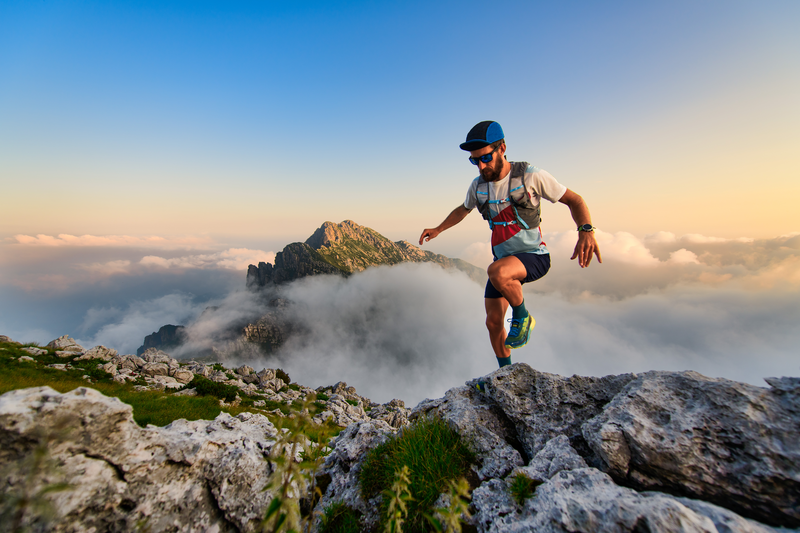
94% of researchers rate our articles as excellent or good
Learn more about the work of our research integrity team to safeguard the quality of each article we publish.
Find out more
ORIGINAL RESEARCH article
Front. Microbiol. , 17 November 2020
Sec. Antimicrobials, Resistance and Chemotherapy
Volume 11 - 2020 | https://doi.org/10.3389/fmicb.2020.601317
This article is part of the Research Topic Antimicrobials in Wildlife and the Environment View all 12 articles
Wildlife has recently been pinpointed as one of the drivers of dissemination of genes conferring resistances to clinically important antimicrobials. The presence of both extended-spectrum beta-lactamase- (ESBL) and carbapenemase-encoding genes has notably been reported in wild birds, that can act as sentinels of antimicrobial resistance (AMR) contamination but also as long-distance spreaders in case of migratory birds. Here, 424 wild birds brought to a rescue center in France were sampled over a 6-month period. These birds encompassed 62 different sedentary or migratory species. A further 16 wild mammals present in the center were also investigated. No carbapenemase-producer was found, but we identified a surprisingly high proportion (24.1%) of ESBL-positive isolates. A total of 144 non-duplicate isolates were collected, including Escherichia coli (n = 88), Enterobacter cloacae (n = 51), and Citrobacter freundii (n = 5), of which 123 carried the blaCTX–M–9 gene. PFGE, phylogroup, and MLST revealed the presence of a limited number of ESBL-positive clones circulating in these animals, all presenting multiple associated resistances. Next-generation sequencing on a subset of isolates, followed by Southern blot hybridization, showed the wide dissemination of an IncHI2/ST1 plasmid carrying the blaCTX–M–9, blaSHV–12 and mcr-9 genes. In all, our results undoubtedly reflect cross transmissions of ESC-resistance (ESC-R) Enterobacteriaceae within the rescue center – similarly to nosocomial spreads observed at hospital, rather than the true bacterial flora of birds. We also showed that the spread of ESC-R in this rescue center did not only rely on clonal but also on a highly successful plasmidic transmission. Since most animals are intended to get back to nature after a few days or weeks, this is obviously an issue with regard to ESBL dissemination in natural environments.
The importance of wildlife in disseminating genes conferring resistances to clinically important antimicrobials (CIAs), such as to extended-spectrum cephalosporins (ESC) or carbapenems (CP), has been recurrently highlighted (Wang et al., 2017; Dolejska and Literak, 2019). Antimicrobial resistance (AMR) in wildlife most likely reflects the pollution of natural environments with AMR genes, plasmids or bacterial clones selected in non-wildlife sectors. AMR acquisition most probably occurs through food opportunities – including dejections from domestic animals or polluted lands – and water sources, but the exact origin of AMR in specific wild individuals is usually unknown (Guenther et al., 2011; Mukerji et al., 2020). In most cases, AMR in wildlife has been found incidentally and, to date, most publications refer to wild birds, which encompass a wide diversity of animal species, habits and behaviors.
In wild birds, major Escherichia coli clones circulating in humans were detected, such as of sequence type (ST)131, ST410, ST648, or ST38 to only name a few, suggesting that these animals were indirectly contaminated by human sources (Schaufler et al., 2016; Atterby et al., 2017; Guenther et al., 2017; Yang et al., 2019). In rare occasions, AMR epidemiology in wild birds more clearly mirrored the one in humans and/or domestic animals in the same country, thereby supporting the hypothesis of local cross-sectorial transmissions. It was notably exemplified for CTX-M-producing Enterobacteriaceae in Sweden and Canada (Bonnedahl et al., 2015; Atterby et al., 2017) or IMP-4-producing Enterobacteriaceae in Australia (Dolejska et al., 2016). In addition to being sentinels of AMR contamination from other sectors, migratory birds can also spread AMR genes or antimicrobial resistant bacteria over very long distances, whose impacts on public health are suspected but not fully clarified (Guenther et al., 2012; Fuentes-Castillo et al., 2019).
