- 1DAICENTER (DBT-AIST International Centre for Translational and Environmental Research) and Bioinformatics Centre, Department of Microbiology, School of Life Sciences, Sikkim University, Gangtok, India
- 2Biotech Hub, Department of Zoology, Nar Bahadur Bhandari Degree College, Gangtok, India
The lesser-known traditionally processed meat products such as beef kargyong, pork kargyong, satchu, and khyopeh are popular food items in the Himalayan state of Sikkim in India. The present study aimed to assess the microbiological safety of traditional meat products by identifying the potential spoilage or pathogenic bacteria, detecting the enterotoxins, and screening the antibiotic susceptibility patterns. The pH and moisture contents of the meat products varied from 5.3 to 5.9 and from 1.5 to 18%, respectively. The microbial loads of aerobic bacteria were 105 to 107 cfu/g, Staphylococcus 103 to 106 cfu/g, Bacillus 104 to 106 cfu/g, and total coliform 102 to 107 cfu/g, respectively. Based on 16S rRNA gene sequencing, the bacterial species isolated from traditionally processed meat products were Staphylococcus piscifermentans, Citrobacter freundii, Enterococcus faecalis, Salmonella enterica, Staphylococcus aureus, Citrobacter werkmanii, Klebsiella pneumoniae, Macrococcus caseolyticus, Klebsiella aerogenes, Staphylococcus saprophyticus, Pseudocitrobacter anthropi, Citrobacter europaeus, Shigella sonnei, Escherichia fergusonii, Klebsiella grimontii, Burkholderia cepacia, and Bacillus cereus. The enzyme-linked immunosorbent assay (ELISA) tests detected Salmonella spp. and enterotoxins produced by B. cereus well as Staphylococcus in a few tested samples. However, the PCR method did not detect the virulence genes of B. cereus and Salmonella in the isolates. Virulence gene (sea) was detected in S. piscifermentans BSLST44 and S. piscifermentans BULST54 isolated from beef kargyong and in S. aureus PSST53 isolated from pork kargyong. No enterotoxins were detected in khyopeh samples. The antibiotic sensitivity test showed that all bacterial strains were susceptible toward gentamicin, cotrimoxazole, norfloxacin, and trimethoprim. Gram-positive bacteria showed 100% sensitivity against clindamycin and erythromycin; however, 50% of the resistance pattern was observed against oxacillin followed by penicillin (33%) and ampicillin (27%).
Introduction
Meat and meat products are important dietary cultures of many communities in the world, among which some popular meat products such as sausages, hams, salami, etc. have been extensively studied in terms of microbiological safety, health benefits, and process technology (Petit et al., 2014; Santiyanont et al., 2019; Tamang et al., 2020). Culturally and organoleptically acceptable meat products are produced by traditional methods of preservation of perishable animal flesh through fermentation (Tamang et al., 2016), sun drying (Aksoy et al., 2019), smoking (Plavsic et al., 2015), and salting (Uğuz et al., 2011). Fermented meat products are usually considered safe for consumption due to low water activity (aw) and low pH, which prevent the growth of pathogenic organisms during fermentation (Holck et al., 2017). Moreover, the presence of some dominant lactic acid bacteria in fermented meat products such as Lactobacillus sakei, Lb. plantarum, Lb. curvatus, Enterococcus faecium, Pediococcus pentosaceus, Leuconostoc carnosum, Leuc. gelidum, Leuc. pseudomesenteroides, and Weissella spp. (Dias et al., 2015; Laranjo et al., 2017) also inhibit the populations of pathogenic organisms in the final products. However, there is a probability of occurrence of pathogenic organisms such as Shiga toxin-producing enterohemorrhagic Escherichia coli, Staphylococcus aureus, Salmonella, Campylobacter spp., and Listeria monocytogenes in meat products (Morales-López et al., 2019).
Varieties of lesser-known and region-specific traditionally processed meat products, produced either by smoking/sun drying or fermentation of domesticated animals such as goat, sheep, pig, ox, and yak, are consumed by different ethnic communities in Asia. Traditionally processed meat (beef, sheep, pig, and yak) products in India are chartayshya, jamma, and arjia of Uttarakhand (Oki et al., 2011); kargyong, satchu, and suka ko masu of Sikkim; and sa-um of Mizoram (Rai et al., 2009; De Mandal et al., 2018). Few species of bacteria were reported earlier from kargyong, satchu, and suka ko masu of Sikkim, purely based on limited phenotypic and biochemical tests, which included Lb. sakei, Lb. divergens, Lb. carnis, Lb. sanfransisco, Lb. curvatus, Leuc. mesenteroides, E. faecium, Bacillus subtilis, B. mycoides, B. thuringiensis, S. aureus, and Micrococcus (Rai et al., 2010).
In this study, four different types of traditionally processed meat products of Sikkim in India were selected viz. kargyong (Figure 1A), a traditional sausage-like product prepared from beef and pork meat; satchu (Figure 1B), a smoked beef meat product; and khyopeh (Figure 1C), a fermented yak meat product. Kargyong is produced by mixing lean meats of beef or pork with salt, garlic, and ginger, and then mixtures are stuffed into the intestine of animals as natural casings. Both ends of casings are then tied up with a rope, put into utensil, and boiled for 30 min, strained out, hooked in a bamboo stick, and smoked above an earthen oven for 10–15 days (Rai et al., 2009). No nitrates and nitrites are added during the preparation of kargyong. Satchu is prepared by slicing red meat of beef or yak into long thread-like strips; mixed with turmeric, salt, and oil; and smoked for 7–10 days (Rai et al., 2009; Rai and Tamang, 2017). Khyopeh is a naturally fermented yak meat product of Sikkim. During its preparation, chopped meats and innards of yak are mixed with salt, and the mixtures are stuffed into the rumen, which is previously removed from the slaughtered yak. Filled up rumen is tied up with twine and hung into bamboo stripes for natural fermentation for 4–6 months above an earthen oven (Bhutia et al., 2020). Kargyong and satchu are commonly eaten as fried side dish or curry, while khyopeh is eaten as soup or curry. However, the presence of pathogenic bacteria in these traditionally processed meat products has not been assessed as food safety measures. Hence, the present study is aimed to isolate and identify the pathogenic and spoilage bacteria, to detect enterotoxin genes, and also to screen antibiotic resistance patterns.
Materials and Methods
Sample Collection
A total of 27 samples of traditionally processed meat products viz. beef kargyong (eight samples), pork kargyong (eight), satchu (six), and khyopeh (five) were collected from different households of Sikkim in India (Table 1). Collected samples were kept in sterile polybags and put into an ice-box carrier and transported to the laboratory. Samples were stored at kept at 4°C for further analysis.
pH and Moisture
The pH was determined with a pH meter (Thermo Scientific, United States) and the moisture contents of samples were measured by a Moisture Analyzer (Ohaus MB, United States). The measurement was taken in triplicates and average values were considered.
Food Sample Preparation
About 25 g of sample was weighed and homogenized with 225 ml buffered peptone water for 2–3 min at medium speed in a Stomacher 400 (Seward, United Kingdom) to obtain 10–1 dilution. Serial dilutions were made by pipetting 1 ml of homogenized samples into 9 ml of sterile diluent. Up to 109 decimal dilutions were made from the previous dilution. An aliquot (0.1 ml) of the diluents was further plated on specific media by the spread plate method (Andrews and Hammack, 2003).
Enumeration of Microbial Load
About 0.1 ml of the homogenized sample in Butterfield’s phosphate-buffered water was inoculated into Plate Count Agar (M091, HiMedia, India), Nutrient Agar (M001, HiMedia, India), Baird Parker agar (M043, HiMedia, India), Bacillus cereus agar (M833, HiMedia, India), and Violet Red Bile Agar (M049, HiMedia, India) using the surface spread method (Feng et al., 2002). The plates were incubated at 35°C for 24 to 48 h and the colonies were counted and results were expressed as colony-forming units per gram (cfu/g). The 3MTM PetrifilmTM aerobic count plate (6400, 3M, United States) was also used to enumerate the microbial load from the collected meat samples, which contained modified standard nutrients, a soluble gelling agent, and a tetrazolium indicator (Nelson et al., 2013).
Enumeration of Staphylococcus
The presence or absence of Staphylococcus in each sample was assessed according to the Food and Drug Administration (FDA) standard method (Tallent et al., 2016). Baird–Parker agar plates were supplemented with Egg Yolk Tellurite Emulsion (FD046L, HiMedia, India). Plates were incubated at 37°C for 36–48 h. Convex, black, shiny colonies with narrow white margin surrounded by a clear zone were regarded as Staphylococcus (Tallent et al., 2016).
Enumeration of Enterococcus
Ten grams of each sample was diluted in 90 ml of peptone water (0.1% w/v) for homogenization by mechanic stirring. In products with high-fat content, 1% v/v Tween 80 (P1754, Merck, Germany) was added. Then, 0.1 ml aliquots of diluents from homogenized food were spread in Bile Esculin Azide Agar (M493, HiMedia, India) plates and incubated for 24 h at 35°C. Colonies that showed black pigmentation on the BEA agar were regarded as Enterococcus (Delpech et al., 2012).
Enumeration of Bacillus
Ten grams of each sample was blended in 90 ml of 0.85% sterile saline in using Stomacher 400 (Seward, United Kingdom) for 3 min followed by serial dilution. Aliquots of 0.1 ml of the appropriate dilutions were surface plated on prepoured plates of B. cereus agar (M833, HiMedia, India). Blue colonies with positive precipitation were regarded as Bacillus (Rai et al., 2010).
Enumeration of Escherichia and Coliform Bacteria
Ten grams of each sample was homogenized in a Stomacher 400 (Seward, United Kingdom) for 3 min in 90 ml buffered peptone water and serial dilution was prepared. An aliquot (0.1 ml) of the homogenate was transferred to Violet Red Bile Agar (M049, HiMedia, India) plates and incubated at 18–24 h at 35°C. Colonies that are purple red, 0.5 mm or larger, and surrounded by a zone of precipitated bile acids were regarded as coliforms. The colonies were further transferred to Brilliant Green Bile Broth (M1211, HiMedia, India) and incubated at 35°C for 24–48 h for gas production. Pure culture of isolates was inoculated in Eosin Methyl Blue Agar (M317, HiMedia, India) and MacConkey Agar (M0081B, HiMedia, India) for differentiation of enteric bacteria (Feng et al., 2002).
Enumeration of Salmonella
The method for isolation of Salmonella was followed as per the protocol described by Santos et al. (2020). About 25 g of the sample was aseptically homogenized and inoculated into 225 ml of buffered peptone water (M614, HiMedia, India) in a sterile jar. The pre-enrichment culture was incubated at 37°C for 18–24 h. Then, 0.1 ml of pre-enrichment culture was inoculated into 10 ml Rappaport–Vassiliadis (RV) medium (M880, HiMedia, India) and incubated at 42°C for 24 h in a circulating, thermostatically controlled water bath (1322, Remi, India). A loopful of cultured broth was streaked onto Xylose-Lysine Deoxycholate Agar (M031, HiMedia, India) plates and incubated at 37°C for 18–24 h. Pink colonies with or without black color were regarded as Salmonella. Each isolate was streaked onto Nutrient Agar (M001, HiMedia, India), incubated at 37°C for 24 h, and phenotypically characterized.
Phenotypic Characterization
The isolated bacteria were characterized on the basis of colony morphology (Tallent et al., 2016), Gram’s reaction, sporulation test (Hussey and Zayaitz, 2007), motility tests (Shields and Cathcart, 2011), enzymatic reactions, and biochemical tests.
