- 1Guangdong Provincial Key Laboratory of Veterinary Pharmaceutics Development and Safety Evaluation, College of Veterinary Medicine, South China Agricultural University, Guangzhou, China
- 2Guangdong Laboratory for Lingnan Modern Agriculture, Guangzhou, China
The aim of this study was to investigate the characteristics of a fosA3 carrying IncC–IncN plasmid from a multidrug-resistant Salmonella isolate HNK130. HNK130 was isolated from a chicken and identified as ST17 Salmonella enterica serovar Indiana and exhibited resistance to 13 antibiotics including the cephalosporins and fosfomycin. S1 nuclease pulsed-field gel electrophoresis and Southern blot assays revealed that HNK130 harbored only one ∼180-kb plasmid carrying fosA3 and blaCTX–M–14, which was not transferable via conjugation. We further examined 107 Escherichia coli electro-transformants and identified 3 different plasmid variants, pT-HNK130-1 (69), pT-HNK130-2 (15), and pT-HNK130-3 (23), in which pT-HNK130-1 seemed to be the same as the plasmid harbored in HNK130. We completely sequenced an example of each of these variants, and all three variants were IncC–IncN multi-incompatible plasmid and showed a mosaic structure. The fosA3 gene was present in all three and bounded by IS26 elements in the same orientation (IS26-322bp-fosA3-1758bp-IS26) that could form a minicircle containing fosA3. The blaCTX–M–14 gene was located within an IS15DI-ΔIS15DI-iroN-IS903B-blaCTX–M–14-ΔISEcp1-IS26 structure separated from the fosA3 gene in pT-HNK130-1, but was adjacent to fosA3 in pT-HNK130-3 in an inverted orientation. Linear comparison of the three variants showed that pT-HNK130-2 and pT-HNK130-3 resulted from the sequence deletion and inversion of pT-HNK130-1. Stability tests demonstrated that pT-HNK130-1 and pT-HNK130-3 could be stably maintained in the transformants without antibiotic selection but pT-HNK130-2 was unstable. This is the first description of an IncC–IncN hybrid plasmid from an ST17 S. Indiana strain and indicates that this plasmid may further facilitate dissemination of fosfomycin and cephalosporin resistance in Salmonella.
Introduction
Fosfomycin is a natural broad-spectrum antibiotic with activity against both Gram-positive and Gram-negative bacteria and has a unique mechanism of action and can synergize with other antibiotics including β-lactams, aminoglycosides, and fluoroquinolones (Raz, 2015; He et al., 2017). The recent rapid increase of multidrug-resistant (MDR) bacteria has limited antibiotic treatment options for infections caused by these pathogens and fosfomycin has become a new choice for the treatment of MDR bacterial infections (Raz, 2015).
Although the use of fosfomycin is prohibited in animals in China, a plasmid-mediated fosfomycin resistance gene fosA3 is often observed in Escherichia coli isolates from pets and food animals (Hou et al., 2012, 2013; He et al., 2013, 2017). Furthermore, fosA3 has been found in association with blaCTX–M, rmtB, floR, blaNDM, and other antibiotic resistance genes (ARGs) on disseminated plasmids, and this has promoted further spread of fosA3 (Hou et al., 2012, 2013; He et al., 2013, 2017; Villa et al., 2015; Hadziabdic et al., 2018). At present, fosA3 has been detected primarily in E. coli isolates from pets, pigs, wild animals, and food animals at rates of 1.1 to 9.0% (Hou et al., 2012, 2013; Ho et al., 2013). However, there are only a few reports that have identified fosA3 in Salmonella isolates (Lin and Chen, 2015; Villa et al., 2015; Wang et al., 2017; Hadziabdic et al., 2018; Fang et al., 2019).
Salmonella is an important global food-borne pathogen of both humans and livestock and 70–80% of annual foodborne diseases in China are attributed to Salmonella infections (Coburn et al., 2007; Wang and Zhang, 2013). Recent reports of Salmonella isolates have found fosA3 in association with blaNDM–1 and blaCMY–16 on an IncA/C plasmid (Villa et al., 2015; Hadziabdic et al., 2018) as well as with mcr-1, oqxAB, and rmtB on HI2 and F33:A-:B- plasmids (Wong et al., 2016; Wang et al., 2017; Fang et al., 2019). However, there is still little information about the prevalence and characteristics of the fosA3 gene among Salmonella isolates. Herein, we report the emergence of an IncC–IncN [IncC, previously termed IncA/C2 (Harmer and Hall, 2016)] plasmid that possesses fosA3, blaCTX–M–14, the heavy metal resistance genes sil and mer as well as other ARGs in Salmonella isolates from chickens in China. This is the first description of an IncC–IncN hybrid plasmid from an ST17 Salmonella Indiana strain, and this hybrid plasmid may have originated from a variety of different plasmids. Our findings also provide important insights into plasmid evolution and the dissemination of fosfomycin resistance in Salmonella.
Materials and Methods
Strains
Salmonella strain HNK130 was isolated from fecal swabs collected from healthy chickens in Henan province of China during 2014 as reported in the previous study (Zhang et al., 2016). In our previous research on the monitoring of drug resistance of Salmonella from food animals (Zhang et al., 2016), this strain was found to be highly resistant to fosfomycin (MIC > 512 μg/ml). The presence of fosA3 and other resistance genes of this strain was screened by PCR amplification using primers described previously (Hou et al., 2012; Li et al., 2015; Lin and Chen, 2015). This strain was then serotyped using slide agglutination with hyperimmune sera (S & A Company, Bangkok, Thailand) and the results were interpreted according to the Kauffman–White scheme as described previously (Zhang et al., 2016). Multilocus sequence typing (MLST) of this strain was performed by PCR and DNA sequence analysis of seven housekeeping genes aroC, dnaN, hemD, hisD, purE, sucA, and thrA to determine the allelic profiles using software available online.1
To detect the prevalence of fosA3-carrying IncC–IncN hybrid plasmid among Salmonella isolates, a total of 288 Salmonella isolates collected and preserved in our laboratory during 2009–2014 were used for screening. Out of the 288 isolates, 126 (44%) isolates were recovered from a total of 1728 pork samples collected from a large-scale slaughterhouse in Guangdong, China, during the period of April 2013 to April 2014. Among the remaining 162 (56%) isolates, except for 3 (1.0%) that were obtained from 180 chicken fecal samples collected from Guangdong, China in October 2009, the other 159 (55%) strains were all isolated from 3850 non-repetitive fecal swabs collected from healthy chickens and pigs in Guangdong, Shandong, Henan, and Hubei provinces of China during 2014. Of these samples collected in 2014, 2090 samples were from chickens and 1760 samples were from pigs; the Salmonella isolates obtained from chickens and pigs were 90 and 69, respectively.
Antimicrobial Susceptibility Testing
The minimum inhibitory concentrations (MICs) of this strain and its transformants for 15 different antibiotics including ampicillin (AMP), cefotaxime (CTX), cefoxitin (CXT), ceftiofur (CTF), ceftriaxone (CTR), nalidixic acid (NAL), ciprofloxacin (CIP), enrofloxacin (ENR), kanamycin (KAN), gentamycin (GEN), amikacin (AMK), tetracycline (TET), chloramphenicol (CHL), florfenicol (FLF), and fosfomycin (FOM) were determined by the agar dilution method according to the CLSI guidelines (CLSI, 2018). E. coli ATCC25922 served as a quality control strain. MIC of AgNO3 was determined by broth microdilution method in an aerobic atmosphere as previously described (Fang et al., 2016); E. coli C600 and E. coli DH5α was used as a reference strain.
