- 1Department of Science and Technology, University of Sannio, Benevento, Italy
- 2Department of Molecular Medicine and Medical Biotechnology, Federico II University, Naples, Italy
- 3Institute of Food Science-National Research Council, Avellino, Italy
- 4CEINGE, Advanced Biotechnologies s.c.ar.l., Naples, Italy
In the last decades, resistant microbial infection rate has dramatically increased, especially infections due to biofilm-producing strains that require increasingly complex treatments and are responsible for the increased mortality percentages compared with other infectious diseases. Considering that biofilms represent a key factor for a wide range of chronic infections with high drug tolerance, the treatment of biofilm-causing bacterial infections represents a great challenge for the future. Among new alternative strategies to conventional antimicrobial agents, the scientific interest has shifted to the study of biologically active compounds from plant-related extracts with known antimicrobial properties, in order to also evaluate their antibiofilm activity. In this regard, the aim of this study has been to assess the antibiofilm activity of polyphenolic extracts from myrtle leaf and pomegranate peel against oral pathogens of dental plaque, an excellent polymicrobial biofilm model. In particular, the in vitro antibiofilm properties of myrtle and pomegranate extracts, also in binary combination, were highlighted. In addition to inhibiting the biofilm formation, the tested polyphenolic extracts have been proven to destroy both preformed single-species and multispecies biofilms formed by Streptococcus mutans, Streptococcus oralis, Streptococcus mitis, and Rothia dentocariosa oral isolates, suggesting that the new natural sources are rich in promising compounds able to counteract biofilm-related infections.
Introduction
Biofilms are complex microbial aggregations encapsulated in a hydrated polymeric matrix of their own synthesis (Aparna and Yadav, 2008). This bacterial complex limits the penetration of antimicrobial drugs, thus protecting the microbial cells entrapped. Furthermore, the biofilm microenvironment is favorable to microbial proliferation and exchange of genetic material, including the transmission of resistance genes (Chambless et al., 2006). About 80% of human bacterial infections were shown to be caused by biofilm-associated microorganisms (Wenzel, 2007), and more than half of infectious diseases related to biofilm formation involves bacterial species that are commensal or common in the human body environment (WHO, 2014). Oral bacteria are an example of human opportunistic species that can cause biofilm-related infections. Epithelial cells, dental surfaces, and orthodontic prosthesis are some of the numerous surfaces prone to the establishment of multispecies biofilms in the oral cavity (Marsh, 2004). They can cause several infectious diseases, including gingivitis, periodontitis, and dental caries, that still represent some of the most common chronic diseases, in both children and adults (Nishikawara et al., 2007). In addition, some oral bacterial species can also be responsible for critical infections outside the oral environment, such as bacteremia (Yang et al., 2009), endocarditis (Keng et al., 2012), and peritonitis (Khan et al., 2014). The oral microbiota can act as a reservoir for respiratory pathogens. Therefore, there is a close association between oral bacterial species and several forms of lung diseases, such as pneumonia (Raghavendran et al., 2007). Streptococcus mutans is considered the most cariogenic of all oral streptococci but is also implicated in subacute bacterial endocarditis and other extraoral pathologies, like nephropathy and atherosclerosis (Lemos et al., 2019). Streptococcus oralis belongs to the human oral microbiota but is also capable of opportunistic infections. It has been related to periodontal diseases and also to bacterial endocarditis (Byers et al., 2000), otitis media, septicemia, and pneumonia in children, in association with Streptococcus pneumoniae (Whalan et al., 2006). Streptococcus mitis and Rothia dentocariosa are commensal bacteria of the human mouth and the upper respiratory tract. These usually represent the etiologic agents in odontogenic infections and may contribute to dental caries. In addition, outside their niche, they can cause a wide range of infectious complications, such as endocarditis and septicemia (Mitchell, 2011).
It has been reported that bacteria living within biofilm, also in oral ones, are more tolerant to antibiotics as they are insensitive to the host’s immune response (Caraher et al., 2007). Therefore, biofilm-related infections can persist for a long time, thus progressing from acute infections to chronic infections (Leid et al., 2002). The increasing critical role of biofilms in pathogenesis and antimicrobial resistance led scientists to consider this complex structure as a drug target to tackle resistant infections. Unconventional antibiofilm agents with demonstrated antimicrobial activity could represent important alternatives for drug development to counteract infections due to major biofilm-forming pathogens.
In the last decades, the natural compounds have attracted the attention of scientists (Cowan, 1999). Since plant extracts have been used for the treatment of various infectious diseases for hundreds of years, they have sparked considerable interest in this context (Ahmad and Beg, 2001). Technological advances in modern science have accelerated the discovery of new phytocompounds of plant origin with therapeutic activity and without side effects (Ahmad and Aqil, 2009). The role of several phytocompounds, like polyphenols, as anti-infective agents is well-established today (Gibbons, 2005; Savoia, 2012). Among constituents of plants, polyphenols have received great scientific interest due to their numerous biological functions, such as promising activity against bacterial and fungal infections (Slobodníková et al., 2016). However, research regarding the antimicrobial effects of active plant-derived constituents against resistant biofilms appears still incomplete, compared to the demonstrated effects against their planktonic counterparts (Costerton et al., 2003).
Among the extensively studied natural sources rich in phytocompounds, especially polyphenols, Myrtus communis L. and Punica granatum L. could represent safe and economical alternatives to antibiotics, in the struggle against resistant infections caused by biofilm-related microorganisms. The antibacterial and antifungal effects of myrtle extracts (M. communis L.) were the object of recent scientific investigations. Crude extracts and essential oils of M. communis seem to be rich in polyphenols and terpenoids (Ben Hsouna et al., 2014), natural constituents of several plant portions that mediate a remarkable antioxidant activity along with strong antimicrobial effects against several pathogens (Aleksic and Knezevic, 2014). A wide plethora of scientific studies have also shown the significant properties of peel and juice pomegranate (P. granatum L.) extracts, in particular their anti-inflammatory and antimicrobial effects derived from the high content of polyphenols, mainly including ellagitannins and anthocyanins (Ismail et al., 2012; Pagliarulo et al., 2016; Ferrazzano et al., 2017). Most of the studies have investigated the antimicrobial activity of natural extracts against planktonic forms. In comparison, the experimental evidences of their antibiofilm effects are still few. Furthermore, the current antibiotic therapies showed very limited effectiveness to contrast the biofilm infection (Aparna and Yadav, 2008). Given the requirement to discover novel agents, preferably natural, with antibiofilm activity, as well as activity against oral biofilms, the aim of this study has been to define the antibiofilm profile of characterized polyphenolic extracts derived from myrtle leaf and pomegranate peel against S. mutans, S. oralis, S. mitis, and R. dentocariosa clinical isolates, important representative members of the dental plaque. Dental plaque bacteria are among the main microorganisms causing biofilm-related infections (from dental caries to systemic diseases). In particular, several studies have revealed that S. mutans represents about the 20–40% of the cultivable flora in biofilms removed from carious lesion (Rosenbloom and Tinanoff, 1991; Koo et al., 2013) and that S. oralis is a cariogenic bacterium significantly concurring in dental plaque formation (Jung et al., 2016). Recent reports also suggest that S. mitis and R. dentocariosa are common inhabitants of biofilms in the oral cavity that could be opportunistic pathogens, causing dental caries and periodontal pathologies (Peros et al., 2011; Banas et al., 2016). These reasons led us to consider the selected pathogenic bacterial strains as good candidates for the study of in vitro biofilm models useful in identifying new antibiofilm natural agents. The development of new knowledge about biofilm interferers/inhibitors could highlight novel, effective, and low-cost antibiofilm compounds that may find useful medical and environmental applications in the future.
Materials and Methods
Polyphenolic Extracts
The hydroethanolic extracts tested in this study were prepared with a solid–liquid solvent-extraction method from samples of dry myrtle leaf and dry pomegranate fruit peel, harvested from plants growing in the southern Italy countryside in the Salerno and Avellino areas, respectively. In particular, the preparation procedure of myrtle (M. communis L.) polyphenolic extract is described by Sateriale et al. (2020). Briefly, the myrtle leaf powder was mixed with a solution of ethanol:water (50:50), reaching a final concentration of 0.1 g ml–1. The suspension was stirred at room temperature (RT) for 30 min, using a rotary shaker, and centrifuged at 10,000 rpm for 15 min at RT. Then the supernatant was filtered through a single-use vacuum filtration unit (Sterilcup®/Steriltop® Filtration System, Merck-Millipore, Darmstadt, Germany) with a 0.45 μm porosity membrane, by using a water vacuum pump. The polyphenolic extract of P. granatum L. fruit peel was prepared according to Pagliarulo et al. (2016), with some minor changes. Pomegranate peel powder (5 g) was homogenized in 25 ml of ethanol:water (50:50) solution for 30 min at RT in the dark. After centrifugation (10,000 rpm for 15 min at RT), the supernatant was filtered by the vacuum filtration systems (0.45 μm porosity membrane) described for myrtle extracts.
Extract volume was reduced by a rotavapor (Heidolph 36001270 Hei-VAP Precision Rotary Evaporator) and finally lyophilized. The resuspension of lyophilized polyphenols in fresh solvent was carried out before each antimicrobial test, adjusting the concentration according to the requirements of the performed assays.
Bacterial Isolates and Growth Conditions
The antibiofilm activity of polyphenolic extracts was assessed against the S. mutans ATCC 25175 (LGC Standards, United Kingdom) strain, isolated from carious dentin, and against the clinical isolates of S. oralis SO1, S. mitis SM2, and R. dentocariosa RD1, obtained from samples of dental plaque from children with tooth decay, provided by the Pediatric Dentistry Department of “Federico II” University, Naples, Italy. Permission to take dental plaque samples was acquired according to the local planning authorities. Furthermore, approval for this study was granted by the ethics committee of the “Federico II” University, Naples, Italy (protocol number 101/14). The isolation of bacterial strains was carried out by culture techniques. The use of selective growth media allowed us to isolate representative colonies from dental plaque samples. The detailed identification of isolates was subsequently performed by mass spectrometry using the matrix-assisted laser desorption/ionization (MALDI) mass spectrometer (Bruker Daltonics, MALDI Biotyper, Fremont, CA, United States), a high-throughput proteomic technique for identification of a variety of bacterial species (Neville et al., 2011; Sogawa et al., 2011), and by a biochemical phenotyping method in a BD Phoenix Automated Microbiology System (Becton Dickinson, BD Franklin Lakes, NJ, United States), according to the manufacturer’s instruction.
