- 1Department of Laboratory Medicine, Research Institute of Bacterial Resistance, Yonsei University College of Medicine, Seoul, South Korea
- 2Department of Laboratory Medicine, College of Medicine, Hallym University, Chuncheon, South Korea
- 3Department of Veterinary Internal Medicine, College of Veterinary Medicine, Konkuk University, Seoul, South Korea
- 4Division of Biotechnology, College of Environmental and Bioresource Sciences, Chonbuk National University, Iksan, South Korea
This study aimed to describe the distribution and characterization of fecal extended-spectrum β-lactamase (ESBL)- and AmpC-producing Escherichia coli isolates from healthy companion animals and cohabiting humans. A total of 968 rectal swab samples from 340 participants, including healthy companion animals and cohabiting humans, were collected from 130 households in South Korea from 2018 to 2019. To determine the bacterial profiles of the participants, several experiments were performed as follows: antimicrobial susceptibility testing, PCR and direct sequencing for ESBL/AmpC production, PFGE, MLST, whole genome sequencing and qRT-PCR. A total of 24.9 and 21.5% of the E. coli isolates from healthy companion animals and cohabiting humans were ESBL/AmpC producers, respectively. The blaCTX–M–14 gene was the most prevalent ESC resistance gene in both pets (n = 25/95, 26.3%) and humans (n = 44/126, 34.9%). The blaCMY–2 gene was also largely detected in pets (n = 19, 20.0%). Overall, intrahousehold pet-human sharing of ESBL/AmpC E. coli isolates occurred in 4.8% of households, and the isolates were all CTX-M-14 producers. In particular, ten CMY-2-producing E. coli isolates from seven dogs and three humans in the different households belonged to the same pulsotype. The MIC values of cefoxitin and the transcription level in CMY-2-producing E. coli isolates were proportional to the blaCMY–2 copy number on the chromosome. Our results showed that the clonal spread of fecal ESBL/AmpC-producing E. coli households’ isolates between healthy companion animals and cohabiting humans was rare, but it could happen. In particular, E. coli ST405 isolates carrying multiple blaCMY–2 genes on the chromosome was sporadically spread between companion animals and humans in South Korea.
Introduction
The close contact between companion animals and humans may cause bacterial transmission across animals or humans by horizontal transfer (indirect transmission) and clonal spread (direct transmission) (Damborg et al., 2016). The majority of studies have focused on the transmission of human-to-companion animal with hospitalized or infected (Bogaerts et al., 2015), but few studies have focused on the fecal transmission of bacteria between healthy companion animals and cohabiting humans as categorized households (Marques et al., 2019).
Since the late 1900s, third- and fourth-generation extended-spectrum cephalosporin (ESC)-resistant Enterobacteriaceae have been frequently reported in both human and veterinary fields (Schmiedel et al., 2014; Lee et al., 2018). ESC resistance in Enterobacteriaceae is often associated with extended-spectrum β-lactamase (ESBL) and AmpC β-lactamase production, mainly CTX-M-type for ESBL in Enterobacteriaceae, CMY-type for AmpC in Escherichia coli, and DHA-type for AmpC in Klebsiella pneumoniae (Lartigue et al., 2004; Verdet et al., 2009; Kameyama et al., 2013). In addition, a wide diversity of carbapenemase-producing Enterobacteriaceae has been also found worldwide (Gauthier et al., 2018).
CMY-2 is the most prevalent AmpC β-lactamase in ESC-resistant E. coli isolates in animals and humans worldwide (Ewers et al., 2012; Lazarus et al., 2015). The dissemination of the blaCMY–2 gene largely corresponds to self-transferable IncA/C or IncI1 plasmids (Ben Sallem et al., 2014; Guo et al., 2014), but chromosomal blaCMY–2 gene transfer through specific ISEcp1-mediated transposition is also responsible for the spread of the blaCMY–2 gene (Verdet et al., 2009; Mata et al., 2012). The transcription of the blaCMY–2 gene could be altered by a putative promoter within ISEcp1, the correlation between ISEcp1 and CMY-2 called the spacer sequence, and the copy number of the blaCMY–2 gene (Smet et al., 2008; Ma et al., 2011; Kurpiel and Hanson, 2012; Manageiro et al., 2015).
Our previous study targeted “diseased” companion animals (Hong et al., 2019), while this study focused on “healthy” companion animals. Namely, the significant difference between our two studies is the study population. This study aimed to compare the distribution and molecular relatedness of fecal ESBL/AmpC-producing E. coli isolates collected from healthy companion animals and cohabiting humans.
Materials and Methods
Study Population
The study population included households with healthy companion animals and humans living together. All human participants were informed of the main goals of this study and were asked to sign a consent form before inclusion. Participants who were considered “healthy” were included if they had no bacterial infection or did not take antibiotics in the previous month. A participant household was made up of healthy companion animals (a dog or a cat) and one or two cohabiting humans (a veterinarian hospital staff and/or a staff’s family). A total of 340 participants (101 dogs, 38 cats, 130 vet’s staff, and 71 staff’s families) living in 130 households belonging to 18 veterinarian hospitals were enrolled for 2018–2019 (Supplementary Material). All human participants were more than 16 years old, and 70% were women. The age of companion animals ranged from less than 1 to 16 years, and 43% were female (data not shown).
Specimen Collection and Bacterial Isolates
This study was carried out in accordance with the recommendations of the ethnic guidelines of KonKuk University College of Veterinary Medicine, South Korea. Individual written informed consent for the use of samples was obtained from all the vet’s staff and the staff’s families.
Enrolled humans collected their own rectal swab samples and rectal swab samples from coliving companion animals into sterile containers. The sample collection was performed with one, two, three, four, and/or five repetitions (first in January 2018, second in March 2018, third in September 2018, fourth in February 2019, and/or fifth in April 2019) per participant (Supplementary Material). Specimens were refrigerated until processing. The rectal swab samples plated onto CHROMagar ESBL plates (CHROMagar, Paris, France) were incubated at 36°C for 18 h. Up to three suspected E. coli colonies (dark pink to reddish colonies) were isolated, and a single colony was subcultured onto a blood agar plate. The subcultured colonies were confirmed as E. coli by matrix-assisted laser desorption ionization-time of flight mass spectrometry (MALDI-TOF MS) with a Vitek-MS (bioMerieux, Marcy-Etoile, France).
Antimicrobial Susceptibility Testing
Antimicrobial susceptibility to ampicillin, piperacillin, ampicillin-sulbactam, cefazolin, cefoxitin, cefotaxime, ceftazidime, cefepime, aztreonam, ertapenem, imipenem, meropenem, amikacin, gentamicin, ciprofloxacin, and trimethoprim-sulfamethoxazole was tested by the agar disk diffusion method on cation-adjusted Mueller-Hinton agar (Difco Laboratories, Detroit, MI, United States). For further characterization, the minimum inhibitory concentrations (MICs) of cefoxitin, cefotaxime, and ceftazidime were determined by the broth microdilution method according to the CLSI guidelines (CLSI, 2019), and the MICs of colistin were assessed by the broth microdilution method using Mueller–Hinton broth (Difco Laboratories), following the criteria of EUCAST (2019). E. coli ATCC 25922 and Pseudomonas aeruginosa ATCC 27853 were used for quality control.
