- Department of Hematology, Rheumatology and Infectious Disease, Faculty of Life Sciences, Graduate School of Medical Sciences, Kumamoto University, Kumamoto, Japan
Human T-cell leukemia virus type 1 (HTLV-1) establishes persistent infection in vivo in two distinct ways: de novo infection and clonal proliferation of infected cells. Two viral genes, Tax and HTLV-1 bZIP factor (HBZ) play critical roles in viral transcription and promotion of T-cell proliferation, respectively. Tax is a potent transactivator not only for viral transcription but also for many cellular oncogenic pathways, such as the NF-κB pathway. HBZ is a suppressor of viral transcription and has the potential to change the immunophenotype of infected cells, conferring an effector regulatory T cell (eTreg)-like signature (CD4+ CD25+ CCR4+ TIGIT+ Foxp3+) and enhancing the proliferation of this subset. Reports that mice transgenic for either gene develop malignant tumors suggest that both Tax and HBZ are involved in leukemogenesis by HTLV-1. However, the immunogenicity of Tax is very high, and its expression is generally suppressed in vivo. Recently, it was found that Tax can be expressed transiently in a small subpopulation of adult T-cell leukemia-lymphoma (ATL) cells and plays a critical role in maintenance of the overall population. HBZ is expressed in almost all infected cells except for the rare Tax-expressing cells, and activates the pathways associated with cell proliferation. These findings indicate that HTLV-1 fine-tunes the expression of viral genes to control the mode of viral propagation. The interplay between Tax and HBZ is the basis of a sophisticated strategy to evade host immune surveillance and increase transmission – and can lead to ATL as a byproduct.
Introduction
Human T-cell leukemia virus type 1 (HTLV-1) belongs to the delta type retroviruses, which also include bovine leukemia virus (BLV), human T-cell leukemia virus type 2 (HTLV-2) and the simian T-cell leukemia viruses (STLVs) (Hajj et al., 2012). HTLV-1 is a causative agent of several human diseases, including adult T-cell leukemia-lymphoma (ATL), HTLV-1 associated myelopathy (HAM)/tropical spastic paraparesis (TSP), and HTLV-1 uveitis (Matsuoka and Jeang, 2007). ATL is a fatal malignancy of CD4+ T lymphocytes, characterized by pleomorphic leukemic cells with hypersegmented nuclei, called “flower cells,” of a particular immunophenotype similar to regulatory T cells (Tregs) (Karube et al., 2004; Satou et al., 2012).
The HTLV-1 provirus encodes several regulatory and accessory proteins in its pX region. These viral products are involved in viral replication, latency and persistence of infected cells (Franchini et al., 2003). It is thought that the prolonged survival and accelerated proliferation of HTLV-1-infected cells caused by viral factors induces cellular transformation, leading to the onset of ATL after a long latent period. Among the viral genes, tax and HTLV-1 bZIP factor (HBZ), which are encoded in the sense and antisense transcripts, respectively, have been shown to have oncogenic properties in transgenic mouse models (Yasunaga and Matsuoka, 2011). Tax is a pleiotropic viral protein that potently activates viral transcription and dysregulates the transcription and function of many cellular genes. Since Tax is highly immunogenic, its expression is often suppressed in vivo (Takeda et al., 2004). On the other hand, transcription of HBZ is consistently detected in all ATL cases (Satou et al., 2006), suggesting that it is critical for the oncogenic mechanism of HTLV-1. The multifarious functions of HBZ have been under study since HBZ was identified in 2002 (Gaudray et al., 2002). It appears that HBZ defines the immunophenotype of HTLV-1-infected and ATL cells, affects the microenvironment and the host immune system, and contributes to persistent infection and pathogenesis. This review summarizes recent findings on the natures and functions of Tax and HBZ and discusses their collaborative role in leukemogenesis by HTLV-1.
Dynamics of Htlv-1-Infected Cells In Vivo
Modes of Viral Propagation
There are two modes of HTLV-1 propagation in vivo: de novo infection and clonal expansion (Figure 1). de novo infection is mediated by infected cells, and is established by integration of the provirus into the host genome. Since HTLV-1 replication is quite low in vivo, HTLV-1 transmits from infected cell to uninfected cell through cell-to-cell contact, not via free virions (Igakura et al., 2003; Yasunaga and Matsuoka, 2011). When de novo infection occurs, a newly infected cell has a unique integration site of the provirus; thus de novo infection increases the variety of HTLV-1-infected clones. On the other hand, clonal expansion is a proliferation of infected cells, which increases the abundance of each clone. It has been reported that HTLV-1-infected clones, in which the provirus is integrated in the same site of the host genome, persisted in infected people over a long period of time (Etoh et al., 1997), indicating that HTLV-1 has the machinery to enhance the proliferation and survival of infected cells. For de novo infection, Tax is critical, since it is required for efficient viral replication. In contrast, HBZ appears to play an important role for clonal expansion, since it promotes the proliferation of CD4+ T cells (Satou et al., 2006). Previous studies of seroconverters showed that, early after initial infection, the number of unique clones is high (i.e., the clonality is low) and the proviral load is variable, while both the clonality and the proviral load stabilize within few years (Manns et al., 1999; Okayama et al., 2001; Tanaka et al., 2005). Since Tax is highly immunogenic and de novo infection provokes immune activation against HTLV-1, clonal expansion is the dominant way of the virus to persist during the long-term carrier state. The clonal proliferation and prolonged survival of host cells caused by HTLV-1 may also promote cellular transformation, and consequently trigger the onset of ATL.