Oral bacterial isolates were aerobically cultured at 37°C in brain heart infusion (BHI) agar/broth (Condalab, Torrejón de Ardoz, Madrid, Spain) and Columbia CNA agar base with 5% sheep blood and with colistin and nalidixic acid (Condalab, Torrejón de Ardoz, Madrid, Spain).
Bacterial isolates were kept in agar media at 4°C and were stored frozen at −80°C in BHI broth with 10% glycerol (v/v) (Carlo Erba, Reagents, Milan, Italy). Working cultures were activated in broth medium at 37°C for 15–18 h before use.
Biofilm in vitro Reproduction
Tube Method
A qualitative and semiquantitative evaluation of biofilm formation was carried out, adapting the “tube method” (TM) described by Christensen et al. (1985) and Ansari et al. (2014). In brief, the bacterial isolates were subcultured in 5 ml of BHI broth tubes, by adjusting turbidity to an optical density (OD) of 0.5 at A600nm, and aerobically incubated for 48 h at 37°C. Subsequently, the medium was discarded, and the tubes were washed with phosphate buffer saline (1xPBS, pH 7.3) and dried. The bacterial cells adherent to the tubes were then stained with crystal violet (2%) for 1 min. After removal of the stain excess, tubes were washed with deionized water, dried in an inverted position, and observed for biofilm formation. The isolates were considered as positive when a visible biofilm lined the wall and bottom of the tube, while a simple ring formation at the liquid interface was not indicative of biofilm formation. Based on the careful observation by the operator, the microbial isolates have been classified as negative (0), when no visible biofilm was observed; weakly positive (+), when a visible biofilm was observed; moderately positive (+ +), when a twice darker visible biofilm was observed with respect to weakly positive cases; and strongly positive (+ + +), when a three times darker visible biofilm was observed with respect to weakly positive cases. Each microorganism suspension at 0.5 OD600 nm was inoculated in a ratio of 1:1:1:1 in mixed culture to form a multispecies biofilm.
Tissue Culture Plate Method
The biofilm biomass measurement of selected oral isolates was evaluated by the quantitative assay known as “tissue culture plate method” (TCPM), similar to that described by Costa et al. (2013). BHI broth (10 ml) was inoculated with test microorganisms from overnight cultures at 37°C for 24 h. After aerobic incubation, each fresh culture was further adjusted by reaching the OD at 0.5, by spectrophotometric reading at A600 nm, and aliquots of 0.2 ml of starter culture were dispensed, in six replicates, into each well of a 96-well flat-bottomed microplate for tissue cultures in hydrophilic polystyrene (NuncTM MicroWell 96-well microplates, Thermo Scientific, Denmark). Each microorganism suspension at 0.5 OD600 nm was inoculated in a ratio of 1:4 with others to form a multispecies biofilm. Sterile BHI broth was used as negative control. The culture plates were incubated at 37°C for 3–6–9–12–18–24–36–48 h, without shaking. Different plates were used for different incubation times. For incubation times longer than 3 h, non-adherent bacteria were removed at regular intervals (T3–T6–T9–T12–T18–T24–T36–T48), new fresh BHI broth medium was added, and plates were further incubated for the remaining time, according to the established total incubation period. After each incubation, the bacterial culture was removed, and the wells were washed with 0.2 ml of 1xPBS (pH 7.3) three times to remove free-floating bacteria. The biofilms adherent to the wells were fixed with 0.2 ml of 85% ethanol (Sigma-Aldrich, Merck KGaA, Darmstadt, Germany) for 15 min and stained with 0.2% crystal violet (Sigma-Aldrich, Merck KGaA, Darmstadt, Germany) for 5 min. The stain excess was washed with deionized water, and plates were dried in a thermostat at a temperature of 30°C for 10 min upside down. The OD of the purple-stained solution was recorded at 600 nm wavelength by a microplate reader (Bio-Rad microplate reader, Model 680). Based on the measurement of OD (ODI) compared to the OD of sterile BHI broth used as negative control (ODC), the microbial isolates have been classified as non-adherent (ODI ≤ ODC), weakly adherent (ODC < ODI ≤ 2∗ODC), moderately adherent (2∗ODC < ODI ≤ 4∗ODC), and strongly adherent (4∗ODC < ODI).
In vitro Antibiofilm Assays With Polyphenolic Extracts
Biofilm Formation Inhibition Assay
To evaluate the ability of the myrtle and pomegranate hydroethanolic polyphenolic extracts to inhibit biofilm formation, some modifications to the tissue culture plate method have been proposed. In particular, a volume of 0.1 ml of extracts, at sub-MIC (1/4 MIC and 1/2 MIC), MIC, and over-MIC (2 MIC and 4 MIC) concentrations, was added to each well of a 96-well microplate. The same volume of BHI broth was added to replace the extracts in the negative control. Finally, 0.1 ml of each single and mixed bacterial culture, with 0.5 OD at A600 nm wavelength, was pipetted to each well, reaching a final volume of 0.2 ml. BHI (0.2 ml) broth was added in wells without bacterial culture as a blank. The plates were wrapped loosely and aerobically incubated at 37°C for 24 h without shaking to allow the cells to attach to the surface. After the incubation, the content of each well was removed, and all the subsequent steps of the above-mentioned TCPM have been performed. After staining with crystal violet, reading at A600 nm by a microplate reader was performed. Results were given as a percentage of biofilm formation inhibition, applying the following formula, according to Bakkiyaraj et al. (2013):
where ODcontrol is the mean OD measured for bacterial biofilms grown without extracts, while ODassay is the mean OD measured for bacterial biofilms grown in the presence of myrtle and pomegranate hydroalcoholic polyphenolic extracts, as single agents and in binary combination.
The lowest concentration of each extract, or extract binary combination, that produced biofilm inhibition was considered to be the minimum biofilm inhibition concentration (MBIC).
Biofilm Eradication Assay
To assess the effects of myrtle and pomegranate hydroethanolic polyphenolic extracts upon mature 1-day biofilms, some adaptations of the TCPM biofilm formation protocol were performed. In particular, aliquots of 0.2 ml of single/mixed starter bacterial culture (with adjusted OD at 0.5 OD600 nm) were dispensed, in six replicates, into each well of a 96-well flat-bottomed microplate. BHI broth (0.2 ml) was added into wells without bacterial culture as a blank. The plates were wrapped loosely and aerobically incubated at 37°C for 24 h without shaking, to allow the cells to attach to the surface. Following incubation, the content of each well was removed, and a volume of 0.1 ml of extracts was added, at MBIC and over-MBIC (2 MBIC, 3 MBIC, and 4 MBIC) concentrations. The same volume of BHI broth was added as a negative control. Finally, 0.1 ml of fresh BHI broth was added to each well, reaching a final volume of 0.2 ml. Fresh BHI broth (0.2 ml) was added in wells without bacteria culture as a blank. The plates were again aerobically incubated at 37°C for 24 h. After the second incubation, the washings with PBS, the staining with 0.2% crystal violet, and the final washing with distilled water were performed before reading the plates at A600 nm wavelength. Results for this test were given as a percentage of biofilm disruption, calculated by the following formula, according to Bakkiyaraj et al. (2013):
where ODcontrol is the mean OD measured for 1-day bacterial biofilms without extracts, while ODassay is the mean OD measured for 1-day bacterial biofilms treated with myrtle and pomegranate hydroalcoholic polyphenolic extracts, as single agents and in binary combination.
The lowest concentration of each extract, or binary combination of extracts, that is able to destroy (eradicate) preformed biofilms was considered to be the minimum biofilm eradication concentration (MBEC).
Biofilm Visualization Through Microscopy Techniques
Light microscopy analysis
The ability of myrtle and pomegranate polyphenolic hydroalcoholic extracts (50% v/v) in binary combination to inhibit biofilm formation has been confirmed by a microscopic technique similar to that described by Chaieb et al. (2011), with minor modifications. Briefly, the biofilm of each separate bacterial strain and also that of the multispecies biofilm formed by S. mutans, S. oralis, S. mitis, and R. dentocariosa were grown on glass cover slides (1 cm2) placed in 24-well polystyrene plates (NuncTM MicroWell 96-well microplates, Thermo Fisher Scientific, Denmark). In particular, aliquots of 0.2 ml of single/mixed starter bacterial culture (with an adjusted OD at 0.5 OD600nm) were dispensed on the cover glass, and then the wells were filled with different concentrations of extracts (0, 20, 40 μg μl–1), reaching a final volume of 0.4 ml in each well, before aerobic incubation at 37°C for 24 h. For negative control, bacterial cultures without extracts were used. After incubation, wells were emptied, washed with PBS, fixed with 90% ethanol for 15 min, and completely dried at 30°C. Then biofilms were stained with 1% crystal violet (Sigma-Aldrich, Merck KGaA, Darmstadt, Germany) for 20 min at RT. The excess dye was washed with distilled water. Finally, dried stained glass pieces were placed on slides and were observed with a trinocular light microscope (Motic B1 Series, Model B1-223 A) at 40X magnification with 40X/0.65/S (WD 0.53 mm) objective.
Fluorescence microscopy analysis
Biofilm architecture, in the absence and in the presence of myrtle and pomegranate hydroalcoholic extracts in binary combination, was evaluated by fluorescence microscopy analysis. The performed method, adapted from Singh et al. (2012), consisted in assessing single and polymicrobial biofilms on glass coverslips (1 cm2) immersed in bacterial culture (0.5 OD600 nm) in 24-well plates. In particular, aliquots of 0.2 ml of single/mixed starter bacterial cultures were dispensed on the cover glass, and then the wells were filled with different concentrations of extracts (0, 20, and 40 μg μl–1), reaching a final volume of 0.4 ml in each well, before. Bacterial cultures without extracts were used as negative control. After aerobic incubation at 37°C for 24 h, mature-biofilm wells were emptied, washed with PBS, fixed with 90% ethanol for 15 min, and completely dried at 30°C. Then biofilms were stained with 1 mM propidium iodide (PI; product code 81845, Sigma-Aldrich, Merck KGaA, Darmstadt, Germany) for 15 min at RT. The excess of dye was washed with distilled water. Finally, biofilms were observed with a fluorescent microscope Nikon Eclipse Ti-S (Nikon, Florence, Italy) equipped with a digital camera DS-Qi2 (Nikon, Florence, Italy) and the acquisition and image analysis software NIS-Elements C (Nikon, Florence, Italy). Digital images were acquired using the 40X objective. PI (excitation wavelength, 543 nm; fluorescence emission wavelength, 617 nm) emits red fluorescence. All fluorescence images were analyzed by Fiji ImageJ software (National Institutes of Health, Bethesda, MD) to obtain the mean fluorescence intensities from biofilms.
