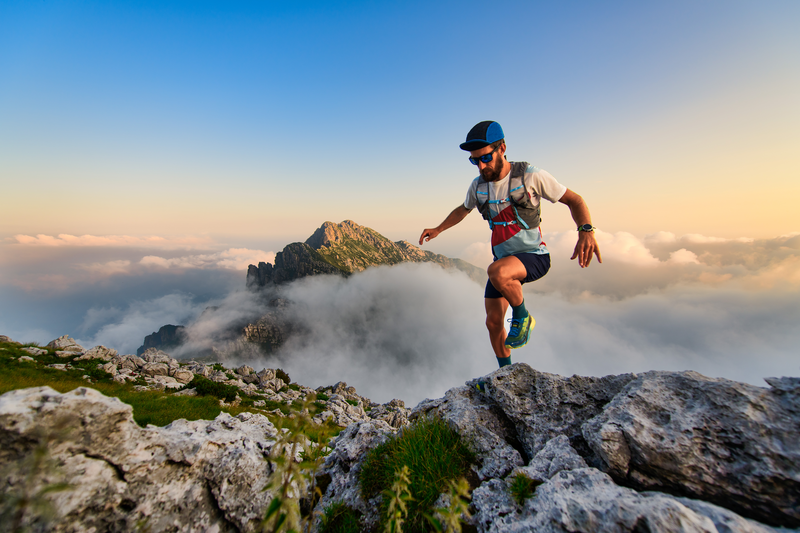
94% of researchers rate our articles as excellent or good
Learn more about the work of our research integrity team to safeguard the quality of each article we publish.
Find out more
REVIEW article
Front. Microbiol. , 02 December 2020
Sec. Microbial Immunology
Volume 11 - 2020 | https://doi.org/10.3389/fmicb.2020.577846
This article is part of the Research Topic Recent Advances in the Immunology of Helminth Infection – Protection, Pathogenesis and Panaceas View all 21 articles
Helminths stage a powerful infection that allows the parasite to damage host tissue through migration and feeding while simultaneously evading the host immune system. This feat is accomplished in part through the release of a diverse set of molecules that contribute to pathogenicity and immune suppression. Many of these molecules have been characterized in terms of their ability to influence the infectious capabilities of helminths across the tree of life. These include nematodes that infect insects, known as entomopathogenic nematodes (EPN) and plants with applications in agriculture and medicine. In this review we will first discuss the nematode virulence factors, which aid parasite colonization or tissue invasion, and cause many of the negative symptoms associated with infection. These include enzymes involved in detoxification, factors essential for parasite development and growth, and highly immunogenic ES proteins. We also explore how these parasites use several classes of molecules (proteins, carbohydrates, and nucleic acids) to evade the host’s immune defenses. For example, helminths release immunomodulatory molecules in extracellular vesicles that may be protective in allergy and inflammatory disease. Collectively, these nematode-derived molecules allow parasites to persist for months or even years in a host, avoiding being killed or expelled by the immune system. Here, we evaluate these molecules, for their individual and combined potential as vaccine candidates, targets for anthelminthic drugs, and therapeutics for allergy and inflammatory disease. Last, we evaluate shared virulence and immunomodulatory mechanisms between mammalian and non-mammalian plant parasitic nematodes and EPNs, and discuss the utility of EPNs as a cost-effective model for studying nematode-derived molecules. Better knowledge of the virulence and immunomodulatory molecules from both entomopathogenic nematodes and soil-based helminths will allow for their use as beneficial agents in fighting disease and pests, divorced from their pathogenic consequences.
Parasitic nematodes infect hosts from almost all branches of the tree of life, often using conserved strategies to successfully invade host tissue while evading the rapid immune response against them (Blaxter and Koutsovoulos, 2015). Their ability to manipulate host immunity is incredible. Consider a 21-year-old woman who presents with no pathology, inflammation, or any symptoms other than the sensation of something moving in her eye (Shah and Saldana, 2010). On examination, a live two cm nematode was found in the superior subconjunctival space of her eye and removed. Subsequent blood testing revealed microfilaremia (i.e., the presence of thousands of juvenile parasitic nematodes in her blood and likely other tissues). Her medical history revealed that she had picked up this infection on a trip to Nigeria 6 years earlier. This carefully coordinated infection is facilitated in part through immunomodulatory excretory/secretory (ES) products that allow the parasite to establish long-lived infection. Given their potent immunomodulatory properties, understanding these nematode-derived products may lead to the development of new anthelminthics and vaccines to overcome host immune suppression, or instead exploited for new therapies in allergy and inflammatory diseases.
Nematode-derived products comprise a diverse range of molecules, including proteins, lipids, carbohydrates, and nucleic acids (Maizels et al., 2018). They have a multitude of effects on the host, from toxic virulence factors that cause tissue damage to powerful immunomodulators. Some of these molecules are homologous to host molecules, allowing the parasite to manipulate immune cell function by mimicking host proteins or producing miRNAs that target host gene expression (Buck et al., 2014; Johnston et al., 2017). Others are unique to parasites and are not found in the host (Periago and Bethony, 2012; Flynn et al., 2019). These virulence factors are important for the parasite’s infectivity and growth within the host, and can cause host tissue damage.
Entomopathogenic nematodes (EPN), which infect and kill their insect hosts within days, are currently used for controlling agricultural pests (Cooper and Eleftherianos, 2016). These parasites also provide a valuable model of parasitic nematode infections with significantly lower costs than rodent models. EPNs produce venom proteins with significant similarity to many proteins found in mammalian pathogenic nematodes (Lu et al., 2017; Chang et al., 2019). EPNs, like nematodes that infect mammals, need to suppress and/or evade the initial immune response (Bai et al., 2013; Brivio and Mastore, 2018). For this reason, identification of EPN-derived molecules and their effects on the host may be translational to nematode parasites of mammals.
Several recent reviews have provided significant insight into the immunomodulatory role of nematode-derived molecules, in particular excreted and secreted proteins (McSorley et al., 2013; Gazzinelli-Guimaraes and Nutman, 2018; Maizels et al., 2018; Zakeri et al., 2018; Maizels, 2020; Ryan et al., 2020). In this review, we highlight recently characterized nematode-derived molecules involved in host-parasite interactions, expand our discussion to non-protein molecules (e.g., lipids, nucleic acids) as potential for therapeutic targets, and investigate the utility of non-mammalian model systems (e.g., insect, plant) to understand host-nematode interactions. We first describe nematode virulence factors, how they mediate host tissue damage, and their utility as anthelminthic or vaccine targets. Next, we discuss nematode-derived products that suppress the host immune response and can be harnessed therapeutically for their immunomodulatory properties. Finally, we evaluate what can be learned from EPNs and plant-parasitic nematodes (PPNs) as models for mammalian-pathogenic nematodes to identify new parasite virulence and immunomodulatory molecules for immunotherapies and drug targets. Studying nematode-derived products’ role in host-parasite interactions provides valuable insight for novel treatments to fight off infection or alleviate allergic and inflammatory diseases.
Many parasitic nematodes transition to parasitism from a developmentally arrested lifecycle stage in order to obtain resources, complete their lifecycle, and find a long-lasting niche (Hotez et al., 2008). To this end, they can produce a wide variety of molecules to assist in their ability to attack, invade, and digest host tissue (Periago and Bethony, 2012). Their arsenal of host virulence molecules can be targeted to develop vaccines or design anthelminthics to reduce worm burden and mitigate host pathology in infected individuals. Significant research on anthelminthics and vaccines is performed in preclinical rodent models to find potential targets for vaccine development prior to the initiation of human research and clinical trials (Table 1 and Figure 1). Here, we highlight promising nematode-derived molecules for anthelminthic and vaccine targets. These include enzymes involved in host tissue invasion and parasite feeding, nematode-derived molecules necessary for parasite development, and immunogenic ES proteins as vaccine candidates.
Table 1. Nematode-derived molecules and their potential for therapeutic use as vaccine, anthelminthic or anti-inflammatory treatments.
Figure 1. The pleiotropic functions of nematode-derived molecules. Functions range from promoting or inhibiting the host immune response (left), to providing essential physiologic functions for the nematode parasite (right). Understanding the virulence (red) and immunomodulatory (green) potential for specific nematode-derived molecules allows us to determine their utility as vaccines, anthelminthics, or new therapeutics for allergic or inflammatory diseases.
Nematodes produce a variety of enzymes for host tissue digestion and feeding that provide useful targets for vaccine development or anthelminthics. For example, enolases are plasminogen-binding surface proteins that are involved in assisting parasites in invading host tissue by promoting the degradation of fibrin (Ayón-Núñez et al., 2018; Jiang et al., 2019). Vaccination with a Trichinella spiralis enolase resulted in the production of specific antibodies in mice, however, this did not lead to a striking reduction in worm burden (Zhang et al., 2018). Additionally, parasite-derived enolases were found in the serum of Brugia malayi-infected individuals, indicating its potential as a diagnostic molecule (Reamtong et al., 2019).
For hookworms, which feed on the blood of their host causing anemia, enzymes involved in processing blood hemoglobin have demonstrated promising vaccine potential (Periago and Bethony, 2012; Hotez et al., 2013). Specifically, the N. americanus hemoglobinase aspartic protease (Na-APR-1) and the heme detoxifying Na-Glutathione S-transferase (Na-GST-1), are currently in clinical trials for a hookworm vaccine, with early results showing the vaccine is safe and immunogenic (Zhan et al., 2010; Bottazzi, 2013, 2017; Diemert et al., 2017). Several phase 1 clinical trials have been completed with recombinant Na-APR-1 vaccine (Bottazzi, 2013). Administration of the recombinant Na-GST-1 vaccine to hookworm-naïve individuals as well as those from hookworm endemic regions in Brazil was found to be safe and immunogenic, and phase 2 clinical trials are underway (Bottazzi, 2017). The success of these trials suggest that safe and effective vaccines could be developed by targeting enzymes integral to nematode feeding and survival. For example, in filarial nematodes, enzymes have provided promising targets for anthelminthic and vaccine development. These include GST, which protects the nematode parasite by neutralizing host cytotoxic products (e.g., reactive oxygen species) and mediating drug resistance, and UDP-glucuronosyltransferase (Bm-UGT), a detoxifying enzyme expressed in the B. malayi intestinal lumen that was essential for its survival (Saeed et al., 2018; Flynn et al., 2019).