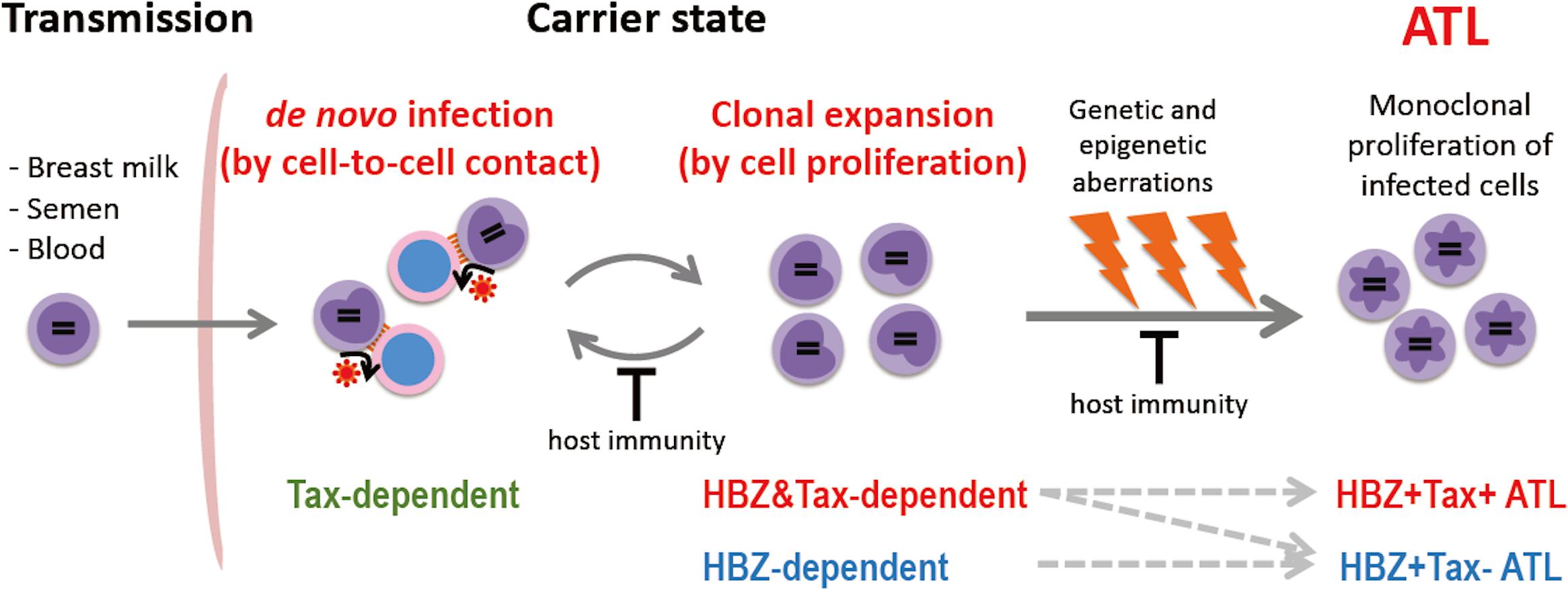
Figure 1. Propagation of HTLV-1-infected cells in vivo. HTLV-1 is transmitted by infected cells in breast milk, semen, and blood, and establishes infection by cell-to-cell contact. HTLV-1 spreads in vivo by de novo infection and clonal expansion. Tax is required for de novo infection since Tax drives viral replication. HBZ is critical for clonal expansion. Host immunity controls the number of infected cells and their clonality. In the carrier state, infected cells survive for a long period; genetic/epigenetic aberrations accumulate, and a malignant clone may emerge, resulting in ATL. Approximately half of ATL cases develop in a Tax-independent manner (i.e., Tax– ATL), while the other half retain the capacity to express Tax (Tax+ ATL).
Characteristics of Infected Cells
In HTLV-1-infected subjects, the majority of infected cells are CD4+ T cells, and several surface molecules, such as CD25 (IL-2Rα), cell adhesion molecule 1 (CADM1), and C-C chemokine receptor 4 (CCR4), are recognized as markers of HTLV-1-infected cells including ATL cells (Yoshie et al., 2002; Sasaki et al., 2005). However, it is known that HTLV-1 utilizes ubiquitously expressed proteins – glucose transporter GLUT1, heparan sulfate proteoglycan (HSPG), and neuropilin-1 – as receptors for entry into cells (Miyazato and Matsuoka, 2014), and in HTLV-1 carriers the provirus can be detected in not only CD4+ T cells, but also in other lineages of hematopoietic cells: CD8+ T cells, monocytes, B cells, and neutrophils (Koyanagi et al., 1993; Yasunaga et al., 2001; Furuta et al., 2017). A recent study also suggested that hematopoietic stem cells in bone marrow are infected with HTLV-1 and act as a reservoir for infection (Furuta et al., 2017). These facts suggest that HTLV-1 promotes the differentiation of infected cells toward CD4+ T cells with a specific immunophenotype. HTLV-1-infected/ATL cells are known to express several proteins associated with Tregs (Karube et al., 2004; Satou et al., 2012; Sugiyama et al., 2013). Among them, FOXP3 is a master transcription factor of Tregs, and controls the expression of a wide range of genes that exert immune-suppressive functions (Wing et al., 2019). HBZ induces transcription of the FOXP3 gene, and the number of Tregs in HBZ transgenic mice is significantly increased compared with wild type mice (Satou et al., 2011; Zhao et al., 2011), suggesting that HBZ defines the immunophenotype of infected cells. The detailed functions of HBZ are described later in this review.
Expression of Tax and HBZ
Promoters of Tax and HBZ
The HTLV-1 provirus has two long terminal repeats (LTRs), one at each end, called the 5′LTR and the 3′LTR, respectively (Figure 2A; Matsuoka and Yasunaga, 2013). The 5′LTR acts as a promoter of all transcripts from the plus strand of the provirus (sense transcripts), and several spliced and unspliced viral mRNAs are induced by activation of 5′LTR. Tax is encoded in ORF IV of the doubly spliced sense transcript, and potently activates the 5′LTR through binding to cyclic AMP-responsive element binding protein (CREB) and recruitment of the complex to the Tax-responsive elements (TREs) in the LTR (Kashanchi and Brady, 2005). Since sense transcripts act as the viral genomic RNA and the templates for all structural proteins, such as Gag, Pol, and Env, Tax is critical for viral replication and de novo infection to uninfected cells. On the other hand, the minus strand of the provirus encodes two different isoforms of HBZ, spliced (sHBZ) and unspliced HBZ (usHBZ) (Gaudray et al., 2002; Cavanagh et al., 2006; Murata et al., 2006; Satou et al., 2006). It has been reported that sHBZ is transcribed from the 3′LTR, and its promoter activity is stronger than that of usHBZ (Yoshida et al., 2008). It has been also shown that the sHBZ protein is more abundant than in cells than that of usHBZ (Cavanagh et al., 2006; Murata et al., 2006; Caceres et al., 2018), suggesting that the effect of sHBZ is dominant in infected cells.