Statistical Data Analysis
All experiments were performed in triplicate, with independent microbial cultures for antimicrobial assays. The results obtained were analyzed and graphically reported by using GraphPad Prism 6 software, validating the statistical significance by the one-way ANOVA test with Tukey correction and the two-way ANOVA test with Bonferroni and Dunnett corrections. In all cases, p < 0.05 were considered statistically significant.
Results
Biofilm Production by S. mutans ATCC 25175, S. oralis SO1, S. mitis SM2, and R. dentocariosa RD1 Oral Isolates
In this study, S. mutans ATCC 25175, S. oralis SO1, S. mitis SM2, and R. dentocariosa RD1 biofilm-forming potential was qualitatively and quantitatively analyzed using the TM and the TCPM. The assigned adherence levels are shown in Table 1. A multispecies biofilm with the mentioned oral isolates was also set up and screened. To quantify the differences between the biofilm-forming ability of oral isolates over time, biofilm formation was monitored using crystal violet staining with regular intervals. Figure 1 shows the microplate measurements generated after static incubations at 37°C from 3 to 48 h (T3–T6–T9–T12–T18–T24–T36–T48).

Table 1. Biofilm adherence levels of S. mutans ATCC 25175, S. oralis SO1, S. mitis SM2, and R. dentocariosa RD1 oral isolates, in pure and mixed cultures.
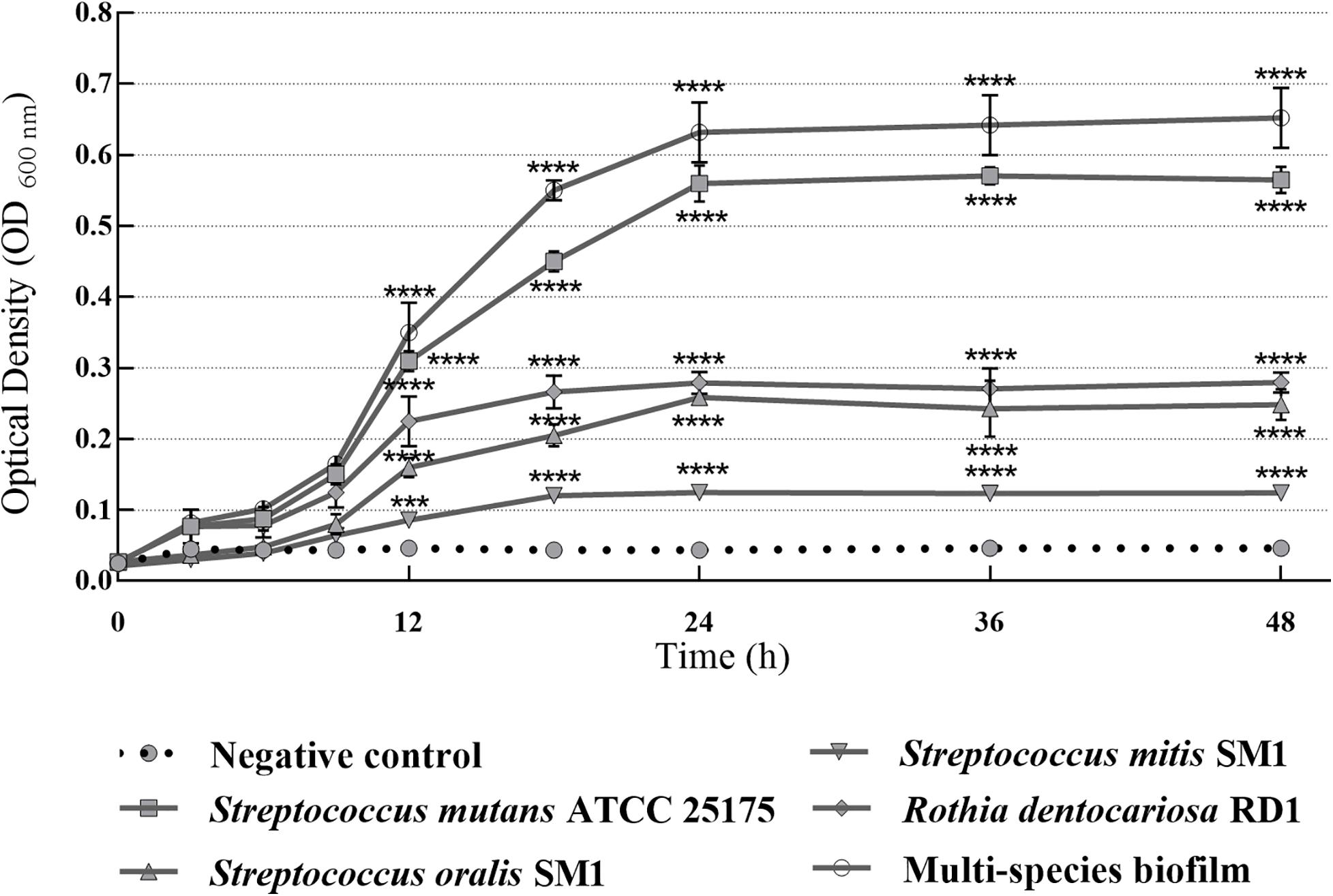
Figure 1. Absorbance values of crystal violet assay with single-species and multispecies biofilms (1:1:1:1 ratio for each isolate) produced by S. mutans ATCC 25175, S. oralis SO1, S. mitis SM2 and R. dentocariosa RD1 oral isolates, plotted over time. OD600 readings were taken after 3, 6, 9, 12, 18, 24, 36, and 48 h. The experiments were performed in triplicate with independent cultures, and statistical significance was examined by the two-way ANOVA test with Bonferroni correction. Results are indicated as means ± SDs. Asterisks indicate statistical significance (***p < 0.001; ****p < 0.0001).
In vitro Antibiofilm Activity of Myrtle Leaf and Pomegranate Peel Polyphenolic Extracts, as Single Agents and in Binary Combination, Against S. mutans ATCC 25175, S. oralis SO1, S. mitis SM2, and R. dentocariosa RD1 Oral Isolates
The antibiofilm activity of polyphenolic hydroethanolic extracts of myrtle leaf (MLE) and pomegranate peel (PPE), also in binary combination (MLE + PPE, 1:1 ratio), was measured against S. mutans ATCC 25175, S. oralis SO1, S. mitis SM2, and R. dentocariosa RD1 oral isolates at several concentrations, including sub-MIC (1/4 MIC and 1/2 MIC), MIC, and over-MIC (2 MIC and 4 MIC) concentrations, in order to assess the impact of the natural extracts upon biofilm formation of dental plaque pathogens involved in caries disease etiology.
MBIC values, which ranged between 10 μg μl–1 (MLE + PPE vs. S. oralis SO1 and S. mitis SM2) and 40 μg μl–1 (MLE vs. S. mutans ATCC 25175 and R. dentocariosa RD1, together with PPE vs. S. mutans ATCC 25175, S. mitis SM2, and R. dentocariosa RD1) (Table 2), were assigned to the lowest concentrations of antimicrobial agents required to inhibit the formation of biofilms, while MBEC values, assigned to the lowest concentration of each antimicrobial agent that is able to destroy (eradicate) preformed biofilms, ranged between 40 μg μl–1 (MLE + PPE vs. S. mutans ATCC 25175, S. oralis SO1, and S. mitis SM2) and 120 μg μl–1 (MLE vs. S. mutans ATCC 25175 and R. dentocariosa RD1, together with PPE vs. S. mutans ATCC 25175, S. mitis SM2, and R. dentocariosa RD1) (Table 2).

Table 2. Quantitative evaluation of effects of myrtle and pomegranate polyphenolic extracts, used individually and in binary combinations, against S. mutans ATCC 25175, S. oralis SO1, S. mitis SM2, and R. dentocariosa RD1 biofilms.
The ability of the MLE, PPE, and MLE + PPE binary combination to disrupt preformed biofilms of S. mutans ATCC 25175, S. oralis SO1, S. mitis SM2, and R. dentocariosa RD1 was tested at MBIC and over-MBIC concentrations (2 MBIC, 3 MBIC, and 4 MBIC).
Biofilm inhibition and eradication activity of myrtle and pomegranate polyphenolic extracts, as single agents and in binary combination, on single-species and multispecies mature biofilms of S. mutans ATCC 25175, S. oralis SO1, S. mitis SM2, and R. dentocariosa RD1 oral isolates are reported in Figures 2, 3, respectively.
