- 1Univ. Lille, INRA, ISA-Yncréa, Univ. Artois, Univ. Littoral Côte d’Opale, EA 7394 – ICV – Institut Charles Viollette, Lille, France
- 2Civil and Geo-Environmental Engineering Laboratory (LGCgE), ISA – Yncréa, Lille, France
- 3MiPI, TERRA Teaching and Research Centre, Gembloux Agro-Bio Tech, University of Liège, Gembloux, Belgium
Within the framework of biocontrol development, three natural substances produced by Bacillus subtilis, called lipopeptides, have been studied: fengycin (F), surfactin (S), and mycosubtilin (M). Their antifungal properties were tested in vitro, in liquid medium, on two strains of Venturia inaequalis, ascomycete fungi causing apple scab. These two strains were, respectively sensitive and less sensitive to tebuconazole, an active substance of the triazole family. These three molecules were tested on their own, in binary (FS, FM, SM) and ternary mixtures (FSM). The antifungal activities of lipopeptides were estimated by calculating an IC50, compared to tebuconazole chemical substance. In tests involving the sensitive strain, all lipopeptide modalities exhibited antifungal activity. However, modalities involving fengycin and its mixtures exhibited the best antifungal activities; the activity of fengycin alone being very similar to that of tebuconazole. Interestingly, regarding the strain with reduced sensitivity to tebuconazole, surfactin and fengycin alone were not efficient while mycosubtilin and the different mixtures showed interesting antifungal activities. Specifically, the antifungal activity of FS and FSM mixture were equivalent to that of tebuconazole. For both fungal strains, microscopic observations revealed important morphological modifications in the presence of fengycin and in a less important proportion in the presence of surfactin but not in the presence of mycosubtilin. Overall, this study highlights the diversity in mode of action of lipopeptides on apple scab strains.
Introduction
Apple scab, also known as black spot, is the foremost disease affecting commercial apple orchards worldwide (Bowen et al., 2011). This disease is among the most economically important ones, being accountable for huge crop losses, with up to 70% reduction in apple production (MacHardy, 1996). The pathogen responsible for apple scab is a hemibiotrophic ascomycete fungus, called Venturia inaequalis Cooke (Winter) for the teleomorph form and Spilocaea pomi Fries (or Fusicladium pomi) for the anamorph. V. inaequalis attacks leaves, flowers and fruits, causing visible lesions, especially at the early stages of plant development, when they are the most sensitive (Bowen et al., 2011). Consequently, yield reductions result directly from unmarketable infected fruits, and indirectly from repeated defoliation. Although apple scab can be managed through integrated practices like genetic and prophylactic methods, the use of fungicides remains the main practice with up to 15–20 treatments per year (Parisi et al., 2004; Brun et al., 2008; Carisse and Jobin, 2012). It has been estimated that triazoles are the most used class of fungicides, accounting for 20% of fungicide use (Parker et al., 2014). However, the repeated use of single-site chemical fungicide, such as demethylation inhibitor fungicide (DMIs), has rapidly led over time to the development of V. inaequalis strains with reduced sensitivity to triazoles fungicides (Köller et al., 1995; Gao et al., 2009; Xu et al., 2010; Villani et al., 2015). Besides, many cases of resistant strains of V. inaequalis have been recorded worldwide: in north and south America (Hildebrand et al., 1988; Köller et al., 1991, 1995; Braun and McRae, 1992; Carisse and Pelletier, 1994; Mondino et al., 2015), in Europa (Kunz et al., 1997; Gao et al., 2009; Xu et al., 2010) and in Asia (Shirane et al., 1996; Vijaya Palani and Lalithakumari, 1999). In organic farming, apple scab can be managed using sulfur or copper. However, the heavy use of copper can lead to significant environmental problems (European Food Safety Authority [EFSA], 2013).
Therefore, there is an increasing need for new safe and environmental-friendly alternatives, such as biopesticides. These are defined as living organisms or products derived from them, which present an antagonistic activity against a targeted pest. Members of the Bacillus species, for instance, are known for producing a wide variety of antimicrobial compounds (Ongena and Jacques, 2008). In particular, the rhizobacterium Bacillus subtilis is one of the most commonly used and well-studied organism (Caulier et al., 2019). With an average of about 4–5% of its genome dedicated to secondary metabolites synthesis, it has the potential to produce more than two dozen structurally diverse antimicrobial compounds (Stein, 2005). Henceforth, some B. subtilis strains have already been registered and commercialized as biopesticides, such as SERENADE® (Bacillus subtilis str. QST 713) to control plant pathogens (Falardeau et al., 2013).
The lipopeptides produced by B. subtilis or Bacillus velezensis are considered as the main compounds involved in its biocontrol effect (Ongena and Jacques, 2008). B. subtilis lipopeptides are amphiphilic secondary metabolites produced by non-ribosomal peptide synthetases (NRPSs), composed of a cyclic peptide moiety (hydrophilic) linked to a fatty acid chain (hydrophobic) (Stein, 2005; Jacques, 2011; Cochrane and Vederas, 2014). According to their amino acid sequence, cyclic lipopeptides (CLPs) are classified in three distinct families, as shown in Figure 1: fengycin (fengycin and plipastatin), iturin (iturin, mycosubtilin, bacillomycin, and mojavensin), and surfactin (lichenysin and pumilacidin) (Ongena and Jacques, 2008; Jacques, 2011). Members of the fengycin family are decapeptides with a β-hydroxy fatty acid chain (C13–C19) (Hamley et al., 2013; Caulier et al., 2019). Interest in fengycin arises from its strong antifungal activity, specifically against filamentous fungi (Deleu et al., 2008). Members of the iturin family are heptapeptides with a β-amino fatty acid (C14–C18), displaying limited antiviral but strong antiyeast and antifungal activity (Maget-Dana and Peypoux, 1994). Surfactins are heptapeptides containing a β-hydroxy fatty acid tail (C12–C16) (Hamley et al., 2013; Caulier et al., 2019). Surfactin are mostly known for their powerful biosurfactant properties (Ongena and Jacques, 2008; Jacques, 2011), but are generally considered to have limited fungitoxicity (Pérez-Garcia et al., 2011). Synergistic activity of these lipopeptides have been already demonstrated for surfactin and iturin, surfactin and fengycin and iturin and fengycin (Ongena and Jacques, 2008).
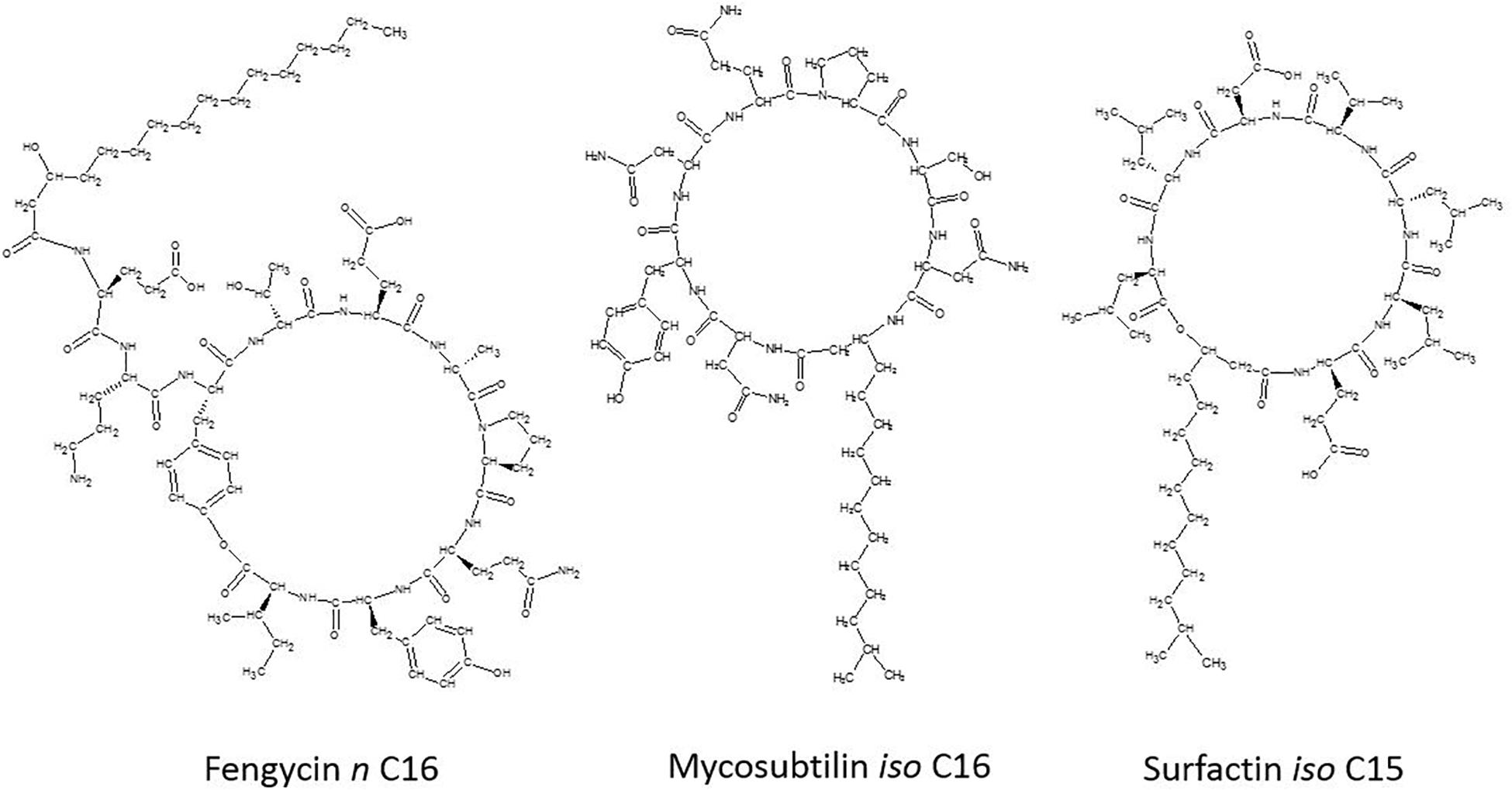
Figure 1. General representation of the chemical structure of lipopeptides from each family: fengycin n C16, mycosubtilin iso C16, and surfactin iso C15.
