- 1College of Pharmacy and Nutrition, University of Saskatchewan, Saskatoon, SK, Canada
- 2Department of Orthopedics, University Medical Center Utrecht, Utrecht, Netherlands
- 3Department of Medicine, Albert Einstein College of Medicine, The Bronx, NY, United States
- 4Western College of Veterinary Medicine, University of Saskatchewan, Saskatoon, SK, Canada
Invasive fungal infections (IFI) cause devastating morbidity and mortality, with the number of IFIs more than tripling since 1979. Our laboratories were the first to demonstrate that radiolabeled microorganism-specific monoclonal antibodies are highly effective for treatment of experimental fungal, bacterial and viral infections. Later we proposed to utilize surface expressed pan-antigens shared by major IFI-causing pathogens such as beta-glucans as RIT targets. Here we evaluated in vivo RIT targeting beta-glucan in Blastomyces dermatitidis which causes serious infections in immunocompromised and immunocompetent individuals and in companion dogs. B. dermatitidis cells were treated with the 400-2 antibody to (1→3)-β-glucans radiolabeled with the beta-emitter 177Lutetium (177Lu) and alpha-emitter 213Bismuth (213Bi) and the efficacy of cell kill was determined by colony forming units (CFUs). To determine the antigen-specific localization of the 400-2 antibody in vivo, C57BL6 mice were infected intratracheally with 2 × 105 B. dermatitidis cells and given 111In-400-2 antibody 24 h later. To evaluate the killing of B. dermatitidis cells with RIT, intratracheally infected mice were treated with 150 μCi 213Bi-400-2 and their lungs analyzed for CFUs 96 h post-infection. 213Bi-400-2 proved to be more effective in killing B. dermatitidis cells in vitro than 177Lu-400-2. Three times more 111In-400-2 accumulated in the lungs of infected mice, than in the non-infected ones. 213Bi-400-2 lowered the fungal burden in the lungs of infected mice more than 2 logs in comparison with non-treated infected controls. In conclusion, our results demonstrate the ability of an anti-(1-3)-beta-D-glucan antibody armed with an alpha-emitter 213Bi to selectively kill B. dermatitidis cells in vitro and in vivo. These first in vivo results of the effectiveness of RIT targeting pan-antigens on fungal pathogens warrant further investigation.
Introduction
Invasive fungal infections (IFI) cause devastating morbidity and mortality, especially in organ transplant patients, cancer patients and patients in intensive care units, with the number of IFIs more than tripling since 1979 (Enoch et al., 2017). There are three main categories of medications used for the treatment of IFI: polyenes (primarily amphotericin B), azoles (fluconazole, itraconazole, voriconazole, posaconazole), and echinocandins (caspofungin, micafungin, anidulafungin). Additionally, flucytosine is primarily utilized in combination with amphotericin B for the treatment of cryptococcosis. Hence, the number of medications available to combat mycostic infections is significantly less than what is available for treating bacterial diseases and the number of targets of these antifungal medications are far fewer (polyenes and azoles: cell membrane sterols; echinocandins: cell wall beta-1,3-glucans; flucytosine: RNA, DNA). Furthermore, the echinocandins, the last new class approved by the Food and Drug Administration (FDA), have been available for ∼10 years with no appearance of new drug classes for the severe fungal diseases.
Radioimmunotherapy (RIT) utilizes antigen-antibody interaction to deliver lethal doses of ionizing radiation to cells and has demonstrated efficacy in several types of cancer (Tomblyn, 2012; Larson et al., 2015). The distinct advantages of RIT over other drugs are: (1) its cytocidal nature, meaning that RIT does not merely abrogate a single cellular pathway but physically destroys targeted cells or cellular machinery; (2) it is less subject to drug resistance mechanisms; (3) its efficacy is independent of the immune status of a host; and (4) it has low toxicity in comparison to chemotherapy due to the specific tumor targeting.
Our laboratories were the first to demonstrate that microorganism-specific monoclonal antibodies (mAbs) are highly effective for the treatment of experimental fungal, bacterial and viral infections (Dadachova et al., 2003; reviewed in Helal and Dadachova, 2018) as well as virally induced cancers (Wang et al., 2007; Phaeton et al., 2016). Using Cryptococcus neoformans as a model organism and the antibodies to its polysaccharide capsule as targeting molecules for the radionuclides we investigated various aspects of RIT of fungal disease such as long term efficacy of the treatment with infected mice being observed for up to 75 days post RIT, high inoculum, acute versus established infection (Dadachova et al., 2003; Bryan et al., 2009; Jiang et al., 2012). We also investigated the radiobiological and immunological mechanisms of actions of infections RIT (Dadachova et al., 2006a, b; Bryan et al., 2008). Several years ago, we proposed that we can utilize surface-expressed antigens shared by major IFI-causing pathogens as targets for radiolabeled mAbs (Nosanchuk and Dadachova, 2012). This approach is different than that utilized to date in cancer RIT, where the selected mAbs target a specific cell type. Fortunately, IFI-causing fungi do share common cell wall associated antigens that also constitute major virulence factors for these fungi, which we called “pan-antigens,” such as melanin, heat shock protein 60 (HSP60) (Guimarães et al., 2009) and beta-glucans. These antigens are exposed on the surface of fungal cells and thus are accessible to the radiolabeled mAbs for binding and delivering cytocidal payloads to those cells. Using C. neoformans and Candida albicans as model organisms, our in vitro experiments showed that antibodies to the above pan-antigens killed 80–100% of fungal cells when radiolabeled with alpha-particles emitting radionuclide 213Bismuth (Bryan et al., 2012).
In this work, we provide first experimental in vivo evidence that pan-antigens on IFI causing pathogens can be targeted with RIT. Blastomycosis dermatitidis was chosen as a model for this project. It is an example of an invasive fungal pathogen that causes serious infections in immunocompromised patients (Pappas et al., 1993), immunocompetent individuals (Gray and Baddour, 2002) and in companion dogs (Davies et al., 2013). It has relatively high prevalence in different areas in Canada and the United States (Davies et al., 2013) and the endemic regions may be increasing, as evidenced by reports in New York, Vermont, Texas, Nebraska, and Kansas (McDonald et al., 2018). We have chosen (1→3)-β-glucan as an RIT target because of the encouraging results in our in vitro work (Bryan et al., 2012) and because the antibodies to this antigen are commercially available. We have demonstrated that those antibodies in the radiolabeled form bind specifically to B. dermatitidis cells in vitro and in vivo and that their administration to infected mice results in several logs reduction of the infectious burden.