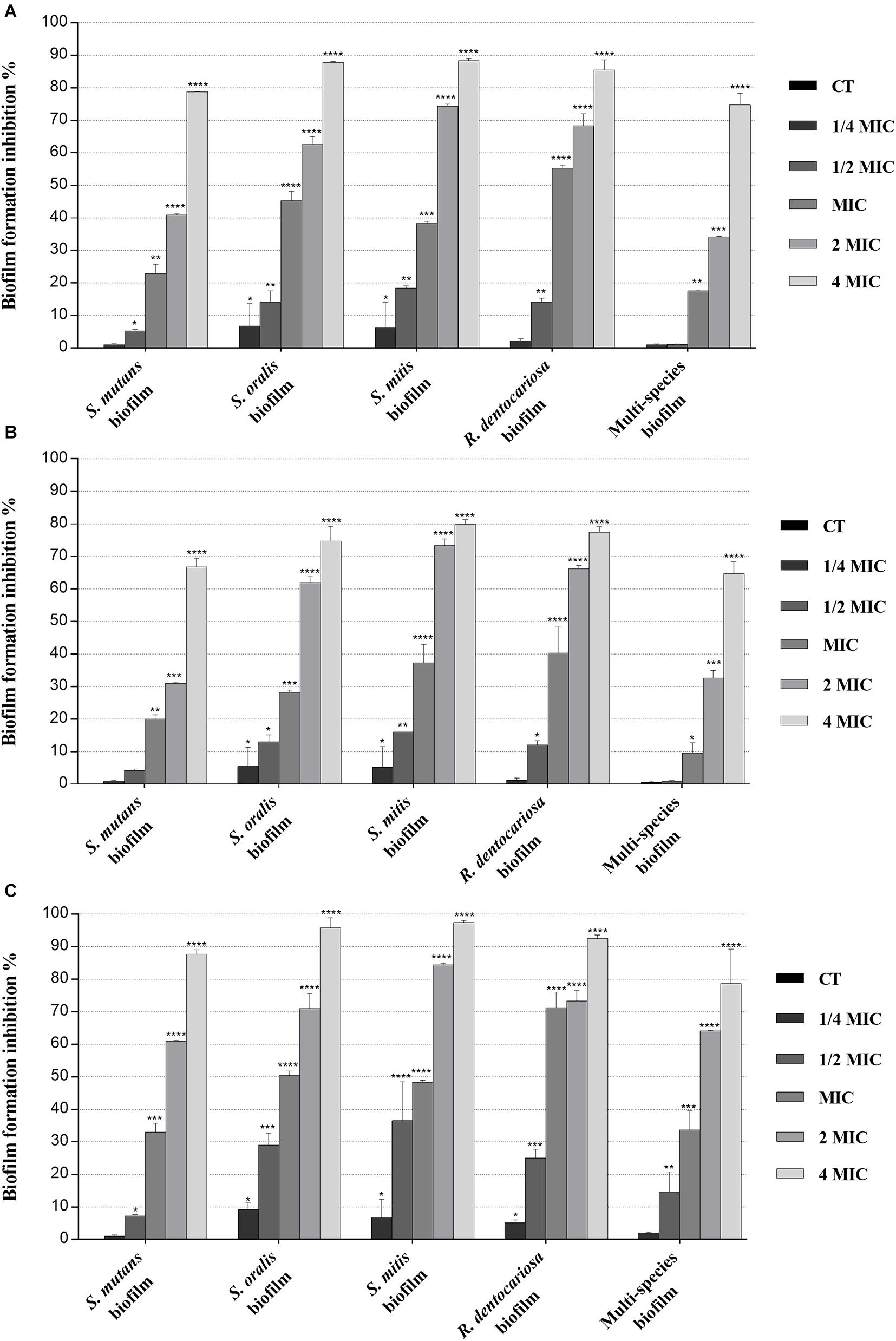
Figure 2. Inhibitory effect of myrtle and pomegranate polyphenolic extracts, used individually and in binary combinations, on single-species and multispecies biofilm formation by S. mutans ATCC 25175, S. oralis SO1, S. mitis SM2, and R. dentocariosa RD1 oral isolates. Figure shows the inhibition percentage values of S. mutans ATCC 25175, S. oralis SO1, S. mitis SM2, and R. dentocariosa RD1 in single-species and multispecies biofilm (1:1:1:1 ratio for each isolate) detected, in the presence of sub-MIC (1/4 MIC and 1/2 MIC), MIC, and over-MIC (2 and 4 MIC) concentrations of myrtle leaf 50% hydroethanolic extract (MLE) (A); pomegranate peel 50% hydroethanolic extract (PPE) (B); myrtle leaf 50% hydroethanolic extract in combination with pomegranate peel 50% hydroethanolic extract (MLE + PPE) added in a 1:1 ratio (C). The experiments were performed in triplicate with independent cultures, and statistical significance was examined by the two-way ANOVA test with Dunnett correction. Results are indicated as means ± SDs. Asterisks indicate statistical significance (*p < 0.05; **p < 0.01;***p < 0.001; ****p < 0.0001).
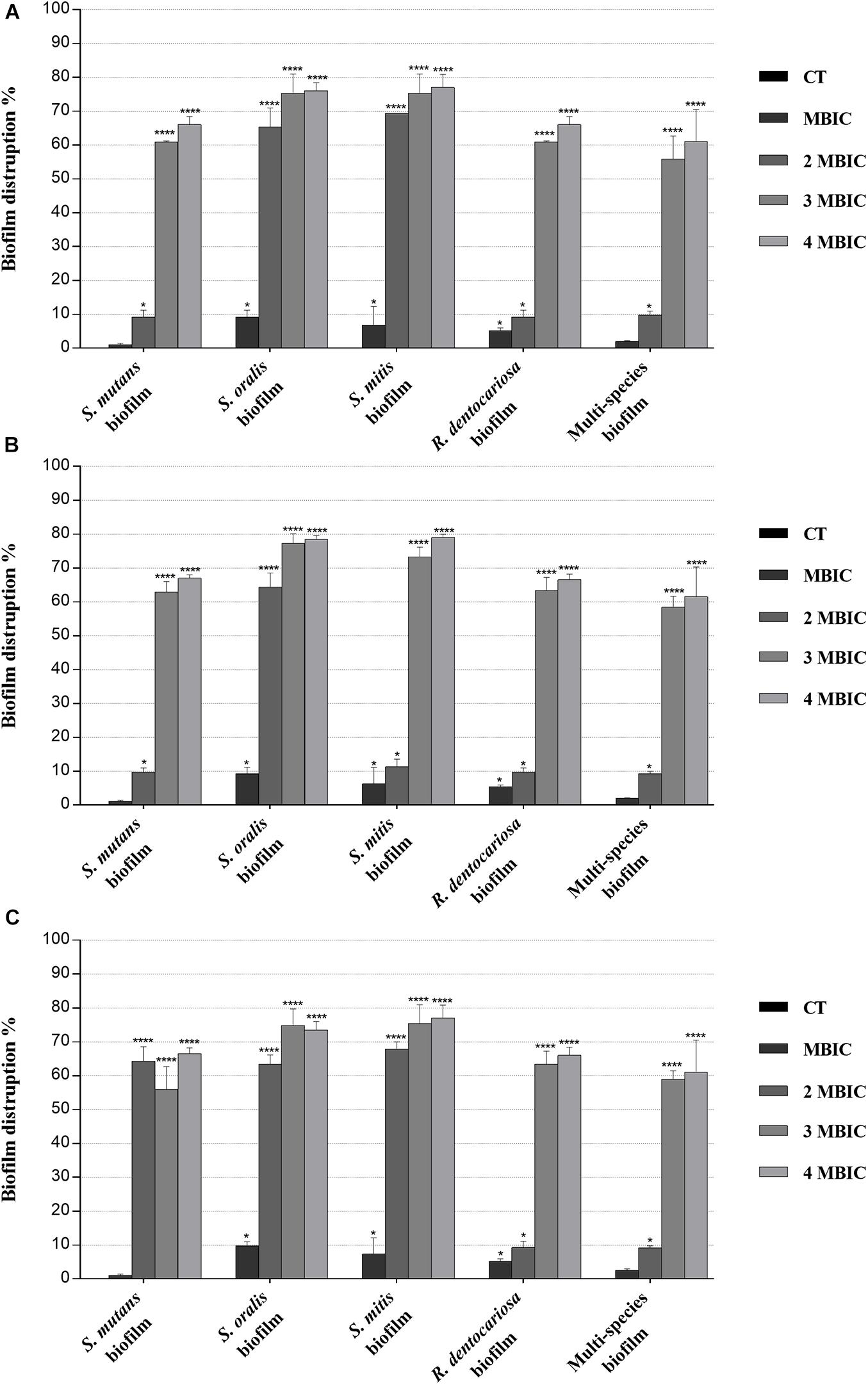
Figure 3. Biofilm eradication activity of myrtle and pomegranate polyphenolic extracts, also in binary combination, on single-species and multispecies mature biofilms of S. mutans ATCC 25175, S. oralis SO1, S. mitis SM2, and R. dentocariosa RD1 oral isolates. Figure shows the disruption percentage values of S. mutans ATCC 25175, S. oralis SO1, S. mitis SM2, and R. dentocariosa RD1 oral isolates in single and multispecies biofilms (1:1:1:1 ratio for each isolate) detected in the presence of MBIC and over-MBIC (2 MBIC, 3 MBIC, and 4 MBIC) concentrations of myrtle leaf 50% hydroethanolic extract (MLE) (A); pomegranate peel 50% hydroethanolic extract (PPE) (B); myrtle leaf 50% hydroethanolic extract in combination with pomegranate peel 50% hydroethanolic extract (MLE + PPE) added in a 1:1 ratio (C). The experiments were performed in triplicate with independent cultures, and statistical significance was examined by the two-way ANOVA test with Dunnett correction. Results are indicated as means ± SDs. Asterisks indicate statistical significance (*p < 0.05; ****p < 0.0001).
Regarding the effect on biofilm formation, the hydroethanolic (50% v/v) polyphenolic extracts of M. communis L. leaf and P. granatum L. fruit peel produced a significant (p < 0.05) inhibition of S. mutans ATCC 25175, S. oralis SO1, S. mitis SM2, and R. dentocariosa RD1 biomass with respect to untreated control, as shown in Figure 2. In detail, both myrtle and pomegranate extracts, also in binary combination, at sub-MIC concentrations caused a slight or not significant decrease for all oral isolates, ranging from 1.2% (PPE vs. four-species biofilm) to 35.7% (MLE + PPE vs. S. mitis biofilm) with respect to the control (Figure 2), while both produced a significant (p < 0.05) inhibition of biofilm formation at MIC and over-MIC concentrations, with inhibition percentages reaching up to 97.3% for MLE + PPE binary combination against the S. mitis biofilm (Figure 2). The ability of the MLE, PPE, and MLE + PPE binary combination to disrupt preformed biofilms of S. mutans ATCC 25175, S. oralis SO1, S. mitis SM2, and R. dentocariosa RD1 was tested at MBIC and over-MBIC concentrations (2 MBIC, 3 MBIC, and 4 MBIC). It was found that preformed biofilms of all tested oral isolates were significantly disrupted at over-MBIC concentrations (Figure 3).
Detection of S. mutans ATCC 25175, S. oralis SO1, S. mitis SM2, R. dentocariosa RD1, and Multispecies Biofilm Architecture in the Absence and in the Presence of Myrtle Leaf and Pomegranate Peel Polyphenolic Extracts
Microscopy techniques allowed us to visually confirm the effects of the polyphenolic extracts against single-species and multispecies biofilms produced by oral pathogens.