Enzymatic Tests
Catalase test was performed by placing a loopful of bacterial isolates into the test tube containing five to six drops of 3% hydrogen peroxide. A positive reaction was represented by the presence of bubbles and no bubble formation represented catalase negative (Reiner, 2010). For the urease test, a heavy inoculum from 24 h pure culture was streaked into the entire slant surface of a tube containing Christensen’s medium and incubated at 35°C. The slant was observed for a color change up to 6 days. Urease production was indicated by a bright pink color on the slant that extends into the butt (Brink, 2010). A gelatin hydrolysis test was performed by stab-inoculating a 24-h culture into the tubes containing nutrient gelatin. The inoculated tubes and uninoculated control tubes were incubated at 25°C for up to 1 week and checked every day for gelatin liquefaction. Liquefaction due to gelatinase activity was confirmed by immersing tubes in an ice bath for 15–30 min, and then tubes were tilted to observe if the gelatin was hydrolyzed. The positive test of hydrolyzed gelatin resulted in a liquid medium after exposure to cold temperature, whereas the negative tests remained solid (dela Cruz and Torres, 2012). For the nitrate reduction test, bacterial cultures were grown in 5 ml nitrate broth for 12–24 h at 35°C. One milliliter of the culture was mixed with three drops of reagents (reagent A and reagent B) and observed for the development of a red/yellow color, indicating the presence/absence of nitrate. A small amount of zinc dust was added to the tube for 5 days to observe for the development of a red color, indicating the absence of nitrate reduction (Buxton, 2011). Coagulase and DNA hydrolysis tests were specifically performed for the characterization of Staphylococcus. For the coagulase test, about three to four isolated colonies of suspected Staphylococcus were emulsified in 0.3 ml of Brain Heart Infusion Broth (LQ210D, HiMedia, India) and incubated at 35–37°C for 18–24 h. Then, 0.5 ml of reconstituted plasma was added into the BHI culture, mixed thoroughly, and incubated at 37°C. The culture was observed for clot formation at intervals for over the next 46 h. The positive cultures exhibited clotting by the end of 24 h and negative cultures showed the absence of clot formation (Bennett et al., 1986). For the DNA hydrolysis test, pure culture of suspected Staphylococcus was streaked on DNase Test Agar w/Methyl Green (M1419, HiMedia, India) and incubated at 37°C for 24 h. A positive result was indicated by a clear halo around the growth (Kateete et al., 2010).
Biochemical Tests
Carbohydrate Fermentation
Aseptically, two to three drops of the test organism from 18 to 24 h BHI broth culture was inoculated in a Phenol Red Carbohydrate Broth containing Durham’s tube and incubated at 37°C for 24 h. A yellow color indicated fermentation of the sugar with lowering of pH to 6.8 or less. A delayed fermentation produces an orange color and bubble trapped within the Durham tube indicated gas production. A reddish or pink color indicated a negative reaction (Reiner, 2012).
IMVic (Indole, Methyl Red, Voges–Proskauer, and Citrate Utilization) and Indole Tests
Pure culture of an isolate was inoculated in the tube of Tryptone Broth (M463, HiMedia, India) and incubated at 37°C for 24–48 h. Five drops of Kovac’s indole reagent (R008, HiMedia, India) was added directly onto the cultured tube. A positive indole test was indicated by the formation of pink to red color (cherry red ring) in the reagent layer on top of the medium. A negative test was indicated by yellow color on top of the reagent layer (MacWilliams, 2012).
Methyl Red and Voges–Proskauer Test
The fresh tested culture was inoculated in 5 ml Methyl Red–Voges–Proskauer (MR-VP) broth (LQ082, HiMedia, India) and incubated for 48 h at 35°C. Then, 2.5 ml of culture was transferred into a new sterile tube and five drops of Methyl Red (MR) Reagent (I007, HiMedia, India) was added. The MR-positive test organism showed red coloration of the medium and the negative test organisms showed yellow coloration of the medium due to low acid production (McDevitt, 2009). In the remaining culture grown in MR-VP broth, 0.6 ml of Barritt’s reagent A (R029, HiMedia, India) and 0.2 ml of Barritt’s reagent B (R030, HiMedia, India) were added. The tubes were then shaken for 30 s to 1 min and the tubes were allowed to stand for 30 min to 1 h. The VP-positive organism showed red coloration on top of the culture and the VP-negative organism showed yellowish color (McDevitt, 2009).
Citrate Test
A Simmon citrate medium (M099, HiMedia, India) was prepared in a tube slant and the fresh pure test organism was inoculated on the surface of the slant, incubated at 37°C for 18–24 h. A positive organism was represented by growth on the surface and change in color from the original green to blue (MacWilliams, 2009).
Genomic Characterization
DNA Extraction
DNA was extracted from each bacterial isolate by the standard phenol/chloroform method of Cheng and Jiang (2006). The quality of DNA was checked by electrophoresis in 0.8% agarose gel and quantified using Nano-Drop ND-1000 spectrometer (Eppendorf, Germany).
PCR Amplification
The PCR of 16S rRNA gene from isolated DNA was amplified using a universal oligonucleotide primer pair 27F (5′-AGAGTTTGATCCTGGCTCAG-3′) and 1492R (5′-TACGGTTACCTTGTTACGACTT-3′) (Lane, 1991) in a thermal cycler (Thermo Fisher, United States). The reaction mixture, conditions, and protocol for the polymerase chain reaction amplification were done following the method of Chagnaud et al. (2001). The PCR amplification was performed in a mixture containing a final volume of 50 μl of GoTaq Green Master Mix (2×) (M7122, Promega, United States), 10 μM of F primer, 10 μM of R primer, and nuclease-free water (NEB). The PCR reaction program was set under the following PCR conditions: 94°C for 10 min; 94°C for 1 min, 65°C for 1 min, and 72°C for 30 s for 35 cycles; and 72°C for 7 min. The PCR products were detected by electrophoresis using 1% agarose, and the bands were stained with 7 μl/100 ml of ethidium bromide and visualized using Gel Doc EZ Imager (Bio-Rad, United States). A standard 100-base pair DNA ladder was used for the verification of amplicon size. The amplified PCR products were purified using the PEG (polyethylene glycol)–NaCl (sodium chloride) (20% w/v of PEG, 2.5 M NaCl) precipitation method of Schmitz and Riesner (2006).
16S rRNA Gene Sequencing
The PCR products were set up in 5 μl volume for a single primer amplification with the same universal primers 27F (5′-AGAGTTTGATCCTGGCTCAG-3′) and 1492R (5′-TACGGTTACCTTGTTACGACTT-3′) (Lane, 1991) for separate reactions of each primer. The PCR reaction was set as follows: denaturation (96°C, 10 s), annealing (50°C, 5 s), and elongation (60°C, 2 min) with a stop reaction at 4°C. The amplicons were then precipitated with 1 μl sodium acetate (3 M, pH 5.2) and 24 μl of absolute alcohol, mixed briefly in vortex, and incubated at room temperature for 15 min, centrifuged at 12,000 rpm for 20 min, further washed with 70% ethanol, air-dried, and suspended in 10 μl formamide. Sequencing of the amplicons was performed by the Sanger sequencing method of Heather and Chain (2016) which was carried out in an automated DNA Analyzer (ABI 3730XL Capillary Sequencers, Applied Biosystems, Foster City, CA, United States).
Data Analysis
The quality of sequences was analyzed by Sequence Scanner v.1.0 (Applied Biosystems, Foster City, CA, United States) followed by DNA sequence assembly using ChromasPro 2.1.81. The contigs were subjected to BLAST (Basic Local Alignment Search Tool) (Benson et al., 2014) for nucleotide similarity search. The sequences were then aligned by pairwise alignment using ClustalW and the phylogenetic tree was constructed by the neighbor-joining method using MEGA7.0 software (Kumar et al., 2016). The genera and species were identified based on the lowest E-value in BLAST.
Detection of Enterotoxin by ELISA
Samples were analyzed for detection of staphylococcal enterotoxin, Bacillus diarrheal enterotoxin, and Salmonella. For the extraction of Bacillus diarrheal enterotoxin, 25 g of the sample was added into 50 ml of 0.25 M Tris buffer (pH 8) and blended for 3 min. The slurry was transferred to a centrifuge bottle and centrifuged for 10 min at 5,000 rpm. The supernatant was then filtered through a prepared syringe and the eluate was adjusted to pH 7.0–8.0. Then, 5 ml of the eluate was mixed thoroughly with 50 μl of the sample additive (Rahmati and Labbe, 2008). The sample was then subjected to an enzyme-linked immunosorbent assay (ELISA) test using Tecra Bacillus diarrheal enterotoxin visual immunoassay (BDEVIA48, 3 M, United States) according to the manufacturer’s instructions. For the extraction of staphylococcal enterotoxin, 10 g of the sample was added into 15 ml PBS (pH 7.4) and homogenized for about 3 min in a blender. The test sample was centrifuged at 5,000 rpm for 10 min at 10°C and 100 μl of the supernatant was tested with a RIDASCREEN staphylococcal enterotoxin assay (SET Total) kit (R4105, R-Biopharm, Germany) for bulk detection of the enterotoxins SEA, SEB, SECl, SEC2, SEC3, SED, and SEE (Bennett, 2005). The test was performed according to the manufacturer’s instructions. For detection of Salmonella spp., 25 g of the sample was mixed with 225 ml of lactose broth and incubated at 36°C (±1°C) for 22–26 h. Then, 0.1 ml of the primary enriched sample was transferred into 10 mL of Rappaport–Vassiliadis broth and further incubated at 42°C (±1°C) for 18–24 h. One milliliter of the enrichment sample was transferred into 25 μl of sample additive in a tube and heated for 15 min in a water bath and then subjected to ELISA test using the Tecra Salmonella Visual immunoassay (SALVIA96, 3 M, United States) according to the manufacturer’s instructions (Perez-Montano et al., 2012). Although all positive results were visible with the naked eye, a microplate reader and PC software were also used to measure the results. The absorbance of the samples was measured by determining optical densities at 450 nm using the iMark Microplate Reader (Bio-Rad, United States). The positive control had an absorbance of ≥1.0 and the negative control had an absorbance of ≤0.2 as per the manufacturer’s instruction.
Detection of Enterotoxin Genes by PCR
The identified bacterial isolates were tested for the detection of few enterotoxin genes viz. Staphylococcus (sea), B. cereus (nhea, nheb), and Salmonella (invA, stn) by PCR analysis. These enterotoxin genes were selected based on their prevalence associated with foodborne illness (Kérouanton et al., 2007; Wallin-Carlquist et al., 2010; Tekale et al., 2015; Amor et al., 2019). The PCR amplification was performed in a total volume of 25 μl and the reaction mixture contained GoTaq Green Master Mix (Taq DNA polymerase, dNTPs, MgCl2, and reaction buffers), 10 μM of each primer [Imperial Life Sciences (P) Ltd, India], and nuclease-free water (P1193, Promega, United States). The amplification target genes, amplicon size in bp, primer sequences, and reaction conditions used in this experiment are given in Table 1. Ten-microliter aliquot of the PCR product was resolved on 2% agarose gel at 80 V and documented with a camera system (Bio-Rad, United States). For the positive control, a reference strain of Salmonella enterica ser. Typhimurium (MTCC 3223), B. cereus (MTCC 1272), and S. aureus (MTCC 740) obtained from IMTECH, India, was used. Bacillus nitratireducens (MK203014), Enterobacter hormaechei (MK748263), and Staphylococcus warneri (MK203007) were used as a negative control for the test.
Antibiotic Sensitivity Test
Nineteen bacterial isolates were evaluated for antimicrobial sensitivity test using the Kirby–Bauer disk diffusion method according to Clinical and Laboratory Standard Institute (CLSI) guidelines (Patel et al., 2017). The inoculum suspension was prepared by selecting four to five pure isolated colonies from overnight growth (16–24 h of incubation) on a non-selective medium with a cotton swab and suspending the colonies into sterile 0.85% physiological saline water. The density of the suspension was compared to a 0.5 McFarland Standard (R092, HiMedia, India), and turbidity was adjusted to McFarland 0.5 by adding saline or more organisms. Another sterile cotton swab was dipped into an inoculum suspension and the excess fluid was removed to avoid overinoculation of plates by pressing against the wall of the inside of the tube above the fluid level. The inoculum was spread evenly over the entire surface of Mueller–Hinton Agar (M173, HiMedia, India) plates by swabbing in three directions and allowed to stand for 3 to 5 min for drying. The standard antibiotic disks (HiMedia, India) containing amoxicillin/clavulanate (30 μg), ceftazidime (30 μg), cefuroxime (30 μg), cefepime (30 μg), trimethoprim (5 μg), nitrofurantoin (300 μg), nalidixic acid (30 μg), tobramycin (10 μg), ciprofloxacin (5 μg), erythromycin (15 μg), chloramphenicol (30 μg), penicillin G (10 μg), ampicillin (10 μg), streptomycin (10 μg), tetracycline (30 μg), gentamicin (10 μg), vancomycin (30 μg), oxacillin (1 μg), rifampicin (5 μg), cefoxitin (30 μg), aztreonam (30 μg), clindamycin (2 μg), cotrimoxazole (25 μg), norfloxacin (10 μg), cefotaxime/clavulanic acid (30 μg), and ceftriaxone (30 μg) were dispensed onto inoculated MHA plates using the disk dispenser. The antibiotic disks used were based on their availability at the laboratory at the time of study. The plates were allowed to stand for a few minutes and were incubated for 24 h at 37°C. Antibiotic sensitivity was checked by measuring the zone of inhibition from the back of the plate to the nearest millimeter using a ruler. The zone of inhibition was recorded and compared with the zone diameter interpretative chart to determine whether the bacterial isolates were susceptible, intermediate, or resistant by referring to the CLSI Performance Standards for Antimicrobial Susceptibility Testing (Patel et al., 2017) and journals (Gao et al., 2018; Thung et al., 2018). The bacteria were reported as sensitive (S), intermediate (I), or resistant (R) to each of the antibiotics used in the test. The positive control strains used for the test includes Escherichia coli (MTCC 443, IMTECH, India) for Gram-negative bacteria and S. aureus (MTCC 96, IMTECH, India) for Gram-positive bacteria. Sterile water was used as negative control.