Materials and Methods
Fungal Cultures and Antibodies
Acapsular C. neoformans (ATCC 208821) cells were cultured in Emmons’ modification of Sabouraud’s agar overnight (37°C) until sufficient cell numbers were reached. Wild type strain of B. dermatitidis Gilchrist et Stokes (ATCC 26199) was used in all experiments. As 26199 strain is a Biological Safety Level (BSL) 3 pathogen in Canada, all manipulations with the cells were conducted using standard operating procedures which enhanced BSL2 (BSL2 +) facilities with the approval by the Public Health Agency of Canada and by the Biosafety Office at University of Saskatchewan. To prevent the formation of the potentially infectious spores, the cells were kept at 37°C at all times except when frozen for storage. B. dermatitidis cells were cultured in Histoplasma macrophage medium (HMM) (Worsham and Goldman, 1988), at 37°C for 5–7 days until sufficient numbers of cells were reached. The media was prepared by dissolving glucose (18.2 g), glutamic acid (1.0 g), cysteine (1.0 g) and HEPES (6.0 g) in 1 L of water. The solution was filter sterilized followed by the addition of 10.6 g of F-12 Nutrient mixture (cat. number: N6760, Sigma). Passage 2–4 were used throughout the work. Agar was prepared by suspending 19.47 g of MiddleBrook 7H10 Agar Base (cat. number: M0303, Sigma) in 900 ml distilled water followed by the addition of 5 ml glycerol. The mixture was then autoclaved and left at room temperature to cool down. Dubos Oleic Albumin Complex (cat. number: 215333, BD Difco) was added as a media additive to enhance the growth of B. dermatitidis. The agar was poured in Petri dishes and left to solidify. The dishes were left at 4°C until needed. Murine IgG2b mAb 2G8 to (1→3)-β-glucan (Torosantucci et al., 2005) was produced by Diatheva Srl, Cartoceto, Italy, and was a kind gift from Dr. A. Torosantucci (Istituto Superiore di Sanità, Rome, Italy). Its Kd is 1.34 × 10–9 M according to the manufacturer’s data. 400-2 murine IgG1 mAb to (1→3)-β-glucan was procured from Biosupplies Australia Pty. Ltd. (Parkville, Australia). The murine isotype control IgG1 antibody MOPC-21 was procured from Invitrogen (Waltham, United States).
Radionuclides and Radiolabeling of Antibodies
400-2, 2G8 and MOPC21 antibodies were first conjugated to a chelating agent C-functionalized trans-cyclohexyldiethylene-triamine pentaacetic acid derivative (CHXA″) (Macrocyclics, San Antonio, TX, United States) with a 10 molar excess to enable its subsequent radiolabeling with 111In, 177Lu or 213Bi. Conjugation and labeling was carried out according to a previously published method (Allen et al., 2018, 2019). In short, the conjugation reaction was carried out for 1.5 h at 37°C in sodium carbonate buffer at pH 8.5. The reaction was followed by exchanging the buffer into 0.15 M ammonium acetate buffer, pH 6.5. 225Ac for construction of the 213Bi/225Ac radionuclide generator was purchased from Oak Ridge National Laboratory, TN, United States. 177Lu in form of 177Lu chloride was acquired from RadioMedix (TX, United States), and 111In chloride – from BWXT Canada. The CHXA″- conjugated antibodies were radiolabeled with 111In, 177Lu and 213Bi. The radiolabeling of CHXA″ conjugates with 111In and 177Lu was performed to achieve the specific activity of 5 μCi/μg of the antibody. 800 μCi of 111In or 177Lu chloride was added to 10 μL 0.15 M ammonium acetate buffer and added to a microcentrifuge tube containing 160 μg of the desired CHXA″ conjugated antibody in 0.15 M ammonium acetate buffer. The reaction mixture was incubated for 60 min at 37°C, and then the reaction was quenched by the addition of 3 μL of 0.05 M EDTA solution. For radiolabeling with 213Bi, it was eluted from a 213Bi/225Ac radionuclide generator with a 300 μL 0.1 M hydroiodic acid (HI) solution followed by 300 μL milliQ H2O. The pH of the solution was adjusted to 6.5 with 80 μL of 5 M ammonium acetate buffer prior to addition to the desired CHXA″ conjugated antibody and the reaction mixture was incubated for 5 min at 37°C. The 213Bi-labeled mAbs were purified from HI on a 0.5 mL Amicon disposable size exclusion filter (30 K MW cut off, Fisher). The percentage of radiolabeling was measured by QQsilica gel-instant thin layer chromatography (SG-iTLC) using 0.15 M ammonium acetate buffer as the eluent (top containing unlabeled 111In/213Bi/177L, bottom containing radiolabeled antibody). SG-iTLCs were read on a PerkinElmer 2470 Automatic Gamma Counter. Greater than 98% labeling was routinely achieved and 111In- and 177Lu-labeled mAbs were used immediately with no need for further purification.
Determination of the Binding Ability of Radiolabeled Antibodies to Fungal Cell Surfaces
We radiolabeled fungal beta-glucan-specific (400-2 or 2G8) mAbs with 177Lu. Five million acapsular C. neoformans cells were incubated for 3 h (37°C) with a radiolabeled mAb in albumin-pre-coated Eppendorf tubes. A radiolabeled isotype antibody (177Lu-MOPC-21) was also used as a control. In addition, samples with radiolabeled mAb without cells were used to rule out non-specific binding to the plastic tubes. Radiolabeled antibody at a specific activity of 5:1 (25 μCi or 50 μCi radioactivity) were used in this experiment. Following incubation, the tube with the cells and the radiolabeled antibody was measured using PerkinElmer© Gamma Counter. The cells were then centrifuged, the supernatant was removed and its radioactivity measured in a gamma counter. The cellular pellet was washed twice with phosphate buffered saline (PBS), and the radioactivity of the pellet was measured in a gamma counter. The percentages of the radiolabeled antibody bound to the cells and of the unbound antibody were calculated using the following formulae:
Determination of RIT Cytotoxicity Against B. dermatitidis
104 B. dermatitidis cells were incubated with 213B-400-2 mAb (1 or 5 μCi at a specific activity of 5 μCi/μg) or 177Lu 400-2 (20 μCi at a the same specific activity) for 1 h. MOPC-21 was used as an isotype control. After 1 h incubation, the cells were centrifuged and washed to remove any radioactivity. Cells were then cultured on MiddleBrook agar at 37°C for 3 days 37°C after which the colony forming units (CFUs) were counted in each group and the percentages of cell survival were calculated.
