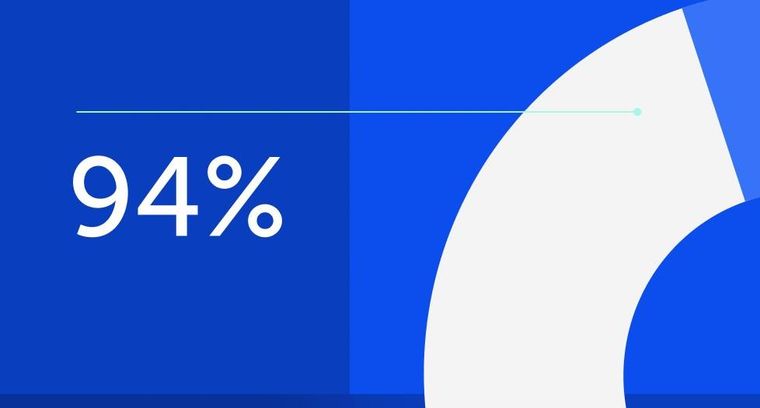
94% of researchers rate our articles as excellent or good
Learn more about the work of our research integrity team to safeguard the quality of each article we publish.
Find out more
ORIGINAL RESEARCH article
Front. Microbiol., 20 March 2020
Sec. Antimicrobials, Resistance and Chemotherapy
Volume 11 - 2020 | https://doi.org/10.3389/fmicb.2020.00428
This article is part of the Research TopicAntimicrobial Resistance As a Global Public Health Problem: How Can We Address It?View all 50 articles
Ciprofloxacin is the choice treatment for infections caused by Salmonella Typhi, however, reduced susceptibility to ciprofloxacin has been reported for this pathogen. Considering the decreased approbation of new antimicrobials and the crisis of resistance, one strategy to combat this problem is to find new targets that enhances the antimicrobial activity for approved antimicrobials. In search of mutants with increased susceptibility to ciprofloxacin; 3,216 EZ-Tn5 transposon mutants of S. Typhi were screened. S. Typhi zxx::EZ-Tn5 mutants susceptible to ciprofloxacin were confirmed by agar diffusion and MIC assays. The genes carrying EZ-Tn5 transposon insertions were sequenced. Null mutants of interrupted genes, as well as inducible genetic constructs, were produced using site-directed mutagenesis, to corroborate phenotypes. SDS-PAGE and Real-time PCR were used to evaluate the expression of proteins and genes, respectively. Five mutants with increased ciprofloxacin susceptibility were found in the screening. The first confirmed mutant was the glutamine synthetase-coding gene glnA. Analysis of outer membrane proteins revealed increased OmpF, a channel for the influx of ciprofloxacin and nalidixic acid, in the glnA mutant. Expression of ompF increased four times in the glnA null mutant compared to WT strain. To understand the relationship between the expression of glnA and ompF, a strain with the glnA gene under control of the tetracycline-inducible Ptet promoter was created, to modulate glnA expression. Induction of glnA decreased expression of ompF, at the same time that reduced susceptibility to ciprofloxacin. Expression of sRNA MicF, a negative regulator of OmpF was reduced to one-fourth in the glnA mutant, compared to WT strain. In addition, expression of glnL and glnG genes (encoding the two-component system NtrC/B that may positively regulate OmpF) were increased in the glnA mutant. Further studies indicate that deletion of glnG decreases susceptibility to CIP, while deletion of micF gene increases susceptibility CIP. Our findings indicate that glnA inactivation promotes ompF expression, that translates into increased OmpF protein, facilitating the entry of ciprofloxacin, thus increasing susceptibility to ciprofloxacin through 2 possible mechanisms.
Salmonella Typhi is the etiological agent of typhoid fever, endemic in many developing countries worldwide. This disease is exclusive of humans with a mortality rate of 10%. The emergence of the extensively drug-resistant S. Typhi H58 strains in Pakistan, which are resistant to the first-line drugs (ampicillin, chloramphenicol, and cotrimoxazole), fluoroquinolones, and third-generation cephalosporin, is a real threat with potential to become typhoid fever untreatable (Cabello, 2018; Johnson et al., 2018; Klemm et al., 2018). Thus, typhoid fever requires alternative pharmacological treatment, including new antimicrobials and development of new combined therapies to revitalize existing antibiotics to prolong its useful life and slow down the emergence of resistance (Cottarel and Wierzbowski, 2007; Fajardo et al., 2008; Liu et al., 2010; Rodas et al., 2010; Sabbagh et al., 2012; González-Bello, 2017).
To find mutants with increased susceptibility to Ciprofloxacin (CIP), a screening was performed over 3,216 insertional mutants of S. Typhi STH2370 with the EZ-Tn5TM transposon exposed to sub-MIC concentrations of CIP. We identified both: a small number of known and new genetic determinants of resistance to quinolones. One such mutant in the glnA gene that encodes for glutamine synthetase (GS) was further characterized. GS produces glutamine from glutamate and ammonia and has a crucial role in nitrogen metabolism. The internal concentration of glutamine is the main intracellular signal for regulating nitrogen availability in enteric bacteria (Zimmer et al., 2000; Switzer et al., 2018). Nitrogen is essential for the biosynthesis of macromolecules in bacteria; thus, the adaptive response to metabolic stress induced by starvation of nitrogen (as it is the case of glutamine auxotrophic bacteria) could affect bacterial physiology, including the susceptibility to antimicrobials which today is known to be modulated by metabolism (Maria-Neto et al., 2012; Peng et al., 2015; Vestergaard et al., 2017; Cui et al., 2019).