At a global scale, CP- or colistin resistance in wild birds has been much less reported than ESC-resistance (ESC-R). It may however reflect a lack of studies, or the accumulation of case reports that do not accurately reflect the true epidemiology. Indeed, investigations on AMR in wild birds sometimes revealed unexpected reservoirs, such as recently where a high proportion of CP-resistant NDM-5-producing Klebsiella pneumoniae was observed in migratory birds in China (Liao et al., 2019). With regard to colistin resistance, a limited number of studies reported the plasmid-mediated mcr-1 gene in wild birds (Tarabai et al., 2019). In France, ESBL-producing Enterobacteriaceae were detected in 2009 in 17/90 (18.9%) of juvenile yellow-legged gulls (Bonnedahl et al., 2009), while the sporadic detection of a VIM-1-producing E. coli was reported in 2012 in the same area (South of France) and the same bird species (Vittecoq et al., 2017). Recently, multi-drug resistant Enterobacteriaceae were again found in yellow-legged gulls in Marseille, France (Ngaiganam et al., 2019).
In all, several works investigating AMR in wild birds resulted from convenient samples from dead animals. Numerous studies were also based on fecal dejections collected in various places where birds live or transit, such as landfills, beaches, urban parks, nests, and other habitats, but associating fecal samples to the right bird species may be challenging. In some situations however, these approaches valuably allowed studying AMR in large cohorts of individuals of the same bird species in their natural environment, as for instance illustrated for black kites (Milvus milvus), corvids (Corvus brachyrhynchos, Corvus corax), gulls (Larus glaucescens, Larus ridibundus), pigeons (Columba livia) or storks (Ciconia ciconia) (Bonnedahl et al., 2010, 2014; Jamborova et al., 2018; Tarabai et al., 2019; Zendri et al., 2020). Here, we adopted a different strategy by systematically sampling all incoming wild birds at a French rescue center over a 6-month period. Such a systematic sampling design, which can for example be set up in rescue centers or during ringing campaigns (Guenther et al., 2010; Schaufler et al., 2016), is still rare in the field of AMR in wild birds. Both sedentary and migratory species were considered and sampled, and AMR genes, plasmids and bacterial clones were further investigated at phenotypic and molecular levels. Wild mammals present in the center were also studied. Our data support interesting hypotheses on the spread of AMR at the interface of wild animals and human communities.
Between April and November 2015, 424 migratory and sedentary birds were sampled at an animal rescue center in the Hérault department, South of France. Cloacal sample was taken using Eswab minitip or pernasal flocked (Labelians, Nemours, France), depending to the size of the bird. Sampling was performed in the first days after arrival at the center, ranging from 24 h to 10 days. During the study, and for epidemiological reasons (see section “Results”), 16 mammals which were temporarily hosted in the rescue center were also sampled using the same procedure as for birds. Within 24 h after sampling, samples were plated on Drigalsky agar as a control of growth, as well as on the selective ChromID ESBL, ChromID OXA-48 and CarbaSMART media for the detection of ESC- and CP resistance. One colony per morphology was picked up and identified by MALDI TOF (VITEK MSVersion 3.0, bioMérieux, Marcy L’Etoile, France).
Susceptibility testing was performed by disc diffusion on Mueller-Hinton agar (Bio-Rad, Marne-la-Coquette, France), according to the guidelines and clinical breakpoints of the Antibiogram Committee of the French Society for Microbiology1. The following discs of human and/or veterinary interest were tested: amoxicillin, amoxicillin + clavulanic acid, cefalotin, cefuroxime, cefotaxime, ceftiofur, ceftazidime, cefoxitin, cefepime, aztreonam, cefquinome, ertapenem, streptomycin, kanamycin, amikacin, apramycin, gentamicin, tobramycin, netilmicin, chloramphenicol, florfenicol, tetracycline, colistin, sulfonamides, trimethoprim, nalidixic acid, and enrofloxacin. E. coli ATCC 25922 was used as a quality control. Minimum inhibitory concentration (MIC) for colistin was determined by the broth microdilution method, as recommended by EUCAST (EUCAST, 2016).
PCRs were performed using specific primers for the detection of blaTEM, blaSHV, blaCTX–M group 1, group 2, and group 9 (Shibata et al., 2006; Dierikx et al., 2010). For all blaCTX–M group 1 and group 9-positive isolates, additional PCRs were performed using the primers ISEcp1L1/P2D and MA1/MA2, respectively. The blaCMY genes were detected using CF1/CF2 primers (Eckert et al., 2004). All positive amplicons were sequenced (Genewiz, London, United Kingdom). The mcr-1 to mcr-5 genes were detected using published primers (Lescat et al., 2018) while detection of the mcr-9 gene was performed using the primers mcr9_int_for (5′-GAAACTAACCCCCAGGAAGC) and mcr9_int_rev (5′-TTTTGGCGATTTCATCATCA).
Phylogenetic grouping of the E. coli isolates was performed using the improved method described by Clermont et al. (2013). PFGE was performed on XbaI-digested DNA. Multi-locus sequence typing (MLST) was performed on one representative of each PFGE profile according to the following websites: https://pubmlst.org/bigsdb?db=pubmlst_mlst_seqdef for E. coli according to the Achtman scheme, and https://pubmlst.org/ecloacae/ for E. cloacae.