Results
Microbial Population
A high pH was recorded in khyopeh with 5.9 and a lower pH in satchu with a mean pH of 5.5, indicating a slightly acidic nature of the meat samples (Table 2). We observed a very low percentage of moisture content in khyopeh with an average value of 2.5% (1.5–3.5%), and a higher moisture content was observed in beef kargyong with a mean value of 14.1%. A higher mean value of aerobic bacterial count was observed in beef kargyong with 9.3 × 106 cfu/g and a lower mean value of 1.2 × 106 cfu/g was found in khyopeh, respectively. Staphylococcal count was found highest in satchu with an average value of 1.8 × 106 cfu/g and least in khyopeh with a mean value of 1.0 × 103 cfu/g, whereas Bacillus count was found highest in beef kargyong with a mean value 4.1 × 105 cfu/g and lowest in satchu (2.2 × 105 cfu/g). Interestingly, growth of Bacillus was not observed in khyopeh. Total coliform count was found highest in satchu with a mean value of 1.2 × 107 cfu/g and lowest in khyopeh (2.1 × 102 cfu/g) (Table 2).
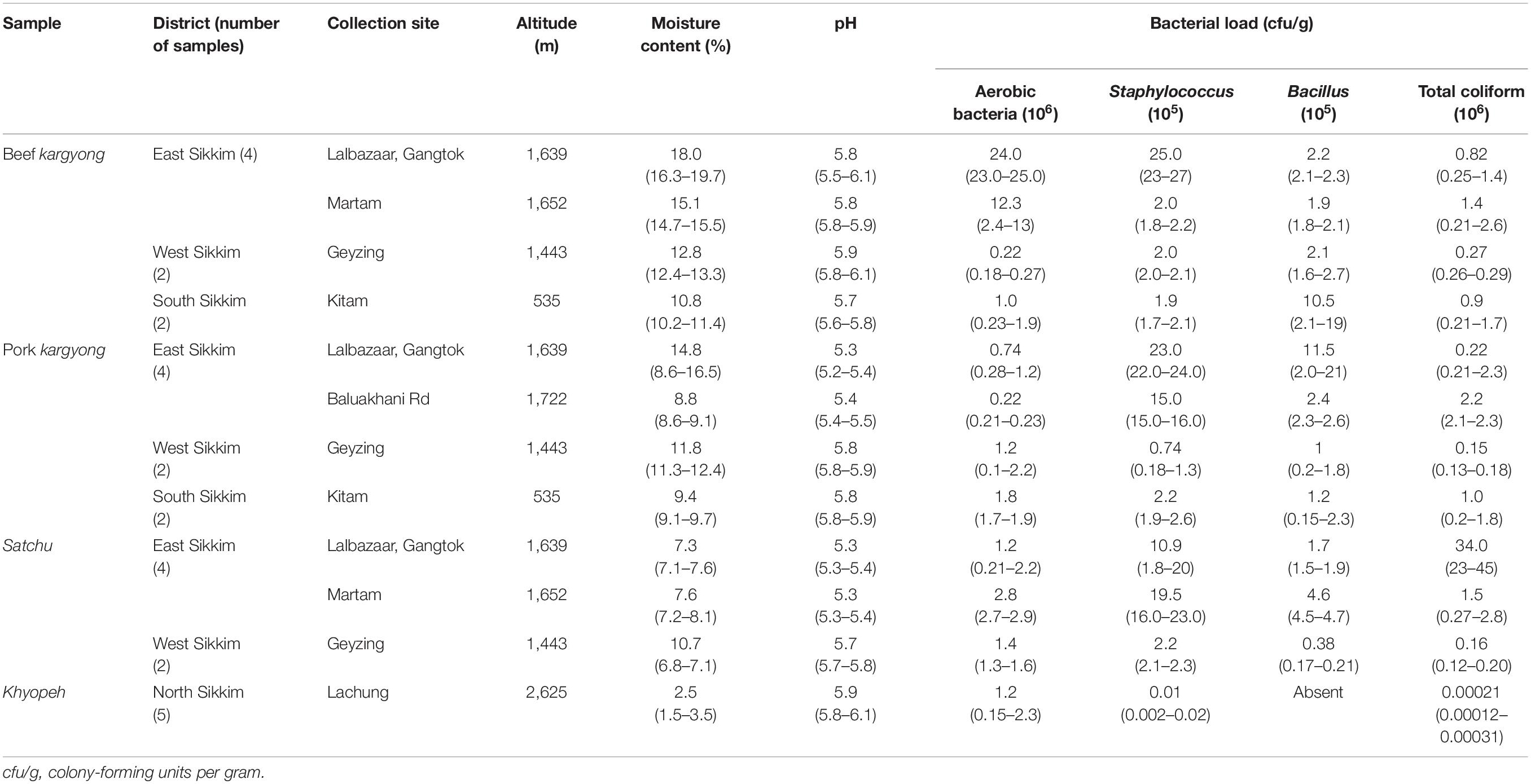
Table 2. Collection sites, altitude, moisture, pH, and bacterial load of traditionally processed meat products of Sikkim.
Identification
Selective media were used to isolate some foodborne bacterial pathogens and spoilage bacteria. A total of 128 bacteria were isolated from traditional meat samples. On the basis of cultural characteristics, cell morphology, and carbohydrate fermentation tests, bacterial genera were preliminarily identified using a taxonomical key (Holt et al., 1994) as Enterobacter, Klebsiella, Escherichia, Salmonella, Enterococcus, Bacillus, Staphylococcus, Citrobacter, and Pseudomonas (Table 3). Out of the 128 bacterial isolates, 19 representative isolates were selected from each grouped strain having similar phenotypic characteristics (data not shown) for molecular identification.
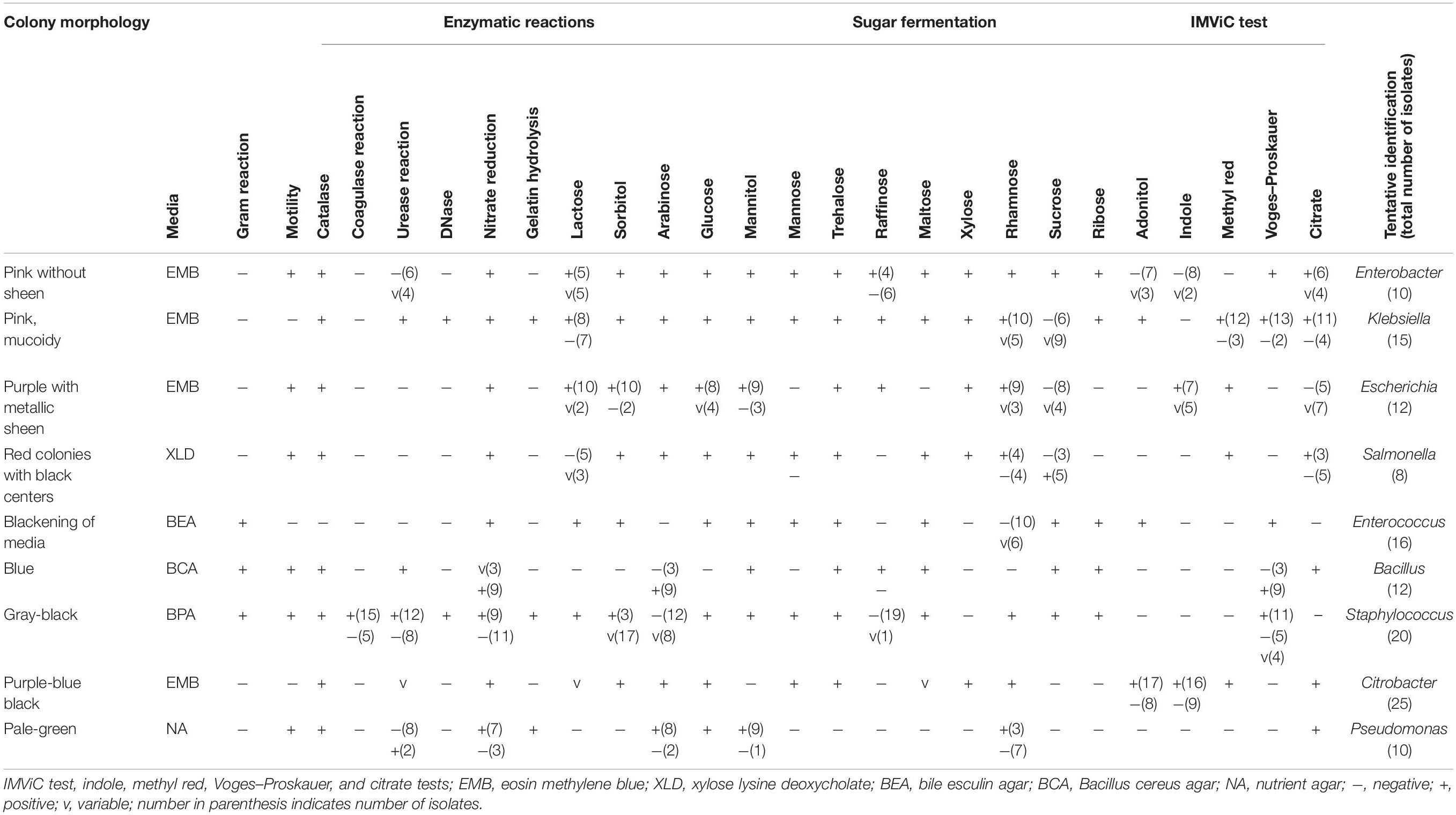
Table 3. Phenotypic and biochemical characterizations of bacteria isolated from traditionally processed meat products of Sikkim.
Based on 16S rRNA gene sequencing (Figure 2 and Table 4), bacterial species were taxonomically confirmed as Staphylococcus piscifermentans, Citrobacter freundii, S. aureus, Enterococcus faecalis, S. enterica, Citrobacter werkmanii, Klebsiella pneumoniae, Macrococcus caseolyticus, Klebsiella aerogenes, Staphylococcus saprophyticus, Pseudocitrobacter anthropi, Citrobacter europaeus, Shigella sonnei, Escherichia fergusonii, Klebsiella grimontii, Burkholderia cepacia, and B. cereus. The percentile compositions of bacterial species in various meat products of Sikkim are shown in Figure 3.