One example that relates nitrogen metabolism and susceptibility to antimicrobials is the observation of Escherichia coli strains resistant to magainin I (a cationic peptide) which overexpress GS (Maria-Neto et al., 2012). In Streptococcus pneumoniae it was demonstrated that glnA was repressed in the presence of penicillin, as well as, the inhibition of GS enhanced susceptibility to penicillin. Hence, glutamine conferred a protective role against penicillin when added to the culture medium (El Khoury et al., 2017). In the same line, methicillin-resistant Staphylococcus aureus and methicillin-susceptible S. aureus with lower expression of GS decreased their level of methicillin resistance (Gustafson et al., 1994). It is proposed that in S. aureus GS participates in the production of constituents of the cell envelope, therefore maintaining the cell wall thickness and the level of crosslinking on peptidoglycan (Gustafson et al., 1994; Lima et al., 2013). In Mycobacterium tuberculosis, GS plays a crucial role in the cell wall biosynthesis; therefore, inhibition of GS in M. tuberculosis with the inhibitor L-methionine-SR-sulfoximine increases susceptibility to isoniazid. Again, this study concludes that inhibition of GS activity affects the synthesis of peptidoglycan layers of the cell wall, changing susceptibility (Harth and Horwitz, 2003).
In this work, we describe that glnA mutants of S. Typhi increase their susceptibility to quinolones. Interestingly OmpF, a porin forming a homotrimer channel for the influx of CIP and nalidixic acid, was augmented in the S. Typhi glnA mutant. Further studies using a tetracycline-inducible system, revealed an inverse correlation between glnA and ompF expression. Our findings suggested that glnA inactivation increases the susceptibility of S. Typhi to CIP through increasing OmpF.
Salmonella Typhi SHT2370 an antibiotic-sensitive virulent clinical strain was obtained from the Infectious Diseases Hospital Lucio Cordova, Santiago, Chile (Valenzuela et al., 2014). Mutants used in this study were derived from S. Typhi STH2370 and are shown in Table 1. Bacteria were grown in LB medium (1% peptone, 0.5% yeast extract, 0.5% NaCl) at 37°C with aeration. When required, the medium was supplemented with Kanamycin (KAN) 50 mg/L, Ampicillin (AMP) 100 mg/L, Chloramphenicol (CAM), CIP (at different concentrations) or Chlortetracycline (CTET at different concentrations). Media were solidified with agar (15 g/L) as required. Mutants in genes glnL and glnG were produced by P22 transducing null mutations with the KANR cassette from S. Typhimurium.
Mutagenesis with the commercial transposon EZ-Tn5TM (Epicentre, CA, United States) was performed as indicated by the provider (protocols available at https://www.lucigen.com/docs/manuals/MA155E-EZ-Tn5-r6kg -Ori-KAN-2-Transposome-Kit.pdf at February 17, 2020) with minor modifications. In brief, S. Typhi STH2370 was grown to OD600 0.4, 5 mL were washed six times with sterile water, resuspended in 100 μL of sterile water and electroporated with 1 μL of EZ-Tn5 transposome at a concentration of 0.1 pmol/μL. Electroporated bacteria were recovered in 1 mL of LB broth, and incubated with aeration for 40 min before plating on LB-agar containing KAN 50 mg/L and overnight incubation. Mutants S. Typhi mutants STH2370 zxx::EZ-Tn5 were organized on a 6 × 8 pattern in 67 lots creating a collection of 3,216 mutants. The primary screening was performed by transferring colonies to LB plates with CIP 0.05 mg/L using a 48-pins microplate replicator. Mutants with increased susceptibility were selected by visual examination and confirmed by disk diffusion assay. The susceptibility of confirmed mutants was quantified by MICs assays before proceeding with cloning and sequencing of interrupted genes.
The mutants with at least two-fold-change in the MIC were subjected to cloning. Briefly, genomic DNA of mutants was extracted, digested with EcoRV and ligated with T4 DNA ligase. Ligated DNA was electroporated in E. coli DH5αλpir, recovered in 1 mL of LB broth, and incubated with aeration for 40 min at 37°C before plating on LB-agar containing KAN 50 mg/L. KANR colonies were grown, and plasmidial DNA was column-purified to perform Sanger sequencing. Sequences were analyzed using the Basic Local Alignment Search Tool available at http://www.ncbi.nlm.nih.gov.
The antibiotic susceptibility tests were performed by Kirby-Bauer disk diffusion method according to CLSI guidelines (CLSI, 2012a). Suspensions of 2 mL of microorganism were grown overnight in liquid culture using LB medium with aeration. The bacterial suspensions were diluted 1:1000 in 0.9% NaCl and 100 μL of this suspension were seeded on Mueller Hinton (MH) agar plates pH 7.3. CIP susceptibilities tests were performed with 5 μg CIP disks onto seeded plates incubated at 37°C for 16–18 h. Halos were recorded as the average of 2-crossed diameters in biological triplicate assays.
MIC was determined using broth microdilution susceptibility testing according to CLSI guidelines (CLSI, 2012b). Briefly, 0.9% NaCl was used to prepare inoculum until turbidity McFarland 0.5, with an equivalent density to 1–2 × 108 CFU/mL. The inoculum was then diluted to reach a concentration of 5 × 104 CFU/well. Antibiotics were serially diluted in base-2 and each well-inoculated with 50 μL of diluted bacteria. The plates were incubated at 37°C for 16 h and read at 600 nm. The MIC was determined as the concentration that allowed less than 50% growth density compared to the non-antibiotic control.