Identification of Antimicrobial Resistance Determinants and the blaCMY–2 Promoter
Template DNA was prepared by the boiling method. blaCTX–M–1–like, blaCTX–M–2–like, blaCTX–M–9–like, and blaCTX–M–25–like for CTX-M-type ESBLs were amplified by PCR and direct sequencing in cefotaxime-non-susceptible E. coli isolates; blaDHA, blaACC, blaACT, and blaFOX for AmpCs were amplified by PCR and direct sequencing in cefoxitin-non-susceptible E. coli isolates; IMP-, VIM-, NDM-, KPC-, GES-, and OXA-48-like for carbapenemases were amplified by PCR and direct sequencing in carbapenem-non-susceptible E. coli isolates: 1138 bp region upstream of the blaCMY–2 gene within ISEcp1 and the 116 bp spacer sequence as the predicted blaCMY–2 promoter between ISEcp1 and CMY-2 for blaCMY–2 promoter as described previously (Smet et al., 2008; Ma et al., 2011; Kurpiel and Hanson, 2012; Manageiro et al., 2015; Hong et al., 2019). The sequences were compared to published DNA sequences using BLAST1.
Epidemiological Bacterial Typing
The representative E. coli isolates carrying the five predominant ESBL/AmpC genes for epidemiological data analysis underwent pulsed-field gel electrophoresis (PFGE) with XbaI. E. coli ATCC 25922 was included as a quality control. Multilocus sequence typing (MLST) was performed on CMY-2-producing E. coli isolates belonged to the same pulsotype.
Whole Genome Sequencing
The three CMY-2 producing E. coli ST405 isolates (E118, E597, and E686) among the same pulsotype were selected. Two (E597 and E686) of the three isolates were recovered from a dog and a human in this study, respectively. The other well-characterized isolate (E118) was recovered from a dog in our previous study in 2017 (Hong et al., 2019). The bacterial DNA was extracted from the three isolates using a Wizard Genomic DNA Purification kit (Promega, Madison, WI, United States). After extraction, DNA shearing was performed using a g-TUBE apparatus (Covaris, Inc., Moburn, MA, United States) and the fragments were purified by using 0.45× of the final volume of washed Agencourt AMPure XP magnetic beads (Beckman Coulter Inc., Brea, CA, United States) (Hong et al., 2016). For bacterial whole genome sequencing (WGS), single-molecule real-time sequencing was carried out on a PacBio RSII instrument (Pacific Biosciences, Menlo Park, CA, United States). The coding sequences, tRNA sequences, and rRNA sequences were annotated using the NCBI Prokaryotic Genome Annotation Pipeline2.
Quantitative Reverse Transcription PCR
The expression level of the blaCMY–2 gene in E. coli ST405 isolates belonged to the same pulsotype was evaluated by quantitative reverse transcription PCR (qRT-PCR) assays. The E. coli isolates were cultured in Muller–Hinton broth at 37°C with vigorous agitation until OD600 = 1.5–2, corresponding to the exponential phase. Bacterial RNA was extracted using the RNeasy Mini Kit (Qiagen, Hilden, Germany), and cDNA was synthesized using the TOPscriptTM cDNA Synthesis Kit (Enzynomics, Daejeon, South Korea). Amplification was carried out in a final volume of 20 μL containing 10 μL of premix (RbTaqTM qPCR 2X preMIX; Enzynomics), 1 μL of each primer, 7 μL of distilled water, and 1 μL of cDNA with a CFX96 system (Bio-Rad, Hercules, CA, United States). Transcript measurement was carried out in quadruplicate, and relative quantification of the blaCMY–2 gene was calculated with the 2–Δ Δ CT method using 16S rRNA as a reference. The E118 strain was measured in every batch for comparison as a reference strain.
Data Analysis
For PFGE, the unweighted-pair group method with averaging based on the Dice similarity coefficient and a clustering algorithm was used to create a dendrogram. Isolates with >80% PFGE similarity were considered as shared clones (Tenover et al., 1995). Statistical analysis was performed using SPSS Statistics version 24.0.0 (IBM Corp., Armonk, NY, United States). The chi-square test was used for comparisons between groups, with a p value of less than 0.05 considered statistically significant.
Results
ESBL/AmpC Genotypes and Antimicrobial Susceptibility
A total of 968 rectal swab samples from healthy companion animals (291 dogs and 91 cats) and their cohabiting humans (381 vet’s staff and 205 of staff’s families) were collected from 340 participants (Supplementary Table S1).
A total of 221 ESBL/AmpC-producing E. coli isolates, including 95 (24.9%, 95/382) healthy companion animals and 126 (21.5%, 126/586) cohabiting humans in the 130 households, were isolated. CTX-M-14 (n = 25, 26.3%) was the most common ESBL/AmpC β-lactamase in companion animals. CMY-2 (n = 19, 20.0%) was the second most predominant ESBL/AmpC β-lactamase, followed by CTX-M-55 (n = 12, 12.6%), CTX-M-15 (n = 9, 9.5%), and CTX-M-27 (n = 7, 7.4%). The blaCTX–M–14 gene (n = 44, 34.9%) was also the most common ESC-resistance gene in cohabiting humans, followed by blaCTX–M–15 (n = 24, 19.0%), blaCTX–M–27 (n = 21, 16.7%), blaCTX–M–55 (n = 8, 6.3%), and blaCMY–2 (n = 8, 6.3%). CTX-M-1 and CTX-M-24 were found only in companion animals, while CTX-M-3, CTX-M-65, and CMY-48 were detected only in humans. Several E. coli isolates cocarrying ESBL/AmpC genes (producing ESBL + ESBL, ESBL + AmpC, or AmpC + AmpC) were also observed (Table 1). Carbapenemases (IMP-, VIM-, NDM-, KPC-, GES-, and OXA-48-like) were not detected in two imipenem-intermediate E. coli isolates (H7h3) along with CTX-M-15 and DHA-1 recovered from vet’s staff and staff’s family.