Genomic DNA of eight selected isolates (four E. coli and four E. cloacae) was extracted from an overnight culture using the GmbH & Co. KG – NucleoSpin® Microbial DNA (Macherey Nagel, Germany). Whole genome sequencing was performed using the NovaSeq technology (Illumina). The paired-end reads (average read length of 151 bp) were generated with a 69-fold to 131-fold coverage. After Trimmomatic cleaning, de novo assembly was performed using Shovill (version 0.9.0). Resistance genes were searched from the assembled genomes using the ResFinder database (CGE2). Pairwise single nucleotide polymorphism (SNP) distances were calculated from core genome alignments generated by Roary using snp-dists3.
Plasmids were typed by PCR-based replicon typing (PBRT) according to the PBRT kit scheme described by Carattoli et al. (2005) using a commercial kit (Diatheva, Cartoceto, Italy). PFGE-S1 gels were performed, followed by Southern blot using adequate probes (blaCTX–M–9, blaSHV–12, mcr-9, IncHI2) according to the manufacturer’s protocol (Roche Diagnostics, Meylan, Germany). Plasmid co-localization was assessed by comparison between the bands corresponding to the resistance genes and those corresponding to the Inc type of the plasmid.
No ethical approval was needed since this study did not involve any experimentation on animals.
The whole genome shotgun project was deposited in DDBJ/EMBL/GenBank under the BioProject accession number PRJNA659767.
Over the 6-month period, a total of 424 wild birds were sampled, that belonged to 62 different species distributed into 25 sedentary ones and 37 species migrating either inside Europe or to Africa. All of these species were represented by less than 20 individuals, except for black swifts (Apus apus, n = 74), rock pigeon (C. livia, n = 55), Turkish turtledove (Streptopelia decaocto, n = 31), and black-billed magpie (Pica pica, n = 21). The vast majority of the birds were referred to the rescue center after serious injury (various traumas, such as broken legs or wings) or because they had been found incidentally. In some occasions, related juveniles from the same litter were collected at the same time. Altogether, all birds were confirmed to be devoid of obvious bacterial or viral infection after veterinary examination so that any AMR in the positive animals should be considered as carriage.
A total of 102 birds (102/424, 24.1%) were positive for the presence of at least one ESC-R Enterobacteriaceae, while no CP-resistant isolate was identified (Supplementary Table 1). Forty-six positive birds were sedentary (14 different species including ducks, sparrows and gulls) while 56 were migratory (19 different species either migrating in Europe, such as tawny owls or in Africa, such as swallows). No bird species was more represented amongst the positive individuals compared to the negative ones. Different bacterial morphologies were identified on numerous selective plates, but only non-duplicate isolates (based on the PFGE profile, phylogroup, CTX-M-type and the antibiogram) were kept for further analysis. Multiple ESC-R isolates were identified in 36/102 samples (n = 35.3%). For each positive animal, one to four different bacterial morphologies were identified, so that a total of 144 different isolates were collected (Supplementary Table 1). ESC-R Enterobacteriaceae were identified as E. coli (n = 88), Enterobacter cloacae (n = 51), and Citrobacter freundii (n = 5). One ESC-R E. coli and one ESC-R E. cloacae were concomitantly identified in 22/102 samples (n = 19.6%), and the same CTX-M-9 enzyme was found in concomitant E. coli and E. cloacae isolates in 20/22 samples.
The blaCTX–M–1 gene was found in 21 isolates (20 E. coli and 1 E. cloacae), while the blaCTX–M–9 gene was dominantly detected in the 123 remaining isolates, i.e., in 68 E. coli, 50 E. cloacae and five C. freundii isolates (Supplementary Table 1). All isolates presented multiple associated resistances (Table 1), the most frequent ones being to sulfonamides (100% in E. cloacae; 98.9% in E. coli), tetracyclines (78.4 and 85.2%, respectively) and chloramphenicol (90.2 and 72.7%, respectively). E. cloacae isolates were also often resistant to gentamicin (64.7%) and enrofloxacin (25.5%). No resistance to amikacin or colistin was detected.
The vast majority of E. coli isolates belonged to the phylogroups A (n = 32) and B1 (n = 53) usually associated to commensal isolates, while only three belonged to the more virulent B2 (n = 2) and D (n = 1) phylogroups. A total of 22 PFGE profiles and 14 different STs were identified among the E. coli isolates (Supplementary Table 1 and Table 2, also see Supplementary Figures 1, 2). The two PFGE profiles A and B were dominant and represented 63.6% (56/88) of the E. coli isolates. These two PFGE profiles corresponded to ST746 (n = 30) and ST1246 (n = 27), respectively, and both produced CTX-M-9. ST155 (n = 14) was also recurrently found and produced CTX-M-1. This ST was more heterogeneous than ST746 and ST1246 since six different PFGE profiles were identified. Dynamics of these three main E. coli lineages over the 6-month period showed that ST1246 sporadically but regularly occurred between May and July, while ST746 had a more epidemic behavior, with 25 isolates detected between the end of June and mid-July (Supplementary Figure 3). For E. cloacae as well, most isolates distributed into a limited number of STs and PFGE profiles and also presented a peak of occurrence between the end of June and mid-July. Indeed, E. cloacae isolates mainly belonged to the new ST corresponding to a single locus variant of ST714 (n = 16; allelic sequence 2/2/gyrB∗/133/51/2/14), ST135 (n = 11), ST78 (n = 10), and ST104 (n = 9), representing 90% (46/51) of all isolates. Contrary to E. coli, no E. cloacae isolate produced CTX-M-1.