Site-directed mutants of S. Typhi STH2370 were constructed by allelic exchange facilitated by the Red/Swap Technique (Datsenko and Wanner, 2000). Briefly, PCR product, with a resistance cassette flanked by regions with homology to the target DNA sequences, was electroporated into strains carrying pKD46, recovered in 1 mL of LB broth and incubated for 1 h at 37°C with aeration before plating onto plates with the corresponding antibiotic to select mutants. When needed the resistance cassette was eliminated to produce FRT scar mutants by introducing pCP20 which encodes the Flippase recombinase that promotes scission from FRT sequences flanking the resistance cassette. The primers used for these constructions are in Supplementary Table 1.
Construction of the tetracycline-dependent (TD) mutants S. Typhi glnATD, S. Typhi ompFTD and S. Typhi STY4173TD, was carried out using a modification of the Red-Swap method for promoter mutation of target genes as described by Hidalgo et al. (2016). The primers used for these constructions are in Supplementary Table 1.
The OMPs fraction of protein was obtained by a method previously described (Lobos and Mora, 1991). Two mL of bacteria were centrifuged at 10,000 g for 10 min. The pellet obtained was resuspended in 10 mM Tris Hydrochloride pH 8, sonicated for 100 s and centrifuged at 7,000 g for 5 min. The supernatant obtained was centrifuged at 13,000 g for 45 min, and the pellet was resuspended in 10 mM Tris Hydrochloride pH 8 with 2% Triton X-100. The suspension was incubated at 37°C for 30 min with occasional stirring and centrifuged at 13,000 g for 45 min. The pellet containing OMPs was resuspended in 100 mM Tris Hydrochloride pH 8, 2% SDS and quantified using BCA protein assay kit. Next, 50 μg of protein were heated at 98°C for 5 min and resolved in an SDS-PAGE at 100 V. The gels were rinsed with water, pre-treated for 60 min with 40% ethanol and 10% acetic acid, washed twice for 10 min and stained in 0.1% acid Coomassie blue R250, 2% orthophosphoric acid, 10% ammonium sulfate, and 20% methanol overnight. Finally, gels were washed with 1% acetic until all Coomassie particles were removed. High resolution pictures of gels were open in the ImageJ software. Using the area selection tool, a horizontal line was traced to cut pick that were then analyzed by their density, which was compared to density of WT strain for the bands corresponding to OmpF (Gallo-Oller et al., 2018).
For extraction of RNA, overnight cultures were diluted 100 times and cultured in fresh LB or LB + CTET as appropriate, until reaching OD600 0.5. RNA was extracted using the Trizol method according to the provider instructions. Briefly, 20 mL of bacterial cell was harvested, washed twice with ice-cold water and treated with lysozyme at RT before adding TrizolTM (Invitrogen). The samples were mixed before adding ice-cold chloroform, centrifugation and recovering the supernatant. RNA was precipitated with isopropanol, washed with 70% v/v ethanol and resuspended in nuclease-free water before treatment with DNase I. Reverse transcriptions of samples were performed with 1 μg of DNase-treated RNA using SuperScriptTM II Reverse Transcriptase (Invitrogen) with Random Hexamers (Invitrogen). Primers for the genes dnaN and lon were used to normalize the expression of target genes. Although dnaN and lon are both good expression controls, dnaN was preferred for assays that used tetracyclines to minimize distortion of results as dnaN remained unchanged compared to lon, in presence of CTet. Quantification was carried out in an EcoTM Real-Time PCR System using Brilliant II SYBRTM Master Mixes. The fold change was calculated as described by Pfaffl (Pfaffl, 2001). The primers used for these assays are listed in Supplementary Table 1.
Student’s t-test was realized if samples obtained of different experiments have passed Shapiro–Wilk test for normality. The data are presented as mean ± SD of at least biological triplicates. Statistical analyses of results from RT-qPCR were calculated using the paired Student’s t-test (GraphPad Prism version 8.0, San Diego, CA, United States).
We screened 3,216 S. Typhi STH2370 zxx::EZ-Tn5 mutants on MH-agar plates with CIP 0.05 mg/L. A total of 43 susceptible mutants with poor or not growth were selected by visual analysis of the colonies. Confirmation of CIP susceptible mutants was performed by using disk diffusion assay. From this test, 10 mutants were confirmed, as their inhibition halos were 6 mm greater compared with WT control and were subjected to MIC assays. Eight mutants were twice as sensitive, one mutant was four-times more sensitive, and one mutant was eight-times more sensitive to CIP, compared with the WT counterpart. These mutants were set for cloning and further sequencing of the interrupted genes. All selected mutants that were sent to sequencing had one transposon insertion only. An extensive search was performed for information linking the mutated genes to antibiotics resistance. Five mutants, recC::EZ-Tn5, cysE::EZ-Tn5, aefA::EZ-Tn5, dacC::EZ-Tn5 and glnA::EZ-Tn5, resulted of especial interest after considering two criteria: (i) the level of susceptibility of the mutant and (ii) the relevance/novelty of the interrupted gene. Supplementary Figure 1 shows the screening workflow to identify genes leading to susceptibility. Subsequently, a secondary screening was performed, over the five mutants of interest, to determine whether observed susceptibility was general or specific for one antimicrobial or a family of them. Diameters of inhibition haloes for the five insertional mutants selected with different antimicrobials were recorded (Supplementary Table 2). After discarding the best candidate (S. Typhi aefA::EZ-Tn5) whose increased susceptibility was product of polar effects produced by the transposon over the acrAB locus upstream of aefAgene, we began the characterization of mutantS. Typhi STH2370 glnA::EZ-Tn5 that showed increased susceptibility to NAL and CIP in the screening (Supplementary Table 2). While the other three mutants were not further characterized.
