- 1Mahidol International Dental School, Faculty of Dentistry, Mahidol University, Bangkok, Thailand
- 2Department of Microbiology, Faculty of Science, Mahidol University, Bangkok, Thailand
- 3Food Technology and Innovation Research Center of Excellence, School of Agricultural Technology, Walailak University, Nakhon Si Thammarat, Thailand
- 4Department of Chemistry and Center for Innovation in Chemistry (PERCH-CIC), Faculty of Science, Mahidol University, Bangkok, Thailand
This study examines treatments of the bacterial pathogen Staphylococcus aureus, namely, in the context of its being a major cause of subclinical bovine mastitis. Such infections caused by S. aureus among dairy cows are difficult to detect and can easily become chronic, leading to reduced productivity and large losses for dairy manufacturers. In this study, the role of alternative sigma factor B (σB), which has been shown to be a global regulator for S. aureus infections, was explored in a mastitis-causing S. aureus strain, RF122. For comparison with the wild-type strain, a sigB null (ΔsigB) mutant was constructed and analyzed for its phenotypes and transcriptome. Our study found that σB is essential for biofilm formation as the ΔsigB mutant strain produced significantly less biofilm than did the wild-type strain at 48 h. σB is involved in response to H2O2 stress. However, σB plays a minor or no role in resistance to antiseptics (e.g., povidone-iodine and chlorhexidine), resistance to tested antibiotics, hemolysin activity, and invasion ability. RNA sequencing identified 225 σB-dependent genes, of which 171 are positively regulated and 54 are negatively regulated. The identified genes are involved in stress response, pathogenesis, and metabolic mechanisms. Quantitative TaqMan RT-PCR was performed to verify the RNA sequencing results; i.e., σB is a positive regulator for asp23, sarA, katA, yabJ, sodA, SAB2006c, and nrdD expressions. In the RF122 strain, σB plays a role in biofilm formation, general stress response (e.g., H2O2), and regulation of virulence factors and virulence-associated genes.
Introduction
Bovine mastitis is an inflammation of the mammary gland (Viguier et al., 2009), which negatively impacts the dairy industry, leading to economic losses due to reduced milk production and increased treatment expenses (Wall et al., 2005; Le Maréchal et al., 2011b). Staphylococcus aureus is one of the most prevalent causative agents of subclinical and clinical mastitis (Dego et al., 2002; Azizoglu et al., 2013). However, unlike clinical mastitis, subclinical mastitis shows few visible symptoms in infected cows (Viguier et al., 2009; Le Maréchal et al., 2011a). The inability to rapidly detect subclinical mastitis leads to a high prevalence of such infections being observed in dairy farms (Gruet et al., 2001). Subclinical mastitis is caused by several species of bacteria including S. aureus (Gruet et al., 2001). Treatment of S. aureus subclinical mastitis can be more difficult because S. aureus is known to invade phagocytes where the concentration of antibiotics is sublethal. Persistent S. aureus can lead to deep-seated abscesses, which further serve as a niche for chronic infection (Hébert et al., 2000; Gruet et al., 2001; Brouillette and Malouin, 2005; Pieterse and Todorov, 2010). Antibiotic-resistant S. aureus, particularly relating to β-lactam drugs, has been reported among mastitis-causing strains (Gruet et al., 2001). Thus, alternative treatments (e.g., anti-CD40 monoclonal antibody) or novel drug targets (e.g., transcription factors) are needed strategies to combat bovine mastitis (Wallemacq et al., 2012).
In bacteria, alternative sigma (σ) factors are transcription factors involved in promoter recognition and RNA polymerase recruitment (Schulthess et al., 2011). They play crucial roles in regulation of gene expression in response to change in environments (Schulthess et al., 2011). The most studied alternative σ factor in Firmicutes (e.g., Bacillus and Staphylococcus spp.) is σB. σB-regulated genes include those involved in general stress response, virulence, capsule formation, and biofilm formation (Nicholas et al., 1999; Hecker et al., 2007; Meier et al., 2007; Kim et al., 2008; Cebrián et al., 2009; Lauderdale et al., 2009; Schulthess et al., 2009, 2011). In S. aureus, σB controls expression of virulence genes via regulation of accessory regulator A (sarA) and accessory gene regulator (agr), which regulate production of extracellular proteins (secreted enzymes and toxins) and cell surface proteins (Bischoff et al., 2001). This study aimed to further explore the roles of σB and the identification of σB regulon in mastitis-causing S. aureus strains. The results validated the potential of σB as an alternative therapeutic target for S. aureus-induced subclinical bovine mastitis.
Methods
Bacterial Strains
Mastitis-causing S. aureus strain RF122 (received as a gift from Professor Vivek Kapur, Penn State University) was used as a wild-type strain. Escherichia coli strain DH5α was used to prepare competent cells for plasmid propagation in the plasmid construction step. S. aureus was cultured in brain heart infusion (BHI) or tryptic soy medium (Difco), and E. coli was cultured in Luria–Bertani (LB) medium (Difco) at 37°C with 200 rpm agitation. For long-term preservation, 20% sterilized glycerol was added into overnight culture and stored at −80°C.
Mutant Construction
A pKSV7 ΔsigB plasmid was constructed using the splicing by overlapping extension polymerase chain reaction (SOE-PCR) method (Wiedmann et al., 1998; Yakhnin and Babitzke, 2004). The SOE-PCR primers for mutant construction are listed in Supplementary Table 1. A pKSV7 ΔsigB plasmid (717 bp in-frame deletion) was transformed into E. coli DH5α for propagation. Competent cells of S. aureus and electroporation were performed as described in Monk et al. (2012). The allelic exchange mutagenesis was carried out following the previously reported procedures of Yakhnin and Babitzke (2004). Deletion of sigB in ΔsigB mutant was confirmed by DNA sequencing (Macrogen, Korea). Growth of wild type and ΔsigB in tryptic soy broth (TSB) media at 37°C with 200 rpm agitation was determined every 2 h for 12 h.The growth experiments were performed in triplicates.
RNA Sequencing and Data Analysis
The post-exponential phase samples, defined as an OD600 of 1.0 with an additional 3 h incubation of S. aureus wild-type and ΔsigB mutant strains, were collected for RNA sequencing (RNA-Seq). RNAprotect (Qiagen) was added to bacterial cultures to stop cellular activity and to stabilize RNA. RNA was extracted using TRIzol (Invitrogen) followed by an RNeasy Mini Kit (Qiagen). Total RNA samples were sent to Molecular Genomics (Singapore) for RNA-Seq. RNA quality and quantity were determined using Agilent 2100 Bioanalyzer (Agilent Technologies, Santa Clara, CA) and Agilent RNA 6000 Pico Kit (Agilent Technologies, Santa Clara, CA). A HiSeq 2500 sequencer (Illumina) was selected as a platform for RNA-Seq in this study. The quality of output sequences was determined by FastQC program version 0.11.7 (https://www.bioinformatics.babraham.ac.uk/projects/fastqc/). Sequences with a Phred quality score of more than 30 were used for further analysis. Genome sequence and gene functions of S. aureus RF122 from NCBI (ASM900v1) were used as reference information to a generated bowtie index using Bowtie2 (Langmead et al., 2018). TopHat 2.1.1 was employed for alignment of RNA sequences in reference to the bowtie index (Kim et al., 2013). HTSeq-count was used to count reads for differential expression analysis (Pyl et al., 2014). DeSeq2 package version 3.2 in R program was also used to test differential expression based on negative binomial generalized linear models (Love et al., 2014). The cut-off values of expression fold change (wild type vs. ΔsigB) determined by DeSeq2 were set at 2, i.e., ≥2 for upregulated genes and −2 or less for downregulated genes, and p-adjusted (padj) values <0.05 were reported as differentially expressed in wild type and in the ΔsigB mutant strains.