In vitro, the antagonism of a variety of B. subtilis strains, or their cell-free supernatant (followed by lipopeptide identification) as well as the direct activity of isolated lipopeptides have been assessed on a broad range of pathogenic fungi (Touré et al., 2004; Leclère et al., 2005; Romero et al., 2007a; Kim et al., 2010; Dunlap et al., 2011; Tao et al., 2011; Liu et al., 2014; Arroyave-Toro et al., 2017; Mejri et al., 2017; Mihalache et al., 2017). A novel approach is to consider these microbial compounds as future biocontrol agent. Purified molecules have been thus tested in vivo on major pathogens such as Botrytis cinerea (Farace et al., 2015), Zymoseptoria tritici (Mejri et al., 2017), and Fusarium oxysporum (Mihalache et al., 2017).
Therefore, as natural substances of microbial origin, lipopeptides represent a promising biocontrol agent, which has been increasingly studied in recent years. The aim of this study is to exhaustively assess, for the first time, the potential antifungal properties of fengycin, mycosubtilin, and surfactin CLPs of B. subtilis lipopeptides against the causal agent of apple scab.
Materials and Methods
Culture Conditions and Inoculum Preparation
The two V. inaequalis strains were provided by IRHS ECOFUN team, INRA Angers-Nantes centre (France). These two strains were distinguished by Muchembled et al. (2018) being respectively sensitive to tebuconazole (S755) and with reduced sensitivity to tebuconazole (rs552). Both strains were maintained on malt agar medium at 20°C, in the dark. Spores were collected from 20 days old cultures in glucose peptone (1.43% glucose and 0.71% bactopeptone). Spore suspensions were calibrated at 5 × 104 spores ml–1.
Lipopeptide Production
Lipopeptides used in this study were produced in shaked flasks using modified Landy media according to Coutte et al. (2010a) for surfactin and fengycin production and Béchet et al. (2013) for mycosubtilin production. They were purified from the fermentation broth using two steps ultrafiltration methods on 10 kDa membrane including four steps of diafiltration as previously described by Coutte et al. (2010b) and Jauregi et al. (2013). After freeze drying, the obtained powders of lipopeptides were then characterized using RP-HPLC and HPLC-MS as previously described (Mejri et al., 2017). In brief, surfactin are composed of isoforms with fatty acid chain of C12–C16, mycosubtilin with fatty acid chain of C15–C18 and fengycin with saturated and unsaturated fatty acid chain of C14–C18.
These three lipopeptides were tested alone (F, M, S), in binary (FS, FM, SM) and ternary mixtures (FSM) (Table 1). The antifungal activities of lipopeptides were compared to the active substance of reference: tebuconazole (Sigma-Aldrich, St. Louis, United States) which is a penetrating and systemic fungicide, from the triazoles family (DMIs).
In vitro Assay
Direct activity of lipopeptides on V. inaequalis was tested in liquid medium within microplates (Muchembled et al., 2018). Sterile flat-bottomed polystyrene 96-well plates were used (Corning® Costar® 3595). Lipopeptides or tebuconazole powders were solubilized in dimethyl sulfoxide (DMSO), and mixed in glucose peptone (1.43% glucose and 0.71% bactopeptone), in order to get a final 0.1% v/v of DMSO. Briefly, for each of the eight treatments (F, M, S, FM, FS, MS, FSM, T), a range of eight concentrations was made (Table 2), and 140 μl per well was distributed in microplates, one concentration per line. Thereafter, 60 μl of a calibrated spore suspension was added to eight wells per line (for a final volume of 200 μl of medium), which corresponds to eight replicates per concentration. The first four columns were left free from spore suspension (60 μl glucose peptone added instead) and used as a control to measure the net optical density (OD). The microplates were then sealed and left agitating (140 rpm) for 6 days at 20°C, in the dark.
Data Analysis
On the sixth day, the OD values were read at 635 nm with a microplate reader (Biotek EL 808; Sharma et al., 2004), in order to determine the IC50 (concentration of the substance to which 50% of the fungal development is inhibited), using a non-linear regression (logistic model). The experiment was repeated four times independently, to manage the intra- and inter-experiments variability. Differences between modalities (treatments × strains) were tested with an F-test comparing a non-linear regression model with distinction between modalities, to a model without this distinction (Ritz and Streibig, 2008). Different F-tests were performed. Some F-test compared lipopeptide treatments for each strain independently: firstly without tebuconazole to compare lipopeptides among each other, and secondly with tebuconazole, to compare lipopeptide activity to that of tebuconazole. Other F-tests compared results of both strains for each modality. Calculated from four independent repetition, each IC50 value is supported by its 95% confidence interval. Statistical analyses were performed using R-software (R Core Team, 2016. R: A language and environment for statistical computing. R Foundation for Statistical Computing, Vienna, Austria)1.
Optical Microscopy
Right after microplates reading, V. inaequalis was observed under an optical microscope in order to check the fungus morphology (Nikon Eclipse 80i, with Nikon Digital Camera Ddxm1200c). Three samples of each single compound (F, M, S, and tebuconazole) were taken from microwells at IC50 concentration and were compared to untreated control. First, a 5 μl drop of lactophenol blue solution was placed on glass slides. Then, 5 μl of sample (mycelium fragment in glucose peptone from microwells) were added before applying the coverslip.
Results
Effect of Lipopeptides on V. inaequalis Growth
The effect of the different samples of lipopeptides were first tested on the tebucanozole sensitive strain, S755 (Figure 2). The fengycin with an IC50 of 0.03 mg l–1 ([0.02–0.04]) was the lipopeptide with the most remarkable antifungal activity among the three lipopeptides tested alone. With respectively 2.84 mg l–1 ([2.23–3.56]) and 5.15 mg l–1 ([4.28–6.18]), mycosubtilin and surfactin were the lipopeptides with lower antifungal activities than fengycin. Among the mixtures, the combination of the three lipopeptides was more effective than fengycin + mycosubtilin, and fengycin + surfactin, while surfactin + mycosubtilin being the least effective. However, this surfactin + mycosubtilin binary mixture is significantly more effective than mycosubtilin or surfactin alone. It can be noted that the three lipopeptide mixture (FSM, 0.045 mg l–1 [0.04–0.05]) was almost as effective as fengycin alone (0.03 mg l–1 [0.02–0.04]).
