- 1Department of Food Science and Technology, Institute of Food Safety and Nutrition, Jinan University, Guangzhou, China
- 2State Key Laboratory of Applied Microbiology Southern China, Guangdong Provincial Key Laboratory of Microbial Safety and Health, Guangdong Open Laboratory of Applied Microbiology, Guangdong Institute of Microbiology, Guangzhou, China
- 3College of Food Science, South China Agricultural University, Guangzhou, China
Bacillus cereus is one of the most important foodborne pathogenic microorganisms, which can lead to gastrointestinal and non-gastrointestinal diseases. However, the potential risk of B. cereus in aquatic products in China has not been comprehensively evaluated yet. In this study, a total of 860 aquatic samples from three types of retail aquatic products were collected from 39 major cities in China from 2011 to 2016. The contamination, distribution of virulence genes, antibiotic resistance and genetic diversity of B. cereus isolates were measured and analyzed. Of all the samples, 219 (25.47%) were positive for B. cereus and 1.83% (4/219) of the samples had contamination levels of more than 1,100 most probable number (MPN)/g. Different isolates had virulence potential, within which 59.6% (164/275) contained all three kinds of enterotoxin genes (nhe, hbl, and cytK-2) and 5.1% (14/275) possessed cereulide encoding gene cesB. The antimicrobial resistance profiles revealed the universal antibiotic resistance to rifampin and most β-lactams, suggesting the necessity to continuously monitor the antibiotic resistance of B. cereus in aquatic products and to control drug use in aquaculture. In sum, our study indicates the potential hazards of B. cereus isolated from aquatic products to customers and may provide a reference for clinical treatment caused by B. cereus.
Introduction
As one of the key sources of nutrition, aquatic products are an indispensable part of consumers’ diets (Thilsted et al., 2014). But, the problem of bacterial contamination in aquatic products always exists (Kim et al., 2017). Consuming raw aquatic products like sashimi is now very popular around the world, making foodborne diseases caused by bacterial contamination in aquatic products more likely (Miguéis et al., 2015). Bacillus cereus, a facultative aerobic and spore-forming Gram-positive bacterium, is a well-known foodborne opportunistic pathogen (Bottone, 2010; Messelhäußer and Ehling-Schulz, 2018). Since B. cereus and its dormant spores are widely present in nature (Andersson et al., 1995; Bottone, 2010), it can easily contaminate different types of food. When B. cereus exceeds 105 CFU/g, it is considered to be unacceptable/potentially hazardous (Gilbert et al., 2000). The contamination incidents of B. cereus in aquatic products have been reported previously (Rahmati and Labbé, 2008; Iwamoto et al., 2010; Miguéis et al., 2016).
As B. cereus in aquatic products may cause food poisoning, it is necessary to investigate the prevalence and potential hazards of different isolates. Food poisoning symptoms induced by B. cereus include diarrhea and vomiting (Stenfors Arnesen et al., 2008), which are mainly caused by non-hemolytic enterotoxin (Nhe), hemolysin BL (Hbl), cytotoxin K (CytK) (Stenfors Arnesen et al., 2008; Messelhäußer and Ehling-Schulz, 2018), and a cyclic dodecadepsipeptide named cereulide (Agata et al., 1995; Ehling-Schulz et al., 2015). The symptoms of gastrointestinal infections caused by B. cereus are generally acute and mild. However, B. cereus can also lead to some severe non-gastrointestinal infections, such as endophthalmitis, bacteremia, septicemia, meningitis, and pneumonia (Hoffmaster et al., 2006; Bottone, 2010; Rishi et al., 2013; Veysseyre et al., 2015). Currently, the most common treatment for the infections and severe food poisoning by B. cereus is antibiotic therapy. If the strain is resistant to the antibiotics used clinically, it will cause the failure of the treatment. Thereby, the resistance profiles of B. cereus isolates to different antibiotics could be used as a reference for the clinical curing.
As the risk of B. cereus in aquatic products in China has not been comprehensively evaluated yet, we aimed to analyze the prevalence of B. cereus in this study, as well as the molecular characteristics (virulence genes, antibiotic resistance profiles, and genetic diversity) of different isolates to explore the potential hazard of B. cereus in aquatic products in major cities of China.
Materials and Methods
Sampling
From 2011 to 2016, 860 aquatic products were collected from one supermarket and two traditional retail markets in 39 major cities (Supplementary Figure S1) according to the Chinese general guidelines of food microbiological examination (The Hygiene Ministry of China, 2010). After collection, aquatic samples were placed in sterile plastic bags, immediately transported back to the laboratory at low temperature (below 4°C) and subjected to further test and analysis. Microbial experiments were operated in class II biosafety cabinets in a BSL2 laboratory.
Isolation and Identification of B. cereus
Qualitative and quantitative detection of B. cereus were performed according to the food microbiological examination guidelines of B. cereus (The Hygiene Ministry of China, 2003) and previous studies (Gao et al., 2018; Yu et al., 2019, 2020) with minor modification. Briefly, 25 grams of aquatic sample were homogenized at 8,000 to 10,000 rpm about 2 min in a sterile bag (Huankai, Guangzhou, China) with 225 mL 0.01 mol/L phosphate-buffered saline (PBS). The homogenates were incubated for 48 ± 2 h at 30 ± 2°C. Then the cultures were streaked onto mannitol-egg yolk-polymyxin (MYP) agar plates and incubated for 24 h at 30°C. Single colonies were then streaked onto chromogenicB. cereus agar plates (Huankai, Guangzhou, China). Typical colonies were further confirmed with biochemical testing (Gao et al., 2018; Yu et al., 2019, 2020). B. cereus ATCC 14579 was used as a reference strain for biochemical characterization. The most probable number (MPN) method was used for the quantitative detection of B. cereus. The detailed procedures were performed as previously described (Yu et al., 2020) and the MPN table is listed in Supplementary Table S1 (The Hygiene Ministry of China, 2014).
ERIC-PCR was used to characterize the clonal isolates of B. cereus identified from the same sample (Gao et al., 2018; Yu et al., 2020). The primer set (named ERIC-F and ERIC-R; Versalovic et al., 1991; Gao et al., 2018) is listed in Supplementary Table S2. If two or more isolates from the same sample had the exact fingerprint, only one of the strains was kept for further test and the others were excluded as clonal isolates.
Detection of Virulence Genes
Seven enterotoxigenic genes (hblA, hblC, hblD, nheA, nheB, nheC, and cytK-2) and one emetic toxin-producing gene (cesB) were detected by PCR using the primers listed in Supplementary Table S2 (Hansen and Hendriksen, 2001; Ehling-Schulz et al., 2005; Oltuszak-Walczak and Walczak, 2013). Genomic DNA was extracted using a HiPure Bacterial DNA extraction kit (Magen, Guangzhou, China) under the instruction of the manufacturer. Concentration and purity of the DNA were measured by Nanodrop 2000 spectrophotometer (Thermo Fisher Scientific, United States). The amplification reactions were performed using ExTaq Mix kit (Takara, China) in a Biometra TOne 96G thermal cycler (Analytik Jena, Jena, Germany). The PCR reaction mixture (25 μL) contained 50 ng of genomic DNA, 1.0 μM of each primer and 12.5 μL ExTaq Mix. Amplification was performed according to the instruction of the manufacturer (ExTaq Mix, Takara, China).