In particular, light microscopy images (Figure 4) show the morphology of the mature biofilms (24 h) of S. mutans ATCC 25175, S. oralis SO1, S. mitis SM2, and R. dentocariosa RD1, in single species and multispecies, grown in the absence and in the presence of the binary combination of myrtle leaf 50% ethanolic extracts and pomegranate peel 50% ethanolic extracts (MLE + PPE). The images of single-species biofilm treated with MLE + PPE at increasing concentrations (Figures 4A2,A3,B2,B3,C2,C3,D2,D3) show a gradual reduction in biofilm mass at 20 and 40 μg μl–1 extract concentrations, compared to their respective untreated controls (Figures 4A1,B1,C1,D1). The extracts also demonstrated the ability to inhibit the multispecies biofilm formation with respect to the unexposed control (Figures 4E1–E3).
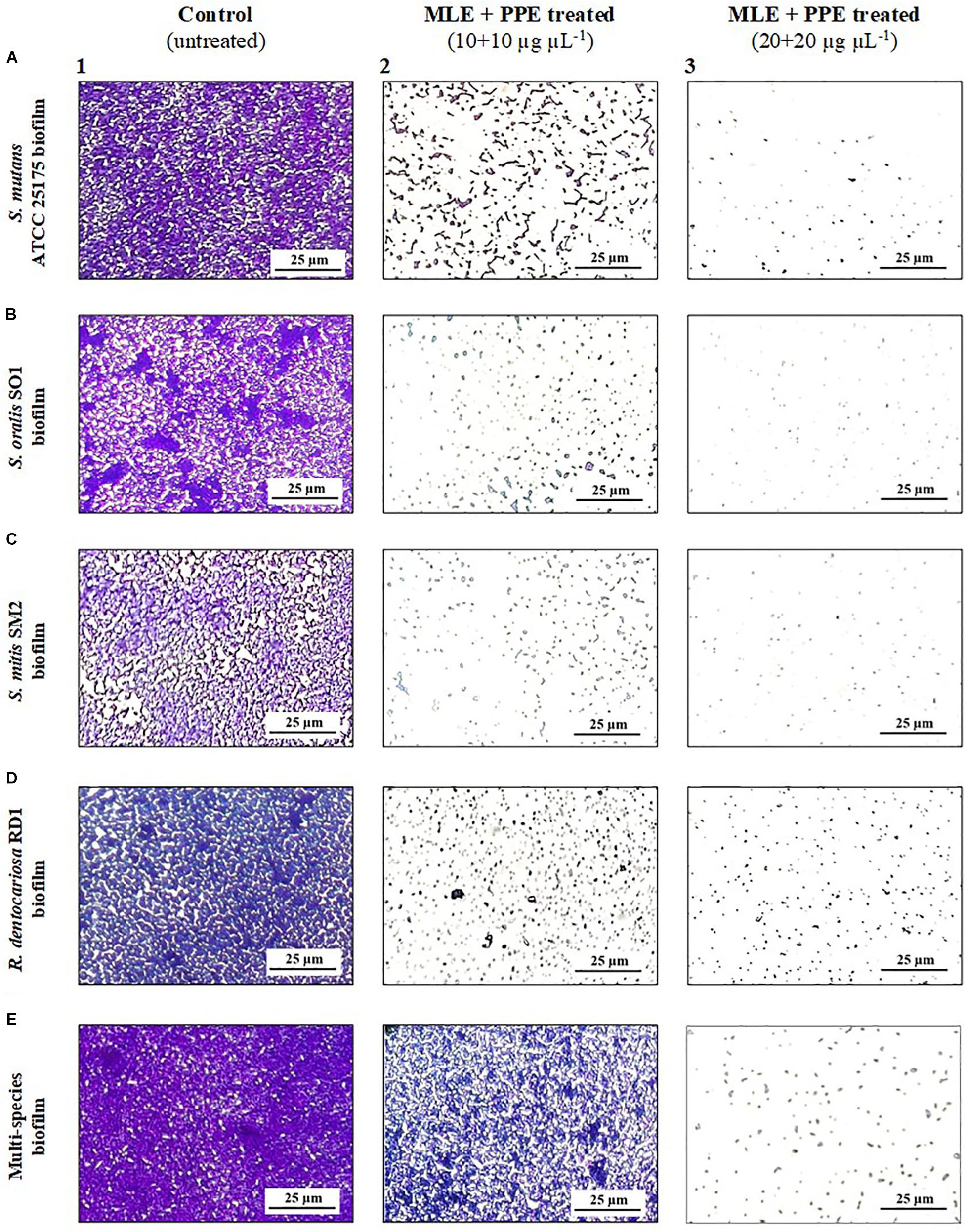
Figure 4. Light microscopy images of mature S. mutans ATCC 25175 (A), S. oralis SO1 (B), S. mitis SM2 (C), R. dentocariosa RD1 (D), and multispecies (E) biofilms (1:1:1:1 ratio for each isolate) grown with and without treatment with myrtle extract in binary combination with pomegranate extract. Crystal violet staining; MLE + PPE, myrtle leaf 50% ethanolic extracts in binary combination with pomegranate peel 50% ethanolic extracts (1:1 ratio).
The effects of the binary combination of MLE and PPE on the architecture of biofilms formed by S. mutans, S. oralis, S. mitis, and R. dentocariosa, in single and mixed cultures, were analyzed also by fluorescence microscopy with PI-based fluorescent staining, as shown in Figure 5.
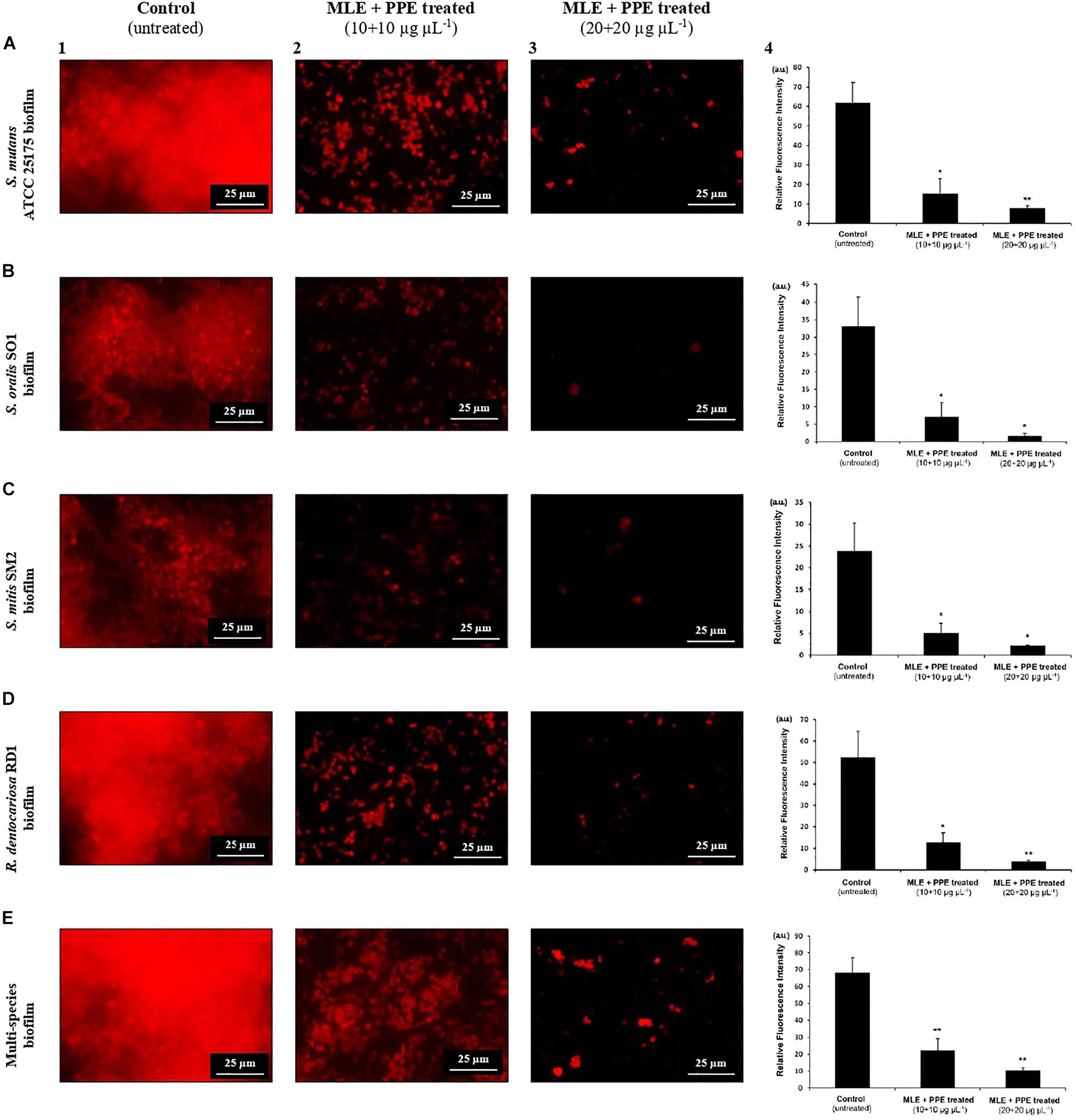
Figure 5. Fluorescence microscopy images of mature S. mutans ATCC 25175 (A), S. oralis SO1 (B), S. mitis SM2 (C), R. dentocariosa RD1 (D), and multispecies (E) biofilms (1:1:1:1 ratio for each isolate) grown with and without treatment with myrtle extract in binary combination with pomegranate extract. PI staining; MLE + PPE, myrtle leaf 50% ethanolic extract in binary combination with pomegranate peel 50% ethanolic extract (1:1 ratio). Relative fluorescence intensity of biofilm architecture in the S. mutans ATCC 25175 (A4), S. oralis SO1 (B4), S. mitis SM2 (C4), R. dentocariosa RD1 (D4) and multispecies (E4) biofilms is reported in arbitrary unit (a.u.). Data are expressed as mean ± SE. **p < 0.001*p < 0.05 compared to the control group.
