- 1School of Biological Sciences and Institute of Microbiology, Seoul National University, Seoul, South Korea
- 2Department of Biology and Chemistry, Changwon National University, Changwon, South Korea
- 3Science Unit, Lingnan University, Tuen Mun, Hong Kong
- 4Forest Biodiversity Division, Korea National Arboretum, Pocheon, South Korea
Trees in forest ecosystems constantly interact with the soil fungal community, and this interaction plays a key role in nutrient cycling. The diversity of soil fungal communities is affected by both environmental factors and host tree species. We investigated the influence of both of these factors by examining the total fungal communities in the rhizospheric soil of climax tree species that have similar ecological roles (Carpinus cordata, an ectomycorrhizal [ECM] tree, and Fraxinus rhynchophylla, an arbuscular mycorrhizal [AM] tree) in temperate forests with continental climates of Mt. Jeombong, South Korea. Fungal communities were assessed by Illumina-MiSeq sequencing the internal transcribed spacer (ITS) region of environmental DNA, and comparing their environmental factors (season and soil properties). We found that soil fungi of the two forest types differed in terms of community structure and ecological guild composition. The total fungal community composition changed significantly with seasons and soil properties in the F. rhynchophylla forest, but not in the C. cordata forest. However, potassium and carbon were significantly correlated with fungal diversity in both forests, and a positive correlation was found only between symbiotrophs of C. cordata and the carbon to nitrogen (C/N) ratio. Thus, the effects of environmental factors on soil fungal communities depended on the host trees, but some factors were common in both forests. Our results indicate that individual tree species should be considered when anticipating how the fungal communities will respond to environmental change.
Introduction
Fungi in forests play key roles in plant diversity and productivity (Van Der Heijden et al., 2008). There is a wide diversity of associations between fungi and plants categorized by how a fungus gets organic matter from plants – symbiotrophic, saprotrophic, and pathotrophic. Among the symbiotrophic fungi, mycorrhizal fungi receive photosynthetic products from plant in exchange for mediating environmental stresses, increasing foraging area, and enhancing water and nutrient absorption through a hyphal network with plants (Smith and Read, 2010; Kramer et al., 2012). Most terrestrial plants require at least one type of mycorrhizal association to properly grow and reproduce (Brundrett and Tedersoo, 2018). Saprotrophic fungi decompose organic matter and are involved in carbon cycling and nutrient mobilization in forests (Kramer et al., 2012; van der Wal et al., 2013), while pathotrophic fungi retrieve nutrients by harming living plants and can control plant populations (Nguyen et al., 2016). Each trophic mode can be divided to several guilds according to their ecological lifestyle (Tedersoo et al., 2014).
Plants can also influence fungal communities by modifying microhabitats with leaf fall and litter (Aponte et al., 2010). The soils surrounding plants have different properties due to decomposition of organic matter, accumulation of carbon, and microbial residues such as amino sugar (Soudzilovskaia et al., 2015; Lin et al., 2017; Craig et al., 2018). This phenomenon results in different carbon and nitrogen cycling rates between host tree species, such as slower carbon and nitrogen cycling in ectomycorrhizal (ECM) forests than in arbuscular mycorrhizal (AM) forests (Phillips et al., 2013; Averill et al., 2014; Sulman et al., 2017). Trees can be divided into four types based on mycorrhizal associations, and the most abundant types being AM and ECM trees (Brundrett, 2009). AM trees include species in the genera Acer and Ulmus and family Podocarpaceae and have symbiotic relationships with fungal species in Glomeromycota (Brundrett, 2009; Helgason and Fitter, 2009). ECM trees include species in the families, such as Betulaceae, Fagaceae, and Pinaceae, and are associated with fungi in Ascomycota and Basidiomycota (Brundrett, 2009; Tedersoo et al., 2010). ECM forests are known to store more carbon and have a higher carbon to nitrogen (C/N) ratio than AM forests (Vesterdal et al., 2013; Averill et al., 2014). However, it should be noted that plants with dual mycorrhization of AM and ECM have been reported (Brundrett, 2017; Tedersoo and Brundrett, 2017).
The heartleaf hornbeam (Carpinus cordata) is an ECM deciduous tree native to northeast Asia that grows in shaded, moist valley forests (Smith and Read, 2010; Kwon et al., 2014). Fraxinus rhynchophylla, a species of ash tree distributed in East Asia, grows well on nutrient-rich soils and is an AM tree (Ambriz et al., 2010). Both C. cordata and F. rhynchophylla are climax species commonly found in Korean temperate forests (Lee et al., 1990; Cho et al., 2005; Park et al., 2016). Previous studies have helped us begin to understand the relationship between fungi and species of Carpinus and Fraxinus. For instance, study on ECM fungi associated with Fagus, Tilia, and Carpinus trees suggested the host preference of ECM fungi, as more than half of species were found in one host (Lang et al., 2011). Meanwhile, the influence of abiotic factors on fungal communities were found in pure F. mandshurica forest, where higher relative abundance of saprotophic fungal were found in soil with higher carbon and nitrogen compared to that of mixed forest of P. koraiensis and F. mandshurica (Wu et al., 2019).
Carpinus cordata and F. rhynchophylla climax forests are both found in well-protected valleys in Korea (Cho et al., 2005; Park et al., 2016). This characteristic provides a unique opportunity to compare fungal communities across soil properties and tree types (ECM vs. AM trees), while controlling for environmental variation because the forests are located so close to each other. This study is part of a larger project by the Korea National Arboretum to understand the interactions between fungi and major tree species in Korea. Various features of the fungal community (alpha diversity, community structure, and ecological guild composition) from the two forest types were compared to determine seasonal variation and the influence of soil properties. We aimed to elucidate the influence of host tree type, in particular AM and ECM trees, on soil fungal communities, while also investigating the effects of season and soil properties (pH, carbon, nitrogen, phosphorus, potassium, and water content).
Materials and Methods
Study Site, Soil Collection, and Chemical Analyses
The study was carried out in temperate forests of Mt. Jeombong, Seoraksan National Park, Inje County, Gangwon Province, South Korea (38.032124, 128.463275, altitude 780–830 m). Sampling was permitted by the Seoraksan National Park Authority. We choose one C. cordata forest and one F. rhynchophylla forest that were adjacent (within 350 m) to minimize the effects of environmental variation. Several shrubs belonging to Ericaceae were found in each sampling site, but forests were composed of single tree species in each stand. This approach allowed us to focus on seasonal variation and the influence of soil properties on the fungal communities. We sampled soil from three individual trees from each forest for each of the four seasons in 2018; selected trees were visually inspected to have no disease symptoms and were at least 20 m away from other sampled trees. Rhizospheric soil samples were collected in triplicate for each tree, from 0 to 10 cm soil layer that was close to root of the host tree, after removing surface litter. In total, 36 soil samples were collected from each forest type (three trees × three replicates × four seasons). Soil samples were placed on ice while being transported to the laboratory. Upon arrival, triplicate soil samples from the same tree were mixed and sieved to 2.0 mm (= 12 sample × 2 forest types = 24 total samples). Half of each Mixed soil sample was sent to National Instrumentation Center for Environmental Management (Seoul National University, Seoul, South Korea) for soil property analysis on the same day of collection. The following soil properties were measured: pH, total organic carbon (TOC), total nitrogen (TN), NH4+, total phosphorus (TP), water content, total potassium (TK), and C/N ratio (based on TOC/TN). The remaining half of each sample was stored at −80°C until DNA extraction.
DNA Extraction, Amplification, and Sequencing
DNA was extracted from each of the 72 soil samples (two forest types × three trees × three replicates of soil mixture × four seasons) within a week of sampling. Extraction was performed from 0.25 g of soil sample with the Power-Soil® DNA Isolation Kit according to the manufacturer’s instructions (MoBio Laboratories, Carlsbad, CA). After extraction, the internal transcribed spacer 2 (ITS2) region was amplified using the primers ITS3 and ITS4 (White et al., 1990) attached with Illumina sequencing adaptors. To minimize the effects of PCR bias, PCR was conducted three times for each DNA sample using an AccuPower PCR PreMix Kit (Bioneer, Deajeon, South Korea). We used the following PCR conditions: 94°C for 5 min; 30 cycles of 94°C for 30 s, 55°C for 30 s, and 72°C for 40 s; and a final extension of 72°C for 10 min. The size of PCR products was visually checked on 1% agarose gel (BIOFACT, Daejeon, South Korea). Triplicate PCR products for a sample were pooled and purified using an Expin™ PCR SV Kit (GeneAll Biotechnology, Seoul, South Korea). To attach multiple index delimiters (MID), we followed the Nextera XT Index Kit protocol (Illumina, San Diego, CA, USA). After purification, DNA quality and concentration from PCR products were quantified with NanoDrop 2000 (Thermo Fisher Scientific, Waltham, MA, USA) and pooled at equimolar concentrations before sequencing. The 72 samples were sequenced by 300 bp × 2 paired-end sequencing on an Illumina MiSeq platform (Macrogen, Seoul, South Korea). Raw sequence data were deposited on NCBI Sequence Read Archive (SRA) under Project ID PRJNA638267.