Testing of Antibiotics Susceptibility
Antimicrobial susceptibility of different strains was evaluated by the Kirby-Bauer (KB) disk diffusion method, which was performed and interpreted as described by the Clinical and Laboratory Standards Institute (The Clinical and Laboratory Standards Institute [CLSI], 2010) and previous publications (Gao et al., 2018; Yu et al., 2019). Twenty antibiotics (Oxoid, United Kingdom; Supplementary Table S3) were selected based on the performance standards for antimicrobial susceptibility testing of the CLSI for Staphylococcus aureus (The Clinical and Laboratory Standards Institute [CLSI], 2010). The diameter of the inhibition zone (Supplementary Table S3) was measured to evaluate the antibiotic resistance of different isolates.
Discrimination of Psychrotrophic and Mesophilic Strains
Rapid discrimination of psychrotrophic and mesophilic B. cereus was done by the detection of the 16S rDNA signatures (von Stetten et al., 1998; Stenfors and Granum, 2001). The primers were listed in Supplementary Table S2 and the PCR program was conducted as described previously (von Stetten et al., 1998) with modifications. The amplification was performed using DreamTaq Green PCR Master Mix (2X) (Thermo Fisher Scientific, United States) following the instruction of the manufacturer.
Growth test of 16 strains, including 14 cesB-positive isolates from aquatic products, B. cereus ATCC14579 and a clinical emetic-type strain B. cereus F4810/72, was conducted according to the previous publication (Luu-Thi et al., 2014) with minor modifications. A single colony of each isolate on BHI agar was inoculated into a 5 mL of BHI broth and then incubated at 30°C until the OD600 reaching 0.6–0.8 (logarithmic growth phase). Afterward, 100 μL of bacterial culture were sprayed on BHI agar plates and incubated at either 7or 43°C within 20 days. Three biological replicates of each strain were performed. If visible colonies could form on the plate incubated at 7°C, the strain was considered to be psychrotrophic isolate.
Multilocus Sequence Typing (MLST) and Phylogenetic Analysis
Seven housekeeping genes were amplified with corresponding primers and conditions as described by the protocol available in PubMLST1. In general, the PCR amplification system (25 μL), referring to the instruction manual of PrimeSTAR Max Premix (Takara, China), contained 12.5 μL PrimeSTAR Max Premix, 50 ng genomic DNA, 1.0 μM of each primer. The sequence type (ST) of each isolate was obtained as described previously (Yu et al., 2019, 2020). A minimal spanning tree was created using PHYLOViZ 2.0 software (Instituto de Microbiologia, Portugal; Nascimento et al., 2017) to visualize the possible evolutionary relationships between different isolates for epidemiological analysis based on MLST alleles. Phylogenetic analysis between the sequence types (STs) of 275 isolates and eight type strains (B. cereus ATCC 14579, Bacillus mycoides DSM 2048, Bacillus pseudomycoides DSM 12442, Bacillus weihenstephanensis WSBC 10204, Bacillus anthracis ATCC 4728, Bacillus thuringiensis ATCC 10792, and two clinical emetic-type strains B. cereus NC7401 and B. cereus F4810/72) was conducted using the BioNumerics software (version 7.6; Applied Maths, Belgium) by the unweighted pair group method of arithmetic averages (UPGMA) method with a 52% similarity level.
Results
Prevalence Analysis of B. cereus in Aquatic Products
The prevalence and contamination level of B. cereus in 860 samples are shown in Table 1. The aquatic products we collected can be divided into three categories: (i) finfish; (ii) mollusks; and (iii) crustaceans (Iwamoto et al., 2010). Through the ERIC-PCR method, 13 strains from 288 were excluded as they present clonal strains (Supplementary Figure S2). Overall, 275 B. cereus isolates were detected in 25.47% (219/860) of the samples, with 25.62% in finfish, 23.32% in mollusks, and 27.78% in crustaceans. In terms of collecting sites, the contamination rate in 15 cities was ≥ 30.00% and even reached 50.00% in Shaoguan (Supplementary Table S4). About the contamination level, 79.45% (174/219) of the samples ranged from 3 to 1,100 MPN/g. In 1.83% (4/219; all from finfish) of total samples, the contamination level exceeded 1,100 MPN/g, indicating that B. cereus in these samples may have a higher risk to cause disease.
Distribution of Virulence Genes and Psychrotolerant Ability of Emetic Strains
The distribution of virulence genes is shown in Figure 1. More than 99.6% of the isolates harbored nhe genes and 61.8% of the isolates possessed hbl ones. Besides, cytK-2 was present in 93.1% of the isolates. In contrast, only 14 isolates (5.1%) possessed cereulide synthetase gene cesB. Eight strains, named 875, 2039-1, 2078, 2931-1A, 3626-1B, 3729-2C, 3831-1A and 3927-1C, harbored all eight virulence genes (nheA-nheB-nheC-hblA-hblC-hblD-cytK-2-cesB; Supplementary Figure S3). About 60.0% (164/275) of the strains were found to contain genes encoding all three types of enterotoxins (nhe, hbl and cytK-2), which suggests that B. cereus strains isolated in this study have a higher potential to cause diarrheal disease.
