- 1Department of Epidemiology, Human Genetics and Environmental Sciences, Center for Infectious Diseases, School of Public Health, University of Texas Health Science Center, Houston, TX, United States
- 2Division of Food Processing Science and Technology, U.S. Food and Drug Administration, Bedford Park, IL, United States
- 3Microbiology and Infectious Diseases Program, University of Texas MD Anderson Cancer Center UTHealth Graduate School of Biomedical Sciences, Houston, TX, United States
In the United States, the consumption of fresh fruits and vegetables has increased during recent years as consumers seek to make healthier lifestyle choices. However, the number of outbreaks associated with fresh produce that involve cases in more than one state (multistate) has increased concomitantly. As the distance along the farm-to-fork continuum has lengthened over time, there are also more opportunities for fresh produce contamination with bacterial pathogens before it reaches the consumer. This review provides an overview of the three bacterial pathogens (i.e., pathogenic Escherichia coli, Listeria monocytogenes, and Salmonella enterica) associated with multistate fresh produce outbreaks that occurred between 2010 and 2017 in the U.S. Possible routes of fresh produce contamination, including pre- and post-harvest, are summarized and outcomes of selected outbreaks within this timeframe are highlighted. Eighty-five multistate outbreaks linked to fresh produce with a confirmed etiology occurred from 2010 to 2017. Cross-contamination within the distribution chain and poor agricultural practices, along with the production of sprouts and importation of fresh produce were frequently implicated contributors to these events. The evolution of the food supply chain in the U.S. necessitates an examination of multistate outbreaks to shed light on factors that increase the scale of these events.
Introduction
The consumption of fresh fruits and vegetables (“fresh produce”) has increased substantially since the 1980s due to consumer demand for a healthier lifestyle and more nutritious foods, especially in high-income countries. The World Health Organization recommends a daily intake of 400 grams of fresh produce (WHO, 2003). A diet rich in fresh produce has been shown to prevent certain chronic diseases such as diabetes, cancer, cardiovascular disease, hypertension, and obesity (WHO, 2003). Due to demand, globalization of trade has aided the distribution of fresh produce worldwide, surmounting the seasonality and location of certain commodities. In 2001, fresh produce encompassed 16.9% ($70 billion) of world agricultural trade, up from 10.6% ($3.4 billion) in 1961 (Huang, 2004). In the U.S., the per capita consumption of fresh produce in 2010 and 2016 was 67.2 and 68.7 kg, respectively (USDA, 2018). Although consumption of fresh produce in the U.S. is steadily increasing, only approximately 6–8% of people achieve the 400 gram daily target (Rekhy and McConchie, 2014); therefore, government and non-government agencies continuously promote nutrition education and encourage consumption of fresh produce to promote public health.
As consumption of fresh produce increases, the likelihood of associated illnesses and outbreaks caused by microbial pathogens is also expected to increase. Since fresh produce is often consumed in its raw state with no processing step to eliminate harmful organisms, there is the potential for contamination with foodborne pathogens and thus, illness upon consumption. For instance, 1779 foodborne outbreaks with a confirmed food vehicle and a confirmed etiology occurred in the U.S. from 2004 to 2010, of which 9.2% (163) were attributed to fresh produce (CDC, 2017d). Out of the 163 produce-associated outbreaks, 27.6% (45) occurred in multiple states of the U.S. A multistate outbreak is defined as an outbreak caused by the same contaminated food item that has been distributed to multiple states. Multistate produce-associated outbreaks from 2004 to 2010 caused a total of 4949 illnesses, 895 hospitalizations, and nine deaths. From 2010 to 2017, 1797 foodborne outbreaks with a confirmed food vehicle and a confirmed etiology occurred in the U.S., of which 12.7% (228) were attributed to fresh produce (CDC, 2017d). Out of these 228 produce-associated outbreaks, a total of 37.3% (85) were multistate. These multistate produce-associated outbreaks resulted in 4748 illnesses, 1190 hospitalizations, and 55 deaths. Overall, the number of produce-associated foodborne outbreaks increased in the U.S. from 2010 to 2017 as compared to 2004 to 2010. The number of produce-associated multistate outbreaks also increased, as did the number of deaths due to these outbreaks. These data suggest that multistate produce-associated outbreaks in the U.S. are on the rise, which is of critical public health concern.
The U.S. Food and Drug Administration (FDA) Food Safety Modernization Act (FSMA) was signed into law in 2011 and allows the FDA to not only respond to food contamination events and foodborne outbreaks but also to focus on prevention-based strategies (FDA, 2019a). The Produce Safety Rule, part of FSMA, provides science-based guidance for safe production, harvest, and handling of fruits and vegetables (FDA, 2019b). The final rule went into effect in January 2016 and indicates that all fresh produce facilities implement mandatory preventive controls and produce safety standards. The key requirements of the rule include a minimum quality for agricultural water, microbial standards for biological soil amendments, specifications for the use of domesticated animals for grazing or working, worker training and hygiene, and standards for tools, equipment, and buildings. The rule also provides draft guidance for sprout growers. In 2017, a total of 13 multistate produce-associated outbreaks occurred in the U.S., whereas the five preceding years (2010–2016) averaged 14 outbreaks per year. Although the number of average outbreaks has not changed largely since the enactment of the final rule, it may be too soon to detect the full effect of the new regulation.
This review will highlight the multistate produce-associated outbreaks in the U.S. from 2010–2017 and the associated bacterial pathogens: Listeria monocytogenes, Escherichia coli, and Salmonella enterica. Further discussion will involve various routes of fresh produce contamination, both pre- and post-harvest, and the risks associated with individual categories of fresh produce including fruits, leafy greens, and vegetable row crops. Finally, this review also explores the incidence and contributing factors of multistate foodborne outbreaks associated with fresh produce consumption. A comprehensive overview of the principal causes and outcomes of multistate outbreaks may establish patterns that can aid in the reduction and possible prevention of these events.
Pathogens Associated With Fresh Produce
Human pathogens generally associated with fresh produce include Hepatitis A, Norovirus, Cyclospora, Aeromonas spp., Bacillus cereus, Campylobacter spp., Clostridium botulinum, E. coli, L. monocytogenes, S. enterica, Shigella spp., Staphylococcus spp., and Yersinia enterocolitica. As there were only three bacterial pathogens involved in multistate fresh produce outbreaks from 2010 to 2017 (see section Multistate Outbreaks of Foodborne Illness Associated With Fresh Produce), this section focuses on E. coli, L. monocytogenes, and S. enterica.
Pathogenic E. coli
E. coli is a Gram-negative, rod-shaped, facultative anaerobe that is associated with the gut microbiota of humans and animals (Croxen et al., 2013). E. coli are coliforms and common fecal indicators as they are associated with fecal matter and are found in proximity to water, soil, and animals (van Elsas et al., 2011). Some strains of E. coli can proliferate at temperatures as low as 7°C and as high as 46°C; optimum temperature for this organism occurs at 37°C (ICMSF, 1996). It has been determined that E. coli can survive in environments containing 6% sodium chloride (NaCl) and pH values > 5.4. All E. coli serotypes are characterized by combinations of somatic (O), flagellar (H), and capsular (K) surface antigens, which can be detected through various molecular techniques including serology (ICMSF, 1996; Nataro and Kaper, 1998).
E. coli can be transmitted via a foodborne route, and there are six major E. coli pathotypes that are broadly distinguished by virulence factors (Croxen et al., 2013; Yang et al., 2017). Enteropathogenic E. coli (EPEC) commonly infects children, yet can also infect adults at infectious doses of 108–1010 CFU. Shiga-toxin producing E. coli/enterohemorrhagic E. coli (STEC/EHEC), also referred to as Verocytotoxin-producing E. coli (VTEC), can cause human illness with an extremely low infectious dose (<1000 CFU). The most common serotype of STEC implicated in outbreaks of foodborne disease is E. coli O157:H7, which is covered in the following section (see section E. coli O157:H7). Infection with enteroinvasive E. coli (EIEC) can resemble Shigella infection, although a greater infectious dose is required for human illness (106–108 CFU vs. 10–100). Both Enteroaggregative E. coli (EAEC) and Enterotoxigenic E. coli (ETEC) are frequent causes of traveler’s diarrhea and are characterized by infectious doses of 1010 and 108 CFU, respectively. Diffusely invasive E. coli (DAEC) is an emerging pathotype of E. coli that causes persistent diarrhea in children and is suspected to contribute to Crohn’s disease in adults (Le Bouguenec and Servin, 2006). Infection with any of these six pathotypes of E. coli can lead to diarrheal disease. The natural history of disease does vary, however, and is dependent on the contributing E. coli pathotype.
More than approximately 112,000 cases of foodborne non-O157 STEC occur each year in the U.S. with a hospitalization rate of 12.8% (Scallan et al., 2011). Between 2009 and 2015, STEC was responsible for 191 (6%) of all reported outbreaks, which remained consistent from outbreaks that occurred from 1998 to 2008 (Dewey-Mattia et al., 2018). The mean total cost of non-O157 E. coli infections in the U.S. is 23.9 million dollars annually (Hoffmann et al., 2012). Approximately 18,000 cases of foodborne ETEC E. coli occur in the U.S. per year, with an additional 12,000 cases that occur due to diarrheagenic E. coli, not including STEC or ETEC pathotypes (Scallan et al., 2011).
E. coli O157:H7
Enterohemorrhagic E. coli O157:H7 is of particular public health concern as it is the primary cause of hemolytic uremic syndrome (HUS) in the U.S. (Banatvala et al., 2001). Transmission generally occurs via a foodborne route, from person-to-person, the consumption of contaminated water, or animal contact (Rangel et al., 1982). Symptoms vary and can include mild gastroenteritis, bloody diarrhea, hemorrhagic colitis, HUS, and even death. An estimated 63,153 cases of foodborne Shiga-toxin producing E. coli O157-related cases occur annually in the U.S with a hospitalization rate of 46.2%, which is a rate similar to that of Vibrio cholerae (43.1%; Scallan et al., 2011). The mean total cost for E. coli O157:H7-related illness in the U.S. is 254.8 million dollars annually (Hoffmann et al., 2012). E. coli O157:H7 shed in bovine and other animal feces likely causes the contamination of fresh produce and the low infectious dose required for illness increases concern (Lim et al., 2010).
L. monocytogenes
The pathogenic bacterium L. monocytogenes is a Gram-positive, rod-shaped, non-spore-forming facultative anaerobe that is ubiquitous in the environment (Farber and Peterkin, 1991). Optimum growth of L. monocytogenes occurs between 30 and 37°C; however, the organism is highly resilient and has been observed to proliferate between temperatures of −2 to 45°C, pH values of 4.0–9.5, and NaCl concentrations as high as 10% (Liu et al., 2005). Of notable concern is the capability of L. monocytogenes to survive and grow at and below the U.S. FDA recommended refrigeration temperatures of ≤4°C (FDA, 2017). L. monocytogenes is also able to form biofilms on various surfaces, which allows for further resistance to environmental stress, and leads to difficulty with surface disinfection and sanitation (da Silva and De Martinis, 2013).
At least 12 serotypes of L. monocytogenes exist and are distributed into genetic categories known as lineages. Lineage I mainly comprises serotypes 1/2b, 3b, 4b, 4d, and 4e and are more often implicated in outbreaks of listeriosis (Ragon et al., 2008; Lomonaco et al., 2015). Lineage II contains serotypes 4a, 1/2c, 3a, and 3c and mainly cause sporadic cases of listeriosis. Lineages III and IV are rare in nature, with only 3% of L. monocytogenes belonging to this category.
The primary route of L. monocytogenes infection is via the consumption of contaminated food. Listeriosis can be asymptomatic or cause febrile gastroenteritis in healthy individuals, however, cases of invasive infection can result in conditions such as septicemia, meningoencephalitis, and fetal loss. Invasive listeriosis has a mortality rate of 20–30% (Swaminathan and Gerner-Smidt, 2007). Populations susceptible to listeriosis include those that are immunosuppressed, elderly, and pregnant, along with neonates. L. monocytogenes has an incubation period that ranges from one to 70 days based on the clinical manifestation of the infection (Hernandez-Milian and Payeras-Cifre, 2014), and an infectious dose of approximately 103 cells for an immunocompromised individual (Pouillot et al., 2015).
Approximately 1600 cases of listeriosis occur in the U.S. annually (Scallan et al., 2011). Although listeriosis has a low incidence, it has the highest estimated hospitalization rate (94%) out of 31 major foodborne pathogens, including Vibrio vulnificus (91.3%) and Clostridium botulinum (82.6%). Monetary loss due to the cost of illness associated with listeriosis in the U.S. is estimated to be 2.6 billion U.S. dollars per year (Hoffmann et al., 2012).
