- 1State Key Laboratory for Biology of Plant Diseases and Insect Pests, Institute of Plant Protection, Chinese Academy of Agricultural Sciences, Beijing, China
- 2College of Plant Science and Technology, Huazhong Agricultural University, Wuhan, China
- 3Department of Agriculture, Abdul Wali Khan University Mardan, Mardan, Pakistan
- 4Scientific Observing and Experimental Station of Crop Pests in Guilin, Ministry of Agriculture, Guilin, China
- 5Southern Regional Collaborative Innovation Center for Grain and Oil Crops in China, Changsha, China
The white-backed planthopper (WBPH), Sogatella furcifera Horváth (Hemiptera: Delphacidae), is an economically significant rice insect pest that harbors a primary fungal yeast-like symbiont (YLS), and some secondary bacterial symbionts like Wolbachia and Cardinium. In the present study, an additional bacterial symbiont in WBPH was characterized. Phylogenetic analysis employing the 16S rRNA gene showed a bacterium closely related to Asaia of Nilaparvata lugens and Nysius expressus, and Asaia krungthepensis. TEM observation of the bacterium showed the typical morphology of Asaia sp. with signature filamentous structures in the nucleoid region. These results indicate that the bacterium belongs to Asaia. The Asaia bacterium was detected in all the tested individual adults and tissues of the laboratory WBPH population but showed varying infection rates (ca 45%) in the field collected WBPH populations. Quantitative PCR analysis revealed that Asaia sp. were significantly more abundant in WBPH females than males, and mainly distributed in the guts, fatty bodies, and salivary glands. Asaia-infected WBPH were of shorter nymphal duration and heavier adult weight than Asaia-free WBPH, while Asaia-free WBPH comparatively fed more, indicating that Asaia plays a role in improving WBPH fitness through involvement in host’s nutrient supply.
Introduction
Insects are colonized by a complex microbial population in a symbiotic relationship (Dillon and Dillon, 2004; Moran et al., 2008). These associations have been formed between symbionts and host insects during long evolutionary processes that are generally categorized as: mutualism, commensalism, and parasitism (Werren et al., 2008; Frago et al., 2012). Some of these symbionts help in important physiological functions for their host insects in many ways. As per the significance of symbionts to the host, symbionts are divided into primary and secondary symbiont (Moran, 2001). The primary symbionts provide nutritional supplements for their hosts such as essential amino acids (Sloan and Moran, 2012), while some secondary symbionts manipulate host reproduction (Werren et al., 2008), or play beneficial roles such as protecting host from parasites and pathogens (Scarborough et al., 2005), increasing heat resistance of host (Dunbar et al., 2007), facilitating host’s digestion of food (Pais et al., 2008), and enhancing host’s insecticide resistance (Tsuchida et al., 2011).
Asaia sp. belongs to family Acetobacteriaceae and is an acetic acid bacterium (AAB) (Yamada et al., 2000). Acetic acid bacteria oxidize wine and sugar to acetic acid, except the genus Asaia (Crotti et al., 2010). Interestingly, Asaia have been reported to be associated with insects that feed on sugar-based diets, particularly those in the order Diptera (Favia et al., 2007; Crotti et al., 2009), Hymenoptera (Kautz et al., 2013; Good et al., 2014), Lepidoptera (Robinson et al., 2010), and Hemiptera (Crotti et al., 2009; Gonella et al., 2012). Asaia was first observed by Favia et al. (2007) in the salivary glands, guts and reproductive organs of Anopheles stephensi, dominating the microbiota of the mosquito. Asaia cells are typically characterized by the signature filamentous structures in the nucleoid region (Favia et al., 2007). Generally, Asaia is vertically transmitted from mother to the offspring by an egg smearing mechanism in A. gambiae (Crotti et al., 2009), but can also be transmitted to the progeny via venereal transfer during mating in A. stephensi (Damiani et al., 2008). Asaia isolated from A. stephensi and Aedes aegypti was able to colonize Scaphoideus titanus (Crotti et al., 2009) and transmitted horizontally via mating and co-feeding (Gonella et al., 2012). Asaia plays a pivotal role in the growth and reproduction of its hosts. For example, Asaia promotes the development of host larvae in A. stephensi and A. gambiae (Chouaia et al., 2012; Mitraka et al., 2013). Asaia co-existing with plasmodium parasites in the guts and salivary glands of A. stephensi play an immunity regulatory role through activating the expression of host antimicrobial peptides without inhibiting itself, leading to the possibility of introducing Asaia as a strong contender for malaria vector control via paratransgenesis technology (Favia et al., 2007; Damiani et al., 2010; Capone et al., 2013; Rami et al., 2018). Recently, Asaia were detected in the small brown planthopper (SBPH, Laodelphax striatellus) and the brown planthopper (BPH, Nilaparvata lugens) through 16S rDNA high-throughput sequencing technology (Li et al., 2017; Zhang et al., 2019).