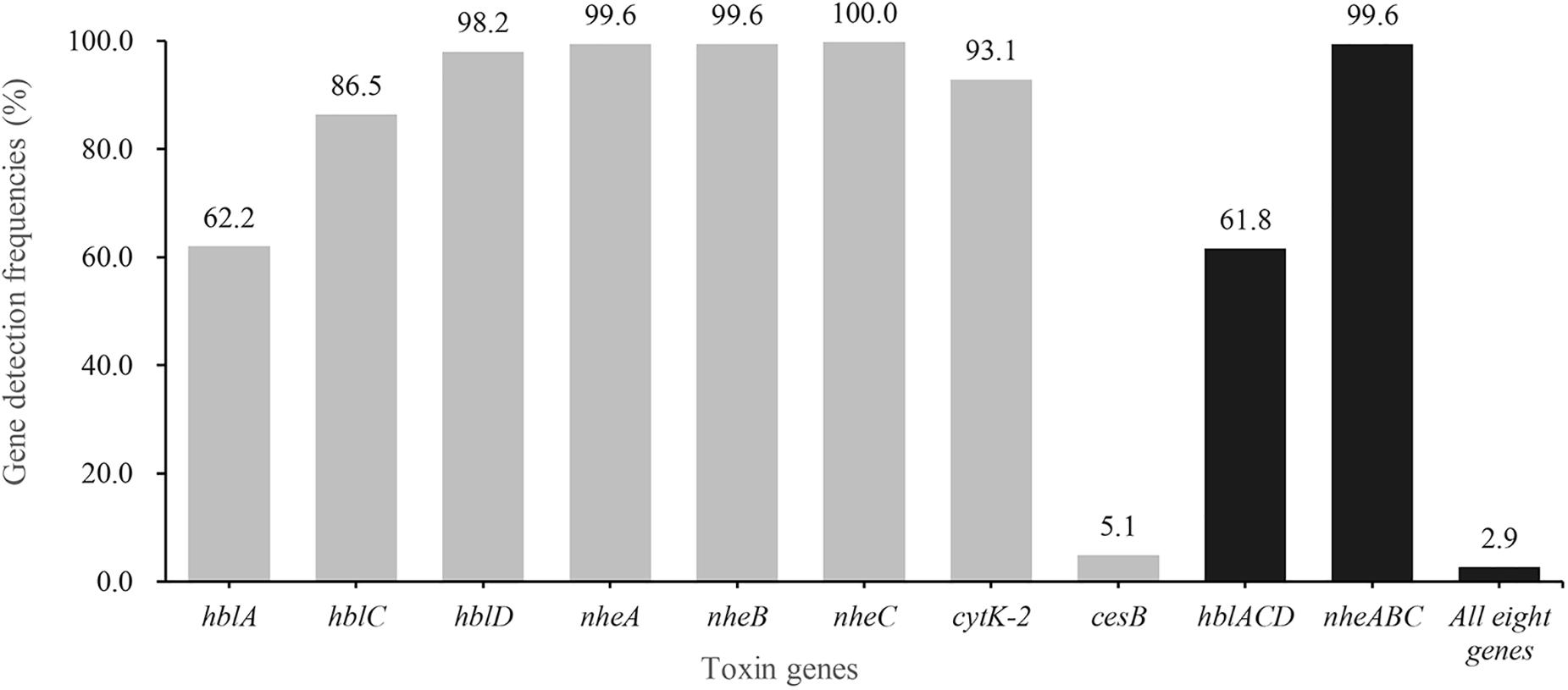
Figure 1. Detection rate of virulence genes in B. cereus from aquatic products. The gray bars of different heights represent the positive rate of hblA, hblC, hblD, nheA, nheB, nheC, cytK-2 and cesB in B. cereus isolates, respectively. The dark bars indicate the positive rate of hbl gene cluster (hblA, hblC, and hblD), or nhe gene group (nheA, nheB, and nheC), or all eight virulence genes.
The psychrotolerant ability of all cesB-positive strains was tested by amplification of specific signatures within 16S rDNA or by growth test at different temperatures. Although all strains showed specific bands representing mesophilic and psychrotrophic signatures within 16S rDNA (Table 2 and Supplementary Figure S4), all cesB-positive strains could grow at 43°C instead of 7°C, revealing their non-psychrotolerant identity (Guinebretière et al., 2008).
Antimicrobial Susceptibility of B. cereus Isolates
According to the results of antimicrobial susceptibility test (Figure 2), nearly all isolates showed resistance to rifampin (RD; 97.5%) and most β-lactams [ampicillin (AMP; 99.3%), penicillin (P; 99.6%), amoxicillin-clavulanic acid (AMC; 98.5%), cephalothin (KF; 83.6%), and cefoxitin (FOX; 97.1%)], whilst they gave less resistance to other β-lactams, such as cefotetan (CTT) and imipenem (IPM) (27.6% and 2.5%, respectively). IPM (96.7%), gentamicin (CN; 97.1%), teicoplanin (TEC; 79.3%) and ciprofloxacin (CIP; 72.0%) could effectively inhibit the growth of different strains.
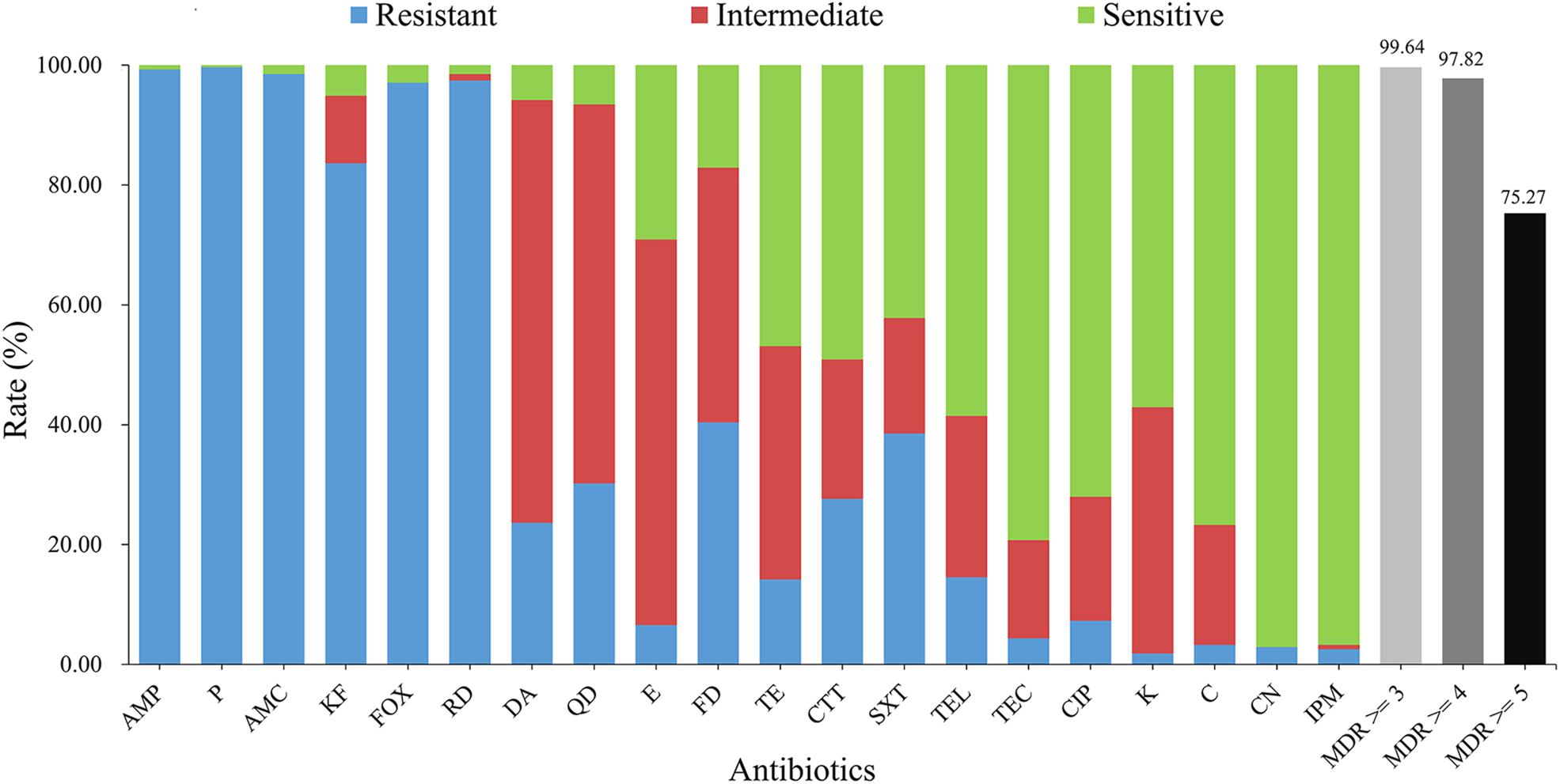
Figure 2. Antimicrobial characteristics of B. cereus from aquatic products. The blue, red, and green bars represent the proportion of resistant, intermediate resistant, and sensitive strains, respectively. The light gray, gray, and black bars show the proportion of strains with multidrug resistance (MDR) to at least three, four, and five classes of antibiotics, respectively. AMP, ampicillin (10 μg); P, penicillin (10 units); AMC, amoxicillin-clavulanic acid (20 μg/10 μg); KF, cephalothin (30 μg); FOX, cefoxitin (30 μg); RD, rifampin (5 μg); DA, clindamycin (2 μg); QD, quinupristin-dalfopristin (15 μg); E, erythromycin (15 μg); FD, nitrofurantoin (300 μg); TE, tetracycline (30 μg); CTT, cefotetan (30 μg); SXT, trimethoprim-sulfamethoxazole (1.25 μg/23.75 μg); TEL, telithromycin (15 μg); TEC, teicoplanin (30 μg); CIP, ciprofloxacin (5 μg); K, kanamycin (30 μg); C, chloramphenicol (30 μg); CN, gentamicin (10 μg); IPM, imipenem (10 μg).