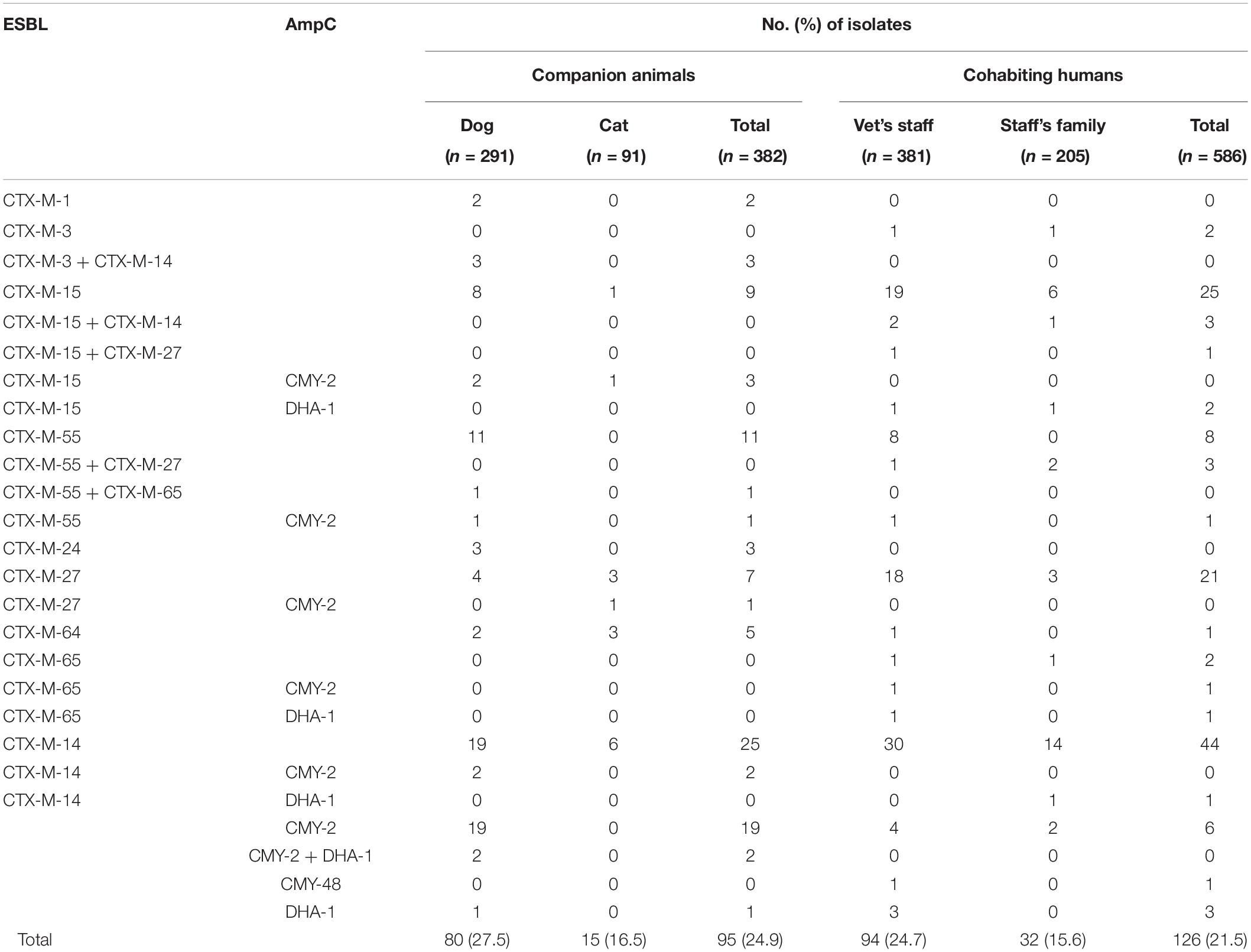
Table 1. Genotypes of ESBL- and AmpC-producing E. coli isolates from healthy companion animals and cohabiting humans.
The ESBL/AmpC-producing E. coli isolates in pets showed a statistically lower susceptibility rate to ampicillin-sulbactam, cefoxitin, ceftazidime, cefepime, aztreonam, gentamicin, and ciprofloxacin than those in cohabiting humans but showed a statistically higher susceptibility rate to trimethoprim-sulfamethoxazole than those in cohabiting humans (p value <0.05). All isolates in companion animals and cohabiting humans were susceptible to colistin (Figure 1).
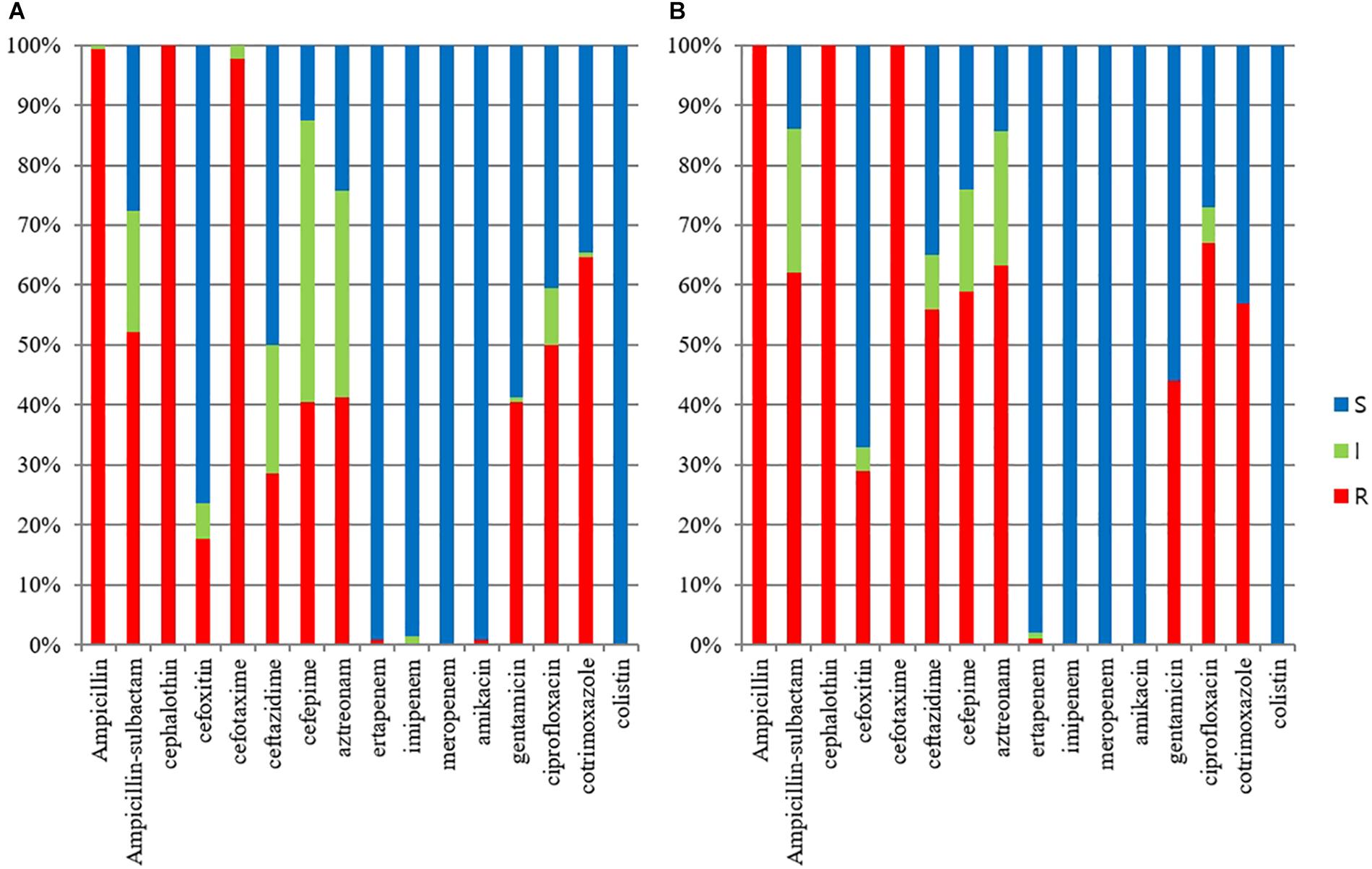
Figure 1. Antimicrobial susceptibilities of ESBL/AmpC-producing E. coli veterinary isolates. (A) E. coli isolates from humans (n = 125); (B) E. coli isolates from companion animals (n = 95).