The white-backed planthopper (WBPH), Sogatella furcifera Horváth, has been reported to harbor the primary fungal yeast-like symbiont (YLS) (Noda et al., 1995) and two secondary bacterial symbionts Wolbachia and Cardinium (Noda et al., 2001; Nakamura et al., 2012; Zhang et al., 2012a). The YLS makes available sterols to WBPH and also contributes in the nitrogen cycle (Noda and Koizumi, 2003). Wolbachia and Cardinium function to regulate WBPH reproduction (Noda et al., 2001; Nakamura et al., 2012; Zhang et al., 2012a). It is further determined that Cardinium helps to accelerate WBPH nymphal development (Zhang et al., 2012b).
In a previous study, we determined the presence and tissue localization of Asaia in WBPH by 16S rDNA high-throughput sequencing and fluorescence in situ hybridization (Li et al., 2019). To further characterize the bacterial symbiont Asaia sp. in WBPH and its functional association with WBPH, in this study, we detected Asaia in WBPH through diagnostic PCR and morphological observation by transmission electron microscopy (TEM), performed phylogenetic analysis by cloning, and sequencing the 16S rDNA of Asaia, determined Asaia densities in WBPH by quantitative PCR, and measured Asaia effects on WBPH fitness.
Materials and Methods
White-Backed Planthopper Populations
A laboratory WBPH population (Lab population) originated from a colony collected in 2014 from rice fields in Xing’an (25°36′18″ N, 110°42′16″ E), China, was maintained using caged rice seedlings (var. Taichung Native 1, TN1) for 27 generations in an insectary (27 ± 5°C, relative humidity 80 ± 5%, and a photoperiod of 14 L: 10 D). Three additional WBPH populations (LS population, WH population, and CS population) were collected from the fields in 2016 (Supplementary Table S1). WBPH adults are characterized by a distinct yellowish-white pronotum and mesonotum and can be easily discriminated from adults of other planthopper species. The field-collected insects were kept initially in 95% alcohol and subsequently stored at −80°C until DNA extraction and were used only in detecting infection rates of symbiotic bacteria.
Detection of Asaia in WBPH
Presence of Asaia in WBPH was previously determined by 16S rDNA high-throughput sequencing and fluorescence in situ hybridization (Li et al., 2019). Diagnostic PCR were used to further detect the presence of Asaia in individual adults of the four WBPH populations and in tissues and individual offspring adults of the Lab population using the Asaia-specific primers. The adult tissues of the Lab population were obtained by dissecting the adults in a drop of sterile 0.01 M PBS buffer (Ph 7.2) under a stereo microscope. The offspring adults (F1 generation) were obtained by pairing parents in glass tubes (180 mm in height and 30 mm in diameter) with 3-leaf rice seedling and rearing the resulting newly hatched nymphs individually in new glass tubes with rice seedlings until they became adults. Total DNA was extracted from individual adults and the tissues using the Wizard® Genomic DNA Purification Kit (Promega, United States), according to the manufacturer’s protocols. The Asaia-specific primers Asafor and Asarev (Table 1) were used to amplify a sequence of 181 bp from the 16S rRNA gene of Asaia (Favia et al., 2007). The PCR conditions were: 1 cycle of 94°C for 5 min; 35 cycles of 94°C for 30 s, 62°C for 30 s, and 72°C for 30 s; and a final extension of 72°C for 10 min. Sterile water was included in all PCRs as a negative control and the DNA sample of WBPH verified by cloning and sequencing was used as a positive control.