Conjugation and Transformation Assays
To test the transferability of the fosA3-positive plasmid, both conjugation experiments and transformation experiments were performed. Conjugation experiments were conducted for strain HNK130 using the sodium azide–resistant E. coli J53 as the recipient by both liquid and solid mating-out assay in Luria-Bertani medium (LB-medium). Transconjugants were selected on MacConkey agar supplemented with 300 mg/L sodium azide and 200 mg/L fosfomycin. For transformation experiments, plasmid DNA from the fosA3-positive strains were extracted using Qiagen Plasmid Midi Kits according to the manufacturer’s instruction (Qiagen, Hilden, Germany). Purified plasmids were transformed into E. coli DH5α (Takara, Dalian, China). Selection of transformants was performed on MacConkey agar containing 200 mg/L fosfomycin, 1 mg/L cefotaxime, and both 200 mg/L fosfomycin and 1 mg/L cefotaxime. The presence of the fosA3 and blaCTX–M–9G genes in the transconjugants and transformants were confirmed by PCR.
Plasmid Characterization
PCR-based replicon typing (PBRT) was performed on transformants using primers as described previously (Carattoli et al., 2005). To determine the location of fosA3 and blaCTX–M–14, pulsed-field gel electrophoresis (PFGE) with S1 nuclease (Takara) digestion of whole genomic DNA was carried out as described previously (Barton et al., 1995). The resulting gels were analyzed by Southern transfer and probing with a DIG-labeled fosA3 gene and blaCTX–M–9G gene fragment according to the manufacturer’s instructions using a DIG High Prime DNA Labeling and Detection Starter Kit I (Roche, Mannheim, Germany).
Plasmid Sequencing and Bioinformatics Analyses
The complete sequence of plasmid was determined by sequencing using long-read MinION (Oxford Nanopore) and short-read Illumina MiSeq technologies. Plasmid DNA was extracted from transformants with the Qiagen Plasmid Midi Kit (Qiagen, Courtaboeuf, France) according to the manufacturer’s instructions and then sent to Biomarker Technologies for sequencing (Biomarker, Beijing, China). The long reads were generated by Oxford Nanopore MinION flowcell R9.4 (Li et al., 2018) with a depth more than 300 times and the 150 bp paired-end short reads were generated by Illumina MiSeq system (Illumina, San Diego, CA, United States) with a depth more than 100 times. The MinION reads were filtered using Filtlong (version 0.2.0) to remove any reads <2000 bp, followed by removal of the lowest 10% of reads by quality. Complete sequence assembly was performed with Canu version 1.5 (Koren et al., 2017) using a combination of short and long reads, followed by error correction by Pilon version 1.12 (Walker et al., 2014). Gene prediction and annotation were done with RAST,2 and IS finder.3 Plasmid replicon types and the plasmid MLST (pMLST) were analyzed using the CGE server.4 Sequences were analyzed and compared using BLAST,5 and map generation was performed using Easyfig (version 2.3) (Sullivan et al., 2011) and BRIG (Alikhan et al., 2011).
Plasmid Stability Testing
The stability of HNK130 and 3 fosA3-positive transformants (T-HNK130-1,-2,-3) was studied by passage in antibiotic-free Luria broth (LB) as previously described (Sandegren et al., 2012). Three separate cultures of each strain carrying the plasmid were grown in 3 ml antibiotic-free LB overnight at 37°C, followed by serial passage of 3 μl overnight culture into 3 ml LB each day, yielding 10 generations for each strain, lasting 20 days. Every 2 days, the cultures were collected and serially diluted using 0.85% saline and grown on LB agar in the absence of antibiotics. Then, 50 colonies were screened on LB agar plates with or without fosfomycin to determine the fraction of plasmid-containing cells, and the plasmid loss was verified by PCR amplification of fosA3 and blaCTX–M–9G.
Accession Number(s)
The plasmid sequences of pT-HNK130-1, pT-HNK130-2, and pT-HNK130-3 have been deposited in GenBank with accession numbers CP045742, CP046032, and CP047128, respectively. We also have submitted the raw reads (both Illumina and Nanorope) of pT-HNK130-1, pT-HNK130-2, and pT-HNK130-3 to the Sequence Read Archive (SRA); the SRA accession was PRJNA631125.
Results
Characterization of fosA3-Bearing Salmonella HNK130
The Salmonella strain HNK130 was found to be ST17 S. enterica serovar Indiana and exhibited an MDR profile for a wide range of antimicrobial agents, including the cephalosporins, aminoglycosides, tetracyclines, and fluoroquinolones (Table 1). The metal susceptibility testing showed that HNK130 and its transformants had the MIC of AgNO3 higher than that for the recipient E. coli C600 and E. coli DH5α (MICAgNO3 = 0.015–0.03 mM vs. 0.008 mM) when compared with E. coli C600 and E. coli DH5α (Table 1). Screening for other resistance genes confirmed that this strain co-harbored blaCTX–M–14 and floR. S1-PFGE and hybridization revealed only one plasmid (∼180 kb) in the strain HNK130 and fosA3 and blaCTX–M–14 were present on this plasmid (Figure 1).
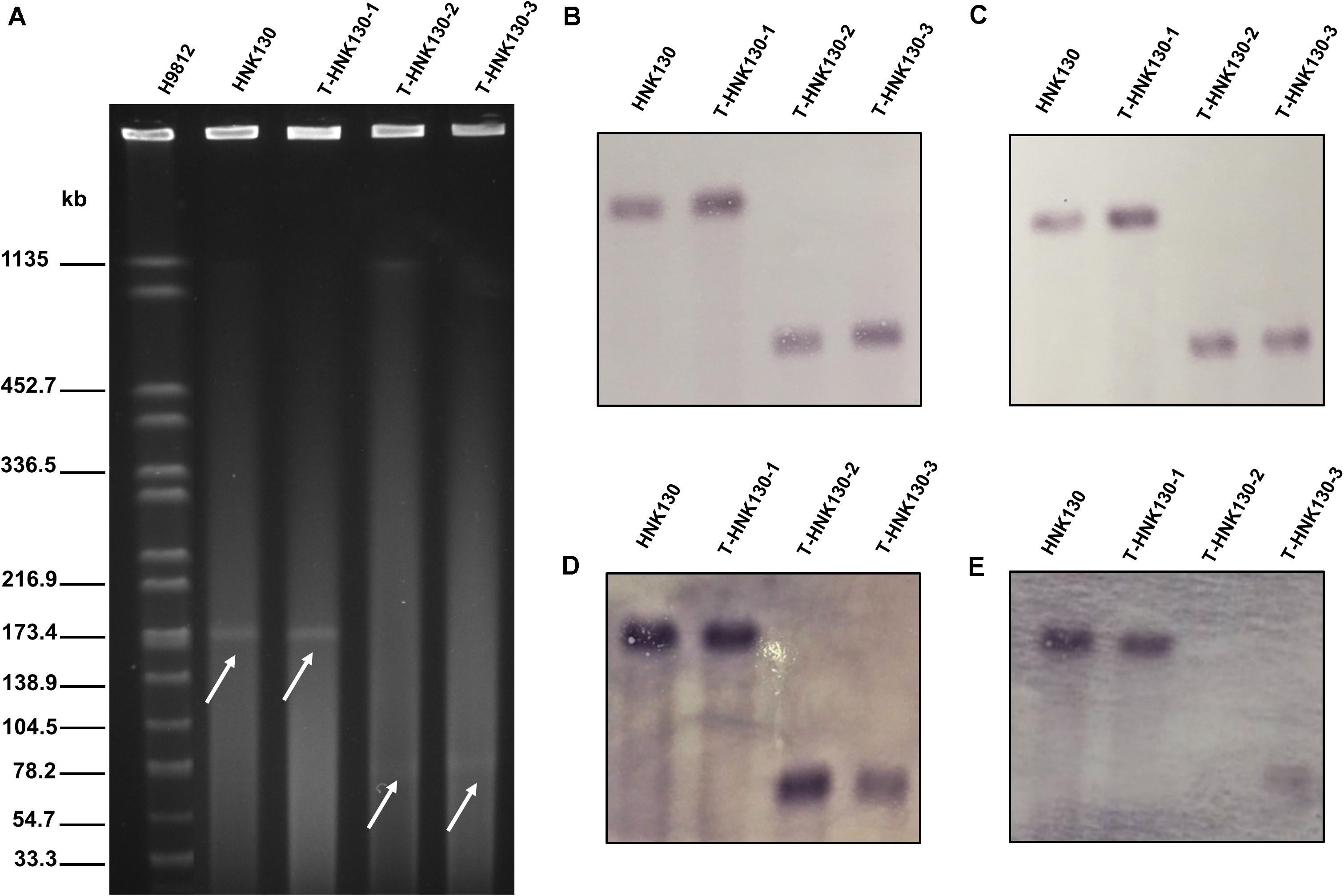
Figure 1. Analysis of HNK130 and its fosA3-positive transformants. (A) S1-PFGE; (B) Southern hybridization with IncC-specific probe; (C) Southern hybridization with IncN-specific probe; (D) Southern hybridization with fosA3-specific probe; (E) Southern hybridization with blaCTX–9G-specific probe.
