- 1Guangdong Provincial Key Laboratory of Microbiology Safety and Health, State Key Laboratory of Applied Microbiology Southern China, Guangdong Institute of Microbiology, Guangdong Academy of Sciences, Guangzhou, China
- 2School of Bioscience and Bioengineering, South China University of Technology, Guangzhou, China
- 3College of Food Science, South China Agricultural University, Guangzhou, China
- 4Biological Testing and Analysis Department, Guangdong Provincial Institute of Food Inspection, Guangzhou, China
Pseudomonas aeruginosa is an important opportunistic pathogen and remains a major threat to the microbial safety of drinking water. There is a lack of comprehensive data on P. aeruginosa contamination in drinking water in China. Therefore, this study aimed to determine the prevalence, genetic diversity, virulence genes, and antimicrobial resistance of P. aeruginosa isolated from mineral water and spring water in China. From January 2013 to January 2014, 314 drinking water samples were collected from 23 cities in China. Of the collected samples, 77 (24.5%) were contaminated with P. aeruginosa, and these comprised 34 raw water (30.4%), 39 activated carbon-filtered water (30.6%), and four final water product (3.9%). A total of 132 P. aeruginosa isolates were obtained, and all of them showed the presence of virulence genes, with the detection rates of ExoU, ExoS, phzM, toxA, and lasB genes being 7.6, 86.3, 95.5, 89.4, and 100%, respectively. All isolates were sensitive to the 14 antibiotics (ciprofloxacin, levofloxacin, ofloxacin, norfloxacin, gentamicin, tobramycin, amikacin, polymyxin B, imipenem, meropenem, aztreonam, ceftazidime, cefepime, and piperacillin/tazobactam) tested. The 132 isolates were categorized into 42 sequence types according to multilocus sequence typing, and ST235 accounted for 8.3% (11) of the total isolates. Thus, this study provides comprehensive data on the prevalence and characteristics of P. aeruginosa in drinking water in China and can aid in developing preventive measures against contamination during the drinking water treatment process.
Introduction
Microbial contamination of drinking water is a common problem that has a serious impact on public health (Li et al., 2017; Wang et al., 2018). Pseudomonas aeruginosa is an important opportunistic pathogen and is frequently detected in drinking water (Vaz-Moreira et al., 2012; Lu et al., 2016). P. aeruginosa widely inhabits diverse environments, including water and soil, and is one of the main pathogens of nosocomial infections, such as cystic fibrosis (Agarwal et al., 2005; Driscoll et al., 2007). P. aeruginosa has a disinfectant resistance gene and can form a biofilm, which makes it the most common contaminant in drinking water production (Guerin-Mechin et al., 2000; Mah et al., 2003). Previous studies have shown that P. aeruginosa is the most suitable indicator of the presence of pathogens in drinking water (Mena and Gerba, 2009). The recommended international code of hygienic practice for collecting, processing, and marketing of natural mineral waters (CAC/RCP 33-1985) and on the quality of water intended for human consumption (98/83/EC) short-listed P. aeruginosa as a bacterial indicator of drinking water quality.
For epidemiological surveillance of P. aeruginosa in drinking water, molecular typing methods have the advantage of discriminatory ability and stability (Mena and Gerba, 2009). In recent studies, multilocus sequence typing (MLST), pulsed field gel electrophoresis (PFGE), enterobacterial repetitive intergenic consensus-polymerase chain reaction, and random-amplified polymorphic DNA have been conducted for tracing sources of P. aeruginosa (Wu et al., 2016; Bel Hadj Ahmed et al., 2019; Slekovec et al., 2019). Among these molecular typing methods, MLST and PFGE have the highest reproducibility and discriminatory ability for typing of P. aeruginosa isolates (De Cesare et al., 2016). Johnson et al. (2007) conducted molecular typing of 90 P. aeruginosa strains using PFGE and MLST, respectively, and the study proved that the resolution of PFGE and MLST was not significantly different. However, PFGE is labor time-consuming and requires sophisticated electrophoresis equipment. MLST typing is performed by comparing seven housekeeping gene sequences of bacteria. According to the result of MLST typing, the genetic diversity of bacteria is consistent in laboratories all over the world.
The pathogenicity of P. aeruginosa is mainly due to its expression of a dozen virulence factors, such as exotoxin A and pyocyanin (Feinbaum et al., 2012). The genes ExoS and ExoU encode the extracellular enzymes ExoS and ExoU, respectively. ExoS can destroy actin cytoskeleton to inhibit phagocytosis in host cells (Horna et al., 2019). ExoU is cytotoxic and has activity of phospholipid enzymes and adenopropionic acid cyclase (Luo et al., 2019). The gene toxA encodes exotoxin A, which can block the synthesis of cellular proteins, leading to host tissue necrosis (Wedekind et al., 2001). The gene phzM encodes a protein that converts phenazine-1-carboxylic acid to pyocyanin to inhibit cellular mitochondrial activity (Parsons et al., 2007). The gene lasB encodes elastase, which hydrolyzes host cell elastin. The synergistic effects of these various virulence factors contribute to the toxicity of P. aeruginosa (Bradbury et al., 2010).