In fluorescence microscopy images, we can observe a large fraction of red PI-stained areas in single-species and multispecies biofilms on untreated glass (Figures 5A1,B1,C1,D1,E1), while the fractions of red-stained areas tended to decrease progressively on treated surfaces with the extracts at increasing concentrations with respect to untreated controls (Figures 5A2,A3,B2,B3,C2,C3,D2,D3,E2,E3). PI is a fluorescent intercalating agent widely used to stain microbial cells, also encapsulated in a biofilm matrix, and nucleic acids, including extracellular DNA (eDNA), which is one of the most abundant components of the exopolysaccharide matrix (Whitchurch et al., 2002). To confirm the decrease of red PI-stained areas in single-species and multispecies biofilms on the surfaces treated with the extracts, a quantitative analysis of the relative fluorescence intensity was performed. In particular, S. mutans single culture showed a significant decrease of relative fluorescence intensity in the presence of myrtle leaf and pomegranate peel polyphenolic extracts with respect to the control [control: 61.78 ± 10.57 vs. MLE + PPE (10 + 10 μg μl–1): 15.44 ± 7.62, p < 0.05; and control: 61.78 ± 10.57 vs. MLE + PPE (20 + 20 μg μl–1): 7.93 ± 1.12, p < 0.001] (Figure 5A4). Similar results were obtained for S. oralis [control: 33.02 ± 8.2 vs. MLE + PPE (10 + 10 μg μl–1): 7.01 ± 4.16, p < 0.05; and control: 33.02 ± 8.2 vs. MLE + PPE (20 + 20 μg μl–1): 1.68 ± 1.19, p < 0.05] (Figure 5B4); S. mitis [control: 23.78 ± 6.45 vs. MLE + PPE (10 + 10 μg μl–1): 5.06 ± 2.24, p < 0.05; and control: 23.78 ± 6.45 vs. MLE + PPE (20 + 20 μg μl–11): 2.18 ± 0.17, p < 0.05] (Figure 5C4); R. dentocariosa [control: 52.33 ± 12.13 vs. MLE + PPE (10 + 10 μg μl–1): 12.49 ± 4.75, p < 0.05; and control: 52.33 ± 12.13 vs. MLE + PPE (20 + 20 μg μl–1): 3.9 ± 0.48, p < 0.001] (Figure 5D4); and also the mixture [control: 68.2 ± 8.71 vs. MLE + PPE (10 + 100 μg μl–1): 22.26 ± 6.92, p < 0.001; and control: 68.2 ± 8.71 vs. MLE + PPE (20 + 20 μg μl–1): 19.25 ± 1.58, p < 0.001] (Figure 5E4).
In addition, the morphology of biofilms on treated glass appeared altered with respect to control, with more intense fluorescent signals. This suggests the presence of dead microbial cells with compromised membranes: PI can easily cross them and intercalate in greater quantity into double-stranded nucleic acids by emitting a clearer red signal (Rosenberg et al., 2019).
Discussion
In a previous work, the authors demonstrated that hydroalcoholic polyphenolic extracts of M. communis L. leaf and P. granatum L. fruit peel showed the ability to inhibit the growth and survival of cariogenic bacteria of dental plaque (Sateriale et al., 2020). Moreover, their remarkable synergistic effects, also in association with amoxicillin, have been demonstrated (Sateriale et al., 2020). The characterization of the hydroalcoholic pomegranate peel extract showed that the most abundant phenolic compounds include pedunculagin 1, punicalin, ellagic acid hexose, ellagic acid pentose, and ellagic acid deoxesose (Pagliarulo et al., 2016), while gallic acid derivatives, tannins, myricetin, and quercetin derivatives are the most abundant phenolic compounds in hydroalcoholic extracts prepared from myrtle leaf (Sateriale et al., 2020). These results allowed us to design the goals of this study and to evaluate the antibiofilm activity of the same natural extracts in order to encourage their use as alternative options to prevent and treat oral infectious diseases, including biofilm-related ones.
Since adherence on oral surfaces is the first crucial step in biofilm formation in the oral cavity (Lendenmann et al., 2000), before analyzing the antibiofilm effects of myrtle and pomegranate extracts against dental plaque bacteria, the first objective of this work was to determine the ability of S. mutans ATCC 25175, S. oralis SO1, S. mitis SM2, and R. dentocariosa RD1 oral isolates to adhere and then to form biofilms. The results obtained by TM and TCPM methods showed the ability to form biofilm both on polystyrene tube walls and on polypropylene surface of 96-well microtiter plates, for all tested oral isolates. No oral isolates were indeed found to be negative for the TM test. In particular, S. mutans strain was shown to be strongly positive (+ + +), as well as the multispecies biofilm, while S. oralis and R. dentocariosa isolates were moderately positive (+ +), and finally the S. mitis isolate was weakly positive (+) (Supplementary Table S1). These results were confirmed by the quantification of microbial biofilm biomass through the recording of the OD (Table 1) at 600 nm by a microtiter reader. Interestingly, the high OD values measured for the S. mutans single biofilm (mean OD600 nm of 0.506 ± 0.045) and for the multispecies biofilm (mean OD600 nm of 0.607 ± 0.028) allow their classification as strongly adherent microbial isolates. S. oralis and R. dentocariosa isolates were moderately adherent, while the S. mitis isolate was weakly adherent. Both TM and TCPM proved to be effective methods to assess in vitro microbial biofilm formation by oral pathogens. However, given several disadvantages related to TM, in terms of major quantity of needed material for each sample and minor number of samples to test simultaneously, TCPM was preferred for subsequent assays. Crystal violet staining is a commonly used technique to quantify biofilm formation, thanks to its relatively easy execution, its reproducibility, and its ability to rapidly analyze multiple samples simultaneously (Stepanović et al., 2007; Agarwal et al., 2011; Liu et al., 2018). Considering these advantages and the interconnection between EPS production and microbial biomass amount in biofilms (Yallop et al., 2000), this staining has been selected as an adequate method to assess biofilm formation in the absence and in the presence of the natural extracts tested in this study. The first 12 h of biofilm formation was shown to correspond to the first adhesion stage, as indicated by the observed increase of OD values. With the increase in cell proliferation and the generation of extracellular matrix, the exponential growth was reached, coming to the plateau (or stationary phase) at about 18 h of monitoring, up to 48 h. No oral isolates showed significant differences in biofilm formation amount between 24 and 48 h of growth. These results, in accordance with other studies (Servetas et al., 2016; Mira et al., 2019), allow us to confirm that 24 h are enough to observe a stable biofilm growth by S. mutans ATCC 25175, S. oralis SO1, S. mitis SM2, and R. dentocariosa RD1 oral isolates and by their multispecies biofilm.
Subsequently, the antibiofilm activity of polyphenolic extracts against S. mutans ATCC 25175, S. oralis SO1, S. mitis SM2, and R. dentocariosa RD1 oral isolates was measured. Both 50% hydroethanolic myrtle leaf polyphenolic extracts (MLE) and pomegranate peel polyphenolic extracts (PPE) were able to inhibit the formation of biofilms made by all mentioned oral isolates, as well as to disrupt preformed 1-day biofilms. Interestingly, the binary combination of myrtle and pomegranate extracts (MLE + PPE) inhibited the biofilm formation and eradicated mature biofilm at lower concentrations with respect to the extracts individually tested.
These results suggest that the antibiofilm activity of polyphenolic myrtle leaf and pomegranate peel extracts, especially in binary combination, against S. mutans ATCC 25175, S. oralis SO1, S. mitis SM2, and R. dentocariosa RD1 oral isolates is in line with other studies that demonstrated the ability of P. granatum L. fruit peel and M. communis L. leaf extracts to inhibit the biofilm formation process and to disrupt preformed biofilms (Bakkiyaraj et al., 2013; Mansouri et al., 2013). The potential of the tested natural extracts in our study to prevent microbial adhesion could be closely linked to the antibiofilm properties of the extracted polyphenolic compounds. Interestingly, flavanols and flavonoids, such as rutin from myrtle extract, exhibited strong sortase inhibitory activity, thus interfering with the aptitude of bacteria to adhere to surfaces (Kang et al., 2006). In general, among the mechanisms underlying the antibacterial activity of polyphenols are the damage to the cytoplasmic membrane and the inhibition of nucleic acids and of energy metabolism but also the inhibition of cell membrane synthesis and cell wall synthesis (Cushnie and Lamb, 2011). As for the mechanisms behind the antibiofilm effects of polyphenols, although they are still unclear, they could be attributable to multiple factors acting in concert. The adhesion process to the surface can be influenced mainly by the physicochemical properties of the surface, but also by the bacterial characteristics and by environmental factors. The alteration of the bacterial surface could interfere with the normal cell–substrate interactions and compromise the attack phase and the normal biofilm development process (Simões et al., 2007). The researches on plant polyphenol antibiofilm activity have revealed several activities leading to biofilm suppression, for example, by affecting the bacterial regulatory mechanisms, such as quorum sensing (Silva et al., 2016) or by damaging the stability of the bacterial cytoplasmic membrane after inhibition of lipid metabolism (Rozalski et al., 2013). In addition, polyphenols could exert an aggregatory effect on bacterial cells, thus determining a preferred interaction of bacterial cells between themselves rather than with the surface (Cushnie et al., 2007). This could explain the ability of polyphenolic extracts to eradicate preformed biofilms, but future studies are needed to clarify their mechanisms of action. Regarding the synergistic antibiofilm effects of myrtle and pomegranate extracts, they could depend on their different polyphenolic contents and relative mechanisms of action. In particular, recent literature data showed that flavonoids, such as quercetin of myrtle extract, are able to inhibit the activities of glucosyltransferases and F-ATPase, and the acid production by S. mutans cells, thus significantly affecting biofilm development and acidogenicity (Duarte et al., 2006), while gallic acid and tannins showed suppressive effect on S. mutans biofilm formation by inhibition of glucosyltransferase and fructosyltransferase (Sendamangalam et al., 2011). On the other hand, the mechanisms of action linked to antibiofilm activity of pomegranate polyphenolic extracts implicate both the precipitation of vital proteins involved in the formation of biofilms, such as adhesins, and the alteration of the cell surface charge thereby interfering with cell–substratum interactions and biofilm development, mediated by ellagic acid and ellagitannins, respectively (Liu et al., 2008).
In addition, to our knowledge, several studies demonstrated that pomegranate peel extracts do not exert cytotoxic effects on human fibroblast (Eid et al., 2015) and that could interfere with gingival fibroblast viability only at high concentrations (Celiksoy et al., 2020). Moreover, literature data reported that phytocompounds from M. communis L. leaves induce apoptosis only in cancer cell lines, but not in non-transformed human fibroblasts (Asgarpanah and Ariamanesh, 2015). In addition, the absence of cytotoxicity of myrtle derivatives is confirmed also by studies in humans. For example, the use myrtle paste was shown to be effective for increasing the quality of life of patients who suffer from painful oral condition, such as aphthous stomatitis, without toxic effects on mouth epithelial (Babaee et al., 2010). However, further research, including cytotoxicity assays and in vivo studies, is required to reach the practical application of these natural antimicrobials in clinical use to counteract oral biofilms.