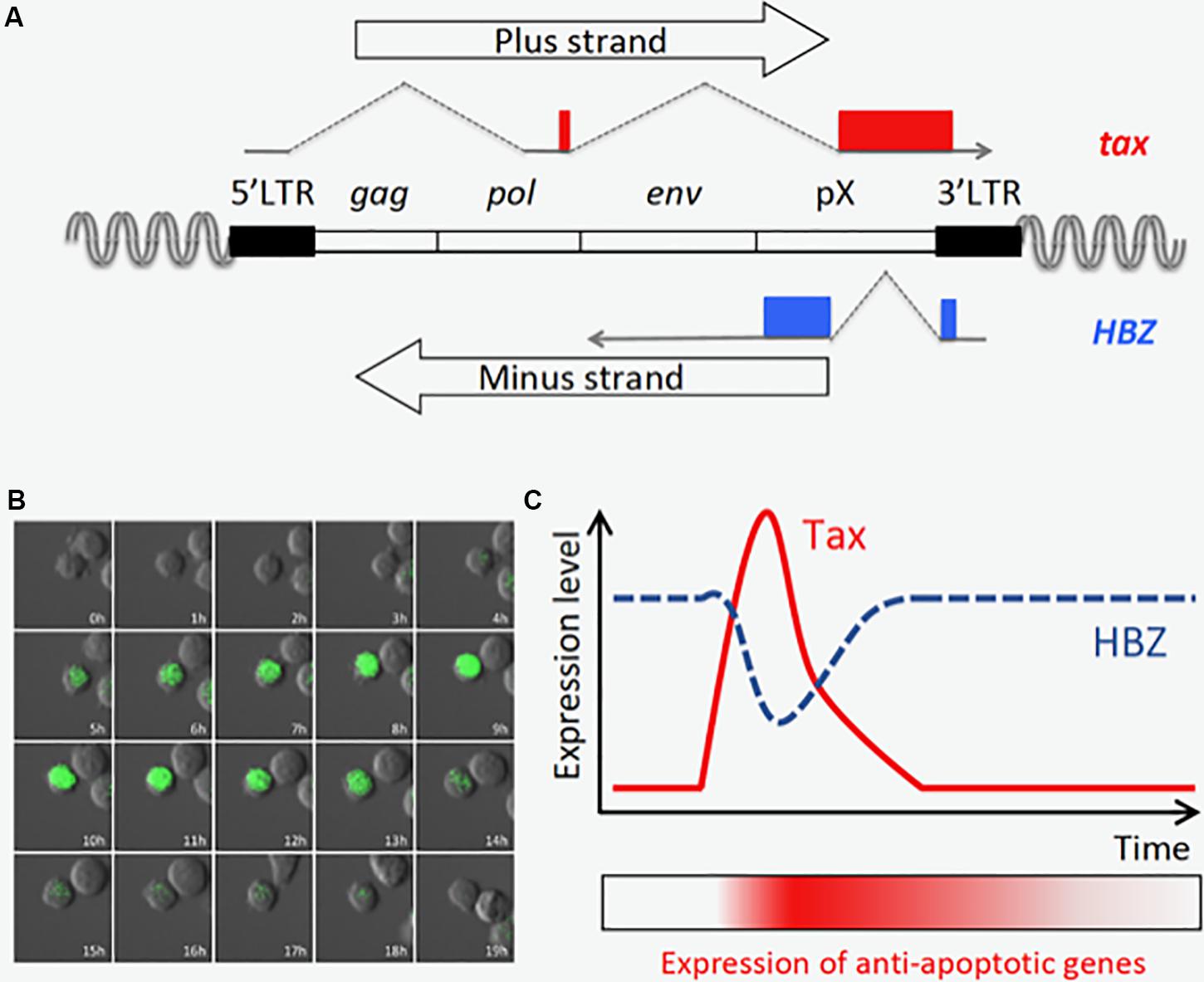
Figure 2. Tax is transiently expressed in an ATL cell line, MT-1. (A) Structure of the provirus and the tax and HBZ genes. (B) Time-lapse imaging of Tax expression in MT-1 cells. Destabilized EGFP protein (d2EGFP) expressed under the control of Tax-responsive elements is used to visualize Tax expression. The image was modified from the one obtained in the author’s previous work (Mahgoub et al., 2018). (C) Tax is transiently expressed, and HBZ expression is oppositely regulated (Mahgoub et al., 2018). Prolonged induction of anti-apoptotic genes is observed even after Tax is diminished, and this may be important for maintenance of the MT-1 cell population.
Differences in Expression Patterns Between Tax and HBZ
The modes of 5′LTR and 3′LTR activation are quite different from each other; the former is inducible, and the latter is continuous (Mahgoub et al., 2018). Recent studies revealed that several stresses, such as genotoxic stimuli and hypoxic conditions, trigger 5′LTR activation and Tax expression in HTLV-1-infected cells (Kulkarni et al., 2017; Mahgoub et al., 2018). In primary ATL cases, Tax expression is generally suppressed, and approximately half of cases completely lose Tax expression. Three mechanisms for this silencing of Tax are reported: the first is genetic aberration, such as a nonsense mutation or an insertion/deletion in the tax gene; the second is deletion of the 5′LTR, and the third is CpG hypermethylation of the 5′LTR (Takeda et al., 2004; Taniguchi et al., 2005). Since Tax is highly immunogenic, silencing Tax expression helps ATL cells evade the host immune system. Importantly, a certain number of infected cells harbor such mutations even in the carrier stage, and these genetic changes seem to occur before proviral integration (Miyazaki et al., 2007; Fan et al., 2010; Katsuya et al., 2019), suggesting that Tax-defective infected cells can survive for a long time and subsequently transform into malignant clones. The other half of ATL cases express low levels of Tax or have the potential to induce Tax. Indeed, it is known that Tax is inducible in half of ATL cases after leukemic cells are transferred to ex vivo culture (Kurihara et al., 2005).
In contrast, HBZ is constantly detected, and the sequences of the HBZ gene and the 3′LTR are conserved in all ATL cases (Satou et al., 2006; Miyazaki et al., 2007; Fan et al., 2010). There are three SP1 transcription factor binding motifs in the 3′LTR (Yoshida et al., 2008). Since the 3′LTR is epigenetically activated in infected cells (Koiwa et al., 2002; Takeda et al., 2004; Taniguchi et al., 2005; Satou et al., 2016; Miura et al., 2018), and SP1 is ubiquitously expressed in many cell types, it is reasonable to believe that HBZ is transcribed in all infected/ATL cells.
Recently, time-lapse imaging showed that Tax is transiently expressed in a small subset (0.5∼3%) of MT-1 cells, a cell line derived from an ATL clone (Figure 2B; Mahgoub et al., 2018). Single cell quantitative RT-PCR also revealed that expression levels of tax and HBZ are inversely correlated; most MT-1 cells express high levels of HBZ but no Tax, while the rare Tax-expressing cells express less HBZ (Mahgoub et al., 2018). It is technically difficult to observe the transient expression of Tax in primary ATL cells; however, it is reported that fresh ATL cells have similar expression levels of Tax and HBZ compared with MT-1 cells (Usui et al., 2008), suggesting those ATL cases have similar expression profiles of Tax and HBZ in vivo. Primary ATL cases can be divided into two subclasses based on Tax status: the Tax-negative type in which Tax is completely silenced, and the Tax-positive type which resembles MT1. Since knockdown of either Tax or HBZ suppresses the proliferation of MT-1 cells (Satou et al., 2006; Mahgoub et al., 2018), both viral factors are thought to be critical for the development of Tax-positive ATL cases.