Although multiple attempts to transfer the fosA3-bearing plasmid from the donor bacteria to E. coli J53 by conjugation were not successful, the fosfomycin resistance trait could be successfully transferred into E. coli DH5α via electro-transformation. We selected three to five clones for blaCTX–M–9G and fosA3 amplification by PCR to verify the transformants. Interestingly, after repeated verifications, we found two different types of transformants: group 1 contained both fosA3 and blaCTX–M–14 while group 2 contained fosA3 and lacked blaCTX–M–14. To further study the presence of two different types of transformants, we randomly selected 107 transformants for PCR detection. Among the 107 transformants, 86% (92/107) of them belong to group 1, and the remaining 14% (15/107) belong to group 2. Plasmid replicon typing revealed that all 107 transformants contained both IncC and IncN replicons.
We then randomly selected nine transformants from groups 1 and 3 from group 2 for S1-PFGE analysis. The nine transformants from group 1 possessed plasmids of two different sizes, i.e., 7 were ∼180 kb and the remaining 2 were ∼80 kb. The group 2 transformants possessed only ∼80 kb plasmids. We examined the genetic features of three of these plasmid variants and designated them as pT-HNK130-1 to pT-HNK130-3 from group 1 fosA3/blaCTX–M–14 positive ∼180 and ∼80 kb plasmids and from group 2 the fosA3 positive ∼80 kb plasmid, respectively. We performed Southern hybridization with DIG-labeled blaCTX–M–9G, fosA3, IncC, and IncN-specific probe in transformants harbored pT-HNK130-1, pT-HNK130-2, and pT-HNK130-3 and showed that the plasmid replicon gene and the antibiotic resistance gene were co-located on the same plasmid in the all three transformants (Figure 1). In addition, based on S1-PFGE and plasmid replicon typing, the plasmid variant pT-HNK130-1 was the same as the plasmid in the wild-type strain.
We also assessed the stability of the three transformants and parent strain HNK130 in the absence of antibiotic selection. The blaCTX–M–14 and fosA3 genes co-transferred plasmids (pT-HNK130-1 and pT-HNK130-3) were stably maintained in the transformants and plasmid loss did not occur. In contrast, the pT-HNK130-2 that contained only the fosA3 gene was unstable and plasmid loss was associated with passage in the absence of antibiotic pressure (Supplementary Figure S1).
Sequence Analysis of pT-HNK130-1, pT-HNK130-2, and pT-HNK130-3
Complete sequences of pT-HNK130-1, pT-HNK130-2, and pT-HNK130-3 were obtained to investigate the genetic features of the three variants and the genetic context of the fosA3 gene and blaCTX–M–14 gene. The largest variant plasmid pT-HNK130-1 was 180,784 bp with a GC content of 51% and carried 255 open reading frames (ORFs). This plasmid belonged to the IncC and IncN incompatibility types. pT-HNK130-1 was 99.88% identical at 53% coverage to plasmid pSE12-01738-2 (CP027679.1), a blaNDM–1-carrying IncC plasmid derived from a Salmonella strain isolated in 2012 from a wild bird in Germany. pT-HNK130-1 also showed high similarity to pRH-1238 (KR091911.1, recovered from a Salmonella isolated from a migratory wild bird in Germany) with 53% coverage and 99.92% identity. pRH-1238 is the first completely sequenced blaNDM–1-fosA3 IncC plasmid and pSE12-01738-2 is a derivative of pRH-1238. Sequence comparisons between pSE12-01738-2 and pT-HNK130-1 showed that in addition to missing and inverted portions of the part of IncC plasmid backbone, there were two regions of (∼70 and ∼20 kb, respectively) of pT-HNK130-1 that did not align to pSE12-01738-2 (Figure 2). The ∼70 kb region of pT-HNK130-1 included backbone elements that encoded functions for horizontal transfer and IncN plasmid replication. BLASTN results for further analysis of this region showed that it was 99.91% identical to the plasmid pCombat13F7-3(CP019248.1) at 77% coverage. pCombat13F7-3 was recovered from a clinical E. coli strain in Hong Kong and belonging to incompatibility groups N and I1. The ∼20 kb region of pT-HNK130-1 contained a Tn7-like transposon (∼5.55 kb) encompassing the tnsABCD genes and silver resistance genes silR, silS, and silE were related to pIncHI2-MU3(MF174859.1) with 86% coverage and 99.64% identity. Sequence analysis indicated that pT-HNK130-1 showed a mosaic structure and may have formed from a variety of plasmids (Figure 2).