S. enterica
S. enterica is a Gram-negative, rod-shaped, non-sporulating facultatively anaerobic organism (Fabrega and Vila, 2013). Pathogenic species of Salmonella are of concern to the food industry as they have been documented to grow at temperatures between 8 and 45°C, pH values from 4.0 to 9.5, and water activity values as low as 0.94 (Chlebicz and Slizewska, 2018). Animal fecal materials are known to harbor S. enterica, which leads to the contamination of water and crops (Wiedemann et al., 2014).
Antigenic formulas are used to classify S. enterica, however, strains are frequently referred to by serotype name. There are six subspecies of S. enterica designated enterica (I), salamae (II), arizonae (IIIa), diarizonae IIIb, houtenae (IV), and indica (IV). S. bongori was formerly referred to as subspecies V, but is now considered a species (Crum-Cianflone, 2008). S. enterica subspecies enterica (I) contains the majority of serotypes that are human pathogens (Fierer and Guiney, 2001).
Salmonella spp. infect humans via various modes, such as contact with an animal carrier and the consumption of contaminated food or water. An estimated 94% of non-typhoidal S. enterica infections in the U.S. are foodborne (Hoffmann et al., 2012). Salmonellosis presents typical gastroenteritis symptoms, and incubation times can range between six and 48 h (Crum-Cianflone, 2008). The infectious dose has not been established, but the disease is generally self-limiting. Invasive salmonellosis can occur, especially in at-risk individuals such as children, the immunocompromised, and the elderly. Treatment with antibiotics for salmonellosis is reserved for severe cases or cases susceptible to complications (Hohmann, 2001).
Over 1 million cases of non-typhoidal S. enterica occur each year in the U.S., of which an estimated 27.2% result in hospitalizations (Hoffmann et al., 2012). Between 1998 and 2008, Salmonella spp. caused 18% of single etiology outbreaks, along with the majority of all hospitalizations (44%) and deaths (30%) recorded. Salmonella spp. also caused 53% of all reported multistate outbreaks in the same time period (Gould et al., 2013). Between 2009 to 2015, S. enterica Enteritidis caused the largest of 177 multistate outbreaks by case-count (1,939). Also, Salmonella spp. caused almost double the number of single etiology outbreaks as recorded in the previous 10 years (30%) (Dewey-Mattia et al., 2018). Annually, non-typhoidal S. enterica illnesses are estimated to cost approximately 3.3 billion U.S. dollars, more than any other of 14 major foodborne pathogens (Hoffmann et al., 2012).
Routes of Fresh Produce Contamination
Fresh produce is generally minimally processed (e.g., washed, sanitized, packaged) and therefore does not go through additional processing steps that would further reduce the microbiological burden and eliminate harmful pathogens (e.g., heating). Since fresh produce is often grown underground or near the ground, it is expected that the saprophytic environmental microorganisms that inhabit soil, water, and vegetation would also be present on these food items. Previous mesophilic evaluation of bacterial levels on fresh produce showed 103–109 CFU/g before and 103–106 CFU/g after minimal processing (Nguyen-the and Carlin, 1994). It is estimated that 80–90% of the mesophilic bacteria are Gram-negative rods including Enterobacter spp., Erwinia spp., and Pseudomonas spp. (Magnuson et al., 1990; Marchetti et al., 1992; Nguyen-the and Carlin, 1994). Yeast and mold levels on fresh produce range from 102 to 106 (Garg et al., 1990; King et al., 1991; Nguyen-the and Carlin, 1994). Even with the high population levels of the native microflora, the quality of fresh produce is often still acceptable.
Due to minimal processing, the concern associated with fresh produce consumption is the possibility for contamination with foodborne pathogens. For example, cantaloupe is grown close to the ground, and the melons can come into contact with soil, other vegetation, irrigation water, and fertilizer. Studies assessing the presence of S. enterica on cantaloupes have observed the prevalence of the pathogen in samples as low as 0.76% (11/1440) (Madden, 1992) and as high as 5.3% (8/151) (FDA, 1999). In addition to pre-processing contamination, fresh produce can also become contaminated with foodborne pathogens during harvesting and various post-harvest stages including processing, transport, and storage.
Pre-harvest Contamination
Prior to harvest, fresh produce can become contaminated with foodborne pathogens from the environment. Pathogens can be introduced into the environment via irrigation water, soil, fertilizers, manure, insects, and dust. Irrigation water is a known vector for foodborne pathogen contamination, and its quality is an indicator of produce safety. Natural sources of irrigation water include rivers, collected rainwater, aquifers, and groundwater (Uyttendaele et al., 2015). Irrigation water can also come from the reuse of wastewater, which could potentially be contaminated with human pathogens depending on how the water was treated. One study found fecal coliform counts as high as 4 log CFU/ml in irrigation water (Materon et al., 2007). Irrigation water can effectively transmit E. coli O157:H7 to row crops such as lettuce (Solomon et al., 2002; Wachtel et al., 2002a); and epidemiological investigations have traced foodborne pathogen contamination of fresh produce to irrigation water (Ackers et al., 1998; Herwaldt, 2000; Wachtel et al., 2002b). For example, irrigation water sourced from a pond was responsible for contaminating tomatoes that led to a multistate S. enterica outbreak in 2005 (Greene et al., 2005).
Soil, fertilizers, and animal manure can also be potential sources for fresh produce contamination. Saprophytic bacteria, such as L. monocytogenes, are ubiquitous in soil and can readily contaminate produce grown underground or low to the ground. Soil amendments, such as fertilizer, compost, and manure can introduce or spread foodborne pathogens to crops. Farm animals such as cows, sheep, pigs, chickens, and deer shed pathogenic E. coli and S. enterica species in their feces, with prevalence in fecal samples as high as 70% (Bailey et al., 2001; Dargatz et al., 2003; Hussein and Bollinger, 2005; Keen et al., 2006; Doane et al., 2007). Fresh produce can be exposed to these pathogens and other enteric pathogens through nearby farm or wildlife animals, along with water runoff containing animal feces. In 2006, feral swine feces was thought to be responsible for the contamination of agricultural and surface water at a ranch which led to an outbreak of E. coli O157:H7 associated with bagged spinach in the U.S. (26 states involved) and Canada; 205 illnesses and three deaths were reported (Jay et al., 2007).
Air, dust, insects, and invertebrates are also vectors for the transfer of foodborne pathogens to fresh produce. Airborne transmission of E. coli O157:H7 from a cattle feedlot to leafy greens fields 60–180 m away has been demonstrated experimentally (Berry et al., 2015). Wildlife that roams and forages in fields may shed foodborne pathogens in fecal matter. Local and migratory birds may also serve as vectors for foodborne pathogens as they frequent fields to obtain food and shelter (Cernicchiaro et al., 2012). In addition, nematodes, slugs, and insects have been shown to harbor bacterial pathogens such as E. coli O157:H7, Campylobacter, Shigella, S. enterica, and L. monocytogenes (Kenney et al., 2006; Sproston et al., 2006; Khamesipour et al., 2018). Insects can facilitate the contamination of fresh produce, for example, various species of flies are capable of transferring E. coli O157:H7 to spinach leaves (Talley et al., 2009; Wasala et al., 2013) and apples (Janisiewicz et al., 1999) through their excreta or regurgitation.
Contamination During Harvesting
Contamination of fresh produce with foodborne pathogens can occur during harvesting through contact with contaminated equipment, transport containers, knives and tools, and human hands and gloves. Mechanical harvesting equipment, including vegetable cutters and corers, can harbor pathogens and disseminate these organisms to fresh produce during harvesting (McEvoy et al., 2009; Taormina et al., 2009; Yang et al., 2012). Leafy greens are often cut and cored in the field by a field-core harvesting technique. During simulated field harvesting, iceberg lettuce was cross-contaminated with E. coli O157:H7 when the pathogen was on the blade of the field coring device (Taormina et al., 2009). A contaminated field coring knife is capable of transferring E. coli O157:H7 to as many as 19 heads of lettuce (McEvoy et al., 2009).
It is estimated that 20% of all foodborne bacterial illnesses are due to food worker transmissions (Greig et al., 2007). In the U.S. during the 2000s, a total of 647 foodborne outbreaks, causing 54,888 illnesses, were attributed to food worker transmissions (Greig et al., 2007). Out of these outbreaks, 23% (151) were due to the consumption of food contaminated with S. enterica; of which 5% (7) were associated with produce and lead to a total of 1263 illnesses. Food worker pathogen transmission to food products may be due to poor hygienic conditions (personnel or environmental), working while sick, or due to improper food safety training and good agricultural practices. Food workers may also be asymptomatic carriers of foodborne pathogens, such as S. enterica Typhi. Various studies have determined that pathogens are transmissible from humans to food surfaces (Jimenez et al., 2007; Sharps et al., 2012; Brar and Danyluk, 2013). For example, S. enterica has been documented to be transmitted from gloves to tomatoes during harvesting in a laboratory setting (Brar and Danyluk, 2013). Proper training for food handlers, including frequent glove changes and handwashing, is essential to prevent the spread of foodborne pathogens.
Post-harvest and Processing Contamination
Post-harvest contamination of fresh produce can occur during transport, storage, and processing. Once harvested from the field, produce is chilled and transported while refrigerated to its next destination. Since rapid cooling of produce is essential to minimize product damage and increase shelf life, coolers, ice or water cooling, and transport vehicles can be possible sources of pathogen contamination. Fresh produce is often transported in a highway trailer either on ice (as is used with broccoli, green onions, and sweet corn) or with a mechanical cooling system (used with lettuce and other leafy greens) (Suslow et al., 2003). In addition to maintaining optimal quality and respiration, strict temperature control of fresh produce is needed to reduce the risk associated with illness due to foodborne pathogens. After transport and once at a processing facility, fresh produce can be stored in a packing house for as little as a few hours or up to several days.
Fresh produce is generally minimally processed with stages that include washing/fluming, shredding and cutting, drying, and packaging. Produce contamination can occur due to contaminated equipment, or due to cross-contamination with other produce. Many studies have assessed the contamination or cross-contamination of produce items during processing (Buchholz et al., 2012a, b, 2014; Davidson et al., 2017; Smolinski et al., 2018). For example, both S. enterica and E. coli O157:H7 have been shown to cross-contaminate spinach, cilantro, and Romaine lettuce during pilot plant-scale processing including washing/fluming (Smolinski et al., 2018). Cross-contamination of pathogens can also occur from processing equipment to food products. A 33.3% (30) frequency of E. coli was observed from environmental samples of a conveyor system at a melon farm in Texas (Castillo et al., 2004). Once washed and packaged, produce can become contaminated during storage if the food is stored in a contaminated location, contaminated box, or enclosure. A 0.8 and a 1.6% (250) frequency of isolation of S. enterica and E. coli, respectively, on washed cantaloupe melons stored in a cooler within a cardboard box at the same melon farm has also been observed (Castillo et al., 2004). The melons could have become contaminated from the field, during harvest or processing, or during storage in the cardboard box.
Once minimally processed, fresh produce is distributed to retail food establishments, food service facilities, and restaurants. Handling of fresh produce by food service personnel, along with the cutting and chopping of fresh produce are all scenarios where these foods can become contaminated. In addition, display temperatures above refrigeration at retail can increase the risk of illness due to possible pathogen proliferation. Before use for cutting or chopping of fresh produce items, equipment including knives and cutting boards should be sanitized. Fresh produce should also be segregated from raw meat and poultry. A knife can cross-contaminate lettuce with S. enterica if it is first used to cut raw chicken (Ravishankar et al., 2010). Once cut, fresh produce is often packaged in deli containers or plastic wrap and is stored in retail display cabinets. Proper refrigeration temperature should be maintained to reduce the risk of bacterial pathogen proliferation. For example, the growth rates of L. monocytogenes were significantly higher on fresh-cut produce items stored in deli containers at 10°C when compared to 5°C (Salazar et al., 2017).
Multistate Outbreaks of Foodborne Illness Associated With Fresh Produce
Data were obtained from the National Outbreak Reporting System (NORS), which tracks foodborne disease outbreaks from public health departments in the U.S (CDC, 2017e). This database does not include outbreaks caused by an exposure event that occurred outside of the U.S. and is limited to outbreaks that are detected, investigated, and reported. Outbreaks considered for inclusion in this review must have resulted in foodborne enteric illness due to a confirmed pathogen in more than one state in the U.S between 2010 and 2017. The implicated food vehicle in each outbreak also must have been classified as produce based on the Food Safety Analytics Collaboration categories (IFSAC, Richardson et al., 2017). In this categorization method, one food category is assigned when the implicated food has a single ingredient and when each ingredient in the food belongs to the same food category. In this review, outbreaks were excluded if they were linked to a food that had multiple ingredients unless the ingredients were all classified within the same category. Outbreaks were also excluded if the implicated food vehicle was dried or frozen. A case study is presented in section A Case Study: Caramel Apples, which is not included in the outbreak tally of this review as it does not meet the inclusion criteria but is discussed as the unique characteristics of the outbreak highlight issues related to multi-component food products that contain fresh produce.