During the 6-month sampling period, a few wild mammals (16) were also healed in the rescue center, using the same facilities as for birds, and that were sampled and analyzed using the same procedures as for birds. Bacterial identification and antimicrobial susceptibility testing proved that eight of these animals carried ESBL-producing E. coli isolates (Table 3 and Supplementary Table 1), including one rabbit (Oryctolagus cuniculus), two hares (Lepus europaeus), one squirrel (Sciurus vulgaris), and four foxes (Vulpes vulpes). Further molecular analysis of ESBL genes together with PFGE and MLST determination concluded that the rabbit, the two hares and one fox carried an ST223 CTX-M-9-producing E. coli. The three other foxes carried an ST155 CTX-M-1-producing E. coli while the squirrel carried an ST1246 CTX-M-9-producing E. coli. All these CTX-M-producing E. coli lineages and corresponding PFGE profiles had also been detected in birds. No E. cloacae was detected in wild mammals.
To further characterize the isolates circulating in the rescue center, four couples of CTX-M-9-producing E. coli/E. cloacae (each couple originating from a single bird) were fully sequenced using Illumina technologies (Table 4). The four bird species were a magpie (P. pica), a jackdaw (Corvus modenula), an eagle owl (Bubo bubo), and a crag martin (Ptyonoprogne rupestris). Except P. rupestris which has a long-distance migratory behavior (Africa), the three other species are considered sedentary. The four E. coli isolates were from the two main lineages (ST746 and ST1246) found in this study, with the B. bubo and P. rupestris harboring a ST746 E. coli, and the P. pica and Corvus modenula harboring a ST1246 E. coli. SNP analysis on the core genome proved that isolates from a same ST were genetically highly similar, which differed by respectively, 8 and 21 SNPs. The same genomic similarities were found among the two E. cloacae of the same ST in the corresponding birds; which differed by respectively 29 and 63 SNPs.
NGS data also revealed additional information on the gene content, which was coherent with the resistance phenotypes observed (Table 4). Moreover, in all eight CTX-M-9-producing E. coli and E. cloacae of the four birds, NGS demonstrated the presence of the IncHI2/ST1 plasmid replicon and the mcr-9 gene. Southern blot experiments on these eight isolates confirmed that blaCTX–M–9 and mcr-9 co-localized on the IncHI2/ST1 plasmid, together with the blaSHV–12 gene when present. The presence of the mcr-9 and blaSHV–12 genes was further assessed by PCR, revealing that blaSHV–12 was present in 99 and mcr-9 in 118 out of the 123 CTX-M-9-producing isolates. Southern blot experiments performed on a representative sub-set of 21 CTX-M-9/SHV-12/MCR-9 positive E. coli and E. cloacae proved that these three genes systematically co-localized on an IncHI2/ST1 plasmid.
In this study, we report a high prevalence of 24.1% of ESC-R in a large collection of 424 wild birds sampled on arrival in an animal rescue center in France over a 6-month period. However, this high prevalence, moreover in a collection encompassing over 50 different bird species, must be cautiously interpreted. Indeed, when considering the delay between entrance and sampling (from 24 h to 10 days) together with the molecular evidence of a limited number of highly prevalent ESC-R clones colonizing the positive animals, this picture most likely reflects cross transmissions of ESC-R within the rescue center, similarly to nosocomial spreads observed at hospital. This interpretation is further reinforced by the evidence of ESBL carriage in several mammals kept in this center with the same bacterial lineages as observed in birds. The presence of ESBL-producing Enterobacteriaceae in wild mammals strongly suggests that they can potentially act as environmental vectors of resistance genes and/or resistant bacteria. This also alerts on the importance of separating animals (here mammals and birds) that can share AMR determinants before being released in nature. In all, the present situation does obviously not reflect the true prevalence of ESC-R in birds in the wild, but reveals a particular bacterial flora specific to the rescue center. A plausible hypothesis would be that some birds have been collected as naturally positive ESBL carriers, and that bird-adapted bacterial clones then colonized the environment of the rescue center – including aqueous solutions, sinks, cages or aviaries as shown in hospitals – thus favoring their persistence and dissemination (Lowe et al., 2012; Chapuis et al., 2016). In particular, E. coli ST746 had already been reported in birds in France in 2009, possibly suggesting specific adaptive properties (Bonnedahl et al., 2009). ST155 has also been reported in wild birds in many occasions (Hernandez et al., 2013; Alcalá et al., 2016).