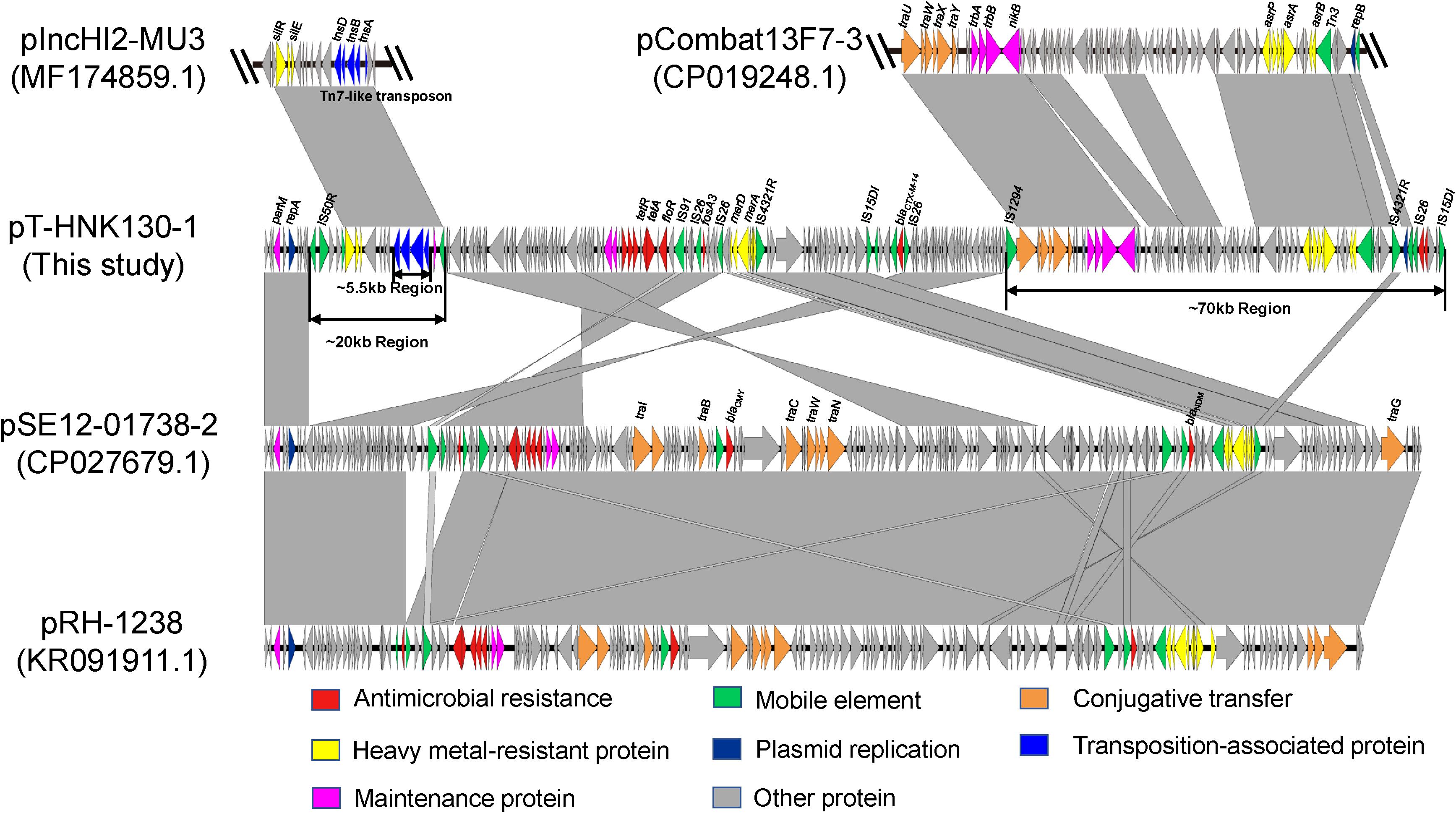
Figure 2. Sequence linear comparison between pT-HNK130-1, pCombat13F7-3 (GenBank accession no. CP019248.1), pIncHI2-MU3 (accession no. MF174859.1), pSE12-01738-2 (accession no. CP027679.1), and pRH-1238 (accession no. KR091911). Gray shading indicates shared regions with a high degree of homology. Genes are represented by arrows and are colored depending on gene function as depicted. Genes are color coded as follows: dark blue, replication; green, mobile element; red, antimicrobial resistance; yellow, heavy metal–resistant protein; blue, transposition-associated protein; orange, conjugative transfer; rose red, maintenance protein; gray, other protein.
The plasmid backbone elements of pT-HNK130-1 encoded functions for plasmid replication, horizontal transfer, maintenance and stability as well as a wide range of mobile genetic elements. In addition, eight predicted ORFs were associated with resistance to aminoglycosides (aph(6)-Id and aph(3″)-Ib), fosfomycin (fosA3), phenicols (floR), β-lactams (blaCTX–M–14), sulfonamides (sul1 and sul2), and tetracycline (tet(A)). These ARGs were grouped together on pT-HNK130-1 with the exception of blaCTX–M–14. This plasmid also contained six ORFs associated with resistance to mercury: merA, merD, merE, merT, merP, and merR (Figure 3).
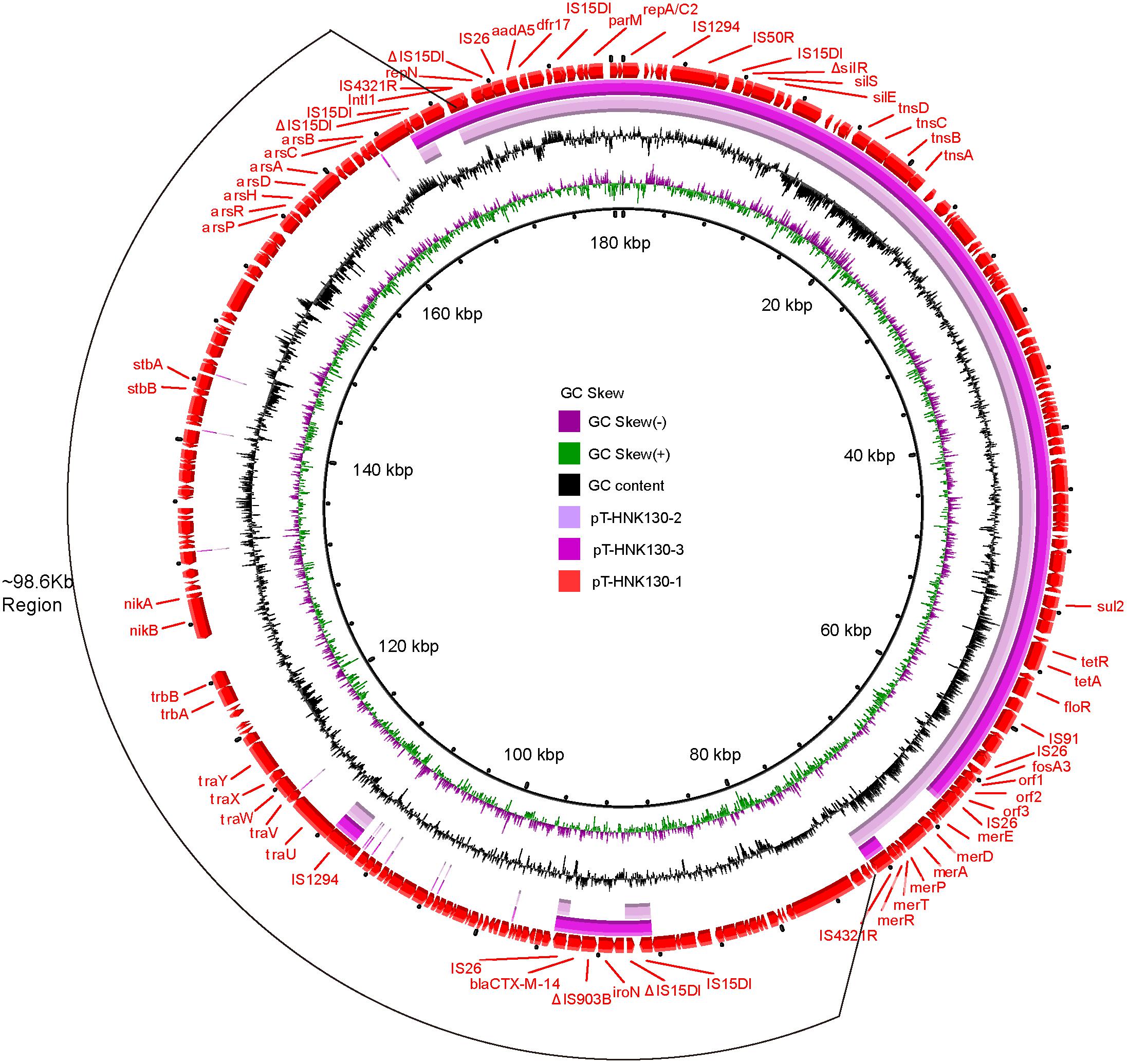
Figure 3. Circular alignment of plasmids pT-HNK130-1, pT-HNK130-2, and pT-HNK130-3. GC content and GC skew are indicated from the inside out. ORFs are indicated by arrows, positions and transcriptional directions of genes depicted in the outer circle belong to pT-HNK130-1, which was included as reference.