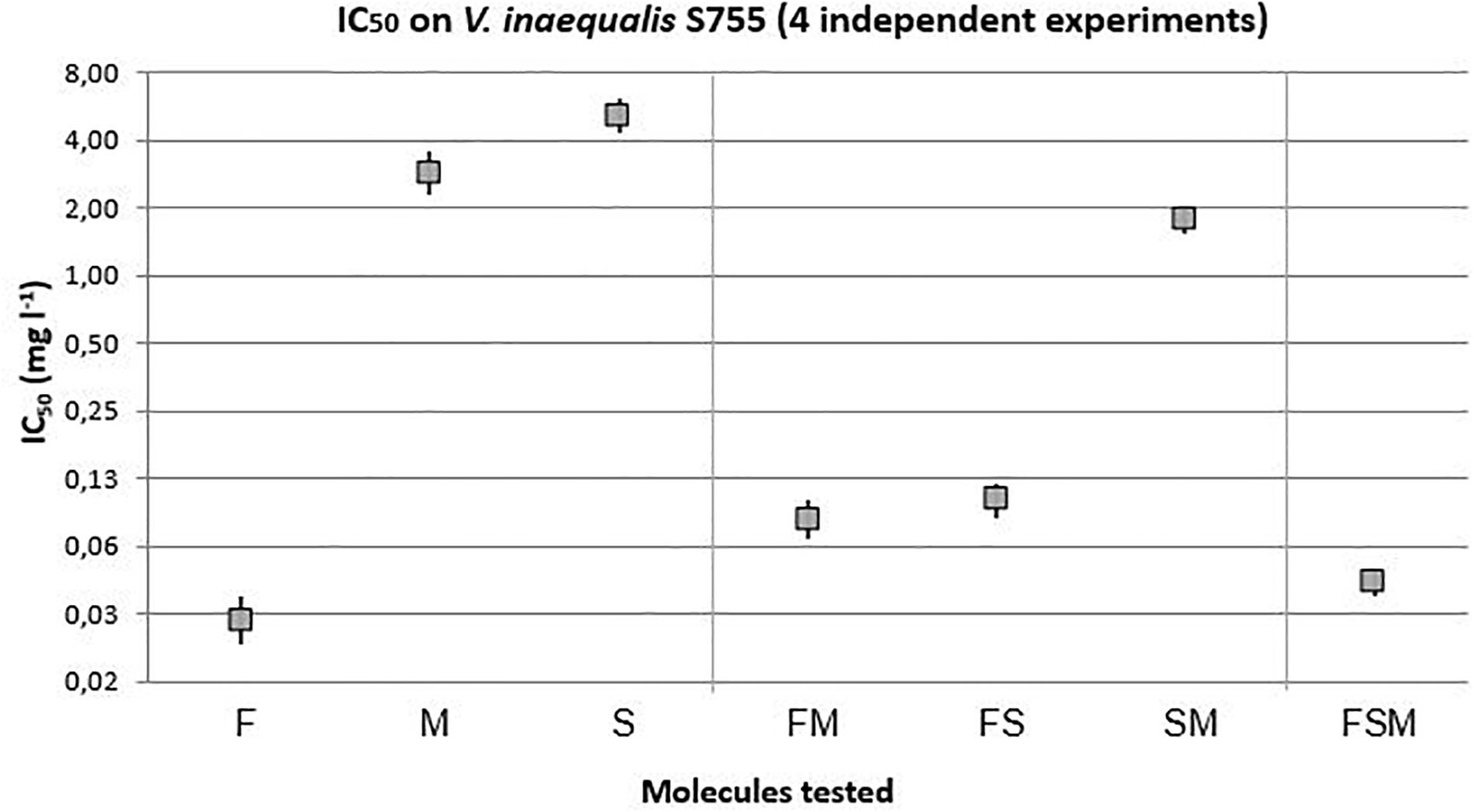
Figure 2. IC50 with 95% confidence interval of lipopeptides modalities on the sensitive strain of V. inaequalis to tebuconazole (F = 256.77, 6 and 1595 df, p-value < 0.0001).
On the tebuconazole reduced sensitivity strain, rs552 (Figure 3), mycosubtilin with an IC50 of 3.28 mg.l–1 [2.21–4.86] was the only lipopeptide with an important antifungal activity in comparison to fengycin and surfactin. In the light of the 95% confidence interval, similar levels of antifungal activity were obtained with M-containing binary mixtures: SM (IC50 = 2.37 mg l–1 [1.92–2.93]) and FM (IC50 = 3.75 mg l–1[2.79–5.04]). Even though the fengycin and surfactin alone did not show any antifungal activity, their combination (FS) have showed a higher antifungal activity than mycosubtilin with an IC50 of 1.79 mg l–1 [1.65–1.94]. A synergistic effect between fengycin and surfactin might therefore be occurring. Moreover, the ternary mixture FSM (1.81 mg l–1 [1.67–1.95]) showed an equivalent antifungal activity to FS.
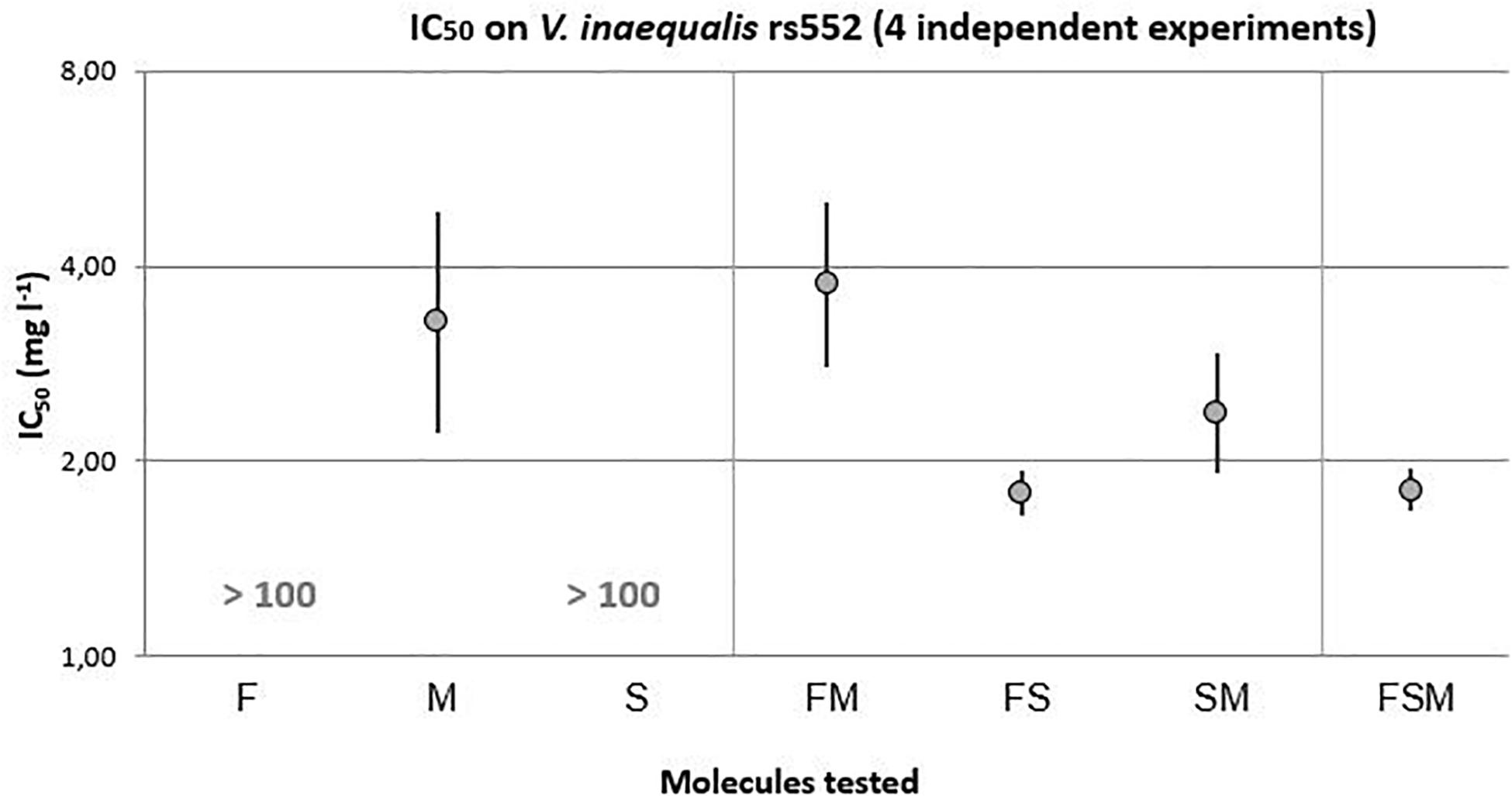
Figure 3. IC50 with 95% confidence interval of lipopeptides modalities on the V. inaequalis strain with reduced sensitivity to tebuconazole (F = 24.559, 4 and 1210 df, p-value < 0.0001).