The high prevalence of ESC-R E. cloacae isolates, often in co-occurrence with ESC-R E. coli, was another interesting feature of this study. Even though E. cloacae alone or as co-contaminant has been sporadically reported in birds (Giacopello et al., 2016), such a high prevalence was an unprecedented situation. It also most probably results from the intra-center spread of a limited number of ESC-R E. cloacae lineages, but this suggests that these lineages may be particularly adapted to the avian hosts. While ST104 and ST135 have only been sporadically reported, ST78 is also considered as a high-risk clone for humans and is a major driver of CP-resistance spread, notably in North America (Izdebski et al., 2015; Annavajhala et al., 2019). Whether this clone had a human source in our study or is also adapted to birds cannot be inferred from our results, and investigations on the microbiota of birds are clearly needed to better understand transmission routes of multi-drug resistant bacteria.
Interestingly, the spread of ESC-R in this rescue center did not only rely on clonal but also on plasmidic transmission. Here, we evidenced the wide dissemination of a single IncHI2/ST1 plasmid bearing mcr-9, blaCTX–M–9, and in most cases blaSHV–12. This plasmid was equally found in ESC-R E. coli and ESC-R E. cloacae, strongly suggesting that plasmid spread within the same bird has occurred as well. Of note, all isolates were phenotypically susceptible to colistin, which is a known feature of mcr-9, a gene that was first described in 2019 in Salmonella enterica from a human patient in the United States and since then has not been shown to confer phenotypic resistance to colistin (Carroll et al., 2019). The study by Chavda et al. (2019) strongly suggested that mcr-9 was associated with large InHI2/IncHI2A plasmid, which was then corroborated by two other publications reporting mcr-9/blaCTX–M–9/blaVIM–4 in E. cloacae from a young patient in the United States and mcr-9/blaSHV–12 in several Enterobacteriaceae from horses in Sweden on IncHI2 plasmids (Borjesson et al., 2019). The occurrence of mcr-9 in both horses and birds may argue for an environmental dissemination and for a large epidemic success of these IncHI2/ST1 plasmids, thanks to plasticity and optimal conjugation properties at low temperatures (around 25°C) (Garcia-Fernandez and Carattoli, 2010). However, since mcr-9 does not confer phenotypic resistance to colistin, its real role remains to be studied.
In terms of CTX-M epidemiology, the over-dominance of blaCTX–M–9 in ESBL-positive animals was unexpected since blaCTX–M–9 is very rare in France, both in domestic animals and humans (Robin et al., 2017). The blaSHV–12 gene was also detected in a significant proportion of isolates, a gene that also remains infrequent in the current ESBL epidemiology in animals, except in some food-producing birds (broilers) in Europe. Therefore, one could argue that the environmental IncHI2 plasmid carrying blaCTX–M–9, mcr-9 and blaSHV–12 may have been introduced occasionally by wild animals before displaying an epidemiological success in the rescue center. It is therefore to consider that veterinarians and all people in contact were highly exposed to ESC-R in this setting, which constitutes an open door for further ESBL spread in the community. Moreover, most animals in the rescue center are also intended to get back to nature after a few days or weeks, and this is obviously another issue with regard to ESBL dissemination in natural environments.
In conclusion, to our best knowledge, this study highlights for the first time the broad dissemination of both ESC-R plasmid and ESC-R E. coli and E. cloacae clones in a rescue center for wild animals, mainly birds. It also reveals a probable dynamic transmission of ESBL genes between E. coli and E. cloacae, which can then further disseminate to the environmental settings, but also ultimately to people in contact and to wildlife once birds are released in nature. The exact source of ESC-R in this center is not easy to clarify. The nature of the ESBL genes found, which are rather rare in domestic animals and humans, may argue for an external reservoir but this remains speculative. Importantly however, this study highlights to what extent such settings at the interface between wildlife and non-wildlife sectors may act as critical points in the amplification of ESC-R prevalence. Even though the epidemic success of the IncHI2 plasmid carrying blaCTX–M–9, mcr-9 and blaSHV–12 can be highlighted, the causes of such a wide dissemination of ESBL-producing bacteria inside the rescue center also remain unknown and may warrant investigation. Antibiotherapy was not common practice in this center (antibiotics were only prescribed in case of open fracture; M-PP, personal communication) but, as demonstrated in a recent study, a single source, such as the use of contaminated disinfectants, may be sufficient for a large and long-term contamination by resistant bacteria (Keck et al., 2020). Other hypotheses include frequent handling of animals, different birds kept in the same cage, difficulties to disinfect surfaces (often in wood) or inadequate hygiene procedures in often crowded settings, such as animal rescue centers. Since that study, the rescue center has implemented measures and procedures to avoid cross contaminations and control intra-center infections.
The datasets presented in this study can be found in online repositories. The names of the repository/repositories and accession number(s) can be found below: https://www.ncbi.nlm.nih.gov/genbank/, PRJNA659767.
Ethical review and approval was not required for the animal study because no ethical approval was needed since this study did not involve any experimentation on animals. Samples used in this study were routine samples taken at the entry of wild birds in the recue center.
NK, MH, and J-YM designed the experiments, analyzed the data, and drafted the manuscript. M-PP performed the sampling campaign. RJ and VM performed the experiments. AD performed the NGS analyses. All authors approved the final version of this manuscript.
This work was funded by the Hôpital pour la Faune Sauvage Garrigues-Cévennes, the Conseil Départemental de l’Hérault and the ANSES, the French Agency for Food, Environmental and Occupational Health & Safety.