To rule out polar effects that might modulate antimicrobial susceptibility, in the S. Typhi glnA::EZ-Tn5, we constructed mutant S. Typhi STH2370 glnA::kan and S. Typhi STH2370 glnA::FRT (Supplementary Figure 2). All mutants in glnA gene (glnA::EZ-Tn5, glnA::kan, and glnA::FRT) presented the same range of increased susceptibility to CIP with a diameter of inhibition haloes ∼11 mm larger and a MIC twice as sensitive compared to the WT strain (Figure 1 and Table 2). The observation that S. Typhi glnA::FRT (as well as all null mutants in glnA gene) shows the same level of susceptibility, rule out any possible polar effect governing the observed phenotype, as FRT sequence is by far less invasive that resistance cassettes.
Figure 1. Salmonella Typhi mutants in glnA gene show susceptibility to ciprofloxacin. A disk diffusion assay was performed to measure inhibition zones of S. Typhi STH2370 (WT), S. Typhi STH2370 glnA::EZ-Tn5, S. Typhi STH2370 glnA::kan and S. Typhi STH2370 glnA::FRT. The strains were seeded onto MH agar plates pH 7.3. Followed, 5 μL of ciprofloxacin 2 mg/mL were spotted onto a filter paper disk and placed on the plates and incubated at 37°C for 16–18 h.
Because inactivating glnA gene increases susceptibility to CIP, we searched for information regarding determinants of susceptibility to this antibiotic. As outer membrane proteins may be implicated in antibacterial entry through the cell envelope (Nikaido, 2003), we decided to study the profiles of OMPs in the glnA::FRT. SDS-PAGE profile of OMPs, showed a threefold increase of OmpF in S. Typhi STH2370 glnA::FRT, compared with WT (Figures 2A,B). Same result was observed for all glnA null mutants in S. Typhi, the results for S. Typhi glnA::FRT and S. Typhi glnA::kan are shown in Supplementary Figure 3, compared to S. Typhi WT and a S. Typhi ompF mutant. Interestingly, deletion of ompF produces changes in the OMPs pattern, at the level of making OMPs unrecognizable by simple Coomassie staining. Previous observation reported changes on the amount of OMPs in an ompF null mutant of a clinical isolate of S. Typhi. However, in this mutant main porins, such as ompC and ompA did not change their migration pattern (Villarreal et al., 2014). It could be the case that the extend of changes produced after ompF is mutated differs between isolates of S. Typhi. Despite that, abundance of OmpF would be the responsible of increased susceptibility to CIP, as ompF is also augmented in the ompF inducible mutant, but not other porins, as shown in further experiments. Next, RT-qPCR was performed to measure ompF expression in glnA::FRT mutant. As shown in Figure 2C, the expression of ompF gene was at least four-times higher in the glnA::FRT mutant compared with WT strain.
Figure 2. The glnA mutant has increased OmpF. (A) OMPs profile from S. Typhi STH2370 (WT) and S. Typhi STH2370 glnA::FRT (glnA). (B) Densitometric analysis of the OmpF porin in the glnA mutant compared with WT strain. Analysis was performed by using the ImageJ software, as described in Section “Materials and Methods.” (C) Expression level of ompF transcripts in the glnA mutant compared with the WT strain. Expression of ompF transcripts was normalized to expression of dnaN and compared to the expression of ompF in the WT strain. The figure shows the mean of three assays. Asterisks indicate significance, calculated by Student’s t-test, of results linked by brackets ∗p ≤ 0.05.
To confirm how changes in glnA expression cause changes in ompF expression and susceptibility to CIP, we modulated the expression of glnA by using a tetracycline-inducible system as previously described (Hidalgo et al., 2016). For that purpose, we successfully replaced the glnA gene promoter with the tetRA cassette (Supplementary Figure 4). This mutant allows modulation of glnA expression by using tetracyclines, to study ompF expression and susceptibility to CIP as a function of glnA expression. Because both OmpF and TetA (the efflux pump for tetracyclines) participate in transport through membranes, we excised tetA gene, from the tetRA cassette, to reduce interferences, caused by the presence of TetA in the inner membrane. This last strain was named as the tetracycline-dependent glnA mutant (or simply glnATD), as shown in Supplementary Figure 4. Using the same strategy, we constructed the tetracycline-dependent ompF mutant (ompFTD), and the same inducible construct was produced for the unrelated gene STY4173 (4173TD) that encodes for a putative protein, as a control. Details regarding these mutants are shown in Supplementary Figure 5. Using the S. Typhi glnATD mutant, we observed that inhibition haloes in the presence of CIP decreased, as the concentration of CTET increases in the plates, to values comparable to the inhibition diameters observed for the WT strain (from 46.0 mm without induction to 38.1 mm with 0.05 μg/mL of CTET). In addition, in uninduced conditions, the glnATD mutant shows an inhibition diameter closer to glnA::FRT null mutant, 46.0 mm and 48.0 mm, respectively (Table 3).
In contrast, using the ompFTD mutant, the inhibition haloes in the presence of CIP increased as the concentration of CTET increases in the plates, to values close to the inhibition diameters observed for the WT strain (from 31.9 mm without induction to 41.0 mm in the plates with 0.05 μg/mL of CTET). Induction of STY4173TD expression did not cause significant changes in the inhibition halo to CIP (Table 3). The treatment with CTET did not produce significant changes in susceptibility of either WT or glnA::FRT strain to CIP.