The presence of Asaia in WBPH was further morphologically detected using transmission electron microscope (TEM). Adult guts of the Lab population were dissected. After the gut samples were fixed in 2% glutaraldehyde overnight at 4°C and then washed with 0.1 M PBS buffer (pH 7.2) four times (30 min each time), they were postfixed in 1% OsO4 in 0.1 M sodium cacodylate buffer (pH 7.2) for 2 h at 4°C and washed with 0.1 M PBS buffer (pH 7.2) two times (15 min each time). Thereafter, the samples were dehydrated through a graded ethanol series (30, 50, 70, 80, 90, 95, and 100%) for 10 min at each concentration, and finally through acetone for three 10-min times. The prepared samples were embedded in 618 epoxy resin (Sigma, United States) and polymerized at 37°C for 12 h, 45°C for 12 h, and 60°C for 48 h, and then sliced into ultrathin sections (60 nm) by a Leica EM UC6 ultrathin slicer (Leica, Germany) and stained with uranyl acetate. The final sample sections were examined under a Hitachi-7500 TEM (Hitachi, Japan) (Crotti et al., 2009).
Molecular Phylogenetic Analysis of Asaia in WBPH
The 16S rRNA gene was used to identify Asaia in the four populations. Total DNA was extracted from individual adults of the four populations as above. The bacterial 16S rRNA gene was amplified using the universal primers 27F (5′-AGAGTTTGATCCTGGCTCAG-3′) and 1492R (5′-ACGGTTACCTTGTTACGACTT-3′) (Weisburg et al., 1991). The PCR analyses were conducted with Taq polymerase (Takara, Japan) in an ABI 9700 thermocycler (Thermo Fisher Scientific, United States). The PCR conditions were: 1 cycle of 94°C for 3 min; 35 cycles of 94°C for 30 s, 52°C for 30 s, and 72°C for 2 min; and a final extension of 72°C for 10 min. Amplified fragments were purified by the AxyPrepTM DNA Gel Extraction Kit, according to the manufacturer’s protocols (Corning, United States), and the PCR products with the expected size were cloned into the pMD-19T plasmid vector (Takara, Japan) and sequenced in an ABI 3730XL DNA analyzer. The 16S rRNA gene sequences of Asaia were registered in GenBank under accession numbers of MK814862, MK811206, MK811207 (Supplementary Table S1), and MK598732 for the LS, WH, CS, and Lab populations, respectively.
A phylogenetic tree was constructed using MEGA v6.0 (Tamura et al., 2013). DNA sequence similarities among Asaia species were investigated using the BLAST search tool1. A multiple alignment of nucleotide sequences was performed using the program package Clustal W with the default parameters (Tamura et al., 2013). The final alignment was manually inspected and corrected (deposited under Supplementary Materials). The phylogenetic tree was constructed using the maximum likelihood (ML) method with HKY + G model. Bootstrap analysis of 1,000 replicates was used to deduce confidence levels.
Asaia Densities in Relation to WBPH Stages and Tissues
Asaia densities were quantified by real-time fluorescence quantitative PCR (qPCR) in various tissues and developmental stages of the Lab population. Twenty newly emerged WBPH adults were dissected as above to obtain a sample of each of the salivary glands, guts, reproductive organs, and fat bodies for qPCR. Asaia densities were also measured in different developmental stages, i.e., nymphs of five instars (20, 10, 5, 5, and 5 insects pooled per sample, respectively) and female and male adults of 8 age groups (0, 2, 4, 6, 8, 10, 12, and 14 d post emergence) (5 insects pooled per sample).