Epidemiological Relatedness Categorized in Households
A total of 130 non-duplicated E. coli isolates carrying the five predominant ESBL/AmpC genes (49 of CTX-M-14, 25 of CTX-M-15, 15 of CTX-M-27, 16 of CTX-M-55, and 25 of CMY-2) in the 84 households were selected for subjecting to PFGE analysis.
Of the 49 E. coli isolates carrying the blaCTX–M–14 gene, 13 cases were considered as shared clones belonged to the same pulsotype. Four cases in four households occurred as intrahousehold pet-human sharing (H9h4, H1h11, H6h6, and H7h16), indicating belonged to same household and same veterinary hospital with >80% clonal similarity. Seven cases occurred as interhousehold pet-human sharing (Figure 2A).
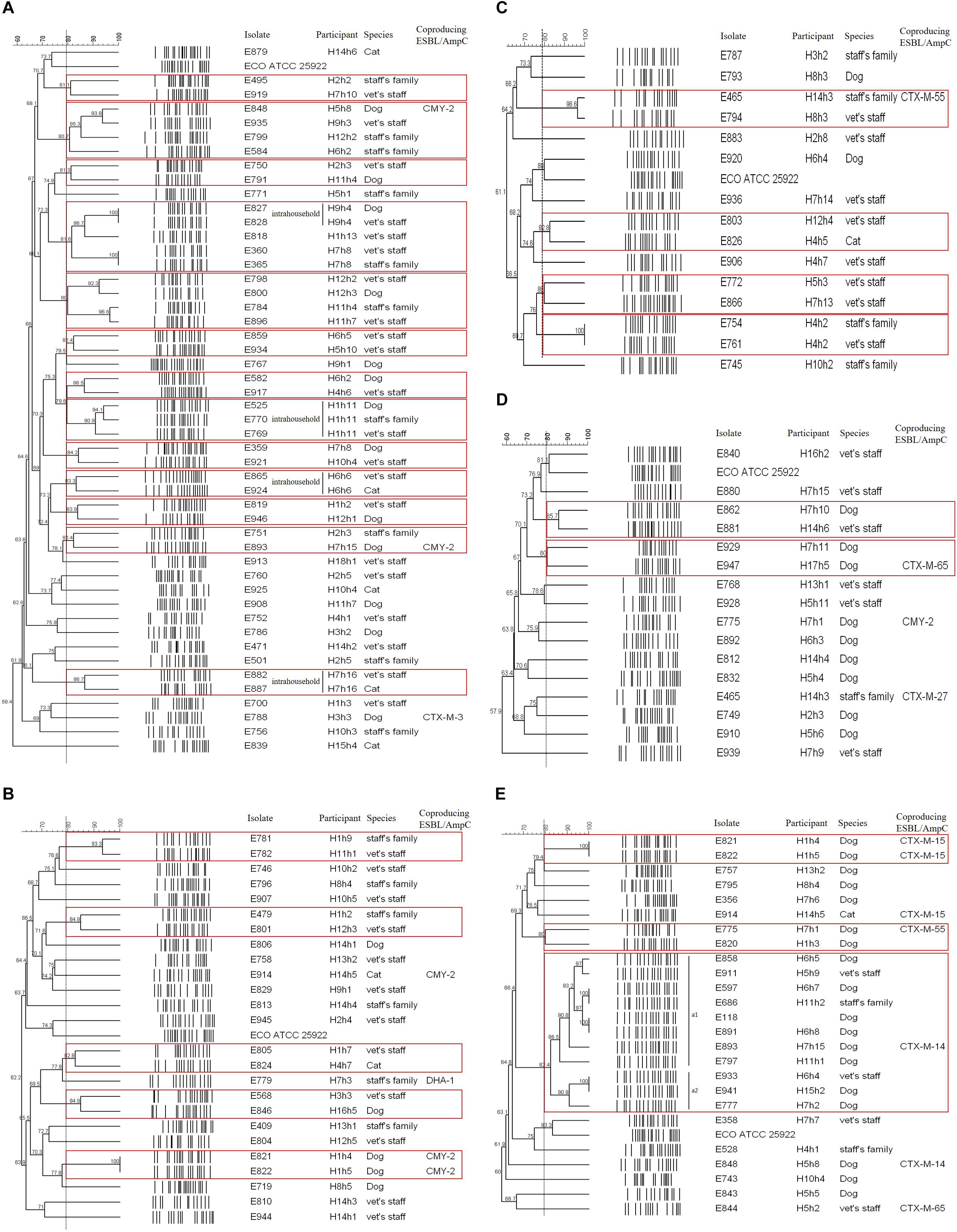
Figure 2. PFGE dendrogram of ESBL/AmpC-producing E. coli isolates by genotype. (A) CTX-M-14; (B) CTX-M-15; (C) CTX-M-27; (D) CTX-M-55; (E) CMY-2. The red box indicates the same pulsotype (>80% similarity) as the closely related case.
Of the 25 E. coli isolates carrying the blaCTX–M–15 gene, five cases had closed bacterial strain typing (cladogram sharing >82%), and intrahousehold pet-human sharing was absent. Two cases of them were observed: interhousehold dog-human sharing and interhousehold dog-dog sharing (Figure 2B).
Of the 15 CTX-M-27-producing E. coli isolates, four cases showed shared clones without intrahousehold pet-human sharing. One case of them was observed as interhousehold cat-human sharing (Figure 2C).
Of the 16 E. coli isolates carrying the blaCTX–M–55 gene, two cases were considered as shared clones without intrahousehold pet-human sharing, but each case occurred as interhousehold dog-human sharing and interhousehold dog-dog sharing (Figure 2D).
Of the 25 E. coli isolates carrying the blaCMY–2 gene, three cases showed closed bacterial strain typing (cladogram sharing >80%) without intrahousehold pet-human sharing. Two cases of them were occurred as interhousehold dog-dog sharing. In the remaining one case, eleven E. coli isolates (eight dogs and three humans from different households) corresponded to ST405 by MLST analysis were included in one cluster (a) by PFGE (pulsotype a1 (six dogs and two humans) with >86% clonal similarity and pulsotype a2 (two dogs and one human) with >90% clonal similarity), similar to the well-characterized E118 strain (E. coli ST405 canine isolate carrying the blaCMY–2 gene) in our previous report (Hong et al., 2019; Figure 2E).
Overall, intrahousehold pet-human sharing was 4.8% (four of the 84 households), and these shared isolates were all CTX-M-14 producers.
Genetic Profiles of CMY-2-Producing E. coli ST405 Isolates
The bacterial profile was further characterized, and 11 CMY-2-producing E. coli ST405 isolates belonged to the a1 and a2 pulsotypes were divided into three groups based on their MIC values to cefoxitin: 256 mg/L was included in group 1, 128 mg/L was included in group 2, and 64 mg/L was included in group 3 (Table 2).
Three isolates (E118, E597, and E686) were selected from each of the three groups, and WGS was carried out. The E597 strain (dog origin) in group 1 was composed of a 5.2 Mb circularized chromosome carrying three copies of blaCMY–2 and two plasmids. The first plasmid of the RepFIB type had 176 CDSs, including only the TEM-1 gene in ca. 14.4 kb. The second repA-type plasmid had 93 CDSs without the antimicrobial resistance (AMR) gene in ca. 8.3 kb. The E118 strain (dog origin) in group 2 was composed of a 5.3 Mb circularized chromosome carrying two copies of blaCMY–2 and one plasmid. The RepFIB replicon plasmid had 175 CDSs, including TEM-1 and tet for tetracycline resistance in ca. 14 kb. The E686 strain (human origin) in group 3 was composed of a 5.2 Mb circularized chromosome carrying one blaCMY–2 and one plasmid. The plasmid of the RepFIB type had 191 CDSs, which included TEM-1, tet, and ant1, for streptomycin resistance in ca. 14.4 kb (Figure 3).