Biodistribution of the Radiolabeled 400-2 Antibody in B. dermatitidis Infected and Healthy Mice
All animal studies were approved by the Animal Research Ethics Board of the University of Saskatchewan. The objective of this experiment was to determine if the 111In-400-2 mAb accumulated in the B. dermatitidis infected lungs in mice to a higher extent than in non-infected animals. Twenty female black C57B16 mice (4–6 weeks old) were randomized into 4 groups (each with 5 mice). Only groups one and two were infected intratracheally with 2 × 105 B. dermatitidis cells while groups three and four received PBS alone Mice received isoflurane with oxygen for anesthesia purposes to enable the intratracheal infection. After 24 h, all mice received intraperitoneal injection of 111In-400-2 antibody (30 μCi). Groups one and three were euthanized at 48 h post-infection while groups two and four were euthanized at 96 h post-infection. Lungs were then isolated. Radioactivity was then measured for each lung tissue using PerkinElmer© Gamma Counter in reference to the radiolabeled antibody standard as described (Allen et al., 2018). Radioactivity was then determined per unit weight of each isolated lung.
Radioimmunotherapy of B. dermatitidis-Infected Mice
This experiment was conducted to measure the in vivo effectiveness of RIT in treating mice infected with B. dermatitidis. For this, 27 female black C57B16 mice were infected intratracheally with 106 B. dermatitidis cells. Twenty four hours after the infection, mice were randomized into 4 groups. The first (9 mice) and second (5 mice) groups received 150 μCi 213Bi-400-2 and 150 μCi 213Bi-MOPC-21, respectively. The third group (8 mice) received no treatment, while the fourth group (5 mice) received only cold 400-2 antibody (30 μg). All treatments were given intraperitoneally. A fifth group consisted of two healthy mice which received neither infection or treatment. Mice were observed for 72 h post treatment and then euthanized. Lungs were isolated and passed through cell strainers. Aseptic conditions were maintained through all the procedures. The cells were centrifuged, concentrated in 700 μL PBS and plated on MiddleBrook agar for 24 h at 37°C. ImageJ software was then used to calculate the area on the colonies formed on the Petri dish in each sample. For the same dish, the average areas of 10 random colonies was also determined. Total area was divided by the average colony area to estimate the number of colonies formed for each plate. In addition, the aliquots of homogenized lungs were obtained from four mice in untreated infected control and 213Bi-400-2 groups. DNA was then isolated from the samples using GenomicPrep Mini Spin kit (Cat. Number 28-9042-58) according to the manufacturer protocol. The levels of BAD1 and C57BL/6L genes were quantified in each sample using real-time PCR system (Bio-Rad CFX96 Real Time System) and SYBR Master Mix. Levels of BAD1 and C57BL/6L correlate to those of B. dermatitidis and mouse cells, respectively. Primers for BAD1 were forward (5′-AAGTGGCTGGGTAGTTATACGCTAC-3′) and reverse (5′-TAGGTTGCTGATTCCATAAGTCAGG-3′ primers) (Sidamonidze et al., 2012) while a genomic marker found in C57BL/6J mice was detected using the forward (5′-AACTCTCAGGGGTCCTGTGT-3′ and reverse (5′-CCTGGGC CTCACTTATTGGG-3′) primers. The quantity of BAD1 was then referenced to that of C57BL/6L genomic marker in both control and 213Bi-400-2 groups.
Statistical Analysis
The data are presented as mean ± standard deviation (SD). One-way ANOVA followed by Tukey’s post hoc test was employed to determine the significant difference between the different groups using GraphPad Prism 5 software (San Diego, CA, United States). A p-value less than 0.05 was considered significant.
Results
The Antibodies to (1→3)-β-Glucan Preserved Their Immunoreactivity After Radiolabeling
Initially, the immunoreactivity toward (1→3)-β-glucan antigen of 400-2 and 2G8 mAbs radiolabeled with 111In was determined by binding to acapsular C. neoformans cells. Results show that both 400-2 and 2G8 antibodies were able to bind significantly higher than the MOPC-21 irrelevant control mAb (Figure 1A) while there was no binding of 400-2 and 2G8 antibodies to the plates alone without the cells. Conversely, there was significantly more non-bound antibody in case of MOPC-21 than 400-2 or 2G8 (Figure 1B). There were approximately 4 × 1013 antibody molecules in 10 μg of the antibody added to 5 × 106 cryptococcal cells. If to assume that every cell expresses around 104 antigen binding sites, it means that around 16 and 50 antibody molecules were bound to every cryptococcal cell for 400-2 and 2G8 mAbs, respectively. As Kd for 2G8 is 1.34 × 10–9 M according to the manufacturer, Kd for 400-2 was estimated from the binding data to be 4 × 10–9 M. As commercial availability of 2G8 antibody for further work was limited, 400-2 mAb was utilized in all subsequent experiments.
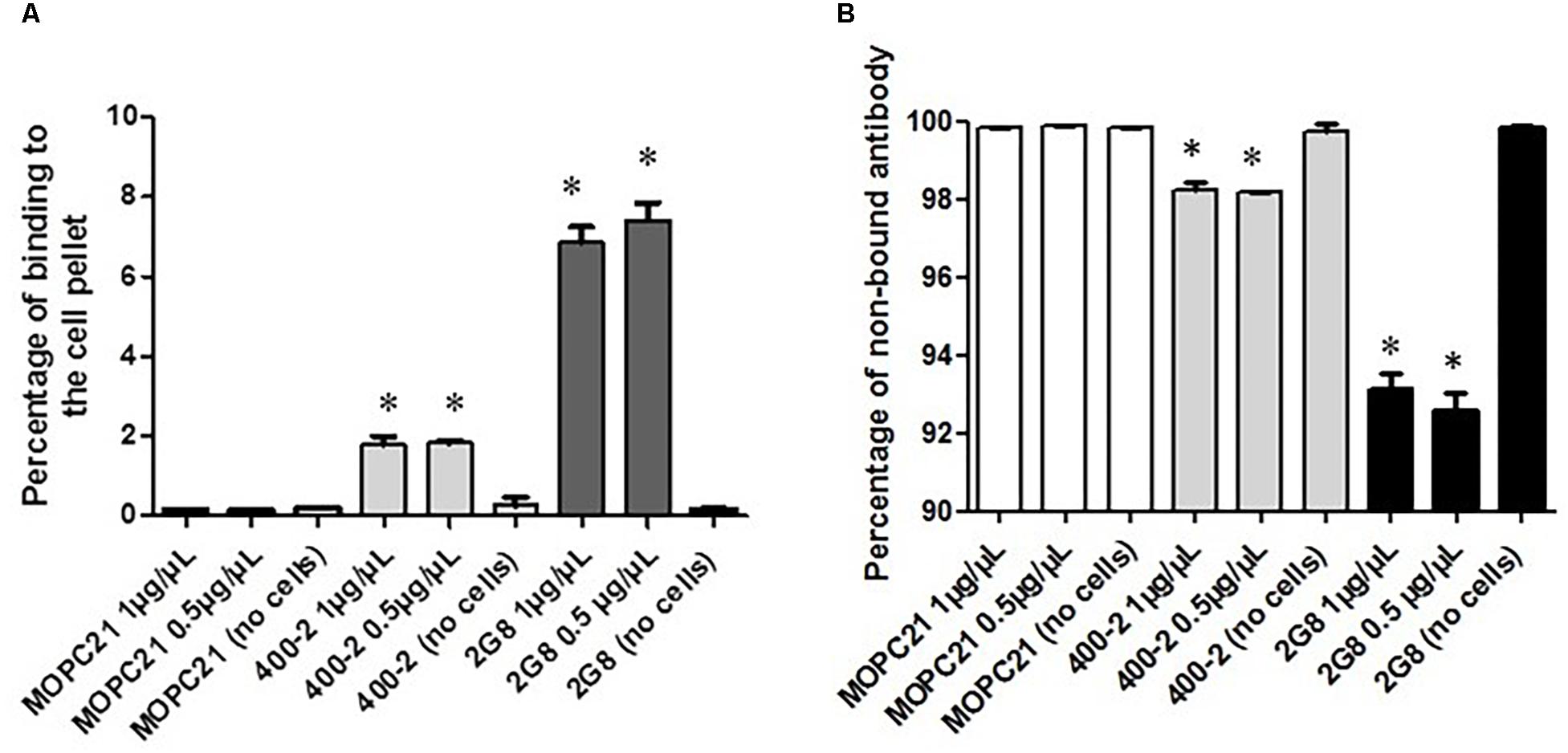
Figure 1. Evaluation of the binding of 177Lu-400-2 and 177Lu-2G8 antibodies to (1→3)-β-glucans antigen after their incubation with acapsular C. neoformans cells for 3 h. 177Lu-MOPC 21 was used as an IgG irrelevant control. (A) percentage of radiolabeled antibody in a sample bound to the cell pellet: (B) percentage of non-bound antibody. ∗Indicates a significant difference between the groups (P value < 0.05).
