- Department of Laboratory Medicine, The Third Xiangya Hospital, Central South University, Changsha, China
Recently, the problem of bacterial resistance has been brought into focus, which makes the development of new antibiotics become a necessity. Compared with traditional development approaches, drug repurposing provides a faster and more effective approach to find new antimicrobial agents. In this study, we found that antispasmodic agent otilonium bromide had strong antibacterial ability and bactericidal activity against Staphylococcus aureus, with minimal inhibitory concentrations (MICs) of 4–8 μg/ml, and bacteria could be killed completely after treatment with 2× MIC of otilonium bromide for 5 h. Furthermore, it had a potent effect on eradicating biofilm at concentrations ranging from 16 to 64 μg/ml. At the same time, it had low tendency to develop resistance and possessed limited cytotoxicity. In the methicillin-resistant S. aureus–infected mouse peritonitis model, it was also effective to cure mice and improve their survival rate. In addition, we observed that otilonium bromide changed the permeability of bacterial membrane and caused membrane damage, and it is probably the antibacterial mechanism of otilonium bromide. Taken together, our results indicated that otilonium bromide could be a new antimicrobial agent to treat S. aureus infections more safely and efficiently.
Introduction
Staphylococcus aureus is a common pathogen that can cause hospital-acquired infections, and its isolation rate was very high among Gram-positive bacteria (Gould et al., 2012). It can cause various diseases such as pneumonia, catheter-related infections, and sepsis (David and Daum, 2017). In addition, owing to the overuse of antibiotics, the problem of bacterial resistance has become more and more serious. The detection rate of methicillin-resistant S. aureus (MRSA) has risen continuously, which poses a huge challenge to clinical treatment. Therefore, how to treat various diseases caused by S. aureus efficiently and economically has become an urgent problem.
Recently, greater attention has been paid to drug repurposing which is a strategy to explore new antimicrobial agents. Most of the drugs discovered through this approach have detailed information about safety and pharmacokinetic profiles, which reduces the cost and time for developing new drugs and accelerates its application in clinical treatment (Miró-Canturri et al., 2019).
Quaternary amine compound otilonium bromide (OB) (Figure 1) is a FDA-certified antispasmodic agent which is commonly used in the treatment of irritable bowel syndrome (IBS). The main mode of action is blocking the L-type Ca2+ channels on smooth muscles and interfering with intracytoplasmatic Ca2+ mobilization, thus relieving excessive intestinal contractions and abdominal pain (Evangelista et al., 2018). It has been reported that OB has potential antibacterial activity against multidrug-resistant Acinetobacter baumannii and S. aureus by damaging cell membrane and disrupting cellular protein homeostasis (Knauf et al., 2018). However, there has been no systematic study to evaluate its antibacterial property, toxicity, and in vivo efficacy. Here, evaluating the antibacterial activity of OB in vitro and in vivo, we believe that OB has a possibility to be used as a novel antibacterial agent for the treatment of staphylococcal infections.
Materials and Methods
Bacterial Strains and Growth Conditions
Ten clinical isolations of S. aureus were collected from patients admitted in the Third Xiangya Hospital of Central South University. S. aureus Newman and ATCC 43300 were provided by Min Li (Shanghai Jiao Tong University, Shanghai, China). Staphylococcus epidermidis RP62A (ATCC 35984) was provided by Di Qu (Fudan University, Shanghai, China). Pseudomonas aeruginosa PAO1 (ATCC 15692) was obtained from Mingqiang Qiao (Nankai University, Tianjin, China). Enterococcus faecalis ATCC 29212, A. baumannii ATCC 1195, Klebsiella pneumoniae ATCC 700603, Escherichia coli ATCC 25922, and S. aureus ATCC 29213 were purchased from the American Type Culture Collection. S. aureus and S. epidermidis strains were grown in tryptic soy broth (TSB) (Solarbio, Beijing, China) medium. E. faecalis strain was cultured in brain–heart infusion broth (Solarbio) medium. Other strains were grown in Luria–Bertani broth (Solarbio) medium.
Reagent Preparation
Oxacillin, vancomycin, OB, melittin, and polymyxin B nonapeptide (PMBN) were purchased from MedChem Express (NJ, United States). Ciprofloxacin and rifampin were purchased from Aladdin (Shanghai, China). Oxacillin, vancomycin, ciprofloxacin, and melittin were prepared in deionized water at 6.4, 25, 6.4, and 1 mg/ml, respectively. OB, PMBN, and rifampin were prepared in DMSO at 100, 10, and 10 mg/ml, respectively.
Antibacterial Assays
The minimal inhibitory concentration (MIC) was determined by microdilution method according to Clinical and Laboratory Standards Institute Guidelines (Harbut et al., 2015). After determining the MIC, 5 μl of bacterial cultures was taken from wells in which the drug concentration was equal to or higher than the MIC, and they were plated on blood agar plates. The minimum bactericidal concentration (MBC) was defined as the lowest concentration for which no bacterial colonies were grown on plates after incubation for 24 h at 37°C (Tan et al., 2019). All experiments were repeated in triplicate.
Time-Kill Assay
An overnight culture of S. aureus ATCC 43300 and ATCC 29213 was adjusted to cell concentration of 1 × 106 CFU/ml in Mueller–Hinton broth (MHB) (Solarbio) medium and was incubated with 0.5× MIC, 1× MIC, 2× MIC, and 4× MIC of OB or without drug. The cultures were incubated at 37°C with shaking at 180 rpm. Samples were taken at 0, 1, 2, 3, 4, 5, 6, 12, and 24 h, then diluted and plated on blood agar plates. After incubation at 37°C for 24 h, colonies were counted and viable cells were determined (CFU/ml) (Thakare et al., 2017). The experiment was repeated in triplicate.
Checkerboard Assay
The bacterial suspension at mid-log phase was adjusted to 1 × 106CFU/ml and dispensed into 96-well microtiter plates (Corning/Costar, United States), and then a two-dimensional checkerboard with serial dilutions of OB and PMBN (ranging from 1 to 128 μg/ml) were set up. The results were detected by a microplate reader (iMark Microplate Absorbance Reader; BIO-RAD, United States) at OD630 nm after incubation at 37°C for 18–24 h. The experiment was repeated in triplicate and the fractional inhibitory concentration index (FICI) was calculated by using the following formula:
Classification criteria: FICI ≤ 0.5 is synergistic; 0.5 < FICI ≤ 1 is additive; 1 < FICI ≤ 4 is irrelevant; >4 is antagonistic (Pengfei et al., 2020).
Anti-biofilm Assays
All S. aureus strains including 10 clinical strains were inoculated into TSB medium with 1% glucose and cultured at 37°C for 24 h, with shaking at 180 rpm. Then 200 μl of bacterial suspension (100-fold dilution) was added to 96-well microtiter plates to form biofilm. After incubation at 37°C for 24 h without shaking, the plates were washed with PBS to remove planktonic cells, stained with 0.5% crystal violet for 15 min, and washed with PBS to remove excess dye. Then the stained dye was dissolved with 200 μl of 95% alcohol for 20 min. The absorbance (A570 nm) was measured in a microplate reader and the biofilm-forming capacity of all S. aureus strains was classified according to the criteria as previously reported (Supplementary Table S1; Hassan et al., 2011). Subsequently, nine strains that were classified as strong biofilm producers were selected to form biofilm as described previously. After 24 h, the medium was discarded and the plates were washed. Biofilm was treated with drugs (ranging from 4 to 128 μg/ml) in 200 μl of TSB medium for 24 h at 37°C. As a control, biofilm was exposed to TSB medium without drug. Finally, the plates were washed again and stained with 0.5% crystal violet. MBEC was defined as the lowest drug concentration of drug which eradicated biofilm by 50% (MBEC50), relative to the drug-free control well (Melo et al., 2011). All experiments were repeated in triplicate.
qPCR
Briefly, S. aureus ATCC 43300 was cultured in a 6-well microtiter plate and treated with or without OB for 24 h. The total RNA was extracted using E.Z.N.A. Bacterial RNA Kit (Omega, United States), cDNA was prepared using TransScript All-in-One First-Strand cDNA Synthesis SuperMix (Transgene, Beijing, China), and qPCR was performed with TransStart Tip Green qPCR SuperMix (Transgene) using a CFX96 Real-Time PCR Detection System (Bio-Rad Laboratories, United Kingdom) (94°Cfor 5 s, 40 cycles of 94°C for 5 s, 58°C for 15 s, and 72°C for 10 s). Primers were used as follows: icaA forward, ACACTTGCTGGCGCAGTCAA; icaA reverse, TCTGGAACCAACATCCAACA; icaD forward, ATGGTCAAGCCCAGACAGAG; and icaD reverse, AGTATTTTCAATGTTTAAAGCAA (Haddad et al., 2018). The primer 16S RNA was used as an internal standard and the experiment was repeated in triplicate.