Systematic data regarding the prevalence of P. aeruginosa in drinking water have not been obtained in China. In addition, information on virulent genes, antimicrobial resistance, and the genetic diversity of P. aeruginosa is also lacking. Therefore, in this study, we determined the prevalence, virulence genes, and antimicrobial resistance of P. aeruginosa in drinking water and further characterized the isolates using MLST.
Materials and Methods
Study Site and Sample Collection
From January 2013 to January 2014, according to the guideline of ISO-16266-2008, we collected water samples from 101drinking water factories (including 58 spring water and 43 mineral water factories) in 23 cities of China (Beijing, Shanghai, Chengdu, Wuhan, Xiamen, Guiyang, Kunming, Nanning, Haikou, Yangjiang, Yufu, Zhaoqing, Qingyuan, Shaoguan, Heyuan, Meizhou, Huizhou, Guangzhou, Dongguan, Foshan, Zhongshan, Zhuhai, and Jiangmen) (Figure 1). As shown in Figure 2, one part of raw water, one part of activated carbon-filtered water, and one part of final water product were collected in each drinking water factories. Since 11 drinking water factories (including seven spring water and four mineral water factories) have two raw water sources, a total of 314 water samples were collected, including 112 raw water samples, 101 activated carbon-filtered water samples, and 101 final water product samples. All samples were placed below 4°C during transportation and testing was performed immediately after receiving the samples.
Isolation and Enumeration of P. aeruginosa
The detection and the enumeration of P. aeruginosa were conducted as described in ISO-16266-2008 International Organization of Standardization [ISO] (2008). Briefly, water sample (250 ml) was filtered through a 0.45-μm membrane (Millipore Co., Billerica, MA, United States) in a stainless steel multi-line filter system (Huankai Co., Guangzhou, China). The membrane was placed on CN agar medium (Huankai Co., Guangzhou, China), a selective medium for P. aeruginosa, and then cultured at 36°C for 48 h. P. aeruginosa colonies were identified based on any of the following: colonies with green color or producing fluorescence and ammonia in ethyl amide broth or forming red color and oxidase positive, producing ammonia in ethyl amide broth and fluorescence on King’s B medium. All P. aeruginosa isolates were further identified by a species-specific ecfX gene. Genomic DNA was extracted using a Bacterial Genomic DNA Purification kit (Dongsheng Biotech, Guangzhou, China), according to the manufacturer’s instruction. P. aeruginosa was identified by amplification of 200-bp fragments with primer pairs ecfX-F (5′ CCTTCCCTCCTTCCCCCCATGCCTATCAGGCGTTCCAT)/ ecfX-R (5′ CCTTCCCTCCTTCCCCCCGGCGATCTGGAAA AGAAATG) (Shi et al., 2012).
Detection of Virulence Genes
Five virulence genes, namely, ExoU, ExoS, phzM, toxA, and lasB, were detected with universal primer multiplex PCR described by Shi et al. (2012). The PCR mixture (30 μl) contained 1 μl template (50 ng), 200 μmol/l dNTPs, 3 μl × 10 Buffer, 1.5 mmol/l Mg2+, primer mixture (0.045 μmol/l EXOU130-F/R, 0.06 μmol/l EXOS276-F/R, 0.09 μmol/l PYO366-F/R, 0.09 μmol/l ETA433-F/R, 0.045 μmol/l ELA556-F/R, and 2.0 μmol/l UP), and 5 U Taq enzyme. The amplification parameters were as follows: initial denaturation at 95°C for 5 min, 30 cycles of 30 s at 95°C, 30 s at 58°C, and 50 s at 72°C, and a final extension at 72°C for 10 min. All primers were synthesized by BGI instrument (Shenzhen, China) (Supplementary Table 1). P. aeruginosa CMCC10104 (Guangdong Culture Collection Centre) was used as the positive control, and distilled water was used as the negative control.
Antibiotic Resistance
Testing the antibiotic resistance of all P. aeruginosa isolates was performed by the Kirby–Bauer disk diffusion method according to the Clinical and Laboratory Standards Institute (CLSI) guidelines (Clinical and Laboratory Standards Institute [CLSI], 2010). P. aeruginosa CMCC 10104 (Guangdong Culture Collection Centre) was tested as positive control. A panel of antibiotics at a specific concentration per disk was tested: ciprofloxacin (5 μg), levofloxacin (5 μg), ofloxacin (5 μg), norfloxacin (10 μg), gentamicin (10 μg), tobramycin (10 μg), amikacin (30 μg), polymyxin B (300 U), imipenem (10 μg), meropenem (10 μg), aztreonam (30 μg), ceftazidime (30 μg), cefepime (30 μg), and piperacillin/tazobactam (110 μg) (Oxoid Co., Hampshire, United Kingdom). All P. aeruginosa isolates were classified as resistant, intermediate, or sensitive using the breakpoints specified by CLSI (Supplementary Table 2).