Radiolabeled mAb 400-2 Specifically Killed B. dermatitidis Cells in vitro
In vitro results show a significantly higher reduction in cell survival when treated with an alpha-emitter labeled 213Bi-400-2 (1 μCi) antibody compared to 213Bi-MOPC-21 (1 μCi), cold 400-2 and PBS controls (Figure 2A). No significant difference was observed between 213Bi-400-2 and 213Bi-MOPC-21 treated cells when the radioactivity was 5 μCi, which can be explained by non-specific killing due to the high radioactivity concentration in a small volume. When a beta-emitter 177Lu was used for radiolabeling, 177Lu-400-2 mAb also demonstrated significant cell killing in comparison to 177Lu-MOPC-21, cold 400-2 and PBS controls (Figure 2B).
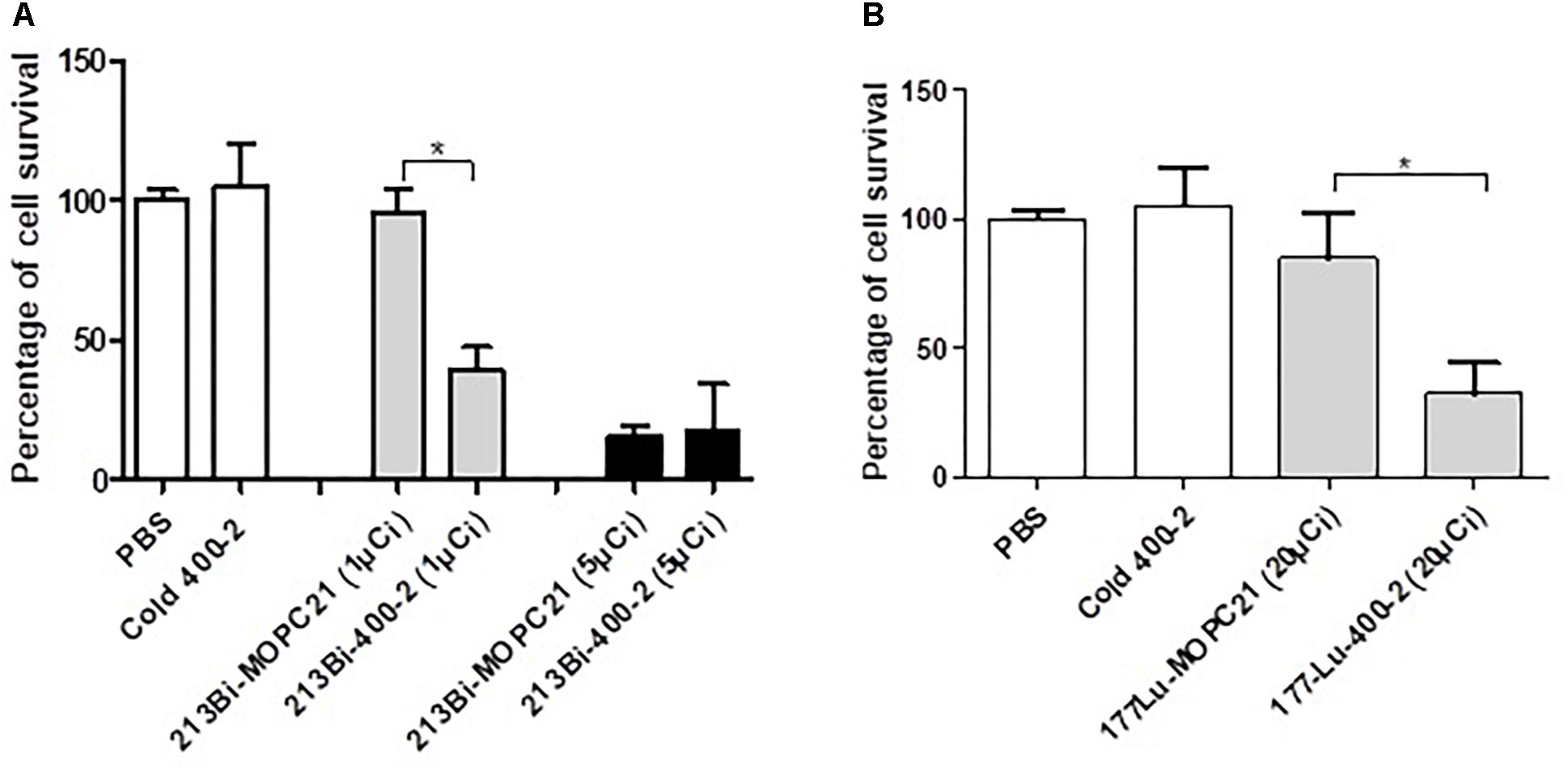
Figure 2. Cytotoxicity of radioimmunotherapy (RIT) toward B. dermatitidis. (A) 213Bi-400-2 mAb; (B) 177Lu-400-2 mAb. Cells were incubated with the radiolabeled antibodies for 1 h at 37°C followed by plating for determination of CFUs. Samples treated with PBS, cold 400-2 mAb and 213Bi- or 177Lu–labeled MOPC-21 mAb were used as controls. *Indicates a significant difference between the groups (P value < 0.05).