In conclusion, this study opens toward new perspectives in using natural antimicrobial combined therapies against oral pathogens able to form biofilm. Although only some of the bacterial species forming the complex oral plaque community have been taken into consideration in this study, our data encourage the promotion of clinical trials for the development of innovative natural tools in the prophylaxis and treatment of biofilm-related oral diseases.
Data Availability Statement
The raw data supporting the conclusions of this article will be made available by the authors, without undue reservation.
Author Contributions
DS and CaP conceived the work. RI contributed to microscopy analyzes. RC, ChP, EV, MV, PS, and MP were involved in the data analysis, wrote and critically reviewed the manuscript. All authors read and approved the final manuscript.
Funding
This study was funded by the FRA 2019-2020.
Conflict of Interest
PS was employed by CEINGE.
The remaining authors declare that the research was conducted in the absence of any commercial or financial relationships that could be construed as a potential conflict of interest.
Acknowledgments
We would like to thank Prof. Gianmaria Fabrizio Ferrazzano, Department of Neuroscience, Reproductive and Oral Sciences, Section of Paediatric Dentistry, University of Naples Federico II, Naples, Italy, for kindly providing the dental plaque samples.
Supplementary Material
The Supplementary Material for this article can be found online at: https://www.frontiersin.org/articles/10.3389/fmicb.2020.592265/full#supplementary-material
References
Agarwal, R. K., Singh, S., Bhilegaonkar, K. N., and Singh, V. P. (2011). Optimization of microtitre plate assay for the testing of biofilm formation ability in different Salmonella serotypes. Int. Food Res. J. 18:1493.
Ahmad, I., and Aqil, F. (2009). New Strategies Combating Bacterial Infection. Hoboken, NJ: John Wiley & Sons.
Ahmad, I., and Beg, A. Z. (2001). Antimicrobial and phytochemical studies on 45 Indian medicinal plants against multi-drug resistant human pathogens. J. Ethnopharmacol. 74, 113–123. doi: 10.1016/S0378-8741(00)00335-4
Aleksic, V., and Knezevic, P. (2014). Antimicrobial and antioxidative activity of extracts and essential oils of Myrtus communis L. Microbiol. Res. 169, 240–254. doi: 10.1016/j.micres.2013.10.003
Ansari, M. A., Khan, H. M., Khan, A. A., Cameotra, S. S., Saquib, Q., and Musarrat, J. (2014). Gum arabic capped-silver nanoparticles inhibit biofilm formation by multi-drug resistant strains of Pseudomonas aeruginosa. J. Basic Microbiol. 54, 688–699. doi: 10.1002/jobm.201300748
Aparna, M. S., and Yadav, S. (2008). Biofilms: microbes and disease. Braz. J. Infect. Dis. 12, 526–530. doi: 10.1590/S1413-86702008000600016
Asgarpanah, J., and Ariamanesh, A. (2015). Phytochemistry and pharmacological properties of Myrtus communis L. Indian J. Traditional Knowl. 14, 82–87.
Babaee, N., Mansourian, A., Momen-Heravi, F., Moghadamnia, A., and Momen-Beitollahi, J. (2010). The efficacy of a paste containing Myrtus communis (Myrtle) in the management of recurrent aphthous stomatitis: A randomized controlled trial. Clin. Oral Investig. 14, 65–70. doi: 10.1007/s00784-009-0267-3
Bakkiyaraj, D., Nandhini, J. R., Malathy, B., and Pandian, S. K. (2013). The anti-biofilm potential of pomegranate (Punica granatum L.) extract against human bacterial and fungal pathogens. Biofouling 29, 929–937. doi: 10.1080/08927014.2013.820825
Banas, J. A., Zhu, M., Dawson, D. V., Blanchette, D. R., Drake, D. R., Gu, H., et al. (2016). Acidogenicity and acid tolerance of Streptococcus oralis and Streptococcus mitis isolated from plaque of healthy and incipient caries teeth. J. Oral Microbiol. 8:32940. doi: 10.3402/jom.v8.32940
Ben Hsouna, A., Hamdi, N., Miladi, R., and Abdelkafi, S. (2014). Myrtus communis essential oil: Chemical composition and antimicrobial activities against food spoilage pathogens. Chem. Biodivers. 11, 571–580. doi: 10.1002/cbdv.201300153
Byers, H. L., Tarelli, E., Homer, K. A., and Beighton, D. (2000). Isolation and characterisation of sialidase from a strain of Streptococcus oralis. J. Med. Microbiol. 49, 235–244. doi: 10.1099/0022-1317-49-3-235
Caraher, E., Reynolds, G., Murphy, P., McClean, S., and Callaghan, M. (2007). Comparison of antibiotic susceptibility of Burkholderia cepacia complex organisms when grown planktonically or as biofilm in vitro. Eur. J. Clin. Microbiol. Infect. Dis. 26, 213–216. doi: 10.1007/s10096-007-0256-x
Celiksoy, V., Moses, R. L., Sloan, A. J., Moseley, R., and Heard, C. M. (2020). Evaluation of the in vitro oral wound healing effects of pomegranate (Punica granatum) rind extract and punicalagin, in combination with Zn (II). Biomolecules 10:1234. doi: 10.3390/biom10091234
Chaieb, K., Kouidhi, B., Jrah, H., Mahdouani, K., and Bakhrouf, A. (2011). Antibacterial activity of Thymoquinone, an active principle of Nigella sativa and its potency to prevent bacterial biofilm formation. BMC Complement. Altern. Med. 11:29. doi: 10.1186/1472-6882-11-29
Chambless, J. D., Hunt, S. M., and Stewart, P. S. (2006). A three-dimensional computer model of four hypothetical mechanisms protecting biofilms from antimicrobials. Appl. Environ. Microbiol. 72, 2005–2013. doi: 10.1128/AEM.72.3.2005-2013.2006
Christensen, G. D., Simpson, W. A., Younger, J. J., Baddour, L. M., Barrett, F. F., Melton, D. M., et al. (1985). Adherence of coagulase-negative staphylococci to plastic tissue culture plates: A quantitative model for the adherence of staphylococci to medical devices. J. Clin. Microbiol. 22, 996–1006. doi: 10.1128/jcm.22.6.996-1006.1985
Costa, E. M., Silva, S., Tavaria, F. K., and Pintado, M. M. (2013). Study of the effects of chitosan upon Streptococcus mutans adherence and biofilm formation. Anaerobe 20, 27–31. doi: 10.1016/j.anaerobe.2013.02.002
Costerton, W., Veeh, R., Shirtliff, M., Pasmore, M., Post, C., and Ehrlich, G. (2003). The application of biofilm science to the study and control of chronic bacterial infections. J. Clin. Investig. 112, 1466–1477. doi: 10.1172/JCI200320365
Cowan, M. M. (1999). Plant products as antimicrobial agents. Clin. Microbiol. Rev. 12, 564–582. doi: 10.1128/cmr.12.4.564
Cushnie, T. P. T., and Lamb, A. J. (2011). Recent advances in understanding the antibacterial properties of flavonoids. Int. J. Antimicrob. Agents 38, 99–107. doi: 10.1016/j.ijantimicag.2011.02.014
Cushnie, T. P. T., Hamilton, V. E. S., Chapman, D. G., Taylor, P. W., and Lamb, A. J. (2007). Aggregation of Staphylococcus aureus following treatment with the antibacterial flavonol galangin. J. Appl. Microbiol. 103, 1562–1567. doi: 10.1111/j.1365-2672.2007.03393.x
Duarte, S., Gregoire, S., Singh, A. P., Vorsa, N., Schaich, K., Bowen, W. H., et al. (2006). Inhibitory effects of cranberry polyphenols on formation and acidogenicity of Streptococcus mutans biofilms. FEMS Microbiol. Lett. 257, 50–56. doi: 10.1111/j.1574-6968.2006.00147.x
Eid, H. A., Abo-Alazm, E. A., Mosleh, M. M., Alshahrani, M. A., Taha, T. H., El-Deeb, N. M., et al. (2015). Assessing the effects of different plant extracts on primary dental plaque colonizer and human fibroblast cells. Asian J. Dentistry 2:1025.
Ferrazzano, G. F., Scioscia, E., Sateriale, D., Pastore, G., Colicchio, R., Pagliuca, C., et al. (2017). In vitro antibacterial activity of pomegranate juice and peel extracts on cariogenic bacteria. BioMed Res. Int. 2017:2152749. doi: 10.1155/2017/2152749
Gibbons, S. (2005). Plants as a source of bacterial resistance modulators and anti-infective agents. Phytochem. Rev. 4, 63–78. doi: 10.1007/s11101-005-2494-9
Ismail, T., Sestili, P., and Akhtar, S. (2012). Pomegranate peel and fruit extracts: a review of potential anti-inflammatory and anti-infective effects. J. Ethnopharmacol. 143, 397–405. doi: 10.1016/j.jep.2012.07.004
Jung, J. E., Cai, J. N., Cho, S. D., Song, K. Y., and Jeon, J. G. (2016). Influence of fluoride on the bacterial composition of a dual-species biofilm composed of Streptococcus mutans and Streptococcus oralis. Biofouling 32, 1079–1087. doi: 10.1080/08927014.2016.1230607
Kang, S. S., Kim, J. G., Lee, T. H., and Oh, K. B. (2006). Flavonols inhibit sortases and sortase-mediated Staphylococcus aureus clumping to fibrinogen. Biol. Pharm. Bull. 29, 1751–1755. doi: 10.1248/bpb.29.1751
Keng, T. C., Ng, K. P., Tan, L. P., Chong, Y. B., Wong, C. M., and Lim, S. K. (2012). Rothia dentocariosa repeat and relapsing peritoneal dialysis-related peritonitis: a case report and literature review. Renal Fail. 34, 804–806. doi: 10.3109/0886022X.2012.678208
Khan, S. T., Ahamed, M., Musarrat, J., and Al-Khedhairy, A. A. (2014). Anti-biofilm and antibacterial activities of zinc oxide nanoparticles against the oral opportunistic pathogens Rothia dentocariosa and Rothia mucilaginosa. Eur. J. Oral Sci. 122, 397–403. doi: 10.1111/eos.12152
Koo, H., Falsetta, M. L., and Klein, M. I. (2013). The exopolysaccharide matrix: a virulence determinant of cariogenic biofilm. J. Dent. Res. 92, 1065–1073. doi: 10.1177/0022034513504218
Leid, J. G., Shirtliff, M. E., Costerton, J. W., and Stoodley, P. (2002). Human leukocytes adhere to, penetrate, and respond to Staphylococcus aureus biofilms. Infect. Immun. 70, 6339–6345. doi: 10.1128/IAI.70.11.6339-6345.2002
Lemos, J. A., Palmer, S. R., Zeng, L., Wen, Z. T., Kajfasz, J. K., Freires, I. A., et al. (2019). The Biology of Streptococcus mutans. Microbiol. Spect. 7:GPP3-0051-2018. doi: 10.1128/microbiolspec.gpp3-0051-2018
Lendenmann, U., Grogan, J., and Oppenheim, F. G. (2000). Saliva and dental pellicle–a review. Adv. Dent. Res. 14, 22–28. doi: 10.1177/08959374000140010301
Liu, L., Xu, Y., Cui, F., Xia, Y., Chen, L., Mou, X., et al. (2018). Monitoring of bacteria biofilms forming process by in-situ impedimetric biosensor chip. Biosens. Bioelectron. 112, 86–92. doi: 10.1016/j.bios.2018.04.019
Liu, Y., Gallardo-Moreno, A. M., Pinzon-Arango, P. A., Reynolds, Y., Rodriguez, G., and Camesano, T. A. (2008). Cranberry changes the physicochemical surface properties of E. coli and adhesion with uroepithelial cells. Coll. Surf. B Biointerf. 65, 35–42. doi: 10.1016/j.colsurfb.2008.02.012
Mansouri, S., Safa, A., Najar, S. G., and Najar, A. G. (2013). Inhibitory activity of Iranian plant extracts on growth and biofilm formation by Pseudomonas aeruginosa. Malaysian J. Microbiol. 9, 176–183.