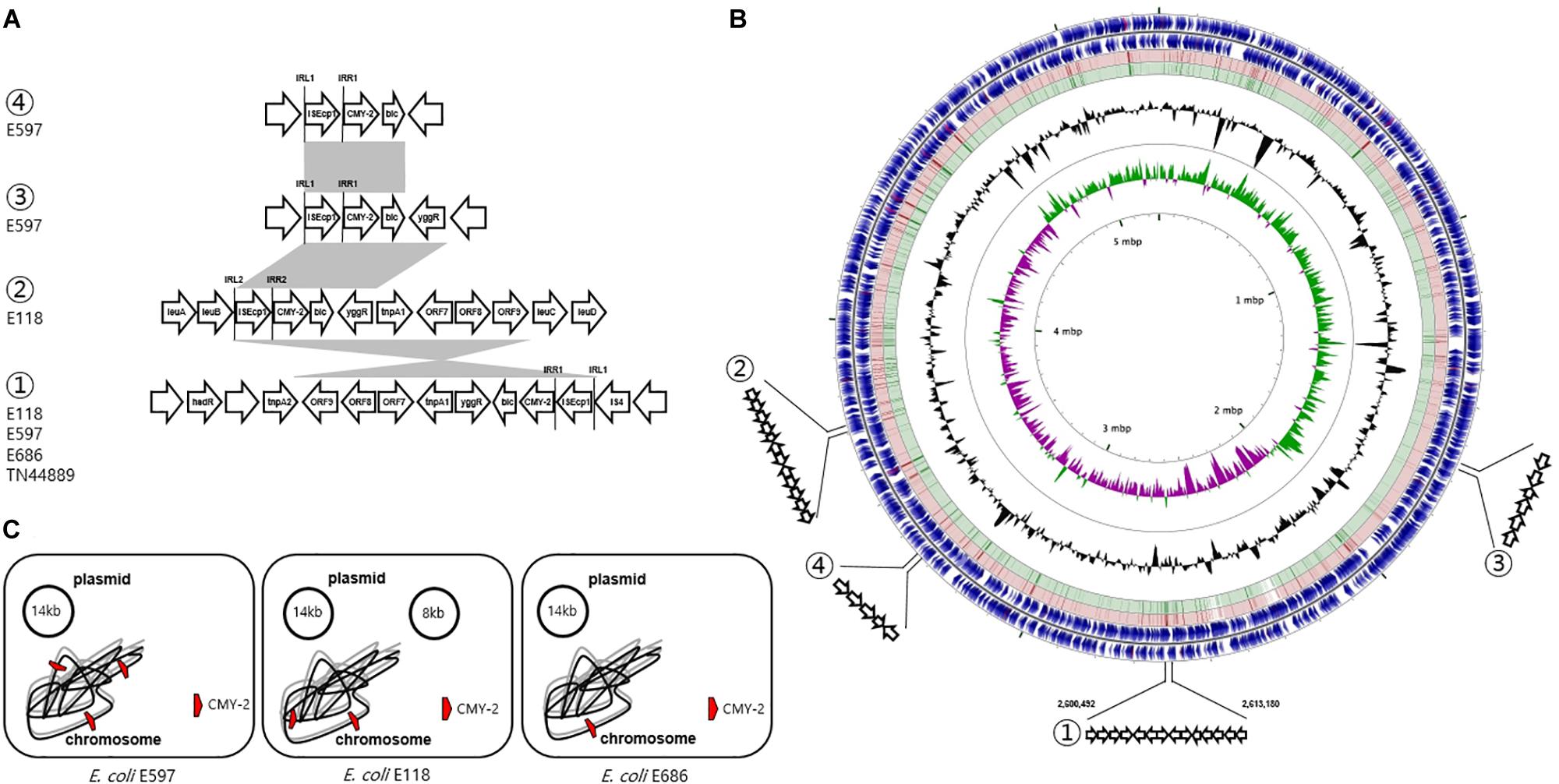
Figure 3. Genetic overview of three E. coli ST405 isolates carrying chromosomally located blaCMY–2. (A) Comparison of the genetic regions surrounding the blaCMY–2 gene. The shaded regions indicate the identical sequences sharing whatever same or converse orientation. (B) Circular genome map representation. The outermost ring: E118 open-reading frames on both forward and reverse strands. Red and green circle represents the blast results of E597 and E686 with E118 representing the positions covered by the BLASTN alignment, respectively. G + C content, black peak; G + C positive skew, green peak; G + C negative skew, purple peak. ① Region of genetic environmental structure from 2,600,492-2,613,180 bp in the E118, E597, and E686 strains identical to E. coli TN44889; ② region from 3,350,844-3,353,575 bp in E118 strain; ③ region from 1,669,367-1,672,220 bp in E597, and ④ region from 3,350,844-3,353,575 bp in E597 strain. (C) The chromosome and plasmid of three CMY-2-producing E. coli isolates.
The genetic environment of the first blaCMY–2 gene by ISEcp1-mediated, truncated by IS4, in the E118 strain was blaCMY–2-blc-yggR-tnp1-orf7-orf8-orf9-tnp2-hsdR. One inverted repeat was located ISEcp1 left (IRL1, GGATCTAAGATGCA), and the paired inverted repeat was ISEcp1 right (IRR1, TGCACCTTAAATCC). The second blaCMY–2 gene sequence by ISEcp1-mediated, adjacent to IRL2 (CCTAGATTCTACGT) and IRR2 (ACGTGGAATTTAGG), was conversely comprised in a different genetic organization (blaCMY–2-blc-yggR-tnp1-orf7-orf8). This context was devoid of the last three CDSs (orf9-tnp2-hsdR) compared to the first blaCMY–2-containing region. These three genes were identical to a part of the Shigella flexneri serotype 2a SRL pathogenicity island, being first reported to be involved in the transfer of antibiotic resistance (Luck et al., 2001). The paired 13 bp sequences for IRL1 and IRR1 adjacent to the configuration (ISEcp1-blaCMY–2) in the E597 strain were identical in the following three flanking regions in different locations on the chromosome: (i) blaCMY–2-blc-yggR, (ii) blaCMY–2-blc-yggR-tnp1-orf7-orf8-orf9-tnp2-hsdR, and (iii) blaCMY–2-blc. In the E686 strain, the genetic context containing-the blaCMY–2 gene by ISEcp1-mediated, adjacent to IRL1 and IRR1, was identified as blaCMY–2-blc-yggR-tnp1-orf7-orf8-orf9-tnp2-hsdR, which was identical to the genetic context of the first blaCMY–2 gene region in the E118 strain and the second blaCMY–2 gene region in the E597 strain. The common chromosomal genetic regions (blaCMY–2-blc-yggR-tnp1-orf7-orf8-orf9-tnp2-hsdR) in the E118, E597, and E686 strains were inserted in the same location, which was from 2,600,492 to 2,613,180 bp (Figure 3).