MLST Analysis
According to the protocols published on the P. aeruginosa pubMLST website,1 MLST analysis was performed using seven housekeeping genes, namely, acsA, aroE, guaA, mutL, nuoD, ppsA, and trpE, and all primers were synthesized by BGI instrument (Shenzhen, China) (Table 1). According to the experimental method described by Curran et al. (2004), the housekeeping genes were amplified for all P. aeruginosa isolates, and PCR products were selected and sent to BGI Instrument (Shenzhen, China) for sequencing. The gene sequences were then compared to the existing sequences on P. aeruginosa pubMLST website2 to assign allele numbers and define sequence types (STs). Then, cluster analysis was conducted using BioNumerics 7.6 software (Applied Maths, Sint-Martens-Latem, Belgium), and a minimum spanning tree was generated from the allelic profiles of the isolates.
Results
Contamination of P. aeruginosa in Drinking Water
In this study, 50 (49.5%) drinking water factories were found to be positive for P. aeruginosa (Supplementary Table 3). Of all the water samples (314), 77 (24.5%) were contaminated with P. aeruginosa, including 34 (30.4%) raw water, 39 (38.6%) activated carbon-filtered water, and four (3.9%) final water products. A total of 132 P. aeruginosa isolates were obtained from the 77 positive samples for P. aeruginosa (Supplementary Table 4). The contamination rates of the different samples are shown in Table 2. Among the 133 mineral water samples, 18 (13.5%) were positive for P. aeruginosa, including eight (17%) raw water and 10 (23.3%) activated carbon-filtered water. The final water product was not found to be positive for P. aeruginosa among the mineral water samples. Among the 181 spring water samples, 59 (32.6%) were positive for P. aeruginosa, including 26 (40%) raw water, 29 (50%) activated carbon-filtered water, and four (6.9%) final water product. Among the 77 contaminated samples with P. aeruginosa (Supplementary Table 3), the contamination level of spring water samples of raw water, activated carbon-filtered water, and final water product was 22.2, 40.2, and 77.5 colony forming units (CFU)/250 ml, respectively, compared with 4.4, 11.8, and 0 CFU/250 ml, respectively, for mineral water samples. Among the 112 raw water samples, there were 98 groundwater and 14 surface raw water samples. As shown in Table 3, 23 (23.5%) underground and six (42.9%) surface raw water samples were contaminated with P. aeruginosa.
Detection of Virulence Genes
In this study, species-specific ecfX gene was found in all 132 P. aeruginosa isolates. The detection results for virulence genes (ExoU, ExoS, phzM, toxA, and lasB) in the 132 isolates are shown in Table 4. Among the isolates, 132 (100%), 126 (95.5%), 118 (89.4%), 10 (7.6%), and 114 (86.3%) were positive for lasB, phzM, toxA, ExoU, and ExoS genes, respectively. The virulence profiles of the isolates are shown in Table 5. Virulence profile B (ExoS, phzM, toxA, and lasB) accounted for 74.2% (n = 98) of all isolates, followed by virulence profile F (ExoS, phzM, and lasB) accounting for 7.6% (n = 10).
Antibiotic Resistance
All 132 isolates were classified as resistant, intermediate, or sensitive according to the diameter of the inhibition zone, as described by CLSI. As shown in Supplementary Figure 1, the result of antimicrobial susceptibilities showed that all 132 isolates were sensitive to 14 antibiotics (ciprofloxacin, levofloxacin, ofloxacin, norfloxacin, gentamicin, tobramycin, amikacin, polymyxin B, imipenem, meropenem, aztreonam, ceftazidime, cefepime, and piperacillin/tazobactam). No P. aeruginosa isolate was resistant to the antibiotics tested.
MLST
All the 132 P. aeruginosa isolates from the 23 cities and different sample types were characterized by MLST analysis, which showed that the isolates could be classified into 42 STs (Supplementary Table 3). ST235 accounted for 8.3% (11) of the total isolates, followed by ST111 at 6.1% (8) and ST277 at 6.1% (8). Additionally, 14 (33.3%) of the 42 STs included a single strain, and eight (19.0%) STs included two isolates. According to the allele numbers of the seven housekeeping genes, a minimum spanning tree was generated to reveal the relationships between the 132 isolates (Figure 3). The STs of the isolates were then further analyzed relative to the sample type and virulence profiles (Figure 3 and Supplementary Figure 2). ST1420, ST1907, ST1974, ST2048, and ST2133 only existed in raw water samples. Five P. aeruginosa isolates from the final water product included five STs (ST175, ST267, ST298, ST324, and ST1182). Furthermore, 11 ST235 isolates included three virulence profiles (B, C, and F), eight ST277 isolates included three virulence profiles (A, B, and D), and eight ST111 isolates showed only one virulence profile (B).