In the view of the decreased susceptibility to CIP when glnA is induced with CTET, we decided to assess the expression of glnA and ompF transcripts in the tetracycline-dependent mutants by RT-qPCR. Expression of glnA was increased as CTET concentrations increased (Figure 3A). Next, we investigated the expression of ompF in the glnATD with and without CTET induction. We found that in the glnATD mutant without induction, ompF is overexpressed close to five-times compared with WT strain (Figure 3B), the expression of ompF in the glnATD mutant without induction, is similar to the expression of ompF observed in S. Typhi glnA::FRT null mutant (Figure 2C). When glnA expression was induced with CTET we observed a significant decrease in ompF expression. Using the ompFTD mutant, we found that without induction, expression of ompF is dramatically reduced compared to WT strain. Induction of ompFTD mutant with 0.05 μg/mL of CTET significantly induced expression of ompF over 150-times compared to uninduced condition and over 1.5-times compared to the WT strain. Higher concentration (0.1 μg/mL) induced ompF only 50 times compared to uninduced condition and produces only half the expression of ompF observed in WT strain (Figure 3C). As a control, we measured the induction of ompF after inducing the unrelated gene STY4173 (Figure 3D). The presence of CTET induced expression of ompF two to threefold compared to uninduced condition and was slightly higher compared to WT strain in the presence of 0.1 μg/mL CTET. The results indicate that expression of glnA inversely correlates with expression of ompF, while increased expression of ompF may be responsible of increased susceptibility to CIP.
Figure 3. Expression of ompF and its dependency in glnA expression. Expression level of glnA transcripts in the mutant glnATD (A) and expression of ompF transcripts in the mutant glnATD (B), ompFTD (C) and 4173TD (D). The mRNA was extracted from tetracycline-dependent mutants under induced (0.05–0.1 μg/mL CTET) and uninduced conditions. Expression of glnA or ompF was normalized to expression of dnaN and compared to expression of glnA or ompF in the WT strain without induction. Results are average of at least three biological replicates. Asterisks indicate significance, calculated by Student’s t-test, of results liked by brackets. *p ≤ 0.05.
MicF is a small RNA that promotes degradation of ompF transcript and blocks translation by interacting with the Shine-Dalgarno sequence (Morillon, 2018). Because of this direct effect of MicF over OmpF, expression of MicF sRNA was studied in the glnA mutant and in the glnATD mutant with different levels of glnA expression. As observed in the Figure 4A, the S. Typhi glnA::FRT null mutant expresses around one-fourth of MicF, compared to the S. Typhi WT. To corroborate this result, MicF expression was studied in the S. Typhi glnATD mutant. Increased expression of glnA with 0.05 and 0.1 μg/mL CTET (as shown in Figure 3A) parallel with increased expression of MicF (Figure 4B). It is also observed that in uninduced conditions, MicF levels are slightly reduced compared with WT strain, probably MicF expression is sensitive to low levels of GlnA that transcribes even in the absence of induction with CTET. Conversely, deletion of micF gene slightly increased susceptibility to CIP in S. Typhi (Table 4). MicF is a well-described sRNA that negatively regulates transcript levels of ompF. Whether the induction of MicF by GlnA is direct or through other factors is unknown.
Figure 4. Expression of MicF transcripts depends on expression of glnA::FRT. Expression of MicF was studied in a glnA null mutant and in the glnATD mutant with different concentrations of chlortetracycline (0, 0.05, or 0.1 μg/mL). Expression of MicF was normalized to the expression of lon (A) or dnaN (B) and compared to the expression of MicF in the WT strain. Results are average of at least three biological replicates. Asterisks indicate significance, calculated by Student’s t-test, of results linked by brackets. *p ≤ 0.05.
Table 4. Susceptibility to ciprofloxacin of S. Typhi null mutants in genes glnL, glnG, micF, and ompF.
In E. coli previous findings indicate that ompF is under the control of NtrBC two-component system encoded by glnL and glnG, as observed in microarray assays (Zimmer et al., 2000). In enterobacteria, these genes are found downstream of glnA and are involved in the stress response of enteric bacteria to nitrogen-limited growth (Bhagirath et al., 2019) (Supplementary Figure 2). The deletion of glnA increases the expression of glnL and glnG, 12- and 8-times respectively, compared to S. Typhi WT (Figure 5), indicating that glnA negatively regulates expression of glnL and glnG through maintaining steady concentrations of glutamine. Mutating glnA reveals a positive regulation of NtrBC on the expression of ompF. This regulation pathway may underly the mechanism of increased susceptibility to CIP in the glnA mutants. To study the phenotype associated to glnL and glnG, null mutations of these genes were transduced into S. Typhi to test for resistance to CIP. As shown in Table 4, deletion of glnL gene has no effect on resistance to CIP. When glnG, which encodes for a transcriptional regulator, is deleted in S. Typhi, the mutant shows moderately, yet significantly, resistant to CIP. It is plausible that while over expression of GlnG correlates with susceptibility, deletion of glnG produces resistance. However, the correlation of activation of NtrBC two-component system [encoded by glnL (NtrB) and glnG (NtrC) genes] need to be further validated.
Figure 5. Expression level of glnL and glnG transcripts in the mutant glnA::FRT compared to WT strain. Expression of transcripts was normalized to the expression of lon and compared to the expression of glnL or glnG in the of WT strain. Results are average of at least three biological replicates. Asterisks indicate significance, calculated by Student’s t-test, of results liked by brackets. *p ≤ 0.05.