qRT-PCR Analysis
Total RNA was extracted from the post–exponential phase culture using TRIzol reagent (Invitrogen), Mini-BeadBeater (Bio-Spec Products), and RNeasy Mini Kit (Qiagen). RNA yield was determined by NanoDrop (ND-1000, NanoDrop Technologies, Inc.). Quantitative reverse transcription-PCR (qRT-PCR) was performed on seven selected genes [asp23, sarA, catalase gene (katA), yabJ, aldehyde dehydrogenase gene (SAB2006c), sodA, and nrdD] to verify σB-dependence identified by RNA-Seq using LightCycler 480 (Roche). Expression levels were normalized with those of the housekeeping gene rpoB. For each RNA sample, qRT-PCR was performed in duplicate reactions. Experiments were performed in biological triplicates.
Phenotypic Characterization of ΔsigB Mutant Strain
Antibiotic and Antiseptic Susceptibilities in S. aureus Wild Type and ΔsigB Strains
S. aureus wild type and ΔsigB mutant strains were grown in TSB at 37°C overnight. Each culture was diluted in 0.85% sterile saline to a density equivalent to 0.5 McFarland standards (approximately OD625 of 0.08–0.13) [Clinical and Laboratory Standards Institute (CLSI, 2012)] and spread onto Mueller Hinton (MH) agar plates using a sterile cotton swab. The antibiotic disks were aseptically placed on the surface of agar. The plates were incubated at 37°C for 18 h, and the inhibitory zones were measured by a digital Vernier caliper. The results were interpreted according to the Clinical and Laboratory Standards Institute CLSI (2012). The antibiotic disks (Oxoid, UK) used were ampicillin (10 μg), penicillin (10 μg), oxacillin (1 μg), cefoxitin (30 μg), ceftiofur (30 μg), cephalothin (30 μg), tetracycline (30 μg), streptomycin (10 μg), erythromycin (15 μg), sulfamethoxazole with trimethoprim (25 μg), sulfamethoxazole (25 μg), and novobiocin (30 μg). The interpretative zone diameters of ceftiofur and streptomycin are not stated in CLSI criteria. Based on previously reported results, susceptibility of ceftiofur was considered ≥21 mm (Soares et al., 2014) and ≤16 mm for resistance to streptomycin (Shittu and Lin, 2006). These antibiotics were selected in this study due to their frequent use in bovine mastitis treatment (Azizoglu et al., 2013).
Iodine and chlorhexidine are typical antiseptics used in dairy farms; their minimum inhibitory concentrations (MICs) and minimum bactericidal concentrations (MBCs) were determined based on the protocol described as follows. Five hundred microliters of OD600 0.4 culture was added into 50 ml of TSB, and the culture was incubated for 6 h. The culture was diluted into MH broth to achieve 5 × 105 colony-forming units (CFUs)/ml and used for the experiments. Ten percent of povidone-iodine solution containing 1% of available iodine (Azizoglu et al., 2013) was used to prepare the concentrations of iodine. The final concentrations of iodine were 0.0001, 0.0005, 0.001, 0.005, 0.01, 0.05, 0.1, 0.15, 0.2, and 0.25%. The treated bacterial culture was grown at 37°C for 18 h. Growth was measured by monitoring OD600, and MIC was reported as the lowest antiseptic concentration which inhibits S. aureus growth. Concentrations that inhibited growth were streaked out onto tryptic soy agar (TSA) plates to determine the lowest antiseptic concentration which completely killed S. aureus or MBC.
The same protocol was used to determine the MIC and MBC for chlorhexidine. The final concentrations of chlorhexidine diacetate were 0.00001, 0.00002, 0.00005, 0.0001, 0.0002, and 0.0004%.
Oxidative Stress Survival Assay
Both wild type and ΔsigB mutant strains were grown to the post-exponential phase. Culture CFUs were determined and recorded by serial dilution and spreading onto TSA. One milliliter of culture was mixed with cumene hydroperoxide (CHP; Sigma) at a final concentration of 7.5 mM. The untreated and treated bacterial culture mixtures were incubated at 37°C, with 200 rpm agitation, for 15, 30, and 60 min. At each time point, samples were spread onto TSA to determine CFU. Survival abilities of wild-type and ΔsigB mutant strains were compared from “log reduction,” which was calculated from log CFU per milliliter of CHP-untreated minus log CFU per milliliter of CHP-treated for each time point. For H2O2 survival, 1 ml of post-exponential phase culture was mixed with H2O2 at 880 mM final concentration and incubated at 37°C, with 200 rpm agitation, for 10 and 20 min. CFU was determined, and survival abilities were compared from log reductions between untreated and treated samples at each time point. All oxidative stress survival assays were repeated at least three times.
Biofilm Formation
To quantify the biofilm formation of S. aureus, a microplate assay was performed based on the previously reported protocol (Mitchell et al., 2010) with slight modifications as follows. A colony of wild-type or ΔsigB mutant strain was inoculated into TSB containing 0.5% glucose and incubated overnight at 37°C, 200 rpm. The culture was diluted to 0.5 McFarland in TSB containing 0.5% glucose and transferred into a 96-well flat-bottom microtiter plate containing a fresh TSB medium in the ratio 1:1. The plates were incubated at 37°C for 24 and 48 h. Culture broth was discarded, and the wells (with attached biofilm) were washed three times with 200 μl of phosphate buffer saline (PBS) pH 7.4. The wells were stained for 30 min with 1% of crystal violet, washed with 100 μl of water to remove excess staining solution, and let dry. Two hundred microliters of 30% acetic acid solution was added to dissolve the crystal violet. The resulting solution was measured at OD560 using the microplate reader (Wallac Victor 1420, PerkinElmer, USA). Biofilm formation was evaluated in three replicates.
Hemolysis Assay
Sheep blood was washed three times in cold PBS and centrifuged at 4,000 rpm for 10 min at 4°C (Rotina 380R, Hettich, UK) (Lauderdale et al., 2009). Sheep red blood cells were then diluted to a final concentration of 1%. The post-exponential phase cultures were 2-fold serially diluted for seven times starting from 109 to 5 × 105 with PBS pH 7.4. One hundred microliters of undiluted and diluted culture were mixed with 900 μl of 1% sheep red blood cells and incubated at 37°C for 30 min. The mixtures were centrifuged at 4,000 rpm for 10 min at 4°C (Centrifuge 5417R, Eppendorf, USA). Hemolytic activity was measured at OD545. Positive control was defined as a complete lysis of red blood cells by 1% Triton-100. The hemolytic unit was defined as the inverse of dilution when the OD545 reading was more than 50% of complete hemolysis [(positive control reading – negative control reading)/2] (Menzies and Kernodle, 1994).