Regarding 133 antimicrobial resistance profiles (Supplementary Figure S3), 58 isolates (21.1%) were resistant to ≥10 antibiotics. The strain 1581-3C, 1705-1C and 1977-3 turned out to be the most highly resistant isolates, which were resistant to 13 antibiotics (Supplementary Figure S3). In contrary, the strain 3004-3A was only resistant to two antibiotics (FOX-RD). Further antimicrobial resistance profile analysis revealed that the most common one was AMP-P-AMC-KF-FOX-RD. Based on the definition of multi-drug resistance (MDR; Magiorakos et al., 2012), a very high proportion (99.64%) of isolates were MDR and 75.27% of the population were resistant to five types of antibiotics.
Analysis of Genetic Diversity
The minimal spanning tree was generated based on the sequences of seven housekeeping genes to estimate the relationships between different strains (Figure 3). Overall, 275 isolates were assigned with 147 STs, which contained 45 new STs (including 52 isolates) (Supplementary Table S5). ST770, the most predominant ST, included 28 isolates, followed by ST4 and ST205 with 18 isolates each. Furthermore, the fourth dominant ST was ST26, which is frequently associated with clinical strains (Carroll et al., 2019). ST26 could be detected in all three types of aquatic products [Finfish (n = 5), mollusks (n = 2), and crustaceans (n = 3)]. Based on the cluster analysis, all isolates were grouped into 162 singletons and eight different clonal complexes, which included ST-142 complex (n = 48, 17.45%), ST-205 complex (n = 32, 11.64%), ST-18 complex (n = 20, 7.27%), ST-111 complex (n = 6, 2.18%), ST-365 complex (n = 3, 1.09%), ST-97 complex (n = 2, 0.73%), ST-8 complex (n = 1, 0.36%), and ST-23 complex (n = 1, 0.36%), indicating the overall high diversity of B. cereus from aquatic products.
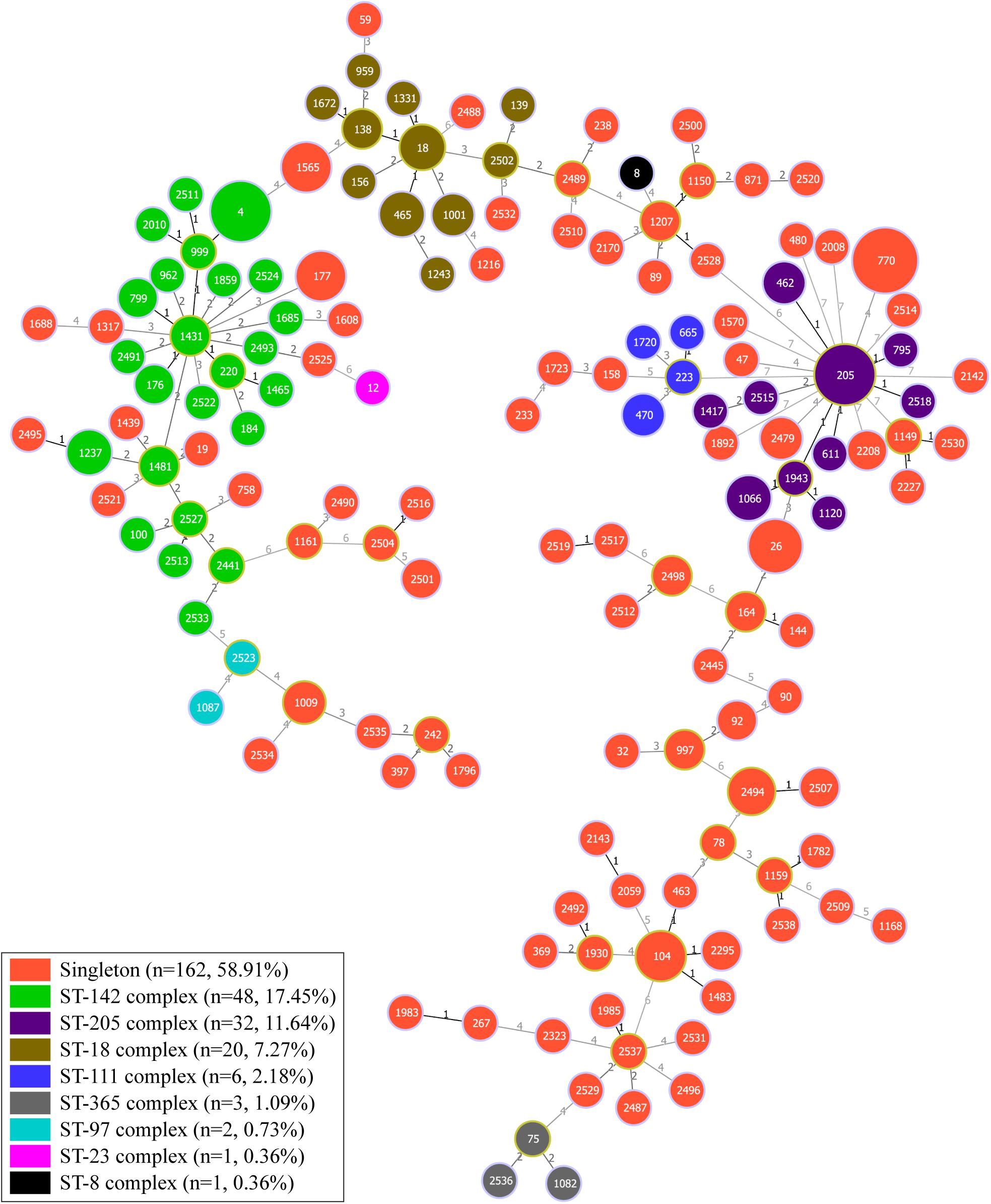
Figure 3. Minimum spanning tree and genetic diversity of 275 B. cereus isolates from aquatic products. Each color represents one clonal complex or a group of singletons. The numbers inside the pie chart are the corresponding sequence types (STs), and the size of the pie chart is proportional to the number of isolates in the corresponding ST. The number along the line indicates the variation of the seven loci between two strains at both ends of the line.