The threat of extensively drug-resistant S. Typhi isolates requires the development of new treatment strategies. One approach is the search for antibiotics adjuvants-compounds (Murima et al., 2014; Baquero and Martínez, 2017). In a non-exhaustive screening of S. Typhi STH2370, we found the mutant S. Typhi glnA::EZ-Tn5, with half the MIC to CIP. This change reveals a low-level resistance, mediated by an intrinsic mechanism of protection in a microorganism that is in principle susceptible to CIP. It is thought that the genes determining such intrinsic low-levels of resistance (2- to 4-times the MIC) might be of clinical importance and they could be target for adjuvant therapies (Baquero and Martínez, 2017). GS plays a crucial role in nitrogen metabolism whose main function is to provide glutamine to the cell. In Enterobacteria, the reaction catalyzed by GS is the only biosynthetic pathway for glutamine synthesis; thus, glnA deletion results in glutamine auxotrophy (Ikeda et al., 1996; Shimizu, 2013). The glnA gene has not been previously related with changes in susceptibility to quinolones, in Gram-negative bacteria. Expression of the major porin OmpF, a well-characterized channel for CIP and NAL entry, was increased across all glnA null mutants tested and the inducible glnA mutant without induction.
Interestingly, the mechanism behind increased susceptibility differs from affecting integrity of the cell wall, as antimicrobials targeting the cell wall have little or no effect on the glnA null mutant (Supplementary Table 2). Our results contrast with previous reports regarding GS and resistance to antimicrobials (Harth and Horwitz, 2003; Lima et al., 2013; El Khoury et al., 2017). It was found an inverse correlation between expression of glnA and ompF and a direct correlation between expression of glnA and micF. Decreased expression of micF in the glnA null mutant might explain at least in part the increased expression of ompF and increased susceptibility to CIP in the glnA mutants.
The growth under nitrogen-limiting conditions activates the Nitrogen regulation stress response, under the control of NtrBC two-component system encoded by glnL (NtrB) and glnG (NtrC) genes. This two-component system is composed of NtrB, the cognate histidine kinase that phosphorylates NtrC to its active form to regulate expression of genes including glnA, as previously described (van Heeswijk et al., 2013). In this study, inactivation of glnA resulted in increased expression of glnL and glnG genes. Importantly, it was found that expression of ompF is controlled by NtrC in E. coli (Zimmer et al., 2000). Therefore, this increase in the expression of glnL and glnG may explain the increased OmpF and increased susceptibility when glnA expression is suppressed or reduced as we illustrate in Figure 6. Indeed, mutation of glnG (encoding for NtrC transcriptional regulator) produces moderate resistance to CIP. This observation is consistent with increased glnLG expression in the glnA mutants, which may trigger ompF expression, finally allowing entry of CIP through OmpF porin.
Figure 6. Integrative model of the regulation of OmpF and its implication in susceptibility to CIP. Expression of OmpF is negatively controlled by GlnA. Proposed model illustrating how glnA gene is necessary to maintain low expression of OmpF. Based in our data, deleting glnA gene promotes ompF expression that in turns facilitates CIP and nalidixic acid entry into the bacterial cell through OmpF.
Other mechanisms, modulating antimicrobial resistance were studied including regulators MarA, MarR, and RamA; efflux-system components AcrB and TolC and porin OmpC. We found no differences in the expression of the genes encoding for such antimicrobial’s resistance factors (Supplementary Figure 6). Thus, enhanced susceptibility to quinolones observed in the glnA mutants could be linked to the increase of OmpF and associated with nutritional stress due to the decrease of glutamine and lower expression of MicF.
The experimental results show that the inactivation of glnA gene drives to increased susceptibility to CIP in S. Typhi, through increasing outer membrane protein OmpF. The finding that genes implicated in nitrogen metabolism, such as glnA, modulate susceptibility to antimicrobials can be useful in search of potential targets to combat antimicrobial resistance.
The datasets generated for this study are available on request to the corresponding author.
ARM and AH participated in the conception of ideas, performed the experiments, analyzed and interpreted the data, and participated in the drafting of manuscript. AYM performed experiments, collected and analyzed the data, and revised the draft. CS, NV, and GM collected and analyzed the data and participated in drafting of manuscript.
This work was supported by the Fondo Nacional de Ciencia y Tecnología (FONDECYT, Government of Chile) Grants 11150588 (AH), 1151393 (GM), and 1160315 (CS); UNAB Regular Grants DI-15-19/RG (AH) and DI-4-17/RG (NV); and ECOS-CONICYT grant C16B04 (AH). ARM has been a predoctoral fellow at Comisión Nacional de Ciencia y Tecnología grant 21120035 (CONICYT, Government of Chile).
The authors declare that the research was conducted in the absence of any commercial or financial relationships that could be construed as a potential conflict of interest.
We thank Dr. Lionello Bossi, Dr. Nara Figueroa-Bossi, and Dr. Felipe Cabello for valuable comments on the manuscript. We thank Mr. Víctor Ahumada for technical assistant. Part of the results included in this manuscript were presented during the XXXVIII Meeting of the Chilean Society for Microbiology 2016, Valdivia, Chile.
The Supplementary Material for this article can be found online at: https://www.frontiersin.org/articles/10.3389/fmicb.2020.00428/full#supplementary-material
Baquero, F., and Martínez, J.-L. (2017). Interventions on metabolism: making antibiotic-susceptible bacteria. MBio 8:e01950-17. doi: 10.1128/mbio.01950-17
Bhagirath, A. Y., Li, Y., Patidar, R., Yerex, K., Ma, X., Kumar, A., et al. (2019). Two component regulatory systems and antibiotic resistance in gram-negative pathogens. Int. J. Mol. Sci. 20:781. doi: 10.3390/ijms20071781
Cabello, F. (2018). Typhoid fever in Chile. Am. J. Trop. Med. Hyg. 99, 1649–1650. doi: 10.4269/ajtmh.18-0677
CLSI, (2012a). Methods for Dilution Antimicrobial Susceptibility Tests for Bacteria That Grow Aerobically; Approved Standard, 9th Edn. Wayne, PA: CLSI.