Bioinformatics and Statistical Analyses
All sequence analyses were performed using the Quantitative Insights Into Microbial Ecology (QIIME) v.1.8.0. pipeline (Caporaso et al., 2010). We used fastq-join to merge paired-end sequences and filter out low-quality sequences (Q < 20, length < 200 bp). operational taxonomic units (OTUs) were clustered based on a 97% similarity threshold using an average linkage method on USEARCH v. 5.2.236 (Edgar, 2010). For taxonomic assignments, the most abundant sequence was selected as the representative sequence of an OTU. Reference sequences from UNITE v. 7.2 (Kõljalg et al., 2013) and Seoul National University Fungal Collection (SFC) were used for taxonomic assignment, following the criteria of Tedersoo et al. (2014). Chimeric sequences were filtered against the reference database with UCHIME (Edgar et al., 2011). Singleton OTUs and non-fungal sequences were removed and all samples were rarified to the minimum number of sequences (30,000 reads) and pooled (90,000 reads) before further analysis. Fungal functional guilds were assigned according to the FUNGuild database (Nguyen et al., 2016). The relative abundance of each OTU was calculated as the ratio of the number of sequences reads of an OTU per total sequence reads in a batch of samples.
Four alpha diversity indices (Chao1 richness, Shannon’s diversity and equitability, and Good’s coverage) were calculated in QIIME and displayed with R software (R Core Team, 2019). A Kruskal-Wallis test was performed to identify the statistically significant relationships between the alpha diversity indices and season with Fisher’s least significant difference (LSD) test as a post hoc test adjusted by the Bonferroni method using the agricolae package (de Mendiburu and de Mendiburu, 2019). Variation in fungal communities among soil samples was visualized by a principal coordinate analysis (PCoA) based on Bray-Curtis dissimilarity index using the phyloseq package (McMurdie and Holmes, 2013). Vectors of soil property factors were fit with the envfit function in the vegan package. To determine the significance of the variations, differences in fungal community compositions across seasons and host trees were evaluated using permutational multivariate analysis of variance (PERMANOVA) with 999 permutations, implemented as “adonis” in the vegan package (Oksanen et al., 2018). Spearman correlation analyses were used to examine the correlation between alpha diversity indices, fungal guilds (in relative abundance), and abiotic factors. The correlation coefficient and value of p were calculated with the ggcorrplot package in R (Kassambara, 2016).
Results
Fungal Composition and Guild Classification
A total of 8,740,440 sequence reads and 17,008 OTUs were initially obtained from the 72 samples. After filtering, 8,301,778 sequence reads and 10,856 OTUs remained. For individual samples, 32,789–202,543 sequence reads were obtained. The rarefaction curves of the observed number of OTUs for each sample were sufficiently saturated, with more than 98.5% Good’s coverage (data not shown). After rarifying the data to 30,000 reads/samples, we obtained 9,580 OTUs. Sequence data of three replicates from the same tree and the same season were combined into a single sample (90,000 reads). The average length of sequences was 389 ± 55 bp. We analyzed data from a total of 24 samples (two forest types × three trees × four seasons).
In the C. cordata forest, a total of 6,549 OTUs were obtained, and 1,292–2,389 OTUs were found from each sample. (Figure 1A). Basidiomycota was the most abundant (46.0%), followed by Ascomycota (45.9%) and Mortierellomycota (7.1%). At the genus level, Inocybe, Sebacina, Mortierella, Russula, and Tomentella were the most abundant (Figure 2A). The relative abundance of sequence reads belonging to genus Inocybe (9.2–12.7%) and Mortierella (6.3–7.5%) were mostly stable across seasons, while that of Sebacina was higher in autumn (12.6%) than in other seasons (5.9–8.9%). The relative abundance of Russula was lower in summer and winter (2.2–3.7%) than in spring and autumn (7.1–7.4%), while that of Tomentella was higher in spring and winter (5.0–5.7%) than in summer (1.7%). For trophic modes, symbiotrophs were the most abundant, followed by saprotrophs and saprotroph-symbiotrophs (Figure 2C). Among the symbiotrophs, an average of 67.9% of OTUs were EM and 0.4% were AM. Among the major genera, Inocybe, Sebacina, Russula, and Tomentella were classified as symbiotrophs. Saprotrophs were represented by Trechispora and Trichocladium. Mortierella was categorized as a saprotroph-symbiotroph. Several pathotrophic fungi were also found (Table 1).
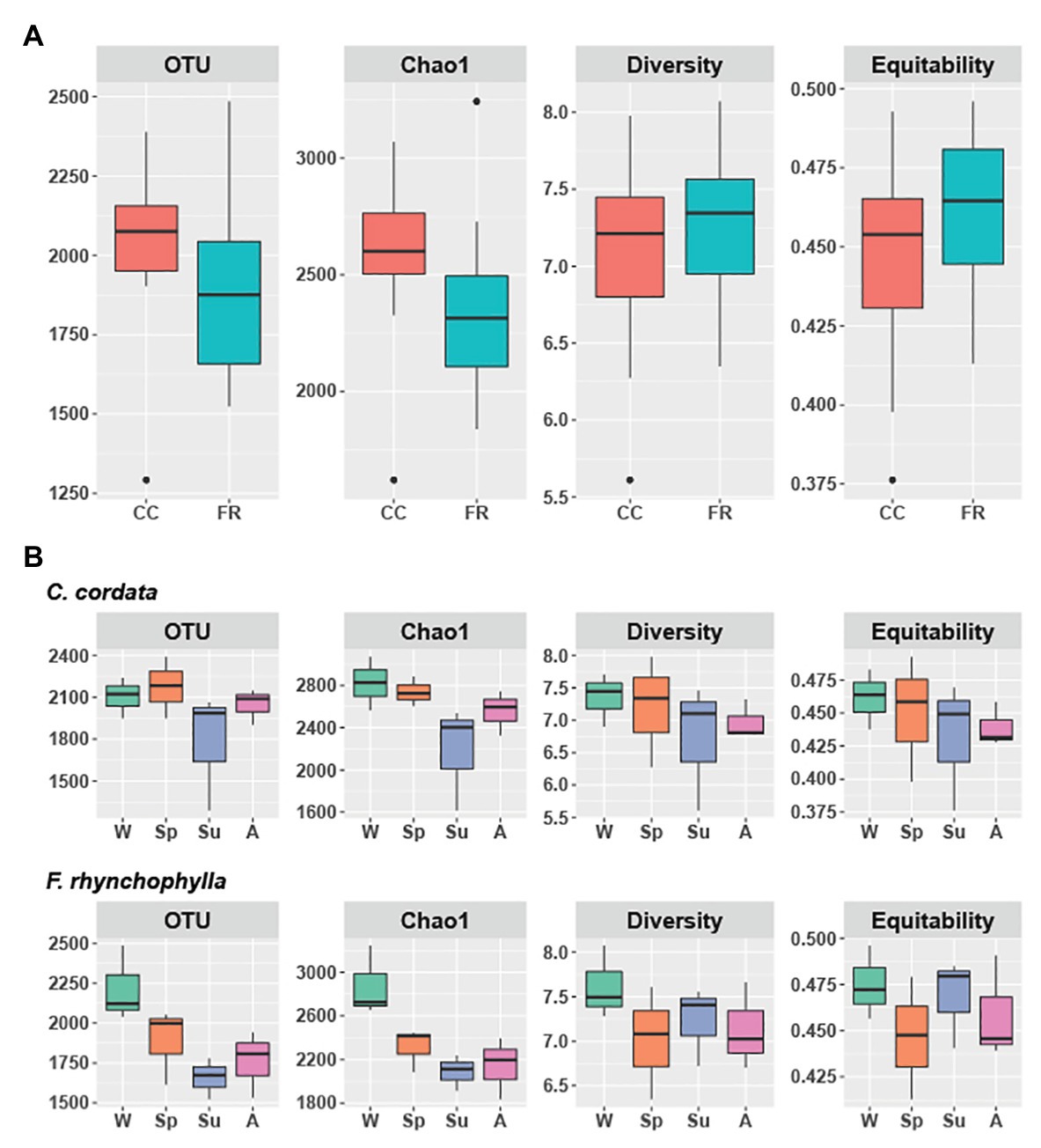
Figure 1. Alpha diversity of soil fungal communities from Carpinus cordata and Fraxinus rhynchophylla forests. (A) Overall alpha diversity of the rhizospheric soil under the dominant tree species in each forest and (B) Seasonal variation in alpha diversity in C. cordata and F. rhynchophylla: W (winter), Sp (spring), Su (summer), and A (autumn). Richness is represented by the number of OTUs and Chao1. Diversity and equitability were estimated with Shannon’s index. Significant differences in alpha diversity indices were tested using Kruskall-Wallis test with LSD Fisher post hoc test adjusted by the Bonferroni method. The box indicates the first and third quartiles, and black line in the middle indicates the median value.