Evaluation of blaCMY–2 Transcript Levels
The gene expression of blaCMY–2 in group 1 was substantially elevated from 4.221- to 7.374-fold compared to the E118 strain. Group 2 and group 3 exhibited 1.385- to 1.935-fold and 0.188- to 0.307-fold increases in blaCMY–2 expression, respectively, compared to the E118 strain (Table 2).
blaCMY–2 Promoter Evaluation
We evaluated two sequences previously presumed to be involved in high levels of ESBL/AmpC gene expression (Smet et al., 2008; Ma et al., 2011; Kurpiel and Hanson, 2012; Manageiro et al., 2015): ISEcp1-provided promoter region sequence and a spacer sequence in front of blaCMY–2 (Figure 4). For the examined sequences, all 11 CMY-2-producing E. coli ST405 isolates shared 100% identity for above two putative promoter sequences related to high levels of ESBL/AmpC gene expression.
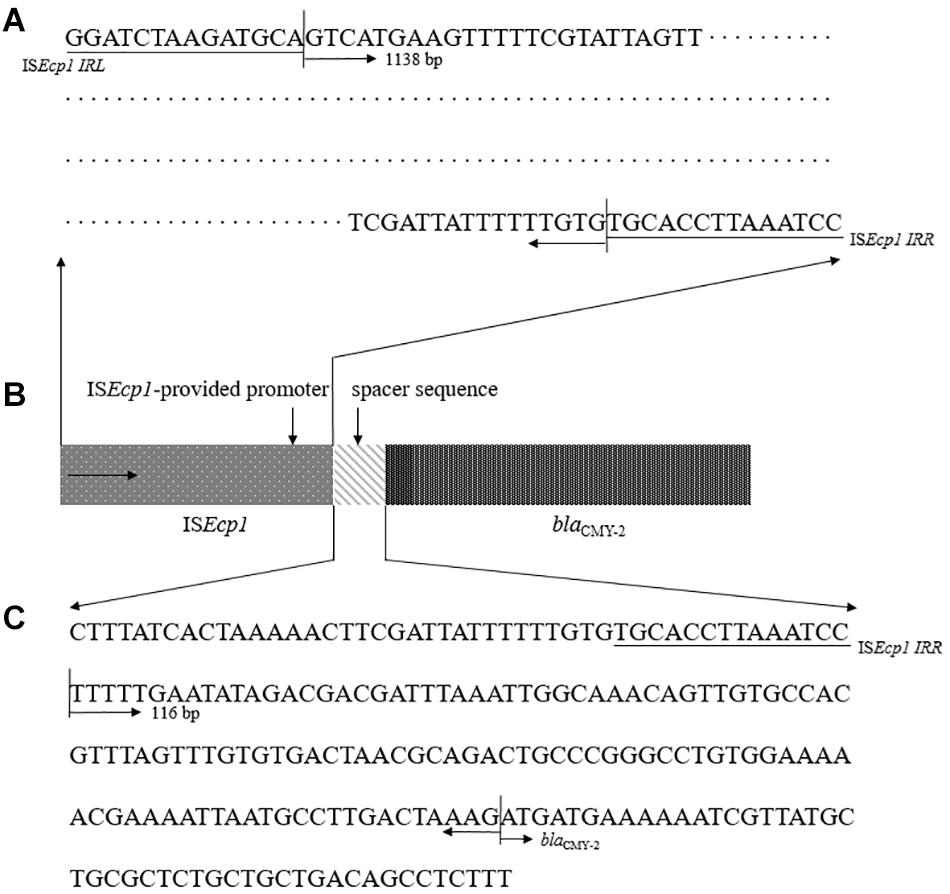
Figure 4. Representative configuration of blaCMY–2 promoters. (A) ISEcp1-provided putative promoter region sequence. (B) Structure of the blaCMY–2 containing region by ISEcp1-mediated transposition. (C) sequence analysis as predicted promoter within the 116 bp spacer between ISEcp1 and blaCMY–2.
Discussion
In our previous study, CMY-2 was the most prevalent ESBL/AmpC enzyme in diseased companion animals, accounting for 26.4% of the ESBL/AmpC enzymes, and CTX-M-14 and CTX-M-55 were the second most abundant enzymes. In addition, NDM-5 was detected in four E. coli isolates from four dogs along with blaCTX–M–15 and blaCMY–2 (Hong et al., 2019). However, in this study, CTX-M-14 was equally highest in healthy companion animals and cohabiting humans, and no carbapenemase genes were detected. Interestingly, the rate of ESBL/AmpC-producing E. coli isolates recovered from healthy companion animals and cohabiting humans was high, observing in more than 20% of the total rectal swab samples. The fact that companion animals could play an important role as a reservoir of AMR bacteria to humans has been reported previously (Day, 2011). In this regard, colonized feces of healthy companion animals can be considered a carrier for potentially transferring ESBL and/or AmpC β-lactamase for ESC resistance to humans.
To analyze clonal relatedness among predominant ESBL/AmpC-producing E. coli isolates from healthy companion animals and cohabiting humans, several closely related clonal lineages belonged to the same pulsotype were observed. Of them, intrahousehold pet-human sharing of AMR bacteria was observed in four cases (4.3%) of the 130 households. Therefore, this result showed that AMR households’ isolate could be shared between companion animals and humans, which was considered intrahousehold pet-human sharing, while largely occurred by interhousehold pet-human sharing with unknown routes of transmission of E. coli isolates colonization.
The clonal relationship of eleven CMY-2-producing E. coli ST405 isolates belonged to the a1 and a2 pulsotypes showed cladograms sharing >82%, but the isolates belonged to different households. In other words, these cases were considered interhousehold pet-human sharing. Chromosomal concordance between three CMY-2-producing E. coli ST405 isolates (E118, E597, and E686) was high (>98%), and prophage sequences were also identical by WGS analysis. Although they shared one identical genetic environment (blaCMY–2-blc-yggR-tnp1-orf7-orf8-orf9-tnp2-hsdR), which was identical to the genetic context of the clinical isolate E. coli TN44889 in 2004 (Verdet et al., 2009), the E118, E597, and E686 strains had different blaCMY–2 copy numbers and different blaCMY–2-containing regions. In this regard, our findings shed doubt whether the three E. coli ST405 isolates were considered as clonal spread based on PFGE analysis, or sporadic spread based on WGS analysis. This is important because if WGS methodology is used, the clonal relatedness of the strains could be different compared to methodology based on PFGE. This doubt demonstrates that epidemiological questions regarding the mechanism for dissemination of resistance determinants should be clarified to better understand the epidemiology of bacteria (de Been et al., 2014). In this study, intrahousehold pet-human sharing cases are likely to be considered as cases by clonal spread, while interhousehold cases seem to be sporadic spread.
We initially prospected the blaCMY–2 gene as the often observed plasmid-mediated AmpC β-lactamase (Verdet et al., 2009; Kameyama et al., 2013), but the locations of three blaCMY–2 genes on the chromosome were confirmed by WGS analysis. In the remaining E. coli ST405 isolates, the blaCMY–2 gene was suspected to be a chromosomal gene. This is because there are two identical 13 bp sequences (paired IRL1 and IRR1) adjacent to ISEcp1 and similar antimicrobial susceptibility phenotypes in 11 CMY-2-producing E. coli ST405 isolates. This result suggested that integration of the blaCMY–2-containing environment into the chromosome of certain ST405 clone appears to be involved in the dissemination of cefoxitin-resistant E. coli in South Korea.