Moreover, all isolates were divided into 13 clusters based on the MLST alleles with the threshold value of 52% similarity (Supplementary Figure S3). Cluster six contained most of the strains (74 strains) and cluster two only contained the strain Y273. Cluster seven had the highest proportion of strains displaying less antibiotic resistance and fewer virulence genes. In cluster seven, eight strains (26.7%) were resistant to less than six classes of antibiotics and 16 strains (53.3%) harbored fewer than six virulence genes. Emetic strains were not evenly distributed into different clusters, with only one strain each in clusters three, four, nine, and ten, two strains in cluster five and 10 strains in cluster six (eight cesB-positive isolates from aquatic products and two clinical emetic-type strains).
Discussion
B. cereus, one of the most important foodborne pathogenic bacteria in China (Paudyal et al., 2018), causes different levels of food poisoning incidents (Schoeni and Wong, 2005; Logan, 2012). Our study here first examined the prevalence of B. cereus in retail aquatic products collected from 39 major cities of China. According to previous studies, the contamination rate of B. cereus in different kinds of food was from 6.8 to 57% (Iurlina et al., 2006; Flores-Urbán et al., 2014; Merzougui et al., 2014; Chon et al., 2015; Tewari et al., 2015; Zhang et al., 2017). In comparison, the contamination rate of B. cereus in our study was 25.47%, which is much higher than the rate in other reports, such as the one in Thailand aquatic products (5%; Ananchaipattana et al., 2012) or in American retail seafood (17.9%; Rahmati and Labbé, 2008). Since aquatic products can be rapidly oxidized and decomposed by microorganisms due to their high moisture content and high unsaturated fatty acid content, they are suitable habitats for B. cereus propagation. Besides, B. cereus can produce endospores that are resistant to heat stress (Stenfors Arnesen et al., 2008), particularly for short heat treatments for aquatic products. Thereby, B. cereus will be present in the cooked food where it may multiply and cause foodborne diseases. Considering the regional distribution, the contamination rate in 39 major cities of China ranged from zero (Hong Kong) to 50.00% (Shaoguan) (Supplementary Table S4). In terms of sample type, the highest proportion of food poisoning incidences caused by B. cereus was from crustaceans which was noted in the United States from 1973 to 2006 (Iwamoto et al., 2010). Of note, crustaceans collected in our study also had the highest contamination rate (27.78%), which reminds the consumers to pay attention to the way they consume crustaceans.
99.6% and 61.8% of the isolates contained nheABC cluster and hblACD group, respectively, which are slightly higher than those in retail seafood of United States (94% for nheABC and 50% for hblACD; Rahmati and Labbé, 2008). Moreover, the prevalence of the nheABC cluster in our isolates is higher than that in food products of Poland (78.6% for nheABC; Berthold-Pluta et al., 2019). cytK-2 was detected in 93.1% of the isolates (256/275), which is much higher than the rate of previous reports (37.4–73%; Gao et al., 2018; Fiedler et al., 2019; Yu et al., 2019). Moreover, the proportion (>5%; 14/275) of the emetic strain is higher than the general level around the world (Chaabouni et al., 2015; Fiedler et al., 2019). The cesB-positive strains we isolated had both psychrotrophic and mesophilic signatures (Table 2 and Supplementary Figure S4), corresponding to an intermediate genotype according to von Stetten et al., 1998. In accordance with the previous study, most emetic strains are mesophilic and none of them have hbl genes, whereas a few emetic strains are psychrotrophic and may have hbl genes (Thorsen et al., 2006). In contrast, all cesB-positive isolates we identified were mesophilic as they were unable to grow at 7°C and possessed hbl genes (Table 2 and Supplementary Figure S4), which is different from previous reports (Ehling-Schulz et al., 2005; Thorsen et al., 2006). When considering the regional distribution, 12 of the cesB-positive isolates were identified from the samples collected from central, eastern, and southwest China. The climate in these areas is mostly the subtropical monsoon climate. Therefore, the geographical distribution of these mesophilic cesB-positive isolates may be in line with the regional climate.
According to the report of Ling et al. (2018), we also divided China into seven regions (Supplementary Figure S1). Of the 147 STs, 11 STs (7.48%) were detected in three or more regions. Among them, ST205 was detected in all regions except for the northern part of China. ST26, the common ST with clinical isolates (Carroll et al., 2019), was detected in four regions of China. 275 isolates from aquatic products and eight reference strains were classified into 13 clusters. Either cluster two or 13 has only one strain (Supplementary Figure S3). Most of the strains in clusters one, three, four, and nine were singleton and the profiles of virulence genes within the same cluster were quite similar (Supplementary Figure S3); however, the profiles of antimicrobial resistance were much diverse. In cluster seven, eight strains were resistant to less than six classes of antibiotics, most of which are common β-lactams, and none of these strains contained hblA virulence genes. The cesB-positive strains from aquatic products were mainly distributed in cluster six (8/14; 57.1%), which also contained two clinical emetic strains B. cereus F4810/72 and NC7401. Therefore, the pathogenic potential of these potential emetic strains within the cluster six should not be neglected. The other six cesB-positive strains were randomly distributed into different clusters, indicating that they may evolve from different origins. Additionally, these 14 strains possessed only two profiles of virulence genes [nheA-nheB-nheC-hblC-hblD-cytK-2-cesB (42.9%) or nheA-nheB-nheC-hblA-hblC-hblD-cytK-2-cesB (57.1%)] and the strains without hblA were only from ST26 and ST205 (Supplementary Figure S3).
B. cereus has been considered as an important bacterial pathogen through foodborne transmission (Glasset et al., 2016; Van Cauteren et al., 2017). For example, B. cereus, detected in both salmon (Labbé and Rahmati, 2012) and tuna (Doménech-Sánchez et al., 2011), produced either enterotoxin or vomiting toxin, respectively. There are some fatal cases through foodborne transmission by B. cereus (Veysseyre et al., 2015). Therefore, the application of effective antibiotics is very important for the clinical treatment of B. cereus if the case is very severe. The results of the antimicrobial analysis revealed that the most common resistant profile was AMP-P-AMC-KF-FOX-RD, which is as same as the profile of our previous study (Gao et al., 2018). Consistent with many reports (Luna et al., 2007; Owusu-Kwarteng et al., 2017), our results demonstrated that B. cereus has developed general resistance to β-lactam antibiotics. According to previous studies, B. cereus isolates also present resistance to different antibiotics used clinically, such as erythromycin, ciprofloxacin, clindamycin, chloramphenicol, etc. (Drobniewski, 1993; Tuladhar et al., 2000). In particular, the proportion of multidrug-resistant strains (99.64%) was higher than in previous reports (Gao et al., 2018; Yu et al., 2019), which may be due to the long-term use of different antibiotics in aquaculture (Alderman and Hastings, 1998; Martinez, 2009; Rico et al., 2013). According to the report by Wang et al. (2017), common medical antibiotics such as macrolides, tetracyclines, and fluoroquinolones have also been detected in aquatic products. It is worth noting that our B. cereus isolates in aquatic samples showed a high proportion of intermediate resistance (Figure 2). The overall situation reminds us to pay attention to the antibiotics used in aquaculture and potential hazard might be caused by these different virulent strains with serious antibiotic resistance.