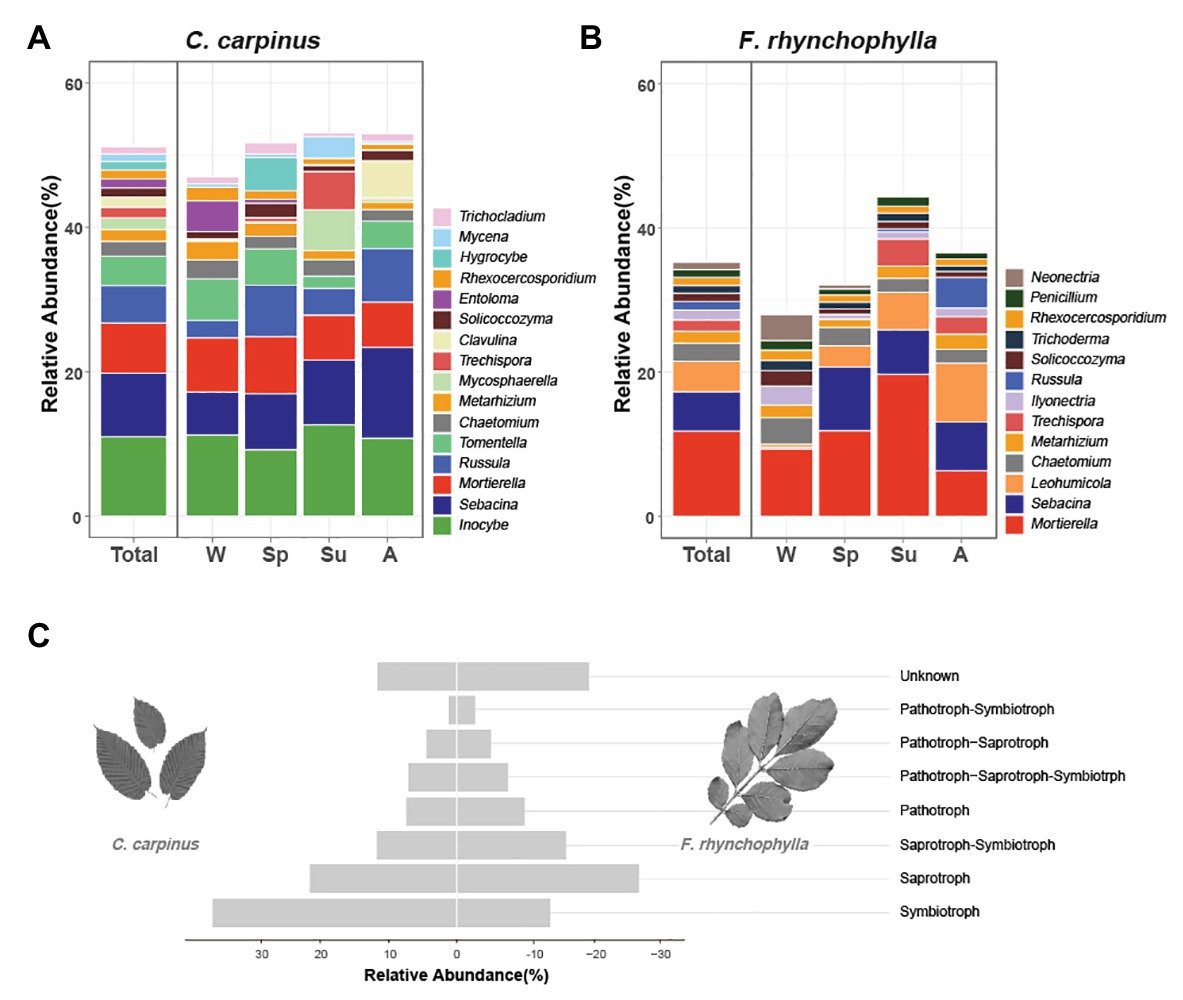
Figure 2. Taxonomic distribution of soil fungal communities in Carpinus cordata and Fraxinus rhynchophylla forests. The average relative abundances of genera in soil fungal communities of (A) C. cordata and (B) F. rhynchophylla forests are represented according to seasonal change. The genera with relative abundances less than 1% are not shown. (C) Average relative abundance of taxa belonging to each trophic mode in fungal communities of C. cordata and F. rhynchophylla forests. Unknown = taxonomic groups with unassigned trophic modes.
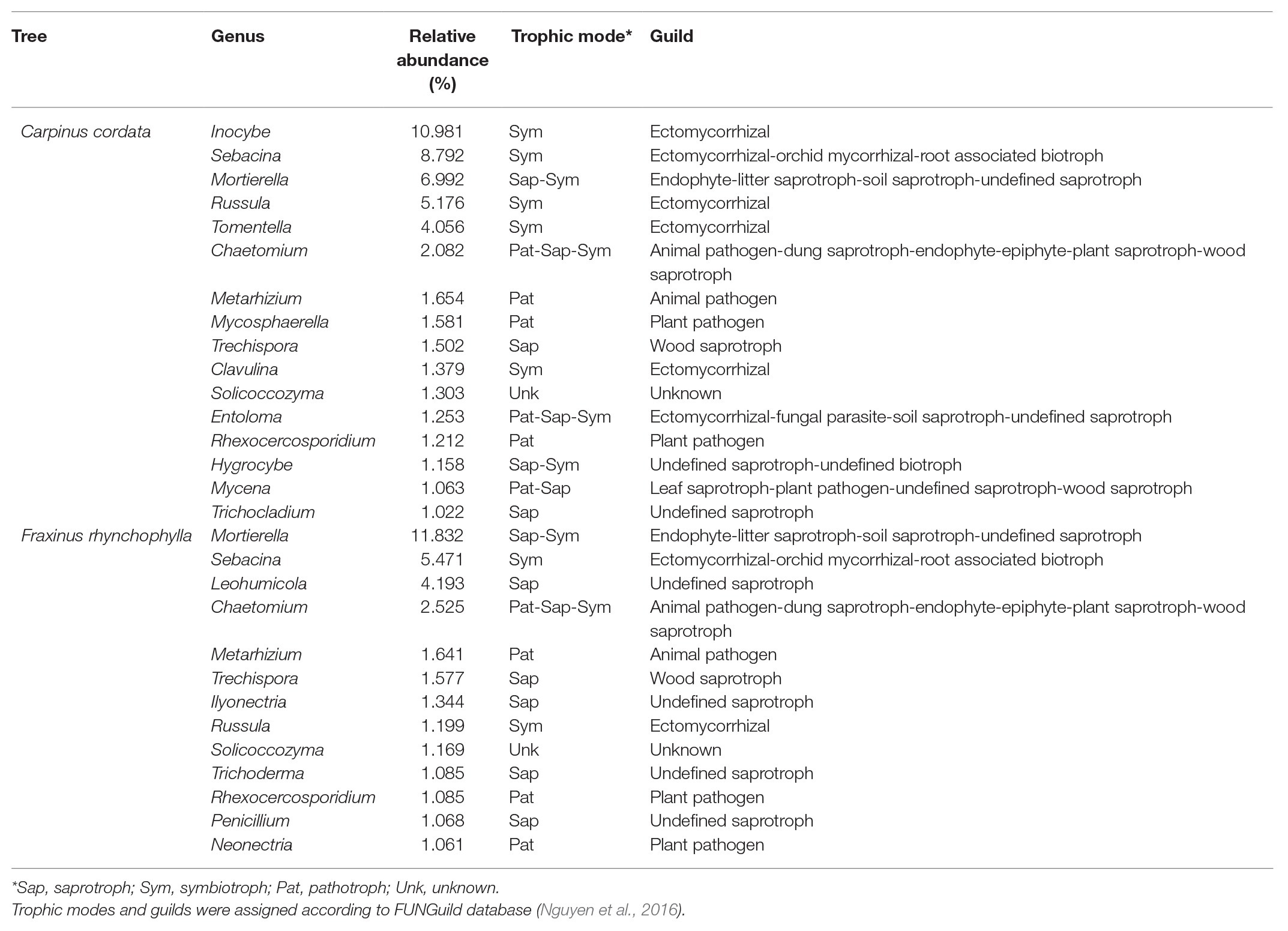
Table 1. Average relative abundance, trophic mode, and guild of major genera (>1%) in the fungal communities of rhizospheric soil in Carpinus cordata and Fraxinus rhynchophylla forests.