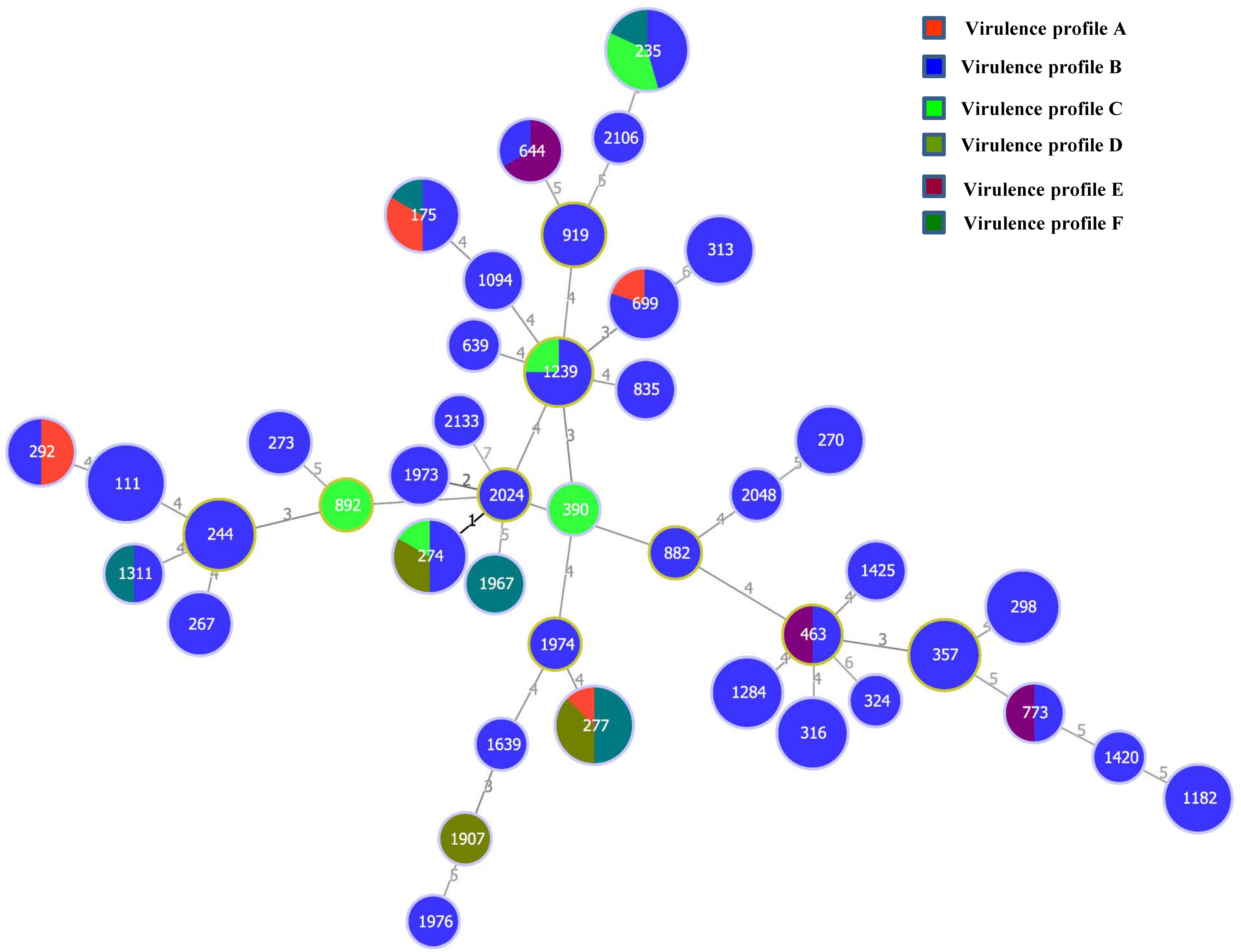
Figure 3. Minimum spanning tree based on multilocus sequence typing data for the 132 P. aeruginosa isolates examined in this study. Each circle represents one sequence type (ST). The size of the circle is related to the number of strains within this ST. The colors in the circles represent the virulence profiles.