Overall, 85 multistate outbreaks associated with fresh produce with confirmed etiologies occurred in the U.S. from 2010 to 2017. A total of five pathogens were responsible for these outbreaks; 83 outbreaks were attributed to three bacterial pathogens, while the remaining two outbreaks were linked to Hepatitis A and Cyclospora cayetanensis. As bacterial pathogens were the principal cause of multistate outbreaks associated with fresh produce in the time period of interest, this review will focus on these organisms. S. enterica was linked to over half of the multistate outbreaks due to bacterial pathogens (67.5%) with 32 different confirmed serotypes. The most frequent single-etiology outbreaks of S. enterica infection were attributable to serotypes Newport (10), Enteritis (6), and Javiana (5). Pathogenic E. coli was responsible for 27.4% of the outbreaks, and L. monocytogenes was responsible for 4.8%. These outbreaks resulted in a known combined total of 4658 illnesses, 1187 hospitalizations, and 55 deaths. S. enterica was the cause of the majority of known illnesses (81.1%) and known hospitalizations (66.2%), however, L. monocytogenes was responsible for the majority of known deaths (67.3%), which were mostly from a single outbreak (33 known deaths; see section Cantaloupe). Vegetables were implicated in 69.9% of outbreaks, and the remainder were linked to fruits (Table 1); however, more deaths occurred due to outbreaks associated with fruits (76.4%) as compared to vegetables (24.6%). The most frequently identified food vehicles within the vegetable category were sprouts (27.6%) and lettuce (13.8%), followed by cucumbers, Romaine, and leafy greens (12.1% each), but outbreaks associated with cucumbers resulted in nearly half (42.8%) of all illnesses linked to vegetables. Melons were the dominant food vehicle for bacterial outbreaks in the fruit category (40.0%) regarding known illnesses (44.9%), hospitalizations (60.0%), and deaths (90.5%).
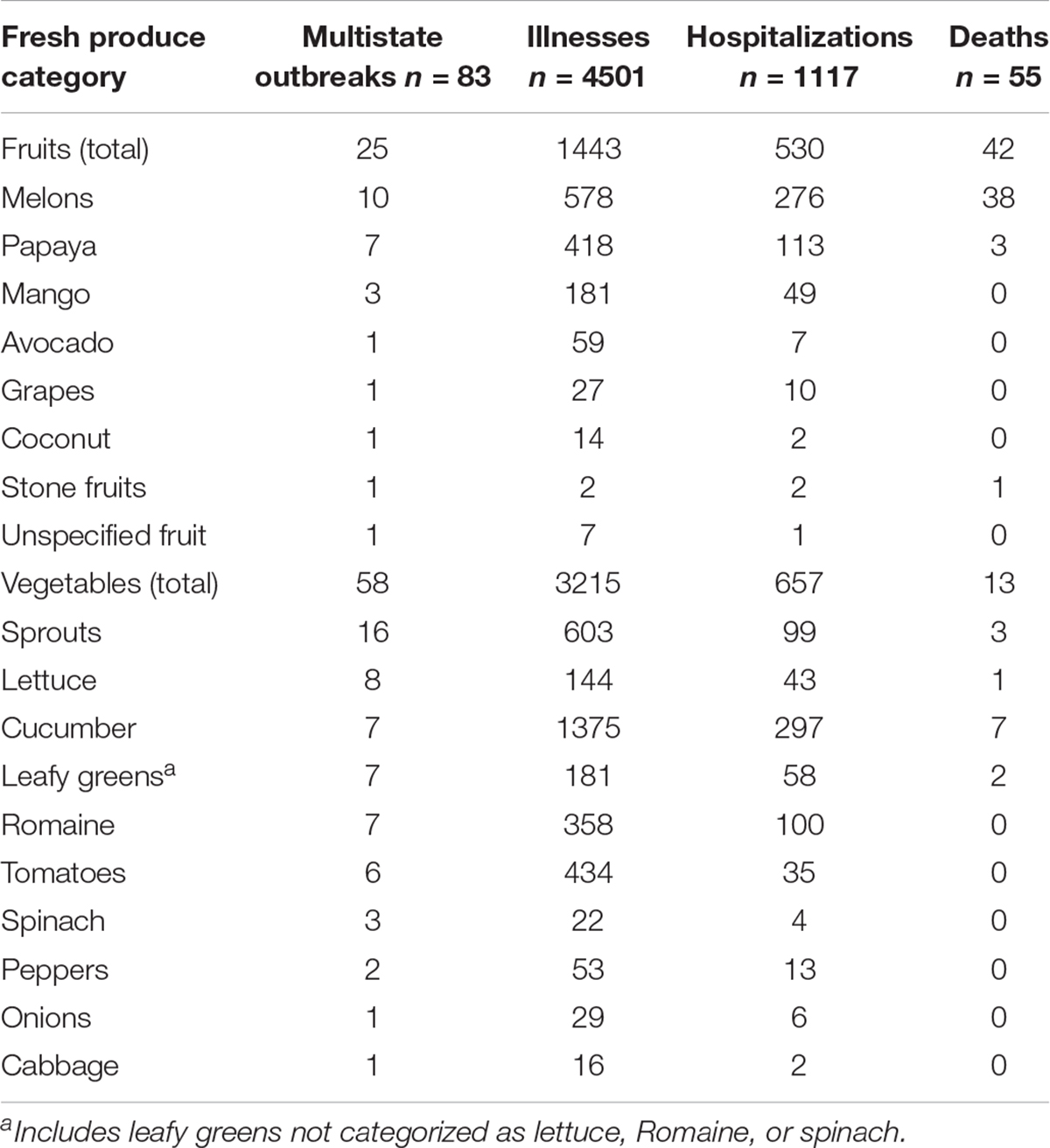
Table 1. Multistate foodborne outbreaks of bacterial infection in the U.S. from 2010 to 2017 associated with different fresh produce categories.
Pathogenic E. coli
A total of 23 multistate outbreaks were attributed to pathogenic E. coli and associated with fresh produce between 2010 and 2017 (Table 2). These outbreaks caused a combined total of 550 illnesses, and E. coli O157:H7 was responsible for over half of these cases (377). Vegetable row crops such as lettuce were identified as food vehicles in the majority of these outbreaks (87.0%), and the remainder were linked to sprouts. No outbreaks were linked to fruits. Six total E. coli serotypes were implicated in these outbreaks, and no single outbreak had more than one confirmed etiology. E. coli O157:H7 was the dominant serotype, responsible for 13 out of the 23 outbreaks (56.5%), of which 100% were associated with vegetable row crops.
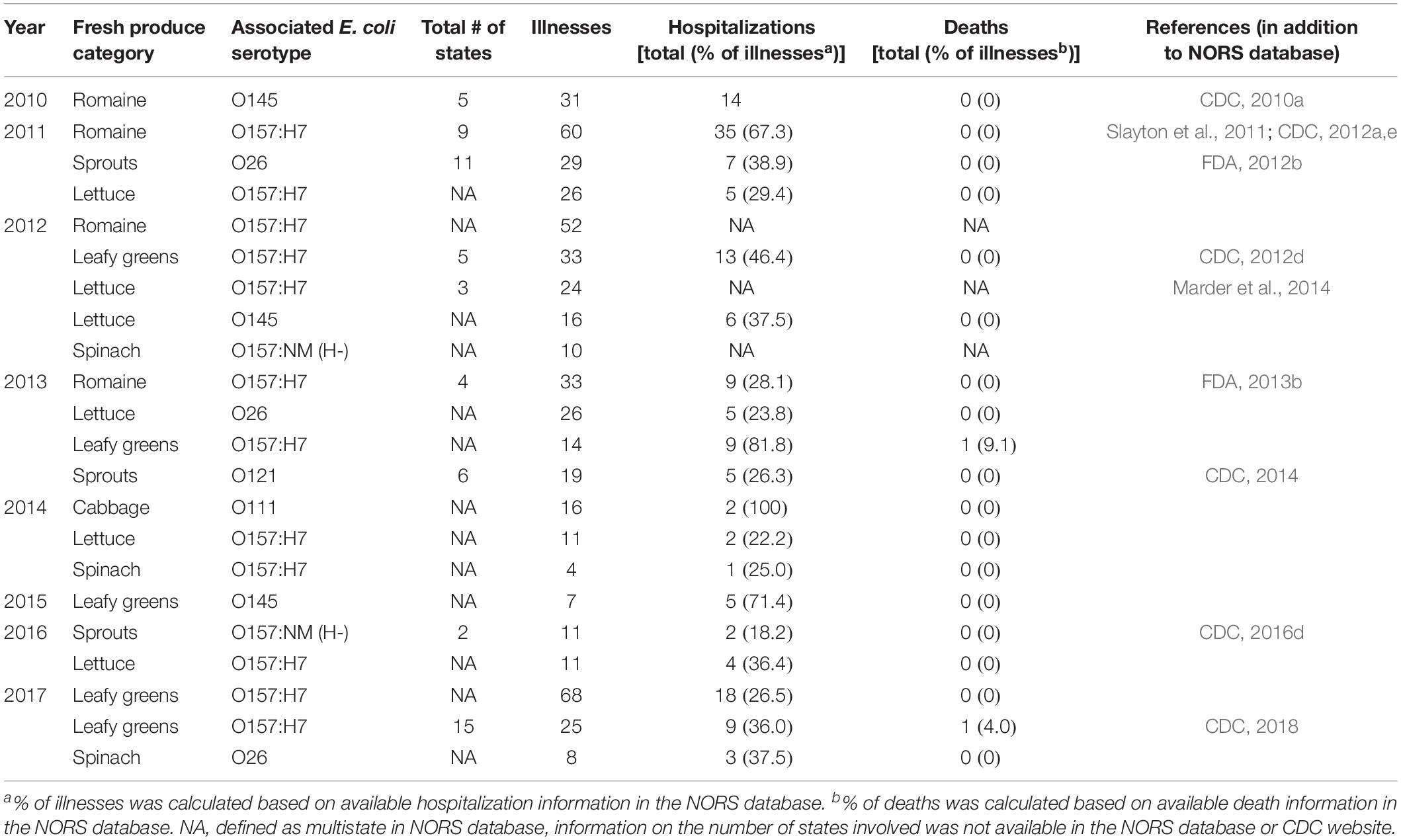
Table 2. Multistate foodborne outbreaks in the U.S. from 2010 to 2017 due to Escherichia coli associated with fresh produce.
Romaine Lettuce
Between 2010 and 2017, five outbreaks of E. coli infection occurred that were associated with Romaine lettuce. Four out of these five outbreaks were linked to E. coli O157:H7 and caused a total of 192 illnesses and no known deaths. An outbreak in 2011 affected the largest number of states (9) and resulted in the largest number of illnesses (58) (Slayton et al., 2011; CDC, 2012a) of all E. coli outbreaks due to contaminated Romaine lettuce in this timeframe. Illness onset occurred from October 9, 2011, to November 7, 2011, and 33 hospitalizations, three cases of HUS, and no deaths were reported (CDC, 2012a). The majority of cases occurred in Missouri (65.5%) and were female (59%). The median age of cases was 28 years, yet the age range was 1–94 years. Epidemiological data pointed to Romaine lettuce as the food vehicle in this outbreak, and one farm was identified as the likely source of the contaminated product, but confirmation via environmental testing was not obtained (Slayton et al., 2011).
Other Leafy Greens
A total of 15 outbreaks of E. coli infection occurred between 2010 and 2017 that were associated with vegetable row crops other than Romaine lettuce (i.e., spinach, iceberg lettuce). These outbreaks resulted in a total of 299 illnesses and two known deaths. Nine of these outbreaks were associated with E. coli O157:H7 infection (60.0%), and this pathogen was the cause of both of the known deaths. In 2011, an outbreak of E. coli O157:H7 infection associated with an organic spinach and spring mix salad blend occurred that caused 33 cases in five states (CDC, 2012d). This outbreak was associated with the most illnesses due to the consumption of contaminated vegetable row crops other than Romaine lettuce that took place in a private residential setting. A total of 13 hospitalizations and no known deaths occurred, but two cases of HUS were reported. Illness onset took place from October 18, 2012 to November 12, 2012, and New York state reported 78.8% of the cases. The ages of cases ranged from four to 66, with a median age of 24, and 63% were female. Epidemiological data and laboratory testing of left-over organic spinach and spring mix blend identified pre-packaged leafy greens produced in Massachusetts as the food vehicle of the outbreak, however, the source of the E. coli O157:H7 contamination was not determined.