Functions of Tax and HBZ
Tax
Tax is a potent transactivator not only of viral transcription but also of many cellular oncogenic molecules and pathways. It is evident that Tax is an oncoprotein, since Tax can transform primary rodent cells and immortalize human CD4+ T cells, and Tax-transgenic animals develop malignant tumors (Yasunaga and Matsuoka, 2011). Activation of the NF-κB pathway is one of the most important functions of Tax for the survival of infected cells (Matsuoka and Jeang, 2007; Harhaj and Giam, 2018). It was shown that a Tax mutant defective for NF-κB activation could not immortalize T cells (Robek and Ratner, 1999), and transgenic mice containing inducible wild type Tax developed a skin inflammation while mice expressing mutant Tax did not (Kwon et al., 2005). Tax strongly activates the NF-κB pathway through interaction with IKKγ (also known as NEMO), which is a subunit of the IκB kinase (IKK) complex (Sun and Yamaoka, 2005; Currer et al., 2012). Recent biochemical studies revealed that Tax hijacks two E3 ubiquitin ligases, RNF8 and LUBAC, and induces the active macromolecular IKK complex via a process mediated by hybrid polyubiquitin chains (Ho et al., 2015; Shibata et al., 2017).
On the other hand, constitutive Tax expression induces hyperactivation of NF-κB and triggers DNA damage and cellular senescence (Kinjo et al., 2010; Zhi et al., 2011). Therefore, it is disadvantageous for infected/ATL cells to express Tax, although Tax is important for de novo infection and cell survival. There must be some way for infected cells to evade Tax-induced cell toxicity and host immune reaction. It was shown that MT-1 cells express Tax for very a short time (∼20 h) in a small subpopulation (Mahgoub et al., 2018), suggesting that transient Tax expression minimizes the risk of its harmful effects. Another way to evade senescence induced by Tax involves HBZ. HBZ suppresses the canonical NF-κB pathway through inhibition of p65/RelA (Zhao et al., 2009; Zhi et al., 2011; Wurm et al., 2012). In addition, in the case of ATL cells, the senescence checkpoint may be inactivated by genetic and/or epigenetic host gene aberrations accumulated during the leukemogenic process (Shudofsky and Giam, 2019).
HBZ
Among the regulatory/accessory genes encoded in HTLV-1, HBZ is the only gene which is conserved and expressed in all ATL cases (Satou et al., 2006; Miyazaki et al., 2007; Fan et al., 2010). The significance of HBZ in HTLV-1-mediated oncogenesis is proven by both in vitro and in vivo studies. Forced expression of HBZ in CD4+ T cells promotes cell proliferation (Satou et al., 2006), inhibits apoptosis (Tanaka-Nakanishi et al., 2014), impedes DNA damage responses (Vernin et al., 2014; Takiuchi et al., 2017; Rushing et al., 2018), and mediates transformation of primary CD4+ T cells (Kasugai et al., 2016). Inhibition of HBZ suppresses the proliferation of ATL cell lines (Satou et al., 2006; Nakagawa et al., 2018), indicating that HBZ is critical for the proliferation and maintenance of ATL cells. HBZ transgenic (HBZ-Tg) mice that express HBZ in CD4+ T cells develop systemic inflammation, such as dermatitis and pulmonary alveolitis, and T-cell lymphoma (Satou et al., 2011). In HBZ-Tg mice, production of the immunosuppressive cytokine IL-10 is higher than in wild-type littermates, suggesting that HBZ contributes to the suppression of host immunity (Yasuma et al., 2016). Another transgenic mouse strain that expresses HBZ under the control of the granzyme B promoter demonstrates a lymphoproliferative disease and hypercalcemia, which is often seen in ATL patients (Esser et al., 2017). HBZ dysregulates the transcription of many cellular genes using its two different molecular forms, HBZ protein and HBZ RNA (Satou et al., 2006; Mitobe et al., 2015). HBZ protein has a bZIP domain in the C-terminus, and forms heterodimers with other host bZIP proteins, such as JUN and CREB/ATF (Ma et al., 2016). In addition, the N-terminus of the HBZ protein contains two LXXLL motifs that are important for its interaction with CBP/p300 (Clerc et al., 2008). Binding of HBZ to these transcription factors and histone modifiers modulates the transcription of cellular and viral genes. HBZ protein targets the Rb/E2F-1 complex and activates the transcription of E2F target genes associated with cell cycle progression and apoptosis (Kawatsuki et al., 2016). It has been shown that HBZ RNA activates the promoters of several host genes, although the precise mechanism is not yet clear (Mitobe et al., 2015). A recent study revealed that HBZ induces a bZIP protein, BATF3, and the BATF3/IRF4 complex promotes the growth of ATL cells by induction of its downstream targets, such as MYC (Nakagawa et al., 2018).
Importantly, HBZ induces the transcription of FOXP3 through the recruitment of Smad proteins and p300 to its promoter region (Satou et al., 2011; Zhao et al., 2011). Since FOXP3 is the master gene of Treg cells, HBZ-expressing cells have an immunophenotype similar to that of Treg cells. CD4+ T cells in HBZ-Tg mice expresses several surface markers of Tregs, such as CD25, cytotoxic T-lymphocyte associated protein 4 (CTLA-4), glucocorticoid-induced TNF-related protein (GITR), CCR4, and T cell immunoreceptor with Ig and ITIM domains (TIGIT) (Satou et al., 2011; Sugata et al., 2016; Yasuma et al., 2016). CCR4 and TIGIT are innately expressed in the subset of Tregs known as effector type Tregs (eTregs), which have strong suppressive function (Sugiyama et al., 2013; Joller et al., 2014). Although HBZ-expressing cells imitate the Treg phenotype, their regulatory function is impaired, since HBZ suppresses the function of FOXP3 via physical interaction (Satou et al., 2011). In Foxp3-expressing T cells in HBZ-Tg mice, the expression level of several Foxp3 target molecules, such as CD25 and CTLA-4, is lower than Treg cells in WT mice (Satou et al., 2011), indicating that dysfunctional Treg cells are induced by HBZ. In addition, those Treg-like cells of HBZ-Tg mice readily lose Foxp3 expression and produce an excessive amount of IFN-γ, which plays important roles in inflammation and lymphomagenesis induced by HBZ (Yamamoto-Taguchi et al., 2013; Mitagami et al., 2015). HBZ represses inhibitory signaling through TIGIT and PD-1, and keeps cells proliferative in response to activation of the T-cell receptor (Kinosada et al., 2017). Taken together, these observations show that HBZ promotes the differentiation of infected cells into eTreg-like CD4+ T cells that are functionally impaired. Indeed, the immunological phenotype of primary ATL cells resembles that of HBZ-Tg CD4+ T cells, and shares certain characteristics of normal Tregs, whereas the regulatory function is often lost (Karube et al., 2004; Chen et al., 2006; Satou et al., 2012). These findings suggest that HBZ fixes the immunophenotype of HTLV-1-infected cells and may accelerate oncogenic changes in this subset by growth promotion.