Discussion
According to the guidelines on the quality of water intended for human consumption (98/83/EC), drinking water should not test positive for P. aeruginosa. Although the prevalence of P. aeruginosa has been systematically studied in the final water product, the data on P. aeruginosa contamination are lacking for the whole treatment process. In our investigation, a large number of samples (112 raw water samples, 101 activated carbon-filtered water samples, and 101 final water product samples) were collected from 101 drinking water factories covering the whole treatment process, and these data can be used for risk assessment of P. aeruginosa. The results of this study showed that 77 (24.5%) samples were contaminated with P. aeruginosa among the 314 water samples. Consistent with previous investigations conducted by Wu et al. (2016), the present result showed that P. aeruginosa contamination of raw water (30.4%) and activated carbon-filtered water (38.6%) was significantly higher than that of raw water (3.9%). Notably, the contamination rate of surface raw water was significantly higher than that of ground raw water. Thus, groundwater is better than surface water as raw water. The main reason for this is that surface raw water is generally drained from pipes to open cistern and is exposed to air for a long time without effective protection measures. In this study, the P. aeruginosa contamination rate of activated carbon-filtered water was the highest among the three kinds of water sample tested. Activated carbon filter system is one of the most important processes in drinking water treatment and is used to adsorb organic pollutants and microbes to purify water (Feng et al., 2013). The P. aeruginosa contamination rate of activated carbon-filtered water can be used to indicate the degree of production specification. Our previous studies have shown that activated carbon filter can get enriched with microorganisms and is the most serious microbial contamination problem in the whole treatment process of drinking water (Wei et al., 2017). Therefore, manufacturers of drinking water must clean, disinfect, and replace activated carbon filters regularly. Stoler et al. (2015) reported that 41% of 80 sachet water samples were positive for P. aeruginosa. In this study, no P. aeruginosa was detected in the final water product of mineral water. However, the contamination rate of the final water product was 6.9% in the case of spring water, which is harmful to the health of the costumers. The high contamination rate of P. aeruginosa in spring water may be caused by the irregular production process during the manufacturing process, including inadequate disinfection, the formation of biofilms of P. aeruginosa in the pipeline, and improper maintenance of activated carbon filter. Hence, manufacturers of drinking water should pay attention to the control and management of the production process to ensure the safety of drinking water.
The pathogenesis of P. aeruginosa is closely related to the virulence genes ExoU, ExoS, phzM, toxA, and lasB (Mavrodi et al., 2001; Strateva et al., 2010; Shi et al., 2012). In this study, all 132 isolates carried at least three virulence genes, a fact which was in contrast to a previous study (Wu et al., 2016). Notably, 132 (100%), 126 (95.5%), 118 (89.4%), 10 (7.6%), and 114 (86.3%) isolates were positive for lasB, phzM, toxA, ExoU, and ExoS genes, respectively, indicating that these virulence genes are widely distributed among P. aeruginosa. Consistent with previous investigations, the virulence genes ExoU and ExoS were not detected in the same isolates (Wolfgang et al., 2003; Shaver and Hauser, 2004). Furthermore, among the six virulent profiles of all isolates (Table 5), virulence profile B was predominant (ExoS, phzM, toxA, and lasB), accounting for 74.2% (n = 98) of the isolates. Importantly, the presence of the virulence genes indicates that P. aeruginosa is a potential pathogenic bacterium. The virulence gene expression in P. aeruginosa is mainly related to quorum sensing. Therefore, future studies should be conducted to study how these virulence genes are expressed in P. aeruginosa. In previous studies, clinical P. aeruginosa was generally resistant to antibiotics (Keating et al., 2013; Munita et al., 2017). However, no P. aeruginosa isolate was resistant to the 14 different antibiotics tested in our study. Silva et al. (2008) isolated 30 P. aeruginosa strains from drinking water that were resistant to one or more antibiotics. The difference in the results may be due to the different sources of the samples. In this study, the raw water mainly came from groundwater, and the small amount of surface raw water samples was also taken from sparsely populated remote areas, with less exposure to outside influences. These environments are rarely contaminated with antibiotics, so P. aeruginosa has not been induced to be antibiotic resistant.
Multilocus sequence typing is a crucial epidemiological typing method and is based on the sequences of seven different housekeeping genes. It has been used in studies on the evolution and population diversity of P. aeruginosa isolates and is advantageous, owing to its discriminatory value and rapid P. aeruginosa typing (Castañeda-Montes et al., 2018; Kainuma et al., 2018). In this study, the MLST results provided a better overview of P. aeruginosa diversity. Of the 42 STs, 14 (33.3%) included a single strain and eight (19.0%) included two isolates, indicating the high genetic diversity of the isolates. As shown in Figure 3, a good correlation among MLST and virulence profiles was found in some isolates. However, there was no significant correlation between ST type and sample type (Supplementary Figure 2). All isolates that belonged to ST111 (n = 8), ST298 (n = 6), ST244 (n = 5), ST316 (n = 6), ST1284 (n = 5), and ST357 (n = 5) showed the same virulence profile B (ExoS, phzM, toxA, and lasB). As no antibiotic-resistant isolate was found in this study, no correlation was observed between the MLST profiles and the antibiotic resistance profiles of the isolates.