Sprouts
Three multistate outbreaks of E. coli associated with sprouts occurred between 2010 and 2017. All outbreaks originated from foods consumed in the restaurant setting and affected a range of six to 11 states (based on available CDC data). Clover sprouts were implicated in two of the three outbreaks, while alfalfa sprouts were implicated in the remaining outbreak. The largest of these outbreaks occurred between 2011 and 2012 and was due to E. coli O26 contamination of clover sprouts (CDC, 2012e; FDA, 2012b). A total of 29 illnesses were reported that resulted in seven hospitalizations and zero deaths with illness onset from December 25, 2011 to March 3, 2012. Out of the 11 affected states, the highest case counts were reported from Michigan (10) and Iowa (5). The majority of cases were female (89%), and the median age was 26 years, with a range of nine to 57 years of age (CDC, 2012e). Traceback analysis revealed that clover sprouts were the food vehicle in this outbreak, which were likely produced from contaminated seeds (FDA, 2012b).
L. monocytogenes
Between 2010 and 2017, four multistate listeriosis outbreaks associated with fresh produce occurred in the U.S (Table 3). A total of 173 illnesses occurred due to these outbreaks, which led to a 99.4% known hospitalization rate and a 21.8% known death rate. Two of these four multistate outbreaks implicated fruits, while vegetable row crops and sprouts were confirmed food vehicles in the remaining two outbreaks.
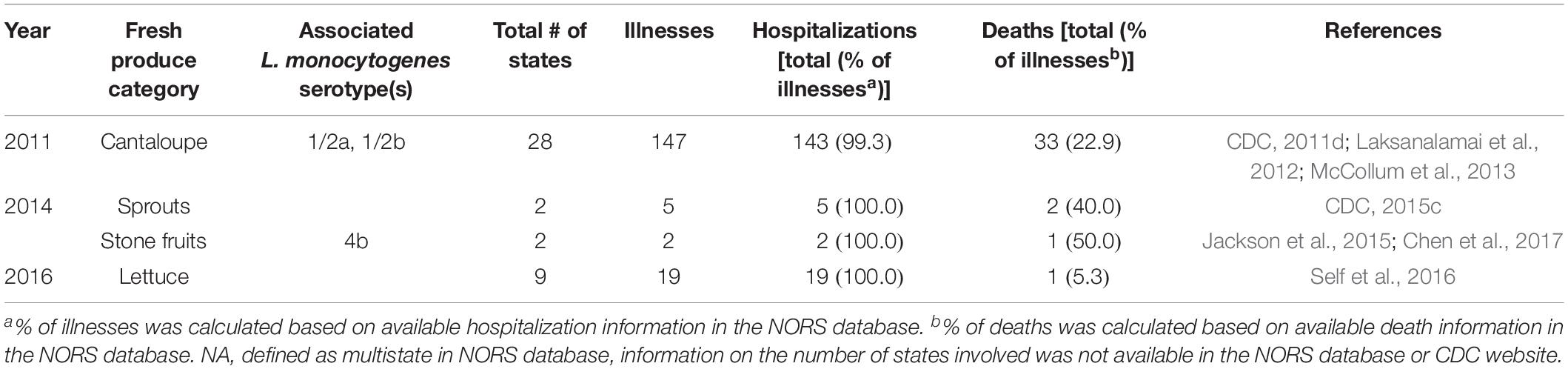
Table 3. Multistate foodborne outbreaks in the U.S. from 2010 to 2017 due to Listeria monocytogenes associated with fresh produce.
Cantaloupe
A total of 147 listeriosis cases were reported to the CDC between July and October 2011, which resulted in 33 known deaths (CDC, 2011d; McCollum et al., 2013). Of the cases with available data, 143 persons out of 145 were hospitalized, three illnesses were in newborns, and four illnesses were in pregnant women, one of which resulted in a miscarriage. Overall, cases were reported in 28 states, with the most cases from Colorado (40), Texas (18), and New Mexico (15). Of patients questioned, 134 out of 144 reported the consumption of cantaloupe within the month before illness. Low sanitation and improper processing equipment were later determined to have been the likely cause of cantaloupe contamination at the implicated farm.
Five subtypes of L. monocytogenes were involved in this outbreak, all of which were isolated from whole cantaloupes and environmental samples that originated from one Colorado-based farm. Initially, three subtypes and the attributable cases were identified through PulseNet (CDC, 2017g). Later, two more subtypes were identified via food and environmental samples, which linked two additional cases. Both L. monocytogenes serotypes 1/2a and 1/2b were implicated in this outbreak (Laksanalamai et al., 2012). Since this outbreak, follow-up studies have been conducted to assess the growth and survival of L. monocytogenes on whole and fresh-cut cantaloupe melons (Ukuku et al., 2012; Huang et al., 2015; Nyarko et al., 2016a, b; Salazar et al., 2017). For example, the growth rate of L. monocytogenes on cantaloupe rind and cut cantaloupe flesh has been modeled at various storage temperatures (Danyluk et al., 2014; Salazar et al., 2017).
Lettuce
Lettuce contaminated with L. monocytogenes caused 19 illnesses in nine states between July 2015 and January 2016 (Self et al., 2016). All cases were hospitalized, one illness was pregnancy related, and one death was reported. Of people who were interviewed, 13 out of 14 reported consuming packaged salad, and nine indicated a specific brand. Routine sampling by the Ohio Department of Agriculture identified L. monocytogenes in the specified brand of packaged salad obtained from a retail location. Whole genome sequencing (WGS) confirmed a high degree of genetic similarity between patient and food isolates. A voluntary recall of packaged salads made at the facility that produced the implicated packaged salad occurred in late January 2016.
Stone Fruits
In July 2014, a packing company recalled various types of stone fruits including whole peaches and nectarines due to suspected Listeria contamination (Jackson et al., 2015). Four patient isolates in PulseNet (CDC, 2017g) matched two pulse-field gel electrophoresis (PFGE) patterns produced by L. monocytogenes isolated from implicated stone fruit once the data were uploaded in August. WGS later confirmed that two of the four isolates from patients in Massachusetts and Minnesota were highly related to outbreak strains. Both confirmed patient isolates were L. monocytogenes serotype 4b (Chen et al., 2017).
Sprouts
The FDA identified L. monocytogenes contamination in mung bean sprouts and irrigation water during routine inspection of a production facility in August 2014. A subsequent investigation cited poor sanitation and equipment maintenance. The company issued a voluntary recall; however, a follow-up inspection demonstrated continued environmental contamination, and the company has since closed its production facility. PFGE and WGS indicated five possible cases of listeriosis associated with this outbreak in Illinois (4) and Michigan (1). All cases were hospitalized, and two deaths were reported (CDC, 2015c). Both interviewed patients indicated consumption of bean sprouts within the month before they became ill.
A Case Study: Caramel Apples
Between October 2014 and January 2015, 35 illnesses from 12 states were reported to the CDC due to consumption of L. monocytogenes-contaminated prepackaged caramel apples (CDC, 2015a). This outbreak warrants discussion as the multi-commodity product consumed (i.e., caramel apple) had a fresh produce component (i.e., whole apple) that was considered low risk because apples have a low internal pH that does not support the growth of pathogens. In addition, the low water activity and temperature of the hot caramel coating applied to the whole apple during processing would hinder pathogen survival if present on the outside surface of the apple. In all, 34 individuals were hospitalized, and 11 cases of listeriosis occurred in pregnant women or newborn infants, including one case that resulted in fetal loss. A total of seven deaths were associated with this outbreak, and at least three were attributable to listeriosis. Three instances of meningitis were reported in healthy children from five to 15 years of age. One individual was determined to be co-infected with two strains of L. monocytogenes.
Although two separate outbreak clusters were identified via both PFGE and WGS, they were investigated together due to the co-infected patient and the comparable regionality and temporality of the clusters. Interviews of 31 infected individuals revealed that 28 had consumed commercially produced prepackaged caramel apples in the month before illness. The remaining three infected individuals interviewed reported consumption of whole or sliced green apples, however, it is unknown if these apples were a food vehicle in the outbreak. Listeria contamination present in an apple packing facility that supplied apples to the implicated caramel apple manufacturers was determined by the FDA to be highly related to the outbreak strains via both PFGE and WGS methods. Follow-up research indicated that stick insertion into the core of the apple creates a microenvironment that allows L. monocytogenes to survive and even grow in an otherwise unsupportive food vehicle (Glass et al., 2015; Salazar et al., 2016). A novel approach for the control of L. monocytogenes in caramel apples through the pretreatment of the wooden sticks with potassium sorbate has since been proposed (Carstens et al., 2018).
S. enterica
S. enterica was the reported etiologic agent for a total of 56 multistate outbreaks associated with fresh produce between 2010 and 2017 (Table 4). A total of 3778 illnesses were linked to these outbreaks, which resulted in a 28.3% known hospitalization rate and 16 known deaths. Fruits were the majority food vehicle implicated in outbreaks attributed to S. enterica (41.1%), followed by seeded vegetables (26.8%), sprouts (21.4%), vegetable row crops (8.9%), and root-underground vegetables (1.8%). However, seeded vegetables (49.3%) rather than fruits were the dominant food vehicle implicated in illnesses due to S. enterica infection (30.1%).
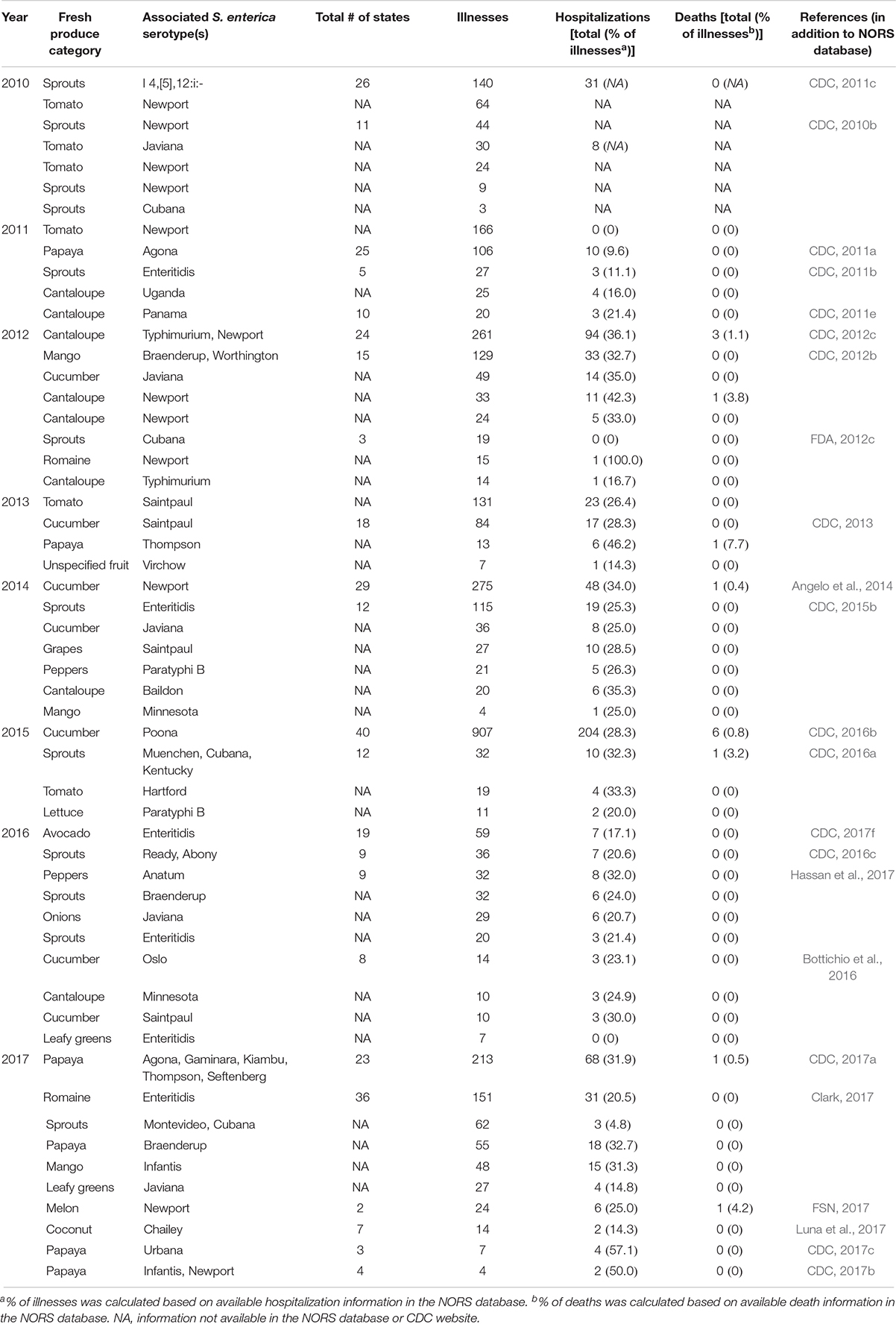
Table 4. Multistate foodborne outbreaks in the U.S. from 2010 to 2017 due to Salmonella enterica associated with fresh produce.