Genetic-environment analysis showed that the fosA3 gene was surrounded by two IS26 elements in the context IS26-322 bp-fosA3-1758 bp-IS26. In this structure, the IS26 elements were in the same orientation forming a minicircle containing fosA3, and this was confirmed by inverse PCR. However, the blaCTX–M–14 gene was located on a IS15DI-ΔIS15DI-iroN-IS903B-blaCTX–M–14-ΔISEcp1-IS26 structure outside the multi-resistance region (MRR) where the fosA3 gene was located (Figures 3, 4A).
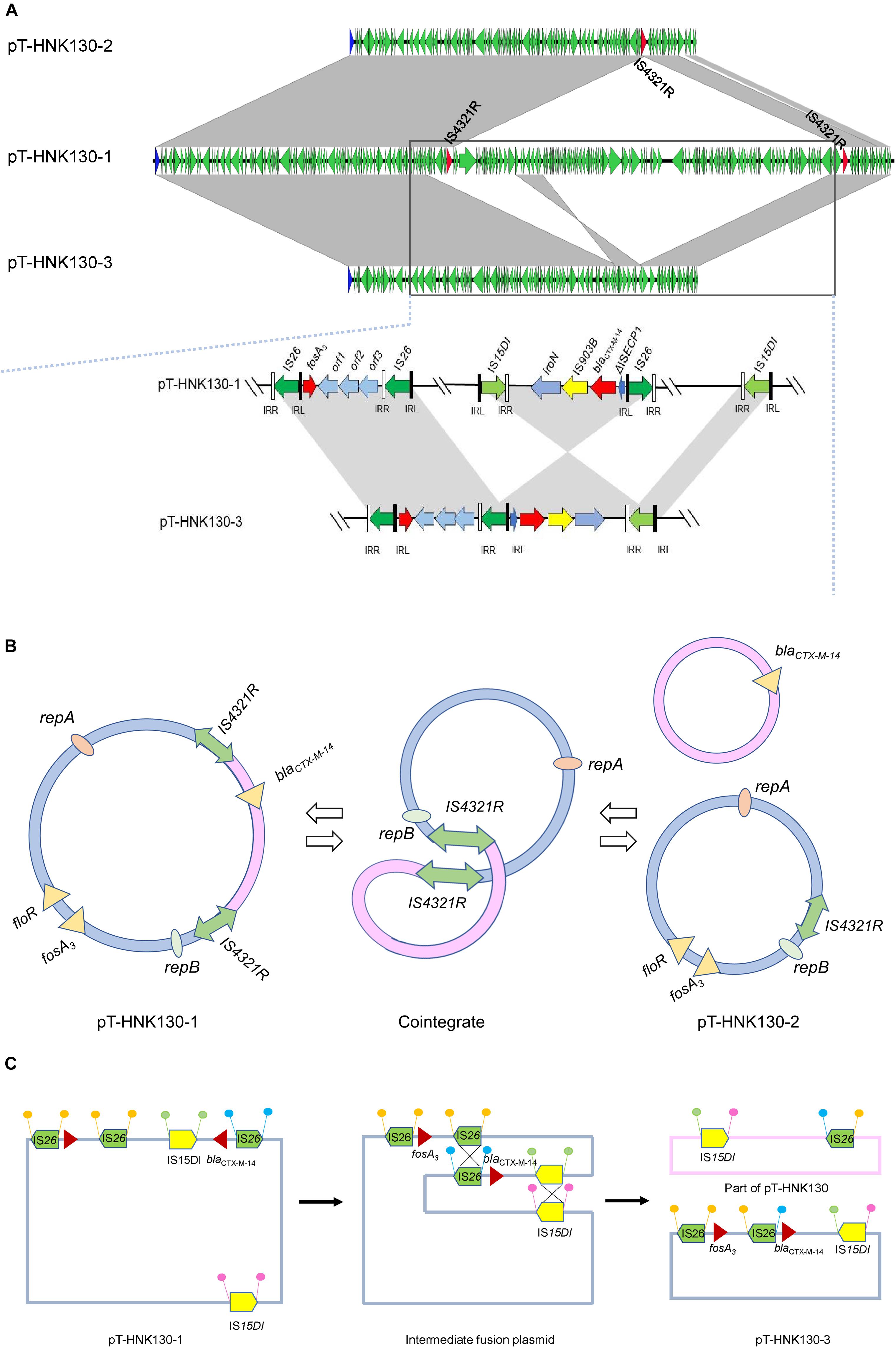
Figure 4. (A) Complete sequence linear comparison of pT-HNK130-1, pT-HNK130-2, and pT-HNK130-3; (B) possible mechanism of pT-HNK130-2 formation; (C) possible mechanism of pT-HNK130-3 formation. Boxed arrows represent the position and transcriptional direction of ORFs. Regions of >99% identity are marked by gray shading.
The second variant plasmid pT-HNK130-2 had a size of 85,132 bp with GC content of 52%, carried 116 ORFs, and belong to the IncC and IncN incompatibility types. Sequence analysis showed that pT-HNK130-2 was identical to pT-HNK130-1, except for a ∼98.6 kb segment between two IS4321Rs of the pT-HNK130-1 backbone that was missing (Figures 3, 4A). The region located between two copies of IS4321R was 99.91% identical to pCombat13F7-3(CP019248.1) at 55% coverage. This region contained the ARG blaCTX–M–14, horizontal transfer associated genes traUVWXY, and many mobile genetic elements and genes associated with plasmid maintenance. These latter were exactly the same as those missing from pT-HNK130-2 compared with plasmid pT-HNK130-1 (Figure 3).
The third variant plasmid pTHNK130-3 was 85,943 bp and composed of 119 ORFs with a GC content of 51% and belonged to the IncC and IncN incompatibility types. A linear comparison with pT-HNK130-1 revealed two segments of ∼23.7 and ∼72.7 kb that were absent from pT-HNK130-3 and blaCTX–M–14 was completely inverted (Figures 3, 4A). This led to the formation of IS26-fosA3-orf1-orf2-orf3-IS26-ΔISEcp1-blaCTX–M–14-IS903B-iroN-ΔIS15DI-IS15DI structure (Figure 4A and Supplementary Figure S3).
Based on the aforementioned sequence analysis, we designed PCR primers to distinguish between these three variants and screened 107 randomly selected transformants. Each transformant possessed only one of the three variants and the percentage we obtained for pT-HNK130-1 to pT-HNK130-3 were 65% (69/107), 14% (15/107), and 21% (23/107), respectively. Interestingly, using these primer sets with the original wild-type strain HNK130 indicated the presence of all three plasmids (Supplementary Table S3 and Supplementary Figure S4).
Prevalence of IncC–IncN Hybrid Plasmid in Salmonella Isolates
These PCR primers were then used to screen 288 Salmonella isolates preserved in our laboratory during 2009–2014 to detect the prevalence of IncC–IncN hybrid plasmid pT-HNK130 among Salmonella isolates. A total of four (1.4%) isolates were detected positive for pT-HNK130. The four isolates were all recovered from chicken fecal swabs collected from 2014, but from a different location (Supplementary Figure S2). They were all resistant to fosfomycin (MIC > 256 μg/ml) and PFGE profiles were identical to that of strain HNK130. In addition, the ST and serotypes of the four strains were also the same as HNK130 and all were ST17 and serovar Indiana (Supplementary Figure S2).