The total DNA was extracted as per the method described above. The copy numbers of the 16S RNA gene of Asaia were quantified using specific primers Asafor and Asarev (Table 2). qPCR analyses were conducted with SYBR® Premix Ex TaqTM II (Takara, Japan) in ABI 7500 Real-Time System (Thermo Fisher Scientific, United States). The PCR temperature profile was 2 min at 95°C 40 cycles of 5 s at 95°C and 34 s at 60°C. The qPCR analyses were biologically performed 3 times. The number of 16S rRNA gene copies of Asaia in the individual tissues and insects was calculated using absolute quantification analysis. A serial of dilutions (102, 103, 104, 105, 106, 107, and 108) of the standard plasmids, containing the sequence of 181 bp from the 16S rRNA gene of Asaia, was used as PCR templates for the establishment of a standard curve.
Asaia Effects on WBPH Fitness
To verify the effects of Asaia on WBPH fitness, an Asaia-free strain (A– strain) was established via oral treatment of WBPH with tetracycline hydrochloride (Amresco, United States). In a glass beaker (180 mm in height and 100 mm in diameter), 150 ml 0.25 mg/ml tetracycline hydrochloride was added to a cotton pad lined on the bottom, and 100 rice seeds (TN1) were seeded on the cotton pad for germination in the insectary. After 7 days, 100 newly hatched nymphs of the Lab population (F0 generation) that were infectious with YLS, Wolbachia, Cardinium and Asaia (A+ strain) were introduced into the beaker and allowed to feed ad lib in the insectary. Twelve days later, the resulting F0 adults were paired in glass tubes with an antibiotic-free 3-leaf rice seedling for oviposition. The hatched nymphs were left to develop on antibiotic-free rice seedlings, the resulting newly emerged F1 adults were paired for oviposition for 7 days and then individually detected for presence of symbionts (YLS, Wolbachia, Cardinium and Asaia) using diagnostic PCR and the specific primers (Table 1). If both F1 parent adults were negative with Asaia while positive with YLS, Wolbachia and Cardinium, their offsprings (A– strain) were reared on antibiotic-free rice plants for another 9 generations to eliminate possible side effects of the antibiotic treatment (Noda et al., 2001) before they were used in fitness measurements. The A+ and A– strains were periodically subjected to qPCR for quantification of YLS, Wolbachia, Cardinium, and Asaia to make sure that the two obtained strains differed only in Asaia.
To measure Asaia effects on WBPH nymphal duration and adult weight, 100 newly hatched nymphs (<12 h) of each of the A+ and A– strains were randomly collected and individually reared on 15 3-leaf rice seedlings in the glass tube in the insectary. Nymphal duration was examined to 30 nymphs and weight was measured to 30 newly emerged male and female adults from each strain.
Newly emerged WBPH adults (<12 h) of each of the A+ and A– strains were randomly selected and paired in the glass tube with 15 3-leaf rice seedlings for test of fecundity and longevity. Upon adult death, fecundity per female (number of hatched nymphs plus number of eggs remaining in the seedlings) and adult longevity were recorded. The tests were run for 30 adult pairs for each strain.
Feeding amount represented by honeydew excretion was measured using a parafilm sachet method (Pathak et al., 1982). Three newly emerged female adults of each of the A+ and A– strains starved for 1 h were aspirated into a parafilm sachet (5 cm × 5 cm) attached to the stem of a tillering rice plant in the insectary. After 24 h, the insects were removed and each of the parafilm sachets was weighed immediately and, after removal of the honeydew, were weighed again using a balance (XP205, Mettler Toledo, Switzerland). The net weight of honeydew excretion was calculated. The tests were repeated for 30 times for each WBPH strain.