Lipid-derived molecules are involved in a variety of biological functions in parasitic nematodes including metabolism and development, making them of particular interest as potential novel anthelminthics that target parasite fitness and development (Ma et al., 2020). Endocannabinoids are lipid-derived molecules important for metabolic homeostasis and immunity, among other functions, and are produced by both mammals and nematodes (Batugedara et al., 2018). The specific function of parasitic nematode-derived endocannabinoids in the host is unclear. However, functional studies for endocannabinoids have been possible in the free-living nematode C. elegans, where endocannabinoids, 2-arachidonoyl glycerol and anandamide played a significant role in metabolism and aversion to pain (Oakes et al., 2017; Galles et al., 2018). Understanding the interplay between host and parasitic nematode-derived endocannabinoids might reveal new immune and metabolic targets to reduce parasite fitness or improve the host response.
The steroidal hormone dafachronic acid (DA) modulates nematode lipid metabolism and development, and the ligand-binding domain for the steroid hormone nuclear receptor for DA (DAF-12) is highly conserved among nematode species, such as C. elegans, N. brasiliensis, Haemonchus contortus, and Strongyloides stercoralis, therefore targeting these receptors may have therapeutic potential to impair parasite fitness (Ogawa et al., 2009; Patton et al., 2018; Ma et al., 2019a,b; Ayoade et al., 2020). In C. elegans, the DAF-12 system acts to inhibit dauer formation, and in H. contortus, DA promoted transition from free-living to parasitism by modulating dauer-like signaling (Ogawa et al., 2009; Ma et al., 2019a,b). Recent investigation of the DAF-12 system in S. stercoralis hyperinfection supports the therapeutic potential of inducing this steroid hormone pathway. In a mouse model of S. stercoralis hyperinfection, which is an often fatal condition in immunocompromised individuals, DA treatment was protective and reduced S. stercoralis parasite burdens by suppressing the development of autoinfective L3a larvae (Patton et al., 2018).
Molecules integral to the parasite’s growth and ability to colonize and feed on the host offer promising vaccine and anthelminthic targets. However other weapons of warfare employed by the worms, such as excreted molecules that are immunogenic and promote a protective anti-helminthic immune response can also be considered as vaccines or adjuvants.
The family of venom allergen-like proteins (VAL) family is one such example. This family has been extensively studied especially given their high expression in many parasitic nematodes (Wilbers et al., 2018). While these proteins are conserved among a wide variety of nematodes, their functions are diverse—including examples of both pro-inflammatory and immunosuppressive molecules. Here we explore some of the most pertinent examples from this family, focusing on the VAL proteins that have demonstrated immunogenic properties for vaccine potential. VAL proteins are homologs of vespid (wasp) venom proteins, the latter of which are locally toxic and can induce allergic and inflammatory responses in humans (King and Valentine, 1987; Tawe et al., 2000; Murray et al., 2001). This makes nematode-derived homologs of these proteins of particular interest in understanding host-nematode interactions that lead to the excessive pathology for the host (King and Valentine, 1987; Wilbers et al., 2018; Tawe et al., 2000; Murray et al., 2001). VAL proteins are conserved in several parasitic nematodes, including Heligmosomoides polygyrus, B. malayi, Trichinella pseudospiralis, and Teladorsagia circumcincta (Ellis et al., 2014; Wang et al., 2017a; Asojo et al., 2018; Darwiche et al., 2018; Wilbers et al., 2018). Notably, VALs are highly expressed: a study of the secreted products from the gastrointestinal murine parasite H. polygyrus revealed that members of the VAL protein family were the most abundant product (Hewitson et al., 2011). Due to their abundance and conserved structure, VALs have been considered as vaccine candidates. For instance, the B. malayi protein Bm-VAL-1 is highly immunogenic, promoting antibody and T cell responses in humans, and conferring protection in vaccination models in mice and jirds (Maizels et al., 1995; Kalyanasundaram and Balumuri, 2011; Darwiche et al., 2018). To target larval stages, Bm-VAL-1 and B. malayi abundant larval transcript-2 (Bm-ALT-2) were combined in a multivalent vaccine, which successfully increased antibody titers and provided enhanced worm killing in a challenge infection in mice (Kalyanasundaram and Balumuri, 2011; Anugraha et al., 2013). The importance of combining antigens as a vaccine strategy for a more effective immune response is increasingly being recognized. In H. polygyrus infection, immunization with a cocktail of three H. polygyrus VALs induced antibody production that protected mice from challenge H. polygyrus infection (Hewitson et al., 2015). The biological function of VALs in infection is unclear, however, given that they are secreted sterol-binding proteins, they may bind immunomodulatory molecules such as prostaglandins and leukotrienes, which have both immune stimulatory and regulatory roles (Honda and Kabashima, 2019).
The immunogenic properties of VALs have made them potential targets for vaccine development. Na-ASP-2 (Ancylostoma-secreted protein), another member of the VAL family, initially showed promise as a hookworm vaccine (Bethony et al., 2005; Diemert et al., 2012). This allergen-like protein was associated with the production of IgE and IgG4 antibody responses that correlate with reduced risk of high Necator americanus burdens in endemically affected areas. Further, validation studies in dogs confirmed that Na-ASP-2 specific antibodies were protective in challenge infections (Bethony et al., 2005). However, early clinical trials resulted in generalized urticarial reactions in many individuals, associated with pre-existing Na-ASP-2-specific IgE (Diemert et al., 2012). This failed clinical trial is a cautionary tale for vaccine development against parasitic nematodes. First, the potential for non-protective allergic-immune responses in previously exposed individuals in endemic areas needs to be considered. Additionally, anti-inflammatory nematode-derived molecules that are necessary to mitigate host tissue damage and inflammation may need to be carefully considered before being used as vaccine or therapeutic targets, since inhibiting these may be more pathogenic than beneficial for the host. Although the effectiveness of VALs as vaccine targets for helminths is challenged by these recent studies, their immunogenic potential may be harnessed for use as adjuvants against other infectious pathogens. For example, recombinant ASP-1 derived from the filarial nematode Onchocerca volvulus, Ov-ASP-1, has shown promise as an adjuvant for vaccines against viral infections, such as HIV, SARS-CoV, and influenza, augmenting viral antigen-specific antibody titers in immunization studies in mice (MacDonald et al., 2005).
Serine protease inhibitors (serpins) constitute another highly conserved family of nematode ES proteins, identified in many nematodes, including B. malayi, Anisakis simplex, and H. contortus (Zang et al., 2000; Bennuru et al., 2009; Yi et al., 2010; Valdivieso et al., 2015). In vitro studies of a serpin derived from H. contortus showed that it reduced blood coagulation (Yi et al., 2010). The anti-coagulation function of serpins is likely an important feeding mechanism for blood-feeding nematodes. B. malayi microfilariae secrete serpins, perhaps to mitigate a coagulation response to excess circulating microfilariae in the bloodstream during chronic infection (Zang et al., 2000). B. malayi serpins are also immunogenic and stimulate mouse and human B and T cell responses, however, this immune response is short-lived, suggesting that serpins alone are not effective vaccine candidates for long-term immunity (Zang et al., 2000). It is interesting to note that despite many preclinical studies on nematode-secreted proteins, only nematode enzymes are currently the subject of ongoing vaccine clinical trials (Table 1). This suggests that targeting virulence factors, which are integral components of the worm’s physiology may offer the most promising vaccination targets.
Parasitic nematodes have evolved multiple mechanisms to evade the host immune system, allowing persistence in their host, in some cases for decades, without being killed (Maizels et al., 2018). Nematode-derived products are key to the immunomodulatory capabilities of the parasites, and investigating their mechanism of action may identify novel therapies for allergic and inflammatory diseases. Reviewing current research into which molecules show the most promise for the development of immunotherapies is an ongoing conversation that has already received a significant amount of attention (Smallwood et al., 2017; Maizels et al., 2018; Bohnacker et al., 2020; Coakley and Harris, 2020; Ryan et al., 2020; White et al., 2020). Here we contribute to that conversation by contextualizing the most current advances in our understanding of immunomodulatory capabilities of nematodes and identifying the molecules that appear to show the most promise for further research.
Identifying the specific molecules within parasitic nematode ES or extract that have the strongest immunomodulatory potential is a main focus in the field of “helminth therapeutics” (Ryan et al., 2020). Nematode-derived immunomodulatory molecules include mimics of host immune mediators as well as novel molecules that are unique to parasites themselves. Many of the products with extensive characterization are proteins. However non-proteins products, such as carbohydrates and small RNAs, are currently being studied for their potential role in immunomodulation (Prasanphanich et al., 2013; Hokke and van Diepen, 2017; Maizels et al., 2018). Mechanisms of immunomodulation for the main nematode-derived molecules discussed here are summarized in Figure 1.
The differential glycosylation of lipids and proteins during the lifecycle of parasitic nematodes provides a unique opportunity for the development of vaccines and novel anthelminthics (Prasanphanich et al., 2013; Hokke and van Diepen, 2017).