With the increasing popularity of aquatic products in Chinese households and the favorable non-heated way to eat aquatic products, consumers and administrative departments should pay more attention to the potential pathogenic risk of B. cereus.
Conclusion
Overall, 275 B. cereus isolates were identified in 219 aquatic products. Of all the samples, 1.83% (4/219) possessed >1,100 MPN/g B. cereus counting. Different isolates had virulence potential, among which 59.6% contained all three types of enterotoxin genes and 5.1% possessed cesB. Based on the MLST analysis, quite high genetic diversity was discovered, and the distribution of ST205 and ST26 in China was widespread. Given that B. cereus from aquatic products had a high proportion of intermediate resistance to different drugs, it is necessary to continuously monitor the antibiotic resistance of B. cereus in aquatic products and control antibiotics use in aquaculture.
Data Availability Statement
All datasets generated for this study are included in the article/Supplementary Material.
Author Contributions
YD, QW, JW, JumZ, YZ, and MeC conceived the project and designed the experiments. YZ, MeC, PY, SY, HG, JunZ, HuZ, MoC, HaZ, SW, RP, QY, LX, SZ, and YL performed the experiments. QW and YD supervised the project. YZ, MeC, and YD analyzed the data and wrote the manuscript. QW, JW, JumZ, and YD complemented the writing. All authors contributed to the article and approved the submitted version.
Funding
The authors would like to acknowledge the financial support of Guangdong Technological Innovation Strategy of Special Funds (Key Areas of Research and Development Program, Grant number 2018B020205003), National Key R&D Program of China (Grant Number 2018YFC1602500), National Natural Science Foundation of China (Grant numbers 31730070 and 31701195), GDAS’ Special Project of Science and Technology Development (Grant number 2017GDASCX-0201), the 1000-Youth Elite Program, and the 111 Project.
Conflict of Interest
The authors declare that the research was conducted in the absence of any commercial or financial relationships that could be construed as a potential conflict of interest.
Supplementary Material
The Supplementary Material for this article can be found online at: https://www.frontiersin.org/articles/10.3389/fmicb.2020.01513/full#supplementary-material
Footnotes
References
Agata, N., Ohta, M., Mori, M., and Isobe, M. (1995). A novel dodecadepsipeptide, cereulide, is an emetic toxin of Bacillus cereus. FEMS Microbiol. Lett. 129, 17–20. doi: 10.1016/0378-1097(95)00119-P
Alderman, D. J., and Hastings, T. S. (1998). Antibiotic use in aquaculture: development of antibiotic resistance-potential for consumer health risks. Int. J. Food Sci. Technol. 33, 139–155. doi: 10.1046/j.1365-2621.1998.3320139.x
Ananchaipattana, C., Hosotani, Y., Kawasaki, S., Pongsawat, S., Latiful, B. M., Isobe, S., et al. (2012). Prevalence of foodborne pathogens in retailed foods in Thailand. Foodborne Pathog. Dis. 9, 835–840. doi: 10.1089/fpd.2012.1169
Andersson, A., Rönner, U., and Granum, P. E. (1995). What problems does the food industry have with the spore-forming pathogens Bacillus cereus and Clostridium perfringens? Int. J. Food Microbiol. 28, 145–155. doi: 10.1016/0168-1605(95)00053-4
Berthold-Pluta, A., Pluta, A., Garbowska, M., and Stefañska, I. (2019). Prevalence and toxicity characterization of Bacillus cereus in food products from Poland. Foods 8:269. doi: 10.3390/foods8070269
Bottone, E. J. (2010). Bacillus cereus, a volatile human pathogen. Clin. Microbiol. Rev. 23, 382–398. doi: 10.1128/CMR.00073-09
Carroll, L. M., Wiedmann, M., Mukherjee, M., Nicholas, D. C., Mingle, L. A., Dumas, N. B., et al. (2019). Characterization of emetic and diarrheal Bacillus cereus strains from a 2016 foodborne outbreak using whole-genome sequencing: addressing the microbiological, epidemiological, and bioinformatic challenges. Front. Microbiol. 10:144. doi: 10.3389/fmicb.2019.00144
Chaabouni, I., Barkallah, I., Hamdi, C., Jouini, A., Saidi, M., Mahillon, J., et al. (2015). Metabolic capacities and toxigenic potential as key drivers of Bacillus cereus ubiquity and adaptation. Ann. Microbiol. 65, 975–983. doi: 10.1007/s13213-014-0941-9
Chon, J. W., Yim, J. H., Kim, H. S., Kim, D. H., Kim, H., Oh, D. H., et al. (2015). Quantitative prevalence and toxin gene profile of Bacillus cereus from ready-to-eat vegetables in South Korea. Foodborne Pathog. Dis. 12, 795–799. doi: 10.1089/fpd.2015.1977
Doménech-Sánchez, A., Laso, E., Pérez, M. J., and Berrocal, C. I. (2011). Emetic disease caused by Bacillus cereus after consumption of tuna fish in a beach club. Foodborne Pathog. Dis. 8, 835–837. doi: 10.1089/fpd.2010.0783
Drobniewski, F. A. (1993). Bacillus cereus and related species. Clin. Microbiol. Rev. 6, 324–338. doi: 10.1128/cmr.6.4.324
Ehling-Schulz, M., Frenzel, E., and Gohar, M. (2015). Food-bacteria interplay: pathometabolism of emetic Bacillus cereus. Front. Microbiol. 6:704. doi: 10.3389/fmicb.2015.00704
Ehling-Schulz, M., Svensson, B., Guinebretiere, M. H., Lindbäck, T., Andersson, M., Schulz, A., et al. (2005). Emetic toxin formation of Bacillus cereus is restricted to a single evolutionary lineage of closely related strains. Microbiology 151(Pt 1), 183–197. doi: 10.1099/mic.0.27607-0
Fiedler, G., Schneider, C., Igbinosa, E. O., Kabisch, J., Brinks, E., Becker, B., et al. (2019). Antibiotics resistance and toxin profiles of Bacillus cereus-group isolates from fresh vegetables from German retail markets. BMC Microbiol. 19:250. doi: 10.1186/s12866-019-1632-2
Flores-Urbán, K. A., Natividad-Bonifacio, I., Vázquez-Quiñones, C. R., Vázquez-Salinas, C., and Quiñones-Ramírez, E. I. (2014). Detection of toxigenic Bacillus cereus strains isolated from vegetables in Mexico City. J. Food Prot. 77, 2144–2147. doi: 10.4315/0362-028x.jfp-13-479
Gao, T., Ding, Y., Wu, Q., Wang, J., Zhang, J., Yu, S., et al. (2018). Prevalence, virulence genes, antimicrobial susceptibility, and genetic diversity of Bacillus cereus isolated from pasteurized milk in China. Front. Microbiol. 9:533. doi: 10.3389/fmicb.2018.00533
Gilbert, R. J., de Louvois, J., Donovan, T., Little, C., Nye, K., Ribeiro, C. D., et al. (2000). Guidelines for the microbiological quality of some ready-to-eat foods sampled at the point of sale. PHLS advisory committee for food and dairy products. Commun. Dis. Public Health 3, 163–167.