The authors declare that the research was conducted in the absence of any commercial or financial relationships that could be construed as a potential conflict of interest.
We thank technicians from the Laboratoire Départemental Vétérinaire de l’Hérault for processing clinical samples.
The Supplementary Material for this article can be found online at: https://www.frontiersin.org/articles/10.3389/fmicb.2020.601317/full#supplementary-material
Alcalá, L., Alonso, C. A., Simón, C., González-Esteban, C., Orós, J., Rezusta, A., et al. (2016). Wild birds, frequent carriers of extended-spectrum β-lactamase (ESBL) producing Escherichia coli of CTX-M and SHV-12 types. Microb. Ecol. 72, 861–869. doi: 10.1007/s00248-015-0718-0
Annavajhala, M. K., Gomez-Simmonds, A., and Uhlemann, A. C. (2019). Multidrug-resistant Enterobacter cloacae complex emerging as a global, diversifying threat. Front. Microbiol. 10:44. doi: 10.3389/fmicb.2019.00044
Atterby, C., Borjesson, S., Ny, S., Jarhult, J. D., Byfors, S., and Bonnedahl, J. (2017). ESBL-producing Escherichia coli in Swedish gulls-A case of environmental pollution from humans? PLoS One 12:e0190380. doi: 10.1371/journal.pone.0190380
Bonnedahl, J., Drobni, M., Gauthier-Clerc, M., Hernandez, J., Granholm, S., Kayser, Y., et al. (2009). Dissemination of Escherichia coli with CTX-M type ESBL between humans and yellow-legged gulls in the south of France. PLoS One 4:e5958. doi: 10.1371/journal.pone.0005958
Bonnedahl, J., Drobni, P., Johansson, A., Hernandez, J., Melhus, A., Stedt, J., et al. (2010). Characterization, and comparison, of human clinical and black-headed gull (Larus ridibundus) extended-spectrum beta-lactamase-producing bacterial isolates from Kalmar, on the southeast coast of Sweden. J. Antimicrob. Chemother. 65, 1939–1944. doi: 10.1093/jac/dkq222
Bonnedahl, J., Hernandez, J., Stedt, J., Waldenstrom, J., Olsen, B., and Drobni, M. (2014). Extended-spectrum beta-lactamases in Escherichia coli and Klebsiella pneumoniae in Gulls. Alaska, USA. Emerg. Infect. Dis. 20, 897–899.
Bonnedahl, J., Stedt, J., Waldenstrom, J., Svensson, L., Drobni, M., and Olsen, B. (2015). Comparison of Extended-Spectrum beta-Lactamase (ESBL) CTX-M genotypes in Franklin gulls from Canada and Chile. PLoS One 10:e0141315. doi: 10.1371/journal.pone.0141315
Borjesson, S., Greko, C., Myrenas, M., Landen, A., Nilsson, O., and Pedersen, K. (2019). A link between the newly described colistin resistance gene mcr-9 and clinical Enterobacteriaceae isolates carrying blaSHV-12 from horses in Sweden. J. Glob. Antimicrob. Resist. 20, 285–289. doi: 10.1016/j.jgar.2019.08.007
Carattoli, A., Bertini, A., Villa, L., Falbo, V., Hopkins, K. L., and Threlfall, E. J. (2005). Identification of plasmids by PCR-based replicon typing. J. Microbiol. Methods 63, 219–228. doi: 10.1016/j.mimet.2005.03.018
Carroll, L. M., Gaballa, A., Guldimann, C., Sullivan, G., Henderson, L. O., and Wiedmann, M. (2019). Identification of novel mobilized colistin resistance gene mcr-9 in a multidrug-resistant, colistin-susceptible Salmonella enterica serotype Typhimurium isolate. MBio 10:e00853-19. doi: 10.1128/mBio.00853-19
Chapuis, A., Amoureux, L., Bador, J., Gavalas, A., Siebor, E., Chretien, M. L., et al. (2016). Outbreak of extended-spectrum beta-lactamase producing Enterobacter cloacae with high MICs of quaternary ammonium compounds in a hematology ward associated with contaminated sinks. Front. Microbiol. 7:1070. doi: 10.3389/fmicb.2016.01070
Chavda, K. D., Westblade, L. F., Satlin, M. J., Hemmert, A. C., Castanheira, M., Jenkins, S. G., et al. (2019). First report of blaVIM-4- and mcr-9-coharboring Enterobacter species isolated from a pediatric patient. mSphere 4:e00629-19.
Clermont, O., Christenson, J. K., Denamur, E., and Gordon, D. M. (2013). The Clermont Escherichia coli phylo-typing method revisited: improvement of specificity and detection of new phylo-groups. Environ. Microbiol. Rep. 5, 58–65. doi: 10.1111/1758-2229.12019
Dierikx, C., Van Essen-Zandbergen, A., Veldman, K., Smith, H., and Mevius, D. (2010). Increased detection of extended spectrum beta-lactamase producing Salmonella enterica and Escherichia coli isolates from poultry. Vet. Microbiol. 145, 273–278. doi: 10.1016/j.vetmic.2010.03.019
Dolejska, M., and Literak, I. (2019). Wildlife is overlooked in the epidemiology of medically important antibiotic-resistant bacteria. Antimicrob. Agents Chemother. 63, e01167–19.