Confocal Laser Scanning Microscope
The efficiency of OB in eradicating biofilm was visually assessed by confocal laser scanning microscope (CLSM). Briefly, an overnight culture of MRSA ATCC 43300 and SA 1420 (clinical strain) was diluted 1:100 with TSB medium, and 2 ml of bacterial suspension was added to a 6-well microtiter plate. Next, sterile glass slides were placed into the plate to form mature biofilm. After incubation at 37°C for 24 h without shaking, the slides were washed with PBS, treated with 4× MIC or 8× MIC of OB, and incubated for another 24 h. As a control, the slides were not treated with drug. The slides were then washed again, stained with SYTO9 fluorescence dye (Thermo Fisher Scientific, United States) according to the manufacturer’s instructions, and observed by CLSM (Zeiss LSM800, Germany). The biofilm quantification was performed with ZEN 3.0 (blue edition) software (She et al., 2019).
Resistance Selection
Single-step resistance selection and multi-step resistance selection were applied to evaluate the drug resistance of S. aureus to OB. For single-step resistance selection, an overnight culture of S. aureus ATCC 43300 and ATCC 29213 was prepared in TSB medium to an OD630 nm of 0.5. Then, 100 μl of diluted bacterial cultures was plated on MH agar (without drug or with 2× MIC, 4× MIC of ciprofloxacin, rifampicin, and OB) to quantitate the starting inoculum or resistant colonies. After incubation at 37°C for 48 h, the resistance frequency was calculated as the number of drug-resistant mutants divided by the number of total colonies (Trombetta et al., 2018). For multi-step resistance selection, on the first day, the MICs of OB and ciprofloxacin against S. aureus ATCC 43300 and ATCC 29213 were determined as previously described. After incubation for 16–18 h, 5 μl of bacterial cultures was taken from wells where the drug concentration was 0.5× MIC and was diluted 1000-fold with MHB medium. Afterward, 50 μl of bacterial suspension and 50 μl of medium containing a serially diluted drug were added to 96-well microtiter plates for the next-day MIC assay, and the protocol was repeated 20 times (Friedman et al., 2006). All experiments were repeated in triplicate.
Membrane Permeability Assays
The effect of OB on the bacterial membrane of S. aureus was assessed by two fluorescence dyes, SYTOX Green and Disc3(5). Nucleic acid stain SYTOX Green could not cross over intact membrane, but it could penetrate through compromised membrane and combine with nucleic acid to exhibit bright green fluorescence (>500-fold fluorescence enhancement). Cationic dye Disc3(5) could detect the membrane depolarization and increase the fluorescence signal (Roth et al., 1997; Miyazaki et al., 2017). Briefly, S. aureus ATCC 43300 and ATCC 29213 in mid-log growth-phases were suspended in 5 mM HEPES (pH 7.2) with an OD630nm of 0.05. The bacterial suspension was incubated with 2 μM SYTOX Green (Thermo Fisher Scientific, United States) in dark for 15 min and then was treated with serially diluted OB. The fluorescence intensity was detected by a microplate reader (PerkinElmer EnVision, United States) for 30 min at the excitation wavelength of 504 nm and emission wavelength of 523 nm (Koh et al., 2013; Lee et al., 2013). The detection of membrane depolarization was slightly different. The bacterial suspension was incubated with 5 mM glucose, 100 mM KCl, and 2 μM Disc3(5) (AAT Bioquest, United States) in dark for 1 h, at which time different concentrations of OB were added and the fluorescence intensity monitored every 30 s for 5 min (excitation wavelength 622 nm; emission wavelength 670 nm) (Miyazaki et al., 2017). Melittin (20 μg/ml) was used as a positive control, and HEPES with 0.1% DMSO was used as a negative control. All experiments were repeated in triplicate.
The β-galactosidase assay was used to detect the inner membrane integrity of E. coli. If the cell membrane was destroyed, ortho-nitrophenol-β-galactoside (ONPG) (Sigma-Aldrich, United States) could enter the cytoplasm and be converted to yellow compound O-nitrophenol by reacting with β-galactosidase produced by E. coli. Briefly, E. coli ATCC 25922 in mid-log growth phase was suspended in PBS with an OD405 nm of 1.2. Thereafter, 100 μl of bacterial suspension, 50 μl of 5 mM ONPG, and 50 μl of OB (0.5× MIC, 1× MIC, and 2× MIC) or DMSO (negative control) were added to the 96-well microtiter plate. The time-dependent color changes of product were monitored by a microplate reader at 405 nm every 10 min for 1.5 h (Han et al., 2016).
Transmission Electron Microscopy
S. aureus ATCC 43300 in mid-log growth phase was suspended in PBS to 2 × 109CFU/ml and was treated with 8× MIC of OB for 1 h at 37°C. As a control, bacteria were not treated with drug. The bacterial suspension was centrifuged at 4000 × g for 5 min to harvest the cell pellets, then the specimens were observed by transmission electron microscope (HITACHI HT7700, Japan) as previously mentioned (de Breij et al., 2018).
Hemolysis Assay and Cytotoxicity Test
Blood samples were collected from a healthy individual at the Third Xiangya Hospital of Central South University and centrifuged at 1000 × g, 4°C for 5 min to harvest the human red blood cells (RBCs). RBCs were washed with PBS three times and suspended in PBS. Thereafter, RBC suspension (100 μl) and serially diluted OB (100 μl) were added to the 96-well microtiter plate to make the final RBC concentration of 4% (v/v). After incubation at 37°C for 1 h, 100 μl of supernatant was taken and the absorbance (A570 nm) was detected. In addition, 0.1% DMSO served as a negative control, 0.1% Triton X-100 was used as a positive control (Gerits et al., 2017). The hemolysis rate was calculated by using the following formula:
The Cell Counting Kit-8 (DojinDo, Japan) was used to test the cytotoxicity of OB on human breast cancer cell line KPL-4 and human colon epithelial cell NCM-460. KPL-4 cells were cultured with DMEM medium containing 10% fetal bovine serum, and NCM-460 cells were grown in RPMI-1640 medium supplemented with 10% fetal bovine serum. Briefly, cells (100 μl) were seeded into 96-well microtiter plates at 4000 cells/well and cultured at 37°C with 5% CO2 for 24 h to make them adhere. Then, the supernatant was discarded and cells were treated with 100 μl of serially diluted OB or 0.1% DMSO for another 24 h. Finally, 10 μl of CCK-8 was added to each well and the absorbance (A450nm) was recorded after 3 h (Tan et al., 2019). The cell viability was calculated according to the following formula:
All experiments were repeated in triplicate.
Mouse Peritonitis Model and in vivo Toxicity
All animal studies were conducted under the approval of the Ethics Committee of the Third Xiangya Hospital, Central South University. The mice used in the experiment were female ICR, 24–26 g, purchased from Hunan SJA Experimental Animal Co. Ltd. (Changsha, China). Mice were maintained on a 12:12 light/dark cycle at 22–24°C in polycarbonate cages. Adequate water and food were provided in accordance with the relevant requirements of animal ethics. For in vivo toxicity determination, the mice (n = 5) were intraperitoneally injected twice (4-h interval) with 40 mg/kg of OB or DMSO (vehicle) and observed for 7 days. In addition, 40 mg/kg of OB or DMSO (vehicle) was injected (i.p.) into mice (n = 5) daily for 7 days and the mice were observed for 5 days after the final injection. On the 12th day, the mice were sacrificed and their blood and tissues were harvested for blood cells analysis and histological analysis (H&E staining). The MRSA-infected mouse peritonitis model was established as previously described (Gómez-Zorrilla et al., 2017; Su et al., 2019). Briefly, the mice were intraperitoneally injected with 500 μl of (2–3) × 108 CFU/ml bacterial suspension (S. aureus ATCC 43300) containing 5% mucin (Cool Chemistry, Beijing, China). Then, groups of mice (n = 5) were given 30 mg/kg of OB, 40 mg/kg of OB, 50 mg/kg of vancomycin (positive control), or saline with 2% DMSO (vehicle) at 1 and 5 h post-infection through intraperitoneal injection. The survival condition and weight changes of the mice were observed continuously for 7 days. The survived mice were euthanized on the seventh day and their liver and kidney were homogenized and plated on blood agar plates to measure the number of viable bacteria.