Cell Invasion Assay
A549 (human lung epithelial cell line) was used to study the invasion assay. In routine A549 maintenance, Dulbecco's Modified Eagle Medium (DMEM) (Gibco) with 10% fetal bovine serum (FBS), 1% final concentration of penicillin/streptomycin, and 5 mM of L-glutamine was used. The cell invasion assay was performed as previously described (Liang et al., 2006). In brief, ~2 × 105 cells of A549 (human lung epithelial cell line) were seeded in 24-well plates and incubated for 18 h at 37°C, 5% CO2. A549 cells were infected by S. aureus wild-type or ΔsigB mutant strain by adding 0.5 ml DMEM containing 5 × 105 CFU of bacteria. The multiplicity of infection value used in this study was 2.5 (2.5 bacterial cells: 1 A549 cell). The plate was incubated at 37°C for 10 min followed by centrifugation at 100 × g for 5 min and continued incubation for 2 h. The culture medium was removed and discarded. Subsequently, the A549-infected cells were washed three times with PBS, pH 7.4. To eliminate extracellular bacteria, 1 ml of DMEM supplemented with 10% FBS, 100 μg/ml gentamicin, and 5 μg/ml lysostaphin was added to each well. The plate was incubated for 1 h; then the supernatant was removed. All wells were washed three times with PBS and then trypsinized by 0.25% trypsin-EDTA. After 5 min, the cells in each well were carefully collected and kept on ice. Four hundred microliters of 0.025% Triton X-100 was added to each tube. The numbers of released bacteria from A549 were determined by CFU on TSA. The percentage of the invasion of ΔsigB mutant was calculated and compared with that of the wild-type strain, which was considered to be 100% (Liang et al., 2006).
Statistical Analysis
An independent t-test and Mann–Whitney U-test were used to analyze data for phenotypic characterization and qRT-PCR. Statistical significance was reported when the p ≤ 0.05.
Results
In this study, ΔsigB strain in RF122 was constructed, and the role of σB in stress response was assessed by comparing the phenotypes of the wild type to those of the mutant. In contrast to the previous studies, our study show that the growth of the ΔsigB mutant strain was different from the wild type (Figure 1). Although the specific growth rates of wild type (0.41 h−1) and ΔsigB mutant (0.44 h−1) were not different, the maximum numbers of CFUs reached in batch cultures were significantly different (p = 0.04). The average maximum CFU reached by wild type was 1.1 × 1010 CFU/ml while that of ΔsigB mutant was 2.5 × 109 CFU/ml. In fact, CFU values during the post-exponential phase and early stationary phase (t = 6, 8, 10, 12) were significantly higher in the wild-type strain. Macroscopically, colonies of the ΔsigB mutant strain on TSA showed less production of the yellow pigment, potentially staphyloxanthin, when compared to the wild-type strain (Supplementary Figure 1). Wild-type and ΔsigB mutant strains were further characterized for their transcriptomes and phenotypes. The following section lists the key findings of this study.
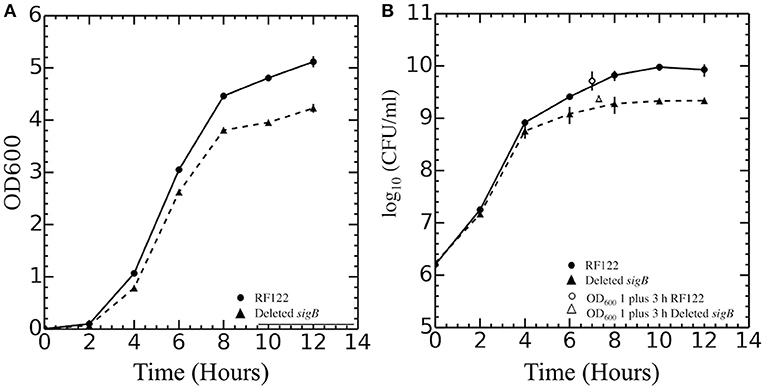
Figure 1. Growth curves of Staphylococcus aureus RF122 and ΔsigB mutant strains. (A) Relationship between OD600 of each strain and time of incubation. (B) Relationship between log10 colony-forming units (CFU) per milliliter of each strain and time of incubation. The experiment was performed in three biological replicates.
σB Regulon in RF122 Strain
σB actively functions throughout the growth phase and peaks at the early stationary phase (Senn et al., 2005; Pané-Farré et al., 2006; Schulthess et al., 2009). Using isogenic ΔsigB and RF122 wild-type strains grown to post–exponential phases, we performed RNA-Seq to determine the members of σB regulon. The raw sequence data (.fastq files) of all samples and summary of RNA-Seq results were submitted to the SRA database under SRA accession number PRJNA548307. The overview of the RNA-Seq result is shown in Supplementary Table 2. We identified 171 positively regulated genes with higher transcription levels in the wild-type strain than in the ΔsigB mutant strain (fold change ≥ 2) (Supplementary Table 3). Fifty-four genes were expressed in higher levels in the ΔsigB mutant strain than in the wild type (fold change ≤ −2) and were defined as negatively σB-regulated genes (Supplementary Table 3). In S. aureus, sigB operon is composed of rsbU, rsbV, rsbW, and sigB (Hecker et al., 2007). The expression of genes in the sigB operon was lower in the ΔsigB mutant compared to the wild-type strain, but only the expression levels of the sigB and rsbW genes differed more than 2-fold with padj being <0.05 (Supplementary Table 3). All affected genes were classified into 16 classes from 17 functional classes of genes in S. aureus. The only one functional class not affected by σB was signal transduction. The highest affected class was the central intermediary metabolism class (Table 1). The percentage of positively and negatively σB-regulated genes in each functional class is shown in Figure 2. Most of the functional classes were affected positively or negatively by σB (Figure 2). Five functional classes in which all affected genes were positively σB-regulated were DNA metabolism, unknown function, mobile and extrachromosomal element functions, transcription, and purines, pyrimidines, nucleosides, and nucleotides (Figure 2). Among the upregulated genes, we found genes involved in general stress response and virulence such as alkaline shock protein (asp23), accessory regulator A (sarA), capsule polysaccharide synthesis enzyme (cap), hemolysin, and translation initiation inhibitor (yabJ). Detoxifying enzymes are catalase and superoxide dismutase (sodA). Several genes are involved in carbohydrate metabolism (glycolysis/gluconeogenesis) as well as fatty acid metabolism such as trimethylamine dehydrogenase and aldehyde dehydrogenase. Genes involved in cell wall synthesis, murE and murD, were also positively regulated by σB.