Cucumbers
Of all multistate outbreaks of S. enterica associated with seeded vegetables from 2010 to 2017, 46.7% were linked to cucumbers. These outbreaks resulted in a combined 1375 illnesses, 297 known hospitalizations, and seven known deaths. S. enterica serotypes Saintpaul (2) and Javiana (2) were most frequently confirmed etiologies in these outbreaks; however, Newport (1), Poona (1), and Oslo (1) were also implicated. The largest outbreak in terms of both the number of states affected (40) and case count (907) occurred in 2015 and was linked to cucumbers imported from Mexico that were contaminated with S. enterica Poona (CDC, 2016b). The majority of cases were female (56%) and under 18 years of age (49%) with illness onset from July 3, 2015, to February 29, 2016. A total of 204 hospitalizations and six deaths were reported as a result of this outbreak. A common distributor of cucumbers from Mexico was linked to this outbreak through testing of cucumbers at retail locations as well as at the distributor’s facility. Two voluntary recalls in September 2015 resulted, after which the number of new cases decreased. Although recalled cucumbers should no longer have been available in stores, 127 cases with illness onset after September 24 were reported, which are suspected to have resulted from cross-contamination during shipping or at the retail level.
Melons
Nine outbreaks of S. enterica infection associated with melons occurred between 2010 and 2017, which comprised 39.1% of all S. enterica outbreaks linked to fruits. Of these melon-attributable outbreaks, eight implicated cantaloupe and the other an unspecified type of melon. The most frequent S. enterica serotype involved in single-etiology outbreaks linked to melons was Newport (3), which was also involved in the only multi-etiology outbreak associated with melons along with Typhimurium. These melon-associated outbreaks collectively caused a total of 431 illnesses, 133 known hospitalization, and five deaths.
Cantaloupe was the food vehicle implicated in the largest outbreak of salmonellosis by case count linked to any fruit type during this period (CDC, 2012c). In 2012, 261 illnesses occurred across 24 states that resulted in a 36.0% hospitalization rate and three deaths. The highest number of illnesses occurred in Kentucky (66) followed by Illinois (36) and Indiana (30); however, all three deaths occurred in Kentucky. Illness onset occurred between July 6 and September 16, 2012, and cases were primarily female (55%) with a median case age of 47 years. Epidemiological data and traceback analysis identified one farm as the source of the contaminated cantaloupes (FDA, 2013a). Environmental samples from the farm’s facilities and cantaloupes obtained at the farm matched the PFGE patterns of the S. enterica Typhimurium and Newport strains identified in clinical isolates; the S. enterica Typhimurium outbreak strain was also isolated from cantaloupes at the retail level in Kentucky. A recall of all implicated cantaloupes was announced in August 2012, and it was ultimately determined that poor agricultural and sanitation practices at the implicated farm contributed to the cause of this outbreak (FDA, 2012a).
Other Fruits
A total of 14 outbreaks due to S. enterica linked to fruits other than melons occurred between 2010 and 2017. Papaya was the most frequent food vehicle implicated in these outbreaks (7), followed by mango (3). Between three and 25 states were affected by these outbreaks (CDC, 2011a,e, 2012b, 2017a,b,c; FDA, 2013a), and an assortment of 17 S. enterica serotypes were implicated, with up to five serotypes confirmed in a single outbreak. These fruit-associated outbreaks resulted in a combined total of 706 illnesses, 182 known hospitalizations, and three known deaths.
The largest outbreak of salmonellosis due to case count in this category was associated with imported papaya and involved 23 states (CDC, 2017a). This outbreak was also the largest of four multistate outbreaks that occurred in 2017 associated with papaya contaminated with Salmonella spp. A total of 213 illnesses, 68 known hospitalizations, and one death occurred as a result of this outbreak, with illness onset between May 17, 2017, and October 4, 2017. The median case age was 40, but ages ranged from one to 95. New York reported the majority of cases (71) along with the only death linked to this outbreak, and New Jersey (41) and Virginia (18) reported the second and third most cases. Traceback analysis pointed toward papaya as the food vehicle in this outbreak, and product sampling of papaya from a grocery store by the Maryland Department of Health yielded two of the five S. enterica serotypes implicated. WGS and PFGE methods were used to confirm the implicated serotypes, and Thompson was linked to the majority of cases (144). A single farm in Mexico was the ultimate source of the contaminated papayas, and three recalls of papayas resulted. Recall dates spanned from July 26 to August 7, 2017, however, the outbreak did not end for another 2 months.
Hot Peppers
Two outbreaks between 2010 and 2017 were linked to S. enterica-contaminated peppers, which constituted 13.3% of all outbreaks associated with seeded vegetables during this period. In 2014, S. enterica serotype Paratyphi B was the confirmed etiology of an outbreak linked to mini peppers, while in 2016, a slightly larger outbreak resulted due to hot peppers contaminated with S. enterica Anatum. The later outbreak caused 32 illnesses, eight known hospitalizations, and zero deaths across nine states (Hassan et al., 2017). Illness onset occurred between May 6 and July 9, 2016, and cases ranged from four to 79 years of age, of which 59% were female. The consolidation of peppers from various sources before distribution and the inability of all patients to identify the type of pepper they had consumed before illness prevented the confirmation of a specific variety of hot pepper as the food vehicle in this outbreak. WGS revealed that 19 clinical isolates were highly correlated to an isolate from a contaminated Anaheim pepper, however, this could not be confirmed by epidemiological data.
Sprouts
Between 2010 and 2017, 12 multistate outbreaks linked to sprouts occurred as a result of S. enterica contamination that affected a range of three to 26 states (CDC, 2011b,c, 2015b, 2016a,c; FDA, 2012c). These outbreaks primarily occurred in a restaurant setting and caused a collective 539 illnesses, 82 known hospitalizations, and one known death. Ten different serotypes of S. enterica were implicated in these outbreaks, with up to three serotypes associated with a single outbreak (CDC, 2011c). The largest outbreak associated with sprouts contaminated with S. enterica occurred in 2010 and caused 140 illnesses in 26 states. Illness onset took place from November 1, 2010, to February 9, 2011, and 31 known hospitalizations were reported along with zero deaths. The majority of illnesses occurred in Illinois (70), Missouri (23), and Indiana (13) and the remaining states involved reported four or fewer cases each. The median case age was 28 years, with a range from one to 85 years, and 63% were female. Several cases consumed contaminated alfalfa sprouts contained in sandwiches from a single fast food restaurant chain. Environmental sampling of the implicated farm identified the confirmed outbreak strain of S. enterica I 4,[5],12:i:- in water run-off, but not in product samples.
The second largest outbreak linked to sprouts contaminated with S. enterica occurred in 2014 and affected 12 states with 115 cases (CDC, 2015b). S. enterica serotype Enteritidis was the confirmed etiology of this outbreak and cases were localized in the Eastern U.S. with the highest case counts reported from Massachusetts (36) and New York (22). One illness occurred in Montana; however, this case had traveled to the Eastern U.S., where the exposure is suspected to have occurred. Illness onset occurred from September 30 to December 15, 2014, and of cases with information available, the hospitalization rate was 25.3%. The majority of cases were female (64%), and the median case age was 32, although ages ranged from one to 83 years of age. Epidemiological data indicated that bean sprouts were the likely food vehicle in this outbreak, and a common supplier was identified who destroyed the remaining product.
Conclusion
In summary, a total of 85 multistate outbreaks that had a confirmed etiology and were associated with fresh produce occurred from 2010 to 2017 in the U.S. The outbreaks discussed in this review draw attention to factors that may contribute to the occurrence of multistate outbreaks. One such factor is that bacterial contamination can be propagated along the distribution chain, which may increase the scope of an outbreak and traceback difficulty. Specific examples include cross-contamination through the consolidation of fresh produce before distribution and in displays at the retail level. Another frequent contributor to multistate outbreaks in fresh produce was poor agricultural practices, especially in relation to melons. Sprouts were repeat offenders as food vehicles for various foodborne pathogens, and further regulation or more regular inspections may be needed to avoid future incidents. Imported fresh produce was also implicated as a food vehicle in several multistate outbreaks. The FDA has the authority to collect and analyze samples from imported goods as these products must meet FDA standards (FDA, 2018a). The FDA can also enact import alerts which enable the detention of imported goods without physical examination and require the importer to demonstrate that the product is in compliance. In the case of imported fresh produce, more frequent screening or import alerts may be necessary for these products to ensure that they are safe for consumption. The case-study included in this review underscores the risks associated with the use of fresh produce in multi-component food products. Surveillance of foodborne diseases and produce recall have proven critical to prevent and reduce the number of illnesses associated with outbreaks during the assessed timeframe.
As a result of a large multistate outbreak of E. coli O157:H7 more recently in 2018, the industry has initiated voluntary labeling of packaged Romaine lettuce with the growing region and harvest date per FDA recommendation (FDA, 2018b). The extension of this labeling practice to other fresh produce items would enable more effective traceback analysis and protect consumers in the case of future outbreaks. It is essential to pinpoint the causes and mechanisms by which contamination occurs in multistate outbreaks in order to prevent their occurrence. This task is difficult, as in some cases, the food vehicle is not confirmed; or a single source of contamination along the farm to fork continuum cannot be identified. In other cases, the root cause of the contamination is not isolated, which is critical for the development of future prevention measures.
Author Contributions
CC worked on the literature review, data collection, data analysis, and wrote the manuscript. JS and CD analyzed the data, wrote the manuscript, and reviewed the manuscript.
Funding
This work was supported in part by NIH R01 Grant No. R01AI116914 from the NIH Institute of Allergy and Infectious Diseases.
Conflict of Interest
The authors declare that the research was conducted in the absence of any commercial or financial relationships that could be construed as a potential conflict of interest.
Acknowledgments
We sincerely thank Mary Lou Tortorello and Diana Stewart for critical reading of the manuscript.
References
Ackers, M. L., Mahon, B. E., Leahy, E., Goode, B., Damrow, T., and Hayes, P. S. (1998). An outbreak of Escherichia coli O157:H7 infections associated with leaf lettuce consumption. J. Infect. Dis. 177, 1588–1593.
Angelo, K. M., Chu, A., Anand, M., Nguyen, T. A., Bottichio, L., Wise, M., et al. (2014). Centers for disease, and prevention, outbreak of Salmonella Newport infections linked to cucumbers–United States. MMWR Morb. Mortal. Wkly. Rep. 64, 144–147.