Results
Antimicrobial Activities of OB Against S. aureus
The results of the MIC and MBC assays are shown in Table 1. S. aureus ATCC 43300 (MRSA) and four clinical isolates of MRSA were resistant to oxacillin (MICs ≥ 64 μg/ml; MBCs ≥ 128 μg/ml). All strains were sensitive to vancomycin, with MICs of 0.25–1 μg/ml and MBCs of 0.5–8 μg/ml. In addition, the spasmolytic agent OB also showed antimicrobial activity against S. aureus, with MICs of 4–8 μg/ml and MBCs of 8–16 μg/ml, as previously reported (Knauf et al., 2018).
To investigate whether the bactericidal activity of OB on S. aureus ATCC 43300 and ATCC 29213 was in a time-dependent and dose-dependent manner, time-kill assays were conducted (Figures 2A,B). Bactericidal activity was observed when S. aureus ATCC 43300 and ATCC 29213 were treated with 2× MIC of OB. No viable bacteria were observed after 5 h. Treatment with 1× MIC of OB also inhibited the growth of S. aureus ATCC 43300 and ATCC 29213, with a reduction in viable cell counts by 5-log10 and 4-log10 after 24 h, respectively.
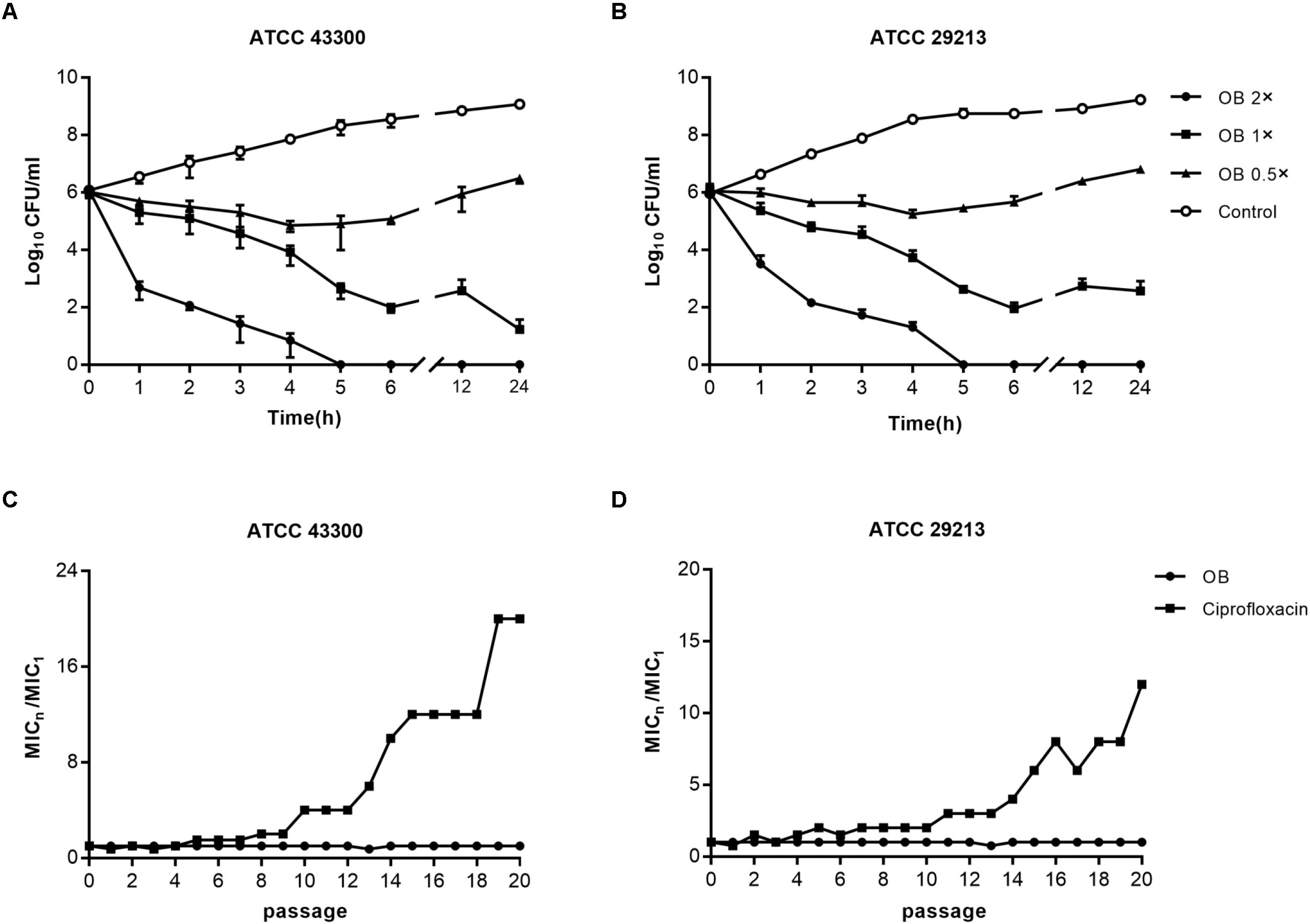
Figure 2. The bactericidal activity of OB against S. aureus and resistance development of S. aureus to OB. Time-kill assays of OB against S. aureus ATCC 43300 (A) and ATCC 29213 (B). S. aureus strains were treated with 0.5× MIC, 1× MIC, 2× MIC of OB and DMSO with PBS (solvent control), respectively. Samples were taken at 0, 1, 2, 3, 4, 5, 6, 12, and 24 h, and the number of viable bacteria was counted as CFU/ml. The data were presented as mean ± SD. Multi-step resistance selection of S. aureus ATCC 43300 (C) and ATCC 29213 (D) to OB and the antibiotic ciprofloxacin. The value of MICn/MIC1 represents the average fold changes of MIC according to the MIC of the first passage.
Antimicrobial Activities of OB Against ESKAPE Pathogens
The MICs of OB against E. faecalis ATCC 29212 and S. epidermidis RP62A were 16 and 8 μg/ml, respectively (data not shown), whereas the MICs for Gram-negative bacteria were higher (ranging from 16 to 128 μg/ml), indicating that OB had greater antibacterial effect on Gram-positive bacteria. To improve the antibacterial potency of OB on Gram-negative bacteria, an outer membrane–disrupting antibacterial peptide PMBN was used to permeabilize the cells. The checkerboard assay was designed to evaluate the effect of OB on Gram-negative bacteria in combination with PMBN (Table 2). All Gram-negative members of the ESKAPE pathogens were insensitive to PMBN (MICs > 128 μg/ml). However, when OB was combined with PMBN (32 μg/ml), the MIC of OB against K. pneumoniae ATCC 700603 was decreased from 128 to 16 μg/ml and the MIC of OB against P. aeruginosa ATCC 15692 was decreased from 64 to 8 μg/ml. When the FICIs were calculated, OB showed significant synergistic effects with PMBN against K. pneumoniae, P. aeruginosa, and E. coli (FICI < 0.375); additive effect was also observed against A. baumannii (0.5 < FICI < 0.75).