In the F. rhynchophylla forest, a total of 7,196 OTUs were obtained, and 1,524–2,485 OTUs were found in each sample (Figure 1A). Ascomycota was the most abundant (56.7%), followed by Basidiomycota (29.9%) and Mortierellomycota (12%). At the genus level, Mortierella, Sebacina, Leohumicola, Chaetomium, and Metarhizium were the most abundant (Figure 2B). The abundances of Chaetomium (1.9–3.7%) and Metarhizium (1.1–2.1%) were stable throughout the year. Sebacina and Leohumicola had the lowest relative abundance in winter (0.2 and 0.5%, respectively), and the abundance of Mortierella was the lowest in autumn (6.3%). For trophic modes, saprotrophs were the most abundant and included genera Leohumicola and Trechispora. The next most abundant trophic modes were saprotroph-symbiotrophs and symbiotrophs (Figure 2C). The average abundance of EM among the symbiotrophs was 40.6% and that of AM was 1.0%. Among the major genera, the proportion of saprotrophic fungi in F. rhynchophylla forest (Mortierella, Leohumicola, Chaetomium, Trechispora, Ilyonectria, Trichoderma, and Penicillium) was higher than that of C. cordata forest (Table 1).
Although the differences between respective alpha diversity indices were non-significant between the two forest types, the Chao1 richness and number of OTUs were higher in C. cordata (p = 0.07 for Chao1 and p = 0.08 for OTUs; Figure 1A). The seasonal differences in alpha diversity indices are shown in Figure 1B. The number of OTUs was the lowest in summer in both forest types. OTU and Chao1 indices in F. rhynchophyla forest showed a marginal association among seasons (p = 0.07 for OTUs and p = 0.06 for Chao1). The number of OTUs was slightly lower in summer than winter (p = 0.07), while Chao1 was different between autumn and winter (p = 0.07) in the post hoc test. No significant seasonal differences were detected in the C. cordata community diversity, but the F. rhynchophylla community showed a significant change in diversity across seasons (Figure 3, Supplementary Table 1). The compositions of fungal communities were significantly different between tree species (R2 = 0.254, p < 0.001; Figure 2).
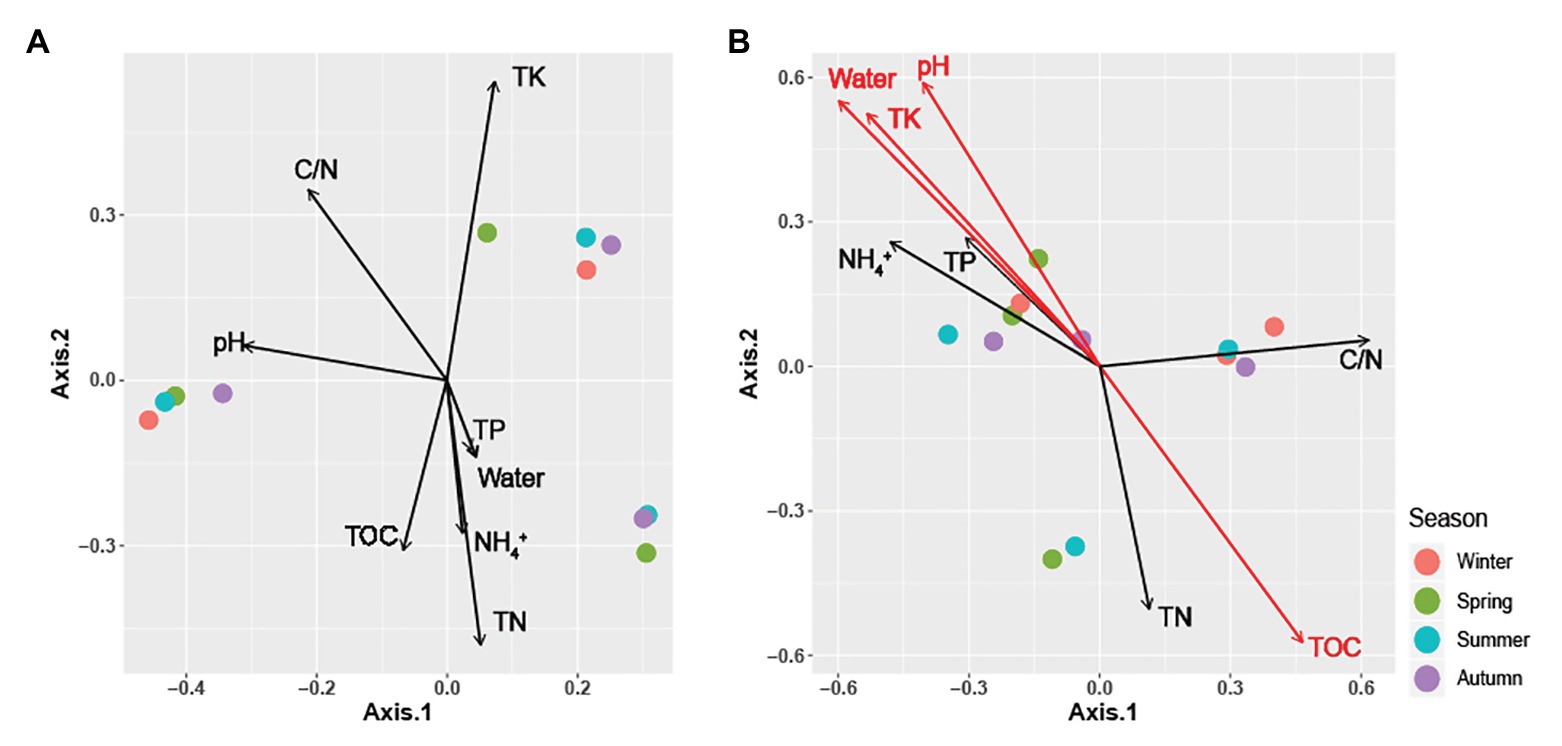
Figure 3. Principal coordinate analysis (PCoA) plots using Bray-Curtis distances matrices of (A) Carpinus cordata and (B) Fraxinus rhynchophylla soil fungal communities. Dot colors represent seasons: purple (Winter), green (Spring), blue (Summer), and red (Autumn). Soil parameters with significant relationships (p < 0.05) are indicated by red arrows.
Correlation Between Soil Properties and Fungal Community
All soil properties except pH showed similar seasonal patterns between forest types (Figure 4). In the PERMANOVA analysis of the C. cordata forest, soil properties were not related to fungal communities (Figure 3A, Supplementary Table 1). However, pH, TOC, water content, TK, and C/N ratio had significant effects on fungal communities of F. rhynchophylla, while TN, NH4+, and TP had non-significant effects (Figure 3B, Supplementary Table 1). Results from the environmental fitness analysis mostly corroborated the PERMANOVA results in both forest types; the one exception was the effect of the C/N ratio on fungal communities, which was not significant in F. rhynchophylla (p = 0.109) for the environmental fitness analysis, but was significant for PERMANOVA analysis. Similarly, the correlation between soil properties and alpha diversity indices was different between forest types (Table 2). For C. cordata, water content and TK showed a significantly positive correlation with the number of OTUs and Chao1 richness index, while TOC showed a significantly negative correlation with the Chao1 richness index (Table 2A). In F. rhynchophylla, a significant positive correlation was detected between TK and four alpha diversity indices (OTU, Chao1, diversity, and equitability), and there were negative correlations among TOC, TN, and Chao1 index (Table 2B). For trophic modes, C/N ratio showed a negative correlation with the relative abundance of pathotroph-saprotroph-symbiotroph group in both forest types. In C. cordata, the relative abundance of symbiotroph group showed a positive correlation with the C/N ratio. In contrast, the relative abundance of saprotroph-symbiotroph group in F. rhynchophylla showed a positive correlation with TOC and TN, while the relative abundance of pathotroph-symbiotroph group showed a negative correlation with TOC and a positive correlation with TK.