Biodistribution of Radiolabeled mAb 400-2 in Infected and Healthy Mice Showed Preferential Uptake in the Infected Lungs
The biodistribution of 111In-400-2 in B. dermatitidis-infected and healthy mice was performed to evaluate the ability of 400-2 mAb to bind to B. dermatitidis in the lungs of infected mice. Our results showed significantly higher accumulation of 111In-400-2 in the lungs of infected mice compared to non-infected ones at 24 h post-injection of the antibody (Figure 3) with the uptake equalizing between infected and non-infected lungs at 72 h after antibody administration. There was no significant difference between injected dose per gram in the blood of infected and non-infected mice at both the 24 and 72 h time points (Figure 3).
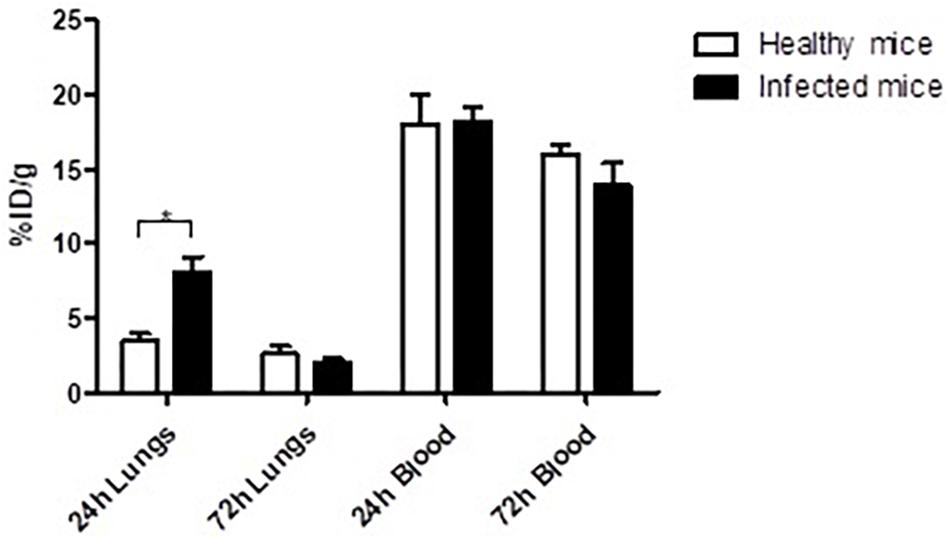
Figure 3. Biodistribution of 111In-400-2 mAb in B. dermatitidis-infected and in healthy mice. C57Bl6 mice were infected intratracheally with B. dermatitidis 2 × 105 cells, injected IP with 111In-400-2 mAb 24 h after infection, and euthanized at 24 or 72 h post antibody administration. Five animals per group were used. %ID/g represents the percentage of injected dose of the radiolabeled antibody per gram tissue (blood and lungs). *Indicates a significant difference between the groups (P value < 0.05).
RIT of B. dermatitidis-Infected Mice Reduced the Fungal Burden in the Lungs by Several Logs
This experiment was conducted to determine the ability of 213Bi-labeled 400-2 mAb to reduce the burden of infection in B. dermatitidis-infected mice. Results demonstrated that single administration of 213Bi-400-2 mAb resulted in more than 2 logs reduction in CFUs in comparison with untreated controls and in more than 1 log reduction – in comparison with the unlabeled (“cold”) and 213Bi-MOPC-21 groups (Figures 4A–F). The cold and 213Bi-MOPC-21 antibodies treatments reduced the CFUs by 1 log, this is probably attributable to opsonisation and non-specific radiation killing of fungal cells, respectively. However, the decrease in fungal burden in control groups was significantly (p < 0.05) less than what was observed in the 213Bi-400-2 group. The PCR results show that B. dermatitidis BAD1 gene levels were significantly (p = 0.001) lowered in the lungs of mice that received 213Bi-400-2 treatment in comparison with the untreated infected controls (Figure 4G).
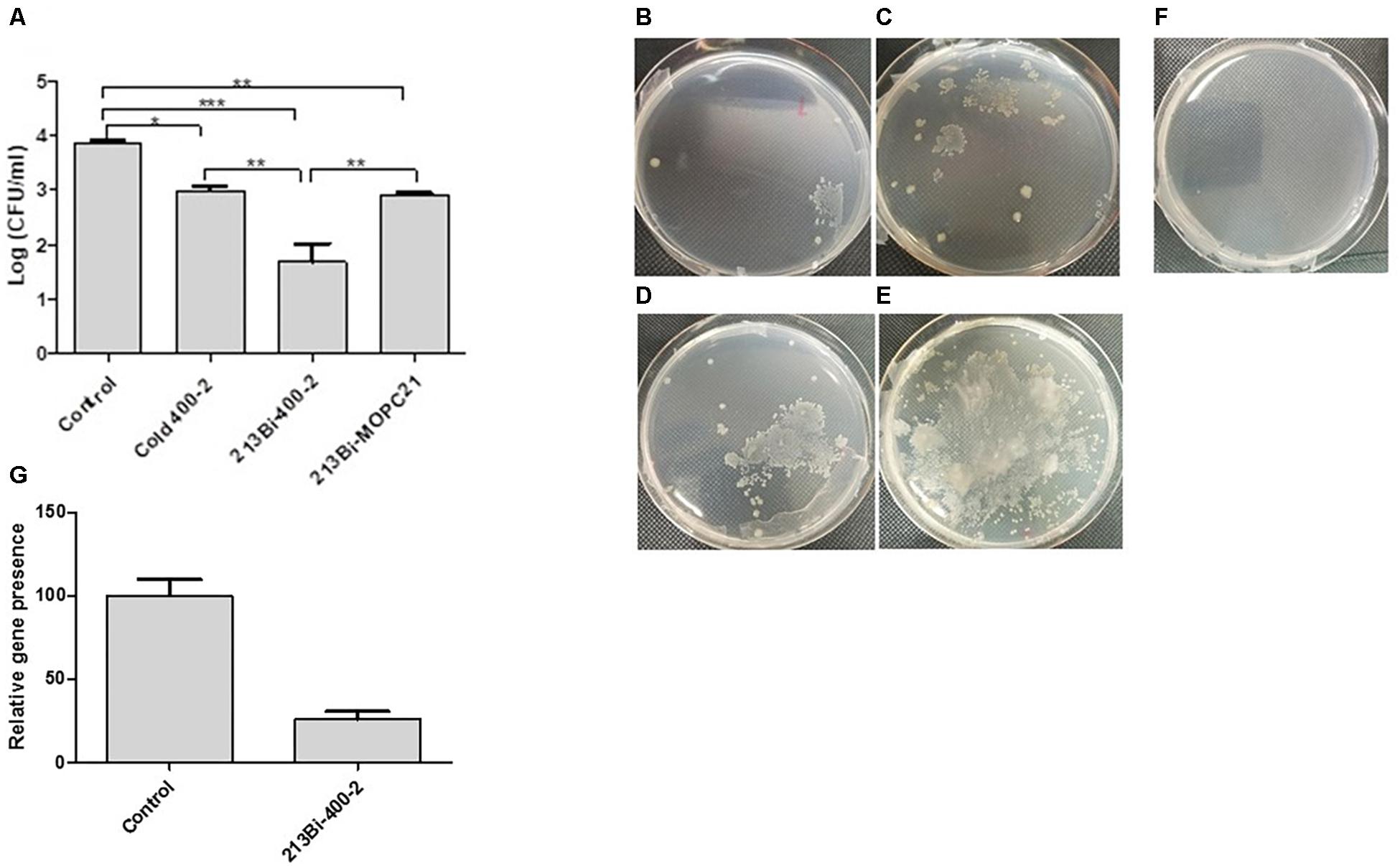
Figure 4. Radioimmunotherapy (RIT) of B. dermatitidis infected mice. (A) CFUs in the lungs of the mice infected with 106 B. dermatitidis cells for 24 h and then either left untreated (control) or treated intraperitoneally with 30 μg 400-2 cold antibody, 150 μCi 213Bi-400-2, or 150 μCi 213Bi-MOPC-21 and were euthanized at 96 h post-infection. * Indicates P value < 0.05; ** < 0.01; *** < 0.001. (B–F) images of representative plates seeded with the homogenates from lungs isolated from various treatment groups: (B) 213Bi-400-2; (C) 213Bi-MOPC-21; 1 (D) 400-2 cold antibody; (E) no treatment; (F) uninfected, untreated mice; (G) real time PCR results, showing B. dermatitidis BAD1 gene levels significantly (p = 0.001) lowered in the lungs of mice that received 213Bi-400-2 treatment in comparison with untreated infected controls.
Discussion
Our laboratories are developing RIT targeting pan-antigens found in all major human pathogenic fungi as a novel approach for the treatment of IFI (Bryan et al., 2012; Nosanchuk and Dadachova, 2012). This approach is a potential alternative to treat invasive infections that are difficult to cure with currently available drugs. In this study, we employed a radiolabeled antibody (213Bi-400-2) that targets fungal (1-3)-beta-D-glucan antigen for delivery of a potent radionuclide as a novel and potent treatment option to treat experimental blastomycosis in mice. Additionally, as (1-3)-beta-D-glucan is expressed in a wide range of fungal cells (Odabasi et al., 2006), we anticipate that radiolabeled antibodies to this antigen could be effective in treating a wide range of fungal diseases.