Statistical Analysis
All the data of symbiotic bacteria densities were converted by logarithm before statistical analysis. Asaia densities in tissues and nymphal instars of the Lab population WBPH were analyzed by one-way analysis of variance (ANOVA), means were separated using a Tukey test when equal variance was assumed or Games-Howell test when equal variance was not assumed. Two-way analysis of variance (ANOVA) was performed to analyze for significant influence of age and sex on densities of Asaia in WBPH adults, differences in Asaia densities between female and male of different ages were analyzed by independent sample T test. The differences in fitness parameters of the A+ and A– strains were analyzed by independent sample T test. All statistical analyses were performed with IBM SPSS Statistics 25.0 (IBM, United States) at P < 0.05.
Results
Detection of Asaia in WBPH
The presence of Asaia sp. in WBPH was verified through detection of the 16S rRNA gene of Asaia in individual adults of the four populations and tissues of the Lab population using Asaia-specific primers (Table 1). Asaia was present in all the individual parental adults and tissues of the Lab population, while the infection rates of both female and male adults of the field-collected populations varied from 36.7 to 63.3% and from 33.3 to 53.3%, respectively, averaging 46.7% (42/90) and 45.6% (41/90) (Table 3). When newly hatched nymphs of the parental insects of the Lab population were reared individually, the Asaia infection in the resulting offspring adults was only 30% (36/120) (Table 3).