Distinct Roles of Tax and HBZ in Viral Persistence and Pathogenesis
As described above, Tax is transiently expressed in only a tiny fraction of MT-1 cells (Mahgoub et al., 2018). In contrast, the expression of HBZ is almost constant; and interestingly, it is contrary to that of tax in Tax-expressing MT-1 cells (Mahgoub et al., 2018). Another study, which detected those viral transcripts in HTLV-infected cell lines by RNA-FISH, also demonstrated that tax mRNA is highly expressed in cells with low HBZ expression (Billman et al., 2017). Since both Tax and HBZ are required for survival of MT-1 cells (Satou et al., 2006; Mahgoub et al., 2018), these two viral products must have collaborative roles in ATL cells. At a single-cell level, Tax-expressing cells express high levels of several anti-apoptotic and NF-κB-related genes, and Tax-negative cells are divided into two subpopulations which expressed medium or low levels of the anti-apoptotic genes. According to these experimental findings and computational simulations, it was hypothesized that transient Tax expression confers an anti-apoptotic property on the expressing cell, and that this effect lasts after Tax expression is diminished (Figure 2C). In contrast, the transcriptome profile in cells that express HBZ dominantly represents activation of the pathways associated with cell proliferation. Hence, in MT-1 cells, the alternating expression of Tax and HBZ seems to execute cooperating programs for survival and proliferation, respectively. Importantly, Tax is induced by various cytotoxic stresses, suggesting that it protects infected cells from apoptosis and triggers viral replication to increase the chance of transmission in a critical situation. The expression patterns and functions of Tax and HBZ comprise a clever strategy of HTLV-1 for persistent infection – a strategy that can also lead to ATL.
Concluding Remarks
Human T-cell leukemia virus type 1 was first discovered in 1980, and intensive studies of viral replication and cellular transformation have revealed the molecular mechanisms of HTLV-1-induced pathogenesis. Viral proteins, especially Tax and HBZ, are attractive therapeutic targets since the former is sensitive to host immunity and the latter is constantly expressed in ATL cells. Further studies are needed to develop new treatments and prophylactic strategies based on the growing knowledge of HTLV-1 biology.
Author Contributions
The author confirms being the sole contributor of this work and has approved it for publication.
Funding
This study was supported by the Friends of Leukemia Research Fund (FLRF) and the Takeda Science Foundation.
Conflict of Interest
The authors declare that the research was conducted in the absence of any commercial or financial relationships that could be construed as a potential conflict of interest.
Acknowledgments
I thank Dr. Linda Kingsbury for proofreading. I apologize, in view of space and format limitations, for failure to cover other examples and issues.
References
Billman, M. R., Rueda, D., and Bangham, C. R. M. (2017). Single-cell heterogeneity and cell-cycle-related viral gene bursts in the human leukaemia virus HTLV-1. Wellcome Open Res. 2:87. doi: 10.12688/wellcomeopenres.12469.2
Caceres, C. J., Angulo, J., Lowy, F., Contreras, N., Walters, B., Olivares, E., et al. (2018). Non-canonical translation initiation of the spliced mRNA encoding the human T-cell leukemia virus type 1 basic leucine zipper protein. Nucleic Acids Res. 46, 11030–11047. doi: 10.1093/nar/gky802
Cavanagh, M. H., Landry, S., Audet, B., Arpin-Andre, C., Hivin, P., Pare, M. E., et al. (2006). HTLV-I antisense transcripts initiating in the 3’LTR are alternatively spliced and polyadenylated. Retrovirology 3:15. doi: 10.1186/1742-4690-3-15
Chen, S., Ishii, N., Ine, S., Ikeda, S., Fujimura, T., Ndhlovu, L. C., et al. (2006). Regulatory T cell-like activity of Foxp3+ adult T cell leukemia cells. Int. Immunol. 18, 269–277. doi: 10.1093/intimm/dxh366
Clerc, I., Polakowski, N., Andre-Arpin, C., Cook, P., Barbeau, B., Mesnard, J. M., et al. (2008). An interaction between the human T cell leukemia virus type 1 basic leucine zipper factor (HBZ) and the KIX domain of p300/CBP contributes to the down-regulation of tax-dependent viral transcription by HBZ. J. Biol. Chem. 283, 23903–23913. doi: 10.1074/jbc.M803116200 [pii]
Currer, R., Van Duyne, R., Jaworski, E., Guendel, I., Sampey, G., Das, R., et al. (2012). HTLV tax: a fascinating multifunctional co-regulator of viral and cellular pathways. Front. Microbiol. 3:406. doi: 10.3389/fmicb.2012.00406
Esser, A. K., Rauch, D. A., Xiang, J., Harding, J. C., Kohart, N. A., Ross, M. H., et al. (2017). HTLV-1 viral oncogene HBZ induces osteolytic bone disease in transgenic mice. Oncotarget 8, 69250–69263. doi: 10.18632/oncotarget.20565
Etoh, K., Tamiya, S., Yamaguchi, K., Okayama, A., Tsubouchi, H., Ideta, T., et al. (1997). Persistent clonal proliferation of human T-lymphotropic virus type I-infected cells in vivo. Cancer Res. 57, 4862–4867.