Marsh, P. D. (2004). Dental plaque as a microbial biofilm. Caries Res. 38, 204–211. doi: 10.1159/000077756
Mira, A., Buetas, E., Rosier, B., Mazurel, D., Villanueva-Castellote, Á, Llena, C., et al. (2019). Development of an in vitro system to study oral biofilms in real time through impedance technology: validation and potential applications. J. Oral Microbiol. 11:1609838. doi: 10.1080/20002297.2019.1609838
Mitchell, J. (2011). Streptococcus mitis: walking the line between commensalism and pathogenesis. Mol. Oral Microbiol. 26, 89–98. doi: 10.1111/j.2041-1014.2010.00601.x
Neville, S. A., Lecordier, A., Ziochos, H., Chater, M. J., Gosbell, I. B., Maley, M. W., et al. (2011). Utility of matrix-assisted laser desorption ionization-time of flight mass spectrometry following introduction for routine laboratory bacterial identification. J. Clin. Microbiol. 49, 2980–2984. doi: 10.1128/JCM.00431-11
Nishikawara, F., Nomura, Y., Imai, S., Senda, A., and Hanada, N. (2007). Evaluation of cariogenic bacteria. Eur. J. Dent. 1, 31–39.
Pagliarulo, C., De Vito, V., Picariello, G., Colicchio, R., Pastore, G., Salvatore, P., et al. (2016). Inhibitory effect of pomegranate (Punica granatum L.) polyphenol extracts on the bacterial growth and survival of clinical isolates of pathogenic Staphylococcus aureus and Escherichia coli. Food Chem. 190, 824–831. doi: 10.1016/j.foodchem.2015.06.028
Peros, K., Mestrovic, S., Anic-Milosevic, S., and Slaj, M. (2011). Salivary microbial and nonmicrobial parameters in children with fixed orthodontic appliances. Angle Orthodontist 81, 901–906. doi: 10.2319/012111-44.1
Raghavendran, K., Mylotte, J. M., and Scannapieco, F. A. (2007). Nursing home-associated pneumonia, hospital-acquired pneumonia and ventilator-associated pneumonia: The contribution of dental biofilms and periodontal inflammation. Periodontology 2000, 164–177. doi: 10.1111/j.1600-0757.2006.00206.x
Rosenberg, M., Azevedo, N. F., and Ivask, A. (2019). Propidium iodide staining underestimates viability of adherent bacterial cells. Sci. Rep. 9:6483. doi: 10.1038/s41598-019-42906-3
Rosenbloom, R. G., and Tinanoff, N. (1991). Salivary Streptococcus mutans levels in patients before, during, and after orthodontic treatment. Am. J. Orthod. Dentofacial Orthopedics 32, 1079–1087. doi: 10.1016/0889-5406(91)70046-Y
Rozalski, M., Micota, B., Sadowska, B., Stochmal, A., Jedrejek, D., Wieckowska-Szakiel, M., et al. (2013). Antiadherent and antibiofilm activity of Humulus lupulus L. derived products: New pharmacological properties. BioMed Res. Int. 2013:101089. doi: 10.1155/2013/101089
Sateriale, D., Facchiano, S., Colicchio, R., Pagliuca, C., Varricchio, E., Paolucci, M., et al. (2020). In vitro synergy of polyphenolic extracts from honey, myrtle and pomegranate against oral pathogens, S. mutans and R. dentocariosa. Front. Microbiol. 11:1465. doi: 10.3389/fmicb.2020.01465
Savoia, D. (2012). Plant-derived antimicrobial compounds: alternatives to antibiotics. Future Microbiol. 7, 979–990. doi: 10.2217/fmb.12.68
Sendamangalam, V., Choi, O. K., Kim, D., and Seo, Y. (2011). The anti-biofouling effect of polyphenols against Streptococcus mutans. Biofouling 27, 13–19. doi: 10.1080/08927014.2010.535897
Servetas, S. L., Carpenter, B. M., Haley, K. P., Gilbreath, J. J., Gaddy, J. A., and Scott Merrell, D. (2016). Characterization of key Helicobacter pylori regulators identifies a role for arsrs in biofilm formation. J. Bacteriol. 198, 2536–2548. doi: 10.1128/JB.00324-16
Silva, L. N., Zimmer, K. R., Macedo, A. J., and Trentin, D. S. (2016). Plant natural products targeting bacterial virulence factors. Chem. Rev. 116, 9162–9236. doi: 10.1021/acs.chemrev.6b00184
Simões, L. C., Simþoes, M., Oliveira, R., and Vieira, M. J. (2007). Potential of the adhesion of bacteria isolated from drinking water to materials. J. Basic Microbiol. 47, 174–183. doi: 10.1002/jobm.200610224
Singh, N., Nayyar, A., Bhattacharjee, G., Singh, A. K., and Pruthi, V. (2012). Assessment of dentifrices against Candida biofilm. Appl. Biochem. Biotechnol. 167, 1688–1698. doi: 10.1007/s12010-012-9574-2
Slobodníková, L., Fialová, S., Rendeková, K., Kováč, J., and Mučaji, P. (2016). Antibiofilm activity of plant polyphenols. Molecules 21:E1717. doi: 10.3390/molecules21121717
Sogawa, K., Watanabe, M., Sato, K., Segawa, S., Ishii, C., Miyabe, A., et al. (2011). Use of the MALDI BioTyper system with MALDI-TOF mass spectrometry for rapid identification of microorganisms. Anal. Bioanal. Chem. 400, 1905–1911. doi: 10.1007/s00216-011-4877-7
Stepanović, S., Vuković, D., Hola, V., Di Bonaventura, G., Djukić, S., Ćirković, I., et al. (2007). Quantification of biofilm in microtiter plates: overview of testing conditions and practical recommendations for assessment of biofilm production by staphylococci. APMIS 115, 891–899. doi: 10.1111/j.1600-0463.2007.apm_630.x
Wenzel, R. P. (2007). Health care–associated infections: major issues in the early years of the 21st century. Clin. Infect. Dis. 45(Suppl. 1), S85–S88. doi: 10.1086/518136
Whalan, R. H., Funnell, S. G. P., Bowler, L. D., Hudson, M. J., Robinson, A., and Dowson, C. G. (2006). Distribution and genetic diversity of the ABC transporter lipoproteins PiuA and PiaA within Streptococcus pneumoniae and related streptococci. J. Bacteriol. 188, 1031–1038. doi: 10.1128/JB.188.3.1031-1038.2006
Whitchurch, C. B., Tolker-Nielsen, T., Ragas, P. C., and Mattick, J. S. (2002). Extracellular DNA required for bacterial biofilm formation. Science 295, 1487–1487. doi: 10.1126/science.295.5559.1487
WHO (2014). Antimicrobial Resistance. Global Report on Surveillance. Geneva: World Health Organization.
Yallop, M. L., Paterson, D. M., and Wellsbury, P. (2000). Interrelationships between rates of microbial production, exopolymer production, microbial biomass, and sediment stability in biofilms of intertidal sediments. Microb. Ecol. 39, 116–127. doi: 10.1007/s002489900186
Keywords: in vitro antibiofilm agents, polyphenolic extracts, dental plaque bacteria, Streptococcus mutans, Streptococcus oralis, Streptococcus mitis, Rothia dentocariosa
Citation: Sateriale D, Imperatore R, Colicchio R, Pagliuca C, Varricchio E, Volpe MG, Salvatore P, Paolucci M and Pagliarulo C (2020) Phytocompounds vs. Dental Plaque Bacteria: In vitro Effects of Myrtle and Pomegranate Polyphenolic Extracts Against Single-Species and Multispecies Oral Biofilms. Front. Microbiol. 11:592265. doi: 10.3389/fmicb.2020.592265
Received: 06 August 2020; Accepted: 09 October 2020;
Published: 05 November 2020.
Edited by:
Rodolfo García-Contreras, National Autonomous University of Mexico, MexicoReviewed by:
Zhejun Wang, University of British Columbia, CanadaBiao Ren, Sichuan University, China
Copyright © 2020 Sateriale, Imperatore, Colicchio, Pagliuca, Varricchio, Volpe, Salvatore, Paolucci and Pagliarulo. This is an open-access article distributed under the terms of the Creative Commons Attribution License (CC BY). The use, distribution or reproduction in other forums is permitted, provided the original author(s) and the copyright owner(s) are credited and that the original publication in this journal is cited, in accordance with accepted academic practice. No use, distribution or reproduction is permitted which does not comply with these terms.
*Correspondence: Caterina Pagliarulo, Y2F0ZXJpbmEucGFnbGlhcnVsb0B1bmlzYW5uaW8uaXQ=