CLSI, (2012b). Performance Standards for Antimicrobial Disk Susceptibility Tests: Approved Standard. Wayne, PA: CLSI.
Cottarel, G., and Wierzbowski, J. (2007). Combination drugs, an emerging option for antibacterial therapy. Trends Biotechnol. 25, 547–555. doi: 10.1016/j.tibtech.2007.09.004
Cui, W.-Q., Qu, Q.-W., Wang, J.-P., Bai, J.-W., Bello-Onaghise, G., Li, Y.-A., et al. (2019). Discovery of potential anti-infective therapy targeting glutamine synthetase in Staphylococcus xylosus. Front. Chem. 7:381. doi: 10.3389/fchem.2019.00381
Datsenko, K. A., and Wanner, B. L. (2000). One-step inactivation of chromosomal genes in Escherichia coli K-12 using PCR products. Proc. Natl. Acad. Sci. U.S.A. 97, 6640–6645. doi: 10.1073/pnas.120163297
El Khoury, J. Y., Boucher, N., Bergeron, M. G., Leprohon, P., and Ouellette, M. (2017). Penicillin induces alterations in glutamine metabolism in Streptococcus pneumoniae. Sci. Rep. 7:14587. doi: 10.1038/s41598-017-15035-y
Fajardo, A., Martínez-Martín, N., Mercadillo, M., Galán, J. C., Ghysels, B., Matthijs, S., et al. (2008). The neglected intrinsic resistome of bacterial pathogens. PLoS One 3:e1619. doi: 10.1371/journal.pone.0001619
Gallo-Oller, G., Ordoñez, R., and Dotor, J. (2018). A new background subtraction method for Western blot densitometry band quantification through image analysis software. J. Immunol. Methods 457, 1–5. doi: 10.1016/j.jim.2018.03.004
González-Bello, C. (2017). Antibiotic adjuvants – A strategy to unlock bacterial resistance to antibiotics. Bioorganic Med. Chem. Lett. 27, 4221–4228. doi: 10.1016/j.bmcl.2017.08.027
Gustafson, J., Strassle, A., Hachler, H., Kayser, F. H., and Berger-Bachi, B. (1994). The femC locus of Staphylococcus aureus required for methicillin resistance includes the glutamine synthetase operon. J. Bacteriol. 176, 1460–1467. doi: 10.1128/jb.176.5.1460-1467.1994
Harth, G., and Horwitz, M. A. (2003). Inhibition of Mycobacterium tuberculosis glutamine synthetase as a novel antibiotic strategy against tuberculosis: demonstration of efficacy in vivo. Infect. Immun. 71, 456–464. doi: 10.1128/IAI.71.1.456-464.2003
Hidalgo, A. A., Villagra, N. A., Jerez, S. A., Fuentes, J. A., and Mora, G. C. (2016). A conditionally lethal mutant of Salmonella Typhimurium induces a protective response in mice. Biochem. Biophys. Res. Commun. 470, 313–318. doi: 10.1016/j.bbrc.2016.01.058
Ikeda, T. P., Shauger, A. E., and Kustu, S. (1996). Salmonella typhimurium apparently perceives external nitrogen limitation as internal glutamine limitation. J. Mol. Biol. 259, 589–607. doi: 10.1006/jmbi.1996.0342
Johnson, R., Mylona, E., and Frankel, G. (2018). Typhoidal Salmonella: distinctive virulence factors and pathogenesis. Cell. Microbiol. 20:e12939. doi: 10.1111/cmi.12939
Klemm, E. J., Shakoor, S., Page, A. J., Qamar, F. N., Judge, K., Saeed, D. K., et al. (2018). Emergence of an extensively drug-resistant Salmonella enterica serovar typhi clone harboring a promiscuous plasmid encoding resistance to fluoroquinolones and third-generation cephalosporins. MBio 9:e00105-18. doi: 10.1128/mbio.00105-18
Lima, T. B., Pinto, M. F. S., Ribeiro, S. M., Lima, L. A., De, Viana, J. C., et al. (2013). Bacterial resistance mechanism: what proteomics can elucidate. FASEB J. 27, 1291–1303. doi: 10.1096/fj.12-221127
Liu, A., Tran, L., Becket, E., Lee, K., Chinn, L., Park, E., et al. (2010). Antibiotic sensitivity profiles determined with an Escherichia coli gene knockout collection: generating an antibiotic bar code. Antimicrob. Agents Chemother. 54, 1393–1403. doi: 10.1128/AAC.00906-09
Lobos, S. R., and Mora, G. C. (1991). Alteration in the electrophoretic mobility of OmpC due to variations in the ammonium persulfate concentration in sodium dodecyl sulfate−polyacrylamide gel electrophoresis. Electrophoresis 12, 448–450. doi: 10.1002/elps.1150120615
Maria-Neto, S., Cândido, E., de, S., Rodrigues, D. R., de Sousa, D. A., da Silva, E. M., et al. (2012). Deciphering the magainin resistance process of Escherichia coli strains in light of the cytosolic proteome. Antimicrob. Agents Chemother. 56, 1714–1724. doi: 10.1128/aac.05558-11
Morillon, A. (2018). “Non-coding RNA, its history and discovery timeline,” in Long Non-coding RNA, ed. M. R. S. Rao, (Amsterdam: Elsevier), 1–24. doi: 10.1016/b978-1-78548-265-6.50001-0
Murima, P., McKinney, J. D., and Pethe, K. (2014). Targeting bacterial central metabolism for drug development. Chem. Biol. 21, 1423–1432. doi: 10.1016/j.chembiol.2014.08.020
Nikaido, H. (2003). Molecular basis of bacterial outer membrane permeability revisited. Microbiol. Mol. Biol. Rev. 67, 593–656. doi: 10.1128/mmbr.67.4.593-656.2003
Peng, B., Su, Y., Bin, Li, H., Han, Y., Guo, C., et al. (2015). Exogenous alanine and/or glucose plus kanamycin kills antibiotic-resistant bacteria. Cell Metab. 21, 249–262. doi: 10.1016/j.cmet.2015.01.008
Pfaffl, M. W. (2001). A new mathematical model for relative quantification in real-time RT-PCR. Nucleic Acids Res. 29, e45.