Bailey, J. S., Stern, N. J., Fedorka-Cray, P., Craven, S. E., Cox, N. A., and Cosby, D. E. (2001). Sources and movement of Salmonella through integrated poultry operations: a multistate epidemiological investigation. J. Food Prot. 64, 1690–1697. doi: 10.4315/0362-028x-64.11.1690
Banatvala, N., Griffin, P. M., Greene, K. D., Barrett, T. J., Bibb, W. F., and Green, J. H. (2001). The United States national prospective hemolytic uremic syndrome study: microbiologic, serologic, clinical, and epidemiologic findings. J. Infect. Dis. 183, 1063–1070. doi: 10.1086/319269
Berry, E. D., Wells, J. E., Bono, J. L., Woodbury, B. L., Kalchayanand, N., and Norman, K. N. (2015). Effect of proximity to a cattle feedlot on Escherichia coli O157:H7 contamination of leafy greens and evaluation of the potential for airborne transmission. Appl. Environ. Microbiol. 81, 1101–1110. doi: 10.1128/AEM.02998-14
Bottichio, L., Medus, C., Sorenson, A., Donovan, D., Sharma, R., Dowell, N., et al. (2016). Outbreak of Salmonella oslo infections linked to persian cucumbers - United States, 2016. MMWR Morb. Mortal. Wkly. Rep. 65, 1430–1433. doi: 10.15585/mmwr.mm655051a3
Brar, P. K., and Danyluk, M. D. (2013). Salmonella transfer potential during hand harvesting of tomatoes under laboratory conditions. J. Food Prot. 76, 1342–1349. doi: 10.4315/0362-028X.JFP-13-048
Buchholz, A. L., Davidson, G. R., Marks, B. P., Todd, E. C., and Ryser, E. T. (2012a). Quantitative transfer of Escherichia coli O157:H7 to equipment during small-scale production of fresh-cut leafy greens. J. Food Prot. 75, 1184–1197. doi: 10.4315/0362-028X.JFP-11-489
Buchholz, A. L., Davidson, G. R., Marks, B. P., Todd, E. C., and Ryser, E. T. (2012b). Transfer of Escherichia coli O157:H7 from equipment surfaces to fresh-cut leafy greens during processing in a model pilot-plant production line with sanitizer-free water. J. Food Prot. 75, 1920–1929. doi: 10.4315/0362-028X.JFP-11-558
Buchholz, A. L., Davidson, G. R., Marks, B. P., Todd, E. C., and Ryser, E. T. (2014). Tracking an Escherichia coli O157:H7-contaminated batch of leafy greens through a pilot-scale fresh-cut processing line. J. Food Prot. 77, 1487–1494. doi: 10.4315/0362-028X.JFP-14-058
Carstens, C. K., Salazar, J. K., Bathija, V. M., Narula, S. S., Wang, P., and Tortorello, M. L. (2018). Control of Listeria monocytogenes in caramel apples by use of sticks pretreated with potassium sorbate. J. Food Prot. 81, 1921–1928. doi: 10.4315/0362-028X.JFP-18-175
Castillo, A., Mercado, I., Lucia, L. M., Martinez-Ruiz, Y., Ponce, de Leon, J., et al. (2004). Salmonella contamination during production of cantaloupe: a binational study. J. Food Prot. 67, 713–720. doi: 10.4315/0362-028x-67.4.713
CDC, (2010a). Multistate Outbreak of Human E. coli O145 Infections Linked to Shredded Romaine Lettuce From a Single Processing Facility (Final Upadate). Available from: https://www.cdc.gov/ecoli/2010/shredded-romaine-5-21-10.html (accessed May 10, 2019).
CDC, (2010b). Multistate Outbreak of Human Salmonella Newport Infections Linked to Raw Alfalfa Sprouts (Final Update). Available from: https://www.cdc.gov/salmonella/2010/newport-alfalfa-sprout-6-29-10.html (accessed May 10, 2019)Google Scholar
CDC, (2011a). Multistate Outbreak of Human Salmonella Agona Infections Linked to Whole, Fresh Imported Papayas (Final Update). Available from: https://www.cdc.gov/salmonella/2011/papayas-8-29-2011.html (accessed April 4, 2019)Google Scholar
CDC, (2011b). Multistate Outbreak of Human Salmonella Enteritidis Infections Linked to Alfalfa Sprouts and Spicy Sprouts (Final Update). Available from: https://www.cdc.gov/salmonella/2011/alfalfa-spicy-sprouts-7-6-2011.html?CDC_AA_refVal=https%3A%2F%2Fwww.cdc.gov%2Fsalmonella%2Fsprouts-enteritidis0611%2F062611%2Findex.html (accessed April 4, 2019)Google Scholar
CDC, (2011c). Multistate Outbreak of Human Salmonella I 4,[5],12:i:- Infections Linked to Alfalfa Sprouts (Final Update). Available from: https://www.cdc.gov/salmonella/2010/alfalfa-sprouts-2-10-11.html (accessed May 10, 2019).
CDC, (2011d). Multistate outbreak of listeriosis associated with Jensen Farms cantaloupe–United States, August-September 2011. MMWR Morb. Mortal. Wkly Rep. 60, 1357–1358.
CDC, (2011e). Multistate Outbreak of Salmonella Panama Infections Linked to Cantaloupe (Final Update). Available from: https://www.cdc.gov/salmonella/2011/cantaloupes-6-23-2011.html?CDC_AA_refVal=https%3A%2F%2Fwww.cdc.gov%2Fsalmonella%2Fpanama0311%2F062311%2Findex.html (accessed April 4, 2019).
CDC, (2012a). Multistate outbreak of E. coli O157:H7 infections linked to Romaine lettuce (final update). Available from: https://www.cdc.gov/ecoli/2011/romaine-lettace-3-23-12.html (accessed April 1, 2019).
CDC, (2012b). Multistate Outbreak of Salmonella Braenderup Infections Associated with Mangoes (Final Update). Available from: https://www.cdc.gov/salmonella/braenderup-08-12/index.html (accessed April 4, 2019).
CDC, (2012c). Multistate outbreak of Salmonella Typhimurium and Salmonella Newport infections linked to cantaloupe (final update). Available from: https://www.cdc.gov/salmonella/typhimurium-cantaloupe-08-12/index.html (accessed April 4, 2019)Google Scholar
CDC, (2012d). Multistate Outbreak of Shiga Toxin-Producing Escherichia coli O157:H7 Infections Linked to Organic Spinach and Spring Mix Blend (Final Update). Available at: https://www.cdc.gov/ecoli/2012/O157H7-11-12/index.html
CDC, (2012e). Multistate Outbreak of Shiga Toxin-Producing Escherichia coli O26 Infections Linked to Raw Clover Sprouts at Jimmy John’srestaurants (Final Update). Available from: https://www.cdc.gov/ecoli/2012/O26-02-12/index.html (accessed April 4, 2019).
CDC, (2013). Multistate Outbreak of Salmonella Saintpaul Infections Linked to Imported Cucumbers (Final Update). Available from: https://www.cdc.gov/salmonella/saintpaul-04-13/index.html (accessed April 4, 2019).
CDC, (2014). Multistate Outbreak of Shiga Toxin-Producing Escherichia coli O121 Infections Linked to Raw Clover Sprouts (Final Update). 2. Available from: https://www.cdc.gov/ecoli/2014/o121-05-14/index.html (accessed April 1, 2019).
CDC, (2015a). Multistate Outbreak of Listeriosis Linked to Commercially Produced Prepackaged Caramel Apples Made from Bidart Bros. Apples (Final Update). Available from: http://www.cdc.gov/listeria/outbreaks/caramel-apples-12-14/index.html (accessed March 19, 2015).
CDC, (2015b). Multistate Outbreak of Salmonella Enteritidis Infections Linked to Bean Sprouts (Final Update). Available at: https://www.cdc.gov/salmonella/enteritidis-11-14/index.html (accessed April 4, 2019).
CDC, (2015c). Wholesome Soy Products, Inc. Sprouts and Investigation of Human Listeriosis Cases (Final Update). Available from: https://www.cdc.gov/listeria/outbreaks/bean-sprouts-11-14/index.html (accessed March 1, 2019)Google Scholar
CDC, (2016a). Multistate Outbreak of Salmonella Infections Linked to Alfalfa Sprouts From One Contaminated Seed Lot (Final Update). Available from: https://www.cdc.gov/salmonella/muenchen-02-16/index.html (accessed April 4, 2019)Google Scholar
CDC, (2016b). Multistate Outbreak of Salmonella Poona Infections Linked to Imported Cucumbers (Final Update). Available from: https://www.cdc.gov/salmonella/poona-09-15/index.html (accessed April 4, 2019).
CDC, (2016c). Multistate Outbreak of Salmonella Reading and Salmonella Abony Infections Linked to Alfalfa Sprouts (Final Update). Available from: https://www.cdc.gov/salmonella/reading-08-16/index.html (accessed April 4, 2019).
CDC, (2016d). Multistate Outbreak of Shiga Toxin-Producing Escherichia coli O157 Infections Linked to Alfalfa Sprouts Produced by Jack & The Green Sprouts (Final Update). Available from: https://www.cdc.gov/ecoli/2016/o157-02-16/index.html (accessed April 3 2019).
CDC, (2017a). Multistate Outbreak of Salmonella Infections Linked to Imported Maradol Papayas (Final Update). Available from: https://www.cdc.gov/salmonella/kiambu-07-17/index.html (accessed May 10, 2019).
CDC, (2017b). Multistate Outbreak of Salmonella Newport and Salmonella Infantis Infections Linked to Imported Maradol Papayas (Final Update). Available from: https://www.cdc.gov/salmonella/newport-09-17/index.html (accessed May 10, 2019)Google Scholar
CDC, (2017c). Multistate Outbreak of Salmonella Urbana Infections Linked to Imported Maradol Papayas (Final Update). Available from: https://www.cdc.gov/salmonella/urbana-09-17/index.html (accessed May 10, 2019).
CDC, (2017d). National Outbreak Reporting System (NORS). Available from: https://wwwn.cdc.gov/norsdashboard/ (accessed October 27, 2018).
CDC, (2017e). National Outbreak Reporting System (NORS). Available from: https://wwwn.cdc.gov/norsdashboard/ (accessed April 4, 2019).
CDC, (2017f). Oklahoma NORS Report, 2016. Available from: http://www.outbreakdatabase.com/reports/OK2016-0144.pdf (accessed April 4, 2019).
CDC, (2017g). PulseNet. Available from: https://www.cdc.gov/pulsenet/index.html (accessed April 4, 2019).
CDC, (2018). Multistate Outbreak of Shiga Toxin-Producing Escherichia coli O157:H7 Infections Linked to Leafy Greens (Final Update). Available from: https://www.cdc.gov/ecoli/2017/o157h7-12-17/index.html (accessed May 10, 2019).
Cernicchiaro, N., Pearl, D. L., McEwen, S. A., Harpster, L., Homan, H. J., and Linz, G. M. (2012). Association of wild bird density and farm management factors with the prevalence of E. coli O157 in dairy herds in Ohio (2007-2009). Zoonoses Public Health 59, 320–329. doi: 10.1111/j.1863-2378.2012.01457.x
Chen, Y., Luo, Y., Pettengill, J., Timme, R., Melka, D., Doyle, M., et al. (2017). Singleton sequence type 382, an emerging clonal group of Listeria monocytogenes associated with three multistate outbreaks linked to contaminated stone fruit, caramel apples, and leafy green salad. J. Clin. Microbiol. 55, 931–941. doi: 10.1128/JCM.02140-16
Chlebicz, A., and Slizewska, K. (2018). Campylobacteriosis, salmonellosis, yersiniosis, and listeriosis as zoonotic foodborne diseases: a review. Int. J. Environ. Res. Public Health 15:E863. doi: 10.3390/ijerph15050863
Clark, M. (2017). Multistate Outbreak of Salmonella Enteritidis Linked to Romaine Lettuce. 2017. Available from: http://www.outbreakdatabase.com/details/2017-multistate-outbreak-of-salmonella-enteritidis-linked-to-romaine-lettuce/?state=60&country=US (accessed May 10, 2019)Google Scholar
Croxen, M. A., Law, R. J., Scholz, R., Keeney, K. M., Wlodarska, M., and Finlay, B. B. (2013). Recent advances in understanding enteric pathogenic Escherichia coli. Clin. Microbiol. Rev. 26, 822–880. doi: 10.1128/CMR.00022-13
Crum-Cianflone, N. F. (2008). Salmonellosis and the gastrointestinal tract: more than just peanut butter. Curr. Gastroenterol. Rep. 10, 424–431. doi: 10.1007/s11894-008-0079-7
da Silva, E. P., and De Martinis, E. C. (2013). Current knowledge and perspectives on biofilm formation: the case of Listeria monocytogenes. Appl. Microbiol. Biotechnol. 97, 957–968. doi: 10.1007/s00253-012-4611-1
Danyluk, M. D., Friedrich, L. M., and Schaffner, D. W. (2014). Modeling the growth of Listeria monocytogenes on cut cantaloupe, honeydew and watermelon. Food Microbiol. 38, 52–55. doi: 10.1016/j.fm.2013.08.001
Dargatz, D. A., Fedorka-Cray, P. J., Ladely, S. R., Kopral, C. A., Ferris, K. E., and Headrick, M. L. (2003). Prevalence and antimicrobial susceptibility of Salmonella spp. isolates from US cattle in feedlots in 1999 and 2000. J. Appl. Microbiol. 95, 753–761. doi: 10.1046/j.1365-2672.2003.02034.x
Davidson, G. R., Kaminski-Davidson, C. N., and Ryser, E. T. (2017). Persistence of Escherichia coli O157:H7 during pilot-scale processing of iceberg lettuce using flume water containing peroxyacetic acid-based sanitizers and various organic loads. Int. J. Food Microbiol. 248, 22–31. doi: 10.1016/j.ijfoodmicro.2017.02.006
Dewey-Mattia, D., Manikonda, K., Hall, A. J., Wise, M. E., and Crowe, S. J. (2018). Surveillance for foodborne disease outbreaks- United States, 2009-2015. MMWR Surveill. Summ. 67, 1–11. doi: 10.15585/mmwr.ss6710a1
Doane, C. A., Pangloli, P., Richards, H. A., Mount, J. R., Golden, D. A., and Draughon, F. A. (2007). Occurrence of Escherichia coli O157:H7 in diverse farm environments. J. Food Prot. 70, 6–10. doi: 10.4315/0362-028x-70.1.6
Fabrega, A., and Vila, J. (2013). Salmonella enterica serovar Typhimurium skills to succeed in the host: virulence and regulation. Clin. Microbiol. Rev. 26, 308–341. doi: 10.1128/CMR.00066-12
Farber, J. M., and Peterkin, P. I. (1991). Listeria monocytogenes, a food-borne pathogen. Microbiol. Rev. 55, 476–511.