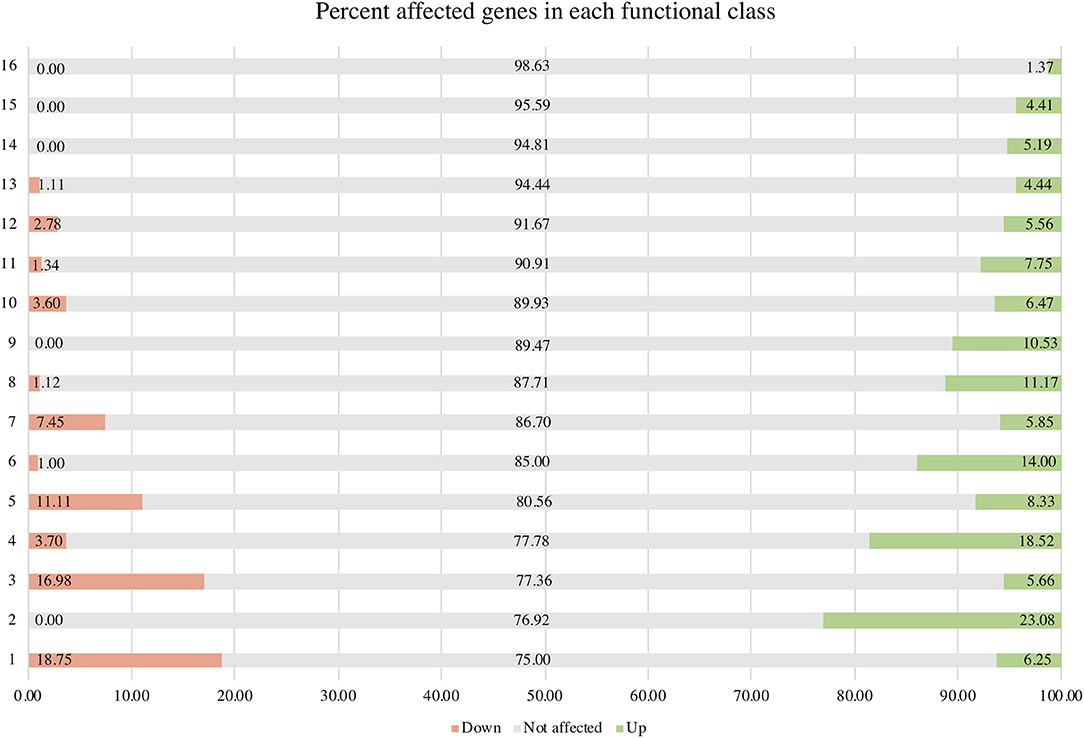
Figure 2. Percentage of positively and negatively σB-regulated genes in each functional class. Functional classes: 1 = central intermediary metabolism; 2 = purines, pyrimidines, nucleoside, and nucleotides; 3 = amino acid biosynthesis; 4 = cellular processes; 5 = fatty acid and phospholipid metabolism; 6 = cellular proteins; 7 = transporter and binding proteins; 8 = cell envelope; 9 = transcription; 10 = energy metabolism; 11 = hypothetical protein; 12 = biosynthesis of cofactors, prosthetic groups, and carriers; 13 = regulatory functions; 14 = mobile and extrachromosomal element function; 15 = unknown function; and 16 = DNA metabolism.
In order to validate the RNA-Seq results, seven genes identified by RNA-Seq to be σB dependent were selected for qRT-PCR. These genes were asp23, sarA, katA, yabJ, sodA, SAB2006c, and nrdD. Expression levels of these genes were normalized to the expression level of a housekeeping gene rpoB. As expected, the wild-type strain showed significantly higher levels of asp23, sarA, katA, yabJ, SAB2006c, sodA, and nrdD expressions when compared to the ΔsigB mutant (p < 0.05). The results correlated to the data obtained from RNA-Seq (Figure 3). The fold change from qRT-PCR of each gene was determined and compared with the RNA-Seq result (Supplementary Table 3). All selected genes showed fold change values of more than 1.5-fold, the cutoff value to determine the differential expression in qRT-PCR. Based on the results from qRT-PCR, the asp23 gene exhibited the highest fold change of 7.14 while the catalase gene showed the lowest fold change of 1.53 (Supplementary Table 4). However, the highest fold change from these seven genes from RNA-Seq was sodA (13.80), contradicting with the fold change result from qRT-PCR (1.60) (Supplementary Table 4).
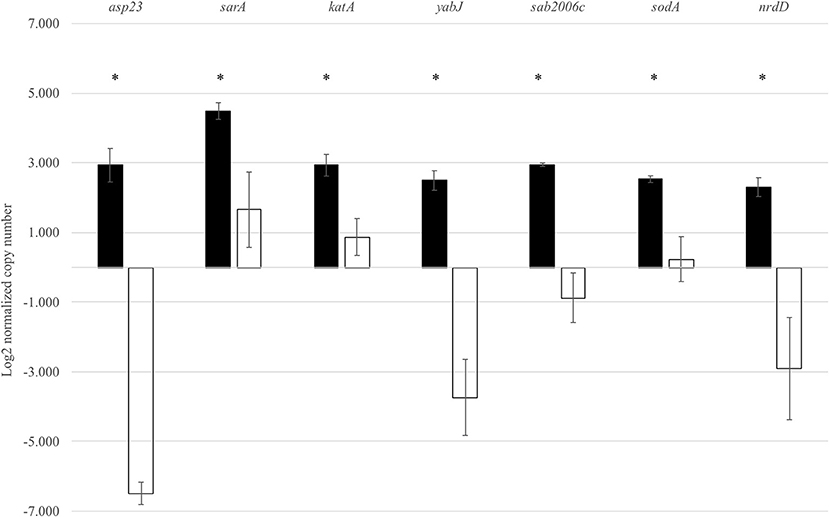
Figure 3. Expression of seven selected genes in both the wild type and ΔsigB mutant determined by qRT-PCR. Differences in mRNA levels of all genes are statistically significant when compared between RF122 wild-type (black) and ΔsigB mutant (white) strains. *significant difference between wild-type and ΔsigB mutant strains at p < 0.05.
σB in Mastitis-Causing S. aureus May Be Involved in Susceptibility to Cell-Wall-Targeting Antibiotics, but Not Antiseptics
As the RNA-Seq results implicated the role of σB in cell wall synthesis, we therefore explored the implication of σB in the susceptibility of mastitis-causing S. aureus to cell-wall-targeting antibiotics. The wild-type RF122 and the ΔsigB mutant strain were subjected to disk diffusion and microdilution assay to determine their susceptibility against cell-wall-targeting antibiotics as well as commonly used antibiotics and antiseptics for bovine mastitis treatments. The results of the antibiotic susceptibility assay indicated that the ΔsigB mutant and the wild type have the same antibiotic profile (Table 2) to cell-wall-targeting β-lactam antibiotics such as ampicillin, penicillin G, cephalothin, cefoxitin, ceftiofur, and oxacillin. The results of the tested antiseptics, iodine, and chlorhexidine, show that both the wild-type and ΔsigB mutant strains have comparable MIC values of 0.005% iodine and 0.00002% chlorhexidine. The MBC values of iodine and chlorhexidine were 0.01 and 0.0002% in both strains, respectively.
σB Plays a Role in Hydrogen Peroxide Stress but Not CHP Stress
The σB proteins of other Gram-positive bacteria such as Bacillus subtilis and Listeria monocytogenes are involved in oxidative stress response (Hecker et al., 2007). Here, we also investigated the role of σB in response to oxidative stressors. The wild-type and ΔsigB mutant strains were assessed for their abilities to survive upon exposure to oxidants. The solution of 880 mM H2O2 was used as an oxidant with exposure times of 10 and 20 min. The results are shown in Table 3. Log reduction values of the ΔsigB mutant at 10 and 20 min are statistically different when compared to those of the wild type (p < 0.05). This finding correlates with previous studies in that σB contributes to the ability to tolerate H2O2 (Kullik et al., 1998; Giachino et al., 2001).
The ability of wild-type and ΔsigB mutant strains to survive inorganic oxidant stress, 7.5 mM CHP, was also determined. Log reduction values of both strains are presented in Table 3. Log reduction values between wild-type and ΔsigB mutant strains at 15, 30, and 60 min are not statistically significant.