Before proceeding with in vivo experiments, we evaluated the specificity of 400-2 antibody binding to B. dermatitidis cells in vitro which was done in the absence of mammalian cells (Figure 1). 400-2 antibody to beta-glucan demonstrated binding to B. dermatitidis cells while the control antibody MOPC-21 – did not. These results provided reassurance that 400-2 is binding specifically to B. dermatitidis. We also compared the in vitro killing ability toward B. dermatitidis cells of 400-2 mAb when conjugated to two different radionuclides, an alpha emitter 213Bi and a beta emitter 177Lu. Although both radioisotopes were able to kill significant percentage of B. dermatitidis cells, 213Bi showed a higher potency than 177Lu. That is, 20 μCi 177Lu-400-2 was required to cause cell death equivalent to the damage caused by 1 μCi 213Bi-400-2. Interestingly, fungal cytotoxicity of radiolabeled 400-2 was observed despite the relatively low expression levels of (1-3)-beta-D-glucan in these cells (Girouard et al., 2007). These results are concordant with our previous results on in vitro killing with 213Bi-2G8 antibody of C. neoformans cells which also has low levels of (1-3)-beta-D-glucans and provide further evidence of the effectiveness of RIT in killing the targeted cells.
Our in vivo biodistribution results showed a significantly higher accumulation of 111In-400-2 antibody in the lungs of the infected mice compared to its accumulation in non-infected ones. This provides a strong evidence of the co-localization of the 400-2 antibody with B. dermatitidis cells. There was almost 3 times more 111In-400-2 mAb in the lungs of infected mice than non-infected once at 24 h post administration of the antibody (Figure 3). Similar results were observed during the biodistribution of capsular polysaccharide-targeting mAb 18B7 in mice infected intratracheally with C. neoformans (Dadachova et al., 2003). The decrease in lung uptake to the background levels in infected mice at 72 h post antibody administration was, most likely, due to the 400-2 mAb coming off (1-3)-beta-D-glucan. However, since the lungs will only experience high radiation doses in the first 4 h after administration of 213Bi-400-2 due to 213Bi short physical half-life of 46 min, the increased accumulation of the antibody in the lungs early after administration will be sufficient to deliver the cytocidal doses of radiation to B. dermatitidis cells.
The biodistribution results provided impetus to pursue RIT experiments. Treatment of infected mice with 213Bi-400-2 antibody was able to decrease the lungs CFUs more than 2 logs compared to non-treated infected mice (Figure 4). To the best of our knowledge, this is the first in vivo demonstration of the efficacy of RIT targeting fungal pan antigens. This 2 logs (1,000 fold) reduction in infection burden compared favorably with cancer RIT, which typically causes 5–100 fold decrease in the tumor size in comparison with untreated controls in experimental cancer models (Jiao et al., 2019; Quelven et al., 2019). In regard to the in vivo specificity of RIT, before performing therapy experiments, we demonstrated that 400-2 antibody was specific for beta-glucan both in vitro (cell binding and cell killing results), and in vivo, when it concentrated 3 times more in the lungs of the infected mice than non-infected mice during the biodistribution study. If it would be binding non-specifically to Fc receptors on resident macrophages and on other cells in the lungs, there would be no difference in the lungs uptake between infected and healthy mice, and the uptake in the infected lungs would be growing with time, as more inflammatory cells are moving in. 213Bi is a powerful alpha-emitter which can cause significant off target effects, including stimulation of immune system. Nevertheless, the effect of 213Bi-labeled control antibody on the infection burden was 10 times lower than that of 213Bi-400-2. This, in our opinion, is the most direct proof of the specificity of RIT treatment. The effect of the unlabeled 400-2 antibody on the fungal burden should be considered separately. In this regard, FDA approved radiolabeled antibody Zevalin for treatment of non-Hodgkin lymphoma is capable of inducing some ADCC which contributes to the antibody overall efficacy.