F-tests were carried out for each modality allowing comparison between strains (Table 3). While fengycin and surfactin did not have any effect on the rs552 strain, mycosubtilin revealed a very close antifungal activity on both strains (2.32 mg l–1 [1.96–2.74] for S755 and, 3.34 mg l–1 [2.62–4.25] for rs552). Moreover, every lipopeptides mixtures (FM, FS, SM, and FSM) were always significantly more effective on the sensitive strain S755. The IC50 of tebuconazole was statistically lower for S755 (0.02 mg l–1 [0.02–0.03]), than for rs552 (1.65 mg l–1 [1.50–1.82]), being less sensitive.
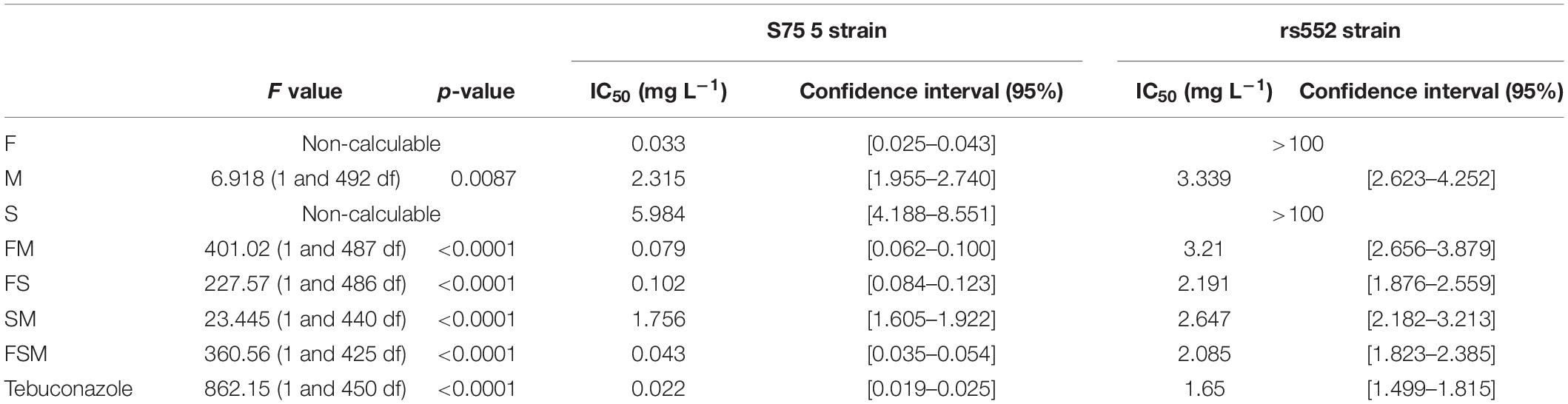
Table 3. Comparison of all modalities IC50 with 95% confidence interval between strains with different sensitivity to tebuconazole (four independent experiments).
Moreover, two other F-tests were run for each strain to compare all modalities with tebuconazole as a reference (data shown in Supplementary Material). On the sensitive strain (F = 305.67, 7, and 1804 df, p-value < 0.0001), it can be outlined that fengycin (0.03 mg l–1 [0.02–0.04]), exhibited a strong antifungal activity at the same level as tebuconazole (0.02 mg l–1 [0.02–0.03]). Likewise, on the strain with reduced sensitivity (F = 21.97, 5 and 1452 df, p-value < 0.0001), the most important antifungal activities were FS (1.8 mg l–1 [1.67–1.94]) and FSM (1.82 mg l–1 [1.7–1.95]) that showed an IC50 as low as tebuconazole (1.86 mg l–1 [1.68–2.05])
Effect of Lipopeptides on V. inaequalis Morphology
The untreated mycelium of V. inaequalis has first been observed under photonic microscopy, in order to confirm the regular morphology in the absence of CLPs (Figure 4). For both strains, mycelium appeared to be well developed, with long hyphae in the control (Figures 4A,B). V. inaequalis has also been observed under tebuconazole treatment. On the sensitive strain, oval-shaped swollen structures, of small sizes, were observed along the mycelium, with a concentration-dependent effect (Figure 4C). However, on the strain with reduced sensitivity, no such morphological changes were noticed. The mycelium looked well developed, although looking slightly more branched and thicken on hyphae’s tips (Figure 4D). In the presence of fengycin, original swollen structures were observed, mainly at the tip of hyphae (Figures 4E,F). Interestingly, these vesicle-like structures seemed of varied type: from slightly swollen cells to large clear bag-like structures, which even looked torn at times. These modifications were observed on both strains, but were less ubiquitous on rs552. These structures were different from the kind observed under tebuconazole treatment. Although the presence of vesicle-like structures is systematic in the presence of fengycin, these same structures can be observed in a less systematic way in the presence of surfactin. On the contrary, in the case of mycosubtilin, no vesicle-like structures were observed on both strains. Therefore, the mycelium in the presence of mycosubtilin had a morphology identical to that of the control.
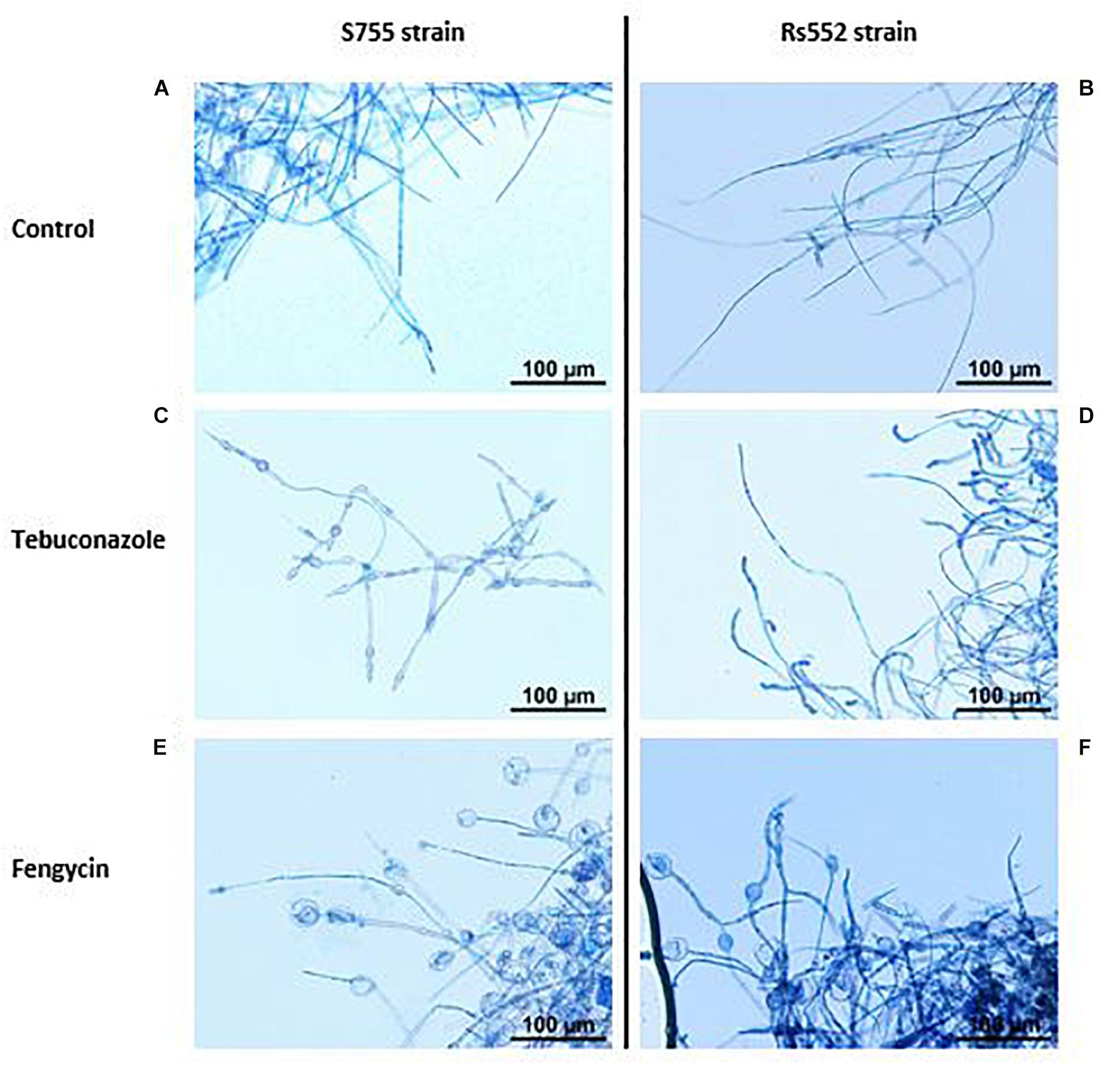
Figure 4. Effect of tebuconazole and fengycin on the morphology of V. inaequalis mycelium. Optical microscopy photos were taken on the sixth day after microplate’s inoculation with calibrated spores’ suspension, with lactophenol blue staining. (A) S755 control; (B) rs552 control; (C) S755 treated with tebuconazole (0.65 mg l–1); (D) rs552 treated with tebuconazole (0.65 mg l–1); (E) S755 treated with fengycin (0.39 mg l–1); (F) rs552 treated with fengycin (0.39 mg l–1).