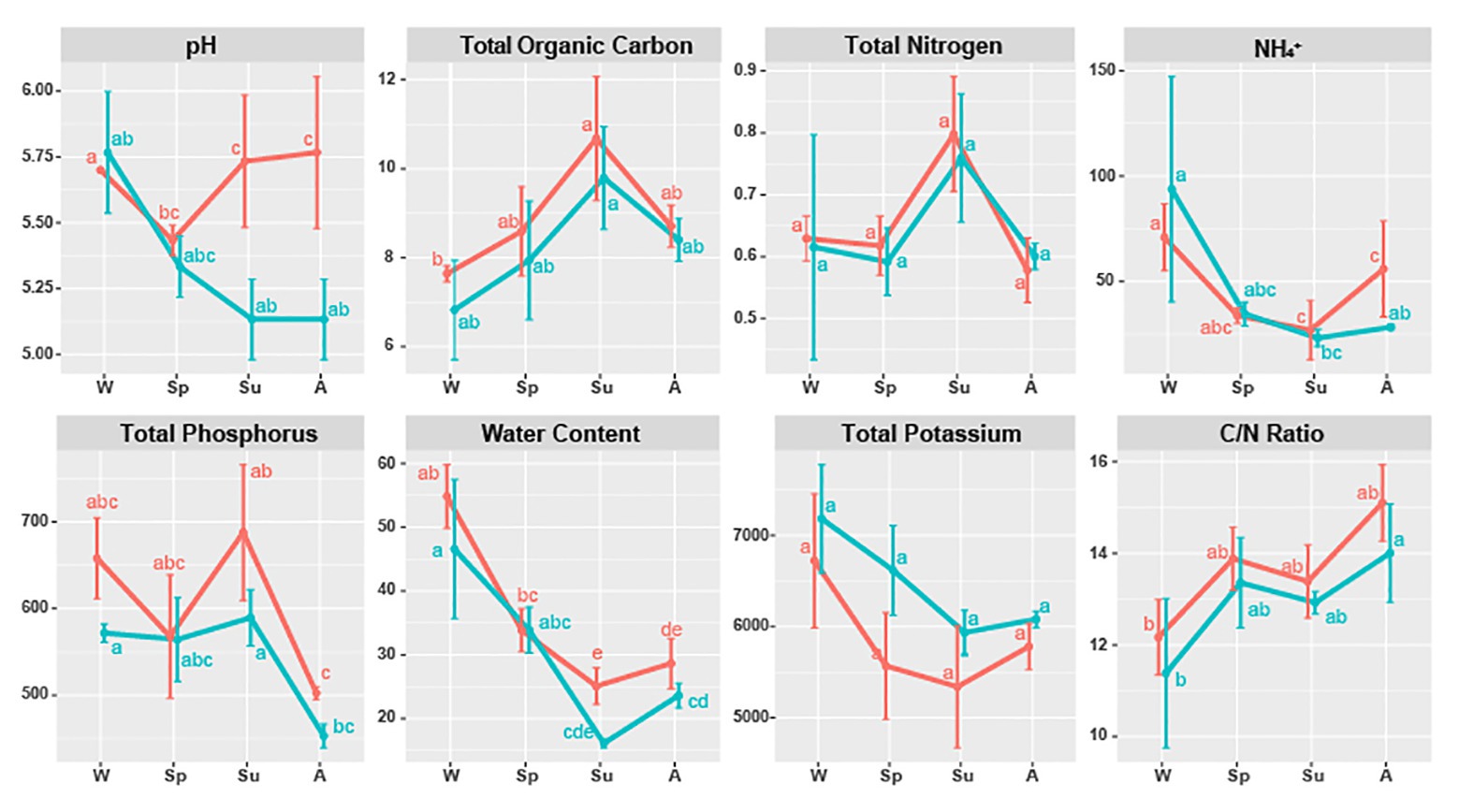
Figure 4. Soil properties of Carpinus cordata (red) and Fraxinus rhynchophylla (blue) forests separated by seasons. Measured soil properties are pH, TOC (Total Organic Carbon, %), TN (Total Nitrogen, %), NH4+ (ammonium ion, mg/kg), TP (Total Phosphorus, mg/kg), water (Water Content, %), TK (Total Potassium, mg/kg), and C/N ratio (carbon to nitrogen ratio, TOC/TN). Letters (a–e) were used as labels to indicate significant differences between means of each soil property in Kruskal-Wallis test with Bonferroni correction.
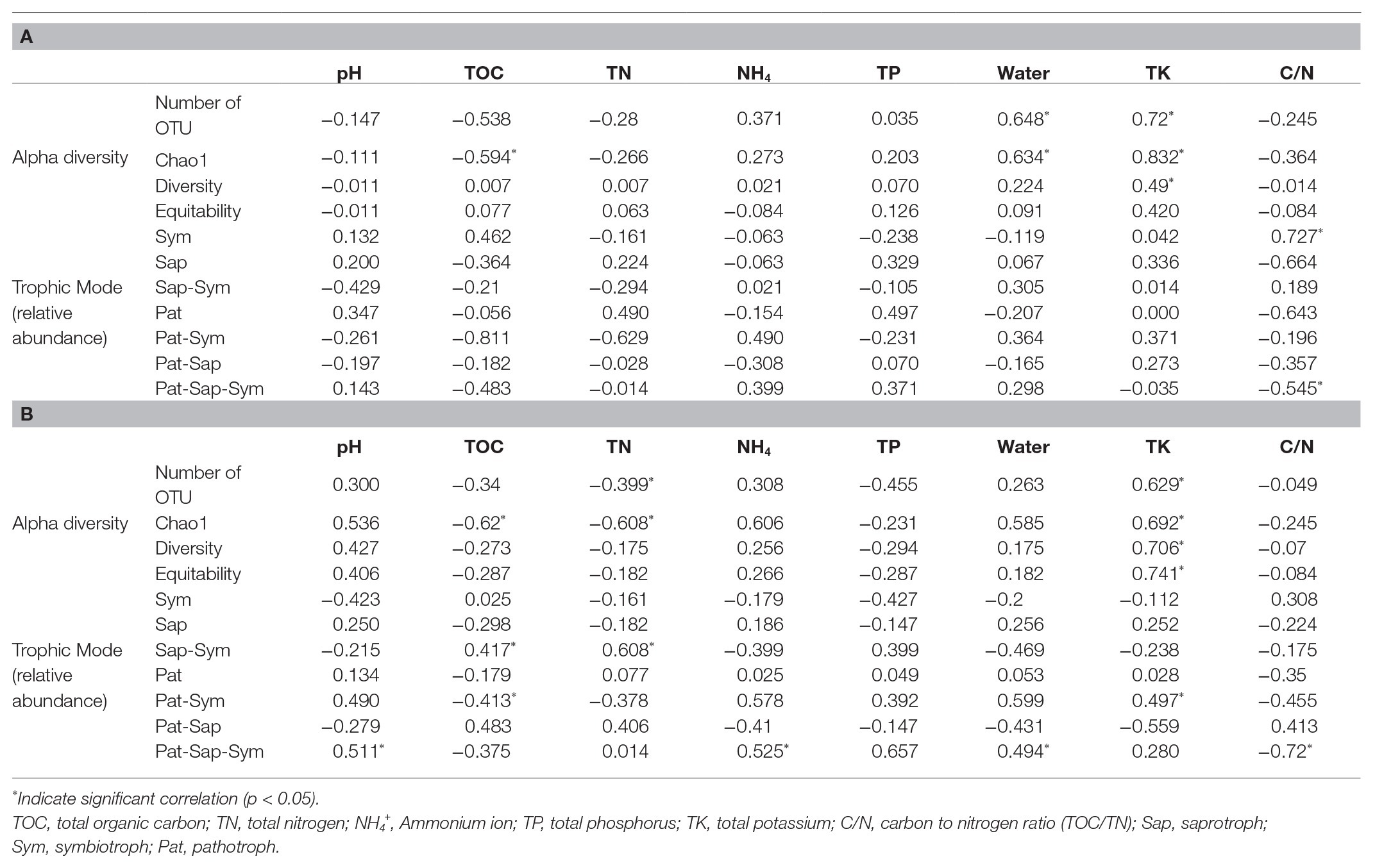
Table 2. The result of Spearman correlation coefficient test between alpha diversity indices, relative abundance of trophic modes and soil properties in (A) Carpinus cordata and (B) Fraxinus rhynchophylla forests.
Discussion
Overall Diversity and Composition of Fungi Associated With ECM and AM Trees
The fungal community was significantly different between the two forest types. A high relative abundance of ECM fungi was found in the C. cordata forest, a result similar to those of previous studies on other Carpinus species (Tyler, 1992; Rudawska et al., 2019). Several ECM fungi were found in rhizopheric soil of F. rhynchophylla as well. Although F. rhynchophylla is known as an AM tree (Ambriz et al., 2010), it was also reported to have a symbiotic relationship with EM fungi (Teste et al., 2020). Sebacina and Russula were the major ECM fungi in both forest types. Sebacina was reported to be a major ECM fungus in oak forests of Mt. Jeombong (Oh et al., 2018) and is known to be abundant in late-stage forests rather than early-stage forests (Long et al., 2016). Likewise, Russula is dominant in the late successional stages of many forests, including those with Carpinus and Fraxinus species (Tedersoo et al., 2010; Tedersoo and Nara, 2010; Li et al., 2013). Mt. Jeombong is a well-preserved national park, so the high relative abundance of late successional ECM fungi in both forests reflects the climax forest stages. Other ECM fungi (Clavulina, Inocybe, and Tomentella), known as late-successional ECM fungi (Smith et al., 2002; Tedersoo and Nara, 2010; Lang et al., 2011; Kałucka and Jagodziński, 2017) were more abundant in the C. cordata forest. AM fungi were two times more abundant in the F. rhynchophylla forest than the C. cordata forest, although overall relative abundance of OTUs belonging to AM fungi in both forest types was low. The reason of low relative abundance of AM OTUs may be that we did not use primers specifically targeting AM fungi, and a complementary study with such primers is needed to complete our understanding of F. rhynchophylla’s fungal community. However, if presence of both AM and ECM is verified, as found in our study, this might reflect dual-mycorrhization of F. rhynchophylla, as reported in roots of Fraxinus species (Ambriz et al., 2010; Lindig-Cisneros et al., 2019; Teste et al., 2020).