FDA, (1999). FDA Survey of Imported Fresh Produce FY Field Assignment. 2001. Available from: https://www.fda.gov/Food/GuidanceRegulation/GuidanceDocumentsRegulatoryInformation/ProducePlantProducts/default.htm (accessed February 28, 2019)Google Scholar
FDA, (2012a). FDA Form 483 Inspectional Observations- Chamberlain Farms, Inc. Available from: https://www.fda.gov/downloads/AboutFDA/CentersOffices/OfficeofGlobalRegulatoryOperationsandPolicy/ORA/ORAElectronicReadingRoom/UCM322103.pdf (accessed April 8, 2019)Google Scholar
FDA, (2012b). Investigation of Multistate Outbreak of E. coli O26 Infections Linked to Consumption of Raw Clover Sprouts. Available from: http://wayback.archive-it.org/7993/20171114154952/https:/www.fda.gov/Food/RecallsOutbreaksEmergencies/Outbreaks/ucm292083.htm (accessed April 1, 2019)Google Scholar
FDA, (2012c). Sprouts: FDA Investigation Summary - Multistate Outbreak of Salmonella Cubana Infections Associated with Sprouts From an Arizona Sprouter. Available from: http://wayback.archive-it.org/7993/20171114154939/https:/www.fda.gov/Food/RecallsOutbreaksEmergencies/Outbreaks/ucm369067.htm (accessed April 5, 2019)Google Scholar
FDA, (2013a). FDA Investigation Summary: Multistate Outbreak of Salmonella Typhimurium and Salmonella Newport Infections Linked to Cantaloupe Grown at Chamberlain Farms in Southwest Indiana. Available from: http://wayback.archive-it.org/7993/20171114154943/https:/www.fda.gov/Food/RecallsOutbreaksEmergencies/Outbreaks/ucm315879.htm (accessed April 8, 2019)Google Scholar
FDA, (2013b). Lettuce: FDA Investigation Summary- Multistate Outbreak of E. coli O157:H7 Illnesses Linked to Ready-to-Eat Salads. Available from: http://wayback.archive-it.org/7993/20171114154923/https:/www.fda.gov/Food/RecallsOutbreaksEmergencies/Outbreaks/ucm374327.htm (accessed April 1, 2019)Google Scholar
FDA, (2017). Refrigerator Thermometers: Cold Facts About Food Safety. Available from: https://www.fda.gov/food/resourcesforyou/consumers/ucm253954.htm (accessed August 18, 2018)Google Scholar
FDA, (2018a). Actions and Enforcement. Available from: https://www.fda.gov/industry/import-program-food-and-drug-administration-fda/actions-enforcement (accessed October 14, 2019)Google Scholar
FDA, (2018b). Statement from FDA Commissioner Scott Gottlieb, M.D., on the Current Romaine Lettuce E. coli O157:H7 Outbreak Investigation. Available from: https://www.fda.gov/news-events/press-announcements/statement-fda-commissioner-scott-gottlieb-md-current-romaine-lettuce-e-coli-o157h7-outbreak (accessed June 7, 2019)Google Scholar
FDA, (2019a). Food Safety and Modernization Act (FSMA). Available from: https://www.fda.gov/food/guidance-regulation-food-and-dietary-supplements/food-safety-modernization-act-fsma (accessed May 13, 2019)Google Scholar
FDA, (2019b). FSMA Final Rule on Produce Safety. 2018. Available from: https://www.fda.gov/food/food-safety-modernization-act-fsma/fsma-final-rule-produce-safety (accessed May 13, 2019)Google Scholar
Fierer, J., and Guiney, D. G. (2001). Diverse virulence traits underlying different clinical outcomes of Salmonella infection. J. Clin. Invest. 107, 775–780. doi: 10.1172/jci12561
FSN, (2017). Pre-cut melon linked to outbreak in the Pacific Northwest. Available from: https://www.foodsafetynews.com/2017/12/pre-cut-melon-linked-to-outbreak-in-the-pacific-northwest/ (accessed May 10, 2019)Google Scholar
Garg, N., Churey, J. J., and Splittstoesser, D. F. (1990). Effect of processing conditions on the microflora of fresh-cut vegetables. J. Food Prot. 53, 701–703. doi: 10.4315/0362-028X-53.8.701
Glass, K. A., Golden, M. C., Wanless, B. J., Bedale, W., and Czuprynski, C. (2015). Growth of Listeria monocytogenes within a caramel-coated apple microenvironment. mBio 6:e1232-15. doi: 10.1128/mBio.01232-15
Gould, L. H., Walsh, K. A., Vieira, A. R., Herman, K., Williams, I. T., Hall, A. J., et al. (2013). Surveillance for foodborne disease outbreaks- United States, 1998-2008. MMWR Surveill. Summ. 62, 1–34.
Greene, S. K., Daly, E. R., Talbot, E. A., Demma, L. J., Holzbauer, S., and Patel, N. J. (2005). Recurrent multistate outbreak of Salmonella Newport associated with tomatoes from contaminated fields. Epidemiol. Infect. 136, 157–165. doi: 10.1017/s095026880700859x
Greig, J. D., Todd, E. C., Bartleson, C. A., and Michaels, B. S. (2007). Outbreaks where food workers have been implicated in the spread of foodborne disease. Part 1. Description of the problem, methods, and agents involved. J. Food Prot. 70, 1752–1761. doi: 10.4315/0362-028x-70.7.1752
Hassan, R., Rounds, J., Sorenson, A., Leos, G., Concepcion-Acevedo, J., Griswold, T., et al. (2017). Multistate outbreak of Salmonella anatum infections linked to imported hot peppers - United States, May-July 2016. MMWR Morb. Mortal. Wkly. Rep. 66, 663–667. doi: 10.15585/mmwr.mm6625a2
Hernandez-Milian, A., and Payeras-Cifre, A. (2014). What is new in listeriosis? Biomed. Res. Int. 2014:358051. doi: 10.1155/2014/358051
Herwaldt, B. L. (2000). Cyclospora cayetanensis: a review, focusing on the outbreaks of cyclosporiasis in the 1990s. Clin. Infect. Dis. 31, 1040–1057. doi: 10.1086/314051
Hoffmann, S., Batz, M. B., and Morris, J. G.Jr. (2012). Annual cost of illness and quality-adjusted life year losses in the United States due to 14 foodborne pathogens. J. Food Prot. 75, 1292–1302. doi: 10.4315/0362-028X.JFP-11-417
Hohmann, E. L. (2001). Nontyphoidal salmonellosis. Clin. Infect. Dis. 32, 263–269. doi: 10.1086/318457
Huang, J., Luo, Y., and Nou, X. (2015). Growth of Salmonella enterica and Listeria monocytogenes on fresh-cut cantaloupe under different temperature abuse scenarios. J. Food Prot. 78, 1125–1131. doi: 10.4315/0362-028X.JFP-14-468
Huang, S. W. (2004). Global Trade Patterns in Fruits and Vegetables. Agriculture and Trade Report Number WRS-04-06. Available from: http://eumed-agpol.iamm.fr/doc/global_trade_fruits_vegetables.pdf (accessed February 21, 2019)Google Scholar
Hussein, H. S., and Bollinger, L. (2005). Prevalence of Shiga toxin-producing Escherichia coli in beef cattle. J. Food Prot. 68, 2224–2241. doi: 10.4315/0362-028x-68.10.2224
ICMSF, (1996). In Microorganisms in Foods 5, Characteristics of Microbial Pathogens. London: Blackie Academic and Professional.
Jackson, B. R., Salter, M., Tarr, C., Conrad, A., Harvey, E., Steinbock, L., et al. (2015). Centers for disease, and prevention, notes from the field: listeriosis associated with stone fruit–United States, 2014. MMWR Morb. Mortal. Wkly. Rep. 64, 282–283.
Janisiewicz, W. J., Conway, W. S., Brown, M. W., Sapers, G. M., and Fratamico, P. (1999). Fate of Escherichia coli O157:H7 on fresh-cut apple tissue and its potential for transmission by fruit flies. Appl. Environ. Microbiol. 65, 1–5.
Jay, M. T., Cooley, M., Carychao, D., Wiscomb, G. W., Sweitzer, R. A., and Crawford-Miksza, L. (2007). Escherichia coli O157:H7 in feral swine near spinach fields and cattle, central California coast. Emerg. Infect. Dis. 13, 1908–1911. doi: 10.3201/eid1312.070763
Jimenez, M., Siller, J. H., Valdez, J. B., Carrillo, A., and Chaidez, C. (2007). Bidirectional Salmonella enterica serovar Typhimurium transfer between bare/glove hands and green bell pepper and its interruption. Int. J. Environ. Health Res. 17, 381–388. doi: 10.1080/09603120701372664
Keen, J. E., Wittum, T. E., Dunn, J. R., Bono, J. L., and Durso, L. M. (2006). Shiga-toxigenic Escherichia coli O157 in agricultural fair livestock, United States. Emerg. Infect. Dis. 12, 780–786.
Kenney, S. J., Anderson, G. L., Williams, P. L., Millner, P. D., and Beuchat, L. R. (2006). Migration of Caenorhabditis elegans to manure and manure compost and potential for transport of Salmonella newport to fruits and vegetables. Int. J. Food Microbiol. 106, 61–68. doi: 10.1016/j.ijfoodmicro.2005.05.011
Khamesipour, F., Lankarani, K. B., Honarvar, B., and Kwenti, T. E. (2018). A systematic review of human pathogens carried by the housefly (Musca domestica L.). BMC Public Health 18:1049. doi: 10.1186/s12889-018-5934-3
King, A. D., Magnusson, J. A., Torok, T., and Goodman, N. (1991). Microflora and storage quality of partially processed lettuce. J. Food Sci. 56, 459–461. doi: 10.1111/j.1365-2621.1991.tb05303.x
Laksanalamai, P., Joseph, L. A., Silk, B. J., Burall, L. S., Tarr, C. L., Gerner-Smidt, P., et al. (2012). Genomic characterization of Listeria monocytogenes strains involved in a multistate listeriosis outbreak associated with cantaloupe in US. PLoS One. 7:e42448. doi: 10.1371/journal.pone.0042448
Le Bouguenec, C., and Servin, A. L. (2006). Diffusely adherent Escherichia coli strains expressing Afa/Dr adhesins (Afa/Dr DAEC): hitherto unrecognized pathogens. FEMS Microbiol. Lett. 256, 185–194. doi: 10.1111/j.1574-6968.2006.00144.x
Lim, J. Y., Yoon, J., and Hovde, C. J. (2010). A brief overview of Escherichia coli O157:H7 and its plasmid O157. J. Microbiol. Biotechnol. 20, 5–14. doi: 10.4014/jmb.0908.08007
Liu, D., Lawrence, M. L., Ainsworth, A. J., and Austin, F. W. (2005). Comparative assessment of acid, alkali and salt tolerance in Listeria monocytogenes virulent and avirulent strains. FEMS Microbiol. Lett. 243, 373–378. doi: 10.1016/j.femsle.2004.12.025
Lomonaco, S., Nucera, D., and Filipello, V. (2015). The evolution and epidemiology of Listeria monocytogenes in Europe and the United States. Infect. Genet. Evol. 35, 172–183. doi: 10.1016/j.meegid.2015.08.008
Luna, S., Taylor, M., Galanis, E., Asplin, R., Huffman, J., Wagner, D., et al. (2017). Outbreak of Salmonella chailey infections linked to precut coconut pieces - United States and Canada. MMWR Morb. Mortal. Wkly. Rep. 67, 1098–1100. doi: 10.15585/mmwr.mm6739a5
Madden, J. M. (1992). Microbial pathogens in fresh produce- the regulatory perspective. J. Food Prot. 55, 821–823. doi: 10.4315/0362-028X-55.10.821
Magnuson, J. A., King, A. D. Jr., and Torok, T. (1990). Microflora of partially processed lettuce. Appl. Environ. Microbiol. 56, 3851–3854.