Discussion
Previous studies have reported that fosA3 genes coexisted with blaCTX–M–14 on IncF II, IncN, IncHI2, and untypeable plasmids (Hou et al., 2012; So-Young et al., 2012; Sato et al., 2013; Yang et al., 2014; He et al., 2017). However, in the present study, the fosA3 gene coexisted with blaCTX–M–14 on the fused plasmids that contained both IncC and IncN replicons. The IncC plasmid is a broad-host range plasmid that can be transmitted not only in Enterobacteriaceae but also in other Gram-negative bacteria such as Pseudomonas aeruginosa and Acinetobacter baumannii (Zhang et al., 2014). Previous studies have identified fosA3 co-located with blaNDM–1 and blaCMY–16 on an IncC plasmid in Salmonella isolated from a wild animal in Germany (Villa et al., 2015; Hadziabdic et al., 2018). To the best of knowledge, our research for the first time reported the presence of both fosA3 and blaCTX–M–14 on IncC-IncN hybrid plasmid in Salmonella.
The fosA3-positive IncC–IncN hybrid plasmid-carrying strains in this study were all MDR ST17-type S. Indiana even if they were collected from different provinces, which may indicate that clonal spread of ST17 S. Indiana was responsible for the dissemination of fosA3. Little information about S. Indiana was available until this serotype was reported in food animals and exhibited resistance to numerous ARGs (Yang et al., 2010). After this, the separation rate of S. enterica serovar Indiana has increased rapidly and is becoming more common in patients, retail meats and food-producing animals (Yang et al., 2010; Bai et al., 2016). In addition, the newly identified Indiana strain also showed resistance to colistin and carbapenem, the last line of defense for treating MDR infections, indicating that MDR ST17-type S. Indiana has become a serious problem (Wang et al., 2017).
In the present study, we completely sequenced the three variant plasmids carried by transformants obtained from parent strain HNK130 and pT-HNK130-1 was the same as the parent plasmid. This plasmid was highly similar to conjugative IncC plasmids pSE12-01738-2 and its derivative pRH-1238 that were identified in S. enterica serovar Corvallis isolated from a wild bird in Germany, and carried blaNDM, fosA3, blaCMY, and other ARGs (Villa et al., 2015; Hadziabdic et al., 2018). Compared with them, in addition to the lack of resistance genes blaNDM and blaCMY, pT-HNK130-1 also lacked some plasmid backbone genes necessary for horizontal transfer including traI, traB, traC, traN, and traG and may explain why pT-HNK130 was not conjugative.
The first variant plasmid pT-HNK130-1 harbored two MRRs, one of which consisted of seven ARGs (sul2, aph(3″)-Ib, aph(6)-Id, tetR, tetA, floR, and fosA3) and six mercury resistance gens (merA, merD, merE, merT, merP, and merR). The other one possessed a single ARG blaCTX–M–14 in the IS15DI-ΔIS15DI-iroN-IS903B-blaCTX–M–14-ΔISEcp1-IS26 structure, a structure similar to the genetic environments of blaCTX–M–14 in the ST131 E. coli isolates from hospitals in the Kyoto and Shiga regions of Japan, in which three CTX-M-14-H30 (non-Rx) isolates had an IS26-ΔISEcp1-blaCTX–M–14-ΔIS903D-IS26-like structure (Matsumura et al., 2015). The blaCTX–M genes are often captured by ISEcp1 forming the transposition units ISEcp1-blaCTX–M-IS903D and ISEcp1-blaCTX–M-orf477 that can be horizontally transferred and disseminated between different host bacteria (D’Andrea et al., 2013). Here, we present the first report of an IS15DI-ΔIS15DI-iroN-IS903B-blaCTX–M–14-ΔISEcp1-IS26 structure and our sequence analysis suggest that the blaCTX–M–14 may originated from other sources via this structure, and the lack of direct repeats may have been caused by multiple transposition processes (Partridge, 2011).
The genetic environment of fosA3 in the largest variant pT-HNK130-1 was IS26-322bp-fosA3-1758 bp-IS26, which was 100% identical to fosA3 genetic structure reported in Salmonella isolates from a wild bird in Germany and E. coli isolates from healthy individuals in Japan and E. coli isolates from chickens in China (So-Young et al., 2012; Ho et al., 2013; Hadziabdic et al., 2018). However, another genetic environment of fosA3 and blaCTX–M–14 was obtained from pT-HNK130-3; IS26-322 bp-fosA3-1758 bp-IS26-ΔISEcp1-blaCTX–M–14-IS903B-iroN-ΔIS15DI-IS15DI. The IS26-associated context of fosA3 has been reported in different plasmids from multiple Enterobacteriaceae and has frequently been associated with several blaCTX–M variants (So-Young et al., 2012; Sato et al., 2013; Yang et al., 2014; Lin and Chen, 2015; He et al., 2017). The genetic environment of both fosA3 and blaCTX–M–14 genes carried by the IncC–IncN plasmid in the current study was very similar to the structure reported from E. coli chicken isolates in China and human clinical isolates in Korea (Hou et al., 2012; So-Young et al., 2012; Ho et al., 2013). In addition, the finding of a circular intermediate carrying fosA3 in this study suggests that horizontal transfer of the IS26 composite transposon might accelerate ARG dissemination. Furthermore, our results also demonstrated that the blaCTX–M–14 and fosA3 co-transferred plasmids were stably maintained even in the absence of antibiotic. A previous study has reported that the association with blaCTX–M likely favored the dissemination and maintenance of fosA3 (Hou et al., 2012). Fosfomycin is used for bacterial infections including urinary tract infections caused by extended spectrum β-lactamase–producing Enterobacteriaceae, thus the persistence of the IS26 transposon-like structure co-carrying blaCTX–M–14 and fosA3 from MDR ST17-type S. Indiana strains may pose a new public health threat.
pT-HNK130-1 also contained a Tn7-like transposon downstream of silver resistance genes silS, silE, and silR. Tn7-like transposons play important roles in cross-genus transfer of the sil and pco operons among Enterobacteriaceae (Fang et al., 2016). There is increasing concern that metal contamination functions as a selective agent in the proliferation of antibiotic resistance (Fang et al., 2016). The coexistence of metal resistance determinants and fosA3, and blaCTX–M–14 may facilitate fosA3 and blaCTX–M–14 dissemination under metal selective pressures in the absence of antibiotics.
The other two sequenced variant plasmids were altered in size compared with the parental plasmid, and linear comparisons of the three variants indicated they were identical except for partial sequence deletions in pT-HNK130-2 and pT-HNK130-3. Detailed analysis based on the three plasmids enabled us to predict the possible mechanism of pT-HNK130-2 and pT-HNK130-3 formation during electro-transformation. pT-HNK130-1 and pT-HNK130-2 shared a common IS element, IS4321R. IS4321R was present in both pT-HNK130-1 and pT-HNK130-2 and could be used as a homologous recombination target to form a cointegrate whereby pT-HNK130-2 is excised (Figures 4A,B). However, the formation of pT-HNK130-3 could be a result of the fusion of pTHNK130-1 via two recombination events (double crossover) (Figures 4A,C). The DNA fragments between two directly oriented copies of IS15DI and IS26 result in the exchange of these segments (Partridge, 2011). Our results revealed that homologous recombination between two copies of IS4321R, IS26, and IS15DI, contributes to changes in plasmid size. Plasmid recombination through IS elements is not uncommon in Gram-negative bacteria and is associated with ARG spread including the New Delhi MBL (NDM) gene (Xie et al., 2018). In addition, plasmid DNA sequences are dynamic and a single plasmid may actually be a cluster of plasmids containing heterogeneous MRRs and is a reflection of its heterogeneity. This would benefit plasmid and host survival to antimicrobial threatening conditions (He et al., 2019). In support of this, we detected all three plasmids in the parent strain using PCR. However, only one plasmid was detected in the original strain by S1-PFGE and further dissection of plasmid identities awaits further investigation.
