: 10.1007/s10482-019-01329-w
Kallscheuer, N., Wiegand, S., Heuer, A., Rensink, S., Boersma, A. S., Jogler, M., et al. (2020). Blastopirellula retiformator sp. nov. isolated from the shallow-sea hydrothermal vent system close to Panarea Island. Antonie Van Leeuwenhoek. 113, 1811–1822. doi: 10.1007/s10482-019-01377-2
Kallscheuer, N., Wiegand, S., Peeters, S. H., Jogler, M., Boedeker, C., Heuer, A., et al. (2019c). Description of three bacterial strains belonging to the new genus Novipirellula gen. nov., reclassificiation of Rhodopirellula rosea and Rhodopirellula caenicola and readjustment of the genus threshold of the phylogenetic marker rpoB for Planctomycetaceae. Antonie Van Leeuwenhoek. 113, 1779–1795. doi: 10.1007/s10482-019-01374-5
Kim, M., Oh, H. -S., Park, S. -C., and Chun, J. (2014). Towards a taxonomic coherence between average nucleotide identity and 16S rRNA gene sequence similarity for species demarcation of prokaryotes. Int. J. Syst. Evol. Microbiol. 64, 346–351. doi: 10.1099/ijs.0.059774-0
Kohn, T., Heuer, A., Jogler, M., Vollmers, J., Boedeker, C., Bunk, B., et al. (2016). Fuerstia marisgermanicae gen. nov., sp. nov., an unusual member of the phylum Planctomycetes from the German Wadden Sea. Front. Microbiol. 7:2079. doi: 10.3389/fmicb.2016.02079
Kohn, T., Rast, P., Wiegand, S., Jetten, M., Kallscheuer, N., Jeske, O., et al. (2020a). The microbiome of Posidonia oceanica seagrass leaves can be dominated by Planctomycetes. Front. Microbiol. 11:1458. doi: 10.3389/fmicb.2020.01458
Kohn, T., Wiegand, S., Boedeker, C., Rast, P., Heuer, A., Schüler, M., et al. (2020b). Planctopirus ephydatiae, a novel Planctomycete species isolated from a freshwater sponge. Syst. Appl. Microbiol. 43:126022. doi: 10.1016/j.syapm.2019.126022
Konstantinidis, K. T., and Tiedje, J. M. (2005). Towards a genome-based taxonomy for prokaryotes. J. Bacteriol. 187, 6258–6264. doi: 10.1128/JB.187.18.6258-6264.2005
Kovaleva, O., Merkel, A. Y., Novikov, A., Baslerov, R., Toshchakov, S., and Bonch-Osmolovskaya, E. (2015). Tepidisphaera mucosa gen. nov., sp. nov., a moderately thermophilic member of the class Phycisphaerae in the phylum Planctomycetes, and proposal of a new family, Tepidisphaeraceae fam. nov., and a new order, Tepidisphaerales ord. nov. Int. J. Syst. Evol. Microbiol. 65, 549–555. doi: 10.1099/ijs.0.070151-0
Lage, O. M., and Bondoso, J. (2014). Planctomycetes and macroalgae, a striking association. Front. Microbiol. 5:267. doi: 10.3389/fmicb.2014.00267
Lechner, M., Findeiss, S., Steiner, L., Marz, M., Stadler, P. F., and Prohaska, S. J. (2011). Proteinortho: detection of (co-)orthologs in large-scale analysis. BMC Bioinform. 12:124. doi: 10.1186/1471-2105-12-124
Lee, I., Ouk Kim, Y., Park, S. C., and Chun, J. (2016). OrthoANI: an improved algorithm and software for calculating average nucleotide identity. Int. J. Syst. Evol. Microbiol. 66, 1100–1103. doi: 10.1099/ijsem.0.000760
Muscholl-Silberhorn, A., Thiel, V., and Imhoff, J. F. (2008). Abundance and bioactivity of cultured sponge-associated bacteria from the Mediterranean Sea. Microb. Ecol. 55, 94–106. doi: 10.1007/s00248-007-9255-9
Neulinger, S. C., Stöhr, R., Thiel, V., Schmaljohann, R., and Imhoff, J. F. (2010). New phylogenetic lineages of the spirochaetes phylum associated with Clathrina species (Porifera). J. Microbiol. 48, 411–418. doi: 10.1007/s12275-010-0017-x
Peeters, S. H., Wiegand, S., Kallscheuer, N., Jogler, M., Heuer, A., Jetten, M. S., et al. (2020). Three marine strains constitute the novel genus and species Crateriforma conspicua in the phylum Planctomycetes. Antonie Van Leeuwenhoek. 113, 1797–1809. doi: 10.1007/s10482-019-01375-4
Pimentel-Elardo, S., Wehrl, M., Friedrich, A. B., Jensen, P. R., and Hentschel, U. (2003). Isolation of Planctomycetes from Aplysina sponges. Aquat. Microb. Ecol. 33, 239–245. doi: 10.3354/ame033239
Pradel, N., Fardeau, M. -L., Tindall, B. J., and Spring, S. (2019). Anaerohalosphaera lusitana gen. nov., sp. nov., and Limihaloglobus sulfuriphilus gen. nov., sp. nov., isolated from solar saltern sediments, and proposal of Anaerohalosphaeraceae fam. nov. within the order Sedimentisphaerales. Int. J. Syst. Evol. Microbiol. 7, 1321–1330. doi: 10.1099/ijsem.0.003919
Pruesse, E., Peplies, J., and Glöckner, F. O. (2012). SINA: accurate high-throughput multiple sequence alignment of ribosomal RNA genes. Bioinformatics 28, 1823–1829. doi: 10.1093/bioinformatics/bts252