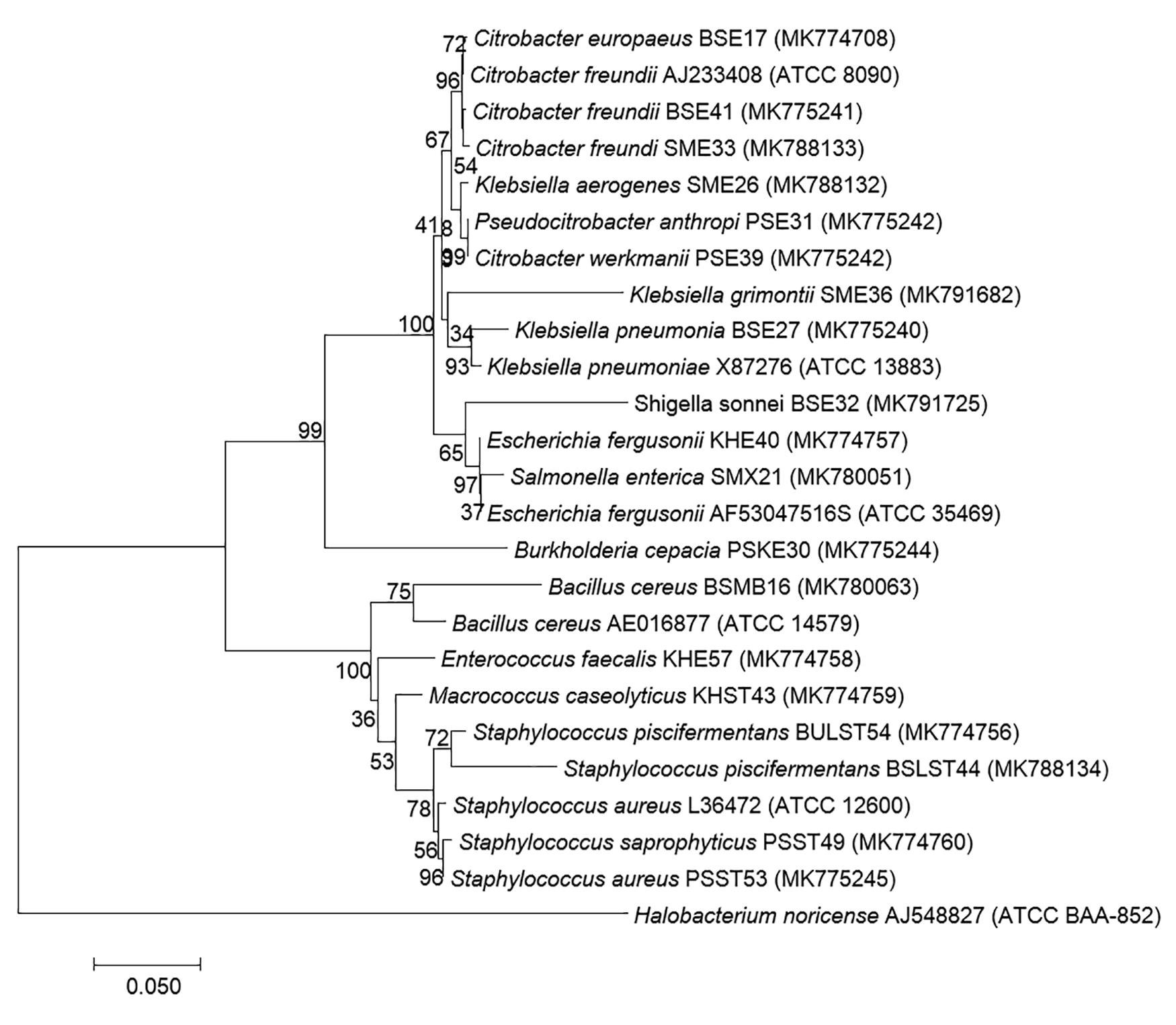
Figure 2. Molecular phylogenetic analysis of 19 bacterial isolates recovered from traditional processed meat products based on 16S rRNA region sequencing. Neighbor-joining phylogenetic tree representation by MEGA 7 with Halobacterium noricence AJ548827 as the outgroup with the evolutionary history (Kumar et al., 2016). The optimal tree with a sum of branch length = 1.13658194 and the percentage of replicate trees in which the clustered associated taxa in the bootstrap test (1,000 replicates) are shown next to the branches. The trees are drawn to scale, with branch lengths in the same units as those of the evolutionary distances used to infer the phylogenetic tree (Tamura et al., 2004), and are expressed in units of the number of base substitutions per site. Analysis involved 25 nucleotide sequences with a total of 569 positions in the final dataset.
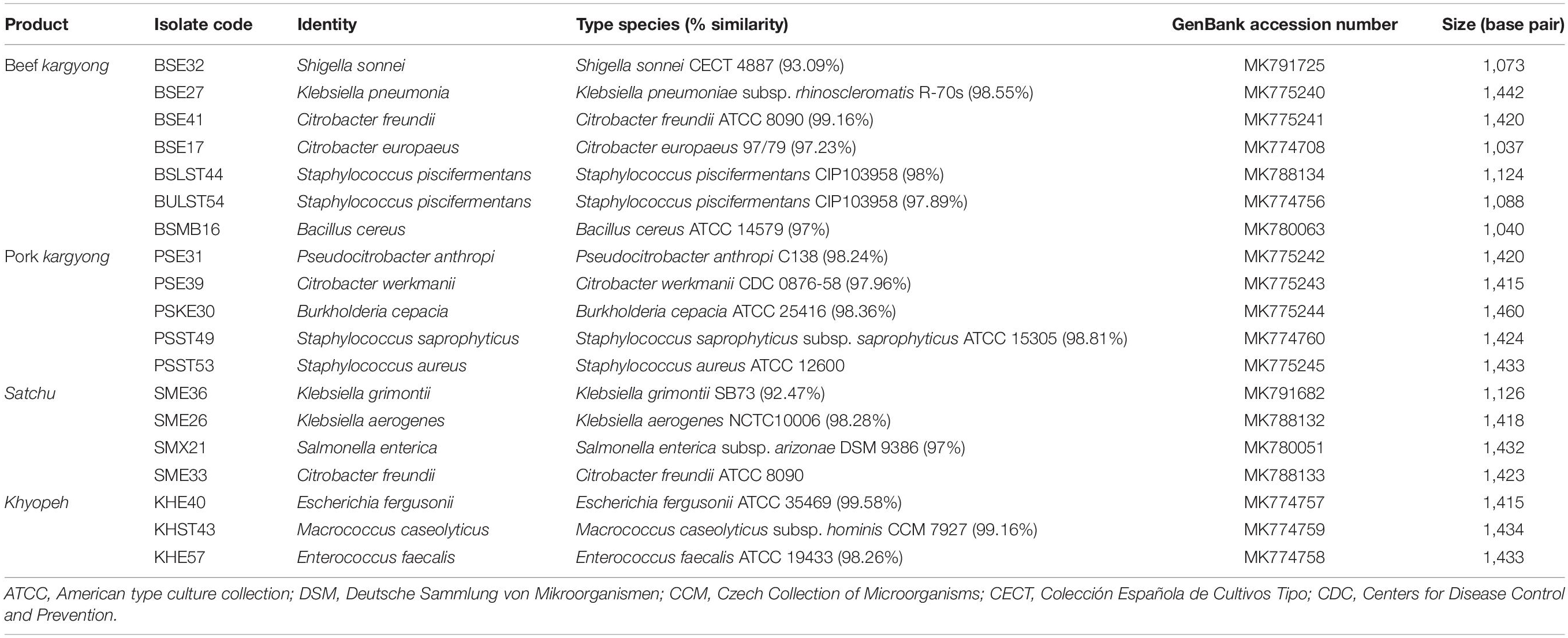
Table 4. Identification of bacterial strains isolated from traditionally processed meat products of Sikkim by 16S rRNA gene sequence based on the Basic Local Alignment Search Tool (BLAST).
Occurrence of Bacterial Enterotoxins
Enzyme-linked immunosorbent assay tests were performed on 27 samples of meat products, out of which two samples of beef kargyong tested positive for Bacillus diarrheal enterotoxins and staphylococcal enterotoxins (Table 5), two samples of pork kargyong tested positive for Salmonella spp. as well staphylococcal enterotoxins, and two samples of satchu tested positive for Bacillus diarrheal enterotoxins and Salmonella spp. However, all the tested enterotoxins were absent in the khyopeh sample. The overall prevalence of enterotoxins in the meat products detected by ELISA test is shown in Figure 4. The optical densities for each extracted sample were also measured at 450 nm with a microtiter reader. The minimum amount of detection limit (LOD) is 0.2 ng/ml of the sample according to the manufacturer’s recommendations. We found varied detectable limits with extracts of beef/pork/yak kargyong, satchu, and khyopeh ranging from 0.08 ng/ml in yak kargyong to 0.35 ng/ml in beef kargyong for Bacillus diarrheal enterotoxins, 0.06 to 0.36 ng/ml in satchu for Salmonella, and 0.08 ng/ml in khyopeh to 0.38 ng/ml in beef kargyong for staphylococcal enterotoxins (Table 6). Additionally, from cultural isolates, molecular detection of a few enterotoxin genes was also performed by the PCR technique using strain-specific primers. S. piscifermentans BSLST44 and S. piscifermentans BULST54 isolated from beef kargyong and S. aureus PSST53 isolated from pork kargyong were found positive for the sea virulent gene (Figure 5A). B. cereus BSMB16 isolated from beef kargyong was found negative for nhea and nheb genes (Figure 5B), and S. enterica SMX21 isolated from satchu was also tested negative for stn and invA virulence genes (Figure 5B).
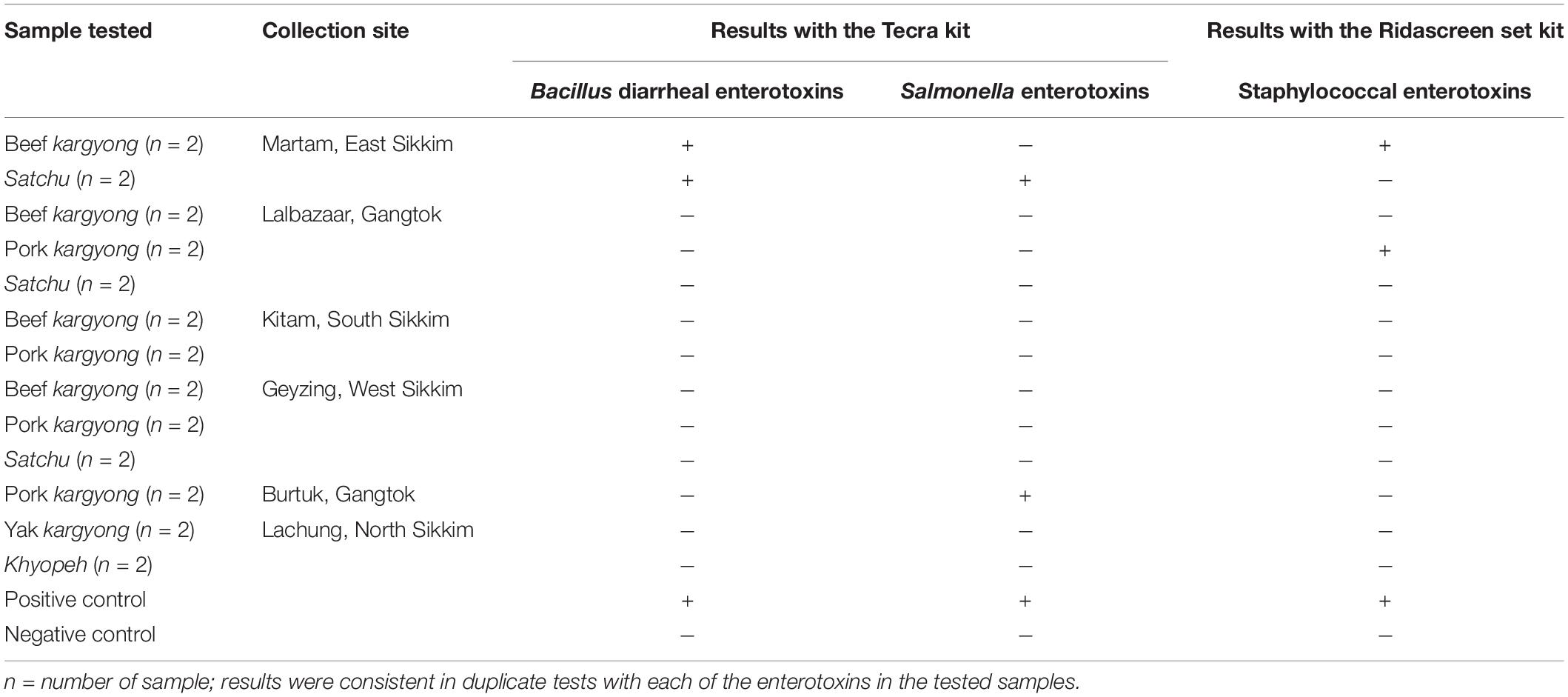
Table 5. Detection of enterotoxins in traditionally processed meat extracts by enzyme-linked immunosorbent assay.