The saprotrophic fungal composition showed the opposite pattern from that of the ECM composition. The relative abundance of saprotrophic taxa among sequence reads of total OTUs (Mortierella, Leohumicola, Chaetomium, and Trechispora) were higher in the F. rhynchophylla forest than the C. cordata forest. This result may be related to different litter decomposition rates of AM and ECM trees (Midgley et al., 2015). Soil and litter quality are known to affect the saprotrophic fungal community (Aponte et al., 2013), and the decomposition rate of litter is faster in AM than ECM trees (Phillips et al., 2013; Averill et al., 2014; Sulman et al., 2017). In addition, AM trees are likely to provide more litter biomass than ECM trees (Rosling et al., 2016). Mortierella was the major saprotrophic-symbiotrophic genus in both forest types. In association with plant roots, Mortierella is known to decompose dead fungal hyphae (Brabcová et al., 2016), promote plant growth, and suppress phytopathogenic nematodes (Eroshin and Dedyukhina, 2002; Al-Shammari et al., 2013). Mortierella was commonly reported in soil and roots samples from various environments (Summerbell, 2005; Curlevski et al., 2010). Metarhizium is a widespread entomopathogen found in soil and insects (Roberts and Leger, 2004). While its activity in soil is unclear, plant growth promotion by suppressing plant pathogenic fungi and entomopathogenic activities were reported (Leger, 2008). Other plant pathogens (Mycosphaerella and Neonectria) are also commonly found in diseased plant tissues or soil as an opportunistic pathogens (Zhang et al., 2005; Menkis and Burokienė, 2012).
Effect of Season and Soil Properties on Soil Fungal Communities
While patterns of soil properties were similar in ECM (C. cordata) and AM (F. rhynchophylla) tree forests, fungal communities associated with C. cordata and F. rhynchophylla showed different responses to changes in season and soil properties. The fungal community associated with F. rhynchophylla showed temporal changes in relative abundance of major genera (Figure 2B). In contrast, no significant seasonal differences were detected in the fungal community associated with C. cordata. While the fungal communities in ECM and AM forests are generally affected by the season (Giachini et al., 2004; Bennett et al., 2013; Guadarrama et al., 2014; Voříšková et al., 2014; Santalahti et al., 2016), the opposite is also reported in ECM forests (Smith et al., 2007; Matsuoka et al., 2016). Priority effects – or the impact a species has on the community due to arriving first – might have caused a lack in seasonal shifts in C. cordata. If the early arriving fungi colonized root tips, later arriving fungi can be excluded from roots independent of environmental fluctuations (Dickie et al., 2012; Fukami, 2015). However, as patterns of fungal communities can change across years (Matsuoka et al., 2016), further research is needed to explain this phenomenon.
The relationship between the composition and abundance of a fungal community and soil properties was reported in previous studies, varying based on the locality and the characteristics of the sampling site (Yang et al., 2011; Voříšková et al., 2014; Žifčáková et al., 2016). In our study, the effect of soil properties on fungal community composition was generally significant in the F. rhynchophylla forest. Especially, we found that pH, potassium, and water content were positively correlated with each other, while TOC was negatively correlated in our result (Figure 3). However, their effect on alpha diversity indexes and trophic modes varied in C. cordata and F. rhynchophylla depending on soil properties (Table 2). Positive correlation between alpha diversity indices and TK were found in both forests, while richness and TOC showed negative correlations. These results indicate similarities among alpha diversity indices of fungal communities with different hosts, even though they had different compositions. There were also positive correlations with richness of soil fungal communities and TK contents in soil, while organic carbon was negatively linked to richness in both trees in both tree species. Potassium uptake is known to be improved by mycorrhization, and this improvement provide benefits to plants in the form of abiotic stress tolerance and phosphorus homeostasis maintenance (Dominguez-Nuñez et al., 2016). Meanwhile, a decrease of fungal biomass, diversity, and degrading activities were reported after carbon input by litter decomposition, but its mechanism is uncertain (Allison et al., 2007). In contrast, a positive correlation between C/N ratio and relative abundance of symbiotrophs was found only in C. cordata. High abundance of ECM in symbiotrophs of C. cordata might be associated with this result, as high C/N ratio was positively correlated with relative abundance of ECM taxa in previous studies (Chen et al., 2019), and ECM facilitate nitrogen uptake by releasing oxidative enzymes (Bödeker et al., 2014), but further investigation would develop our understanding of these processes.
Conclusion
In this study, we uncovered differences in fungal diversity and soil properties between neighboring climax forests (C. cordata and F. rhynchophylla) using a metabarcoding approach. Although seasonal patterns of soil properties were similar across the two forest types, soil fungal communities differed based on the season and soil properties only in F. rhynchophylla. Our results suggest the importance of considering characteristics of host trees, as different climax forests may respond to changes (seasonal and soil properties) in different ways.
Data Availability Statement
The datasets presented in this study can be found in online repositories. The names of the repository/repositories and accession number(s) can be found at: https://www.ncbi.nlm.nih.gov/bioproject/PRJNA638267/.
Author Contributions
CK, JJ, and YL contributed to conceiving and designing the experiments. KP performed the experiments and analyzed the data with assistance from S-YO. KP and SY wrote the manuscript with revisions from JF, S-YO, and YL. All authors contributed to the article and approved the submitted version.
Funding
This project was supported by the research project for exploring potential fungal diversity in forest soil (KNA1-1-25, 19-2) from the Korea National Arboretum.
Conflict of Interest
The authors declare that the research was conducted in the absence of any commercial or financial relationships that could be construed as a potential conflict of interest.
Acknowledgments
We would like to thank Jae Young Park, Min-Ji Kim, and Abel Severin Lupala for their help in sample collection.
Supplementary Material
The Supplementary Material for this article can be found online at: https://www.frontiersin.org/articles/10.3389/fmicb.2020.572706/full#supplementary-material
References
Allison, S. D., Hanson, C. A., and Treseder, K. K. (2007). Nitrogen fertilization reduces diversity and alters community structure of active fungi in boreal ecosystems. Soil Biol. Biochem. 39, 1878–1887. doi: 10.1016/j.soilbio.2007.02.001
Al-Shammari, T. A., Baha’i, A. H., Elgorban, A. M., El-Kahky, M. T., and Al-Sum, B. A. (2013). The use of Trichoderma longibrachiatum and Mortierella alpina against root-knot nematode, Meloidogyne javanica on tomato. J. Pure Appl. Microbiol. 7, 199–207.
Ambriz, E., Báez-Pérez, A., Sánchez-Yáñez, J. M., Moutoglis, P., and Villegas, J. (2010). Fraxinus-Glomus-Pisolithus symbiosis: plant growth and soil aggregation effects. Pedobiologia 53, 369–373. doi: 10.1016/j.pedobi.2010.07.001
Aponte, C., García, L. V., and Marañón, T. (2013). Tree species effects on nutrient cycling and soil biota: a feedback mechanism favouring species coexistence. Forest Ecol. Manag. 309, 36–46. doi: 10.1016/j.foreco.2013.05.035
Aponte, C., García, L. V., Marañón, T., and Gardes, M. (2010). Indirect host effect on ectomycorrhizal fungi: leaf fall and litter quality explain changes in fungal communities on the roots of co-occurring Mediterranean oaks. Soil Biol. Biochem. 42, 788–796. doi: 10.1016/j.soilbio.2010.01.014
Averill, C., Turner, B. L., and Finzi, A. C. (2014). Mycorrhiza-mediated competition between plants and decomposers drives soil carbon storage. Nature 505, 543–545. doi: 10.1038/nature12901
Bennett, A. E., Daniell, T. J., Öpik, M., Davison, J., Moora, M., Zobel, M., et al. (2013). Arbuscular mycorrhizal fungal networks vary throughout the growing season and between successional stages. PLoS One 8:e83241. doi: 10.1371/journal.pone.0083241
Bödeker, I. T., Clemmensen, K. E., de Boer, W., Martin, F., Olson, Å., and Lindahl, B. D. (2014). Ectomycorrhizal Cortinarius species participate in enzymatic oxidation of humus in northern forest ecosystems. New Phytol. 203, 245–256. doi: 10.1111/nph.12791
Brabcová, V., Nováková, M., Davidová, A., and Baldrian, P. (2016). Dead fungal mycelium in forest soil represents a decomposition hotspot and a habitat for a specific microbial community. New Phytol. 210, 1369–1381. doi: 10.1111/nph.13849
Brundrett, M. C. (2009). Mycorrhizal associations and other means of nutrition of vascular plants: understanding the global diversity of host plants by resolving conflicting information and developing reliable means of diagnosis. Plant Soil 320, 37–77. doi: 10.1007/s11104-008-9877-9
Brundrett, M. C. (2017). “Global diversity and importance of mycorrhizal and nonmycorrhizal plants” in Biogeography of mycorrhizal symbiosis. ed. L. Tedersoo (New York, NY: Springer), 533–556.