Glycosylation patterns unique to the parasite are potential vaccine targets, because they are distinct from host glycosylation patterns and potentially more immunogenic, acting as pathogen-associated molecular patterns. On the other hand, glycosylation patterns that mimic the host can be explored for their immunomodulatory potential, providing novel immunotherapies. Gala1–3GalNAc-R (α-Gal), a parasite-specific glycan epitope produced by the sheep pathogen H. contortus induced an IgG response in lambs and is implicated in protection against H. contortus challenge infections (van Stijn et al., 2010). Similarly, glycosylation patterns are essential for the host to recognize the glycans on the surfaces of mucin-like proteins expressed by T. canis, and led to pro-inflammatory cytokine expression by human THP-1 macrophages (Długosz et al., 2019).
In the anaphylactic reaction known as α-Gal Syndrome (AGS), humans produce IgE in response to α-Gal present in red meat. However, humans infected with T. canis had reduced IgE antibodies to α-Gal caps on N-glycans, indicating that the parasites may be able to downregulate the allergic response, even though this is not an epitope that the worms themselves make (Hodžić et al., 2020). The ability of the parasite to suppress the immune response to oligosaccharides provides evidence for the “hygiene hypothesis” which argues that an increased sensitivity to a wide variety of allergens may result from a reduction in helminth infections in countries with stronger sanitation infrastructure (Yazdanbakhsh et al., 2002). In another example of immunomodulation, N-glycans produced by the canine heartworm Dirofilaria immitis allowed the worm to hide from the host immune system, by imitating host glycosylation patterns and also using unique glycosylation patterns that interfered with host binding to other nematode-derived molecules, a technique known as glycol-gimmickry (Martini et al., 2019). Further, changing the glycosylation patterns on proteins in H. polygyrus resulted in an increase in proinflammatory cytokines and a decrease in nematode-specific IgG1 in Balb/c mice (Doligalska et al., 2013). Together these studies show the importance of glycosylation patterns in both immunogenicity and evasion of the host immune system by parasitic nematodes.
ES proteins have systemic effects on the immune system, which could be harnessed as therapies for allergy and inflammatory diseases. For example, in vitro treatment of macrophages with T. spiralis ES generated a regulatory phenotype that prevented airway allergic inflammation in mice (Kang et al., 2019). Similarly, in the dog hookworm A. caninum, the secreted anti-inflammatory protein-2 (AIP-2) suppressed airway inflammation in an asthma model in mice in a dendritic cell and Treg-dependent pathway (Navarro et al., 2016). The H. polygyrus ES proteins, H. polygyrus Alarmin Release Inhibitor (HpARI) and H. polygyrus Binds Alarmin Receptor and Inhibits (HpBARI), were identified due to their ability to downregulate the initiation of both type 2 allergic and parasitic responses through the IL-33-ST2 pathway. HpARI bound to the alarmin IL-33 in necrotic cells and prevented its release, while HpBARI binds IL-33 receptor ST2, preventing IL-33 engagement (Osbourn et al., 2017; Vacca et al., 2020). Intranasal administration of HpARI followed by infection with the skin-penetrating Nippostrongylus brasiliensis lead to greater intestinal worm burdens in comparison to untreated infected mice, indicating that a H. polygryus-specific product could impair immune responses to a different but related parasitic worm. HpBARI administration suppressed Th2 inflammatory responses to the extract from the allergenic fungus Alternaria. While H. polygyrus is a nematode parasite of mice, and HpBARI targets murine ST2, a homolog of HpBARI (HpBARI_Hom2), was identified that could effectively suppress the human ST2, supporting the clinical relevance of these findings. A similar strategy to inhibit Th2 cytokine responses is employed by T. muris with p43, the most abundant protein in its excretome/secretome (Bancroft et al., 2019). T. muris p43 contains structural domains homologous to thrombospondin and the IL-13 receptor, which allowed it to tether to matrix proteoglycans and bind and inhibit IL-13. Functionally, p43 inhibits its function in promoting worm expulsion. Another recently identified candidate for immune modulation is the enzyme H. polygyrus-derived Hpb glutamate dehydrogenase (GDH), which reduced allergic airway inflammation in mice by inducing a switch from pro-inflammatory to anti-inflammatory eicosanoids (e.g., prostaglandins). Hpb-GDH was effective at suppressing inflammatory pathways in both mouse and human macrophages and granulocytes by inhibiting the 5-lipooxygenase and instead promoting the cyclooxygenase pathway leading to the synthesis of prostanoids and the downregulation of 5-LOX metabolites (de Los Reyes et al., 2020). Identifying nematode-derived enzymes that target host immune-metabolic pathways with the resulting effect of suppressing inflammatory responses is an exciting new research avenue that may offer novel immunotherapeutics of allergic diseases.
Nematode-derived cysteine protease inhibitors (cystatins) also have demonstrated anti-inflammatory functions. Cystatin from the filarial nematode Acanthocheilonema viteae downregulated Th2 cytokine responses in an airway allergy model in Balb/c mice, including decreased IL-5 and IL-13 in the broncho-alveolar lavage (Daniłowicz-Luebert et al., 2013). In in vitro microglial cultures from cells harvested from rat brains and stimulated with LPS, A. viteae cystatin downregulated nitric oxide and TNFα expression as well as mRNA expression of the pro-inflammatory cytokines iNOS and COX-2, providing promise for therapies for neurodegenerative diseases, such as Parkinson’s disease (Behrendt et al., 2016). Cystatins from filarial nematode B. malayi were also immunosuppressive: treatment with recombinant Bm cystatin was able to reduce dextran sulfate sodium (DSS)-induced colitis in mice (Bisht et al., 2019). Specifically, Bm cystatin led to increased Tregs in the colon and alternative activation of peritoneal macrophages. Recently, cystatin from the ES products of the zoonotic nematode T. spiralis, rTsCstN, was discovered as structurally homologous to human cystatin (Kobpornchai et al., 2020). Functionally, rTsCstN suppressed inflammatory cytokine production by LPS-treated mouse bone marrow derived macrophages. Cystatins are found in a wide variety of nematode species, including O. volvulus, H. contortus, and B. malayi (Hartmann et al., 2002; Hartmann and Lucius, 2003; Gregory and Maizels, 2008; Arumugam et al., 2014; Wang et al., 2017c) and their potential as immunomodulators is being explored.
Cytokines are commonly mimicked by parasitic nematodes in their efforts to modulate the host immune system. One such ortholog is Macrophage Inhibitory Factor (MIF), which is produced by many nematodes including B. malayi, Anisakis simplex, Wuchereria bancrofti, and H. contortus (Bennuru et al., 2009; Chauhan et al., 2015; Wang et al., 2017b; Harischandra et al., 2018). Murine MIF is important for alternative activation of macrophages, promotes the Th2 response during nematode infection, and is required for optimal worm clearance (Filbey et al., 2019). On the other hand, nematode MIF homologs appear to have an immunosuppressive effect (Cho et al., 2011). MIF isolated from A. simplex increased Treg responses and reduced colitis severity. Here, mice treated with rAs-MIF regained previously lost weight and had lower disease activity indices in DSS-induced colitis. Another modulator of Tregs are TGF-β orthologs which are found in several parasitic nematodes, including H. polygyrus, N. brasiliensis, and T. circumcincta (McSorley et al., 2010). Hp-TGM was recently identified as a TGF-β mimic produced by H. polygyrus (Johnston et al., 2017). Interestingly, this mimic is structurally distinct from mammalian TGF-β, however, it is able to bind to mouse and human TGF-β receptors and induce Foxp3 expression in Treg cells. Furthermore, Hp-TGM was immunosuppressive in an allogenic skin graft model where it delayed graft rejection.
Across the proteomes of parasitic nematodes, there is consistency in the presence of amino acid motifs recognizable by T-cell receptors, known as T-cell-exposed motifs or TCEMs (Homan and Bremel, 2018). Using bioinformatics to analyze the proteomes of a wide variety of nematodes, many proteins have been identified with extremely high indices of predicted immunosuppression, indicating that the protein is likely to promote Treg responses. For instance, the hookworm, A. ceylanicum alone had over 500 peptides with a highly suppressive index (Homan and Bremel, 2018). Given its rapidity and cost-effectiveness, the ability to screen in silico for immunotherapeutic nematode-derived proteins may constitute an important frontier for research in nematode immunomodulation.
Some products have an effect on a wide range of immune cells. These include ES-62, a secreted protein from the nematode A. viteae, which interacts with B cells, dendritic cells, macrophages, and mast cells to downregulate inflammatory responses (Pineda et al., 2014). This protein’s anti-inflammatory potential is reliant on post-translational modification including the attachment of phosphorlycholines. Current research on characterizing small molecule analogs for ES-62 is an example of the potential for nematode-derived products to be the impetus for the development of immunotherapies (Suckling et al., 2018).
A recent study highlighted nematode-secreted DNases as a novel mechanism for impairing neutrophil-mediated killing (Bouchery et al., 2020). Within hours post-infection with rat hookworm N. brasiliensis, host neutrophils swarmed invading nematodes and released neutrophil extracellular traps (NETs) comprised of nucleic acids, histones, and granular proteins. This provides evidence that NETs, originally identified in bacterial killing, are also used against helminths. However, at the same time, hookworms have developed an excretory/secretory deoxyribonuclease protein, known as Nb-DNase II, that can degrade the NETs, in both in vitro and in vivo models. This new finding provides an exciting avenue of targeting parasitic nematode DNAses as vaccine or therapeutic targets to promote NET-mediated nematode killing.