In summary, this study investigated the prevalence, virulence, antimicrobial resistance, and molecular characteristics of P. aeruginosa isolates from drinking water samples across different cities of China. Of the water samples tested, 24.5% were contaminated, and the contamination rate of the final water product was 6.9% in the case of spring water, suggesting that drinking water products could be a potential vehicle for the transmission of P. aeruginosa. In addition, since activated carbon filters, the most serious potential contamination site in the whole production process, also showed a high degree of P. aeruginosa contamination, manufacturers of drinking water should regularly clean, disinfect, and replace activated carbon filters. Furthermore, our data indicated that all 132 isolates carried at least three virulence genes, indicating that they may have a potentially pathogenic effect on the health of consumers. The MLST data from this study can be used to trace the possible origins of P. aeruginosa, which would aid in developing effective and precise prevention and control measures against P. aeruginosa contamination of the whole drinking water production process.
Data Availability Statement
The original contributions presented in the study are included in the article/Supplementary Material, further inquiries can be directed to the corresponding author.
Author Contributions
QW, JZ, and LW conceived and designed the experiments. LW, XW, and WG performed the experiments. LW, QG, and JW analyzed the data. LX, YZ, and XZ contributed reagents, materials, and analysis tools. LW, HW, and TL contributed to the writing of the manuscript. All authors contributed to the article and approved the submitted version.
Funding
We would like to acknowledge the financial support of the National Key R&D Program of China (2018YFD0400901), the Local Innovative and Research Teams Project of Guangdong Pearl River Talents Program (2017BT01S174), the GDAS’ Project of Science and Technology Development (2018GDASCX-0910), and China Postdoctoral Science Foundation (2019M662829).
Conflict of Interest
The authors declare that the research was conducted in the absence of any commercial or financial relationships that could be construed as a potential conflict of interest.
Supplementary Material
The Supplementary Material for this article can be found online at: https://www.frontiersin.org/articles/10.3389/fmicb.2020.544653/full#supplementary-material
Footnotes
References
Agarwal, G., Kapil, A., Kabra, S. K., Das, B. K., and Dwivedi, S. N. (2005). Characterization of Pseudomonas aeruginosa isolated from chronically infected children with cystic fibrosis in India. BMC Microbiol. 5:43. doi: 10.1186/1471-2180-5-43
Bel Hadj Ahmed, A., Salah Abbassi, M., Rojo-Bezares, B., Ruiz-Roldán, L., Dhahri, R., Mehri, I., et al. (2019). Characterization of Pseudomonas aeruginosa isolated from various environmental niches: new STs and occurrence of antibiotic susceptible “high-risk clones”. Intern. J. Environ. Health Res. 30, 643–652. doi: 10.1080/09603123.2019.1616080
Bradbury, R. S., Roddam, L. F., Merritt, A., Reid, D. W., and Champion, A. C. (2010). Virulence gene distribution in clinical, nosocomial and environmental isolates of Pseudomonas aeruginosa. J. Med. Microbiol. 59, 881–890. doi: 10.1099/jmm.0.018283-0
Castañeda-Montes, F. J., Avitia, M., Sepúlveda-Robles, O., Cruz-Sánchez, V., Kameyama, L., Guarneros, G., et al. (2018). Population structure of Pseudomonas aeruginosa through a MLST approach and antibiotic resistance profiling of a Mexican clinical collection. Infect. Genet. Evol. 65, 43–54. doi: 10.1016/j.meegid.2018.06.009
Clinical and Laboratory Standards Institute [CLSI] (2010). Performance Standards for Antimicrobial Disk Susceptibility Tests. Wayne, PA: CLSI.
Curran, B., Jonas, D., Grundmann, H., Pitt, T., and Dowson, C. G. (2004). Development of a multilocus sequence typing scheme for the opportunistic pathogen Pseudomonas aeruginosa. J. Clin. Microbiol. 42, 5644–5649. doi: 10.1128/jcm.42.12.5644-5649.2004
De Cesare, A., Parisi, A., Giacometti, F., Serraino, A., Piva, S., Caruso, M., et al. (2016). Multilocus sequence typing of Arcobacter butzleri isolates collected from dairy plants and their products, and comparison with their PFGE types. J. Appl. Microbiol. 120, 165–174. doi: 10.1111/jam.12977
Driscoll, J. A., Brody, S. L., and Kollef, M. H. (2007). The epidemiology, pathogenesis and treatment of Pseudomonas aeruginosa infections. Drugs 67, 351–368. doi: 10.2165/00003495-200767030-00003
Feinbaum, R. L., Urbach, J. M., Liberati, N. T., Djonovic, S., Adonizio, A., Carvunis, A.-R., et al. (2012). Genome-wide identification of Pseudomonas aeruginosa virulence-related genes using a Caenorhabditis elegans infection model. PLoS Pathog. 8:e1002813. doi: 10.1371/journal.ppat.1002813
Feng, S., Chen, C., Wang, Q. F., Zhang, X. J., Yang, Z. Y., and Xie, S. G. (2013). Characterization of microbial communities in a granular activated carbon-sand dual media filter for drinking water treatment. Intern. J. Environ. Sci. Technol. 10, 917–922. doi: 10.1007/s13762-013-0188-1
Guerin-Mechin, L., Dubois-Brissonnet, F., Heyd, B., and Leveau, J. (2000). Quaternary ammonium compound stresses induce specific variations in fatty acid composition of Pseudomonas aeruginosa. Intern. J. Food Microbiol. 55, 157–159. doi: 10.1016/s0168-1605(00)00189-6
Horna, G., Amaro, C., Palacios, A., Guerra, H., and Ruiz, J. (2019). High frequency of the exoU+/exoS+ genotype associated with multidrug-resistant “high-risk clones” of Pseudomonas aeruginosa clinical isolates from Peruvian hospitals. Sci. Rep. 9:10874.