Brundrett, M. C., and Tedersoo, L. (2018). Evolutionary history of mycorrhizal symbioses and global host plant diversity. New Phytol. 220, 1108–1115. doi: 10.1111/nph.14976
Caporaso, J. G., Kuczynski, J., Stombaugh, J., Bittinger, K., Bushman, F. D., Costello, E. K., et al. (2010). QIIME allows analysis of high-throughput community sequencing data. Nat. Methods 7, 335–336. doi: 10.1038/nmeth.f.303
Chen, L., Xiang, W., Wu, H., Ouyang, S., Zhou, B., Zeng, Y., et al. (2019). Tree species identity surpasses richness in affecting soil microbial richness and community composition in subtropical forests. Soil Biol. Biochem. 130, 113–121. doi: 10.1016/j.soilbio.2018.12.008
Cho, H. S., Kim, G. T., and Choo, G. C. (2005). Studies on the structure of forest community at the Danggol valley in Taebaeksan area, the Baekdudaegan. Korean J. Environ. Ecol. 19, 55–62 (In Korean).
Craig, M. E., Turner, B. L., Liang, C., Clay, K., Johnson, D. J., and Phillips, R. P. (2018). Tree mycorrhizal type predicts within-site variability in the storage and distribution of soil organic matter. Glob. Chang. Biol. 24, 3317–3330. doi: 10.1111/gcb.14132
Curlevski, N. J. A., Xu, Z. H., Anderson, I. C., and Cairney, J. W. (2010). Diversity of soil and rhizosphere fungi under Araucaria bidwillii (Bunya pine) at an Australian tropical montane rainforest site. Fungal Divers. 40, 12–22. doi: 10.1007/s13225-009-0001-0
Dickie, I. A., Fukami, T., Wilkie, J. P., Allen, R. B., and Buchanan, P. K. (2012). Do assembly history effects attenuate from species to ecosystem properties? A field test with wood-inhabiting fungi. Ecol. Lett. 15, 133–141. doi: 10.1111/j.1461-0248.2011.01722.x
Dominguez-Nuñez, J. A., Benito, B., Berrocal-Lobo, M., and Albanesi, A. (2016). “Mycorrhizal fungi: role in the solubilization of potassium” in Potassium solubilizing microorganisms for sustainable agriculture. eds. B. R. Maurya, J. P. Verma, and R. S. Meena (New Delhi: Springer), 77–98.
Edgar, R. C. (2010). Search and clustering orders of magnitude faster than BLAST. Bioinformatics 26, 2460–2461. doi: 10.1093/bioinformatics/btq461
Edgar, R. C., Haas, B. J., Clemente, J. C., Quince, C., and Knight, R. (2011). UCHIME improves sensitivity and speed of chimera detection. Bioinformatics 27, 2194–2200. doi: 10.1093/bioinformatics/btr381
Eroshin, V. K., and Dedyukhina, E. G. (2002). Effect of lipids from Mortierella hygrophila on plant resistance to phytopathogens. World. J. Microb. Biot. 18, 165–167. doi: 10.1023/A:1014429527591
Fukami, T. (2015). Historical contingency in community assembly: integrating niches, species pools, and priority effects. Annu. Rev. Ecol. Evol. Syst. 46, 1–23. doi: 10.1146/annurev-ecolsys-110411-160340
Giachini, A. J., Souza, L. A., and Oliveira, V. L. (2004). Species richness and seasonal abundance of ectomycorrhizal fungi in plantations of Eucalyptus dunnii and Pinus taeda in southern Brazil. Mycorrhiza 14, 375–381. doi: 10.1007/s00572-004-0297-2
Guadarrama, P., Castillo, S., Ramos-Zapata, J. A., Hernández-Cuevas, L. V., and Camargo-Ricalde, S. L. (2014). Arbuscular mycorrhizal fungal communities in changing environments: the effects of seasonality and anthropogenic disturbance in a seasonal dry forest. Pedobiologia 57, 87–95. doi: 10.1016/j.pedobi.2014.01.002
Helgason, T., and Fitter, A. H. (2009). Natural selection and the evolutionary ecology of the arbuscular mycorrhizal fungi (phylum Glomeromycota). J. Exp. Bot. 60, 2465–2480. doi: 10.1093/jxb/erp144
Kałucka, I. L., and Jagodziński, A. M. (2017). “Ectomycorrhizal Fungi: a major player in early succession” in Mycorrhiza-function, diversity, state of the art. eds. A. Varma, R. Prasad, and N. Tuteja (Cham: Springer), 187–229.
Kõljalg, U., Nilsson, R. H., Abarenkov, K., Tedersoo, L., Taylor, A. F., Bahram, M., et al. (2013). Towards a unified paradigm for sequence-based identification of fungi. Mol. Ecol. 22, 5271–5277. doi: 10.1111/mec.12481
Kramer, S., Marhan, S., Ruess, L., Armbruster, W., Butenschoen, O., Haslwimmer, H., et al. (2012). Carbon flow into microbial and fungal biomass as a basis for the belowground food web of agroecosystems. Pedobiologia 55, 111–119. doi: 10.1016/j.pedobi.2011.12.001
Kwon, B., Heo, N., Shin, H., Kim, H. S., Park, P. S., and Yi, M. J. (2014). Nutrient use strategy of Carpinus cordata saplings growing under different forest stand conditions. Korean J. Agric. For. Meteorol. 16, 188–196. doi: 10.5532/KJAFM.2014.16.3.188 (In Korean).
Lang, C., Seven, J., and Polle, A. (2011). Host preferences and differential contributions of deciduous tree species shape mycorrhizal species richness in a mixed central European forest. Mycorrhiza 21, 297–308. doi: 10.1007/s00572-010-0338-y
Lee, K. J., Jo, J. C., Lee, B. S., and Lee, D. S. (1990). The structure of plant community in Kwangnung forest. I. Analysis of the forest community of Soribong area by the classification and ordination techniques. J. Korean. For. Soc. 79, 173–186 (In Korean).
Leger, R. J. S. (2008). Studies on adaptations of Metarhizium anisopliae to life in the soil. J. Invertebr. Pathol. 98, 271–276. doi: 10.1016/j.jip.2008.01.007
Li, G. J., Zhao, Q., Zhao, D., Yue, S. F., Li, S. F., Wen, H. A., et al. (2013). Russula atroaeruginea and R. sichuanensis spp. nov. from Southwest China. Mycotaxon 124, 173–188. doi: 10.5248/124.173
Lin, G., McCormack, M. L., Ma, C., and Guo, D. (2017). Similar below-ground carbon cycling dynamics but contrasting modes of nitrogen cycling between arbuscular mycorrhizal and ectomycorrhizal forests. New Phytol. 213, 1440–1451. doi: 10.1111/nph.14206
Lindig-Cisneros, R., Villegas, J., Báez-Pérez, A. L., and Gómez-Romero, M. (2019). Biomass and iron accumulation in plants of Pinus pseudotrobus and Fraxinus uhdei in Acrisols from Western-Mexico with endo- and ectomycorrhiza. Catena 173, 410–413. doi: 10.1016/j.catena.2018.10.033
Long, D., Liu, J., Han, Q., Wang, X., and Huang, J. (2016). Ectomycorrhizal fungal communities associated with Populus simonii and Pinus tabuliformis in the hilly-gully region of the Loess Plateau, China. Sci. Rep. 6:24336. doi: 10.1038/srep24336
Matsuoka, S., Kawaguchi, E., and Osono, T. (2016). Temporal distance decay of similarity of ectomycorrhizal fungal community composition in a subtropical evergreen forest in Japan. FEMS Microbiol. Ecol. 92:fiw061. doi: 10.1093/femsec/fiw061
McMurdie, P. J., and Holmes, S. (2013). Phyloseq: an R package for reproducible interactive analysis and graphics of microbiome census data. PLoS One 8:e61217. doi: 10.1371/journal.pone.0061217
Menkis, A., and Burokienė, D. (2012). Distribution and genetic diversity of the root-rot pathogen Neonectria macrodidyma in a forest nursery. For. Pathol. 42, 79–83. doi: 10.1111/j.1439-0329.2011.00712.x
Midgley, M. G., Brzostek, E., and Phillips, R. P. (2015). Decay rates of leaf litters from arbuscular mycorrhizal trees are more sensitive to soil effects than litters from ectomycorrhizal trees. J. Ecol. 103, 1454–1463. doi: 10.1111/1365-2745.12467
Nguyen, N. H., Song, Z., Bates, S. T., Branco, S., Tedersoo, L., Menke, J., et al. (2016). FUNGuild: an open annotation tool for parsing fungal community datasets by ecological guild. Fungal Ecol. 20, 241–248. doi: 10.1016/j.funeco.2015.06.006
Oh, S. Y., Cho, H. J., Eimes, J. A., Han, S. K., Kim, C. S., and Lim, Y. W. (2018). Guild patterns of basidiomycetes community associated with Quercus mongolica in Mt. Jeombong, Republic of Korea. Mycobiology 46, 13–23. doi: 10.1080/12298093.2018.1454009
Oksanen, J., Blanchet, F. G., Friendly, M., Kindt, R., Legendre, P., McGlinn, D., et al. (2018). Vegan: community ecology package. R package version 2.5–2.