σB Is Important to Biofilm Formation but Does Not Influence Cell Invasion and Hemolysis Activity in RF122
The ability of bacteria to form biofilm could suggest the cause of chronic mastitis. Hence, the biofilm formation assay was performed in the wild-type and ΔsigB mutant strains. As shown in Table 3, at 24 h, the wild type and ΔsigB mutant could form biofilm in comparable extent. However, at 48 h, the ΔsigB mutant formed significantly less biofilm than the wild-type strain (p = 0.038). Our results support and correlate with the results of previous studies that σB contributes to biofilm formation (Lauderdale et al., 2009; Mitchell et al., 2010).
We also investigated the ability of ΔsigB mutant and wild-type strains to invade the host cells. Both strains did not show significant difference in the ability to invade host cells (data not shown, p = 0.11). In addition, we also looked into the ability of the wild-type and ΔsigB mutant strains to lyse red blood cells. We found that the hemolytic activities of wild-type and ΔsigB mutant strains were also not significantly different; the hemolytic units of the wild type and ΔsigB mutant were 4.
Discussion
σB in S. aureus is involved in stress response and highly expressed during the stationary phase (Hecker et al., 2007). In previous studies, most of the sigB mutant strains were constructed by insertion mutations, and inactivation of σB was done through disruption of a gene encoding regulator of sigma B rsbU located upstream of the rsbV–rsbW–sigB operon (Bischoff et al., 2004; Tuchscherr et al., 2015). The growth characteristics of ΔsigB mutants in these other backgrounds (e.g., human isolate Newman and murine isolate LS1) were comparable to those of their wild-type strains (Bischoff et al., 2004; Tuchscherr et al., 2015). In contrast, in this study, the ΔsigB mutant strain was constructed by an in-frame deletion of the sigB gene. Our ΔsigB mutant strain showed impaired growth in comparison to the RF122 wild type. The reason comparable growth characteristics were found between the wild type and its ΔsigB mutant in previous studies could be due to the leaky activity of σB from its intact sigB gene under the activation pathway independent of RsbU. In addition to change in growth characteristics, RF122 ΔsigB also appears off-white. σB has been shown to positively regulate the SAB2176 gene, which encodes a secretory antigen staphyloxanthin precursor. Lower expression of this gene in the ΔsigB mutant could correlate to less production of the yellowish pigment on its colony (Supplementary Figure 1).
Effect of σB on Other Virulence Regulators
The well-known virulence regulators in S. aureus are Agr, SarA, SaeRS, ArlRS, and σB (Jenul and Horswill, 2018). Transcriptome profiles in this study show a positive influence of σB on SarA, which is similar to other studies (Bischoff et al., 2004). Bischoff et al. (2001) found that σB has a negative influence on expression of RNAIII, which is an agr effector molecule (Bischoff et al., 2001). From our transcriptome analysis, no significant changes in agr operon expression in the wild type and ΔsigB mutant strains were observed. In RF122, σB negatively affected expression of SAB1004 (RNAIII), but the fold change is <2 (Supplementary Table 3). These findings suggest that the agr in RF122 is not directly regulated by σB like other strains (Bischoff et al., 2001; Figueiredo et al., 2017). However, similar to previous findings, our RNA-Seq and qRT-PCR results show that sarA are upregulated by σB and that σB has a negative effect on several genes encoding exotoxins and secreted enzymes. These genes include staphylococcal exotoxin genes (set2,3,4,5.7), which are positively regulated by the yabJ-spoVG operon and lipase (lip) (Supplementary Table 3) (Meier et al., 2007; Schulthess et al., 2011). In comparison to human clinical isolates (Bischoff et al., 2004), the influence of σB on virulence genes varies among S. aureus strains (Table 4). The differences in σB regulons might be due to the different nature of bacterial strains. Our invasion assay showed no effects of σB during invasion, which is similar to the result in the LS1 strain (Tuchscherr et al., 2015). In S. aureus LS1, σB is responsible for intracellular persistence, whereas sarA and agr are involved in invasion and infection of the strain (Tuchscherr et al., 2015).
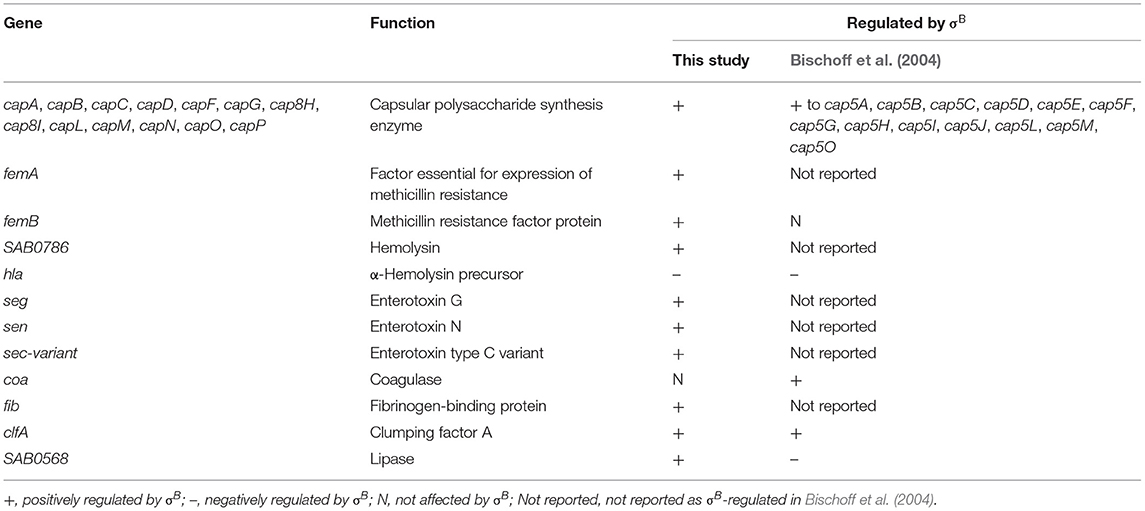
Table 4. List of virulence genes affected by σB in this study in comparison to Bischoff et al. (2004).
Reduction of Biofilm Production Related to Lack of σB
With regard to the ability of the pathogen to form biofilm, our finding with the bovine mastitis RF122 strain correlates with previous findings in MA12 and Newbould strains (Rachid et al., 2000; Lorenz et al., 2008; Mitchell et al., 2010). At 48 h under the experimental conditions (TSB containing 0.5% glucose), the ΔsigB mutant showed a significant decrease in biofilm formation when compared to the wild type, indicating that σB contributes to biofilm formation in RF122. Biofilm is composed of extracellular polymeric substances (EPS) while major compositions are polysaccharides, proteins, nucleic acid, and lipids (Flemming and Wingender, 2010). Several capsular polysaccharide synthesis genes (cap) have reduced expression levels in the ΔsigB mutant. A strong correlation between the capsule genotype/phenotype and the amount of formed biofilm were identified (Salimena et al., 2016). In S. aureus, biofilm formation is related to production of polysaccharide intercellular adhesion (PIA), which depends on the expression of the icaADBC operon (Valle et al., 2003). However, based on our RNA-Seq results, σB did not influence the expression of icaA. We propose that the role of σB on biofilm formation in mastitis-causing S. aureus is ica independent. Figueiredo et al. (2017) summarized ica-independent biofilm formation genes, and some of those previously reported genes were also identified to be σB dependent in this study, i.e., clfA, hla, and SAB1679c (serine protease precursor).