Discussion
Lipopeptide Have a Good Efficacy Against V. inaequalis
Our results revealed, for the first time, the existence of in vitro antifungal properties of CLPs from B. subtilis on V. inaequalis, and in some cases, the concentration range was comparable with the chemical reference tebuconazole.
Even though surfactin is generally considered as having little or no antifungal properties on its own (Ongena and Jacques, 2008; Gonzalez-Jaramillo et al., 2017), we highlighted a clear antifungal activity against S755 strain like other studies (Inès and Dhouha, 2015; Krishnan et al., 2019). Mycosubtilin inhibited both strains, with a statistically similar antifungal activity (IC50 = 2.32 mg l–1 [1.96–2.74] on S755 and 3.34 mg l–1 [2.62–4.25 mg l–1] on rs552). Mycosubtilin has already been showed to inhibit several phytopathogenic fungi (Leclère et al., 2005; Mejri et al., 2017). Comparing our IC50 results to other studies is not straightforward, since most of them evaluated the CLPs antifungal efficacy by using inhibition or growth rate/percentage (Touré et al., 2004; Leclère et al., 2005; Romero et al., 2007b; Dunlap et al., 2011; Tao et al., 2011; Liu et al., 2014; Arroyave-Toro et al., 2017; Mihalache et al., 2017). However, in a recent work of Mejri et al. (2017), the IC50 of mycosubtilin, in liquid medium, was evaluated at 1.4 mg l–1 on Z. tritici, which is close to our results. In this study on Z. tritici, IC50 were above 100 mg l–1 for fengycin and surfactin treatments (Mejri et al., 2017), like our results on rs552. Interestingly, this study was conducted on the T01193 strain, which also has reduced sensitivity to several DMIs (S. Mejri, personal communication).
Furthermore, little is known about lipopeptides’ activity while in mixtures, although they could act in a synergistic manner (Ongena and Jacques, 2008). In our experiments, better efficacies were obtained with SM mixtures on S755 than lipopeptides on their own. On rs552, the M-containing binary mixtures (FM and SM) were relatively as effective as mycosubtilin alone. Also, a very strong synergistic effect was observed with the mixture FS transforming two non-inhibitory molecules in an efficient complex. Synergistic activities have already been showed on phytopathogenic fungi, with the mixture surfactins + mycosubtilins or surfactins + iturins (Maget-Dana et al., 1992; Romero et al., 2007b; Deravel et al., 2014; Mejri et al., 2017; Mihalache et al., 2017). It is also known that anionic and non-ionic biosurfactants as for example surfactins and iturins can form mixed micelles, acting more efficiently than simple micelles on biological membranes (Maget-Dana et al., 1992; Muñoz et al., 2004; Jauregi et al., 2013). However, less information are available about the potential synergistic effect of fengycin and surfactin which are both anionic biosurfactants.
Lipopeptides Differently Induce Fungal Morphological Modifications
Through our microscopic observations, we noticed consistent morphological modifications on V. inaequalis under fengycin treatments. Many vesicle-like swollen structures were observed on both strains. Morphological damages by B. subtilis lipopeptides have already been noticed on other fungi. However, this is the first time such swollen structures on mycelium are well characterized in the presence of isolated fengycin. Under scanning electron microscopy (SEM), Romero et al. (2007a) observed a loss of turgidity of Podosphaera fusca conidia following treatment with a cell-free supernatant of B. subtilis. Increase vacuolization and disorganization of the cytoplasm, resulting from membrane disruption by small vesicle-like structures, was visualized with transmission electron microscopy (TEM) (Romero et al., 2007a). Mihalache et al. (2017) also spotted deeply folded, shrunken, wrinkled and partially distorted hyphae, as well as subterminal vesicles and intercalary swelling on F. oxysporum mycelium, under M or SM treatments, using SEM. More recent data, from Park et al. (2019), have shown vesicle-like structures with surfactin (from B. velenzenis) on Colletotrichum gloeosporioides. The surface of the mycelium was rugged and unusually swollen and rough, suggesting the peptide affects directly the fungal cell wall components (chitin, glucans, and glycoproteins) to exhibit antifungal activity (Park et al., 2019).
Clear disruptions were observed after linking antifungal activity data with morphological microscopic observations. Indeed, on S755, for the same level of antifungal activity, we did not observe the same type of morphological modifications according to treatments: large vesicle-like structures could be seen in the presence of fengycin, while small oval-shaped swollen structures, of small sizes, could be observed with tebuconazole. Moreover, in the case of fengycin, the same kind of vesicle-like structures were observed on both strains even though fengycin had a strong activity on S755 but did not show any activity on rs552. The same goes for surfactin-treated fungi, where similar vesicle-like structures were seen on both strains, irrespective of the activity. A work by Vanittanakom et al. (1986) also relates for the first time morphological changes in different fungi (bulging, curling, emptying) but this does not seem directly related to antifungal activity. This is in agreement with our results, for which the presence of original vesicle-like structures does not seem correlated with antifungal activity. Regarding mycosubtilin, we never observed vesicles (neither on S755, nor on rs552), even if there was always antifungal activity for both strains.
Lipopeptides Differently Inhibit Tebuconazole Sensitive and Less Sensitive V. inaequalis Strains
Another originality of our approach is to compare, for the first time, CLPs activity on fungal strains possessing different sensitivity to fungicides. Our tests clearly confirmed the difference in tebuconazole sensitivity between both 755 and 552 (Muchembled et al., 2018). Tebuconazole is an inhibitor of sterol biosynthesis (DMIs), which specifically targets the 14α-demethylase (or CYP51), an important regulatory enzyme in the ergosterol biosynthetic pathway (Yoshida and Aoyama, 1987). Inhibition of this enzyme disrupts ergosterol synthesis, leading to the reduction of ergosterol content and thus the formation of cell membranes with altered structure and functions, such as fluidity and permeability (Leroux et al., 2008; Price et al., 2015). Three major mechanisms may be implicated in DMI-resistance (Cools et al., 2013; Price et al., 2015). The first is the alteration of the target enzyme, which results in reduced affinity to fungicides (Leroux et al., 2008; Cools et al., 2013; Parker et al., 2014). The second mechanism involves the overexpression of CYP51 (Schnabel and Jones, 2001; Pfeufer and Ngugi, 2012; Villani et al., 2016) while the third mechanism is an efflux phenomenon, enabling a decrease of the intracellular drug accumulation, attributed to overexpression of membrane transporter proteins involved in multidrug resistance (MDR) (Leroux et al., 2008; Cools et al., 2013). Two efflux systems are involved in MDR: the ATP-Binding Cassette (ABC), which utilizes energy from ATP hydrolysis, and the Major Facilitators Superfamily (MFS) transporters, which employ the proton motive force (Deising et al., 2008).
Moreover, Vijaya Palani and Lalithakumari (1999) found that some DMIs-resistant V. inaequalis strains were characterized by significantly reduced total lipid and ergosterol biosynthesis. They postulated that the reduction in ergosterol content could lower membrane permeability, thus hampering the transport of toxicant in the resistant strains. Another study stated that low ergosterol content is linked with increased membrane interaction and destabilization (Falardeau et al., 2013). High fungal ergosterol could buffer fluidity changes, increasing CLPs tolerance (Falardeau et al., 2013).