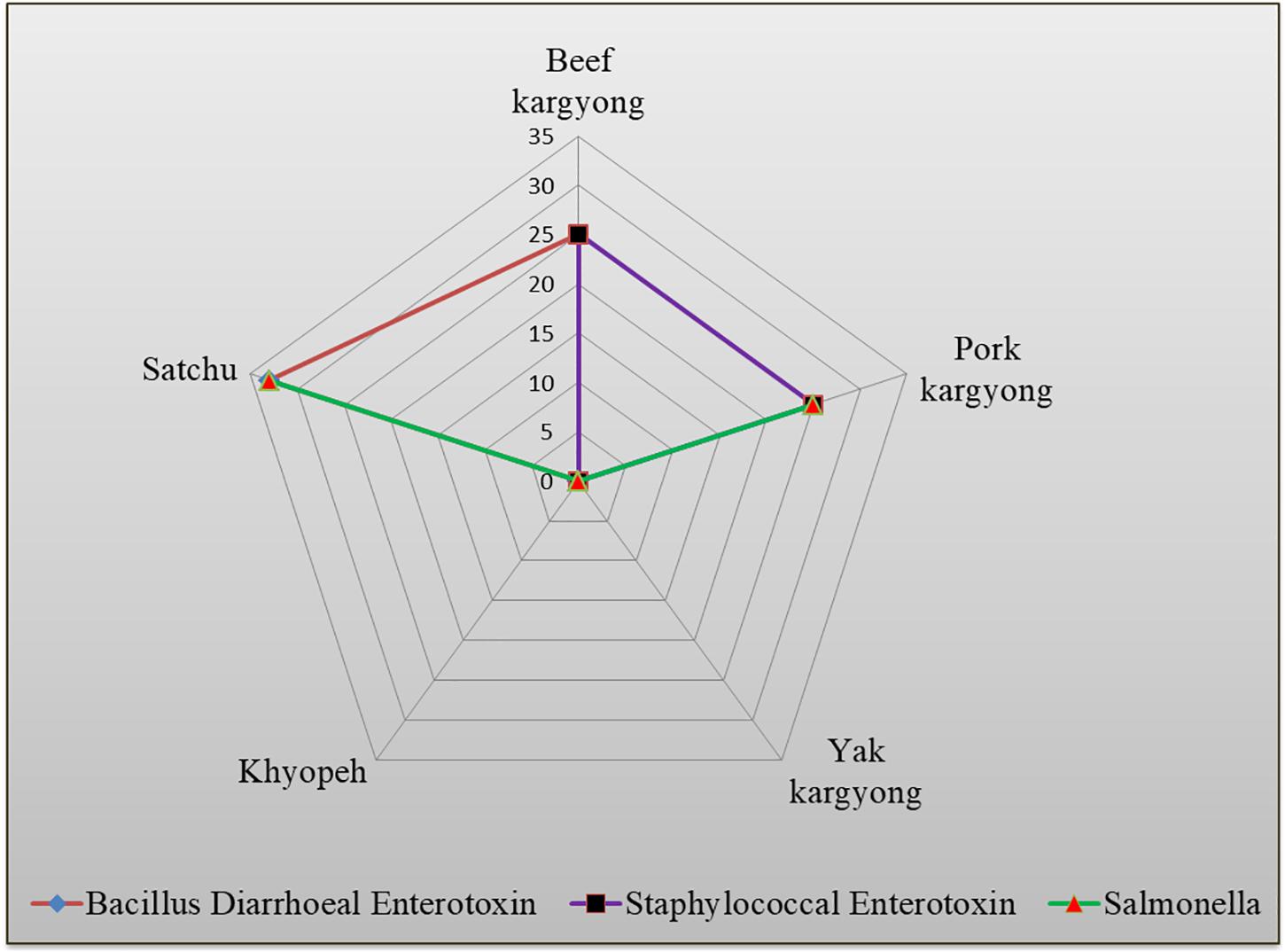
Figure 4. Percentile distribution of different bacterial enterotoxins present in traditional meat products.
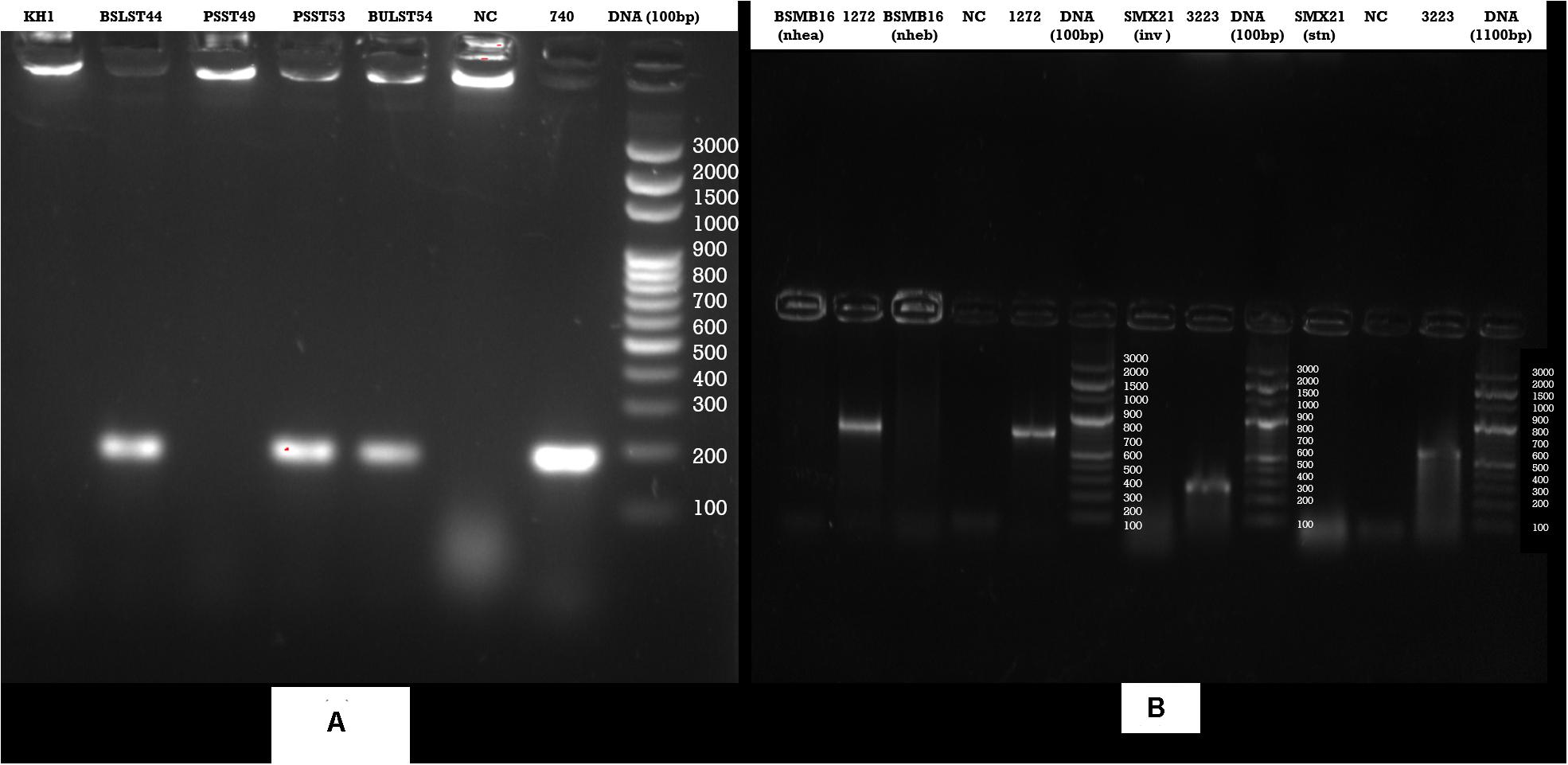
Figure 5. Agarose gel analysis of target strains after PCR amplification of DNA (A) Staphylococcus enterotoxin gene (sea), (B) Bacillus cereus enterotoxin genes (nhea, nheab) and Salmonella (inv, stn) enterotoxin genes isolated from traditional meat products.
Prevalence of Antimicrobial Resistant Bacteria
Bacteria isolated from traditionally processed meat products showed 100% susceptibility against gentamicin, cotrimoxazole, norfloxacin, and trimethoprim. However, a variable resistance pattern was also observed (Supplementary Tables 1–3). Overall, the highest level of resistance was observed in amoxicillin–clavulanate (58%) followed by ampicillin (27%). All Gram-positive bacteria were found sensitive against clindamycin and erythromycin, but a resistance pattern was observed in oxacillin (50%) followed by penicillin (33%) and ampicillin (27%) (Supplementary Tables 3, 4). Nitrofurantoin was found resistant to K. pneumoniae (BSE27) isolated from beef kargyong. Cotrimoxazole was found resistant to E. faecalis KHE57 isolated from khyopeh. The bacterial isolates from different meat samples showed variable patterns of being sensitive, intermediate, and resistant toward various classes of antibiotics which are shown in Figure 6.
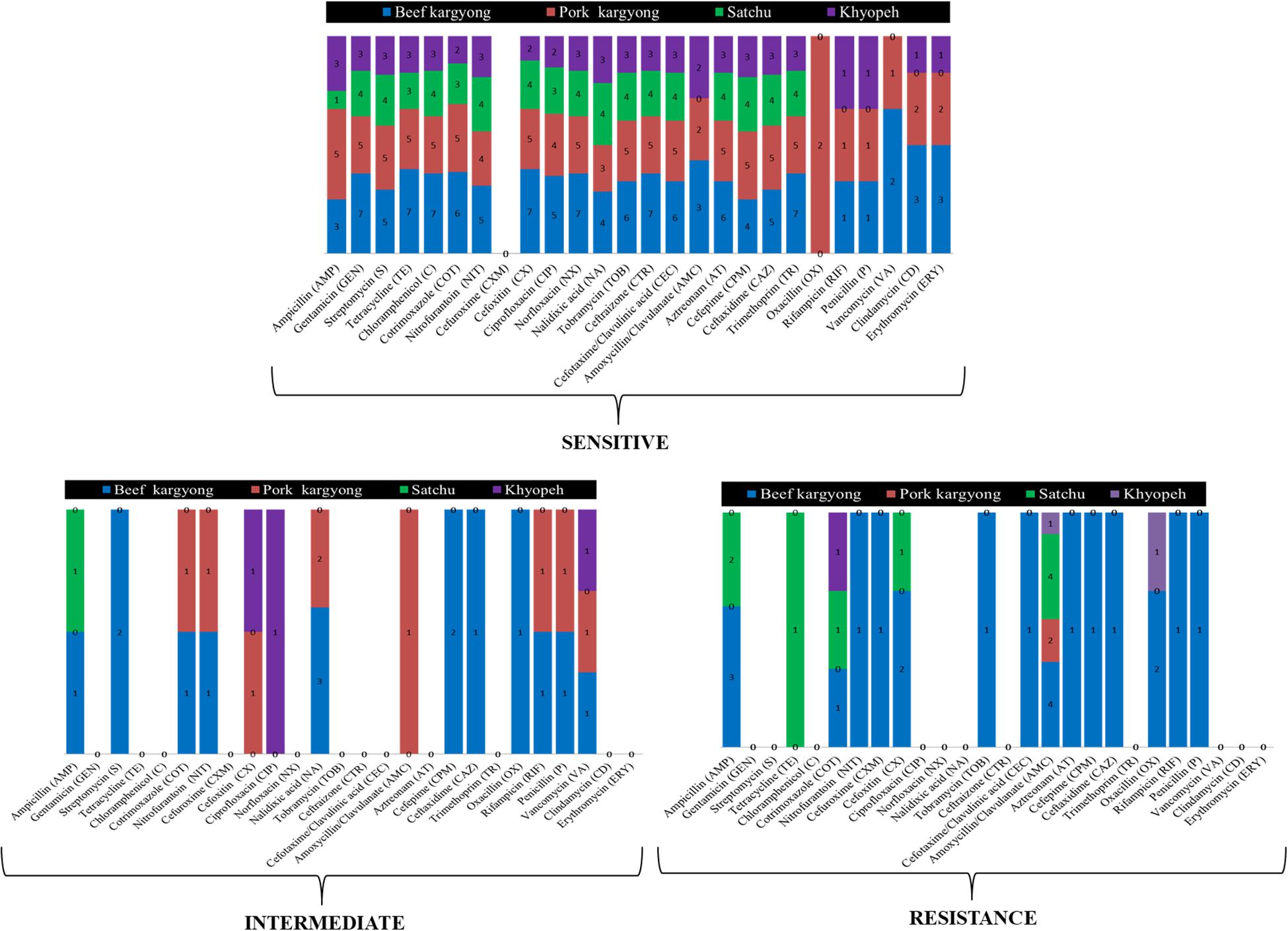
Figure 6. Bacterial isolates from different meat samples showing variable patterns of being sensitive, intermediate, and resistant against the tested antibiotics.