Anti-biofilm Activities of OB Against S. aureus
The biofilm-forming capacity of all S. aureus strains are shown in Supplementary Table S2 with strong biofilm producers selected for the following experiments. The eradication effect of OB on mature biofilm was illustrated (Table 1). Oxacillin had no obvious anti-biofilm effect on most of the tested isolates (MBEC50 > 128 μg/ml). Although vancomycin had potent antibacterial activity against MRSA, it did not eradicate biofilm obviously, and there were only three tested isolates with MBEC50 of 16 μg/ml. However, OB was able to eradicate biofilm partially with MBEC50 of 16–64 μg/ml, and the eradication rate for certain MRSA strains reached 80%. The CLSM also showed that as the concentration of OB increased, the green fluorescence signal (viable bacteria stained green) was significantly weakened, and the thickness and density of the biofilm was decreased (Figure 3A), which was consistent with the results of quantitative analysis (Figure 3B). There is a research reported that hydroxypropyltrimethyl ammonium chloride chitosan inhibited the expression of icaA, which mediates the production of extracellular polysaccharides, both in new biofilms and in pre-existing biofilms on titanium (Peng et al., 2011). As a quaternary ammonium derivative, OB may also affect the expression of some biofilm-related genes. The qPCR analysis results showed that compared with the control group, the relative expression of icaA and icaD genes decreased after treatment with 24 μg/ml of OB (P < 0.01) (Figure 3C).
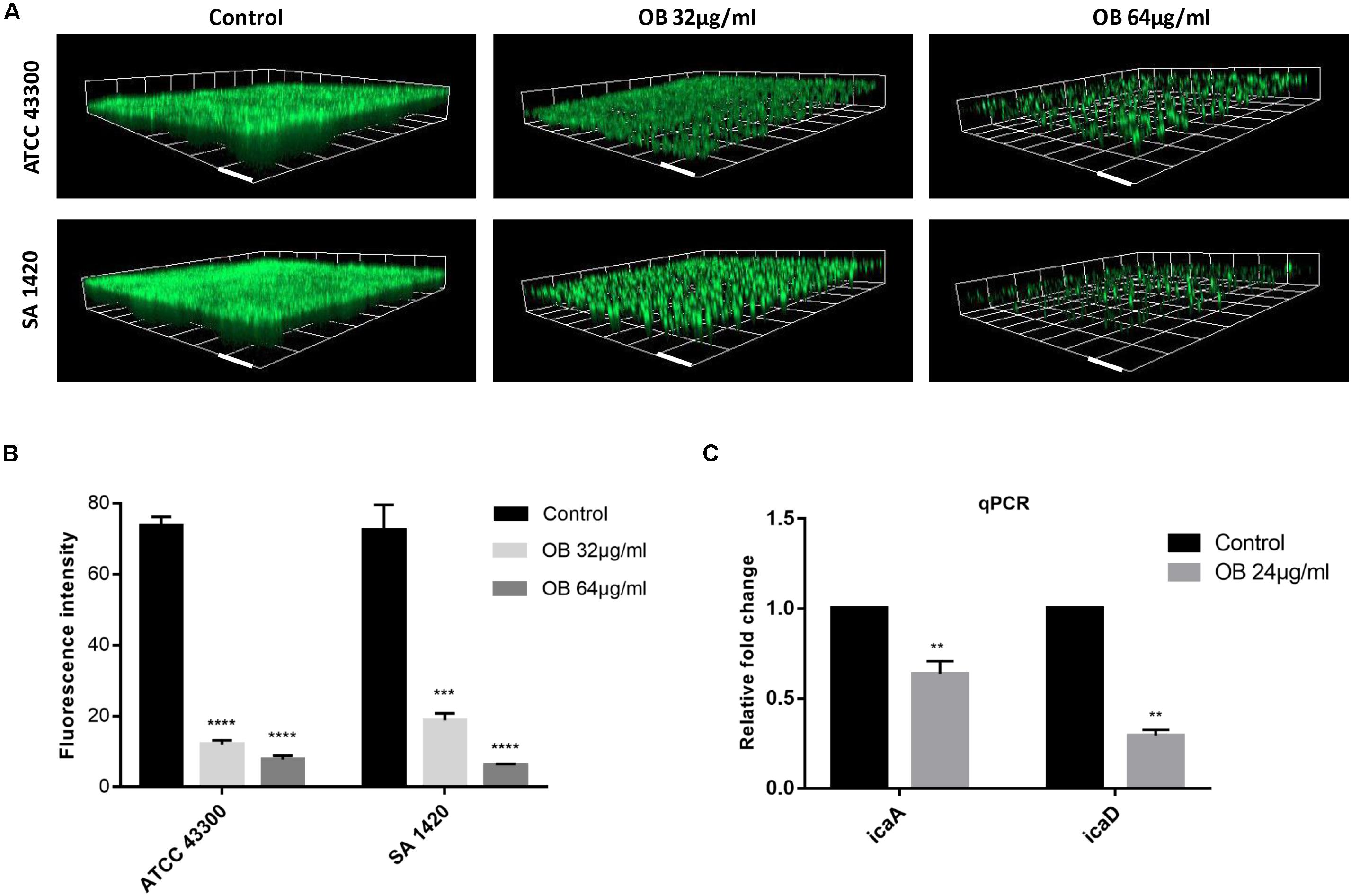
Figure 3. The anti-biofilm activities of OB against S. aureus. (A) Three-dimensional CLSM images of biofilm formed on glass slides using the fluorescence dye SYTO9. The mature biofilm formed by S. aureus ATCC 43300 and SA 1420 (clinical MRSA strain) was treated with DMSO (solvent control), 32 or 64 μg/ml of OB for 24 h. The images were processed with Zen 3.0. Scale bars, 20 μm. (B) Corresponding quantitative histogram of fluorescent intensity detected by CLSM. Compared with control group, ***P < 0.001;****P < 0.0001 (unpaired two-tailed Student’s t-test). (C) Relative expression of icaA and icaD. S. aureus ATCC 43300 was cultured with or without OB (24 μg/ml) for 24 h, and the transcription of icaA and icaD were measured by qPCR. Compared with control group, **P < 0.01(unpaired two-tailed Student’s t-test).
Resistance Selection of OB for S. aureus
The results of multi-step resistance selection are shown in Figures 2C,D. When S. aureus ATCC 43300 and ATCC 29213 were cultured in the presence of sub-inhibitory concentration of ciprofloxacin, the MICs increased 20- and 12-fold after 20 passages, respectively. However, the MICs of OB almost did not change from the first passage to the last passage. For single-step resistance selection (Table 3), the spontaneous mutation frequency to OB was lower than comparator agents like rifampicin and ciprofloxacin. The spontaneous mutation frequency of S. aureus ATCC 43300 and ATCC 29213 treatment with 4× MIC of OB were < 5.92 × 10–10 (± 8.37 × 10–10) and < 3.81 × 10–10 (± 5.39 × 10–10), whereas treatment with 4× MIC of rifampicin were 9.81 × 10–9 (± 4.80 × 10–9) and 6.62 × 10–9 (± 1.69 × 10–9), respectively. In summary, regardless of the long-term or short-term process of in vitro drug-resistance evolution, OB had a greater advantage over rifampicin and ciprofloxacin in reducing the production of resistant colonies.
Effect of OB on Membrane Permeability
To further investigate the antibacterial mechanism of OB against S. aureus, the nucleic acid dye SYTOX Green was used to detect the integrity of the cell membrane. Treatment with varying concentrations of OB on S. aureus ATCC 43300 and ATCC 29213 caused a dose-dependent increase in fluorescence intensity in 5 min, and the fluorescence signal did not decrease in the next 25 min (Figures 4A,B). A similar phenomenon was observed using the membrane potential sensitive dye Disc3(5). Treatment with 4× MIC of OB to S. aureus strains resulted in an increase in fluorescence intensity (400–450 AU) within 300 s (Figures 4C,D). Melittin as a positive control has been confirmed to have strong membrane lytic property, so the enhancement of fluorescence signal could also be detected. Together, these results demonstrated that the antimicrobial mechanism of OB was likely similar to melittin which was able to combine within the cell membrane and cause membrane damage.