Fan, J., Ma, G., Nosaka, K., Tanabe, J., Satou, Y., Koito, A., et al. (2010). APOBEC3G generates nonsense mutations in human T-cell leukemia virus type 1 proviral genomes in vivo. J. Virol. 84, 7278–7287. doi: 10.1128/JVI.02239-09 [pii]
Franchini, G., Fukumoto, R., and Fullen, J. R. (2003). T-cell control by human T-cell leukemia/lymphoma virus type 1. Int. J. Hematol. 78, 280–296. doi: 10.1007/bf02983552
Furuta, R., Yasunaga, J. I., Miura, M., Sugata, K., Saito, A., Akari, H., et al. (2017). Human T-cell leukemia virus type 1 infects multiple lineage hematopoietic cells in vivo. PLoS Pathog. 13:e1006722. doi: 10.1371/journal.ppat.1006722
Gaudray, G., Gachon, F., Basbous, J., Biard-Piechaczyk, M., Devaux, C., and Mesnard, J. M. (2002). The complementary strand of the human T-cell leukemia virus type 1 RNA genome encodes a bZIP transcription factor that down-regulates viral transcription. J. Virol. 76, 12813–12822. doi: 10.1128/jvi.76.24.12813-12822.2002
Hajj, H. E., Nasr, R., Kfoury, Y., Dassouki, Z., Nasser, R., Kchour, G., et al. (2012). Animal models on HTLV-1 and related viruses: what did we learn? Front. Microbiol. 3:333. doi: 10.3389/fmicb.2012.00333
Harhaj, E. W., and Giam, C. Z. (2018). NF-kappaB signaling mechanisms in HTLV-1-induced adult T-cell leukemia/lymphoma. FEBS J. 285, 3324–3336. doi: 10.1111/febs.14492
Ho, Y. K., Zhi, H., Bowlin, T., Dorjbal, B., Philip, S., Zahoor, M. A., et al. (2015). HTLV-1 tax stimulates ubiquitin E3 ligase, ring finger protein 8, to assemble lysine 63-linked polyubiquitin chains for TAK1 and IKK activation. PLoS Pathog. 11:e1005102. doi: 10.1371/journal.ppat.1005102
Igakura, T., Stinchcombe, J. C., Goon, P. K., Taylor, G. P., Weber, J. N., Griffiths, G. M., et al. (2003). Spread of HTLV-I between lymphocytes by virus-induced polarization of the cytoskeleton. Science 299, 1713–1716. doi: 10.1126/science.1080115
Joller, N., Lozano, E., Burkett, P. R., Patel, B., Xiao, S., Zhu, C., et al. (2014). Treg cells expressing the coinhibitory molecule TIGIT selectively inhibit proinflammatory Th1 and Th17 cell responses. Immunity 40, 569–581. doi: 10.1016/j.immuni.2014.02.012
Karube, K., Ohshima, K., Tsuchiya, T., Yamaguchi, T., Kawano, R., Suzumiya, J., et al. (2004). Expression of FoxP3, a key molecule in CD4CD25 regulatory T cells, in adult T-cell leukaemia/lymphoma cells. Br. J. Haematol. 126, 81–84. doi: 10.1111/j.1365-2141.2004.04999.x
Kashanchi, F., and Brady, J. N. (2005). Transcriptional and post-transcriptional gene regulation of HTLV-1. Oncogene 24, 5938–5951. doi: 10.1038/sj.onc.1208973
Kasugai, Y., Yoshida, N., Ohshima, K., Matsuo, K., Seto, M., and Tsuzuki, S. (2016). New mouse model of acute adult T-cell leukemia generated by transplantation of AKT. BCLxL, and HBZ-transduced T cells. Cancer Sci. 107, 1072–1078. doi: 10.1111/cas.12974
Katsuya, H., Islam, S., Tan, B. J. Y., Ito, J., Miyazato, P., Matsuo, M., et al. (2019). The Nature of the HTLV-1 Provirus in Naturally Infected Individuals Analyzed by the Viral DNA-Capture-Seq Approach. Cell Rep. 29, 724–735.e724. doi: 10.1016/j.celrep.2019.09.016
Kawatsuki, A., Yasunaga, J. I., Mitobe, Y., Green, P. L., and Matsuoka, M. (2016). HTLV-1 bZIP factor protein targets the Rb/E2F-1 pathway to promote proliferation and apoptosis of primary CD4 T cells. Oncogene 35, 4509–4517. doi: 10.1038/onc.2015.510
Kinjo, T., Ham-Terhune, J., Peloponese, J. M. Jr., and Jeang, K. T. (2010). Induction of reactive oxygen species by human T-cell leukemia virus type 1 tax correlates with DNA damage and expression of cellular senescence marker. J. Virol. 84, 5431–5437. doi: 10.1128/JVI.02460-09
Kinosada, H., Yasunaga, J. I., Shimura, K., Miyazato, P., Onishi, C., Iyoda, T., et al. (2017). HTLV-1 bZIP factor enhances T-cell proliferation by impeding the suppressive signaling of co-inhibitory receptors. PLoS Pathog. 13:e1006120. doi: 10.1371/journal.ppat.1006120
Koiwa, T., Hamano-Usami, A., Ishida, T., Okayama, A., Yamaguchi, K., Kamihira, S., et al. (2002). 5’-long terminal repeat-selective CpG methylation of latent human T-cell leukemia virus type 1 provirus in vitro and in vivo. J. Virol. 76, 9389–9397. doi: 10.1128/jvi.76.18.9389-9397.2002
Koyanagi, Y., Itoyama, Y., Nakamura, N., Takamatsu, K., Kira, J., Iwamasa, T., et al. (1993). In vivo infection of human T-cell leukemia virus type I in non-T cells. Virology 196, 25–33. doi: 10.1006/viro.1993.1451
Kulkarni, A., Mateus, M., Thinnes, C. C., McCullagh, J. S., Schofield, C. J., Taylor, G. P., et al. (2017). Glucose metabolism and oxygen availability govern reactivation of the latent human retrovirus HTLV-1. Cell Chem. Biol. 24, 1377–1387e1373. doi: 10.1016/j.chembiol.2017.08.016
Kurihara, K., Harashima, N., Hanabuchi, S., Masuda, M., Utsunomiya, A., Tanosaki, R., et al. (2005). Potential immunogenicity of adult T cell leukemia cells in vivo. Int. J. Cancer 114, 257–267. doi: 10.1002/ijc.20737
Kwon, H., Ogle, L., Benitez, B., Bohuslav, J., Montano, M., Felsher, D. W., et al. (2005). Lethal cutaneous disease in transgenic mice conditionally expressing type I human T cell leukemia virus Tax. J. Biol. Chem. 280, 35713–35722. doi: 10.1074/jbc.M504848200
Ma, G., Yasunaga, J., and Matsuoka, M. (2016). Multifaceted functions and roles of HBZ in HTLV-1 pathogenesis. Retrovirology 13:16. doi: 10.1186/s12977-016-0249-x