Dolejska, M., Masarikova, M., Dobiasova, H., Jamborova, I., Karpiskova, R., Havlicek, M., et al. (2016). High prevalence of Salmonella and IMP-4-producing Enterobacteriaceae in the silver gull on Five Islands, Australia. J. Antimicrob. Chemother. 71, 63–70. doi: 10.1093/jac/dkv306
Eckert, C., Gautier, V., Saladin-Allard, M., Hidri, N., Verdet, C., Ould-Hocine, Z., et al. (2004). Dissemination of CTX-M-type beta-lactamases among clinical isolates of Enterobacteriaceae in Paris, France. Antimicrob. Agents Chemother. 48, 1249–1255. doi: 10.1128/aac.48.4.1249-1255.2004
EUCAST (2016). Recommendations for MIC Determination of Colistin (polymyxin E) as Recommended by the Joint CLSI-EUCAST Polymyxin Breakpoints Working Group. Sweden: EUCAST.
Fuentes-Castillo, D., Farfan-Lopez, M., Esposito, F., Moura, Q., Fernandes, M. R., Lopes, R., et al. (2019). Wild owls colonized by international clones of extended-spectrum beta-lactamase (CTX-M)-producing Escherichia coli and Salmonella Infantis in the Southern Cone of America. Sci. Total Environ. 674, 554–562. doi: 10.1016/j.scitotenv.2019.04.149
Garcia-Fernandez, A., and Carattoli, A. (2010). Plasmid double locus sequence typing for IncHI2 plasmids, a subtyping scheme for the characterization of IncHI2 plasmids carrying extended-spectrum beta-lactamase and quinolone resistance genes. J. Antimicrob. Chemother. 65, 1155–1161. doi: 10.1093/jac/dkq101
Giacopello, C., Foti, M., Mascetti, A., Grosso, F., Ricciardi, D., Fisichella, V., et al. (2016). Antimicrobial resistance patterns of Enterobacteriaceae in European wild bird species admitted in a wildlife rescue centre. Vet. Ital. 52, 139–144. doi: 10.12834/vetit.327.1374.2
Guenther, S., Aschenbrenner, K., Stamm, I., Bethe, A., Semmler, T., Stubbe, A., et al. (2012). Comparable high rates of extended-spectrum-beta-lactamase-producing Escherichia coli in birds of prey from Germany and Mongolia. PLoS One 7:e53039. doi: 10.1371/journal.pone.0053039
Guenther, S., Ewers, C., and Wieler, L. H. (2011). Extended-spectrum beta-lactamases producing E. coli in wildlife, yet another form of environmental pollution? Front. Microbiol. 2:246. doi: 10.3389/fmicb.2011.00246
Guenther, S., Grobbel, M., Lubke-Becker, A., Goedecke, A., Friedrich, N. D., Wieler, L. H., et al. (2010). Antimicrobial resistance profiles of Escherichia coli from common European wild bird species. Vet. Microbiol. 144, 219–225. doi: 10.1016/j.vetmic.2009.12.016
Guenther, S., Semmler, T., Stubbe, A., Stubbe, M., Wieler, L. H., and Schaufler, K. (2017). Chromosomally encoded ESBL genes in Escherichia coli of ST38 from Mongolian wild birds. J. Antimicrob. Chemother. 72, 1310–1313. doi: 10.1093/jac/dkx006
Hernandez, J., Johansson, A., Stedt, J., Bengtsson, S., Porczak, A., Granholm, S., et al. (2013). Characterization and comparison of extended-spectrum β-lactamase (ESBL) resistance genotypes and population structure of Escherichia coli isolated from Franklin’s Gulls (Leucophaeus pipixcan) and humans in Chile. PLoS One 8:e76150. doi: 10.1371/journal.pone.0076150
Izdebski, R., Baraniak, A., Herda, M., Fiett, J., Bonten, M. J., Carmeli, Y., et al. (2015). MLST reveals potentially high-risk international clones of Enterobacter cloacae. J. Antimicrob. Chemother. 70, 48–56. doi: 10.1093/jac/dku359
Jamborova, I., Janecko, N., Halova, D., Sedmik, J., Mezerova, K., Papousek, I., et al. (2018). Molecular characterization of plasmid-mediated AmpC beta-lactamase- and extended-spectrum beta-lactamase-producing Escherichia coli and Klebsiella pneumoniae among corvids (Corvus brachyrhynchos and Corvus corax) roosting in Canada. FEMS Microbiol. Ecol. 94:fiy166. doi: 10.1093/femsec/fiy166
Keck, N., Dunie-Merigot, A., Dazas, M., Hirchaud, E., Laurence, S., Gervais, B., et al. (2020). Long-lasting nosocomial persistence of chlorhexidine-resistant Serratia marcescens in a veterinary hospital. Vet. Microbiol. 245:108686. doi: 10.1016/j.vetmic.2020.108686
Lescat, M., Poirel, L., and Nordmann, P. (2018). Rapid multiplex polymerase chain reaction for detection of mcr-1 to mcr-5 genes. Diagn. Microbiol. Infect. Dis. 92, 267–269. doi: 10.1016/j.diagmicrobio.2018.04.010
Liao, X., Yang, R. S., Xia, J., Chen, L., Zhang, R., Fang, L. X., et al. (2019). High colonization rate of a novel carbapenem-resistant Klebsiella lineage among migratory birds at Qinghai Lake. China. J. Antimicrob. Chemother. 74, 2895–2903. doi: 10.1093/jac/dkz268
Lowe, C., Willey, B., O’shaughnessy, A., Lee, W., Lum, M., Pike, K., et al. (2012). Outbreak of extended-spectrum beta-lactamase-producing Klebsiella oxytoca infections associated with contaminated handwashing sinks(1). Emerg. Infect. Dis. 18, 1242–1247. doi: 10.3201/eid1808.111268
Mukerji, S., Gunasekera, S., Dunlop, J. N., Stegger, M., Jordan, D., Laird, T., et al. (2020). Implications of foraging and interspecies interactions of birds for carriage of Escherichia coli strains resistant to critically important antimicrobials. Appl. Environ. Microbiol. 86, e01610–e01620.