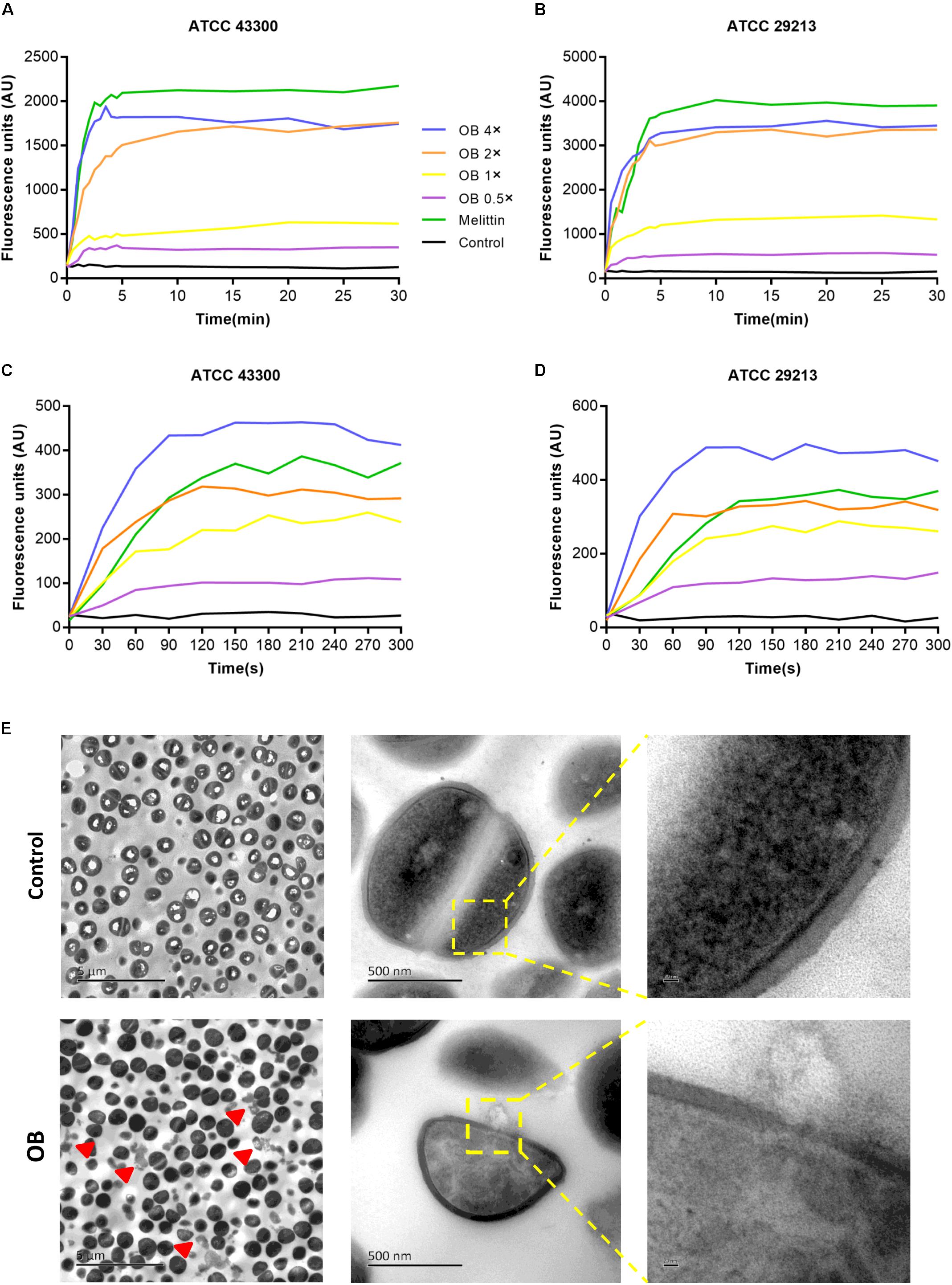
Figure 4. Effect of OB on membrane permeability. S. aureus ATCC43300 and ATCC29213 were incubated with 0.5× MIC, 1× MIC, 2× MIC, 4× MIC of OB, and the effect of OB on the fluorescence intensity of Disc3(5) (A,B) and SYTOX Green (C,D) were detected. 20 μg/ml melittin was used as a positive control and 0.1% DMSO with HEPES was a negative control. (E) The TEM images of S. aureus ATCC43300 exposed to DMSO with PBS (solvent control) or 64 μg/ml of OB. Red arrowheads show areas of cytoplasmic leakage. Scale bars, 5 μm (left), 500 nm (middle), and 20 nm (right).
To visually observe bacterial membrane disruption by OB, transmission electron microscopy (TEM) was employed. S. aureus ATCC 43300 (2 × 109 CFU/ml) was incubated with 64 μg/ml of OB (which was a sub-inhibitory concentration for this high inoculum, no statistical difference in the number of viable bacteria before and after treatment, from 2.57 ± 0.74 × 109 to 1.43 ± 0.17 × 109, P < 0.05, unpaired two-tailed Student’s t-test) for 1 h at 37°C. The TEM showed that the boundary of cell membrane became blurred after treatment and intracellular contents were observed to leak from the cell. In contrast, the control group showed that the cell membrane was unaffected. Therefore, these results further demonstrated that OB targets the membrane (Figure 4E).
Because OB also showed antimicrobial activity against Gram-negative bacteria with MICs of 16–128 μg/ml, we hypothesized that OB probably achieved this effect by acting on the inner membrane of Gram-negative bacteria. To detect the inner membrane integrity, the ONPG experiment was performed with E. coli as an example. E. coli ATCC 29212 incubated with OB caused a dose-dependent and time-dependent increase in optical density value (A405 nm). In treatment with 2× MIC of OB for 1.5 h, the optical density value increased from 1.3 to 1.6. In contrast, no increase of optical density value was detected according to the control group (Supplementary Figure S1).
Hemolytic Activity and Cytotoxicity
The results of hemolysis assay and cytotoxicity test are shown in Supplementary Figure S2. The HC50 of OB for human RBCs was 51.67 μg/ml, whereas the IC50 value of OB for human breast cancer cell lines KPL-4 and human colonic epithelial cell NCM-460 were 36.66 and 42.61 μg/ml, respectively. The MICs and MBCs of OB was lower than the IC50 and HC50 values of OB, suggesting that OB had great antimicrobial activity and low toxicity.
Therapeutic Efficacy of OB in MRSA-Infected Mouse Peritonitis Model
Before in vivo efficacy testing, toxicity of OB was evaluated in mice (Supplementary Figure S3). Intraperitoneal injection of OB with a dose of 40 mg/kg twice was well-tolerated in all mice, with no death observed up to 7 days post-injection, and there was almost no change in body weight compared with the vehicle group, indicating that this dose is relatively safe for treatment of peritonitis. When the OB was used consecutively for 7 days, no death was observed but the weight of mice decreased slightly compared with the vehicle group. For blood cell analysis, there was no statistical difference among the parameters (Supplementary Table S3). For histological analysis, the results showed that OB did not cause significant injury on the tissues, but there were mild morphological changes with hepatocytes in the liver and a little hemorrhagic focus in the kidney as compared with the vehicle group.
The antibacterial activity in vivo was evaluated by MRSA-infected mouse peritonitis model. The mice (n = 5) in vehicle group were intraperitoneally injected with (2–3) × 108 CFU/ml of MRSA (500 μl), which resulted in a 100% mortality rate within 48 h. Therefore, the inoculation amount of MRSA used in this model was a lethal dose that could lead to acute peritonitis. Compared with the vehicle group, treatment with 30 or 40 mg/kg of OB at 1 and 5 h post-infection cured 80% of mice, whereas treatment with 50 mg/kg of vancomycin (positive control) cured 100% of mice (Figure 5A). The weight of all mice decreased slightly on the first day after infection and then increased to the initial value after 7 days (Figure 5B). The mice in the vehicle group died within 48 h, and the number of viable bacteria in liver and kidney was estimated to determine the actual amount of infected bacteria. The bacterial loads of MRSA in liver and kidney were approximately 2.5 × 108 and 5.2 × 107CFU/mouse (data not shown), which is in accordance with the initial injection amount. Moreover, the number of viable bacteria in liver and kidney of the survived mice was also counted. Only a few bacteria could be detected in liver or kidney of the mice treated with 50 mg/kg of vancomycin. There was still a certain amount of bacteria in the liver or kidney of the mice treated with OB, but there was no statistical difference in the number of bacteria in liver of the mice treated with 40 mg/kg of OB and 50 mg/kg of vancomycin. Compared with the group treated with 30 mg/kg of OB, the number of viable bacteria in liver exhibited a 3-log10 reduction in the group treated with 40 mg/kg of OB (P < 0.01) (Figures 5C,D).