Extracellular vesicles (EVs) released by parasitic nematodes during infection may provide a powerful strategy for the parasitic nematode to generate widespread effects on host cells (Coakley et al., 2015). Of therapeutic promise, treatment with EVs from T. spiralis and N. brasiliensis suppressed colitis of mice and protection was associated with reduced proinflammatory cytokines and increased Th2 and Treg responses (Eichenberger et al., 2018; Yang et al., 2020). In addition to containing lipids and proteins that may be immunomodulatory, EVs may also serve as cargos to deliver small RNAs to host cells, such as macrophages and intestinal cells, where they target and suppress host RNA. sRNAs have been identified in EVs generated by several parasitic nematodes, including T. spiralis, N. brasiliensis, Trichuris muris, and H. polygyrus, where they are predicted to target host immune gene networks (Tritten et al., 2017; Eichenberger et al., 2018; Chow et al., 2019; Yang et al., 2020). For example, H. polygyrus EVs were able to suppress macrophage responses and IL-33 signaling, and contained miRNAs that specifically targeted host DUSP1 RNA, a regulator of MAPK signaling (Buck et al., 2014; Coakley et al., 2017). miRNA generation has been reported in several nematode species, including Ascaris suum, which infects pigs, where miRNA sequence analysis predicted that they targeted the host Th2 immune response (IL-13, IL-25, IL-33) (Hansen et al., 2019). Circulating filarial nematode-derived miRNAs were detected in the blood of Litomosomoides sigmodontis-infected mice, O. volvulus-infected humans, Loa loa-infected baboons and Onchocerca ochengi-infected cattle (Buck et al., 2014; Tritten et al., 2014; Quintana et al., 2015), however, whether they were present in EVs, or targeted host gene expression, is unclear.
The products included in EVs can differ by lifecycle stage and sex of the nematode (Gu et al., 2017; Harischandra et al., 2018). Examining the molecules found in EVs for all lifecycle stages will allow for the discovery of a wide variety of potential drug targets. Molecules found in all developmental stages associated with the host could be strong candidates for the development of vaccines, allowing the immune system to recognize parasites throughout an infection, such as Galectin-2 (Hertz et al., 2020). On the other hand, molecules unique to adults may assist the worm in evading the host immune system in order to maintain a chronic infection, making them of particular interest for the development of anthelminthics and as immunotherapies. Interestingly, adult female B. malayi EVs had far more complex proteomes than males, with nearly four times as many proteins, including a MIF homolog, which may be involved in regulating the immune system (Harischandra et al., 2018). EVs may offer containment and protection from degradation of a multitude of immunogenic nematode antigens that could allow for more effective host immune responses to helminths. For example, intact EVs, but not lysed EVs, from T. muris were able to reduce egg burden in a subsequent infection of this worm, making them potential vaccine candidates for improved immunogenicity (Coakley et al., 2017; Shears et al., 2018). EVs present a unique opportunity to study the parasitic lifecycle, allowing for a greater understanding of molecules that are required to initiate and maintain an infection.
Model systems have proven to be valuable to the study of human disease (Rubin et al., 2000). For example, initial analysis of the Drosophila melanogaster genome identified that over 60% of human disease-associated genes had orthologs in the fly. Studies of fly immunity led to the discovery and description of the Toll pathway and subsequently Toll-like receptors in mammals (Lemaitre, 2004). Similarly, determining the mechanistic function of conserved parasitic nematode effectors may benefit from the use of the EPN-insect model system, which is cheaper, faster, and allows for more individual hosts to be used per experiment than could possibly be done in a mammalian system. Effectors could be mechanistically described in the model system, providing an elevated starting point for experimentation in parasitic infections of mammals.
EPNs form a mutualistic relationship with bacteria, carrying them inside their intestine when they infect their hosts, releasing the bacteria into the hemolymph of their insect host. The bacteria assist in killing the host, and, along with liquified host tissue, serve as a food source (Bai et al., 2013; Brivio and Mastore, 2018). Similar to skin-penetrating nematode parasites of mammals, the initial infection process is entirely dependent on the ability of the nematode to enter the host and suppress its immune system. EPNs suppress the host immune system early in infection, causing it to tolerate not only nematode parasites but their symbiotic bacteria, until the host succumbs to infection (Toubarro et al., 2013). The specific molecules excreted/secreted from EPNs could be used for pest control in agricultural settings and also for immunoregulatory studies. Here we discuss the current research and known functions of EPN-derived virulence and immunomodulatory molecules, and how they relate to molecules employed by mammalian pathogenic nematodes. We also discuss main virulence factors that are present in plant parasitic nematodes, highlighting the striking conservation of these parasitic virulence mechanisms across the tree of life.
The ES products from the EPN Heterorhabditis bacteriophora are lethal to their insect hosts at high concentrations (Kenney et al., 2019). Treatment of Drosophila with proteins extracted from the supernatant of activated H. bacteriophora suppressed expression of antimicrobial protein diptericin, a product of the immune deficiency (Imd) pathway in insects (Kenney et al., 2019). This immunomodulatory mechanism is swift enough to allow for the infection of not just the nematode, but its mutualistic bacterial co-infector Photorhabdus luminescens, which would otherwise be killed by its insect host. In a similar manner, S. carpocapsae suppresses the immune response of its Drosophila host, allowing for the propagation of the endosymbiotic bacteria Xenorhabdus nematophila (Garriga et al., 2020). Shortly after infection, and before the bacteria is released from the gut of the infected nematode, there is a significant reduction in total insect hemocytes, suggesting that the nematode itself is capable of suppressing the host immune system, to the benefit of its endosymbiotic bacteria. The mechanism for immunomodulatory products from EPNs remains to be determined, however it appears to be time sensitive. After 3 h of exposure to live S. carpocapsae, insect hemocytes had reduced phagocytic activity, which was not apparent after only 1 h (Brivio et al., 2018). Identification of the specific EPN-derived molecules that target this innate immune Imd pathway in Drosophila, and whether they are conserved in mammalian parasitic nematodes could allow discovery of new anthelminthics and immunotherapies.
There are striking differences between the EPN lifecycle and that of mammalian pathogenic nematodes, most importantly the fate of the host, which is swiftly killed by EPNs in contrast to mammalian parasitic nematodes which establish chronic infections (Cooper and Eleftherianos, 2016). In order to evade the host immune system, contribute to host killing, and then feed on the dead body, the parasite must be able to successfully suppress the host immune response, release toxins, and then digest host tissue, making EPNs a strong model for identifying anti-inflammatory molecules as well as strong virulence factors. Recently, studies characterizing the specific proteins present in EPN ES revealed the remarkable resemblance to mammalian parasitic nematode-derived proteins with regards to structure and function. These include VAL proteins, enolases, serpins, and cystatins (Figure 2). For instance, Steinernema glaseri was shown to express enolases only at the activated infectious juveniles (IJs) stage, suggesting that the protein has a role in staging an infection, likely to digest the insect tissue (Liu et al., 2012). In infected insects, this secreted enolase was present in the insect hemolymph, and alone was sufficient to allow for quicker propagation of the bacteria, Xenorhabdus poinarii. Many venom proteins, with similarity to mammalian parasitic nematode VAL proteins, were identified in the ES of activated Steinernema carpocapsae and S. feltiae infective juveniles (IJs), and may contribute to the high toxicity of these parasites to their insect hosts (Lu et al., 2017; Chang et al., 2019). S. carpocapsae also expressed the serpin-like inhibitor Sc-SRP-6. Sc-SRP-6 impaired clot formation in its insect host by preventing the incorporation of melanin, known as melanization, which is an important defense mechanism in insects (Toubarro et al., 2013). Likewise, Sc-SRP-6 inhibited the hydrolysis of insect gut juices, a function that is thought to be conserved in A. ceylanicum. This serpin-like protein not only modulated the immune system, but inhibited digestion as the nematode passes through the gut of its host. Similar serpin-like genes were also found in mammalian-pathogenic nematodes, such as B. malayi (Zang et al., 1999). ES products from EPNs therefore have similar functions to those from mammalian-pathogenic nematodes, and may serve as powerful models for rapid discovery of useful targets for anthelminthics, given the comparative affordability and shorter lifecycles of EPNs. EPNs also produce cystatin, particularly when they detect insect hemolymph, as a location cue for their presence in the insect (Hao et al., 2008). Further research comparing the similarities between cystatins from EPNs and mammalian pathogenic nematodes would be valuable in validating the connections between these models.
Figure 2. Entomopathogenic nematodes as a model to identify virulent and immunomodulatory nematode-secreted molecules.
Like nematode parasites of animals, plant-parasitic nematodes (PPNs) are masters of immune modulation, most of which is mediated by their secreted proteins and molecules. Because of their devastating effects in agriculture, PPNs are well-studied, and hundreds of secreted effectors have been identified, though, similar to other nematodes, few have been studied in mechanistic detail (Rehman et al., 2016; Vieira and Gleason, 2019). A detailed discussion of PPN effectors is beyond the scope of this review, however several recent reviews focus on PPN virulence factors and host-pathogen interaction (Goverse and Smant, 2014; Rehman et al., 2016; Vieira and Gleason, 2019). Here we discuss PPN virulence factors that are shared with EPN and mammalian parasitic nematodes, including VALs and cystatins. VAL genes have been identified in many PPNs, and their expression is associated with host invasion and migration through host tissues (Lozano-Torres et al., 2014; Wilbers et al., 2018). PPN VALs have been shown to be important for modulating host immunity, especially in the early stages of infection (Kang et al., 2012; Wang et al., 2007). Several VAL-family proteins have been characterized, and they appear to modulate similar processes in both plants and animals, suggesting that mechanistic studies in one model system will be valuable to our understanding of how these effectors work in general. Many cystatin genes have been predicted in plant-parasitic nematode genomes, but little is known about their role in parasitism. A recent description of a cystatin from the pine wood nematodes (PWN) Bursaphelenchus xylophilus, found that Bx-cpi-1 is involved in the development and pathogenic process of the nematode (Xue and Wu, 2019).