σB Plays a Minor Role in Resistance to Antiseptics and Antibiotics Used in Dairy Farms
In our study, the sigB mutant and its wild type were susceptible to β-lactams. However, the sigB mutant showed wider clear zones, in comparison to the wild type, when exposed to the drugs. The phenotypes suggest the role of σB in resistance to β-lactam antibiotics. In terms of gene expression, murG and murC, which are involved in biosynthesis of murein sacculus and peptidoglycan, were positively regulated by σB. This is in agreement with previous findings which reported that cell-wall-targeting antibiotics such as β-lactams and vancomycin as well as DNA-synthesis-targeting antibiotics such as sulfamethoxazole/trimethoprim can activate σB in vancomycin resistance strains (VRSA) (Chen et al., 2011).
Povidone-iodine and chlorhexidine are commonly used antiseptics in dairy cows. We therefore determined the susceptibility of wild-type and ΔsigB mutant strains to iodine and chlorhexidine. MICs of povidone-iodine in wild-type and ΔsigB mutant strains are equivalent (0.005%). Interestingly, the MIC is still lower than the recommended concentration (0.1%) used in dairy farms (Azizoglu et al., 2013). Similar to the results of povidone-iodine, the MIC of chlorhexidine antiseptic in ΔsigB mutant strain is also comparable (0.0002%) to that of the wild type. The MIC was identical to the previously reported value (0.0002%) by Azizoglu et al. (2013) when tested with seven other mastitis S. aureus strains. The results suggested that σB is not involved in the ability of S. aureus to tolerate commonly used antiseptics such as povidone-iodine and chlorhexidine.
Previous reports showed that the ΔsigB mutant strain of human isolates (ATCC12598, FDA486, SH1000, LAC, Newman, GP268, and BB255) had significant increases in α-hemolysin production in comparison to their wild-type strains (Giachino et al., 2001; Palma and Cheung, 2001; Lauderdale et al., 2009; Chen et al., 2011). σB negatively regulates hla (α-hemolysin) expression (Bischoff et al., 2004; Chen et al., 2011). However, bovine S. aureus isolates including RF122 have been reported to produce higher levels of α-toxin than human S. aureus isolates perhaps due to higher levels of agrA, arlR, saeR, and sarZ (positive regulators of hla) expression and lower levels of rot (negative regulator of hla) expression (Liang et al., 2011). To assess the role of σB in pathogenicity, hemolysis assays and biofilm formation assays were performed. Our study demonstrated that the RF122 ΔsigB mutant and wild-type strains could lyse the sheep red blood cells to a comparable extent, suggesting that σB may play a trivial role in α-hemolytic activity in S. aureus-causing mastitis RF122 strain.
σB Plays a Role in General Stress Responses
RNA-Seq and qRT-PCR results confirm that σB directly regulates the expression of alkaline shock protein (asp23) in response to general stress. On the contrary, Schulthess et al. (2011) showed that asp23 is downregulated by yabJ-spoVG and upregulated by σB. For oxidative stress response, σB positively controls sodA and katA expressions, and the phenotype of the ΔsigB mutant was significantly different from that of the wild type upon H2O2 exposure. In contrast, CHP (organic hydroperoxide) had no effect on the survival of the ΔsigB mutant strain compared to the wild-type strain. Perhaps, the regulators or genes which respond to CHP (organic hydroperoxide) stress are not regulated by σB. In Mycobacterium smegmatis, a regulator responding to organic hydroperoxide is OhrR, a MarR type of transcriptional regulator (Saikolappan et al., 2015). Although a regulator similar to OhrR was not found in this study, none of the MarR transcriptional regulator family is influenced by σB.
Newly Identified Genes Regulated by σB
Our transcriptomic result identified newly identified σB-dependent genes. Most of these genes are located in operons, which are the murQ-PTS system (SAB0131-0132), nrdG-nrdD (SAB2490c-2491c), and yabJ-spoVG (SAB0446-0447). Kozytska et al. (2010) identified a list of genes which are found only in S. aureus bovine strains. Among the bovine strain genes, two genes (SAB1880 and SAB1889) are shown to be regulated by σB in our study. The finding suggests that the role of σB is diverse among S. aureus strains.
Conclusion
This RNA-Seq study demonstrates that the alternative sigma factor B in bovine, the S. aureus mastitis RF122 strain, contributes to the expression of several virulence determinants and stress responses. RF122 σB is important for biofilm formation and H2O2 response while playing a minor role in α-hemolytic activity and tolerance against iodine, chlorhexidine, and alkyl hydroperoxide stresses. Even though σB does not contribute to invasion of this bacterial strain, other studies suggested that σB is important to intracellular survival. The biofilm formation and intracellular persistence are important factors for mastitis onset. For future studies, the roles of σB in biofilm formation and intracellular persistence are to be elucidated. Better understanding of S. aureus persistence mechanisms in bovine mastitis could validate σB as a potential drug target.
Data Availability Statement
The datasets generated for this study can be found in the NCBI bioproject accession number PRJNA548307 (https://www.ncbi.nlm.nih.gov/bioproject/PRJNA548307).
Author Contributions
SS collaborated in the experimental designs, performed parts of the experiments, analyzed the results, and prepared the manuscript. PM performed parts of the experiments, analyzed the result, and prepared the manuscript. PS assisted with mutant construction. PC and WN performed invasion assay. CJ supervised the invasion assay and prepared the manuscript. SC designed and supervised the experiments and the results analyses and prepared the manuscript.
Funding
This research project was supported by grants from the Agricultural Research Development Agency (CRP550010440) to SC and CJ and Mahidol University to SS.
Conflict of Interest
The authors declare that the research was conducted in the absence of any commercial or financial relationships that could be construed as a potential conflict of interest.
Acknowledgments
We thank Professor Vivek Kapur who gave RF122 wild type to SC and Professor Martin Wiedmann for initial supervision on the project at the Department of Food Science, Cornell University.
Supplementary Material
The Supplementary Material for this article can be found online at: https://www.frontiersin.org/articles/10.3389/fmicb.2019.02493/full#supplementary-material
References
Azizoglu, R. O., Lyman, R., and Anderson, K. L. (2013). Bovine Staphylococcus aureus: dose response to iodine and chlorhexidine and effect of iodine challenge on antibiotic susceptibility. J. Dairy Sci. 96, 993–999. doi: 10.3168/jds.2012-5857
Bischoff, M., Dunman, P., Kormanec, J., Macapagal, D., Murphy, E., Mounts, W., et al. (2004). Microarray-based analysis of the Staphylococcus aureus σB regulon. J. Bacteriol. 186, 4085–4099. doi: 10.1128/JB.186.13.4085-4099.2004
Bischoff, M., Entenza, J. M., and Giachino, P. (2001). Influence of a functional sigB operon on the global regulators sar and agr in Staphylococcus aureus. J. Bacteriol. 183, 5171–5179. doi: 10.1128/JB.183.17.5171-5179.2001
Brouillette, E., and Malouin, F. (2005). The pathogenesis and control of Staphylococcus aureus-induced mastitis: study models in the mouse. Microbes Infect. 7, 560–568. doi: 10.1016/j.micinf.2004.11.008
Cebrián, G., Sagarzazu, N., Aertsen, A., Pagán, R., Condón, S., and Mañas, P. (2009). Role of the alternative sigma factor σB on Staphylococcus aureus resistance to stresses of relevance to food preservation. J. Appl. Microbiol. 107, 187–196. doi: 10.1111/j.1365-2672.2009.04194.x
Chen, H.-Y., Chen, C.-C., Fang, C.-S., Hsieh, Y.-T., Lin, M.-H., and Shu, J.-C. (2011). Vancomycin activates σB in vancomycin-resistant Staphylococcus aureus resulting in the enhancement of cytotoxicity. PLOS ONE 6:e24472. doi: 10.1371/journal.pone.0024472
CLSI (2012). Performance Standards for Antimicrobial Susceptibility Testing; Twenty Second Informational Supplement. CLSI.