Discussion
In the present study, we collected four types of traditionally processed meat products (beef kargyong, pork kargyong, satchu, and khyopeh) from different places of Sikkim, and they were analyzed for pH, moisture, microbial load, spoilage/pathogenic bacteria, enterotoxins, and antibiotic susceptibility test. The pH value of the samples varied from 5.3 to 5.9 which was similar to the pH value found in traditional dry-cured lacon (5.0–6.0) (Lorenzo et al., 2015), beef and pork jerky (5.5–6.07) (Yang et al., 2018), and biltong (5.0–6.2) (Petit et al., 2014). Preservation of meat by drying has been practiced for centuries and the traditional meat products of Sikkim are preserved either by sun drying or smoking. Among the tested samples, we observed a very less moisture content in khyopeh with 2.5 to 18% in beef kargyong. The quality and stability of meat products are greatly affected by pH and moisture content (Frazier and Westhoff, 2014). Phenotypic characterization of the 128 bacterial isolates was performed for presumptive identification followed by molecular characterization of representative strains (19) targeting the 16S rRNA gene. The identified bacteria include S. piscifermentans, C. freundii, E. faecalis, S. enterica, S. aureus, C. werkmanii, K. pneumoniae, M. caseolyticus, K. aerogenes, S. saprophyticus, P. anthropi, C. europaeus, S. sonnei, E. fergusonii, K. grimontii, B. cepacia, and B. cereus. S. aureus is usually considered as a food-poisoning bacterium (Hennekinne et al., 2012), but it is frequently underreported since the illness caused by it is mild (Paparella et al., 2018). Foodborne illness caused by S. aureus is often associated with the consumption of meat and meat products (Madahi et al., 2014; Hasanpour Dehkordi et al., 2017). The prevalence of S. aureus in meat and dairy products has been reported earlier (Guven et al., 2010; Momtaz et al., 2013; Hasanpour Dehkordi et al., 2017; Rahi et al., 2020). Coagulase-negative staphylococci (CoNS) like S. saprophyticus and S. piscifermentans have been reported as flavoring microorganisms capable of reducing nitrite, enhancing color stability, and preventing rancidity by inhibiting the oxidation of unsaturated free fatty acids (Talon and Leroy, 2006). Many dried/salted/fermented meat products are often reported to be dominated by coagulase-negative staphylococci (Lorenzo et al., 2015), which may contribute to bioprotection against foodborne pathogens by producing bacteriocins (Mainar et al., 2017). We found sea enterotoxin gene from isolated strains of S. aureus and a few CoNS. Similar studies were reported in ground beef where enterotoxin and biofilm genes in coagulase-negative Staphylococcus were detected, indicating it as a potential pathogen (Pavan et al., 2019). Although Staphylococcus produces different types of enterotoxins like the classical enterotoxins (sea–see) and the newer enterotoxins (seg–sely) (Fisher et al., 2018), however, strains producing sea are implicated in the majority of cases of staphylococcal food poisoning (Kérouanton et al., 2007; Wallin-Carlquist et al., 2010). The presence of sea virulent gene in a few samples of kargyong advocates the concern for food safety of the product, which might have been contaminated during traditional processing; however, there has been no official report of food-poisoning cases in Sikkim after the consumption of kargyong.
We also identified various bacteria belonging to the family Enterobacteriaceae, which are commonly present in the intestines of human (Martinson et al., 2019) and animal (Schierack et al., 2007) that may survive in diverse environments (Martinson et al., 2019). However, they may also cause a variety of community-acquired (foodborne) and nosocomial infections (Bereket et al., 2012). Klebsiella has often been reported in meat products as a contaminant from water, containers, and feces of animal or human origin (Gundogan et al., 2013). Foods are commonly contaminated with Salmonella and Shigella by an infected food handler who practices poor hygiene and inadequate cooking (Garedew et al., 2016; Liu et al., 2018; Ranjbar et al., 2020). Salmonella is one of the most common bacterial pathogens in laboratory-confirmed foodborne illness cases causing gastroenteritis, typhoid fever, and bacteremia (Eng et al., 2015). The major identified virulence genes like inv, stn, fimA, and spv responsible for salmonellosis are linked to a combination of plasmid and chromosomal factors (Chaudhary et al., 2015). Dry meat products are traditionally considered as safe food; however, the outbreak of foodborne illness caused by S. enterica is often reported due to its ability to withstand the adverse environment leading to cross-protection against abiotic stress (Mutz et al., 2020). Although the ELISA test detected Salmonella spp. in a few tested samples, the PCR method showed the absence of invA and stn virulence genes in the isolated strain. The invA gene is primarily targeted for the identification of Salmonella in food of animal origin, since it is present in pathogenic serovars and has been demonstrated to be a rapid and effective method for diagnosis of salmonellosis (Karmi, 2013; Carraturo et al., 2016). However, there are few reports on the absence of invA gene in certain Salmonella strains, which indicate that they are not invasive or may have other invasive mechanisms (Kaushik et al., 2014; Sharma and Das, 2016; Buehler et al., 2019; Kadry et al., 2019; Yanestria et al., 2019).
Enterococci isolated from meat products have the ability to produce enterocins harboring antimicrobial activity against L. monocytogenes (da Costa et al., 2019). B. cereus is an endospore-forming bacterium responsible for foodborne illness in humans and is frequently involved in foodborne outbreaks (Tewari et al., 2015). Bacillus enterotoxins have been reported from fermented legume products like soumbala and bikalga of Africa (Ouoba et al., 2008); doenjang, a fermented soybean food of Korea (Lee et al., 2017); dairy milk (Saleh-Lakha et al., 2017); some street food of Indian Himalayas (Kharel et al., 2016); and meat products (Soleimani et al., 2018). B. cepacia is identified as a spoilage microorganism in food (Cunningham-Oakes et al., 2019).
The widespread use of antibiotics in animals for the treatment of diseases and other purposes may develop antibiotic resistance in bacteria resulting into cross-transmission of resistance genes from animals to humans through food (Safarpoor Dehkordi et al., 2017; Tang et al., 2017). The antibiogram of bacterial isolates showed a maximum rate of sensitivity against gentamicin and trimethoprim, which is in accordance with a similar report where 90% of sensitivity was observed in bacterial isolates from street foods (Amor et al., 2019). The majority of isolated strains showed the highest resistance pattern against amoxicillin–clavulanate followed by ampicillin. Amoxicillin–clavulanate-resistant bacteria were also reported from retail sausage in Malaysia which exhibited 100% resistance along with penicillin followed by ampicillin (83.3%) and cefotaxime (71.4%) (Tew et al., 2016). The increasing prevalence of amoxicillin–clavulanate resistance has been reported due to the continued spread of β-lactamase-mediated resistance (White et al., 2004). We also found a strain of bacteria identified as K. pneumoniae that showed resistance against nitrofurantoin which is a broad-spectrum antibiotic used for the treatment of uncomplicated urinary tract infections (UTI) (Osei Sekyere, 2018). Most of the Gram-positive cocci were oxacillin resistant; however, the resistance pattern was not observed in any cephalosporin class of antibiotics except for B. cereus. Overall, the majority of the isolated strains from traditionally processed meat products of Sikkim showed a maximum percentage of sensitivity against the tested antibiotics.
Conclusion
The traditional processing of perishable meat into flavor-enhanced products by drying, smoking, and fermentation is culturally and organoleptically accepted by ethnic people of Sikkim in India. This study showed the prevalence of some spoilage and pathogenic bacteria; however, enterotoxin virulent genes were not detected in bacterial strains isolated from traditional meat products except for the sea virulent gene which tested positive from two samples of kargyong. It was interesting to find out the absence of pathogenic bacteria and enterotoxins in khyopeh, which is a fermented yak meat product found only in the north district of Sikkim. Most of the bacterial strains in traditionally processed meat products of Sikkim showed a maximum percentage of sensitivity against the tested antibiotics. Some samples of meat products showed the prevalence of spoilage bacteria probably as contaminants during the traditional processing method. Hence, proper monitoring of hygienic conditions during preparation practices is highly recommended for the safety of these culturally acceptable meat products.
Data Availability Statement
The datasets generated for this study can be found in online repositories. The names of the repository/repositories and accession number(s) can be found in the article/Supplementary Material.
Author Contributions
NT and JT: conceptualization, resources, supervision, and writing—review and editing. MB: data curation, formal analysis, methodology, software, visualization, and roles/writing—original draft. JT: funding acquisition and project administration. MB and NT: investigation. MB, NT, and JT: validation. All authors contributed to the article and approved the submitted version.
Funding
The authors are grateful to the Department of Biotechnology (DBT), Government of India for financial support through DAICENTER project under sanction order: BT/B1/14/042/2017.
Conflict of Interest
The authors declare that the research was conducted in the absence of any commercial or financial relationships that could be construed as a potential conflict of interest.
Supplementary Material
The Supplementary Material for this article can be found online at: https://www.frontiersin.org/articles/10.3389/fmicb.2020.599606/full#supplementary-material
Footnotes
References
Aksoy, A., Karasu, S., Akcicek, A., and Kayacan, S. (2019). Effects of different drying methods on drying kinetics, microstructure, color, and the rehydration ratio of minced meat. Foods 8:216. doi: 10.3390/foods8060216
Amor, M. G. B., Jan, S., Baron, F., Grosset, N., Culot, A., Gdoura, R., et al. (2019). Toxigenic potential and antimicrobial susceptibility of Bacillus cereus group bacteria isolated from Tunisian foodstuffs. BMC Microbiol. 19:196. doi: 10.1186/s12866-019-1571-y
Andrews, W. H., and Hammack, T. S. (2003). BAM: Food Sampling/Preparation of Sample Homogenate. Bacteriological Analytical Manual. Maryland, MD: U.S Food and Drug Administration.
Bennett, R. W. (2005). Staphylococcal enterotoxin and its rapid identification in foods by Enzyme-linked Immunosorbent assay–based methodology. J. Food Protect. 68, 1264–1270. doi: 10.4315/0362-028x-68.6.1264
Bennett, R. W., Yeterian, M., Smith, W., Coles, C. M., Sassaman, M., and McClure, F. D. (1986). Staphylococcus aureus identification characteristics and enterotoxigenicity. J. Food Sci. 51, 1337–1339. doi: 10.1111/j.1365-2621.1986.tb13117.x
Benson, D. A., Clark, K., Karsch-Mizrachi, I., Lipman, D. J., Ostell, J., and Sayers, E. W. (2014). GenBank. Nucleic Acids Res. 43, 30–35.
Bereket, W., Hemalatha, K., Getenet, B., Wondwossen, T., Solomon, A., Zeynudin, A., et al. (2012). Update on bacterial nosocomial infections. Eur. Rev. Med. Pharmacol. Sci. 16, 1039–1044.
Bhutia, M., Thapa, N., and Tamang, J. P. (2020). Khyopeh, a traditional fermented yak meat product of Sikkim. Indian J. Trad. Knowl. 19, 187–191.
Buehler, A. J., Wiedmann, M., Kassaify, Z., and Cheng, R. A. (2019). Evaluation of invA diversity among Salmonella species suggests why some commercially available rapid detection kits may fail to detect multiple Salmonella subspecies and species. J. Food Prot. 82, 710–717. doi: 10.4315/0362-028x.jfp-18-525
Buxton, R. (2011). Nitrate and Nitrite Reduction Test Protocols. Washington, DC: American Society for Microbiology.
Carraturo, F., Gargiulo, G., Giorgio, A., Aliberti, F., and Guida, M. (2016). Prevalence, distribution, and diversity of Salmonella spp. in meat samples collected from Italian slaughterhouses. J. Food Sci. 81, M2545–M2551.