Rodas, P. I., Contreras, I., and Mora, G. C. (2010). Salmonella enterica serovar Typhi has a 4.1 kb genetic Island inserted within the sapABCDF operon that causes loss of resistance to the antimicrobial peptide protamine. J. Antimicrob. Chemother. 65, 1624–1630. doi: 10.1093/jac/dkq197
Sabbagh, S. C., Lepage, C., McClelland, M., and Daigle, F. (2012). Selection of salmonella enterica serovar typhi genes involved during interaction with human macrophages by screening of a transposon mutant library. PLoS One 7:e36643. doi: 10.1371/journal.pone.0036643
Shimizu, K. (2013). Metabolic regulation of a bacterial cell system with Emphasis on Escherichia coli metabolism. ISRN Biochem. 2013:645983. doi: 10.1155/2013/645983
Switzer, A., Brown, D. R., and Wigneshweraraj, S. (2018). New insights into the adaptive transcriptional response to nitrogen starvation in Escherichia coli. Biochem. Soc. Trans. 46, 1721–1728. doi: 10.1042/bst20180502
Valenzuela, C., Ugalde, J. A., Mora, G. C., Alvarez, S., Contreras, I., and Santiviago, C. A. (2014). Draft genome sequence of Salmonella enterica serovar typhi strain STH2370. Genome Announc. 2, e104–e114. doi: 10.1128/genomeA.00104-14
van Heeswijk, W. C., Westerhoff, H. V., and Boogerd, F. C. (2013). Nitrogen assimilation in Escherichia coli: putting molecular data into a systems perspective. Microbiol. Mol. Biol. Rev. 77, 628–695. doi: 10.1128/mmbr.00025-13
Vestergaard, M., Nøhr-Meldgaard, K., Bojer, M. S., Krogsgård Nielsen, C., Meyer, R. L., Slavetinsky, C., et al. (2017). Inhibition of the ATP synthase eliminates the intrinsic resistance of staphylococcus aureus towards polymyxins. MBio 8:e01114-17. doi: 10.1128/mbio.01114-17
Villarreal, J. M., Becerra-Lobato, N., Rebollar-Flores, J. E., Medina-Aparicio, L., Carbajal-Gómez, E., Zavala-García, M. L., et al. (2014). The Salmonella enterica serovar Typhi ltrR-ompR-ompC-ompF genes are involved in resistance to the bile salt sodium deoxycholate and in bacterial transformation. Mol. Microbiol. 92, 1005–1024. doi: 10.1111/mmi.12610
Zimmer, D. P., Soupene, E., Lee, H. L., Wendisch, V. F., Khodursky, A. B., Peter, B. J., et al. (2000). Nitrogen regulatory protein C-controlled genes of Escherichia coli: scavenging as a defense against nitrogen limitation. Proc. Natl. Acad. Sci. U.S.A. 97, 14674–14679. doi: 10.1073/pnas.97.26.14674
Keywords: glnA, ciprofloxacin, OmpF, S. Typhi, transposon
Citation: Millanao AR, Mora AY, Saavedra CP, Villagra NA, Mora GC and Hidalgo AA (2020) Inactivation of Glutamine Synthetase-Coding Gene glnA Increases Susceptibility to Quinolones Through Increasing Outer Membrane Protein F in Salmonella enterica Serovar Typhi. Front. Microbiol. 11:428. doi: 10.3389/fmicb.2020.00428
Received: 30 July 2019; Accepted: 27 February 2020;
Published: 20 March 2020.
Edited by:
Kunihiko Nishino, Osaka University, JapanReviewed by:
Ricardo Oropeza, National Autonomous University of Mexico, MexicoCopyright © 2020 Millanao, Mora, Saavedra, Villagra, Mora and Hidalgo. This is an open-access article distributed under the terms of the Creative Commons Attribution License (CC BY). The use, distribution or reproduction in other forums is permitted, provided the original author(s) and the copyright owner(s) are credited and that the original publication in this journal is cited, in accordance with accepted academic practice. No use, distribution or reproduction is permitted which does not comply with these terms.
*Correspondence: Alejandro A. Hidalgo, YWxlamFuZHJvLmhpZGFsZ29AdW5hYi5jbA==
Disclaimer: All claims expressed in this article are solely those of the authors and do not necessarily represent those of their affiliated organizations, or those of the publisher, the editors and the reviewers. Any product that may be evaluated in this article or claim that may be made by its manufacturer is not guaranteed or endorsed by the publisher.
Research integrity at Frontiers
Learn more about the work of our research integrity team to safeguard the quality of each article we publish.