The shared ancestry and parasitic behavior of these nematodes could be an explanation for their similar strategies of immune modulation. The conservation of molecular mechanisms of parasitism could allow for the identification of more proteins as well as small molecules that could be used to optimize an immune response during nematode infections, balancing an inflammatory response with worm burden. Further research will allow for ES products to be harnessed for the modulation of the immune system in mammals beyond the context of nematode infections, with applications in allergy and inflammatory diseases, without the risks of infection with live worms. The early stages of EPN infections are of particular interest, as the parasite focuses on suppressing the immune system without killing its host, prior to the release of mutualistic bacteria. This highly immunosuppressive stage may have applications with the mammal-infecting parasites that persist in their hosts for months or even years, such as hookworms and filarial nematodes (Hotez et al., 2008).
Here we discussed some of the main nematode-derived effectors in virulence and host immunomodulation. With improved high throughput technologies, further research is being conducted to identify more specific molecules that are involved in host-parasite interaction, that could provide therapeutic insight for controlling helminth infections, and at the same time curing debilitating inflammatory diseases. We also discussed EPNs and PPNs as cheaper and faster models for understanding how nematode-derived molecules influence host-pathogen interactions. The combined strategies from screening for novel nematode-derived molecules, to determining their therapeutic abilities by mechanistic studies in vertebrate, invertebrate, and plant models, and finally testing them in clinical studies will allow for faster development of anthelminthic drugs, vaccines, and therapies for allergy and inflammatory disease.
MN and SB contributed writing as well as the organization and idea to the work. AD contributed significant writing. All authors have approved it for publication.
This work was supported by the NIH (R01AI153195, R21AI142121, and R21AI135500) to MN and AD.
The authors declare that the research was conducted in the absence of any commercial or financial relationships that could be construed as a potential conflict of interest.
Anugraha, G., Jeyaprita, P. J., Madhumathi, J., Sheeba, T., and Kaliraj, P. (2013). Immune responses of B. malayi thioredoxin (TRX) and venom allergen homologue (VAH) chimeric multiple antigen for lymphatic filariasis. Acta Parasitol. 58, 468–477.
Arumugam, S., Zhan, B., Abraham, D., Ward, D., Lustigman, S., and Klei, T. R. (2014). Vaccination with recombinant Brugia malayi cystatin proteins alters worm migration., homing and final niche selection following a subcutaneous challenge of Mongolian gerbils (Meriones unguiculatus) with B. malayi infective larvae. Parasit. Vectors 7:43. doi: 10.1186/1756-3305-7-43
Asojo, O. A., Darwiche, R., Gebremedhin, S., Smant, G., Lozano-Torres, J. L., Drurey, C., et al. (2018). Heligmosomoides polygyrus venom allergen-like Protein-4 (HpVAL-4) is a sterol binding protein. Int. J. Parasitol. 48, 359–369. doi: 10.1016/j.ijpara.2018.01.002
Ayoade, K. O., Carranza, F. R., Cho, W. H., Wang, Z., Kliewer, S. A., Mangelsdorf, D. J., et al. (2020). Dafachronic acid and temperature regulate canonical dauer pathways during Nippostrongylus brasiliensis infectious larvae activation. Parasit Vectors 13:162.
Ayón-Núñez, D. A., Fragoso, G., Bobes, R. J., and Laclette, J. P. (2018). Plasminogen-binding proteins as an evasion mechanism of the host’s innate immunity in infectious diseases. Biosci. Rep. 38:BSR20180705.
Bai, X., Adams, B. J., Ciche, T. A., Clifton, S., Gaugler, R., Kim, K. S., et al. (2013). A lover and a fighter: the genome sequence of an entomopathogenic nematode Heterorhabditis bacteriophora. PLoS One 8:e69618. doi: 10.1371/journal.pone.0069618
Bancroft, A. J., Levy, C. W., Jowitt, T. A., Hayes, K. S., Thompson, S., McKenzie, E. A., et al. (2019). The major secreted protein of the whipworm parasite tethers to matrix and inhibits interleukin-13 function. Nat. Commun. 10:2344.
Batugedara, H. M., Argueta, D., Jang, J. C., Lu, D., Macchietto, M., Kaur, J., et al. (2018). Host- and helminth-derived endocannabinoids that have effects on host immunity are generated during infection. Infect. Immun. 86:e00441-18.
Behrendt, P., Arnold, P., Brueck, M., Rickert, U., Lucius, R., Hartmann, S., et al. (2016). A helminth protease inhibitor modulates the lipopolysaccharide-induced proinflammatory phenotype of microglia in vitro. Neuroimmunomodulation 23, 109–121. doi: 10.1159/000444756
Bennuru, S., Semnani, R., Meng, Z., Ribeiro, J. M., Veenstra, T. D., and Nutman, T. B. (2009). Brugia malayi excreted/secreted proteins at the host/parasite interface: stage- and gender-specific proteomic profiling. PLoS Negl. Trop. Dis. 3:e410. doi: 10.1371/journal.pntd.0000410
Bethony, J., Loukas, A., Smout, M., Brooker, S., Mendez, S., Plieskatt, J., et al. (2005). Antibodies against a secreted protein from hookworm larvae reduce the intensity of hookworm infection in humans and vaccinated laboratory animals. FASEB J. 19, 1743–1745. doi: 10.1096/fj.05-3936fje
Bisht, N., Khatri, V., Chauhan, N., and Kalyanasundaram, R. (2019). Cystatin from filarial parasites suppress the clinical symptoms and pathology of experimentally induced colitis in mice by inducing T-Regulatory Cells., B1-Cells., and alternatively activated macrophages. Biomedicines 7:85. doi: 10.3390/biomedicines7040085
Blaxter, M., and Koutsovoulos, G. (2015). The evolution of parasitism in Nematoda. Parasitology 142(Suppl. 1), S26–S39.
Bohnacker, S., Troisi, F., de Los Reyes, Jiménez, M., and Esser-von Bieren, J. (2020). What can parasites tell us about the pathogenesis and treatment of Asthma and Allergic diseases. Front. Immunol. 11:2106. doi: 10.3389/fimmu.2020.02106
Bottazzi, M. (2013). Safety and Immunogenecity of the Na-APR-1 Hookworm Vaccine in Healthy Adults. Baylor College of Medicine. (2017-2020) [Identifier: NCT03172975]. Houston, TX: USA b Department of Pediatrics
Bottazzi, M. (2017). Efficacy of Na-GST-1/Alhydrogel Hookworm Vaccine Assessed by Controlled Challenge of Infection. Baylor College of Medicine. (2013-2015) [NCT01717950]. Houston, TX: USA b Department of Pediatrics
Bouchery, T., Moyat, M., Sotillo, J., Silverstein, S., Volpe, B., Coakley, G., et al. (2020). Hookworms evade host immunity by secreting a Deoxyribonuclease to degrade neutrophil extracellular traps. Cell Host Microbe 27:277-289.e6.
Brivio, M. F., and Mastore, M. (2018). Nematobacterial complexes and insect hosts: different weapons for the same war. Insects 9:117. doi: 10.3390/insects9030117
Brivio, M. F., Toscano, A., De Pasquale, S. M., De Lerma Barbaro, A., Giovannardi, S., Finzi, G., et al. (2018). Surface protein components from entomopathogenic nematodes and their symbiotic bacteria: effects on immune responses of the greater wax moth., Galleria mellonella (Lepidoptera: Pyralidae). Pest. Manag. Sci. 74, 2089–2099. doi: 10.1002/ps.4905
Buck, A. H., Coakley, G., Simbari, F., McSorley, H. J., Quintana, J. F., Le Bihan, T., et al. (2014). Exosomes secreted by nematode parasites transfer small RNAs to mammalian cells and modulate innate immunity. Nat. Commun. 5:5488.
Chang, D. Z., Serra, L., Lu, D., Mortazavi, A., and Dillman, A. R. (2019). A core set of venom proteins is released by entomopathogenic nematodes in the genus Steinernema. PLoS Pathog 15:e1007626. doi: 10.1371/journal.ppat.1007626
Chauhan, N., Sharma, R., and Hoti, S. L. (2015). Identification and biochemical characterization of macrophage migration inhibitory factor-2 (MIF-2) homologue of human lymphatic filarial parasite. Wuchereria bancrofti. Acta Trop. 142, 71–78. doi: 10.1016/j.actatropica.2014.10.009
Cho, M. K., Lee, C. H., and Yu, H. S. (2011). Amelioration of intestinal colitis by macrophage migration inhibitory factor isolated from intestinal parasites through toll-like receptor 2. Parasite Immunol. 33, 265–275. doi: 10.1111/j.1365-3024.2010.01276.x
Chow, F. W., Koutsovoulos, G., Ovando-Vázquez, C., Neophytou, K., Bermúdez-Barrientos, J. R., Laetsch, D. R., et al. (2019). Secretion of an argonaute protein by a parasitic nematode and the evolution of its siRNA guides. Nucleic Acids Res. 47, 3594–3606. doi: 10.1093/nar/gkz142
Coakley, G., and Harris, N. L. (2020). Interactions between macrophages and helminths. Parasite Immunol. 42:e12717.