Chagnaud, P., Machinis, K., Coutte, L. A., Marecat, A., and Mercenier, A. (2001). Rapid PCR –based procedure to identify lactic acid bacteria: application to six common Lactobacillus species. J. Microbiol. Methods 44, 139–148. doi: 10.1016/s0167-7012(00)00244-x
Chaudhary, J. H., Nayak, J. B., Brahmbhatt, M. N., and Makwana, P. P. (2015). Virulence genes detection of Salmonella serovars isolated from pork and slaughterhouse environment in Ahmedabad. Gujarat. Vet. World 8, 121–124. doi: 10.14202/vetworld.2015.121-124
Cheng, H. R., and Jiang, N. (2006). Extremely rapid extraction of DNA from bacteria and yeasts. Biotechnol. Lett. 28, 55–59. doi: 10.1007/s10529-005-4688-z
Cremonesi, P., Luzzana, M., Brasca, M., Morandi, S., Lodi, R., Vimercati, C., et al. (2005). Development of a multiplex PCR assay for the identification of Staphylococcus aureus enterotoxigenic strains isolated from milk and dairy products. Mol. Cell. Probes 19, 299–305. doi: 10.1016/j.mcp.2005.03.002
Cunningham-Oakes, E., Weiser, R., Pointon, T., and Mahenthiralingam, E. (2019). Understanding the challenges of non-food industrial product contamination. FEMS Microbiol. Lett. 366:fnaa010. doi: 10.1093/femsle/fnaa010
da Costa, R. J., Voloski, F. L. S., Mondadori, R. G., Duval, E. H., and Fiorentini, A. M. (2019). Preservation of meat products with bacteriocins produced by lactic acid bacteria isolated from meat. J. Food Qual. 2019, 1–12. doi: 10.1155/2019/4726510
De Mandal, S., Singh, S. S., Bose Muthukumaran, R., Thanzami, K., Kumar, V., and Senthil Kumar, N. (2018). Metagenomic analysis and the functional profiles of traditional fermented pork fat ‘sa-um’ of Northeast India. AMB Express 8:163. doi: 10.1186/s13568-018-0695-z
dela Cruz, T. E. E., and Torres, J. M. O. (2012). Gelatin Hydrolysis Test Protocol. Washington, DC: American Society for Microbiology.
Delpech, G., Pourcel, G., Schell, C., De Luca, M., Basualdo, J., Bernstein, J., et al. (2012). Antimicrobial resistance profiles of Enterococcus faecalis and Enterococcus faecium isolated from artisanal food of animal origin in Argentina. Foodborne Pathog. Dis. 9, 939–944.
Dias, F. S., Santos, M. R. R. M., and Schwan, R. F. (2015). Enumeration, identification and safety proprieties of lactic acid bacteria isolated from pork sausage. Arq. Bras. Med. Vet. Zootec. 67, 918–926. doi: 10.1590/1678-4162-8119
Eng, S. K., Pusparajah, P., Ab Mutalib, N. S., Ser, H. L., Chan, K. G., and Lee, L. H. (2015). Salmonella: a review on pathogenesis, epidemiology and antibiotic resistance. Front. Life Sci. 8:284–293. doi: 10.1080/21553769.2015.1051243
Feng, P., Weagant, S. D., Grant, M. A., Burkhardt, W., Shellfish, M., and Water, B. (2002). Enumeration of Escherichia coli and the coliform bacteria. Bacteriological Analytical Manual. Maryland, MD: U.S Food and Drug Administration.
Fisher, E. L., Otto, M., and Cheung, G. Y. (2018). Basis of virulence in enterotoxin-mediated staphylococcal food poisoning. Front. Microbiol. 9:436. doi: 10.3389/fmicb.2018.00436
Frazier, C. W., and Westhoff, C. D. (2014). “Contamination, preservation, and spoilage of meats and meat products∗,” in Food Microbiology, 5th Edn, (New York, NY: McGraw-Hill Education).
Gao, T., Ding, Y., Wu, Q., Wang, J., Zhang, J., Yu, S., et al. (2018). Prevalence, virulence genes, antimicrobial susceptibility, and genetic diversity of Bacillus cereus isolated from pasteurized milk in China. Front. Microbiol. 9:533. doi: 10.3389/fmicb.2018.00533
Garedew, L., Hagos, Z., Zegeye, B., and Addis, Z. (2016). The detection and antimicrobial susceptibility profile of Shigella isolates from meat and swab samples at butchers’ shops in Gondar town, Northwest Ethiopia. J. Infect. Public Health 9, 348–355. doi: 10.1016/j.jiph.2015.10.015
Guinebretière, M. H., and Broussolle, V. (2002). Enterotoxigenic profiles of food-poisoning and food-borne Bacillus cereus strains. J. Clin. Microbiol. 40, 3053–3056. doi: 10.1128/jcm.40.8.3053-3056.2002
Gundogan, N., Ataol, O., and Ozturk, F. (2013). Determination of virulence factors in Staphylococcus aureus, Enterococcus faecalis and Enterococcus faecium isolated from meat and milk products. J. Food Safety 33, 387–393. doi: 10.1111/jfs.12062
Guven, K., Mutlu, M. B., Gulbandilar, A., and Cakir, P. (2010). Occurrence and characterization of Staphylococcus aureus isolated from meat and dairy products consumed in Turkey. J. Food Safety 30, 196–212. doi: 10.1111/j.1745-4565.2009.00200.x
Hasanpour Dehkordi, A., Khaji, L., Sakhaei Shahreza, M. H., Mashak, Z., Safarpoor Dehkordi, F., Safaee, Y., et al. (2017). One-year prevalence of antimicrobial susceptibility pattern of methicillin-resistant Staphylococcus aureus recovered from raw meat. Trop. Biomed. 34, 396–404.
Heather, J. M., and Chain, B. (2016). The sequence of sequencers: the history of sequencing DNA. Genomics 107, 1–8. doi: 10.1016/j.ygeno.2015.11.003
Hennekinne, J. A., De Buyser, M. K., and Dragacci, S. (2012). Staphylococcus aureus and its food poisoning toxins: characterization and outbreak investigation. FEMS Microbiol. Rev. 36, 815–836. doi: 10.1111/j.1574-6976.2011.00311.x
Holck, A., Axelsson, L., McLeod, A., Rode, T. M., and Heir, E. (2017). Health and safety considerations of fermented sausages. J. Food Quality 2017, 1–25. doi: 10.1155/2017/9753894
Holt, J. G., Krieg, N. R., Sneath, P. H. A., Staley, J. T., and Williams, S. T. (1994). Bergey’sManual of Determinative Bacteriology, 9th Edn. Baltimore, MD: Williams & Wilkins, 965–1599.
Hussey, M. A., and Zayaitz, A. (2007). Endospore Stain Protocol. Washington DC: American Society for Microbiology.
Kadry, M., Nader, S. M., Dorgham, S. M., and Kandil, M. M. (2019). Molecular diversity of the invA gene obtained from human and egg samples. Vet. World 12, 1033–1038. doi: 10.14202/vetworld.2019.1033-1038
Karmi, M. (2013). Detection of virulence gene (invA) in Salmonella isolated from meat and poultry products. Int. J. Genet. 3, 7–12.
Kateete, D. P., Kimani, C. N., Katabazi, F. A., Okeng, A., Okee, M. S., Nanteza, A., et al. (2010). Identification of Staphylococcus aureus: DNase and mannitol salt agar improve the efficiency of the tube coagulase test. Ann. Clin. Microbiol. Antimicro. 9:23. doi: 10.1186/1476-0711-9-23
Kaushik, P., Kumari, S., Bharti, S. K., and Dayal, S. (2014). Isolation and prevalence of Salmonella from chicken meat and cattle milk collected from local markets of Patna. India. Vet. World 7, 62–65. doi: 10.14202/vetworld.2014.62-65
Kérouanton, A., Hennekinne, J. A., Letertre, C., Petit, L., Chesneau, O., Brisabois, A., et al. (2007). Characterization of Staphylococcus aureus strains associated with food poisoning outbreaks in France. Int. J. Food Microbiol. 115, 369–375. doi: 10.1016/j.ijfoodmicro.2006.10.050
Kharel, N., Palni, U., and Tamang, J. P. (2016). Microbiological assessment of ethnic street foods of the Himalayas. J. Ethn. Foods 3, 235–241. doi: 10.1016/j.jef.2016.01.001
Kumar, S., Stecher, G., and Tamura, K. (2016). MEGA7: molecular evolutionary genetics analysis version 7.0 for bigger datasets. Mol. Biol. Evol. 33, 1870–1874. doi: 10.1093/molbev/msw054
Lane, D. J. (1991). “16S/23S rRNA sequencing,” in Nucleic Acid Techniques in Bacterial Systematic, eds E. Stackebrandt and M. Goodfellow (New York: John Wiley and Sons), 115–175.
Laranjo, M., Elias, M., and Fraqueza, M. J. (2017). The use of starter cultures in traditional meat products. J. Food Qual. 2017:9546026. doi: 10.1155/2017/9546026
Lee, N., Kim, M. D., Chang, H. J., Choi, S. W., and Chun, H. S. (2017). Genetic diversity, antimicrobial resistance, toxin gene profiles, and toxin production ability of Bacillus cereus isolates from doenjang, a Korean fermented soybean paste. J. Food Saf. 37.
Liu, H., Whitehouse, C. A., and Li, B. (2018). Presence and persistence of Salmonella in water: the impact on microbial quality of water and food safety. Front. Public Health 6:159. doi: 10.3389/fpubh.2018.00159
Lorenzo, J. M., Bermúdez, R., Domínguez, R., Guiotto, A., Franco, D., and Purriños, L. (2015). Physicochemical and microbial changes during the manufacturing process of dry-cured lacón salted with potassium, calcium and magnesium chloride as a partial replacement for sodium chloride. Food Contr. 50, 763–769. doi: 10.1016/j.foodcont.2014.10.019
MacWilliams, M. P. (2009). Citrate Test Protocol. Washington, DC: American Society for Microbiology.
Madahi, H., Rostami, F., Rahimi, E., and Dehkordi, F. S. (2014). Prevalence of enterotoxigenic Staphylococcus aureus isolated from chicken nugget in Iran. Jundishapur. J. Microbiol. 7:e10237. doi: 10.5812/jjm.10237
Mainar, M. S., Stavropoulou, D. A., and Leroy, F. (2017). Exploring the metabolic heterogeneity of coagulase-negative staphylococci to improve the quality and safety of fermented meats: a review. Int. J. Food Microbiol. 247, 24–37. doi: 10.1016/j.ijfoodmicro.2016.05.021
Martinson, J. N. V., Pinkham, N. V., Peters, G. W., Cho, H., Heng, J., Rauch, M., et al. (2019). Rethinking gut microbiome residency and the Enterobacteriaceae in healthy human adults. J. ISME. 13, 2306–2318. doi: 10.1038/s41396-019-0435-7
McDevitt, S. (2009). Methyl Red and Voges-Proskauer Test Protocols. Washington, DC: American Society for Microbiology.
Momtaz, H., Dehkordi, F. S., Rahimi, E., Asgarifar, A., and Momeni, M. (2013). Virulence genes and antimicrobial resistance profiles of Staphylococcus aureus isolated from chicken meat in Isfahan province. Iran. J. Appl. Poul. Res. 22, 913–921. doi: 10.3382/japr.2012-00673
Morales-López, S., Yepes, J. A., Prada-Herrera, J. C., and Torres-Jiménez, A. (2019). Enterobacteria in the 21st century: a review focused on taxonomic changes. J. of Infect. in Dev. Countr. 13, 265–273. doi: 10.3855/jidc.11216
Murugkar, H. V., Rahman, H., and Dutta, P. K. (2003). Distribution of virulence genes in Salmonella serovars isolated from man and animals. Indian J. Med. Res. 117, 66–70.