In the past we have performed extensive evaluation of RIT toxicity in mouse models of fungal infection using the same radioactive doses of 213Bi-labeled antibodies used in the current study. In this regard, the toxicity evaluation in RIT-treated mice infected intratracheally with C. neoformans showed the absence of acute hematologic and long-term pulmonary toxicity (Dadachova et al., 2004a,b,c). RIT proved to be much better tolerated by the infected mice than current standard of care Amphotericin B (Bryan et al., 2010). Finally, RIT spared bystander mammalian cells such as macrophages which were able to perform their functions such as nitric oxide production post-RIT exposure (Bryan et al., 2013). The technology of attaching radionuclides to mAbs is mature and can be easily translated from the cancer field into infectious diseases. Importantly, nuclear medicine departments in hospitals world-wide are now routinely treating cancer patients with RIT and are fully equipped for deployment of infectious diseases RIT. Portable imaging equipment is available for imaging patients receiving RIT to ascertain the targeting of radiolabeled mAbs and to monitor the progression of the disease.
In conclusion, our results demonstrate the ability of an anti-(1-3)-beta-D-glucan antibody armed with an alpha-emitter 213Bi to selectively kill B. dermatitidis cells in vitro and in vivo. These results provide encouraging first in vivo results of the effectiveness of RIT targeting pan-antigens on fungal pathogens. The goal of our group is to pursue a clinical trial of RIT in companion dogs with spontaneous blastomycosis; this research is in progress.
Data Availability Statement
The datasets generated for this study are available on request to the corresponding author.
Ethics Statement
The animal study was reviewed and approved by the Animal Research Ethics Board of the University of Saskatchewan.
Author Contributions
MH, KA, and BD performed the experiment. ED and ES came up with the concept of the study. JN participated in the discussion of the concept and results. MH and ED wrote the manuscript. All authors edited the manuscript.
Funding
This research was funded by the Saskatchewan Health Research Foundation and partially supported by the Sylvia Fedoruk Canadian Centre for Nuclear Innovation.
Conflict of Interest
The authors declare that the research was conducted in the absence of any commercial or financial relationships that could be construed as a potential conflict of interest.
Acknowledgments
The authors would like to thank Dr. Marcel Wüthrich from the Department of Pediatrics, University of Wisconsin School of Medicine and Public Health, for the encouraging discussions at the beginning of the project.
References
Allen, K. J. H., Jiao, R., Malo, M. E., Frank, C., and Dadachova, E. (2018). Biodistribution of a radiolabeled antibody in mice as an approach to evaluating antibody pharmacokinetics. Pharmaceutics 10:E262. doi: 10.3390/pharmaceutics10040262
Allen, K. J. H., Jiao, R., Malo, M. E., Frank, C., Fisher, D. R., Rickles, D., et al. (2019). Comparative radioimmunotherapy of experimental melanoma with novel humanized antibody to melanin labeled with 213Bismuth and 177Lutetium. Pharmaceutics 11, 348–360.
Bryan, R. A., Guimaraes, A. J., Hopcraft, S., Jiang, Z., Bonilla, K., Morgenstern, A., et al. (2012). Toward developing a universal treatment for fungal disease using radioimmunotherapy targeting common fungal antigens. Mycopathologia 173, 463–471. doi: 10.1007/s11046-011-9476-9
Bryan, R. A., Huang, X., Morgenstern, A., Bruchertseifer, F., Casadevall, A., and Dadachova, E. (2008). Radiofungicidal effects of external gamma radiation and antibody-targeted beta and alpha radiation on Cryptococcus neoformans. Antimicrob. Agents Chemother. 52, 2232–2235. doi: 10.1128/AAC.01245-07
Bryan, R. A., Jiang, Z., Howell, R. C., Morgenstern, A., Bruchertseifer, F., Casadevall, A., et al. (2010). Radioimmunotherapy is more effective than antifungal treatment in experimental cryptococcal infection. J. Infect. Dis. 202, 633–637. doi: 10.1086/654813
Bryan, R. A., Jiang, Z., Huang, X., Morgenstern, A., Bruchertseifer, F., Sellers, R., et al. (2009). Radioimmunotherapy is effective against a high infection burden of Cryptococcus neoformans in mice and does not select for radiation-resistant phenotypes in cryptococcal cells. Antimicrob. Agents Chemother. 53, 1679–1682. doi: 10.1128/AAC.01334-08
Bryan, R. A., Jiang, Z., Morgenstern, A., Bruchertseifer, F., Casadevall, A., and Dadachova, E. (2013). Radioimmunotherapy of Cryptococcus neoformans spares bystander mammalian cells. Future Microbiol. 8, 1–9. doi: 10.2217/fmb.13.79
Dadachova, E., Bryan, R. A., Apostolidis, C., Morgenstern, A., Zhang, T., Moadel, T., et al. (2006a). Interaction of radiolabeled antibodies with fungal cells and components of immune system in vitro and during radioimmunotherapy of experimental fungal infection. J. Infect. Dis. 193, 1427–1436. doi: 10.1086/503369
Dadachova, E., Bryan, R. A., Frenkel, A., Zhang, T., Apostolidis, C., Nosanchuk, J. S., et al. (2004a). Evaluation of acute hematological and long-term pulmonary toxicity of radioimmunotherapy of Cryptococcus neoformans infection in murine models. Antimicrob. Agents Chemother. 48, 1004–1006. doi: 10.1128/aac.48.3.1004-1006.2004
Dadachova, E., Burns, T., Bryan, R. A., Apostolidis, C., Brechbiel, M. W., Nosanchuk, J. D., et al. (2004b). Feasibility of radioimmunotherapy of experimental pneumococcal infection. Antimicrob. Agents Chemother. 48, 1624–1629. doi: 10.1128/aac.48.5.1624-1629.2004
Dadachova, E., Howell, R. W., Bryan, R. A., Frenkel, A., Nosanchuk, J. D., and Casadevall, A. (2004c). Susceptibility of the human pathogenic fungi Cryptococcus neoformans and Histoplasma capsulatum to γ-radiation versus radioimmunotherapy with α- and β-emitting radioisotopes. J. Nucl. Med. 45, 313–320.
Dadachova, E., Nakouzi, A., Bryan, R. A., and Casadevall, A. (2003). Ionizing radiation delivered by specific antibody is therapeutic against a fungal infection. Proc. Natl. Acad. Sci. U.S.A. 100, 10942–109427.
Dadachova, E., Patel, M. C., Toussi, S., Apostolidis, C., Morgenstern, A., Brechbiel, M. W., et al. (2006b). Targeted killing of virally infected cells by radiolabeled antibodies to viral proteins. PLoS Med. 3:e427. doi: 10.1371/journal.pmed.0030427
Davies, J. L., Epp, T., and Burgess, H. J. (2013). Prevalence and geographic distribution of canine and feline blastomycosis in the Canadian prairies. Can. Vet. J. 54, 753–760.