Presence of Asaia in WBPH was further morphologically detected by TEM in hindgut sections of WBPH. Bacterial cells resembling Asaia were observed (Figure 1), confirming the results of PCR analysis. Figure 1A shows a portion of the hindgut of WBPH adults harboring a polymorphic bacterial flora. Some of the bacteria presented the morphological signatures of Asaia sp., i.e., bright filamentous nucleoid region and electron-dense cytoplasm microinclusions resembling enterosomes (Figure 1B), previously described for Asaia cells in A. stephensi, A. aegypti, and S. titanus (Favia et al., 2007; Crotti et al., 2009). These signatures of bacterial cells suggest the presence of Asaia in WBPH.
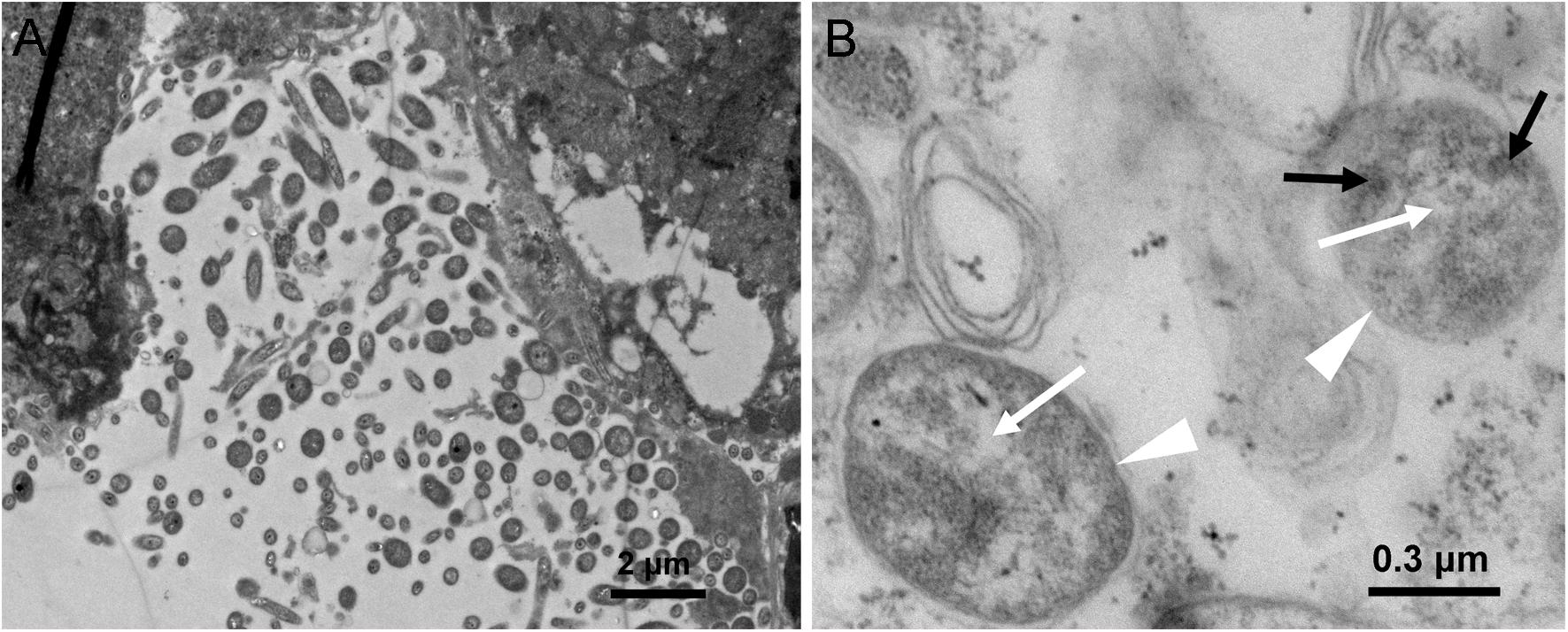
Figure 1. TEM micrographs of an WBPH adult female hindgut. (A) Hindgut lumen of female WBPH. (B) Detail of panel A, showing two bacteria (indicated by white triangle), where black arrowheads indicate enterosomes in the bacterial cytoplasm, and white arrowheads indicate bright filamentous nucleoid region.
Molecular Phylogenetic Analysis of Asaia in WBPH
Four 1452 bp 16S rRNA gene sequences of Asaia were obtained from the four WBPH populations, which were deposited in GenBank. The sequences showed limited variation between the four populations (0–1 nucleotide differences), and the 16S rRNA gene sequence of Asaia in the Lab population was used in phylogenetic analysis. According to BLAST analysis, the 16S rRNA sequence of WBPH showed 99.93% similarity to that from Asaia of BPH (GenBank no. FJ774959), 97.93% to that from Asaia of Nysius expressus (GenBank no. JQ726820), and 96.33% to that from Asaia krungthepensis (GenBank no. AB682130). Phylogenetic analysis of the 16S rRNA sequences using ML method clustered Asaia of WBPH, BPH and N. expressus to a single clade that was distinct from the known Asaia of mosquitoes and A. krungthepensis (Figure 2).