These modifications of membrane properties and ergosterol concentrations could be correlated with the biological activities of the CLPs on both V. inaequalis strains. Previous studies acknowledged that CLPs can interact with biological membranes and especially ergosterol (Pérez-Garcia et al., 2011). However, the mode of action can differ according to the family of CLPs considering that fengycin and surfactin are negatively charged molecules while mycosubtilin is a neutral compound (Falardeau et al., 2013). Polarity appears to be key in these membrane-CLPs interactions depending on the charge of both lipopeptides and membranes (Deleu et al., 2003; Buchoux et al., 2008; Razafindralambo et al., 2009; Juhaniewicz-Dębińska et al., 2019). While iturin is known to possess an ion-conducting pore-forming activity (Maget-Dana and Peypoux, 1994), fengycin and surfactin have concentration-dependent mode of action. At low concentration, fengycin and surfactin induce limited perturbation, such as pore formation (Deleu et al., 2005; Heerklotz and Seelig, 2007; Pérez-Garcia et al., 2011; Cochrane and Vederas, 2014). At higher concentrations, they operate by a detergent mechanism, with complete disruption and solubilization of the lipid bilayer (Deleu et al., 2003, 2005; Ongena and Jacques, 2008; Cochrane and Vederas, 2014). In a study, Patel et al. (2011) suggested the all-or-none leakage/membrane permeabilization effect of fengycin, highlighting crucial interaction with membrane and lipids.
Fluid state of membrane lipids may also be key for effective CLPs insertion (Deleu et al., 2008; Mantil et al., 2019). In agreement, more recent studies, on model membrane, showed that bilayers containing higher levels of ergosterol exhibited an increased tolerance to activity of fengycin at low doses (Mantil et al., 2019). All these information could explain the differences measured in lipopeptide antifungal activity between tebuconazole sensitive and reduced sensitivity strains of V. inaequalis.
Conclusion
Finally, this study revealed a diversity of responses of two strains of V. inaequalis exposed to different families of lipopeptides, highlighting the distinct modes of action of each lipopeptide. On both strains, the lipopeptides with the most remarkable antifungal activities (F for S755; FS and FSM for rs552) were very close to the activity of tebuconazole. These antifungal activities obtained in vitro present promising results for CLP development as biopesticides. Moreover, according to several ecotoxicity evaluations, CLPs are amongst the least toxic substances and could thus constitute eco-friendly alternatives to chemical pesticides. In the future, we suggest further investigation under in vivo and in situ experiments, to validate lipopeptides’ efficacy as biocontrol agents in apple orchards.
Data Availability Statement
The raw data supporting the conclusions of this manuscript will be made available by the authors, without undue reservation, to any qualified researcher.
Author Contributions
All authors have made direct experimental and/or intellectual contribution to the work, and have read and approved the final version. HD, CD, and JJ have conducted all the in vitro experiment with V. inaequalis. FC has produced and purified the different lipopeptides from Bacillus subtilis. HD, CD, JM, KS, FC, and PJ were principally responsible for analyzing the results and writing the manuscript. CD, JM, FC, and PJ have defined the general design of the experiments. FC and PJ were respectively the scientific coordinator of the AgriBioPOM and ALIBIOTECH funding projects.
Funding
This work was supported by the funding from the AgriBioPOM research program and from the ALIBIOTECH program funding administered by the Hauts-de-France Region. Avec le soutien du fonds européen de développement régional.
Conflict of Interest
The authors declare that the research was conducted in the absence of any commercial or financial relationships that could be construed as a potential conflict of interest.
Acknowledgments
The authors want to thank Jonathan Paucellier for his technical assistance during the AgriBioPOM project.
Supplementary Material
The Supplementary Material for this article can be found online at: https://www.frontiersin.org/articles/10.3389/fmicb.2019.02327/full#supplementary-material
Footnotes
References
Arroyave-Toro, J. J., Mosquera, S., and Villegas-Escobar, V. (2017). Biocontrol activity of Bacillus subtilis EA-CB0015 cells and lipopeptides against postharvest fungal pathogens. Biol. Control 114, 195–200. doi: 10.1016/j.biocontrol.2017.08.014
Béchet, M., Castéra-Guy, J., Guez, J.-S., Chihib, N.-E., Coucheney, F., Coutte, F., et al. (2013). Production of a novel mixture of mycosubtilins by mutants of Bacillus subtilis. Bioresour. Technol. 145, 264–270. doi: 10.1016/j.biortech.2013.03.123
Bowen, J. K., Mesarich, C. H., Bus, V. G. M., Beresford, R. M., Plummer, K. M., and Templeton, M. D. (2011). V. inaequalis: the causal agent of apple scab. Mol. Plant. Pathol. 12, 105–122. doi: 10.1111/j.1364-3703.2010.00656.x
Brun, L., Didelot, F., and Parisi, L. (2008). Effects of apple cultivar susceptibility to V. inaequalis on scab epidemics in apple orchards. Crop Prot. 27, 1009–1019. doi: 10.1016/j.cropro.2007.12.009
Braun, P. G., and McRae, K. B. (1992). Composition of a population of V. inaequalis resistant to myclobutanil. Can. J. Plant Pathol. 14, 215–220. doi: 10.1080/07060669209500878
Buchoux, S., Lai-Kee-Him, J., Garnier, M., Tsan, P., Besson, F., Brisson, A., et al. (2008). Surfactin-triggered small vesicle formation of negatively charged membranes: a novel membrane-lysis mechanism. Biophys. J. 95, 3840–3849. doi: 10.1529/biophysj.107.128322
Carisse, O., and Jobin, T. (2012). Managing summer apple scab epidemics using leaf scab incidence threshold values for fungicide sprays. Crop Prot. 35, 36–40. doi: 10.1016/j.cropro.2011.12.014
Carisse, O., and Pelletier, J. R. (1994). Sensitivity distribution of V. inaequalis to fenarimol in Québec apple orchards. Phytoprotection 75, 35–43.
Caulier, S., Nannan, C., Gillis, A., Licciardi, F., Bragard, C., and Mahillon, J. (2019). Overview of the antimicrobial compounds produced by members of the Bacillus subtilis group. Front. Microbiol. 10:302. doi: 10.3389/fmicb.2019.00302
Cochrane, S. A., and Vederas, J. C. (2014). Lipopeptides from Bacillus and Paenibacillus spp.: a gold mine of antibiotic candidates. Med. Res. Rev. 36, 4–31. doi: 10.1002/med.21321
Cools, H. J., Hawkins, N. J., and Fraaije, B. A. (2013). Constraints on the evolution of azole resistance in plant pathogenic fungi. Plant Pathol. 62, 36–42. doi: 10.1111/ppa.12128
Coutte, F., Leclère, V., Béchet, M., Guez, J.-S., Lecouturier, D., Chollet-Imbert, M., et al. (2010a). Effect of pps disruption and constitutive expression of srfA on surfactin productivity, spreading and antagonistic properties of Bacillus subtilis 168 derivatives. J. Appl. Microbiol. 109, 480–491. doi: 10.1111/j.1365-2672.2010.04683.x
Coutte, F., Lecouturier, D., Ait Yahia, S., Leclère, V., Béchet, M., and Jacques, P. (2010b). Production of surfactin and fengycin by Bacillus subtilis in a bubbleless membrane bioreactor. Appl. Microbiol. Biot. 87, 499–507. doi: 10.1007/s00253-010-2504-8
Deising, H. B., Reimann, S., and Pascholati, S. F. (2008). Mechanisms and significance of fungicide resistance. Braz. J. Microbiol. 39, 286–295. doi: 10.1590/S1517-838220080002000017
Deleu, M., Bouffioux, O., Razafindralambo, H., Paquot, M., Hbid, C., Thonart, P., et al. (2003). Interaction of surfactin with membranes: a computational approach. Langmuir 19, 3377–3385. doi: 10.1021/la026543z
Deleu, M., Paquot, M., and Nylander, T. (2005). Fengycin Interaction with lipid monolayers at the air–aqueous interface - implications for the effect of fengycin on biological membranes. J. Colloid Interface Sci. 283, 358–365. doi: 10.1016/j.jcis.2004.09.036
Deleu, M., Paquot, M., and Nylander, T. (2008). Effect of fengycin, a lipopeptide produced by bacillus subtilis, on model biomembrance. Biophys. J. 94, 2667–2679. doi: 10.1529/biophysj.107.114090
Deravel, J., Lemière, S., Coutte, F., Krier, F., Van Hese, N., Béchet, M., et al. (2014). Mycosubtilin and surfactin are efficient, low ecotoxicity molecules for the biocontrol of lettuce downy mildew. Appl. Microbiol. Biot. 98, 6255–6264. doi: 10.1007/s00253-014-5663-1
Dunlap, C. A., Schisler, D. A., Price, N. P., and Vaughn, S. F. (2011). Cyclic lipopeptide profile of three Bacillus subtilis strains; antagonists of fusarium head blight. J. Microbiol. 49, 603–609. doi: 10.1007/s12275-011-1044-y
European Food Safety Authority [EFSA] (2013). Conclusion of the peer review of the pesticide risk assessment of confirmatory data submitted for the active substance copper (I), copper (II) variants namely copper hydroxide, copper oxychloride, tribasic copper sulphate, copper (I) oxide. Bordeaux mixture. EFSA J. 11, 3235–3275.