In conclusion, this study for the first time identified and characterized a hybrid IncC–IncN plasmid carrying fosA3, blaCTX–M–14 as well as heavy metal resistance genes (sil and mer) in MDR ST17-type S. Indiana Salmonella isolates from chickens in China. The presence of ARGs and metal resistance genes in a single plasmid can further enhance stability in the absence of antibiotic selective pressure in the presence of metal contamination. Fosfomycin is an alternative treatment for multidrug-resistant Enterobacteriaceae infections in the clinic, so it cannot be excluded that this isolate may spread to humans via direct contact or the food chain. The emergence of this plasmid from S. Indiana isolates will seriously limit future therapeutic options and the further spread of this plasmid among Salmonella would be a serious public health concern.
Data Availability Statement
The datasets generated for this study can be found in the NCBI, pT-HNK130-1 (CP045742), pT-HNK130-2 (CP046032), pTHNK130-3 (CP047128), and PRJNA631125.
Ethics Statement
This study protocol was approved by the South China Agriculture University Animal ethics committee. The strains of food animal origin were isolated from fecal swabs of healthy chickens and the owners of the animals gave permission for their animals to be used in this study.
Author Contributions
L-JZ wrote the manuscript, performed the experiments, and analyzed the data. X-XG, JZ, and Y-WL performed the experiments. LY and L-XF contributed to data analysis and manuscript modification. H-XJ designed the study and supervised the project. All authors contributed to the article and approved the submitted version.
Funding
This work was funded by the National Natural Science Foundation of China (31772792) and National Key Research and Development Program of China (2016YFD0501300).
Conflict of Interest
The authors declare that the research was conducted in the absence of any commercial or financial relationships that could be construed as a potential conflict of interest.
Supplementary Material
The Supplementary Material for this article can be found online at: https://www.frontiersin.org/articles/10.3389/fmicb.2020.01582/full#supplementary-material
Footnotes
- ^ http://mlst.warwick.ac.uk/mlst/dbs/Senterica
- ^ http://rast.nmpdr.org
- ^ https://www-is.biotoul.fr
- ^ https://cge.cbs.dtu.dk/services/
- ^ http://blast.ncbi.nlm.nih.gov
References
Alikhan, N. F., Petty, N. K., Ben Zakour, N. L., and Beatson, S. A. (2011). BLAST Ring Image Generator (BRIG): simple prokaryote genome comparisons. BMC Genomics 12:402. doi: 10.1186/1471-2164-12-402
Bai, L., Zhao, J., Gan, X., Wang, J., Zhang, X., Cui, S., et al. (2016). Emergence and diversity of Salmonella enterica serovar Indiana isolates with concurrent resistance to ciprofloxacin and cefotaxime from food-producing animals and patients in China. Antimicrob. Agents Chemother. 60, 3365–3371. doi: 10.1128/aac.02849-15
Barton, B. M., Harding, G. P., and Zuccarelli, A. J. (1995). A general method for detecting and sizing large plasmids. Anal. Biochem. 226:235. doi: 10.1006/abio.1995.1220
Carattoli, A., Bertini, A., Villa, L., Falbo, V., Hopkins, K. L., and Threlfall, E. J. (2005). Identification of plasmids by PCR-based replicon typing. J. Microbiol. Methods 63:219. doi: 10.1016/j.mimet.2005.03.018
CLSI, (2018). Performance Standards for Antimicrobial Susceptibility Testing, 28th Edn. Wayne, PA: CLSI.
Coburn, B., Grassl, G. A., and Finlay, B. B. (2007). Salmonella, the host and disease: a brief review. Immunol. Cell Biol. 85, 112–118. doi: 10.1038/sj.icb.7100007
D’Andrea, M. M., Arena, F., Pallecchi, L., and Rossolini, G. M. (2013). CTX-M-type β-lactamases: a successful story of antibiotic resistance. Int. J. Med. Microbiol. Ijmm 303, 305–317. doi: 10.1016/j.ijmm.2013.02.008
Fang, L., Li, X., Li, L., Li, S., Liao, X., Sun, J., et al. (2016). Co-spread of metal and antibiotic resistance within ST3-IncHI2 plasmids from E. coli isolates of food-producing animals. Sci. Rep. 6:25312.
Fang, L.-X., Jiang, Q., Deng, G.-H., He, B., Sun, R.-Y., Zhang, J.-F., et al. (2019). Diverse and flexible transmission of fosA3 associated with heterogeneous MDR regions in Salmonella enterica serovars Typhimurium and Indiana isolates. Antimicrob. Agents Chemother. 64:e02001-19.
Hadziabdic, S., Borowiak, M., Bloch, A., Malorny, B., Szabo, I., Guerra, B., et al. (2018). Complete genome sequence of an avian native NDM-1-producing Salmonella enterica subsp. enterica Serovar corvallis strain. Genome Announc 6:e00593-18.
Harmer, C. J., and Hall, R. M. (2016). PCR-based typing of IncC plasmids. Plasmid 87-88, 37–42. doi: 10.1016/j.plasmid.2016.08.002
He, D., Liu, L., Guo, B., Wu, S., Chen, X., Wang, J., et al. (2017). Chromosomal location of the fosA3 and blaCTX-M genes in Proteus mirabilis and clonal spread of Escherichia coli ST117 carrying fosA3-positive IncHI2/ST3 or F2:A-:B- plasmids in a chicken farm. Int. J. Antimicrob. Agents 49, 443–448. doi: 10.1016/j.ijantimicag.2016.12.009
He, D. D., Zhao, S. Y., Wu, H., Hu, G. Z., Zhao, J. F., Zong, Z. Y., et al. (2019). Antimicrobial resistance-encoding plasmid clusters with heterogeneous MDR regions driven by IS26 in a single Escherichia coli isolate. J. Antimicrob. Chemother. 74, 1511–1516. doi: 10.1093/jac/dkz044
He, L., Partridge, S. R., Yang, X., Hou, J., Deng, Y., Yao, Q., et al. (2013). Complete nucleotide sequence of pHN7A8, an F33:A-:B-type epidemic plasmid carrying blaCTX-M-65, fosA3 and rmtB from China. J. Antimicrob. Chemother. 68, 46–50. doi: 10.1093/jac/dks369
Ho, P. L., Chan, J., Lo, W. U., Law, P. Y., Li, Z., Lai, E. L., et al. (2013). Dissemination of plasmid-mediated fosfomycin resistance fosA3 among multidrug-resistant Escherichia coli from livestock and other animals. J. Appl. Microbiol. 114, 695–702. doi: 10.1111/jam.12099
Hou, J., Huang, X., Deng, Y., He, L., Yang, T., Zeng, Z., et al. (2012). Dissemination of the fosfomycin resistance gene fosA3 with CTX-M beta-lactamase genes and rmtB carried on IncFII plasmids among Escherichia coli isolates from pets in China. Antimicrob. Agents Chemother. 56, 2135–2138. doi: 10.1128/aac.05104-11
Hou, J., Yang, X., Zeng, Z., Lv, L., Yang, T., Lin, D., et al. (2013). Detection of the plasmid-encoded fosfomycin resistance gene fosA3 in Escherichia coli of food-animal origin. J. Antimicrob. Chemother. 68, 766–770. doi: 10.1093/jac/dks465
Koren, S., Walenz, B. P., Berlin, K., Miller, J. R., Bergman, N. H., and Phillippy, A. M. (2017). Canu: scalable and accurate long-read assembly via adaptive k-mer weighting and repeat separation. Genome Res. 27, 722–736. doi: 10.1101/gr.215087.116
Li, B., Zhang, Y., Wei, J., Shao, D., Liu, K., Shi, Y., et al. (2015). Characterization of a novel small plasmid carrying the florfenicol resistance gene floR in Haemophilus parasuis. J. Antimicrob. Chemother. 70, 3159–3161. doi: 10.1093/jac/dkv230
Li, R., Xie, M., Ning, D., Lin, D., Yang, X., Yin, W. M. H., et al. (2018). Efficient generation of complete sequences of MDR-encoding plasmids by rapid assembly of MinION barcoding sequencing data. Gigascience 3, 1–9.