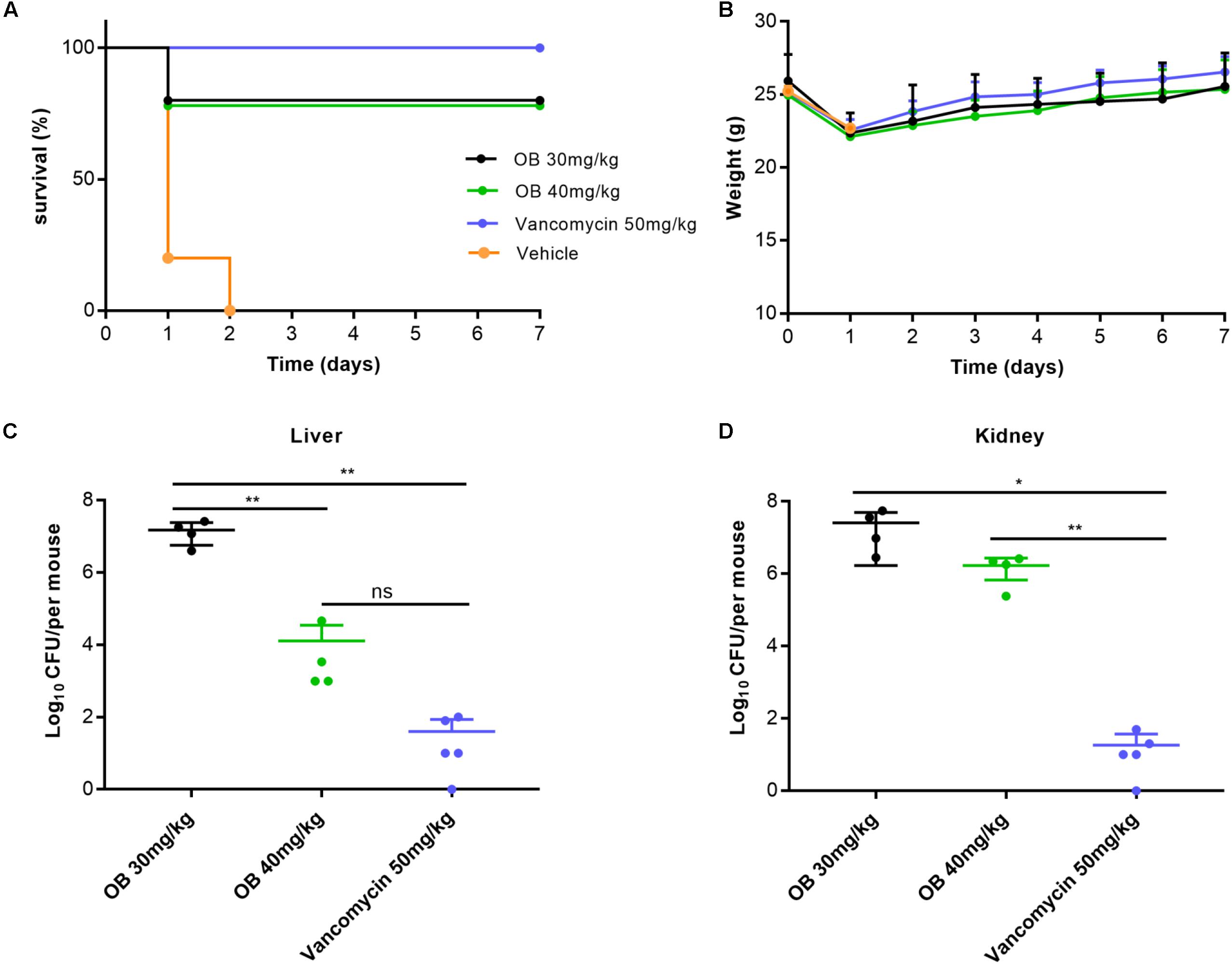
Figure 5. Therapeutic efficacy of OB in vivo. Groups of mice (n = 5) were given 30 mg/kg of OB, 40 mg/kg of OB, 50 mg/kg of vancomycin (positive control), or saline with 2% DMSO (vehicle) at 1 and 5 h post-infection. The survival condition (A) and weight changes (B) of the infected mice were observed for 7 days. The number of viable bacteria in liver (C) and kidney (D) of the survived mice after 7 days were counted. The data were presented as mean ± SD. *P < 0.05; **P < 0.01 (one-way ANOVA, Dunnett’s multiple-comparison test).
Discussion
In this report, we found that compared with Gram-negative bacteria, OB had stronger antibacterial activity against Gram-positive bacteria, which might be a result of the differences in the composition and structure of the cell wall. The cell wall structure of Gram-negative bacteria is more complicated, and its unique component outer membrane has selective permeability to molecules, thus reducing the sensitivity of bacteria to certain drugs. PMBN had no obvious antibacterial activity as well as bactericidal activity, but it increased the permeability of the outer membrane and promoted the entry of OB into the Gram-negative bacteria (Tsubery et al., 2000). Therefore, the application of PMBN improved the antibacterial efficiency of OB and expanded its antibacterial spectrum. As we know, biofilm is the structured communities of bacteria and is more resistant to antibiotic treatment (Coenye and Nelis, 2010). Our results showed that OB had a stronger effect on biofilm eradication than vancomycin, which reflects its superiority as an antibacterial agent in the treatment of biofilm-related infections. In addition, it could also reduce the expression of icaA and icaD genes which mediated the synthesis of polysaccharide intercellular adhesin, the main exopolysaccharide component of staphylococcal biofilm matrix (Arciola et al., 2015).
Quaternary amine compounds (QACs) are widely used in clinical disinfection to prevent the spread of bacteria and act through membrane disruption. The positively charged head group in compounds can be attracted by the negative charge on the bacterial surface to form an electrostatic bond, which increases the pressure on the cell wall and promotes the penetration of long-chain alkyl into the cell membrane, and eventually leads to the leakage of intracellular contents and cell death (Oblak et al., 2019). Because OB has similar chemical features, we speculated that it likely also disrupts the cell membrane. Therefore, we performed the assay using fluorescent dyes and found that OB could depolarize the cell membrane and increase its permeability. Subsequently, we microscopically observed that the integrity of cell membrane was destroyed after treatment with OB. ONPG experiment also showed that OB could penetrate the inner membrane of Gram-negative bacteria. Moreover, membrane-targeting antimicrobials reconfigure the membrane and interfere with the basic function of bacterial membrane, which makes it extremely hard for bacteria to survive and develop drug resistance (Koh et al., 2016; Lin et al., 2017). Our result also showed that OB had rapid bactericidal ability and a low tendency for resistance development, which further confirmed our hypothesis that OB was a membrane-targeting agent.
In addition to its membrane-disrupting activity, several researches propose that QACs may also affect other cellular components and this contributes to their antimicrobial action. Bore et al. (2007) reported that benzalkonium chloride treatment might result in superoxide stress in E. coli K-12. Knauf et al. (2018) suggested that benzalkonium chloride might act directly on the ribosome or influence ribosome association with other important proteostasis components to induce accumulation of protein aggregates in A. baumannii. Because OB has a similar scaffold, we hypothesize it is possible that OB has very specific targets within the bacterial cell to induce cell death, but its binding site and molecular mechanism need to be further investigated.
OB has no obvious side effects and slight toxicity. OB can neither affect the gastric secretion nor produce atropine-like side effects during treatment in patients with IBS (Heading et al., 2006). Acute toxicity experiments revealed that the non-lethal dose for oral administration was 1500 mg/kg for rats and 1000 mg/kg for dogs. In the chronic toxicity experiment, a dose of 80 mg/kg of OB was orally administered to the experimental animals for 180 days, and no change was found in blood chemistry or histologic profiles (Triantafillidis and Malgarinos, 2014). Our data also showed that OB had low hemolysis rate and limited cytotoxicity. The HC50 and IC50 values are significantly higher than the MIC and MBC values, indicating that the recommended antibacterial dose is likely safe. In addition, in in vivo toxicity testing, OB did not cause mice death and significant injury on tissues after treatment with 40 mg/kg of drug by intraperitoneal injection for 7 days. In MRSA-infected mouse peritonitis model, although the weight of survived mice reduced after infection, it could return to the initial level after treatment with OB, which also illustrated that the toxicity of OB was slight.