Falardeau, J., Wise, C., Novitsky, L., and Avis, T. J. (2013). Ecological and mechanistic insights into the direct and indirect antimicrobial properties of Bacillus subtilis lipopeptides on plant pathogens. J. Chem. Ecol. 39, 869–878. doi: 10.1007/s10886-013-0319-7
Farace, G., Fernandez, O., Jacquens, L., Coutte, F., Krier, F., Jacques, P., et al. (2015). Cyclic lipopeptides from Bacillus subtilis activate distinct patterns of defence responses in grapevine. Mol. Plant Pathol. 16, 177–187. doi: 10.1111/mpp.12170
Gao, L., Berrie, A., Yang, J., and Xu, X. (2009). Within- and between-orchard variability in the sensitivity of V. inaequalis to myclobutanil, a DMI fungicide, in the UK. Pest Manag. Sci. 65, 1241–1249. doi: 10.1002/ps.1816
Gonzalez-Jaramillo, L. M., Aranda, F. J., Teruel, J. A., Villegas-Escobar, V., and Ortiz, A. (2017). Antimycotic activity of fengycin C biosurfactant and its interaction with phosphatidylcholine model membranes. Colloid Surf. B 156, 114–22. doi: 10.1016/j.colsurfb.2017.05.021
Hamley, I. W., Dehsorkhi, A., Jauregi, P., Seitsonen, J., Ruokolainen, J., Coutte, F., et al. (2013). Self-assembly of three bacterially-derived bioactive lipopeptides. Soft Matter 9, 9572–9578.
Heerklotz, H., and Seelig, J. (2007). Leakage and lysis of lipid membranes induced by the lipopeptide surfactin. Eur. Biophys. J. 36, 305–314. doi: 10.1007/s00249-006-0091-5
Hildebrand, P. D., Lockhart, C. L., Newbery, R. J., and Ross, R. G. (1988). Resistance of V. inaequalis to bitertanol and other demethylation-inhibiting fungicides. Can. J. Plant Pathol. 10, 311–316. doi: 10.1080/07060668809501704
Inès, M., and Dhouha, G. (2015). Lipopeptide surfactants: production, recovery and pore forming capacity. Peptides 71, 100–112. doi: 10.1016/j.peptides.2015.07.006
Jacques, P. (2011). “Surfactin and other lipopeptides from Bacillus spp.,” in Microbiology Monographs, vol 20, eds Edn, ed. G. Soberón-Chávez (Berlin: Springer).
Jauregi, P., Coutte, F., Catiau, L., Lecouturier, D., and Jacques, P. (2013). Micelle size characterization of lipopeptides produced by B. subtilis and their recovery by the two-step ultrafiltration process. Sep. Purif. Technol. 104, 175–182. doi: 10.1016/j.seppur.2012.11.017
Juhaniewicz-Dębińska, J., Tymecka, D., and Sęk, S. (2019). Diverse effect of cationic lipopeptide on negatively charged and neutral lipid bilayers supported on gold electrodes. Electrochim. Acta 298, 735–44.
Kim, P. I., Ryu, J., Kim, Y. H., and Chi, Y. T. (2010). Production of biosurfactant lipopeptides Iturin A, fengycin and surfactin A from Bacillus subtilis CMB32 for control of Colletotrichum gloeosporioides. J. Microbiol. Biotechnol. 20, 138–145.
Köller, W., Parker, D. M., and Reynolds, K. L. (1991). Baseline sensitivities of V. inaequalis to sterol demethylation inhibitors. Plant Dis. 75, 726–728. doi: 10.1094/PHYTO.1997.87.12.1272
Köller, W., Smith, F. D., Reynolds, J. K. L., Wilcox, W. F., and Burri, J. A. (1995). Seasonal changes of sensitivities to sterol demethylation inhibitors in V. inaequalis populations. Mycol. Res. 99, 689–692. doi: 10.1016/s0953-7562(09)80529-7
Krishnan, N., Velramar, B., and Velu, R. K. (2019). Investigation of antifungal activity of surfactin against mycotoxigenic phytopathogenic fungus fusarium moniliforme and its impact in seed germination and mycotoxicosis. Pestic. Biochem. Phys. 155, 101–7. doi: 10.1016/j.pestbp.2019.01.010
Kunz, S., Deising, H., and Mendgen, K. (1997). Acquisition of resistance to sterol demethylation inhibitors by populations of V. inaequalis. Phytopathology 87, 1272–1278. doi: 10.1094/PHYTO.1997.87.12.1272
Leclère, V., Bechet, M., Adam, A., Guez, J.-S., Wathelet, B., Ongena, M., et al. (2005). Mycosubtilin overproduction by Bacillus subtilis BBG100 enhances the organism’s antagonistic and biocontrol activities. Appl. Environ. Microb. 71, 4577–4584. doi: 10.1128/aem.71.8.4577-4584.2005
Leroux, P., Bach, J., Debieu, D., Fillinger, S., Fritz, R., et al. (2008). “Mode of action of biosynthesis inhibitors and resistance phenomena in fungi,” in Modern Fungicides and Antifungal Compounds V, eds H. W. Dehne, H. B. Deising, U. Gisi, K. H. Kuck, P. E. Russell, and H. Lyr (Germany: Deutsche Phytomedizinische Gesellschaft e.V. Verlag), 45–51.
Liu, J., Hagberg, I., Novitsky, L., Hadj-Moussa, H., and Avis, T. J. (2014). Interaction of antimicrobial cyclic lipopeptides from Bacillus subtilis influences their effect on spore germination and membrane permeability in fungal plant pathogens. Fungal Biol. 118, 855–861. doi: 10.1016/j.funbio.2014.07.004
MacHardy, W. E. (1996). Apple Scab, Biology, Epidemiology, and Management. St. Paul, Minn, USA: APS.