Coakley, G., Maizels, R. M., and Buck, A. H. (2015). Exosomes and other extracellular vesicles: the new communicators in parasite infections. Trends Parasitol. 31, 477–489. doi: 10.1016/j.pt.2015.06.009
Coakley, G., McCaskill, J. L., Borger, J. G., Simbari, F., Robertson, E., Millar, M., et al. (2017). Extracellular vesicles from a helminth parasite suppress macrophage activation and constitute an effective vaccine for protective immunity. Cell Rep. 19, 1545–1557. doi: 10.1016/j.celrep.2017.05.001
Cooper, D., and Eleftherianos, I. (2016). Parasitic nematode immunomodulatory strategies: recent advances and perspectives. Pathogens 5:58. doi: 10.3390/pathogens5030058
Daniłowicz-Luebert, E., Steinfelder, S., Kühl, A. A., Drozdenko, G., Lucius, R., Worm, M., et al. (2013). A nematode immunomodulator suppresses grass pollen-specific allergic responses by controlling excessive Th2 inflammation. Int. J. Parasitol. 43, 201–210. doi: 10.1016/j.ijpara.2012.10.014
Darwiche, R., Lugo, F., Drurey, C., Varossieau, K., Smant, G., Wilbers, R. H. P., et al. (2018). Crystal structure of Brugia malayi venom allergen-like protein-1 (BmVAL-1)., a vaccine candidate for lymphatic filariasis. Int. J. Parasitol. 48, 371–378. doi: 10.1016/j.ijpara.2017.12.003
de Los Reyes, Jiménez, M., Lechner, A., Alessandrini, F., Bohnacker, S., Schindela, S., et al. (2020). An anti-inflammatory eicosanoid switch mediates the suppression of type-2 inflammation by helminth larval products. Sci. Transl. Med. 12:eaay0605. doi: 10.1126/scitranslmed.aay0605
Diemert, D. J., Freire, J., Valente, V., Fraga, C. G., Talles, F., Grahek, S., et al. (2017). Safety and immunogenicity of the Na-GST-1 hookworm vaccine in Brazilian and American adults. PLoS Negl. Trop. Dis. 11:e0005574. doi: 10.1371/journal.pntd.0005574
Diemert, D. J., Pinto, A. G., Freire, J., Jariwala, A., Santiago, H., Hamilton, R. G., et al. (2012). Generalized urticaria induced by the Na-ASP-2 hookworm vaccine: implications for the development of vaccines against helminths. J. Allergy Clin. Immunol. 130:169-76.e6.
Długosz, E., Basałaj, K., and Zawistowska-Deniziak, A. (2019). Cytokine production and signalling in human THP-1 macrophages is dependent on Toxocara canis glycans. Parasitol. Res. 118, 2925–2933. doi: 10.1007/s00436-019-06405-8
Doligalska, M., Joźwicka, K., Laskowska, M., Donskow-Łysoniewska, K., Pa̧czkowski, C., and Janiszowska, W. (2013). Changes in Heligmosomoides polygyrus glycoprotein pattern by saponins impact the BALB/c mice immune response. Exp. Parasitol. 135, 524–531. doi: 10.1016/j.exppara.2013.09.005
Eichenberger, R. M., Ryan, S., Jones, L., Buitrago, G., Polster, R., Montes, et al. (2018). Hookworm secreted extracellular vesicles interact with host cells and prevent inducible colitis in mice. Front. Immunol. 9:850. doi: 10.3389/fimmu.2018.00850
Ellis, S., Matthews, J. B., Shaw, D. J., Paterson, S., McWilliam, H. E., Inglis, N. F., et al. (2014). Ovine IgA-reactive proteins from teladorsagia circumcincta infective larvae. Int. J. Parasitol. 44, 743–750. doi: 10.1016/j.ijpara.2014.05.007
Filbey, K. J., Varyani, F., Harcus, Y., Hewitson, J. P., Smyth, D. J., McSorley, H. J., et al. (2019). Macrophage Migration Inhibitory Factor (MIF) is essential for type 2 effector cell immunity to an intestinal helminth parasite. Front. Immunol. 10:2375. doi: 10.3389/fimmu.2019.02375
Flynn, A. F., Joyce, M. G., Taylor, R. T., Bennuru, S., Lindrose, A. R., Sterling, S. L., et al. (2019). Intestinal UDP-glucuronosyltransferase as a potential target for the treatment and prevention of lymphatic filariasis. PLoS Negl. Trop. Dis. 13:e0007687. doi: 10.1371/journal.pntd.0007687
Galles, C., Prez, G. M., Penkov, S., Boland, S., Porta, E. O. J., Altabe, S. G., et al. (2018). Endocannabinoids in caenorhabditis elegans are essential for the mobilization of cholesterol from internal reserves. Sci. Rep. 8:6398.
Garriga, A., Mastore, M., Morton, A., Pino, F. G. D., and Brivio, M. F. (2020). Immune response of Drosophila suzukii larvae to infection with the nematobacterial complex steinernema Carpocapsae-Xenorhabdus nematophila. Insects 11:210. doi: 10.3390/insects11040210
Gazzinelli-Guimaraes, P. H., and Nutman, T. B. (2018). Helminth parasites and immune regulation. F1000Res 7:F1000FacultyRev-1685.
Goverse, A., and Smant, G. (2014). The activation and suppression of plant innate immunity by parasitic nematodes. Annu. Rev. Phytopathol. 52, 243–265. doi: 10.1146/annurev-phyto-102313-050118
Gregory, W. F., and Maizels, R. M. (2008). Cystatins from filarial parasites: evolution., adaptation and function in the host-parasite relationship. Int. J. Biochem. Cell Biol. 40, 1389–1398. doi: 10.1016/j.biocel.2007.11.012
Gu, H. Y., Marks, N. D., Winter, A. D., Weir, W., Tzelos, T., McNeilly, T. N., et al. (2017). Conservation of a microRNA cluster in parasitic nematodes and profiling of miRNAs in excretory-secretory products and microvesicles of Haemonchus contortus. PLoS Negl. Trop. Dis. 11:e0006056. doi: 10.1371/journal.pntd.0006056
Hansen, E. P., Fromm, B., Andersen, S. D., Marcilla, A., Andersen, K. L., Borup, A., et al. (2019). Exploration of extracellular vesicles from Ascaris suum provides evidence of parasite-host cross talk. J. Extracell Vesicles 8:1578116. doi: 10.1080/20013078.2019.1578116
Hao, Y. J., Montiel, R., Nascimento, G., Toubarro, D., and Simoes, N. (2008). Identification., characterization of functional candidate genes for host-parasite interactions in entomopathogenetic nematode Steinernema carpocapsae by suppressive subtractive hybridization. Parasitol Res. 103, 671–683. doi: 10.1007/s00436-008-1030-4
Harischandra, H., Yuan, W., Loghry, H. J., Zamanian, M., and Kimber, M. J. (2018). Profiling extracellular vesicle release by the filarial nematode Brugia malayi reveals sex-specific differences in cargo and a sensitivity to ivermectin. PLoS Negl. Trop. Dis. 12:e0006438. doi: 10.1371/journal.pntd.0006438
Hartmann, S., and Lucius, R. (2003). Modulation of host immune responses by nematode cystatins. Int. J. Parasitol. 33, 1291–1302. doi: 10.1016/s0020-7519(03)00163-2
Hartmann, S., Schönemeyer, A., Sonnenburg, B., Vray, B., and Lucius, R. (2002). Cystatins of filarial nematodes up-regulate the nitric oxide production of interferon-gamma-activated murine macrophages. Parasite Immunol. 24, 253–262. doi: 10.1046/j.1365-3024.2002.00459.x
Hertz, M. I., Glaessner, P. M., Rush, A., and Budge, P. J. (2020). Brugia malayi galectin 2 is a tandem-repeat type galectin capable of binding mammalian polysaccharides. Mol. Biochem. Parasitol. 235:111233. doi: 10.1016/j.molbiopara.2019.111233
Hewitson, J. P., Filbey, K. J., Esser-von Bieren, J., Camberis, M., Schwartz, C., Murray, J., et al. (2015). Concerted activity of IgG1 antibodies and IL-4/IL-25-dependent effector cells trap helminth larvae in the tissues following vaccination with defined secreted antigens., providing sterile immunity to challenge infection. PLoS Pathog 11:e1004676. doi: 10.1371/journal.ppat.1004676
Hewitson, J. P., Harcus, Y., Murray, J., van Agtmaal, M., Filbey, K. J., Grainger, J. R., et al. (2011). Proteomic analysis of secretory products from the model gastrointestinal nematode Heligmosomoides polygyrus reveals dominance of venom allergen-like (VAL) proteins. J. Proteomics. 74, 1573–1594. doi: 10.1016/j.jprot.2011.06.002
Hodžić, A., Mateos-Hernández, L., Fréalle, E., Román-Carrasco, P., Alberdi, P., Pichavant, M., et al. (2020). Infection with Toxocara canis inhibits the production of IgE antibodies to α-Gal in humans: towards a conceptual framework of the hygiene hypothesis? Vaccines (Basel) 8:167. doi: 10.3390/vaccines8020167
Hokke, C. H., and van Diepen, A. (2017). Helminth glycomics - glycan repertoires and host-parasite interactions. Mol. Biochem. Parasitol. 215, 47–57. doi: 10.1016/j.molbiopara.2016.12.001
Homan, E. J., and Bremel, R. D. (2018). A role for epitope networking in immunomodulation by helminths. Front. Immunol. 9:1763. doi: 10.3389/fimmu.2018.01763
Honda, T., and Kabashima, K. (2019). Prostanoids and leukotrienes in the pathophysiology of atopic dermatitis and psoriasis. Int. Immunol. 31, 589–595. doi: 10.1093/intimm/dxy087
Hotez, P. J., Brindley, P. J., Bethony, J. M., King, C. H., Pearce, E. J., and Jacobson, J. (2008). Helminth infections: the great neglected tropical diseases. J. Clin. Invest. 118, 1311–1321. doi: 10.1172/jci34261
Hotez, P. J., Diemert, D., Bacon, K. M., Beaumier, C., Bethony, J. M., Bottazzi, M. E., et al. (2013). The human hookworm vaccine. Vaccine 31(Suppl. 2), B227–B232.