Enoch, D. A., Yang, H., Aliyu, S. H., and Micallef, C. (2017). The changing epidemiology of invasive fungal infections. Methods Mol. Biol. 1508, 17–65. doi: 10.1007/978-1-4939-6515-1_2
Girouard, G., Lachance, C., and Pelletier, R. (2007). Observations on (1-3)-beta-D-glucan detection as a diagnostic tool in endemic mycosis caused by Histoplasma or Blastomyces. J. Med. Microbiol. 56, 1001–1002. doi: 10.1099/jmm.0.47162-0
Gray, N. A., and Baddour, L. M. (2002). Cutaneous inoculation blastomycosis. Clin. Infect. Dis. 34, E44–E49.
Guimarães, A. J., Frases, S., Gomez, F. J., Zancopé-Oliveira, R. M., and Nosanchuk, J. D. (2009). Monoclonal antibodies to heat shock protein 60 alter the pathogenesis of Histoplasma capsulatum. Infect. Immun. 77, 1357–1367. doi: 10.1128/IAI.01443-08
Helal, M., and Dadachova, E. (2018). Radioimmunotherapy as a novel approach in HIV, bacterial, and fungal infectious diseases. Cancer Biother. Radiopharm. 33, 330–335. doi: 10.1089/cbr.2018.2481
Jiang, Z., Bryan, R. A., Morgenstern, A., Bruchertseifer, F., Casadevall, A., and Dadachova, E. (2012). Treatment of early and established Cryptococcus neoformans infection with radiolabeled antibodies in immunocompetent mice. Antimicrob. Agents Chemother. 56, 552–554. doi: 10.1128/AAC.00473-11
Jiao, R., Allen, K. J. H., Malo, M. E., Helal, M., Jiang, Z., Smart, K., et al. (2019). Evaluation of novel highly specific antibodies to cancer testis antigen Centrin-1 for radioimmunoimaging and radioimmunotherapy of pancreatic cancer. Cancer Med. 8, 5289–5300. doi: 10.1002/cam4.2379
Larson, S. M., Carrasquillo, J. A., Cheung, N. K., and Press, O. W. (2015). Radioimmunotherapy of human tumours. Nat. Rev. Cancer 15, 347–360. doi: 10.1038/nrc3925
McDonald, R., Dufort, E., Jackson, B. R., Tobin, E. H., Newman, A., Benedict, K., et al. (2018). Notes from the field: blastomycosis cases occurring outside of regions with known endemicity - New York, 2007-2017. MMWR Morb. Mortal Wkly. Rep. 67, 1077–1078. doi: 10.15585/mmwr.mm6738a8
Nosanchuk, J. D., and Dadachova, E. (2012). Radioimmunotherapy of fungal diseases: the therapeutic potential of cytocidal radiation delivered by antibody targeting fungal cell surface antigens. Front. Microbiol. 12:283. doi: 10.3389/fmicb.2011.00283
Odabasi, Z., Paetznick, V. L., Rodriguez, J. R., Chen, E., McGinnis, M. R., and Ostrosky-Zeichner, L. (2006). Differences in beta-glucan levels in culture supernatants of a variety of fungi. Med. Mycol. 44, 267–272. doi: 10.1080/13693780500474327
Pappas, P. G., Threlkeld, M. G., Bedsole, G. D., Cleveland, K. O., Gelfand, M. S., and Dismukes, W. E. (1993). Blastomycosis in immunocompromised patients. Medicine 72, 311–325. doi: 10.1097/00005792-199309000-00003
Phaeton, R., Jiang, Z., Revskaya, E., Fisher, D. R., Goldberg, G. L., and Dadachova, E. (2016). Beta emitters Rhenium-188 and Lutetium-177 are equally effective in radioimmunotherapy of HPV-positive experimental cervical cancer. Cancer Med. 5, 9–16. doi: 10.1002/cam4.562
Quelven, I., Monteil, J., Sage, M., Saidi, A., Mounier, J., Bayout, A., et al. (2019). 212Pb Alpha-Radioimmunotherapy targeting CD38 in multiple Myeloma: a preclinical study. J. Nucl. Med. doi: 10.2967/jnumed.119.239491 [Epub ahead of print],
Sidamonidze, K., Peck, M. K., Perez, M., Baumgardner, D., Smith, G., Chaturvedi, V., et al. (2012). Real-time PCR assay for identification of Blastomyces dermatitidis in culture and in tissue. J. Clin. Microbiol. 50, 1783–1786. doi: 10.1128/JCM.00310-12
Tomblyn, M. (2012). Radioimmunotherapy for B-cell non-hodgkin lymphomas. Cancer Control 19, 196–203. doi: 10.1177/107327481201900304
Torosantucci, A., Bromuro, C., Chiani, P., Bernardis, F. D., Berti, F., Galli, C., et al. (2005). A novel glyco-conjugate vaccine against fungal pathogens. J. Exp. Med. 202, 597–606. doi: 10.1084/jem.20050749
Wang, X. G., Revskaya, E., Bryan, R. A., Strickler, H. D., Burk, R. D., Casadevall, A., et al. (2007). Treating cancer as an infectious disease–viral antigens as novel targets for treatment and potential prevention of tumors of viral etiology. PLoS One 2:e1114. doi: 10.1371/journal.pone.0001114
Keywords: radioimmunotherapy, Blastomyces dermatitidis, (1→3)-β-glucan, mouse model, 213Bismuth
Citation: Helal M, Allen KJH, van Dijk B, Nosanchuk JD, Snead E and Dadachova E (2020) Radioimmunotherapy of Blastomycosis in a Mouse Model With a (1→3)-β-Glucans Targeting Antibody. Front. Microbiol. 11:147. doi: 10.3389/fmicb.2020.00147
Received: 30 July 2019; Accepted: 21 January 2020;
Published: 07 February 2020.
Edited by:
Carlos Pelleschi Taborda, University of São Paulo, BrazilReviewed by:
Bruce Klein, University of Wisconsin-Madison, United StatesTodd B. Reynolds, The University of Tennessee, Knoxville, United States
Bettina Fries, Stony Brook Medicine, United States
Copyright © 2020 Helal, Allen, van Dijk, Nosanchuk, Snead and Dadachova. This is an open-access article distributed under the terms of the Creative Commons Attribution License (CC BY). The use, distribution or reproduction in other forums is permitted, provided the original author(s) and the copyright owner(s) are credited and that the original publication in this journal is cited, in accordance with accepted academic practice. No use, distribution or reproduction is permitted which does not comply with these terms.
*Correspondence: Ekaterina Dadachova, ekaterina.dadachova@usask.ca