In the present study, we used mouse peritonitis model to evaluate the therapeutic effect of OB in vivo. This model was chosen because it is easy to master, the end points are clear (death or survival), and the model has been validated with marketed antibiotics (Ford et al., 1996; Bouley et al., 2016). However, OB has poor systemic absorption and primarily remains in the gastrointestinal tract after oral administration. Pharmacokinetic studies revealed that the drug is rapidly eliminated from the circulation within 4 h (Evangelista, 1999). To improve the utilization of the drug and avoid gastrointestinal side reactions, intraperitoneal route of administration was used in this model. There are abundant capillaries on the peritoneum of mice, which can increase the absorption area of the drug and accelerate its circulation (Al Shoyaib et al., 2020). Through animal experiment, we found that OB was effective in vivo and improved the survival rate of mice. However, it is still worth exploring how to optimize pharmacologic properties and reduce toxicity of OB for treatment of S. aureus infections more efficiently. This may include drug combination, new chemical analogs, and nanoscale carriers (Pham et al., 2019).
In summary, OB not only has strong antibacterial ability against S. aureus but also has a potent effect on eradicating mature biofilm. Furthermore, it lacks resistance development and has low cytotoxicity. In vivo, OB is effective for peritonitis infection model caused by MRSA. All these findings emphasize the potential of OB for further clinical development.
Data Availability Statement
All datasets generated for this study are included in the article/Supplementary Material.
Ethics Statement
Clinical samples collection and animal experiments were conducted under the approval of the Ethics Committee of the Third Xiangya Hospital, Central South University (Nos. 2019sydw0233 and 2019-S021). Strains and blood were isolated from clinical samples routinely collected from patients, and the identification of patients was not needed. Therefore, the need for written informed consent was waived and oral informed consent was obtained.
Author Contributions
YW, PS, and LZ designed the experiments. LZ performed most of the experiments, analyzed the results, and wrote the article. LC, ZL, and FT provided essential reagents and methods. SL and XZ performed the supporting experiments. YW conceived and supervised the study. All authors contributed to the article and approved the submitted version.
Funding
This study was supported by the Natural Science Foundation of Hunan Province (2019JJ80029).
Conflict of Interest
The authors declare that the research was conducted in the absence of any commercial or financial relationships that could be construed as a potential conflict of interest.
Acknowledgments
We are grateful to Min Li (Shanghai Jiao Tong University, Shanghai, China), Di Qu (Fudan University, Shanghai, China), and Mingqiang Qiao (Nankai University, Tianjin, China) for providing bacterial strains.
Supplementary Material
The Supplementary Material for this article can be found online at: https://www.frontiersin.org/articles/10.3389/fmicb.2020.01720/full#supplementary-material
FIGURE S1 | Effect of OB on inner membrane permeability. E. coli ATCC 25922 was incubated with 0.5× MIC, 1× MIC, 2× MIC of OB or DMSO (solvent control), and the absorbance at 405 nm were monitored with ONPG for 1.5 h. The data were presented as mean ± SD.
FIGURE S2 | Hemolytic activity and cytotoxicity of OB. (A) 4% (v/v) human RBCs were treated with serially diluted OB at 37°C for 1 h, and the absorbance of the supernatants was measured at 540 nm to calculate the hemolysis (%). 0.1% DMSO with PBS was a negative control, and 0.1% Triton X-100 was a positive control. Human breast cancer cell line KPL-4 (B) and human colon epithelial cell NCM-460 (C) were treated with OB for 24 h, 10 μl of CCK-8 was added and the absorbance at 450 nm was recorded to calculate the cell viability (%). The data were presented as mean ± SD.
FIGURE S3 | In vivo toxicity of OB. (A) Weight changes of mice treated with 40 mg/kg of OB twice (i.p) and observed for 7 days. Weight changes (B) and H&E staining (C) of the mice treated with 40 mg/kg of OB daily for consecutive 7 days (i.p.) and observed for 12 days. Scale bars, 50 μm.
References
Al Shoyaib, A., Archie, S. R., and Karamyan, V. T. (2020). Intraperitoneal route of drug administration: should it be used in experimental animal studies? Pharm. Res. 37:12. doi: 10.1007/s11095-019-2745-x
Arciola, C. R., Campoccia, D., Ravaioli, S., and Montanaro, L. (2015). Polysaccharide intercellular adhesin in biofilm: structural and regulatory aspects. Front. Cell. Infect. Microbiol. 5:7. doi: 10.3389/fcimb.2015.00007
Bore, E., Hebraud, M., Chafsey, I., Chambon, C., Skjaeret, C., Moen, B., et al. (2007). Adapted tolerance to benzalkonium chloride in Escherichia coli K-12 studied by transcriptome and proteome analyses. Microbiology 153(Pt 4), 935–946. doi: 10.1099/mic.0.29288-29280
Bouley, R., Ding, D., Peng, Z., Bastian, M., Lastochkin, E., Song, W., et al. (2016). Structure–activity relationship for the 4(3H)-quinazolinone antibacterials. J. Med. Chem. 59, 5011–5021. doi: 10.1021/acs.jmedchem.6b00372
Coenye, T., and Nelis, H. J. (2010). In vitro and in vivo model systems to study microbial biofilm formation. J. Microbiol. Methods 83, 89–105. doi: 10.1016/j.mimet.2010.08.018
David, M. Z., and Daum, R. S. (2017). Treatment of Staphylococcus aureus Infections. Curr. Top. Microbiol. Immunol. 409, 325–383. doi: 10.1007/82_2017_42
de Breij, A., Riool, M., Cordfunke, R. A., Malanovic, N., de Boer, L., Koning, R. I., et al. (2018). The antimicrobial peptide SAAP-148 combats drug-resistant bacteria and biofilms. Sci. Transl. Med. 10:eaan4044. doi: 10.1126/scitranslmed.aan4044
Evangelista, S. (1999). Otilonium bromide: a selective spasmolytic for the gastrointestinal tract. J. Int. Med. Res. 27, 207–222. doi: 10.1177/030006059902700501
Evangelista, S., Traini, C., and Vannucchi, M. G. (2018). otilonium bromide: a drug with a complex mechanism of action. Curr. Pharm. Des. 24, 1772–1779. doi: 10.2174/1381612824666180507122935
Ford, C. W., Hamel, J. C., Wilson, D. M., Moerman, J. K., Stapert, D., Yancey, R. J., et al. (1996). In vivo activities of U-100592 and U-100766, novel oxazolidinone antimicrobial agents, against experimental bacterial infections. Antimicrob. Agents Chemother. 40, 1508–1513. doi: 10.1128/aac.40.6.1508
Friedman, L., Alder, J. D., and Silverman, J. A. (2006). Genetic changes that correlate with reduced susceptibility to daptomycin in Staphylococcus aureus. Antimicrob. Agents Chemother. 50, 2137–2145. doi: 10.1128/AAC.00039-36
Gerits, E., Defraine, V., Vandamme, K., De Cremer, K., De Brucker, K., Thevissen, K., et al. (2017). Repurposing Toremifene for Treatment of Oral Bacterial Infections. Antimicrob. Agents Chemother. 61:e01846-16. doi: 10.1128/AAC.01846-1816
Gómez-Zorrilla, S., Calatayud, L., Juan, C., Cabot, G., Tubau, F., Oliver, A., et al. (2017). Understanding the acute inflammatory response to Pseudomonas aeruginosa infection: differences between susceptible and multidrug-resistant strains in a mouse peritonitis model. Int. J. Antimicrob. Agents 49, 198–203. doi: 10.1016/j.ijantimicag.2016.10.016