Qin, Q. -L., Xie, B. -B., Zhang, X. -Y., Chen, X. -L., Zhou, B. -C., Zhou, J., et al. (2014). A proposed genus boundary for the prokaryotes based on genomic insights. J. Bacteriol. 196, 2210–2215. doi: 10.1128/JB.01688-14
Quast, C., Pruesse, E., Yilmaz, P., Gerken, J., Schweer, T., Yarza, P., et al. (2012). The SILVA ribosomal RNA gene database project: improved data processing and web-based tools. Nucleic Acids Res. 41, D590–D596. doi: 10.1093/nar/gks1219
Quévrain, E., Roué, M., Domart-Coulon, I., and Bourguet-Kondracki, M.-L. (2014). Assessing the potential bacterial origin of the chemical diversity in calcareous sponges. J. Mar. Sci. Technol. 22, 36–49. doi: 10.6119/JMST-013-0718-2
Rodriguez-R, L. M., and Konstantinidis, K. T. (2016). The enveomics collection: a toolbox for specialized analyses of microbial genomes and metagenomes. PeerJ Preprints 4:e1900v1. doi: 10.7287/peerj.preprints.1900v1
Roué, M., Quévrain, E., Domart-Coulon, I., and Bourguet-Kondracki, M. -L. (2012). Assessing calcareous sponges and their associated bacteria for the discovery of new bioactive natural products. Nat. Prod. Rep. 29, 739–751. doi: 10.1039/c2np20040f
Ruys, A. J. (2013). Biomimetic biomaterials: Structure and applications. Cambridge, UK: Woodhead Publishing.
Stackebrandt, E., and Ebers, J. (2006). Taxonomic parameters revisited: tarnished gold standards. Microbiol. Today 8, 6–9.
Stamatakis, A. (2014). RAxML version 8: a tool for phylogenetic analysis and post-analysis of large phylogenies. Bioinformatics 30, 1312–1313. doi: 10.1093/bioinformatics/btu033
Terrapon, N., Lombard, V., Drula, E., Coutinho, P. M., and Henrissat, B. (2017). “The CAZy database/the carbohydrate-active enzyme (CAZy) database: principles and usage guidelines” in A practical guide to using glycomics databases. ed. K. F. Aoki-Kinoshita (Tokyo: Springer, Japan), 117–131.
Wiegand, S., Jogler, M., Boedeker, C., Pinto, D., Vollmers, J., Rivas-Marín, E., et al. (2020). Cultivation and functional characterization of 79 Planctomycetes uncovers their unique biology. Nat. Microbiol. 5, 126–140. doi: 10.1038/s41564-019-0588-1
Wiegand, S., Jogler, M., and Jogler, C. (2018). On the maverick Planctomycetes. FEMS Microbiol. Rev. 42, 739–760. doi: 10.1093/femsre/fuy029
Wiegand, S., Jogler, M., Kohn, T., Awal, R. P., Oberbeckmann, S., Kesy, K., et al. (2019). The novel shapeshifting bacterial phylum Saltatorellota. bioRxiv 817700. [Preprint]. doi: 10.1101/817700
Yarza, P., Yilmaz, P., Pruesse, E., Glöckner, F. O., Ludwig, W., Schleifer, K. H., et al. (2014). Uniting the classification of cultured and uncultured bacteria and archaea using 16S rRNA gene sequences. Nat. Rev. Microbiol. 12, 635–645. doi: 10.1038/nrmicro3330
Yoon, J., Jang, J. -H., and Kasai, H. (2014). Algisphaera agarilytica gen. nov., sp. nov., a novel representative of the class Phycisphaerae within the phylum Planctomycetes isolated from a marine alga. Antonie Van Leeuwenhoek 105, 317–324. doi: 10.1007/s10482-013-0076-1
Keywords: marine bacteria, sponge, 16S rRNA gene, planctomycetes, Mediterranean Sea, bacterial community
Citation: Kallscheuer N, Wiegand S, Kohn T, Boedeker C, Jeske O, Rast P, Müller R-W, Brümmer F, Heuer A, Jetten MSM, Rohde M, Jogler M and Jogler C (2020) Cultivation-Independent Analysis of the Bacterial Community Associated With the Calcareous Sponge Clathrina clathrus and Isolation of Poriferisphaera corsica Gen. Nov., Sp. Nov., Belonging to the Barely Studied Class Phycisphaerae in the Phylum Planctomycetes. Front. Microbiol. 11:602250. doi: 10.3389/fmicb.2020.602250
Edited by:
Marcelino T. Suzuki, Sorbonne Universités, FranceReviewed by:
Detmer Sipkema, Wageningen University and Research, NetherlandsJoana Bondoso, Independent Researcher, Toulouse, France
Copyright © 2020 Kallscheuer, Wiegand, Kohn, Boedeker, Jeske, Rast, Müller, Brümmer, Heuer, Jetten, Rohde, Jogler and Jogler. This is an open-access article distributed under the terms of the Creative Commons Attribution License (CC BY). The use, distribution or reproduction in other forums is permitted, provided the original author(s) and the copyright owner(s) are credited and that the original publication in this journal is cited, in accordance with accepted academic practice. No use, distribution or reproduction is permitted which does not comply with these terms.
*Correspondence: Christian Jogler, Y2hyaXN0aWFuLmpvZ2xlckB1bmktamVuYS5kZQ==