Mahgoub, M., Yasunaga, J. I., Iwami, S., Nakaoka, S., Koizumi, Y., Shimura, K., et al. (2018). Sporadic on/off switching of HTLV-1 Tax expression is crucial to maintain the whole population of virus-induced leukemic cells. Proc. Natl. Acad. Sci. U.S.A. 115, E1269–E1278. doi: 10.1073/pnas.1715724115
Manns, A., Miley, W. J., Wilks, R. J., Morgan, O. S., Hanchard, B., Wharfe, G., et al. (1999). Quantitative proviral DNA and antibody levels in the natural history of HTLV-I infection. J. Infect. Dis. 180, 1487–1493. doi: 10.1086/315088
Matsuoka, M., and Jeang, K. T. (2007). Human T-cell leukaemia virus type 1 (HTLV-1) infectivity and cellular transformation. Nat. Rev. Cancer 7, 270–280. doi: 10.1038/nrc2111 [pii]
Matsuoka, M., and Yasunaga, J. I. (2013). Human T-cell leukemia virus type 1: replication, proliferation and propagation by Tax and HTLV-1 bZIP factor. Curr. Opin. Virol. 13, 146–156. doi: 10.1016/j.coviro.2013.08.010
Mitagami, Y., Yasunaga, J., Kinosada, H., Ohshima, K., and Matsuoka, M. (2015). Interferon-gamma promotes inflammation and development of T-cell lymphoma in HTLV-1 bZIP factor transgenic mice. PLoS Pathog. 11:e1005120. doi: 10.1371/journal.ppat.1005120
Mitobe, Y., Yasunaga, J., Furuta, R., and Matsuoka, M. (2015). HTLV-1 bZIP factor RNA and protein impart distinct functions on T-cell proliferation and survival. Cancer Res. 75, 4143–4152. doi: 10.1158/0008-5472.CAN-15-0942
Miura, M., Miyazato, P., Satou, Y., Tanaka, Y., and Bangham, C. R. M. (2018). Epigenetic changes around the pX region and spontaneous HTLV-1 transcription are CTCF-independent. Wellcome Open Res. 3:105. doi: 10.12688/wellcomeopenres.14741.2
Miyazaki, M., Yasunaga, J., Taniguchi, Y., Tamiya, S., Nakahata, T., and Matsuoka, M. (2007). Preferential selection of human T-cell leukemia virus type 1 provirus lacking the 5’ long terminal repeat during oncogenesis. J. Virol. 81, 5714–5723. doi: 10.1128/JVI.02511-06
Miyazato, P., and Matsuoka, M. (2014). Human T-cell leukemia virus type 1 and Foxp3 expression: viral strategy in vivo. Int. Immunol. 26, 419–425. doi: 10.1093/intimm/dxu048
Murata, K., Hayashibara, T., Sugahara, K., Uemura, A., Yamaguchi, T., Harasawa, H., et al. (2006). A novel alternative splicing isoform of human T-cell leukemia virus type 1 bZIP factor (HBZ-SI) targets distinct subnuclear localization. J. Virol. 80, 2495–2505. doi: 10.1128/JVI.80.5.2495-2505.2006
Nakagawa, M., Shaffer, A. L. lll, Ceribelli, M., Zhang, M., Wright, G., Huang, D. W., et al. (2018). Targeting the HTLV-I-regulated BATF3/IRF4 transcriptional network in adult T cell leukemia/lymphoma. Cancer Cell 34, 286–297.e210. doi: 10.1016/j.ccell.2018.06.014
Okayama, A., Stuver, S., Iga, M., Okamoto, M., Mueller, N., Matsuoka, M., et al. (2001). Sequential change of virus markers in seroconverters with community-acquired infection of human T lymphotropic virus type I. J. Infect. Dis. 183, 1031–1037. doi: 10.1086/319282
Robek, M. D., and Ratner, L. (1999). Immortalization of CD4(+) and CD8(+) T lymphocytes by human T-cell leukemia virus type 1 Tax mutants expressed in a functional molecular clone. J. Virol. 73, 4856–4865.
Rushing, A. W., Hoang, K., Polakowski, N., and Lemasson, I. (2018). The Human T-Cell Leukemia virus type 1 basic leucine zipper factor attenuates repair of double-stranded DNA breaks via nonhomologous end joining. J. Virol. 92, 672–618. doi: 10.1128/JVI.00672-18
Sasaki, H., Nishikata, I., Shiraga, T., Akamatsu, E., Fukami, T., Hidaka, T., et al. (2005). Overexpression of a cell adhesion molecule, TSLC1, as a possible molecular marker for acute-type adult T-cell leukemia. Blood 105, 1204–1213. doi: 10.1182/blood-2004-03-1222
Satou, Y., Miyazato, P., Ishihara, K., Yaguchi, H., Melamed, A., Miura, M., et al. (2016). The retrovirus HTLV-1 inserts an ectopic CTCF-binding site into the human genome. Proc. Natl. Acad. Sci. U.S.A. 113, 3054–3059. doi: 10.1073/pnas.1423199113
Satou, Y., Utsunomiya, A., Tanabe, J., Nakagawa, M., Nosaka, K., and Matsuoka, M. (2012). HTLV-1 modulates the frequency and phenotype of FoxP3+CD4+ T cells in virus-infected individuals. Retrovirology 9:46. doi: 10.1186/1742-4690-9-46
Satou, Y., Yasunaga, J., Yoshida, M., and Matsuoka, M. (2006). HTLV-I basic leucine zipper factor gene mRNA supports proliferation of adult T cell leukemia cells. Proc. Natl. Acad. Sci. U.S.A. 103, 720–725. doi: 10.1073/pnas.0507631103
Satou, Y., Yasunaga, J., Zhao, T., Yoshida, M., Miyazato, P., Takai, K., et al. (2011). HTLV-1 bZIP factor induces T-cell lymphoma and systemic inflammation in vivo. PLoS Pathog. 7:e1001274. doi: 10.1371/journal.ppat.1001274
Shibata, Y., Tokunaga, F., Goto, E., Komatsu, G., Gohda, J., Saeki, Y., et al. (2017). HTLV-1 tax induces formation of the active macromolecular IKK complex by generating Lys63- and Met1-linked hybrid polyubiquitin chains. PLoS Pathog. 13:e1006162. doi: 10.1371/journal.ppat.1006162
Shudofsky, A. M. D., and Giam, C. Z. (2019). Cells of adult T-cell leukemia evade HTLV-1 Tax/NF-kappaB hyperactivation-induced senescence. Blood Adv. 3, 564–569. doi: 10.1182/bloodadvances.2018029322
Sugata, K., Yasunaga, J., Kinosada, H., Mitobe, Y., Furuta, R., Mahgoub, M., et al. (2016). HTLV-1 Viral Factor HBZ Induces CCR4 to Promote T-cell Migration and Proliferation. Cancer Res. 76, 5068–5079. doi: 10.1158/0008-5472.CAN-16-0361