Park, B. J., Kim, J. J., Byeon, J. G., Cheon, K., Joo, S. H., and Lee, Y. G. (2016). The classification of forest community and character of stand structure in Mt. Myeonbong-focused on research Forest in Kyungpook National University, Cheongsong. J. Korean. For. Soc. 105, 391–400. doi: 10.14578/jkfs.2016.105.4.391 (In Korean).
Phillips, R. P., Brzostek, E., and Midgley, M. G. (2013). The mycorrhizal-associated nutrient economy: a new framework for predicting carbon-nutrient couplings in temperate forests. New Phytol. 199, 41–51. doi: 10.1111/nph.12221
R Core Team (2019). R: a language and environment for statistical computing. R Foundation for Statistical Computing. Vienna, Austria. https://www.R-project.org/ (Accessed May 27, 2020).
Roberts, D. W., and Leger, R. J. S. (2004). Metarhizium spp., cosmopolitan insect-pathogenic fungi: mycological aspects. Adv. Appl. Microbiol. 54, 1–70. doi: 10.1016/S0065-2164(04)54001-7
Rosling, A., Midgley, M. G., Cheeke, T., Urbina, H., Fransson, P., and Phillips, R. P. (2016). Phosphorus cycling in deciduous forest soil differs between stands dominated by ecto- and arbuscular mycorrhizal trees. New Phytol. 209, 1184–1195. doi: 10.1111/nph.13720
Rudawska, M., Kujawska, M., Leski, T., Janowski, D., Karliński, L., and Wilgan, R. (2019). Ectomycorrhizal community structure of the admixture tree species Betula pendula, Carpinus betulus, and Tilia cordata grown in bare-root forest nurseries. For. Ecol. Manag. 437, 113–125. doi: 10.1016/j.foreco.2019.01.009
Santalahti, M., Sun, H., Jumpponen, A., Pennanen, T., and Heinonsalo, J. (2016). Vertical and seasonal dynamics of fungal communities in boreal scots pine forest soil. FEMS Microbiol. Ecol. 92:fiw170. doi: 10.1093/femsec/fiw170
Smith, M. E., Douhan, G. W., and Rizzo, D. M. (2007). Ectomycorrhizal community structure in a xeric Quercus woodland based on rDNA sequence analysis of sporocarps and pooled roots. New Phytol. 174, 847–863. doi: 10.1111/j.1469-8137.2007.02040.x
Smith, J. E., Molina, R., Huso, M. M., Luoma, D. L., McKay, D., Castellano, M. A., et al. (2002). Species richness, abundance, and composition of hypogeous and epigeous ectomycorrhizal fungal sporocarps in young, rotation-age, and old-growth stands of Douglas-fir (Pseudotsuga menziesii) in the Cascade Range of Oregon, USA. Can. J. Bot. 80, 186–204. doi: 10.1139/b02-003
Soudzilovskaia, N. A., van der Heijden, M. G., Cornelissen, J. H., Makarov, M. I., Onipchenko, V. G., Maslov, M. N., et al. (2015). Quantitative assessment of the differential impacts of arbuscular and ectomycorrhiza on soil carbon cycling. New Phytol. 208, 280–293. doi: 10.1111/nph.13447
Sulman, B. N., Brzostek, E. R., Medici, C., Shevliakova, E., Menge, D. N., and Phillips, R. P. (2017). Feedbacks between plant N demand and rhizosphere priming depend on type of mycorrhizal association. Ecol. Lett. 20, 1043–1053. doi: 10.1111/ele.12802
Summerbell, R. C. (2005). Root endophyte and mycorrhizosphere fungi of black spruce, Picea mariana, in a boreal forest habitat: influence of site factors on fungal distributions. Stud. Mycol. 53, 121–145. doi: 10.3114/sim.53.1.121
Tedersoo, L., Bahram, M., Põlme, S., Kõljalg, U., Yorou, N. S., Wijesundera, R., et al. (2014). Global diversity and geography of soil fungi. Science 346:1256688. doi: 10.1126/science.1256688
Tedersoo, L., and Brundrett, M. C. (2017). “Evolution of ectomycorrhizal symbiosis in plants” in Biogeography of mycorrhizal symbiosis. ed. L. Tedersoo (New York, USA: Springer International Publishing), 407–467.
Tedersoo, L., May, T. W., and Smith, M. E. (2010). Ectomycorrhizal lifestyle in fungi: global diversity, distribution, and evolution of phylogenetic lineages. Mycorrhiza 20, 217–263. doi: 10.1007/s00572-009-0274-x
Tedersoo, L., and Nara, K. (2010). General latitudinal gradient of biodiversity is reversed in ectomycorrhizal fungi. New Phytol. 185, 351–354. doi: 10.1111/j.1469-8137.2009.03134.x
Teste, F. P., Jones, M. D., and Dickie, I. A. (2020). Dual-mycorrhizal plants: their ecology and relevance. New Phytol. 225, 1835–1851. doi: 10.1111/nph.16190
Tyler, G. (1992). Tree species affinity of decomposer and ectomycorrhizal macrofungi in beech (Fagus sylvatica L.), oak (Quercus robur L.) and hornbeam (Carpinus betulus L.) forests. For. Ecol. Manag. 47, 269–284. doi: 10.1016/0378-1127(92)90279-I
Van Der Heijden, M. G., Bardgett, R. D., and Van Straalen, N. M. (2008). The unseen majority: soil microbes as drivers of plant diversity and productivity in terrestrial ecosystems. Ecol. Lett. 11, 296–310. doi: 10.1111/j.1461-0248.2007.01139.x
van der Wal, A., Geydan, T. D., Kuyper, T. W., and De Boer, W. (2013). A thready affair: linking fungal diversity and community dynamics to terrestrial decomposition processes. FEMS Microbiol. Rev. 37, 477–494. doi: 10.1111/1574-6976.12001
Vesterdal, L., Clarke, N., Sigurdsson, B. D., and Gundersen, P. (2013). Do tree species influence soil carbon stocks in temperate and boreal forests? For. Ecol. Manag. 309, 4–18. doi: 10.1016/j.foreco.2013.01.017
Voříšková, J., Brabcová, V., Cajthaml, T., and Baldrian, P. (2014). Seasonal dynamics of fungal communities in a temperate oak forest soil. New Phytol. 201, 269–278. doi: 10.1111/nph.12481
White, T. J., Bruns, T., Lee, S. J. W. T., and Taylor, J. (1990). “Amplification and direct sequencing of fungal ribosomal RNA genes for phylogenetics” in PCR protocols: A guide to methods and applications. eds. M. A. Innis, D. H. Gelfand, J. J. Sninsky, and T. J. White (New York: Academic Press, Inc.), 315–322.
Wu, D., Zhang, M., Peng, M., Sui, X., Li, W., and Sun, G. Y. (2019). Variations in soil functional fungal community structure associated with pure and mixed plantations in typical temperate forests of China. Front. Microbiol. 10:1636. doi: 10.3389/fmicb.2019.01636
Yang, H., Yuan, Y., Zhang, Q., Tang, J., Liu, Y., and Chen, X. (2011). Changes in soil organic carbon, total nitrogen, and abundance of arbuscular mycorrhizal fungi along a large-scale aridity gradient. Catena 87, 70–77. doi: 10.1016/j.catena.2011.05.009
Zhang, Z., Zhang, J., Wang, Y., and Zheng, X. (2005). Molecular detection of Fusarium oxysporum f. sp. niveum and Mycosphaerella melonis in infected plant tissues and soil. FEMS Microbiol. Lett. 24, 39–47. doi: 10.1016/j.femsle.2005.05.057
Keywords: arbuscular mycorrhizal fungi, ectomycorrhizal fungi, temperate forest, plant-soil interaction, soil fungal community
Citation: Park KH, Oh S-Y, Yoo S, Fong JJ, Kim CS, Jo JW and Lim YW (2020) Influence of Season and Soil Properties on Fungal Communities of Neighboring Climax Forests (Carpinus cordata and Fraxinus rhynchophylla). Front. Microbiol. 11:572706. doi: 10.3389/fmicb.2020.572706
Edited by:
Nuria Ferrol, Experimental Station of Zaidín (EEZ), SpainReviewed by:
Mélanie Roy, Université Toulouse III Paul Sabatier, FranceLucie Vincenot, Université de Rouen, France
Copyright © 2020 Park, Oh, Yoo, Fong, Kim, Jo and Lim. This is an open-access article distributed under the terms of the Creative Commons Attribution License (CC BY). The use, distribution or reproduction in other forums is permitted, provided the original author(s) and the copyright owner(s) are credited and that the original publication in this journal is cited, in accordance with accepted academic practice. No use, distribution or reproduction is permitted which does not comply with these terms.
*Correspondence: Young Woon Lim, eXdsaW1Ac251LmFjLmty
†These authors have contributed equally to this work