Mutz, Y. D. S., Rosario, D. K. A., Paschoalin, V. M. F., and Conte-Junior, C. A. (2020). Salmonella enterica: a hidden risk for dry-cured meat consumption? Crit. Rev. Food Sci. Nutr. 60, 976–990. doi: 10.1080/10408398.2018.1555132
Nayak, R., Stewart, T., Wang, R. F., Lin, J., Cerniglia, C. E., and Kenney, P. B. (2004). Genetic diversity and virulence gene determinants of antibiotic-resistant Salmonella isolated from preharvest turkey production sources. Int. J. Food Microbiol. 91, 51–62. doi: 10.1016/s0168-1605(03)00330-1
Nelson, M. T., LaBudde, R. A., Tomasino, S. F., Pines, R. M., Bennett, M., Dormstetter, K., et al. (2013). Comparison of 3MTM petrifilmTM aerobic count plates to standard plating methodology for use with AOAC antimicrobial efficacy methods 955.14, 955.15, 964.02, and 966.04 as an alternative enumeration procedure: collaborative study. J. AOAC Int. 96, 717–722. doi: 10.5740/jaoacint.12-469
Oki, K., Rai, A. K., Sato, S., Watanabe, K., and Tamang, J. P. (2011). Lactic acid bacteria isolated from ethnic preserved meat products of the Western Himalayas. Food Microbiol. 28, 1308–1315. doi: 10.1016/j.fm.2011.06.001
Osei Sekyere, J. (2018). Genomic insights into nitrofurantoin resistance mechanisms and epidemiology in clinical Enterobacteriaceae. Future Sci. OA 4:FSO293. doi: 10.4155/fsoa-2017-0156
Ouoba, L. I. I., Thorsen, L., and Varnam, A. H. (2008). Enterotoxins and emetic toxins production by Bacillus cereus and other species of Bacillus isolated from Soumbala and Bikalga, African alkaline fermented food condiments. Int. J. Food Microbiol. 124, 224–230. doi: 10.1016/j.ijfoodmicro.2008.03.026
Paparella, A., Serio, A., Rossi, C., Mazzarrino, G., and López, C. C. (2018). “Food-Borne transmission of staphylococci,” in Pet-To-Man Travelling Staphylococci, ed. V. Savini (Cambridge: Academic Press), 71–94. doi: 10.1016/b978-0-12-813547-1.00006-6
Patel, J. B., Cockerill, F. III, Eliopoulos, G., Jenkins, S., Lewis, J., Limbago, B., et al. (2017). M100 Performance Standards for Antimicrobial Susceptibility Testing, 27th Edn. Wayne, PA: CLSI.
Pavan, A. C. L., Nakadomari, G. H., Vignoto, V. K. C., Sfaciotte, R. A. P., Melo, F. D., Ferraz, S. M., et al. (2019). Enterotoxin and slime gene detection in Staphylococcus coagulase negative isolated from ground beef. Rev. Ciênc. Vet. E Saúde Pública 6, 30–40.
Perez-Montano, J. A., Gonzalez-Aguilar, D., Barba, J., Pacheco-Gallardo, C., Campos-Bravo, C. A., Garcia, S., et al. (2012). Frequency and antimicrobial resistance of Salmonella serotypes on beef carcasses at small abattoirs in Jalisco State, Mexico. J. Food Protect. 75, 67–873.
Petit, T., Caro, Y., Petit, A. S., Santchurn, S. J., and Collignan, A. (2014). Physicochemical and microbiological characteristics of biltong, a traditional salted dried meat of South Africa. Meat Sci. 9, 1313–1317. doi: 10.1016/j.meatsci.2013.11.003
Plavsic, D., Okanovic, D., Gubic, J., and Njezic, Z. (2015). Microbiological and chemical evaluation of dried smoked meat product. Procedia Food Sci. 5, 239–242. doi: 10.1016/j.profoo.2015.09.061
Rahi, A., Kazemeini, H., Jafariaskari, S., Seif, A., Hosseini, S., and Dehkordi, F. S. (2020). Genotypic and phenotypic-based assessment of antibiotic resistance and profile of staphylococcal cassette chromosome mec in the methicillin-resistant Staphylococcus aureus recovered from raw milk. Infect. Drug Resist. 13:273. doi: 10.2147/IDR.S229499
Rahmati, T., and Labbe, R. (2008). Levels and toxigenicity of Bacillus cereus and Clostridium perfringens from retail seafood. J. Food Protect. 71, 1178–1185. doi: 10.4315/0362-028x-71.6.1178
Rai, A. K., Palni, U., and Tamang, J. P. (2009). Traditional knowledge of the Himalayan people on production of indigenous meat products. Indian J. Trad. Knowl. 8, 104–109.
Rai, A. K., and Tamang, J. P. (2017). Prevalence of Staphylococcus and Micrococcus in traditionally prepared meat products. J. Sci. Indus. Res. 76, 351–354.
Rai, A. K., Tamang, J. P., and Palni, U. (2010). Microbiological studies of ethnic meat products of the Eastern Himalayas. Meat Sci. 85, 560–567. doi: 10.1016/j.meatsci.2010.03.006
Ranjbar, R., Safarpoor Dehkordi, F., and Heiat, M. (2020). The frequency of resistance genes in Salmonella enteritidis strains isolated from Cattle. Iran J. Publ. Health 49, 967–984.
Reiner, K. (2012). Carbohydrate Fermentation Protocol. Washington, DC: American Society for Microbiology.
Safarpoor Dehkordi, F., Gandomi, H., Akhondzadeh Basti, A., Misaghi, A., and Rahimi, E. (2017). Phenotypic and genotypic characterization of antibiotic resistance of methicillin-resistant Staphylococcus aureus isolated from hospital food. Antimicrob. Resist. Infect. Contr. 6, 104–104.
Saleh-Lakha, S., Leon-Velarde, C. G., Chen, S., Lee, S., Shannon, K., Fabri, M., et al. (2017). A study to assess the numbers and prevalence of Bacillus cereus and its toxins in pasteurized fluid milk. J. Food Prot. 80, 1085–1089. doi: 10.4315/0362-028x.jfp-16-521
Santiyanont, P., Chantarasakha, K., Tepkasikul, P., Srimarut, Y., Mhuantong, W., Tangphatsornruang, S., et al. (2019). Dynamics of biogenic amines and bacterial communities in a Thai fermented pork product Nham. Food Res. Int. 119, 110–118. doi: 10.1016/j.foodres.2019.01.060
Santos, P. D. M., Widmer, K. W., and Rivera, W. L. (2020). PCR-based detection and serovar identification of Salmonella in retail meat collected from wet markets in Metro Manila, Philippines. PLoS One 15:e0239457. doi: 10.1371/journal.pone.0239457
Schierack, P., Walk, N., Reiter, K., Weyrauch, K., and Wieler, L. (2007). Composition of intestinal Enterobacteriaceae populations of healthy domestic pigs. Microbiology 153, 3830–3837. doi: 10.1099/mic.0.2007/010173-0
Schmitz, A., and Riesner, D. (2006). Purification of nucleic acids by selective precipitation with polyethylene glycol 6000. Anal. Biochem. 354, 311–313. doi: 10.1016/j.ab.2006.03.014
Sharma, I., and Das, K. (2016). Detection of invA gene in isolated Salmonella from marketed poultry meat by PCR assay. J Food Process Technol. 7:564. doi: 10.4172/2157-7110.1000564
Shields, P., and Cathcart, L. (2011). Motility Test Medium Protocol. Washington, DC: American Society for Microbiology.
Soleimani, M., Hosseini, H., Pilevar, Z., Mehdizadeh, M., and Carlin, F. (2018). Prevalence, molecular identification and characterization of Bacillus cereus isolated from beef burgers. J. Food Saf. 38, 1–8. doi: 10.1111/jfs.12414
Tallent, S., Hait, Jennifer, Bennett, R. W., and Lancette, G. A. (2016). Staphylococcus aureus. Bacteriological Analytical Manual. Maryland, MD: U.S. Food and Drug Administration.
Talon, R., and Leroy, S. (2006). “Latest developments in meat bacterial starters,” in Advanced Technologies for Meat Processing, eds L. M. L. Nollet and F. Toldra (New York, NY: CRC Press), 401–418. doi: 10.1201/9781420017311.ch16
Tamang, J. P., Cotter, P. D., Endo, A., Han, N. S., Kort, R., Liu, S. Q., et al. (2020). Fermented foods in a global age: east meets West. Compreh. Rev. Food Sci. Food Saf. 19, 184–217. doi: 10.1111/1541-4337.12520
Tamang, J. P., Holzapfel, W. H., and Watanabe, K. (2016). Diversity of microorganisms in global fermented foods and beverages. Front. Microbiol. 7:377. doi: 10.3389/fmicb.2016.00377
Tamura, K., Nei, M., and Kumar, S. (2004). Prospects for inferring very large phylogenies by using the neighbor-joining method. Proc. Nat. Aca. Sci. U.S.A. 101, 11030–11035. doi: 10.1073/pnas.0404206101
Tang, K. L., Caffrey, N. P., Nóbrega, D. B., Cork, S. C., Ronksley, P. E., Barkema, H. W., et al. (2017). Restricting the use of antibiotics in food-producing animals and its associations with antibiotic resistance in food-producing animals and human beings: a systematic review and meta-analysis. Lancet Plan. Health 1, 316–327.
Tekale, A. A., Savalia, C. V., Kshirsagar, D. P., Brahmbhatt, M. N., and Chatur, Y. A. (2015). Detection and virulence gene characterization of Salmonella isolates from fish by conventional and molecular methods. J. Vet. Pub. Health 13, 43–46.
Tew, L. S., She, L. Y., and Chew, C. H. (2016). Isolation, antimicrobial susceptibility profile and detection of Sul1, blaTEM, and blaSHV in amoxicillin-clavulanate-resistant bacteria isolated from retail sausages in Kampar, Malaysia. Jundishapur. J. Microbiol. 9, 37897–37897.
Tewari, A., Singh, S. P., and Singh, R. (2015). Incidence and enterotoxigenic profile of Bacillus cereus in meat and meat products of Uttarakhand, India. J. Food Sci. Technol. 52, 1796–1801. doi: 10.1007/s13197-013-1162-0
Thung, T. Y., Radu, S., Mahyudin, N. A., Rukayadi, Y., Zakaria, Z., Mazlan, N., et al. (2018). Prevalence, virulence genes and antimicrobial resistance profiles of Salmonella serovars from retail beef in Selangor, Malaysia. Front. Microbiol. 8:2697. doi: 10.3389/fmicb.2017.02697
Uğuz, Ş, Soyer, A., and Dalmiş, Ü (2011). Effects of different salt contents on some quality characteristics during processing of dry-cured Turkish Pastirma. J. Food Qual. 34, 204–211. doi: 10.1111/J.1745-4557.2011.00382.X
Wallin-Carlquist, N., Márta, D., Borch, E., and Rådström, P. (2010). Prolonged expression and production of Staphylococcus aureus enterotoxin A in processed pork meat. Int. J. Food Microbiol. 141, 69–74.
White, A. R., Kaye, C., Poupard, J., Pypstra, R., Woodnutt, G., and Wynne, B. (2004). Augmentin® (amoxicillin/clavulanate) in the treatment of community-acquired respiratory tract infection: a review of the continuing development of an innovative antimicrobial agent. J. Antimicrob. Chemother. 53, 3–20.
Yanestria, S. M., Rahmaniar, R. P., Wibisono, F. J., and Effendi, M. H. (2019). Detection of invA gene of Salmonella from milkfish (Chanos chanos) at Sidoarjo wet fish market, Indonesia, using polymerase chain reaction technique. Vet. World 12, 170–175. doi: 10.14202/vetworld.2019.170-175
Keywords: meat products, 16S rRNA, ELISA, pathogens, enterotoxin, virulent genes
Citation: Bhutia MO, Thapa N and Tamang JP (2021) Molecular Characterization of Bacteria, Detection of Enterotoxin Genes, and Screening of Antibiotic Susceptibility Patterns in Traditionally Processed Meat Products of Sikkim, India. Front. Microbiol. 11:599606. doi: 10.3389/fmicb.2020.599606
Received: 27 August 2020; Accepted: 07 December 2020;
Published: 11 January 2021.
Edited by:
Eugenia Bezirtzoglou, Democritus University of Thrace, GreeceReviewed by:
Farhad Safarpoor Dehkordi, University of Tehran, IranThomas S. Hammack, United States Food and Drug Administration, United States
Copyright © 2021 Bhutia, Thapa and Tamang. This is an open-access article distributed under the terms of the Creative Commons Attribution License (CC BY). The use, distribution or reproduction in other forums is permitted, provided the original author(s) and the copyright owner(s) are credited and that the original publication in this journal is cited, in accordance with accepted academic practice. No use, distribution or reproduction is permitted which does not comply with these terms.
*Correspondence: Namrata Thapa, bmFtdW1hbmlAaG90bWFpbC5jb20=; Jyoti Prakash Tamang, anlvdGlfdGFtYW5nQGhvdG1haWwuY29t