Gould, I. M., David, M. Z., Esposito, S., Garau, J., Lina, G., Mazzei, T., et al. (2012). New insights into meticillin-resistant Staphylococcus aureus (MRSA) pathogenesis, treatment and resistance. Int. J. Antimicrob. Agents 39, 96–104. doi: 10.1016/j.ijantimicag.2011.09.028
Haddad, O., Merghni, A., Elargoubi, A., Rhim, H., Kadri, Y., and Mastouri, M. (2018). Comparative study of virulence factors among methicillin resistant Staphylococcus aureus clinical isolates. BMC Infect. Dis. 18:560. doi: 10.1186/s12879-018-3457-3452
Han, H. M., Gopal, R., and Park, Y. (2016). Design and membrane-disruption mechanism of charge-enriched AMPs exhibiting cell selectivity, high-salt resistance, and anti-biofilm properties. Amino Acids 48, 505–522. doi: 10.1007/s00726-015-2104-2100
Harbut, M. B., Vilchèze, C., Luo, X., Hensler, M. E., Guo, H., Yang, B., et al. (2015). Auranofin exerts broad-spectrum bactericidal activities by targeting thiol-redox homeostasis. Proc. Natl. Acad. Sci. U.S.A. 112, 4453–4458. doi: 10.1073/pnas.1504022112
Hassan, A., Usman, J., Kaleem, F., Omair, M., Khalid, A., and Iqbal, M. (2011). Evaluation of different detection methods of biofilm formation in the clinical isolates. Braz. J. Infect. Dis. 15, 305–311. doi: 10.1590/s1413-86702011000400002
Heading, R., Bardhan, K., Hollerbach, S., Lanas, A., and Fisher, G. (2006). Systematic review: the safety and tolerability of pharmacological agents for treatment of irritable bowel syndrome–a european perspective. Aliment. Pharmacol. Ther. 24, 207–236. doi: 10.1111/j.1365-2036.2006.02937.x
Knauf, G. A., Cunningham, A. L., Kazi, M. I., Riddington, I. M., Crofts, A. A., Cattoir, V., et al. (2018). Exploring the Antimicrobial Action of Quaternary Amines against Acinetobacter baumannii. mBio 9, e2317–e2394. doi: 10.1128/mBio.02394-2317
Koh, J. J., Qiu, S., Zou, H., Lakshminarayanan, R., Li, J., Zhou, X., et al. (2013). Rapid bactericidal action of alpha-mangostin against MRSA as an outcome of membrane targeting. Biochim. Biophys. Acta 1828, 834–844. doi: 10.1016/j.bbamem.2012.09.004
Koh, J. J., Zou, H., Lin, S., Lin, H., Soh, R. T., Lim, F. H., et al. (2016). Nonpeptidic amphiphilic xanthone derivatives: structure-activity relationship and membrane-targeting properties. J. Med. Chem. 59, 171–193. doi: 10.1021/acs.jmedchem.5b01500
Lee, J. K., Park, S. C., Hahm, K. S., and Park, Y. (2013). Antimicrobial HPA3NT3 peptide analogs: placement of aromatic rings and positive charges are key determinants for cell selectivity and mechanism of action. Biochim. Biophys. Acta 1828, 443–454. doi: 10.1016/j.bbamem.2012.09.005
Lin, S., Koh, J., Aung, T. T., Sin, W. L. W., Lim, F., Wang, L., et al. (2017). Semisynthetic flavone-derived antimicrobials with therapeutic potential against methicillin-resistant Staphylococcus aureus (MRSA). J. Med. Chem. 60, 6152–6165. doi: 10.1021/acs.jmedchem.7b00380
Melo, A. S., Bizerra, F. C., Freymuller, E., Arthington-Skaggs, B. A., and Colombo, A. L. (2011). Biofilm production and evaluation of antifungal susceptibility amongst clinical Candida spp. isolates, including strains of the Candida parapsilosis complex. Med. Mycol. 49, 253–262. doi: 10.3109/13693786.2010.530032
Miró-Canturri, A., Ayerbe-Algaba, R., and Smani, Y. (2019). Drug repurposing for the treatment of bacterial and fungal infections. Front. Microbiol. 10:41. doi: 10.3389/fmicb.2019.00041
Miyazaki, H., Midorikawa, N., Fujimoto, S., Miyoshi, N., Yoshida, H., and Matsumoto, T. (2017). Antimicrobial effects of lysophosphatidylcholine on methicillin-resistant Staphylococcus aureus. Ther. Adv. Infect. Dis. 4, 89–94. doi: 10.1177/2049936117714920
Oblak, E., Piecuch, A., Rewak-Soroczynska, J., and Paluch, E. (2019). Activity of gemini quaternary ammonium salts against microorganisms. Appl. Microbiol. Biotechnol. 103, 625–632. doi: 10.1007/s00253-018-9523-9522
Peng, Z., Tu, B., Shen, Y., Du, L., Wang, L., Guo, S., et al. (2011). Quaternized chitosan inhibits icaA transcription and biofilm formation by Staphylococcus on a titanium surface. Antimicrob. Agents Chemother. 55, 860–866. doi: 10.1128/AAC.01005-1010
Pengfei, S., Shijia, L., Linying, Z., Zhen, L., Jinfeng, L., Lanlan, X., et al. (2020). Insights into idarubicin antimicrobial activity against methicillin-resistant Staphylococcus aureus. Virulence 11, 636–651. doi: 10.1080/21505594.2020.1770493
Pham, T. N., Loupias, P., Dassonville-Klimpt, A., and Sonnet, P. (2019). Drug delivery systems designed to overcome antimicrobial resistance. Med. Res. Rev. 39, 2343–2396. doi: 10.1002/med.21588
Roth, B. L., Poot, M., Yue, S. T., and Millard, P. J. (1997). Bacterial viability and antibiotic susceptibility testing with SYTOX green nucleic acid stain. Appl. Environ. Microbiol. 63, 2421–2431.
She, P., Luo, Z., Chen, L., and Wu, Y. (2019). Efficacy of levofloxacin against biofilms of Pseudomonas aeruginosa isolated from patients with respiratory tract infections in vitro. Microbiologyopen 8:e720. doi: 10.1002/mbo3.720
Su, M., Qiu, L., Deng, Y., Ruiz, C. H., Rudolf, J. D., Dong, L., et al. (2019). Evaluation of platensimycin and platensimycin-inspired thioether analogues against methicillin-resistant Staphylococcus aureus in topical and systemic infection mouse models. Mol. Pharm. 16, 3065–3071. doi: 10.1021/acs.molpharmaceut.9b00293
Tan, F., She, P., Zhou, L., Liu, Y., Chen, L., Luo, Z., et al. (2019). Bactericidal and anti-biofilm activity of the retinoid compound CD437 against Enterococcus faecalis. Front. Microbiol. 10:2301. doi: 10.3389/fmicb.2019.02301
Thakare, R., Singh, A. K., Das, S., Vasudevan, N., Jachak, G. R., Reddy, D. S., et al. (2017). Repurposing ivacaftor for treatment of Staphylococcus aureus infections. Int. J. Antimicrob. Agents 50, 389–392. doi: 10.1016/j.ijantimicag.2017.03.020
Triantafillidis, J. K., and Malgarinos, G. (2014). Long-term efficacy and safety of otilonium bromide in the management of irritable bowel syndrome: a literature review. Clin. Exp. Gastroenterol. 7, 75–82. doi: 10.2147/CEG.S46291
Trombetta, R. P., Dunman, P. M., Schwarz, E. M., Kates, S. L., and Awad, H. A. (2018). A high-throughput screening approach to repurpose fda-approved drugs for bactericidal applications against Staphylococcus aureus small-colony variants. mSphere 3:e00422-18. doi: 10.1128/mSphere.00422-418
Keywords: otilonium bromide, Staphylococcus aureus, antibacterial, biofilm, membrane permeability
Citation: Zhou L, She P, Tan F, Li S, Zeng X, Chen L, Luo Z and Wu Y (2020) Repurposing Antispasmodic Agent Otilonium Bromide for Treatment of Staphylococcus aureus Infections. Front. Microbiol. 11:1720. doi: 10.3389/fmicb.2020.01720
Received: 31 March 2020; Accepted: 30 June 2020;
Published: 31 July 2020.
Edited by:
Santi M. Mandal, Indian Institute of Technology Kharagpur, IndiaReviewed by:
Nagendran Tharmalingam, Brown University, United StatesSteven W. Polyak, University of South Australia, Australia
Copyright © 2020 Zhou, She, Tan, Li, Zeng, Chen, Luo and Wu. This is an open-access article distributed under the terms of the Creative Commons Attribution License (CC BY). The use, distribution or reproduction in other forums is permitted, provided the original author(s) and the copyright owner(s) are credited and that the original publication in this journal is cited, in accordance with accepted academic practice. No use, distribution or reproduction is permitted which does not comply with these terms.
*Correspondence: Yong Wu, wuyong_zn@csu.edu.cn