CTX-M-type ESBL, especially CTX-M-15, is commonly more dominant than CMY-type AmpC β-lactamases in humans (Lee et al., 2018), but CMY-2-producing E. coli isolates have been frequently detected as much as CTX-M-type in veterinary medicine worldwide (Pietsch et al., 2018; Donà et al., 2019; Hong et al., 2019). Interestingly, cefoxitin is often used in the veterinary field for treating gram-positive and anaerobic bacterial infections (Brook et al., 2013; Lappin et al., 2017). It is likely that veterinary gram-negative isolates can also become resistant by multiple transpositions of the blaCMY–2 gene owing to cefoxitin selective pressure and then may accelerate the dissemination of cefoxitin-resistant E. coli.
For the three classified groups in 11 CMY-2-producing E. coli ST405 isolates, regarding the relationship between the MIC values of cefoxitin and the transcription level, the MIC values of cefoxitin were proportional to the blaCMY–2 copy number, varying from one to three copies. However, no mutations were observed in the 1138 bp upstream sequence of the blaCMY–2 gene and the 116 bp spacer sequence (Smet et al., 2008; Ma et al., 2011; Kurpiel and Hanson, 2012; Manageiro et al., 2015). This finding indicated that the higher level of blaCMY–2 expression correlated with multiple copy numbers of the blaCMY–2 gene is a key factor rather than the blaCMY–2 promoter mutation, but it could not be excluded a limitation that the total number of CMY-2-producing E. coli ST405 isolates analyzed in this study was only eleven.
Unfortunately, we initially tried to isolate samples up to five times per participants during the collection procedure to enhance the accuracy of the study, but the number of sampling actually varied from one to five due to individual circumstances of the participants.
Conclusion
This study presented the intrahousehold pet-human sharing of fecal ESBL/AmpC-producing E. coli isolates in healthy companion animals and cohabiting humans, indicating that the colonized feces of healthy companion animals could be a reservoir for spreading AMR bacteria to humans. Notably, E. coli ST405 isolates carrying multiple blaCMY–2 genes on the chromosome sporadically spread between companion animals and humans in South Korea. In addition, the gene copy number on the chromosome seems to be the most important factor affecting the transcription level of the AMR gene.
Data Availability Statement
The datasets generated for this study can be found in the NCBI GenBank: https://www.ncbi.nlm.nih.gov/nuccore/cp049196; https://www.ncbi.nlm.nih.gov/nuccore/cp049197; https://www.ncbi.nlm.nih.gov/nuccore/cp049198.
Ethics Statement
This study was carried out in accordance with the recommendation of ethical guidelines of KonKuk University College of Veterinary Medicine, South Korea. Written informed consent was obtained from the owners for the participation of their animals in this study.
Author Contributions
WS is the corresponding author who was in charge of the study design, data analysis and proofreading of the manuscript. JH performed an examination of molecular work (resistance gene, PFGE, and MLST), analyzed the experimental data, and wrote the manuscript. H-MP, J-CC, and SHJ designed the sample collection and the experiments. J-YO designed the experiments and performed the experiment. SJ conducted the statistical analysis.
Funding
This research was supported by a fund from the Korean Center for Disease Control and Prevention (2017-ER5405-01).
Conflict of Interest
The authors declare that the research was conducted in the absence of any commercial or financial relationships that could be construed as a potential conflict of interest.
Acknowledgments
We thank all participants for their contribution to the program.
Supplementary Material
The Supplementary Material for this article can be found online at: https://www.frontiersin.org/articles/10.3389/fmicb.2020.00674/full#supplementary-material
Footnotes
References
Ben Sallem, R., Ben Slama, K., Rojo-Bezares, B., Porres-Osante, N., Jouini, A., Klibi, N., et al. (2014). IncI1 plasmids carrying bla(CTX-M-1) or bla(CMY-2) genes in Escherichia coli from healthy humans and animals in Tunisia. Microb. Drug Resist. 20, 495–500. doi: 10.1089/mdr.2013.0224
Bogaerts, P., Huang, T. D., Bouchahrouf, W., Bauraing, C., Berhin, C., El Garch, F., et al. (2015). Characterization of ESBL- and AmpC-producing Enterobacteriaceae from diseased companion animals in Europe. Microb. Drug Resist. 21, 643–650. doi: 10.1089/mdr.2014.0284
Brook, I., Wexler, H. M., and Goldstein, E. J. (2013). Antianaerobic antimicrobials: spectrum and susceptibility testing. Clin. Microbiol. Rev. 26, 526–546. doi: 10.1128/CMR.00086-12
CLSI, (2019). Performance Standards for Antimicrobial Susceptibility Testing; Twenty-eighth Informational Supplement. Wayne, PA: Clinical and Laboratory Standards Institute.
Damborg, P., Broens, E. M., Chomel, B. B., Guenther, S., Pasmans, F., Wagenaar, J. A., et al. (2016). Bacterial zoonoses transmitted by household pets: state-of-the-art and future perspectives for targeted research and policy actions. J. Comp. Pathol. 155, S27–S40. doi: 10.1016/j.jcpa.2015.03.004
Day, M. J. (2011). One health: the importance of companion animal vector-borne diseases. Parasit Vectors 4:49. doi: 10.1186/1756-3305-4-49
de Been, M., Lanza, V. F., de Toro, M., Scharringa, J., Dohmen, W., Du, Y., et al. (2014). Dissemination of cephalosporin resistance genes between Escherichia coli strains from farm animals and humans by specific plasmid lineages. PLoS Genet. 10:e1004776. doi: 10.1371/journal.pgen.1004776
Donà, V., Scheidegger, M., Pires, J., Furrer, H., Atkinson, A., and Babouee Flury, B. (2019). Gradual in vitro evolution of cefepime resistance in an ST131 Escherichia coli strain expressing a plasmid-encoded CMY-2 β-Lactamase. Front. Microbiol. 10:1311. doi: 10.3389/fmicb.2019.01311
EUCAST (2019). The European Committee on Antimicrobial Susceptibility Testing. Breakpoint tables for interpretation of MICs and zone diameters, version 9.0.
Ewers, C., Bethe, A., Semmler, T., Guenther, S., and Wieler, L. H. (2012). Extended-spectrum β-lactamase-producing and AmpC-producing Escherichia coli from livestock and companion animals, and their putative impact on public health: a global perspective. Clin. Microbiol. Infect. 18, 646–655. doi: 10.1111/j.1469-0691.2012.03850.x
Gauthier, L., Dortet, L., Cotellon, G., Creton, E., Cuzon, G., Ponties, V., et al. (2018). Diversity of carbapenemase-producing Escherichia coli isolates in France in 2012-2013. Antimicrob. AgentsChemother. 62:e00266-18. doi: 10.1128/AAC.00266-18
Guo, Y. F., Zhang, W. H., Ren, S. Q., Yang, L., Lü, D. H., Zeng, Z. L., et al. (2014). IncA/C plasmid-mediated spread of CMY-2 in multidrug-resistant Escherichia coli from food animals in China. PLoS One 9:e96738. doi: 10.1371/journal.pone.0096738
Hong, J. S., Song, W., Park, H. M., Oh, J. Y., Chae, J. C., Shin, S., et al. (2019). Clonal spread of extended-spectrum cephalosporin-resistant Enterobacteriaceae between companion animals and humans in South Korea. Front. Microbiol. 10:1371. doi: 10.3389/fmicb.2019.01371
Hong, J. S., Yoon, E. J., Lee, H., Jeong, S. H., and Lee, K. (2016). Clonal dissemination of Pseudomonas aeruginosa sequence type 235 isolates carrying blaIMP-6 and emergence of blaGES-24 and blaIMP-10 on novel genomic Islands PAGI-15 and -16 in South Korea. Antimicrob. AgentsChemother. 60, 7216–7223.