Glasset, B., Herbin, S., Guillier, L., Cadel-Six, S., Vignaud, M. L., Grout, J., et al. (2016). Bacillus cereus-induced food-borne outbreaks in France, 2007 to 2014: epidemiology and genetic characterisation. Euro Surveill. 21:30413. doi: 10.2807/1560-7917.ES.2016.21.48.30413
Guinebretière, M. H., Thompson, F. L., Sorokin, A., Normand, P., Dawyndt, P., Ehling-Schulz, M., et al. (2008). Ecological diversification in the Bacillus cereus group. Environ. Microbiol. 10, 851–865. doi: 10.1111/j.1462-2920.2007.01495.x
Hansen, B. M., and Hendriksen, N. B. (2001). Detection of enterotoxic Bacillus cereus and Bacillus thuringiensis strains by PCR analysis. Appl. Environ. Microbiol. 67, 185–189. doi: 10.1128/AEM.67.1.185-189.2001
Hoffmaster, A. R., Hill, K. K., Gee, J. E., Marston, C. K., De, B. K., Popovic, T., et al. (2006). Characterization of Bacillus cereus isolates associated with fatal pneumonias: strains are closely related to Bacillus anthracis and harbor B. anthracis virulence genes. J. Clin. Microbiol. 44, 3352–3360. doi: 10.1128/JCM.00561-06
Iurlina, M. O., Saiz, A. I., Fuselli, S. R., and Fritz, R. (2006). Prevalence of Bacillus spp. in different food products collected in Argentina. LWT – Food Sci. Technol. 39, 105–110. doi: 10.1016/j.lwt.2005.01.006
Iwamoto, M., Ayers, T., Mahon, B. E., and Swerdlow, D. L. (2010). Epidemiology of seafood-associated infections in the United States. Clin. Microbiol. Rev. 23, 399–411. doi: 10.1128/CMR.00059-09
Kim, H. W., Hong, Y. J., Jo, J. I., Ha, S. D., Kim, S. H., Lee, H. J., et al. (2017). Raw ready-to-eat seafood safety: microbiological quality of the various seafood species available in fishery, hyper and online markets. Lett. Appl. Microbiol. 64, 27–34. doi: 10.1111/lam.12688
Labbé, R., and Rahmati, T. (2012). Growth of enterotoxigenic Bacillus cereus on salmon (Oncorhynchus nerka). J. Food Prot. 75, 1153–1156. doi: 10.4315/0362-028X.JFP-11-485
Ling, N., Li, C., Zhang, J., Wu, Q., Zeng, H., He, W., et al. (2018). Prevalence and molecular and antimicrobial characteristics of Cronobacter spp. isolated from raw vegetables in China. Front. Microbiol. 9:1149. doi: 10.3389/fmicb.2018.01149
Logan, N. A. (2012). Bacillus and relatives in foodborne illness. J. Appl. Microbiol. 112, 417–429. doi: 10.1111/j.1365-2672.2011.05204.x
Luna, V. A., King, D. S., Gulledge, J., Cannons, A. C., Amuso, P. T., and Cattani, J. (2007). Susceptibility of Bacillus anthracis, Bacillus cereus, Bacillus mycoides, Bacillus pseudomycoides and Bacillus thuringiensis to 24 antimicrobials using Sensititre automated microbroth dilution and Etest agar gradient diffusion methods. J. Antimicrob. Chemother. 60, 555–567. doi: 10.1093/jac/dkm213
Luu-Thi, H., Khadka, D. B., and Michiels, C. W. (2014). Thermal inactivation parameters of spores from different phylogenetic groups of Bacillus cereus. Int. J. Food Microbiol. 189, 183–188. doi: 10.1016/j.ijfoodmicro.2014.07.027
Magiorakos, A. P., Srinivasan, A., Carey, R. B., Carmeli, Y., Falagas, M. E., Giske, C. G., et al. (2012). Multidrug-resistant, extensively drug-resistant and pandrug-resistant bacteria: an international expert proposal for interim standard definitions for acquired resistance. Clin. Microbiol. Infect. 18, 268–281. doi: 10.1111/j.1469-0691.2011.03570.x
Martinez, J. L. (2009). Environmental pollution by antibiotics and by antibiotic resistance determinants. Environ. Pollut. 157, 2893–2902. doi: 10.1016/j.envpol.2009.05.051
Merzougui, S., Lkhider, M., Grosset, N., Gautier, M., and Cohen, N. (2014). Prevalence, PFGE typing, and antibiotic resistance of Bacillus cereus group isolated from food in Morocco. Foodborne Pathog. Dis. 11, 145–149. doi: 10.1089/fpd.2013.1615
Messelhäußer, U., and Ehling-Schulz, M. (2018). Bacillus cereus—a multifaceted opportunistic pathogen. Curr. Clin. Micro. Rpt. 5, 120–125. doi: 10.1007/s40588-018-0095-9
Miguéis, S., Moura, A. T., Saraiva, C., and Esteves, A. (2016). Influence of season and type of restaurants on sashimi microbiota. Eur. J. Public Health 26, 877–881. doi: 10.1093/eurpub/ckw009
Miguéis, S., Santos, C., Saraiva, C., and Esteves, A. (2015). Evaluation of ready to eat sashimi in northern Portugal restaurants. Food Control 47, 32–36. doi: 10.1016/j.foodcont.2014.06.025
Nascimento, M., Sousa, A., Ramirez, M., Francisco, A. P., Carriço, J. A., and Vaz, C. (2017). PHYLOViZ 2.0: providing scalable data integration and visualization for multiple phylogenetic inference methods. Bioinformatics 33, 128–129. doi: 10.1093/bioinformatics/btw582
Oltuszak-Walczak, E., and Walczak, P. (2013). PCR detection of cytK gene in Bacillus cereus group strains isolated from food samples. J. Microbiol. Methods 95, 295–301. doi: 10.1016/j.mimet.2013.09.012
Owusu-Kwarteng, J., Wuni, A., Akabanda, F., Tano-Debrah, K., and Jespersen, L. (2017). Prevalence, virulence factor genes and antibiotic resistance of Bacillus cereus sensu lato isolated from dairy farms and traditional dairy products. BMC Microbiol. 17:65. doi: 10.1186/s12866-017-0975-9
Paudyal, N., Pan, H., Liao, X., Zhang, X., Li, X., Fang, W., et al. (2018). A meta-analysis of major foodborne pathogens in Chinese food commodities between 2006 and 2016. Foodborne Pathog. Dis. 15, 187–197. doi: 10.1089/fpd.2017.2417
Rahmati, T., and Labbé, R. (2008). Levels and toxigenicity of Bacillus cereus and Clostridium perfringens from retail seafood. J. Food Prot. 71, 1178–1185. doi: 10.4315/0362-028x-71.6.1178
Rico, A., Phu, T. M., Satapornvanit, K., Min, J., Shahabuddin, A. M., Henriksson, P. J. G., et al. (2013). Use of veterinary medicines, feed additives and probiotics in four major internationally traded aquaculture species farmed in Asia. Aquaculture 412–413, 231–243. doi: 10.1016/j.aquaculture.2013.07.028
Rishi, E., Rishi, P., Sengupta, S., Jambulingam, M., Madhavan, H. N., Gopal, L., et al. (2013). Acute postoperative Bacillus cereus endophthalmitis mimicking toxic anterior segment syndrome. Ophthalmology 120, 181–185. doi: 10.1016/j.ophtha.2012.07.009
Schoeni, J. L., and Wong, A. C. L. (2005). Bacillus cereus food poisoning and its toxins. J. Food Prot. 68, 636–648. doi: 10.4315/0362-028x-68.3.636
Stenfors, L. P., and Granum, P. E. (2001). Psychrotolerant species from the Bacillus cereus group are not necessarily Bacillus weihenstephanensis. FEMS Microbiol. Lett. 197, 223–228. doi: 10.1111/j.1574-6968.2001.tb10607.x
Stenfors Arnesen, L. P., Fagerlund, A., and Granum, P. E. (2008). From soil to gut: Bacillus cereus and its food poisoning toxins. FEMS Microbiol. Rev. 32, 579–606. doi: 10.1111/j.1574-6976.2008.00112.x
Tewari, A., Singh, S. P., and Singh, R. (2015). Incidence and enterotoxigenic profile of Bacillus cereus in meat and meat products of Uttarakhand. India. J. Food Sci. Technol. 52, 1796–1801. doi: 10.1007/s13197-013-1162-0
The Clinical and Laboratory Standards Institute [CLSI] (2010). Performance Standards for Antimicrobial Susceptibility Testing; Twentieth Informational Supplement. Approved Standard-M100-S20. Wayne, PA: The Clinical and Laboratory Standards Institute.