Marchetti, R., Casadei, M. A., and Guerzoni, M. E. (1992). Microbial population dynamics in ready-to-use vegetable salads. Ital. J. Food Sci. 2, 92–108.
Marder, E. P., Garman, K. N., Ingram, L. A., and Dunn, J. R. (2014). Multistate outbreak of Escherichia coli O157:H7 associated with bagged salad. Foodborne Pathog. Dis. 11, 593–595. doi: 10.1089/fpd.2013.1726
Materon, L. A., Martinez-Garcia, M., and McDonald, V. (2007). Identification of sources of microbial pathogens on cantaloupe rinds from pre-harvest to post-harvest operations. World J. Microbiol. Biotechnol. 23, 1281–1287. doi: 10.1007/s11274-007-9362-2
McCollum, J. T., Cronquist, A. B., Silk, B. J., Jackson, K. A., O’Connor, K. A., Cosgrove, S., et al. (2013). Multistate outbreak of listeriosis associated with cantaloupe. N. Engl. J. Med. 369, 944–953. doi: 10.1056/NEJMoa1215837
McEvoy, J. L., Luo, Y., Conway, W., Zhou, B., and Feng, H. (2009). Potential of Escherichia coli O157:H7 to grow on field-cored lettuce as impacted by postharvest storage time and temperature. Int. J. Food Microbiol. 128, 506–509. doi: 10.1016/j.ijfoodmicro.2008.08.008
Nataro, J. P., and Kaper, J. B. (1998). Diarrheagenic Escherichia coli. Clin. Microbiol. Rev. 11, 142–201.
Nguyen-the, C., and Carlin, F. (1994). The microbiology of minimally processed fresh fruits and vegetables. Crit. Rev. Food Sci. Nutr. 34, 371–401.
Nyarko, E., Kniel, K. E., Millner, P. D., Luo, Y., Handy, E. T., Reynnells, R., et al. (2016a). Survival and growth of Listeria monocytogenes on whole cantaloupes is dependent on site of contamination and storage temperature. Int. J. Food Microbiol. 234, 65–70. doi: 10.1016/j.ijfoodmicro.2016.06.030
Nyarko, E., Kniel, K. E., Reynnells, R., East, C., Handy, E. T., Luo, Y., et al. (2016b). Survival and growth of Listeria monocytogenes on Fresh-Cut “Athena” and “Rocky Ford” cantaloupes during storage at 4 degrees C and 10 degrees C. Foodborne Pathog. Dis. 13, 587–591. doi: 10.1089/fpd.2016.2160
Pouillot, R., Klontz, K. C., Chen, Y., Burall, L. S., Macarisin, D., and Doyle, M. (2015). Infectious dose of Listeria monocytogenes in outbreak linked to ice cream, United States. Emerg. Infect. Dis. 22, 2113–2119. doi: 10.3201/eid2212.160165
Ragon, M., Wirth, T., Hollandt, F., Lavenir, R., Lecuit, M., Le Monnier, A., et al. (2008). A new perspective on Listeria monocytogenes evolution. PLoS Pathog. 4:e1000146. doi: 10.1371/journal.ppat.1000146
Rangel, J. M., Sparling, P. H., Crowe, C., Griffin, P. M., and Swerdlow, D. L. (1982). Epidemiology of Escherichia coli O157:H7 outbreaks, United States, -2002. Emerg. Infect. Dis. 11, 603–609.
Ravishankar, S., Zhu, L., and Jaroni, D. (2010). Assessing the cross contamination and transfer rates of Salmonella enterica from chicken to lettuce under different food-handling scenarios. Food Microbiol. 27, 791–794. doi: 10.1016/j.fm.2010.04.011
Rekhy, R., and McConchie, R. (2014). Promoting consumption of fruit and vegetables for better health. Have compaigns delivered on the goals? Appetite 79, 113–123. doi: 10.1016/j.appet.2014.04.012
Richardson, L. C., Bazaco, M. C., Parker, C. C., Dewey-Mattia, D., Golden, N., Jones, K., et al. (2017). An updated scheme for categorizing foods implicated in foodborne disease outbreaks: a tri-agency collaboration. Foodborne Pathog. Dis. 14, 701–710. doi: 10.1089/fpd.2017.2324
Salazar, J. K., Carstens, C. K., Bathija, V. M., Narula, S. S., Parish, M., and Tortorello, M. L. (2016). Fate of Listeria monocytogenes in fresh apples and caramel apples. J. Food Prot. 79, 696–702. doi: 10.4315/0362-028X.JFP-15-442
Salazar, J. K., Sahu, S. N., Hildebrandt, I. M., Zhang, L., Qi, Y., Liggans, G., et al. (2017). Growth Kinetics of Listeria monocytogenes in cut produce. J. Food Prot. 80, 1328–1336. doi: 10.4315/0362-028X.JFP-16-516
Scallan, E., Hoekstra, R. M., Angulo, F. J., Tauxe, R. V., Widdowson, M. A., and Roy, S. L. (2011). Foodborne illness acquired in the United States–major pathogens. Emerg. Infect. Dis. 17, 7–15. doi: 10.3201/eid1701.P11101
Self, J. L., Conrad, A., Stroika, S., Jackson, A., Burnworth, L., Beal, J., et al. (2016). Notes from the field: outbreak of listeriosis associated with consumption of packaged salad - United States and Canada, 2015-2016. MMWR Morb. Mortal. Wkly. Rep. 65, 879–881. doi: 10.15585/mmwr.mm6533a6
Sharps, C. P., Kotwal, G., and Cannon, J. L. (2012). Human norovirus transfer to stainless steel and small fruits during handling. J. Food Prot. 75, 1437–1446. doi: 10.4315/0362-028X.JFP-12-052
Slayton, R. B., Turabelidze, G., Bennett, S. D., Schwensohn, C. A., Yaffee, A. Q., Khan, F., et al. (2011). Outbreak of Shiga toxin-producing Escherichia coli (STEC) O157:H7 associated with romaine lettuce consumption. PLoS One 8:e55300. doi: 10.1371/journal.pone.0055300
Smolinski, H. S., Wang, S., Ren, L., Chen, Y., Kowalcyk, B., Thomas, E., et al. (2018). Transfer and redistribution of Salmonella Typhimurium LT2 and Escherichia coli O157:H7 during pilot-scale processing of baby spinach, cilantro, and romaine lettuce. J. Food Prot. 81, 953–962. doi: 10.4315/0362-028X.JFP-17-420
Solomon, E. B., Yaron, S., and Matthews, K. R. (2002). Transmission of Escherichia coli O157:H7 from contaminated manure and irrigation water to lettuce plant tissue and its subsequent internalization. Appl. Environ. Microbiol. 68, 397–400. doi: 10.1128/aem.68.1.397-400.2002
Sproston, E. L., Macrae, M., Ogden, I. D., Wilson, M. J., and Strachan, N. J. (2006). Slugs: potential novel vectors of Escherichia coli O157. Appl. Environ. Microbiol. 72, 144–149. doi: 10.1128/aem.72.1.144-149.2006
Suslow, T. V., Oria, M. P., Beuchat, L. R., Garrett, E. H., Parish, M. E., Harris, L. J., et al. (2003). Chapter II: production practices as risk factors in microbial food safety and fresh and fresh-cut produce. CRFSFS 2, 38–77. doi: 10.1111/j.1541-4337.2003.tb00030.x
Swaminathan, B., and Gerner-Smidt, P. (2007). The epidemiology of human listeriosis. Microbes Infect. 9, 1236–1243. doi: 10.1016/j.micinf.2007.05.011
Talley, J. L., Wayadande, A. C., Wasala, L. P., Gerry, A. C., Fletcher, J., DeSilva, U., et al. (2009). Association of Escherichia coli O157:H7 with filth flies (Muscidae and Calliphoridae) captured in leafy greens fields and experimental transmission of E. coli O157:H7 to spinach leaves by house flies (Diptera: Muscidae). J. Food Prot. 72, 1547–1552. doi: 10.4315/0362-028x-72.7.1547
Taormina, P. J., Beuchat, L. R., Erickson, M. C., Ma, L., Zhang, G., and Doyle, M. P. (2009). Transfer of Escherichia coli O157:H7 to iceberg lettuce via simulated field coring. J. Food Prot. 72, 465–472. doi: 10.4315/0362-028x-72.3.465
Ukuku, D. O., Olanya, M., Geveke, D. J., and Sommers, C. H. (2012). Effect of native microflora, waiting period, and storage temperature on Listeria monocytogenes serovars transferred from cantaloupe rind to fresh-cut pieces during preparation. J. Food Prot. 75, 1912–1919. doi: 10.4315/0362-028X.JFP-12-191
USDA, (2018). Food Availability (Per Capita) Data System. “Fruit and Vegetables”. Available at: https://www.ers.usda.gov/data-products/food-availability-per-capita-data-system/ (accessed February 2, 2019).
Uyttendaele, M., Jaykus, L. A., Amoah, P., Chiodini, A., Cunliffe, D., and Jacxsens, L. (2015). Microbial hazards in irrigation water: standards, norms, and testing to manage use of water in fresh produce primary production. CRFSFS 14, 336–356. doi: 10.1111/1541-4337.12133
van Elsas, J. D., Semenov, A. V., Costa, R., and Trevors, J. T. (2011). Survival of Escherichia coli in the environment: fundamental and public health aspects. ISME J. 5, 173–183. doi: 10.1038/ismej.2010.80
Wachtel, M. R., Whitehand, L. C., and Mandrell, R. E. (2002a). Association of Escherichia coli O157:H7 with preharvest leaf lettuce upon exposure to contaminated irrigation water. J. Food Prot. 65, 18–25. doi: 10.4315/0362-028x-65.1.18
Wachtel, M. R., Whitehand, L. C., and Mandrell, R. E. (2002b). Prevalence of Escherichia coli associated with a cabbage crop inadvertently irrigated with partially treated sewage wastewater. J. Food Prot. 65, 471–475.
Wasala, L., Talley, J. L., Desilva, U., Fletcher, J., and Wayadande, A. (2013). Transfer of Escherichia coli O157:H7 to spinach by house flies, Musca domestica (Diptera: Muscidae). Phytopathology 103, 373–380. doi: 10.1094/PHYTO-09-12-0217-FI
WHO, (2003). Diet, Nutrition and the Prevention of Chronic Diseases. Report of a Joint FAO/WHO Expert Consultation. WHO Technical Report Series, No. 916. Available from: https://apps.who.int/iris/bitstream/handle/10665/42665/WHO_TRS_916.pdf;sequence=1 (accessed Februrary 20, 2019).
Wiedemann, A., Virlogeux-Payant, I., Chausse, A. M., Schikora, A., and Velge, P. (2014). Interactions of Salmonella with animals and plants. Front. Microbiol. 5:791. doi: 10.3389/fmicb.2014.00791
Yang, S. C., Lin, C. H., Aljuffali, I. A., and Fang, J. Y. (2017). Current pathogenic Escherichia coli foodborne outbreak cases and therapy development. Arch. Microbiol. 199, 811–825. doi: 10.1007/s00203-017-1393-y
Keywords: multistate, foodborne outbreaks, produce, E. coli, L. monocytogenes, S. enterica, diarrheal disease
Citation: Carstens CK, Salazar JK and Darkoh C (2019) Multistate Outbreaks of Foodborne Illness in the United States Associated With Fresh Produce From 2010 to 2017. Front. Microbiol. 10:2667. doi: 10.3389/fmicb.2019.02667
Received: 22 August 2019; Accepted: 01 November 2019;
Published: 22 November 2019.
Edited by:
Dario De Medici, National Institute of Health (ISS), ItalyReviewed by:
Anca Ioana Nicolau, Dunarea de Jos University, RomaniaWerner Ruppitsch, Austrian Agency for Health and Food Safety (AGES), Austria
Copyright © 2019 Carstens, Salazar and Darkoh. This is an open-access article distributed under the terms of the Creative Commons Attribution License (CC BY). The use, distribution or reproduction in other forums is permitted, provided the original author(s) and the copyright owner(s) are credited and that the original publication in this journal is cited, in accordance with accepted academic practice. No use, distribution or reproduction is permitted which does not comply with these terms.
*Correspondence: Charles Darkoh, Charles.Darkoh@uth.tmc.edu