International Organization of Standardization [ISO] (2008). Water Quality-Detection and Enumeration of Pseudomon asaeruginosa-Method by Membrane Filtration. Geneva: ISO.
Johnson, J. K., Arduino, S. M., Stine, O. C., Johnson, J. A., and Harris, A. D. (2007). Multilocus sequence typing compared to pulsed-field gel electrophoresis for molecular typing of Pseudomonas aeruginosa. J. Clin. Microbiol. 45, 3707–3712. doi: 10.1128/jcm.00560-07
Kainuma, A., Momiyama, K., Kimura, T., Akiyama, K., Inoue, K., Naito, Y., et al. (2018). An outbreak of fluoroquinolone-resistant Pseudomonas aeruginosa ST357 harboring the exoU gene. J. Infect. Chemother. 24, 615–622. doi: 10.1016/j.jiac.2018.03.008
Keating, D., Crowe, M. J., Kennedy, B., Salmon, A., Britton, D., Gallagher, C. G., et al. (2013). Molecular detection of an atypical, highly resistant, clonal Pseudomonas aeruginosa isolate in cystic fibrosis patients. J. Cystic Fibros. 12, 141–146. doi: 10.1016/j.jcf.2012.07.007
Li, C., Ling, F., Zhang, M., Liu, W.-T., Li, Y., and Liu, W. (2017). Characterization of bacterial community dynamics in a full-scale drinking water treatment plant. J. Environ. Sci. 51, 21–30. doi: 10.1016/j.jes.2016.05.042
Lu, J., Struewing, I., Vereen, E., Kirby, A. E., Levy, K., Moe, C., et al. (2016). Molecular detection of Legionella spp. and their associations with Mycobacterium spp., Pseudomonas aeruginosa and amoeba hosts in a drinking water distribution system. J. Appl. Microbiol. 120, 509–521.
Luo, R. G., Miao, X. Y., Luo, L. L., Mao, B., Yu, F. Y., and Xu, J. F. (2019). Presence of pldA and exoU in mucoid Pseudomonas aeruginosa is associated with high risk of exacerbations in non-cystic fibrosis bronchiectasis patients. Clin. Microbiol. Infect. 25, 601–606. doi: 10.1016/j.cmi.2018.07.008
Mah, T.-F., Pitts, B., Pellock, B., Walker, G. C., Stewart, P. S., and O’toole, G. A. (2003). A genetic basis for Pseudomonas aeruginosa biofilm antibiotic resistance. Nature 426, 306–310. doi: 10.1038/nature02122
Mavrodi, D., Bonsall, R., Delaney, S., Soule, M., Phillips, G., and Thomashow, L. (2001). Functional analysis of genes for biosynthesis of pyocyanin and phenazine-1 carboxamide from Pseudomonas aeruginosa PAO1. J. Bacteriol. 183, 6454–6465. doi: 10.1128/jb.183.21.6454-6465.2001
Mena, K. D., and Gerba, C. P. (2009). “Risk assessment of Pseudomonas aeruginosa in water,” in Reviews of Environmental Contamination and Toxicology, Vol. 201, ed. D. M. Whitacre (Boston, MA: Springer), 71–115. doi: 10.1007/978-1-4419-0032-6_3
Munita, J. M., Aitken, S. L., Miller, W. R., Perez, F., Rosa, R., Shimose, L. A., et al. (2017). Multicenter evaluation of Ceftolozane/Tazobactam for serious infections caused by carbapenem-resistant Pseudomonas aeruginosa. Clin. Infect. Dis. 65, 158–161. doi: 10.1093/cid/cix014
Parsons, J. F., Greenhagen, B. T., Shi, K., Calabrese, K., Robinson, H., and Ladner, J. E. (2007). Structural and functional analysis of the pyocyanin biosynthetic protein PhzM from Pseudomonas aeruginosa. Biochemistry 46, 1821–1828. doi: 10.1021/bi6024403
Shaver, C. M., and Hauser, A. R. (2004). Relative contributions of Pseudomonas aeruginosa ExoU, ExoS, and ExoT to virulence in the lung. Infect. Immun. 72, 6969–6977. doi: 10.1128/iai.72.12.6969-6977.2004
Shi, H., Trinh, Q., Xu, W., Zhai, B., Luo, Y., and Huang, K. (2012). A universal primer multiplex PCR method for typing of toxinogenic Pseudomonas aeruginosa. Appl. Microbiol. Biotechnol. 95, 1579–1587. doi: 10.1007/s00253-012-4277-8