Lin, D., and Chen, S. (2015). First detection of conjugative plasmid-borne fosfomycin resistance gene fosA3 in Salmonella isolates of food origin. Antimicrob. Agents Chemother. 59, 1381–1383. doi: 10.1128/aac.04750-14
Matsumura, Y., Johnson, J. R., Yamamoto, M., Nagao, M., Tanaka, M., Takakura, S., et al. (2015). CTX-M-27- and CTX-M-14-producing, ciprofloxacin-resistant Escherichia coli of the H30 subclonal group within ST131 drive a Japanese regional ESBL epidemic. J. Antimicrob. Chemother. 70, 1639–1649.
Partridge, S. R. (2011). Analysis of antibiotic resistance regions in Gram-negative bacteria. FEMS Microbiol. Rev. 35, 820–855. doi: 10.1111/j.1574-6976.2011.00277.x
Raz, R. (2015). Fosfomycin: an old-new antibiotic. Clin. Microbiol. Infect. Off. Publ. Eur. Soc. Clin. Microbiol. Infect. Dis. 18, 4–7. doi: 10.1111/j.1469-0691.2011.03636.x
Sandegren, L., Linkevicius, M., Lytsy, B., Melhus, A., and Andersson, D. I. (2012). Transfer of an Escherichia coli ST131 multiresistance cassette has created a Klebsiella pneumoniae-specific plasmid associated with a major nosocomial outbreak. J. Antimicrob. Chemother. 67, 74–83. doi: 10.1093/jac/dkr405
Sato, N., Kawamura, K., Nakane, K., Wachino, J., and Arakawa, Y. (2013). First detection of fosfomycin resistance gene fosA3 in CTX-M-producing Escherichia coli isolates from healthy individuals in Japan. Microb. Drug Resist. 19, 477–482. doi: 10.1089/mdr.2013.0061
So-Young, L., Yeon-Joon, P., Kyung, Y. J., Seungwon, J., Yoonjoo, K., Seok Hoon, J., et al. (2012). Prevalence of acquired fosfomycin resistance among extended-spectrum β-lactamase-producing Escherichia coli and Klebsiella pneumoniae clinical isolates in Korea and IS26-composite transposon surrounding fosA3. J. Antimicrob. Chemother. 67, 2843–2847. doi: 10.1093/jac/dks319
Sullivan, M. J., Petty, N. K., and Beatson, S. A. (2011). Easyfig: a genome comparison visualizer. Bioinformatics 27, 1009–1010. doi: 10.1093/bioinformatics/btr039
Villa, L., Guerra, B., Schmoger, S., Fischer, J., Helmuth, R., Zong, Z., et al. (2015). IncA/C Plasmid Carrying blaNDM-1, blaCMY-16, and fosA3 in a Salmonella enterica serovar corvallis strain isolated from a migratory wild bird in Germany. Antimicrob. Agents Chemother. 59, 6597–6600. doi: 10.1128/aac.00944-15
Walker, B. J., Abeel, T., Shea, T., Priest, M., Abouelliel, A., Sakthikumar, S., et al. (2014). Pilon: an integrated tool for comprehensive microbial variant detection and genome assembly improvement. PLoS One 9:e112963. doi: 10.1371/journal.pone.0112963
Wang, J., Li, X., Li, J., Hurley, D., Bai, X., Yu, Z., et al. (2017). Complete genetic analysis of a Salmonella enterica serovar Indiana isolate accompanying four plasmids carrying mcr-1, ESBL and other resistance genes in China. Vet. Microbiol. 210, 142–146. doi: 10.1016/j.vetmic.2017.08.024
Wang, S., and Zhang, D. W. (2013). Analysis of bacterial foodborne disease outbreaks in China between 1994 and 2005. FEMS Immunol. Med. Microbiol. 51, 8–13. doi: 10.1111/j.1574-695x.2007.00305.x
Wong, M. H., Chan, E. W., Xie, L., Li, R., and Chen, S. (2016). IncHI2 plasmids are the key vectors responsible for oqxAB transmission among Salmonella species. Antimicrob. Agents Chemother. 60, 6911–6915. doi: 10.1128/aac.01555-16
Xie, M., Li, R., Liu, Z., Chan, E. W. C., and Chen, S. (2018). Recombination of plasmids in a carbapenem-resistant NDM-5-producing clinical Escherichia coli isolate. J. Antimicrob. Chemother. 73, 1230–1234. doi: 10.1093/jac/dkx540
Yang, B., Qu, D., Zhang, X., Shen, J., Cui, S., Shi, Y., et al. (2010). Prevalence and characterization of Salmonella serovars in retail meats of marketplace in Shaanxi, China. Int. J. Food Microbiol. 141, 63–72. doi: 10.1016/j.ijfoodmicro.2010.04.015
Yang, X., Liu, W., Liu, Y., Wang, J., Lv, L., Chen, X., et al. (2014). F33: A-: B-, IncHI2/ST3, and IncI1/ST71 plasmids drive the dissemination of fosA3 and blaCTX-M-55/-14/-65 in Escherichia coli from chickens in China. Front. Microbiol. 5:688. doi: 10.3389/fmicb.2014.00688
Zhang, G. Q., Yao, Y. H., Yu, X. L., and Niu, J. J. (2014). A survey of five broad-host-range plasmids in gram-negative bacilli isolated from patients. Plasmid 74, 9–14. doi: 10.1016/j.plasmid.2014.05.002
Keywords: Salmonella, IncC–IncN plasmid, fosA3, blaCTX–M–14, multidrug-resistant
Citation: Zhang L-J, Gu X-X, Zhang J, Yang L, Lu Y-W, Fang L-X and Jiang H-X (2020) Characterization of a fosA3 Carrying IncC–IncN Plasmid From a Multidrug-Resistant ST17 Salmonella Indiana Isolate. Front. Microbiol. 11:1582. doi: 10.3389/fmicb.2020.01582
Received: 12 February 2020; Accepted: 17 June 2020;
Published: 21 July 2020.
Edited by:
Eva M. Top, University of Idaho, United StatesReviewed by:
Timothy J. Johnson, University of Minnesota Twin Cities, United StatesXiang-Dang Du, Henan Agricultural University, China
Steven Lee Foley, National Center for Toxicological Research (FDA), United States
Copyright © 2020 Zhang, Gu, Zhang, Yang, Lu, Fang and Jiang. This is an open-access article distributed under the terms of the Creative Commons Attribution License (CC BY). The use, distribution or reproduction in other forums is permitted, provided the original author(s) and the copyright owner(s) are credited and that the original publication in this journal is cited, in accordance with accepted academic practice. No use, distribution or reproduction is permitted which does not comply with these terms.
*Correspondence: Hong-Xia Jiang, hxjiang@scau.edu.cn
†These authors have contributed equally to this work
‡Present address: Xi-Xi Gu, Henan University of Animal Husbandry and Economy, Zhengzhou, China