The Hygiene Ministry of China (2003). National Food Safety Standard. Food Microbiological Examination: Bacillus Cereus TEST. Beijing: The Hygiene Ministry of China.
The Hygiene Ministry of China (2010). National Food Safety Standard. Food Microbiological Examination: General Guidelines. Beijing: The Hygiene Ministry of China.
The Hygiene Ministry of China (2014). National Food Safety Standard. Food Microbiological Examination: General Guidelines. Beijing: The Hygiene Ministry of China.
Thilsted, S. H., James, D., Toppe, J., Subasinghe, R., and Karunasagar, I. (2014). “Maximizing the contribution of fish to human nutrition,” in Proceedings of the ICN2 Second International Conference on Nutrition, Rome: FAO.
Thorsen, L., Hansen, B. M., Nielsen, K. F., Hendriksen, N. B., Phipps, R. K., and Budde, B. B. (2006). Characterization of emetic Bacillus weihenstephanensis, a new cereulide-producing bacterium. Appl. Environ. Microbiol. 72, 5118–5121. doi: 10.1128/AEM.00170-06
Tuladhar, R., Patole, S. K., Koh, T. H., Norton, R., and Whitehall, J. S. (2000). Refractory Bacillus cereus infection in a neonate. Int. J. Clin. Pract. 54, 345–347.
Van Cauteren, D., Le Strat, Y., Sommen, C., Bruyand, M., Tourdjman, M., Da Silva, N. J., et al. (2017). Estimated annual numbers of foodborne pathogen-associated illnesses, hospitalizations, and deaths, France, 2008-2013. Emerg. Infect. Dis. 23, 1486–1492. doi: 10.3201/eid2309.170081
Versalovic, J., Koeuth, T., and Lupski, J. R. (1991). Distribution of repetitive DNA-sequences in eubacteria and application to fingerprinting of bacterial genomes. Nucleic Acids Res. 19, 6823–6831. doi: 10.1093/nar/19.24.6823
Veysseyre, F., Fourcade, C., Lavigne, J. P., and Sotto, A. (2015). Bacillus cereus infection: 57 case patients and a literature review. Med. Mal. Infect. 45, 436–440. doi: 10.1016/j.medmal.2015.09.011
von Stetten, F., Francis, K. P., Lechner, S., Neuhaus, K., and Scherer, S. (1998). Rapid discrimination of psychrotolerant and mesophilic strains of the Bacillus cereus group by PCR targeting of 16S rDNA. J. Microbiol. Meth. 34, 99–106. doi: 10.1016/s0167-7012(98)00077-3
Wang, H., Ren, L., Yu, X., Hu, J., Chen, Y., He, G., et al. (2017). Antibiotic residues in meat, milk and aquatic products in Shanghai and human exposure assessment. Food Control 80, 217–225. doi: 10.1016/j.foodcont.2017.04.034
Yu, P., Yu, S., Wang, J., Guo, H., Zhang, Y., Liao, X., et al. (2019). Bacillus cereus isolated from vegetables in China: incidence, genetic diversity, virulence genes, and antimicrobial resistance. Front. Microbiol. 10:948. doi: 10.3389/fmicb.2019.00948
Yu, S., Yu, P., Wang, J., Li, C., Guo, H., Liu, C., et al. (2020). A study on prevalence and characterization of Bacillus cereus in ready-to-eat foods in China. Front. Microbiol. 10:3043. doi: 10.3389/fmicb.2019.03043
Keywords: aquatic products, Bacillus cereus, prevalence, MLST, multi-drug resistance
Citation: Zhang Y, Chen M, Yu P, Yu S, Wang J, Guo H, Zhang J, Zhou H, Chen M, Zeng H, Wu S, Pang R, Ye Q, Xue L, Zhang S, Li Y, Zhang J, Wu Q and Ding Y (2020) Prevalence, Virulence Feature, Antibiotic Resistance and MLST Typing of Bacillus cereus Isolated From Retail Aquatic Products in China. Front. Microbiol. 11:1513. doi: 10.3389/fmicb.2020.01513
Received: 09 March 2020; Accepted: 10 June 2020;
Published: 03 July 2020.
Edited by:
Giovanna Suzzi, University of Teramo, ItalyReviewed by:
Christophe Nguyen-The, Institut National de la Recherche Agronomique (INRA), FranceLucilla Iacumin, University of Udine, Italy
Copyright © 2020 Zhang, Chen, Yu, Yu, Wang, Guo, Zhang, Zhou, Chen, Zeng, Wu, Pang, Ye, Xue, Zhang, Li, Zhang, Wu and Ding. This is an open-access article distributed under the terms of the Creative Commons Attribution License (CC BY). The use, distribution or reproduction in other forums is permitted, provided the original author(s) and the copyright owner(s) are credited and that the original publication in this journal is cited, in accordance with accepted academic practice. No use, distribution or reproduction is permitted which does not comply with these terms.
*Correspondence: Qingping Wu, wuqp203@163.com; Yu Ding, dingyu@jnu.edu.cn
†These authors have contributed equally to this work