Sugiyama, D., Nishikawa, H., Maeda, Y., Nishioka, M., Tanemura, A., Katayama, I., et al. (2013). Anti-CCR4 mAb selectively depletes effector-type FoxP3+CD4+ regulatory T cells, evoking antitumor immune responses in humans. Proc. Natl. Acad. Sci. U.S.A. 110, 17945–17950. doi: 10.1073/pnas.1316796110
Sun, S. C., and Yamaoka, S. (2005). Activation of NF-kappaB by HTLV-I and implications for cell transformation. Oncogene 24, 5952–5964. doi: 10.1038/sj.onc.1208969
Takeda, S., Maeda, M., Morikawa, S., Taniguchi, Y., Yasunaga, J., Nosaka, K., et al. (2004). Genetic and epigenetic inactivation of tax gene in adult T-cell leukemia cells. Int. J. Cancer 109, 559–567. doi: 10.1002/ijc.20007
Takiuchi, Y., Kobayashi, M., Tada, K., Iwai, F., Sakurada, M., Hirabayashi, S., et al. (2017). HTLV-1 bZIP factor suppresses TDP1 expression through inhibition of NRF-1 in adult T-cell leukemia. Sci. Rep. 7:12849. doi: 10.1038/s41598-017-12924-0
Tanaka, G., Okayama, A., Watanabe, T., Aizawa, S., Stuver, S., Mueller, N., et al. (2005). The clonal expansion of human T lymphotropic virus type 1-infected T cells: a comparison between seroconverters and long-term carriers. J. Infect. Dis. 191, 1140–1147. doi: 10.1086/428625
Tanaka-Nakanishi, A., Yasunaga, J., Takai, K., and Matsuoka, M. (2014). HTLV-1 bZIP factor suppresses apoptosis by attenuating the function of FoxO3a and altering its localization. Cancer Res. 74, 188–200. doi: 10.1158/0008-5472.CAN-13-0436
Taniguchi, Y., Nosaka, K., Yasunaga, J., Maeda, M., Mueller, N., Okayama, A., et al. (2005). Silencing of human T-cell leukemia virus type I gene transcription by epigenetic mechanisms. Retrovirology 2:64. doi: 10.1186/1742-4690-2-64
Usui, T., Yanagihara, K., Tsukasaki, K., Murata, K., Hasegawa, H., Yamada, Y., et al. (2008). Characteristic expression of HTLV-1 basic zipper factor (HBZ) transcripts in HTLV-1 provirus-positive cells. Retrovirology 5:34. doi: 10.1186/1742-4690-5-34
Vernin, C., Thenoz, M., Pinatel, C., Gessain, A., Gout, O., Delfau-Larue, M. H., et al. (2014). HTLV-1 bZIP factor HBZ promotes cell proliferation and genetic instability by activating OncomiRs. Cancer Res. 74, 6082–6093. doi: 10.1158/0008-5472.CAN-13-3564
Wing, J. B., Tanaka, A., and Sakaguchi, S. (2019). Human FOXP3(+) regulatory T Cell heterogeneity and function in autoimmunity and cancer. Immunity 50, 302–316. doi: 10.1016/j.immuni.2019.01.020
Wurm, T., Wright, D. G., Polakowski, N., Mesnard, J. M., and Lemasson, I. (2012). The HTLV-1-encoded protein HBZ directly inhibits the acetyl transferase activity of p300/CBP. Nucleic Acids Res. 40, 5910–5925.
Yamamoto-Taguchi, N., Satou, Y., Miyazato, P., Ohshima, K., Nakagawa, M., Katagiri, K., et al. (2013). HTLV-1 bZIP factor induces inflammation through Labile Foxp3 expression. PLoS Pathog. 9:e1003630. doi: 10.1371/journal.ppat.1003630
Yasuma, K., Yasunaga, J., Takemoto, K., Sugata, K., Mitobe, Y., Takenouchi, N., et al. (2016). HTLV-1 bZIP factor impairs anti-viral immunity by inducing co-inhibitory molecule, T cell immunoglobulin and ITIM domain (TIGIT). PLoS Pathog. 12:e1005372. doi: 10.1371/journal.ppat.1005372
Yasunaga, J., and Matsuoka, M. (2011). Molecular mechanisms of HTLV-1 infection and pathogenesis. Int. J. Hematol. 94, 435–442. doi: 10.1007/s12185-011-0937-1
Yasunaga, J., Sakai, T., Nosaka, K., Etoh, K., Tamiya, S., Koga, S., et al. (2001). Impaired production of naive T lymphocytes in human T-cell leukemia virus type I-infected individuals: its implications in the immunodeficient state. Blood 97, 3177–3183.
Yoshida, M., Satou, Y., Yasunaga, J., Fujisawa, J., and Matsuoka, M. (2008). Transcriptional control of spliced and unspliced human T-cell leukemia virus type 1 bZIP factor (HBZ) gene. J. Virol. 82, 9359–9368. doi: 10.1128/JVI.00242-08
Yoshie, O., Fujisawa, R., Nakayama, T., Harasawa, H., Tago, H., Izawa, D., et al. (2002). Frequent expression of CCR4 in adult T-cell leukemia and human T-cell leukemia virus type 1-transformed T cells. Blood 99, 1505– 1511.
Zhao, T., Satou, Y., Sugata, K., Miyazato, P., Green, P. L., Imamura, T., et al. (2011). HTLV-1 bZIP factor enhances TGF-beta signaling through p300 coactivator. Blood 118, 1865–1876.
Zhao, T., Yasunaga, J., Satou, Y., Nakao, M., Takahashi, M., Fujii, M., et al. (2009). Human T-cell leukemia virus type 1 bZIP factor selectively suppresses the classical pathway of NF-kappaB. Blood 113, 2755–2764.
Keywords: human T-cell leukemia virus type 1, Tax, HTLV-1 bZIP factor, adult T-cell leukemia-lymphoma, Foxp3
Citation: Yasunaga J (2020) Strategies of Human T-Cell Leukemia Virus Type 1 for Persistent Infection: Implications for Leukemogenesis of Adult T-Cell Leukemia-Lymphoma. Front. Microbiol. 11:979. doi: 10.3389/fmicb.2020.00979
Received: 10 March 2020; Accepted: 23 April 2020;
Published: 19 May 2020.
Edited by:
Toshiki Watanabe, The University of Tokyo, JapanReviewed by:
Jun-ichi Fujisawa, Kansai Medical University, JapanIsabelle Lemasson, East Carolina University, United States
Copyright © 2020 Yasunaga. This is an open-access article distributed under the terms of the Creative Commons Attribution License (CC BY). The use, distribution or reproduction in other forums is permitted, provided the original author(s) and the copyright owner(s) are credited and that the original publication in this journal is cited, in accordance with accepted academic practice. No use, distribution or reproduction is permitted which does not comply with these terms.
*Correspondence: Jun-ichirou Yasunaga, jyasunag@kumamoto-u.ac.jp