Silva, M. E. Z. D., Filho, I. C., Endo, E. H., Nakamura, C. V., Ueda-Nakamura, T., and Filho, B. P. D. (2008). Characterisation of potential virulence markers in Pseudomonas aeruginosa isolated from drinking water. Antonie Leeuwenhoek 93, 323–334. doi: 10.1007/s10482-007-9209-8
Slekovec, C., Robert, J., Van Der Mee-Marquet, N., Berthelot, P., Rogues, A.-M., Derouin, V., et al. (2019). Molecular epidemiology of Pseudomonas aeruginosa isolated from infected ICU patients: a French multicenter 2012-2013 study. Eur. J. Clin. Microbiol. Infect. Dis. 38, 921–926. doi: 10.1007/s10096-019-03519-w
Stoler, J., Ahmed, H., Asantewa Frimpong, L., and Bello, M. (2015). Presence of Pseudomonas aeruginosa in coliform-free sachet drinking water in Ghana. Food Control 55, 242–247. doi: 10.1016/j.foodcont.2015.02.038
Strateva, T., Markova, B., Ivanova, D., and Mitov, I. (2010). Distribution of the type III effector proteins-encoding genes among nosocomial Pseudomonas aeruginosa isolates from Bulgaria. Ann. Microbiol. 60, 503–509. doi: 10.1007/s13213-010-0079-3
Vaz-Moreira, I., Nunes, O. C., and Manaia, C. M. (2012). Diversity and antibiotic resistance in Pseudomonas spp. from drinking water. Sci. Total Environ. 426, 366–374. doi: 10.1016/j.scitotenv.2012.03.046
Wang, F., Li, W., Li, Y., Zhang, J., Chen, J., Zhang, W., et al. (2018). Molecular analysis of bacterial community in the tap water with different water ages of a drinking water distribution system. Front. Environ. Sci. Eng. 12:6. doi: 10.1007/s11783-018-1020-4
Wedekind, J. E., Trame, C. B., Dorywalska, M., Koehl, P., Raschke, T. M., Mckee, M., et al. (2001). Refined crystallographic structure of Pseudomonas aeruginosa exotoxin A and its implications for the molecular mechanism of toxicity. J. Mol. Biol. 314, 823–837. doi: 10.1006/jmbi.2001.5195
Wei, L., Wu, Q., Zhang, J., Guo, W., Chen, M., Xue, L., et al. (2017). Prevalence and genetic diversity of Enterococcus faecalis isolates from mineral water and spring water in China. Front. Microbiol. 8:1109. doi: 10.3389/fmicb.2017.01109
Wolfgang, M., Kulasekara, B., Liang, X., Boyd, D., Wu, K., Yang, Q., et al. (2003). Conservation of genome content and virulence determinants among clinical and environmental isolates of Pseudomonas aeruginosa. Proc. Natl. Acad. Sci. U.S.A. 100, 8484–8489. doi: 10.1073/pnas.0832438100
Keywords: Pseudomonas aeruginosa, mineral water, spring water, virulence genes, multilocus sequence typing
Citation: Wei L, Wu Q, Zhang J, Guo W, Gu Q, Wu H, Wang J, Lei T, Xue L, Zhang Y, Wei X and Zeng X (2020) Prevalence, Virulence, Antimicrobial Resistance, and Molecular Characterization of Pseudomonas aeruginosa Isolates From Drinking Water in China. Front. Microbiol. 11:544653. doi: 10.3389/fmicb.2020.544653
Received: 21 March 2020; Accepted: 02 November 2020;
Published: 03 December 2020.
Edited by:
Natalia V. Kirienko, Rice University, United StatesReviewed by:
Yanhong Liu, United States Department of Agriculture (USDA), United StatesDingqiang Chen, Zhujiang Hospital, Southern Medical University, China
Yanmei Li, University of Maryland, Baltimore, United States
Guangchao Yu, Jinan University, China
Copyright © 2020 Wei, Wu, Zhang, Guo, Gu, Wu, Wang, Lei, Xue, Zhang, Wei and Zeng. This is an open-access article distributed under the terms of the Creative Commons Attribution License (CC BY). The use, distribution or reproduction in other forums is permitted, provided the original author(s) and the copyright owner(s) are credited and that the original publication in this journal is cited, in accordance with accepted academic practice. No use, distribution or reproduction is permitted which does not comply with these terms.
*Correspondence: Qingping Wu, d3VxcDIwM0AxNjMuY29t