Ngaiganam, E. P., Pagnier, I., Chaalal, W., Leangapichart, T., Chabou, S., Rolain, J. M., et al. (2019). Investigation of urban birds as source of beta-lactamase-producing Gram-negative bacteria in Marseille city. France. Acta Vet. Scand. 61:51.
Robin, F., Beyrouthy, R., Bonacorsi, S., Aissa, N., Bret, L., Brieu, N., et al. (2017). Inventory of extended-spectrum-β-lactamase-producing Enterobacteriaceae in France as assessed by a multicenter study. Antimicrob. Agents Chemother 61:e01911-16. doi: 10.1128/AAC.01911-16
Schaufler, K., Semmler, T., Wieler, L. H., Wohrmann, M., Baddam, R., Ahmed, N., et al. (2016). Clonal spread and interspecies transmission of clinically relevant ESBL-producing Escherichia coli of ST410–another successful pandemic clone? FEMS Microbiol. Ecol. 92:fiv155. doi: 10.1093/femsec/fiv155
Shibata, N., Kurokawa, H., Doi, Y., Yagi, T., Yamane, K., Wachino, J.-I., et al. (2006). PCR classification of CTX-M-type beta-lactamase genes identified in clinically isolated gram-negative bacilli in Japan. Antimicrob. Agents Chemother. 50, 791–795. doi: 10.1128/aac.50.2.791-795.2006
Tarabai, H., Valcek, A., Jamborova, I., Vazhov, S. V., Karyakin, I. V., Raab, R., et al. (2019). Plasmid-mediated mcr-1 colistin resistance in Escherichia coli from a black kite in Russia. Antimicrob. Agents Chemother. 63, e01266–19.
Vittecoq, M., Laurens, C., Brazier, L., Durand, P., Elguero, E., Arnal, A., et al. (2017). VIM-1 carbapenemase-producing Escherichia coli in gulls from southern France. Ecol. Evol. 7, 1224–1232. doi: 10.1002/ece3.2707
Wang, J., Ma, Z. B., Zeng, Z. L., Yang, X. W., Huang, Y., and Liu, J. H. (2017). The role of wildlife (wild birds) in the global transmission of antimicrobial resistance genes. Zool. Res. 38, 55–80.
Yang, H., Rehman, M. U., Zhang, S., Yang, J., Li, Y., Gao, J., et al. (2019). High prevalence of CTX-M belonging to ST410 and ST889 among ESBL producing E. coli isolates from waterfowl birds in China’s tropical island Hainan. Acta Trop. 194, 30–35. doi: 10.1016/j.actatropica.2019.03.008
Keywords: CTX-M, mcr-9, wild bird, E. coli, E. cloacae, IncHI2
Citation: Haenni M, Métayer V, Jarry R, Drapeau A, Puech M-P, Madec J-Y and Keck N (2020) Wide Spread of blaCTX–M–9/mcr-9 IncHI2/ST1 Plasmids and CTX-M-9-Producing Escherichia coli and Enterobacter cloacae in Rescued Wild Animals. Front. Microbiol. 11:601317. doi: 10.3389/fmicb.2020.601317
Received: 31 August 2020; Accepted: 26 October 2020;
Published: 17 November 2020.
Edited by:
Alain Hartmann, Institut National de Recherche pour l’Agriculture, l’Alimentation et l’Environnement (INRAE), FranceReviewed by:
Sebastian Guenther, University of Greifswald, GermanyCopyright © 2020 Haenni, Métayer, Jarry, Drapeau, Puech, Madec and Keck. This is an open-access article distributed under the terms of the Creative Commons Attribution License (CC BY). The use, distribution or reproduction in other forums is permitted, provided the original author(s) and the copyright owner(s) are credited and that the original publication in this journal is cited, in accordance with accepted academic practice. No use, distribution or reproduction is permitted which does not comply with these terms.
*Correspondence: Marisa Haenni, bWFyaXNhLmhhZW5uaUBhbnNlcy5mcg==
Disclaimer: All claims expressed in this article are solely those of the authors and do not necessarily represent those of their affiliated organizations, or those of the publisher, the editors and the reviewers. Any product that may be evaluated in this article or claim that may be made by its manufacturer is not guaranteed or endorsed by the publisher.
Research integrity at Frontiers
Learn more about the work of our research integrity team to safeguard the quality of each article we publish.