Jiang, P., Zao, Y. J., Yan, S. W., Song, Y. Y., Yang, D. M., Dai, L. Y., et al. (2019). Molecular characterization of a Trichinella spiralis enolase and its interaction with the host’s plasminogen. Vet. Res. 50:106.
Johnston, C. J. C., Smyth, D. J., Kodali, R. B., White, M. P. J., Harcus, Y., Filbey, K. J., et al. (2017). A structurally distinct TGF-β mimic from an intestinal helminth parasite potently induces regulatory T cells. Nat. Commun. 8:1741.
Kalyanasundaram, R., and Balumuri, P. (2011). Multivalent vaccine formulation with BmVAL-1 and BmALT-2 confer significant protection against challenge infections with Brugia malayi in mice and jirds. Res. Rep. Trop. Med. 2011, 45–56. doi: 10.2147/rrtm.s13679
Kang, J. S., Koh, Y. H., Moon, Y. S., and Lee, S. H. (2012). Molecular properties of a venom allergen-like protein suggest a parasitic function in the pinewood nematode Bursaphelenchus xylophilus. Int. J. Parasitol. 42, 63–70. doi: 10.1016/j.ijpara.2011.10.006
Kang, S. A., Park, M. K., Park, S. K., Choi, J. H., Lee, D. I., Song, S. M., et al. (2019). Adoptive transfer of Trichinella spiralis-activated macrophages can ameliorate both Th1- and Th2-activated inflammation in murine models. Sci. Rep. 9:6547.
Kenney, E., Hawdon, J. M., O’Halloran, D., and Eleftherianos, I. (2019). Heterorhabditis bacteriophora excreted-secreted products enable infection by Photorhabdus luminescens through suppression of the imd pathway. Front. Immunol. 10:2372. doi: 10.3389/fimmu.2019.02372
King, T. P., and Valentine, M. D. (1987). Allergens of hymenopteran venoms. Clin. Rev. Allergy 5, 137–148.
Kobpornchai, P., Flynn, R. J., Reamtong, O., Rittisoonthorn, N., Kosoltanapiwat, N., Boonnak, K., et al. (2020). A novel cystatin derived from Trichinella spiralis suppresses macrophage-mediated inflammatory responses. PLoS Negl. Trop. Dis. 14:e0008192. doi: 10.1371/journal.pntd.0008192
Liu, H., Zeng, H., Yao, Q., Yuan, J., Zhang, Y., Qiu, D., et al. (2012). Steinernema glaseri surface enolase: molecular cloning., biological characterization., and role in host immune suppression. Mol. Biochem. Parasitol. 185, 89–98. doi: 10.1016/j.molbiopara.2012.06.006
Lozano-Torres, J. L., Wilbers, R. H., Warmerdam, S., Finkers-Tomczak, A., Diaz-Granados, A., van Schaik, C. C., et al. (2014). Apoplastic venom allergen-like proteins of cyst nematodes modulate the activation of basal plant innate immunity by cell surface receptors. PLoS Pathog 10:e1004569. doi: 10.1371/journal.ppat.1004569
Lu, D., Macchietto, M., Chang, D., Barros, M. M., Baldwin, J., Mortazavi, A., et al. (2017). Activated entomopathogenic nematode infective juveniles release lethal venom proteins. PLoS Pathog 13:e1006302. doi: 10.1371/journal.ppat.1006302
Ma, G., Wang, T., Korhonen, P. K., Hofmann, A., Sternberg, P. W., Young, N. D., et al. (2020). Elucidating the molecular and developmental biology of parasitic nematodes: moving to a multiomics paradigm. Adv. Parasitol. 108, 175–229. doi: 10.1016/bs.apar.2019.12.005
Ma, G., Wang, T., Korhonen, P. K., Stroehlein, A. J., Young, N. D., and Gasser, R. B. (2019a). Dauer signalling pathway model for Haemonchus contortus. Parasit Vectors 12:187.
Ma, G., Wang, T., Korhonen, P. K., Young, N. D., Nie, S., Ang, C. S., et al. (2019b). Dafachronic acid promotes larval development in Haemonchus contortus by modulating dauer signalling and lipid metabolism. PLoS Pathog 15:e1007960. doi: 10.1371/journal.ppat.1007960
MacDonald, A. J., Cao, L., He, Y., Zhao, Q., Jiang, S., and Lustigman, S. (2005). rOv-ASP-1., a recombinant secreted protein of the helminth Onchocercavolvulus. is a potent adjuvant for inducing antibodies to ovalbumin., HIV-1 polypeptide and SARS-CoV peptide antigens. Vaccine 23, 3446–3452. doi: 10.1016/j.vaccine.2005.01.098
Maizels, R. M. (2020). Regulation of immunity and allergy by helminth parasites. Allergy 75, 524–534. doi: 10.1111/all.13944
Maizels, R. M., Sartono, E., Kurniawan, A., Partono, F., Selkirk, M. E., and Yazdanbakhsh, M. (1995). T-cell activation and the balance of antibody isotypes in human lymphatic filariasis. Parasitol. Today 11, 50–56. doi: 10.1016/0169-4758(95)80116-2
Maizels, R. M., Smits, H. H., and McSorley, H. J. (2018). Modulation of host immunity by helminths: the expanding repertoire of parasite effector molecules. Immunity 49, 801–818. doi: 10.1016/j.immuni.2018.10.016
Martini, F., Eckmair, B., Štefaniæ, S., Jin, C., Garg, M., Yan, S., et al. (2019). Highly modified and immunoactive N-glycans of the canine heartworm. Nat. Commun. 10:75.
McSorley, H. J., Grainger, J. R., Harcus, Y., Murray, J., Nisbet, A. J., Knox, D. P., et al. (2010). daf-7-related TGF-beta homologues from Trichostrongyloid nematodes show contrasting life-cycle expression patterns. Parasitology 137, 159–171. doi: 10.1017/s0031182009990321
McSorley, H. J., Hewitson, J. P., and Maizels, R. M. (2013). Immunomodulation by helminth parasites: defining mechanisms and mediators. Int. J. Parasitol. 43, 301–310. doi: 10.1016/j.ijpara.2012.11.011
Murray, J., Gregory, W. F., Gomez-Escobar, N., Atmadja, A. K., and Maizels, R. M. (2001). Expression and immune recognition of Brugia malayi VAL-1., a homologue of vespid venom allergens and Ancylostoma secreted proteins. Mol. Biochem. Parasitol. 118, 89–96. doi: 10.1016/s0166-6851(01)00374-7
Navarro, S., Pickering, D. A., Ferreira, I. B., Jones, L., Ryan, S., Troy, S., et al. (2016). Hookworm recombinant protein promotes regulatory T cell responses that suppress experimental asthma. Sci. Transl. Med. 8:362ra143. doi: 10.1126/scitranslmed.aaf8807
Oakes, M. D., Law, W. J., Clark, T., Bamber, B. A., and Komuniecki, R. (2017). Cannabinoids activate monoaminergic signaling to modulate Key C. elegans behaviors. J. Neurosci. 37, 2859–2869. doi: 10.1523/jneurosci.3151-16.2017
Ogawa, A., Streit, A., Antebi, A., and Sommer, R. J. (2009). A conserved endocrine mechanism controls the formation of dauer and infective larvae in nematodes. Curr. Biol. 19, 67–71. doi: 10.1016/j.cub.2008.11.063
Osbourn, M., Soares, D. C., Vacca, F., Cohen, E. S., Scott, I. C., Gregory, W. F., et al. (2017). HpARI protein secreted by a helminth parasite suppresses interleukin-33. Immunity 47, 739–751.e5.
Patton, J. B., Bonne-Année, S., Deckman, J., Hess, J. A., Torigian, A., Nolan, T. J., et al. (2018). Methylprednisolone acetate induces., and Δ7-dafachronic acid suppresses., Strongyloides stercoralis hyperinfection in NSG mice. Proc. Natl. Acad. Sci. U S A. 115, 204–209. doi: 10.1073/pnas.1712235114
Periago, M. V., and Bethony, J. M. (2012). Hookworm virulence factors: making the most of the host. Microbes Infect. 14, 1451–1464. doi: 10.1016/j.micinf.2012.09.002
Pineda, M. A., Lumb, F., Harnett, M. M., and Harnett, W. (2014). ES-62., a therapeutic anti-inflammatory agent evolved by the filarial nematode Acanthocheilonema viteae. Mol. Biochem. Parasitol. 194, 1–8. doi: 10.1016/j.molbiopara.2014.03.003
Prasanphanich, N. S., Mickum, M. L., Heimburg-Molinaro, J., and Cummings, R. D. (2013). Glycoconjugates in host-helminth interactions. Front. Immunol. 4:240. doi: 10.3389/fimmu.2013.00240
Quintana, J. F., Makepeace, B. L., Babayan, S. A., Ivens, A., Pfarr, K. M., Blaxter, M., et al. (2015). Extracellular Onchocerca-derived small RNAs in host nodules and blood. Parasit Vectors 8:58. doi: 10.1186/s13071-015-0656-1
Reamtong, O., Rujimongkon, K., Sookrung, N., Saeung, A., Thiangtrongjit, T., Sakolvaree, Y., et al. (2019). Immunome and immune complex-forming components of Brugia malayi identified by microfilaremic human sera. Exp. Parasitol. 200, 92–98. doi: 10.1016/j.exppara.2019.04.005
Rehman, S., Gupta, V. K., and Goyal, A. K. (2016). Identification and functional analysis of secreted effectors from phytoparasitic nematodes. BMC Microbiol. 16:48. doi: 10.1186/s12866-016-0632-8
Rubin, G. M., Yandell, M. D., Wortman, J. R., Gabor Miklos, G. L., Nelson, C. R., Hariharan, I. K., et al. (2000). Comparative genomics of the eukaryotes. Science 287, 2204–2215. doi: 10.1126/science.287.5461.2204
Ryan, S. M., Eichenberger, R. M., Ruscher, R., Giacomin, P. R., and Loukas, A. (2020). Harnessing helminth-driven immunoregulation in the search for novel therapeutic modalities. PLoS Pathog 16:e1008508. doi: 10.1371/journal.ppat.1008508
Saeed, M., Imran, M., Baig, M. H., Kausar, M. A., Shahid, S., and Ahmad, I. (2018). Virtual screening of natural anti-filarial compounds against glutathione-S-transferase of Brugia malayi and Wuchereria bancrofti. Cell Mol. Biol. (Noisy-le-grand). 64, 69–73. doi: 10.14715/cmb/2018.64.13.13
Shah, A. N., and Saldana, M. (2010). Images in clinical medicine. Ocular loiasis. N. Engl. J. Med. 363, e16. doi: 10.1056/nejmicm1002020
Shears, R. K., Bancroft, A. J., Hughes, G. W., Grencis, R. K., and Thornton, D. J. (2018). Extracellular vesicles induce protective immunity against Trichuris muris. Parasite Immunol. 40:e12536. doi: 10.1111/pim.12536
Smallwood, T. B., Giacomin, P. R., Loukas, A., Mulvenna, J. P., Clark, R. J., and Miles, J. J. (2017). Helminth immunomodulation in autoimmune disease. Front. Immunol. 8:453. doi: 10.3389/fimmu.2017.00453
Suckling, C. J., Alam, S., Olson, M. A., Saikh, K. U., Harnett, M. M., and Harnett, W. (2018). Small molecule analogues of the parasitic worm product ES-62 interact with the TIR domain of MyD88 to inhibit pro-inflammatory signalling. Sci. Rep. 8:2123.