Kameyama, M., Chuma, T., Yabata, J., Tominaga, K., Iwata, H., and Okamoto, K. (2013). Prevalence and epidemiological relationship of CMY-2 AmpC β-lactamase and CTX-M extended-spectrum β-lactamase-producing Escherichia coli isolates from broiler farms in Japan. J. Vet. Med. Sci. 75, 1009–1015. doi: 10.1292/jvms.12-0453
Kurpiel, P. M., and Hanson, N. D. (2012). Point mutations in the inc antisense RNA gene are associated with increased plasmid copy number, expression of blaCMY-2 and resistance to piperacillin/tazobactam in Escherichia coli. J. Antimicrob. Chemother. 67, 339–345. doi: 10.1093/jac/dkr479
Lappin, M. R., Blondeau, J., Boothe, D., Breitschwerdt, E. B., Guardabassi, L., Lloyd, D. H., et al. (2017). Antimicrobial use guidelines for treatment of respiratory tract disease in dogs and cats: antimicrobial guidelines working group of the international society for companion animal infectious diseases. J. Vet. Intern. Med. 31, 279–294. doi: 10.1111/jvim.14627
Lartigue, M. F., Poirel, L., and Nordmann, P. (2004). Diversity of genetic environment of bla(CTX-M) genes. FEMS. Microbiol. Lett. 234, 201–207.
Lazarus, B., Paterson, D. L., Mollinger, J. L., and Rogers, B. A. (2015). Do human extraintestinal Escherichia coli infections resistant to expanded-spectrum cephalosporins originate from food-producing animals? A systematic review. Clin. Infect. Dis. 60, 439–452. doi: 10.1093/cid/ciu785
Lee, H., Yoon, E. J., Kim, D., Jeong, S. H., Won, E. J., Shin, J. H., et al. (2018). Antimicrobial resistance of major clinical pathogens in South Korea, May 2016 to April 2017: first one-year report from Kor-GLASS. Euro Surveill. 23:1800047. doi: 10.2807/1560-7917.ES.2018.23.42.1800047
Luck, S. N., Turner, S. A., Rajakumar, K., Sakellaris, H., and Adler, B. (2001). Ferric dicitrate transport system (Fec) of Shigella flexneri 2a YSH6000 is encoded on a novel pathogenicity island carrying multiple antibiotic resistance genes. Infect. Immun. 10, 6012–6021. doi: 10.1128/iai.69.10.6012-6021.2001
Ma, L., Siu, L. K., and Lu, P. L. (2011). Effect of spacer sequences between bla(CTX-M) and ISEcp1 on bla(CTX-M) expression. J. Med. Microbiol. 60, 1787–1792. doi: 10.1099/jmm.0.033910-0
Manageiro, V., Ferreira, E., Pinto, M., Fonseca, F., Ferreira, M., Bonnet, R., et al. (2015). Two novel CMY-2-type β-lactamases encountered in clinical Escherichia coli isolates. Ann. Clin. Microbiol. Antimicrob. 14:12. doi: 10.1186/s12941-015-0070-8
Marques, C., Belas, A., Aboim, C., Cavaco-Silva, P., Trigueiro, G., Gama, L. T., et al. (2019). Evidence of sharing of Klebsiella pneumoniae strains between healthy companion animals and cohabiting humans. J. Clin. Microbiol. 57:e01537-18. doi: 10.1128/JCM.01537-18
Mata, C., Miró, E., Alvarado, A., Garcillán-Barcia, M. P., Toleman, M., Walsh, T. R., et al. (2012). Plasmid typing and genetic context of AmpC β-lactamases in Enterobacteriaceae lacking inducible chromosomal ampC genes: findings from a Spanish hospital 1999-2007. J. Antimicrob. Chemother. 67, 115–122. doi: 10.1093/jac/dkr412
Pietsch, M., Irrgang, A., Roschanski, N., Brenner Michael, G., Hamprecht, A., Rieber, H., et al. (2018). Whole genome analyses of CMY-2-producing Escherichia coli isolates from humans, animals and food in Germany. BMC Genomics 19:601. doi: 10.1186/s12864-018-4976-3
Schmiedel, J., Falgenhauer, L., Domann, E., Bauerfeind, R., Prenger-Berninghoff, E., Imirzalioglu, C., et al. (2014). Multiresistant extended-spectrum β-lactamase-producing Enterobacteriaceae from humans, companion animals and horses in central Hesse, Germany. BMC Microbiol. 14:187. doi: 10.1186/1471-2180-14-187
Smet, A., Martel, A., Persoons, D., Dewulf, J., Heyndrickx, M., Catry, B., et al. (2008). Diversity of extended-spectrum beta-lactamases and class C beta-lactamases among cloacal Escherichia coli Isolates in Belgian broiler farms. Antimicrob. Agents Chemother. 52, 1238–1243. doi: 10.1128/AAC.01285-07
Tenover, F. C., Arbeit, R. D., Goering, R. V., Mickelsen, P. A., Murray, B. E., Persing, D. H., et al. (1995). Interpreting chromosomal DNA restriction patterns produced by pulsed-field gel electrophoresis: criteria for bacterial strain typing. J. Clin. Microbiol. 33, 2233–2239. doi: 10.1128/jcm.33.9.2233-2239.1995
Keywords: Escherichia coli, CTX-M, CMY-2, healthy companion animal, human, copy number
Citation: Hong JS, Song W, Park H-M, Oh J-Y, Chae J-C, Jeong S and Jeong SH (2020) Molecular Characterization of Fecal Extended-Spectrum β-Lactamase- and AmpC β-Lactamase-Producing Escherichia coli From Healthy Companion Animals and Cohabiting Humans in South Korea. Front. Microbiol. 11:674. doi: 10.3389/fmicb.2020.00674
Received: 17 February 2020; Accepted: 24 March 2020;
Published: 15 April 2020.
Edited by:
Shaolin Wang, China Agricultural University, ChinaReviewed by:
Hong Yao, Henan Agricultural University, ChinaRongmin Zhang, South China Agricultural University, China
Copyright © 2020 Hong, Song, Park, Oh, Chae, Jeong and Jeong. This is an open-access article distributed under the terms of the Creative Commons Attribution License (CC BY). The use, distribution or reproduction in other forums is permitted, provided the original author(s) and the copyright owner(s) are credited and that the original publication in this journal is cited, in accordance with accepted academic practice. No use, distribution or reproduction is permitted which does not comply with these terms.
*Correspondence: Wonkeun Song, swonkeun@naver.com