Maget-Dana, R., Thimon, L., Peypoux, F., and Ptak, M. (1992). Surfactin/iturin A interactions may explain the synergistic effect of surfactin on the biological properties of iturin A. Biochimie 74, 1047–1051. doi: 10.1016/0300-9084(92)90002-v
Maget-Dana, R., and Peypoux, F. (1994). Iturins, a special class of pore-forming lipopeptides: biological and physicochemical properties. Toxicology 87, 151–174. doi: 10.1016/0300-483x(94)90159-7
Mantil, E., Crippin, T., and Avis, T. J. (2019). Domain redistribution within ergosterol-containing model membranes in the presence of the antimicrobial compound fengycin. Biochim. Biophys. Acta 1861, 738–47. doi: 10.1016/j.bbamem.2019.01.003
Mejri, S., Siah, A., Coutte, F., Magnin-Robert, M., Randoux, B., Tisserant, B., et al. (2017). Biocontrol of the wheat pathogen zymoseptoria tritici using cyclic lipopeptides from Bacillus subtilis. Environ. Sci. Pollut. Res. 25, 29822–29833. doi: 10.1007/s11356-017-9241-9
Mihalache, G., Balaes, T., Gostin, I., Stefan, M., Coutte, F., and Krier, F. (2017). Lipopeptides produced by Bacillus subtilis as new biocontrol products against fusariosis in ornamental plants. Environ. Sci. Pollut. Res. 25, 29784–29793. doi: 10.1007/s11356-017-9162-7
Mondino, P., Casanova, L., Celio, A., Bentancur, O., Leoni, C., and Alaniz, S. (2015). Sensitivity of V. Inaequalis to trifloxystrobin and difenoconazole in uruguay. J. Phytopathol. 163, 1–10. doi: 10.1111/jph.12274
Muchembled, J., Deweer, C., Sahmer, K., and Halama, P. (2018). Antifungal activity of essential oils on two Venturia inaequalis strains with different sensitivities to tebuconazole. Environ. Sci. Pollut. Res. 25, 29921–29928. doi: 10.1007/s11356-017-0507-z
Muñoz, M., Rodríguez, A., Graciani, M., and Moyá, M. L. (2004). Conductometric, surface tension, and kinetic studies in mixed SDS-Tween 20 and SDS-SB3-12 micellar solutions. Langmuir 20, 10858–10867. doi: 10.1021/la048247n
Ongena, M., Jourdan, E., Adam, A., Paquot, M., Brans, A., Joris, B., et al. (2007). Surfactin and fengycin lipopeptides of Bacillus subtilis as elicitors of induced systemic resistance in plants. Environ. Microbiol. 9, 1084–1090. doi: 10.1111/j.1462-2920.2006.01202.x
Ongena, M., and Jacques, P. (2008). Bacillus lipopeptides: versatile weapons for plant disease biocontrol. Trends Microbiol. 16, 115–125. doi: 10.1016/j.tim.2007.12.009
Parisi, L., Fouillet, V., Schouten, H. J., Groenwold, R., Laurens, F., Didelot, F., et al. (2004). Variability of the pathogenicity of Venturia inaequalis in Europe. Acta Hortic. 663, 107–113. doi: 10.17660/ActaHortic.2004.663.13
Park, G., Nam, J., Kim, J., Song, J., Kim, P. I., Min, H. J., et al. (2019). Structure and mechanism of surfactin peptide from Bacillus velezensis antagonistic to fungi plant pathogens. Bull. Korean Chem. Soc. 40, 704–709. doi: 10.1002/bkcs.11757
Parker, J., Warrilow, A., Price, C., Mullins, J., Kelly, D., and Kelly, S. (2014). Resistance to antifungals that target CYP51. J. Chem. Biol. 7, 143–161. doi: 10.1007/s12154-014-0121-1
Patel, H., Tscheka, C., Edwards, K., Karlsson, G., and Heerklotz, H. (2011). All-or-none membrane permeabilization by fengycin-type lipopeptides from Bacillus subtilis QST713. Biochim. Biophys. Acta 1808, 2000–2008. doi: 10.1016/j.bbamem.2011.04.008
Pérez-Garcia, A., Romero, D., and De Vicente, A. (2011). Plant protection and growth stimulation by microorganisms: biotechnological applications of Bacilli in agriculture. Curr. Opin. Biotech. 22, 187–193. doi: 10.1016/j.copbio.2010.12.003
Pfeufer, E. E., and Ngugi, H. K. (2012). Orchard factors associated with resistance and cross resistance to sterol demethylation inhibitor fungicides in populations of Venturia inaequalis from Pennsylvania. Phytopathology 102, 272–282. doi: 10.1094/PHYTO-04-11-0117
Price, C. L., Parker, J. E., Warrilow, A. G. S., Kelly, D. E., and Kelly, S. L. (2015). Azole fungicides - understanding resistance mechanisms in agricultural fungal pathogens. Pest Manag. Sci. 71, 1054–1058. doi: 10.1002/ps.4029
R Core Team (2016). R: A Language and Environment for Statistical Computing. Vienna: R Foundation for Statistical Computing. Available at: https://www.R-project.org/
Razafindralambo, H., Dufour, S., Paquot, M., and Deleu, M. (2009). Thermodynamic studies of the binding interactions of surfactin analogues to lipid vesicles. J. Therm. Anal. Calorim. 95, 817–821. doi: 10.1007/s10973-008-9403-6
Romero, D., De Vicente, A., Olmos, J. L., Dávila, J. C., and Pérez-García, A. (2007a). Effect of lipopeptides of antagonistic strains of Bacillus subtilis on the morphology and ultrastructure of the cucurbit fungal pathogen Podosphaera fusca. J. Appl. Microbiol. 103, 969–976. doi: 10.1111/j.1365-2672.2007.03323.x
Romero, D., De Vicente, A., Rakotoaly, R. H., Dufour, S. E., Veening, J.-W., Arrebola, E., et al. (2007b). The iturin and fengycin families of lipopeptides are key factors in antagonism of Bacillus subtilis toward Podosphaera fusca. Mol. Plant. Microbe Interact. 20, 430–440. doi: 10.1094/mpmi-20-4-0430
Schnabel, G., and Jones, A. L. (2001). The 14α-demethylase (CYP51A1) gene is overexpressed in V. inaequalis strains resistant to myclobutanil. Phytopathology 91, 102–110. doi: 10.1094/PHYTO.2001.91.1.102
Sharma, N., Gruszewski, H. A., Park, S. W., Holm, D. G., and Vivanco, J. M. (2004). Purification of an isoform of patatin with antimicrobial activity against Phytophthora infestans. Plant Physiol. Bioch. 42, 647–655. doi: 10.1016/j.plaphy.2004.05.010
Shirane, N., Takenaka, H., Ueda, K., Hashimoto, Y., Katoh, K., and Ishii, H. (1996). Sterol analysis of DMI-resistant and –sensitive strains of V. inaequalis. Phytochemistry 41, 1301–1308. doi: 10.1016/0031-9422(95)00787-3
Stein, T. (2005). Bacillus subtilis antibiotics: structures, syntheses and specific functions. Mol. Microbiol. 56, 845–857. doi: 10.1111/j.1365-2958.2005.04587.x
Tao, Y., Bie, X. M., Lv, F. X., Zhao, H. Z., and Lu, Z. X. (2011). Antifungal activity and mechanism of fengycin in the presence and absence of commercial surfactin against Rhizopus stolonifer. J. Microbiol. 49, 146–150. doi: 10.1007/s12275-011-0171-9
Touré, Y., Ongena, M., Jacques, P., Guiro, A., and Thonart, P. (2004). Role of lipopeptides produced by Bacillus subtilis GA1 in the reduction of grey mould disease caused by Botrytis cinerea on apple. J. Appl. Microbiol. 96, 1151–1160. doi: 10.1111/j.1365-2672.2004.02252.x
Vanittanakom, N., Loeffler, W., Koch, U., and Jung, G. (1986). Fengycin-a novel antifungal lipopeptide antibiotic produced by Bacillus subtilis F-29-3. J. Antibiot. 39, 888–901. doi: 10.7164/antibiotics.39.888
Vijaya Palani, P., and Lalithakumari, D. (1999). Resistance of V. inaequalis to the sterol biosynthesis inhibiting fungicide, penconazole [1-(2-(2,4-dichlorophenyl) pentyl)-1H-1,2,4-triazole]. Mycol. Res. 103, 1157–1164. doi: 10.1017/s0953756299008321
Villani, S. M., Biggs, A. R., Cooley, D. R., Raes, J. J., and Cox, K. D. (2015). Prevalence of myclobutanil resistance and difenoconazole insensitivity in populations of V. inaequalis. Plant Dis. 99, 1526–1536. doi: 10.1094/PDIS-01-15-0002-RE
Villani, S. M., Hulvey, J., Hily, J. M., and Cox, K. D. (2016). Overexpression of the CYP51A1 gene and repeated elements are associated with differential sensitivity to DMI fungicides in Venturia inaequalis. Phytopathology 106, 562–71. doi: 10.1094/PHYTO-10-15-0254-R
Xu, X. M., Gao, L. Q., and Yang, J. R. (2010). Are insensitivities of Venturia inaequalis to myclobutanil and fenbuconazole correlated?. Crop Prot. 29, 183–189. doi: 10.1016/j.cropro.2009.07.002
Keywords: V. inaequalis, Bacillus subtilis, lipopeptides, biofungicide, antifungal activity
Citation: Desmyttere H, Deweer C, Muchembled J, Sahmer K, Jacquin J, Coutte F and Jacques P (2019) Antifungal Activities of Bacillus subtilis Lipopeptides to Two Venturia inaequalis Strains Possessing Different Tebuconazole Sensitivity. Front. Microbiol. 10:2327. doi: 10.3389/fmicb.2019.02327
Received: 27 July 2019; Accepted: 24 September 2019;
Published: 22 October 2019.
Edited by:
Fred Asiegbu, University of Helsinki, FinlandReviewed by:
Mate Viragh, Hungarian Academy of Sciences (MTA), HungaryFeyisara Eyiwumi Oni, Ghent University, Belgium
Copyright © 2019 Desmyttere, Deweer, Muchembled, Sahmer, Jacquin, Coutte and Jacques. This is an open-access article distributed under the terms of the Creative Commons Attribution License (CC BY). The use, distribution or reproduction in other forums is permitted, provided the original author(s) and the copyright owner(s) are credited and that the original publication in this journal is cited, in accordance with accepted academic practice. No use, distribution or reproduction is permitted which does not comply with these terms.
*Correspondence: Jérôme Muchembled, jerome.muchembled@isa-lille.fr; jerome.muchembled@yncrea.fr