Dego, O. K., van Dijk, J. E., and Nederbragt, H. (2002). Factors involved in the early pathogenesis of bovine Staphylococcus aureus mastitis with emphasis on bacterial adhesion and invasion. A review. Vet. Q. 24, 181–198. doi: 10.1080/01652176.2002.9695135
Figueiredo, A. M. S., Ferreira, F. A., Beltrame, C. O., and Côrtes, M. F. (2017). The role of biofilms in persistent infections and factors involved in ica-independent biofilm development and gene regulation in Staphylococcus aureus. Crit. Rev. Microbiol. 43, 602–620. doi: 10.1080/1040841X.2017.1282941
Flemming, H.-C., and Wingender, J. (2010). The biofilm matrix. Nat. Rev. Microbiol. 8:623. doi: 10.1038/nrmicro2415
Giachino, P., Engelmann, S., and Bischoff, M. (2001). SigmaB activity depends on RsbU in Staphylococcus aureus. J. Bacteriol. 183, 1843–1852. doi: 10.1128/JB.183.6.1843-1852.2001
Gruet, P., Maincent, P., Berthelot, X., and Kaltsatos, V. (2001). Bovine mastitis and intramammary drug delivery: review and perspectives. Adv. Drug Deliv. Rev. 50, 245–259. doi: 10.1016/S0169-409X(01)00160-0
Hébert, A., Sayasith, K., Sénéchal, S., Dubreuil, P., and Lagacé, J. (2000). Demonstration of intracellular Staphylococcus aureus in bovine mastitis alveolar cells and macrophages isolated from naturally infected cow milk. FEMS Microbiol. Lett. 193, 57–62. doi: 10.1016/S0378-1097(00)00455-9
Hecker, M., Pané-Farré, J., and Uwe, V. (2007). SigB-dependent general stress response in Bacillus subtilis and related Gram-positive bacteria. Annu. Rev. Microbiol. 61, 215–236. doi: 10.1146/annurev.micro.61.080706.093445
Jenul, C., and Horswill, A. R. (2018). Regulation of Staphylococcus aureus virulence. Microbiol. Spectr. 6, 1–34. doi: 10.1128/microbiolspec.GPP3-0031-2018
Kim, D., Pertea, G., Trapnell, C., Pimentel, H., Kelley, R., and Salzberg, S. L. (2013). TopHat2: accurate alignment of transcriptomes in the presence of insertions, deletions and gene fusions. Genome Biol. 14:R36. doi: 10.1186/gb-2013-14-4-r36
Kim, J. H., Kim, C. H., Hacker, J., Ziebuhr, W., Lee, B. K., and Cho, S. H. (2008). Molecular characterization of regulatory genes associated with biofilm variation in a Staphylococcus aureus strain. J. Microbiol. Biotechnol. 18, 28–34.
Kozytska, S., Stauß, D., Pawlik, M.-C., Hensen, S., Eckart, M., Ziebuhr, W., et al. (2010). Identification of specific genes in Staphylococcus aureus strains associated with bovine mastitis. Vet. Microbiol. 145, 360–365. doi: 10.1016/j.vetmic.2010.03.020
Kullik, I., Giachino, P., and Fuchs, T. (1998). Deletion of the alternative sigma factor B Staphylococcus aureus reveals its function as a global regulator of virulence genes. J. Bacteriol. 180, 4814–4820.
Langmead, B., Wilks, C., Antonescu, V., and Charles, R. (2018). Scaling read aligners to hundreds of threads on general-purpose processors. Bioinformatics 35, 421–432. doi: 10.1093/bioinformatics/bty648
Lauderdale, K. J., Boles, B. R., Cheung, A. L., and Horswill, A. R. (2009). Interconnections between Sigma B, agr, and Proteolytic Activity in Staphylococcus aureus biofilm maturation. Infect. Immun. 77, 1623–1635. doi: 10.1128/IAI.01036-08
Le Maréchal, C., Seyffert, N., Jardin, J., Hernandez, D., Jan, G., Rault, L., et al. (2011a). Molecular basis of virulence in Staphylococcus aureus Mastitis. PLOS ONE 6:e27354. doi: 10.1371/journal.pone.0027354
Le Maréchal, C., Thiéry, R., Vautor, E., and Le Loir, Y. (2011b). Mastitis impact on technological properties of milk and quality of milk products—a review. Dairy Sci. Technol. 91, 247–282. doi: 10.1007/s13594-011-0009-6
Liang, X., Hall, J. W., Yang, J., Yan, M., Doll, K., Bey, R., et al. (2011). Identification of single nucleotide polymorphisms associated with hyperproduction of alpha-toxin in Staphylococcus aureus. PLOS ONE 6:e18428. doi: 10.1371/journal.pone.0018428
Liang, X., Yu, C., Sun, J., Liu, H., Landwehr, C., Holmes, D., et al. (2006). Inactivation of a two-component signal transduction system, saeRS, eliminates adherence and attenuates virulence of Staphylococcus aureus. Infect. Immun. 74, 4655–4665. doi: 10.1128/IAI.00322-06
Lorenz, U., Hüttinger, C., Schäfer, T., Ziebuhr, W., Thiede, A., Hacker, J., et al. (2008). The alternative sigma factor sigma B of Staphylococcus aureus modulates virulence in experimental central venous catheter-related infections. Microbes Infect. 10, 217–223. doi: 10.1016/j.micinf.2007.11.006
Love, M. I., Huber, W., and Anders, S. (2014). Moderated estimation of fold change and dispersion for RNA-Seq data with DeSeq2. Genome Biol. 15:550. doi: 10.1186/s13059-014-0550-8
Meier, S., Goerke, C., Wolz, C., Seidl, K., Homerova, D., Schulthess, B., et al. (2007). SigmaB and the sigmaB-dependent arlRS and yabJ-spoVG loci affect capsule formation in Staphylococcus aureus. Infect. Immun. 75, 4562–4571. doi: 10.1128/IAI.00392-07
Menzies, B. E., and Kernodle, D. S. (1994). Site-directed mutagenesis of the alpha-toxin gene of Staphylococcus aureus: role of histidines in toxin activity in vitro and in a murine model. Infect. Immun. 62, 1843–1847.
Mitchell, G., Brouillette, E., Séguin, D. L., Asselin, A.-E., Jacob, C. L., and Malouin, F. (2010). A role for sigma factor B in the emergence of Staphylococcus aureus small-colony variants and elevated biofilm production resulting from an exposure to aminoglycosides. Microb. Pathog. 48, 18–27. doi: 10.1016/j.micpath.2009.10.003
Monk, I. R., Shah, I. M., Xu, M., Tan, M.-W., and Foster, T. J. (2012). Transforming the untransformable: application of direct transformation to manipulate genetically Staphylococcus aureus and Staphylococcus epidermidis. mBio 3, e00277–e00211. doi: 10.1128/mBio.00277-11
Nicholas, R. O., Li, T., McDevitt, D., Marra, A., Sucoloski, S., Demarsh, P. L., et al. (1999). Isolation and characterization of a sigB deletion mutant of Staphylococcus aureus. Infect. Immun. 67, 3667–3669.