Tawe, W., Pearlman, E., Unnasch, T. R., and Lustigman, S. (2000). Angiogenic activity of Onchocerca volvulus recombinant proteins similar to vespid venom antigen 5. Mol. Biochem. Parasitol. 109, 91–99. doi: 10.1016/s0166-6851(00)00231-0
Toubarro, D., Avila, M. M., Hao, Y., Balasubramanian, N., Jing, Y., Montiel, R., et al. (2013). A serpin released by an entomopathogen impairs clot formation in insect defense system. PLoS One 8:e69161. doi: 10.1371/journal.pone.0069161
Tritten, L., O’Neill, M., Nutting, C., Wanji, S., Njouendoui, A., Fombad, F., et al. (2014). Loa loa and Onchocerca ochengi miRNAs detected in host circulation. Mol. Biochem. Parasitol. 198, 14–17. doi: 10.1016/j.molbiopara.2014.11.001
Tritten, L., Tam, M., Vargas, M., Jardim, A., Stevenson, M. M., Keiser, J., et al. (2017). Excretory/secretory products from the gastrointestinal nematode Trichuris muris. Exp. Parasitol. 178, 30–36. doi: 10.1016/j.exppara.2017.05.003
Vacca, F., Chauché, C., Jamwal, A., Hinchy, E. C., Heieis, G., Webster, H., et al. (2020). A helminth-derived suppressor of ST2 blocks allergic responses. Elife 9:e54017.
Valdivieso, E., Perteguer, M. J., Hurtado, C., Campioli, P., Rodríguez, E., Saborido, A., et al. (2015). ANISERP: a new serpin from the parasite anisakis simplex. Parasit Vectors 8:399.
van Stijn, C. M., van den Broek, M., Vervelde, L., Alvarez, R. A., Cummings, R. D., Tefsen, B., et al. (2010). Vaccination-induced IgG response to Galalpha1-3GalNAc glycan epitopes in lambs protected against Haemonchus contortus challenge infection. Int. J. Parasitol. 40, 215–222. doi: 10.1016/j.ijpara.2009.07.009
Vieira, P., and Gleason, C. (2019). Plant-parasitic nematode effectors - insights into their diversity and new tools for their identification. Curr. Opin. Plant Biol. 50, 37–43. doi: 10.1016/j.pbi.2019.02.007
Wang, X., Li, H., Hu, Y., Fu, P., and Xu, J. (2007). Molecular cloning and analysis of a new venom allergen-like protein gene from the root-knot nematode Meloidogyne incognita. Exp. Parasitol. 117, 133–140. doi: 10.1016/j.exppara.2007.03.017
Wang, Y., Bai, X., Zhu, H., Wang, X., Shi, H., Tang, B., et al. (2017a). Immunoproteomic analysis of the excretory-secretory products of Trichinella pseudospiralis adult worms and newborn larvae. Parasit Vectors 10:579.
Wang, Y., Lu, M., Wang, S., Ehsan, M., Yan, R., Song, X., et al. (2017b). Characterization of a secreted macrophage migration inhibitory factor homologue of the parasitic nematode haemonchus contortus acting at the parasite-host cell interface. Oncotarget 8, 40052–40064. doi: 10.18632/oncotarget.16675
Wang, Y., Wu, L., Liu, X., Wang, S., Ehsan, M., Yan, R., et al. (2017c). Characterization of a secreted cystatin of the parasitic nematode Haemonchus contortus and its immune-modulatory effect on goat monocytes. Parasit Vectors 10:425.
White, M. P. J., McManus, C. M., and Maizels, R. M. (2020). Regulatory T-cells in helminth infection: induction., function and therapeutic potential. Immunology 160, 248–260. doi: 10.1111/imm.13190
Wilbers, R. H. P., Schneiter, R., Holterman, M. H. M., Drurey, C., Smant, G., Asojo, O. A., et al. (2018). Secreted venom allergen-like proteins of helminths: conserved modulators of host responses in animals and plants. PLoS Pathog 14:e1007300. doi: 10.1371/journal.ppat.1007300
Xue, Q., and Wu, X. Q. (2019). Characteristics and function of a novel cystatin gene in the pine wood nematode Bursaphelenchus xylophilus. Biol. Open 8:bio042655. doi: 10.1242/bio.042655
Yang, Y., Liu, L., Liu, X., Zhang, Y., Shi, H., Jia, W., et al. (2020). Extracellular vesicles derived from trichinella spiralis muscle larvae ameliorate TNBS-induced colitis in mice. Front. Immunol. 11:1174. doi: 10.3389/fimmu.2020.01174
Yazdanbakhsh, M., Kremsner, P. G., and van Ree, R. (2002). Allergy., parasites., and the hygiene hypothesis. Science 296, 490–494. doi: 10.1126/science.296.5567.490
Yi, D., Xu, L., Yan, R., and Li, X. (2010). Haemonchus contortus: cloning and characterization of serpin. Exp. Parasitol. 125, 363–370. doi: 10.1016/j.exppara.2010.03.002
Zakeri, A., Hansen, E. P., Andersen, S. D., Williams, A. R., and Nejsum, P. (2018). Immunomodulation by helminths: intracellular pathways and extracellular vesicles. Front. Immunol. 9:2349. doi: 10.3389/fimmu.2018.02349
Zang, X., Atmadja, A. K., Gray, P., Allen, J. E., Gray, C. A., Lawrence, R. A., et al. (2000). The serpin secreted by Brugia malayi microfilariae., Bm-SPN-2., elicits strong., but short-lived., immune responses in mice and humans. J. Immunol. 165, 5161–5169. doi: 10.4049/jimmunol.165.9.5161
Zang, X., Yazdanbakhsh, M., Jiang, H., Kanost, M. R., and Maizels, R. M. (1999). A novel serpin expressed by blood-borne microfilariae of the parasitic nematode Brugia malayi inhibits human neutrophil serine proteinases. Blood 94, 1418–1428. doi: 10.1182/blood.v94.4.1418
Zhan, B., Perally, S., Brophy, P. M., Xue, J., Goud, G., Liu, S., et al. (2010). Molecular cloning., biochemical characterization., and partial protective immunity of the heme-binding glutathione S-transferases from the human hookworm Necator americanus. Infect. Immun. 78, 1552–1563. doi: 10.1128/iai.00848-09
Keywords: entomopathogenic nematode, inflammatory disorders, vaccination, excretory and secretory products, soil-transmitted helminth
Citation: Bobardt SD, Dillman AR and Nair MG (2020) The Two Faces of Nematode Infection: Virulence and Immunomodulatory Molecules From Nematode Parasites of Mammals, Insects and Plants. Front. Microbiol. 11:577846. doi: 10.3389/fmicb.2020.577846
Received: 30 June 2020; Accepted: 02 November 2020;
Published: 02 December 2020.
Edited by:
Mark C. Siracusa, Rutgers Biomedical and Health Sciences, United StatesReviewed by:
Rick M. Maizels, University of Edinburgh, United KingdomCopyright © 2020 Bobardt, Dillman and Nair. This is an open-access article distributed under the terms of the Creative Commons Attribution License (CC BY). The use, distribution or reproduction in other forums is permitted, provided the original author(s) and the copyright owner(s) are credited and that the original publication in this journal is cited, in accordance with accepted academic practice. No use, distribution or reproduction is permitted which does not comply with these terms.
*Correspondence: Meera G. Nair, bWVlcmEubmFpckBtZWRzY2gudWNyLmVkdQ==
Disclaimer: All claims expressed in this article are solely those of the authors and do not necessarily represent those of their affiliated organizations, or those of the publisher, the editors and the reviewers. Any product that may be evaluated in this article or claim that may be made by its manufacturer is not guaranteed or endorsed by the publisher.
Research integrity at Frontiers
Learn more about the work of our research integrity team to safeguard the quality of each article we publish.