Palma, M., and Cheung, A. L. (2001). Sigma(B) activity in Staphylococcus aureus is controlled by RsbU and an additional factor(s) during bacterial growth. Infect. Immun. 69, 7858–7865. doi: 10.1128/IAI.69.12.7858-7865.2001
Pané-Farré, J., Jonas, B., Förstner, K., Engelmann, S., and Hecker, M. (2006). The σB regulon in Staphylococcus aureus and its regulation. Int. J. Med. Microbiol. 296, 237–258. doi: 10.1016/j.ijmm.2005.11.011
Pieterse, R., and Todorov, S. D. (2010). Bacteriocins - exploring alternatives to antibiotics in mastitis treatment. Braz. J. Microbiol. 41, 542–562. doi: 10.1590/S1517-83822010000300003
Pyl, P. T., Anders, S., and Huber, W. (2014). HTSeq—a Python framework to work with high-throughput sequencing data. Bioinformatics 31, 166–169. doi: 10.1093/bioinformatics/btu638
Rachid, S., Ohlsen, K., Wallner, U., Hacker, J., Hecker, M., and Ziebuhr, W. (2000). Alternative transcription factor sigma(B) is involved in regulation of biofilm expression in a Staphylococcus aureus mucosal isolate. J. Bacteriol. 182, 6824–6826. doi: 10.1128/JB.182.23.6824-6826.2000
Saikolappan, S., Das, K., and Dhandayuthapani, S. (2015). Inactivation of the organic hydroperoxide stress resistance regulator OhrR enhances resistance to oxidative stress and isoniazid in Mycobacterium smegmatis. J. Bacteriol. 197, 51–62. doi: 10.1128/JB.02252-14
Salimena, A. P. S., Lange, C. C., Camussone, C., Signorini, M., Calvinho, L. F., Brito, M. A., et al. (2016). Genotypic and phenotypic detection of capsular polysaccharide and biofilm formation in Staphylococcus aureus isolated from bovine milk collected from Brazilian dairy farms. Vet. Res. Commun. 40, 97–106. doi: 10.1007/s11259-016-9658-5
Schulthess, B., Bloes, D. A., François, P., Girard, M., Schrenzel, J., Bischoff, M., et al. (2011). The σB-dependent yabJ-spoVG operon is involved in the regulation of extracellular nuclease, lipase, and protease expression in Staphylococcus aureus. J. Bacteriol. 193, 4954–4962. doi: 10.1128/JB.05362-11
Schulthess, B., Meier, S., Homerova, D., Goerke, C., Wolz, C., Kormanec, J., et al. (2009). Functional characterization of the σB-dependent yabJ and spoVG operon in Staphylococcus aureus role in methicillin and glycopeptide resistance. Antimicrob. Agents Chemother. 53, 1832–1839. doi: 10.1128/AAC.01255-08
Senn, M. M., Giachino, P., Homerova, D., Steinhuber, A., Strassner, J., Kormanec, J., et al. (2005). Molecular analysis and organization of the σB operon in Staphylococcus aureus. J. Bacteriol. 187, 8006–8019. doi: 10.1128/JB.187.23.8006-8019.2005
Shittu, A. O., and Lin, J. (2006). Antimicrobial susceptibility patterns and characterization of clinical isolates of Staphylococcus aureus in KwaZulu-Natal province, South Africa. BMC Infect. Dis. 6:125. doi: 10.1186/1471-2334-6-125
Soares, T. C. S., Paes, A. C., Megid, J., Ribolla, P. E. M., Paduan, K. S., Gottschalk, M., et al. (2014). Antimicrobial susceptibility of Streptococcus suis isolated from clinically healthy swine in Brazil. Can. J. Vet. Res. 78, 145–149.
Therapeutic (2007). Cephalosporins (Veterinary-Systemic). Available online at: www.aavpt.org/associations/12658/files/cephalosporins.pdf (accessed June 20, 2019).
Tuchscherr, L., Bischoff, M., Lattar, S. M., Noto Llana, M., Pförtner, H., Niemann, S., et al. (2015). Sigma factor SigB is crucial to mediate Staphylococcus aureus adaptation during chronic infections. PLOS Pathog. 11:e1004870. doi: 10.1371/journal.ppat.1004870
Valle, J., Toledo-Arana, A., Berasain, C., Ghigo, J.-M., Amorena, B., Penadés, J. R., et al. (2003). SarA and not σB is essential for biofilm development by Staphylococcus aureus. Mol. Microbiol. 48, 1075–1087. doi: 10.1046/j.1365-2958.2003.03493.x
Viguier, C., Arora, S., Gilmartin, N., Welbeck, K., and O'Kennedy, R. (2009). Mastitis detection: current trends and future perspectives. Trends Biotechnol. 27, 486–493. doi: 10.1016/j.tibtech.2009.05.004
Wall, R. J., Powell, A. M., Paape, M. J., Kerr, D. E., Bannerman, D. D., Pursel, V. G., et al. (2005). Genetically enhanced cows resist intramammary Staphylococcus aureus infection. Nat. Biotechnol. 23, 445–451. doi: 10.1038/nbt1078
Wallemacq, H., Bedoret, D., Pujol, J., Desmet, C., Drion, P.-V., Farnir, F., et al. (2012). CD40 triggering induces strong cytotoxic T lymphocyte responses to heat-killed Staphylococcus aureus immunization in mice: a new vaccine strategy for staphylococcal mastitis. Vaccine 30, 2116–2124. doi: 10.1016/j.vaccine.2012.01.039
Wiedmann, M., Arvik, T. J., Hurley, R. J., and Boor, K. J. (1998). General stress transcription factor σB and its role in acid tolerance and virulence of Listeria monocytogenes. J. Bacteriol. 180, 3650–3656.
Keywords: Staphylococcus aureus, alternative sigma factor B, staphylococcal bovine mastitis, transcriptome, bacterial stress response
Citation: Supa-amornkul S, Mongkolsuk P, Summpunn P, Chaiyakunvat P, Navaratdusit W, Jiarpinitnun C and Chaturongakul S (2019) Alternative Sigma Factor B in Bovine Mastitis-Causing Staphylococcus aureus: Characterization of Its Role in Biofilm Formation, Resistance to Hydrogen Peroxide Stress, Regulon Members. Front. Microbiol. 10:2493. doi: 10.3389/fmicb.2019.02493
Received: 04 July 2019; Accepted: 16 October 2019;
Published: 07 November 2019.
Edited by:
Harold J. Schreier, University of Maryland, Baltimore County, United StatesReviewed by:
Fernanda Buzzola, CONICET Research Institute in Microbiology and Medical Parasitology (IMPaM), ArgentinaMarat R. Sadykov, University of Nebraska Medical Center, United States
Copyright © 2019 Supa-amornkul, Mongkolsuk, Summpunn, Chaiyakunvat, Navaratdusit, Jiarpinitnun and Chaturongakul. This is an open-access article distributed under the terms of the Creative Commons Attribution License (CC BY). The use, distribution or reproduction in other forums is permitted, provided the original author(s) and the copyright owner(s) are credited and that the original publication in this journal is cited, in accordance with accepted academic practice. No use, distribution or reproduction is permitted which does not comply with these terms.
*Correspondence: Soraya Chaturongakul, soraya.cha@mahidol.ac.th