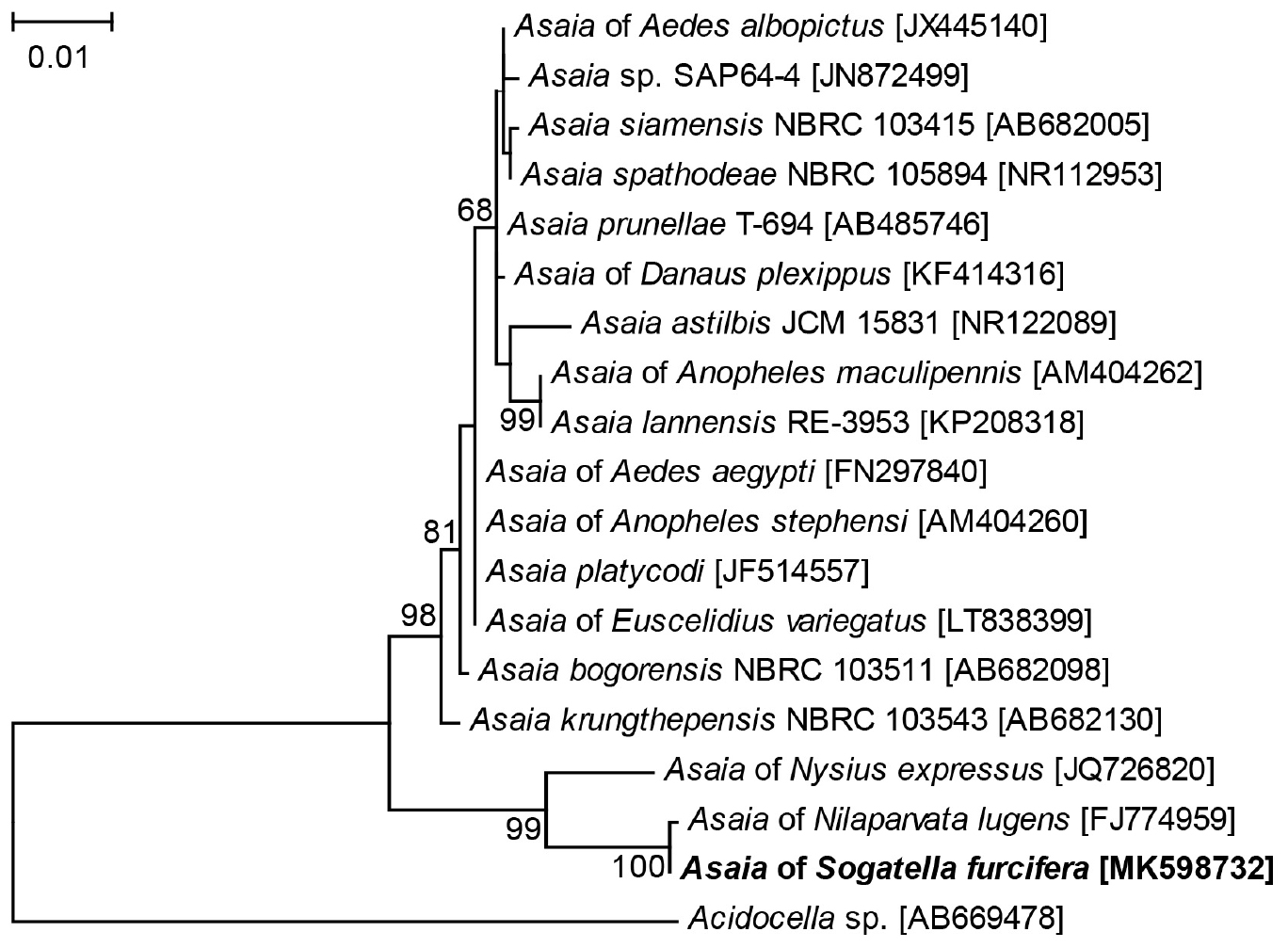
Figure 2. Phylogenetic tree based on Asaia 16S rRNA gene sequences. The tree was constructed using HKY + G model for ML method. The names and sequence GenBank accession numbers (in parentheses) are shown. Sequences obtained in this study are shown in bold. Only Bootstrap values >50 are represented. Acidocella sp. of the family Acetobacteriaceae is used as outgroup.
Asaia Densities in Relation to WBPH Stages and Tissues
When expressed as the number of 16S rRNA gene copies per insect, Asaia densities increased with the nymphal instar (F = 1033.081, df = 4,10, P < 0.001; Figure 3A), being the lowest in the first instars and increasing significantly as the nymphs developed through the second to the fourth instars (Tukey test, P ≤ 0.001). In the adult stage, Asaia densities showed a unimodal type change with adult age, peaking in 8 days old females and males (Figure 3B). ANOVA showed that the Asaia densities in WBPH adults were significantly affected by both age (F = 221.682, df = 7,32, P < 0.001) and sex (F = 273.767, df = 1,32, P < 0.001) and their interaction (F = 6.775, df = 7,32, P < 0.001). Asaia densities in females were significantly higher than those in males with the exception of 2 days old adults (t ≥ 3.552, df = 4, P ≤ 0.024; Figure 3B). Among the tissues, Asaia abundance differed significantly (F = 1305.572, df = 4, 10, P < 0.001; Figure 3C), being the most in the guts and the fatty bodies, significantly lower in the salivary glands, and still significantly lower in the reproductive organs (ovaries and testes) (Games-Howell test, P ≤ 0.023).
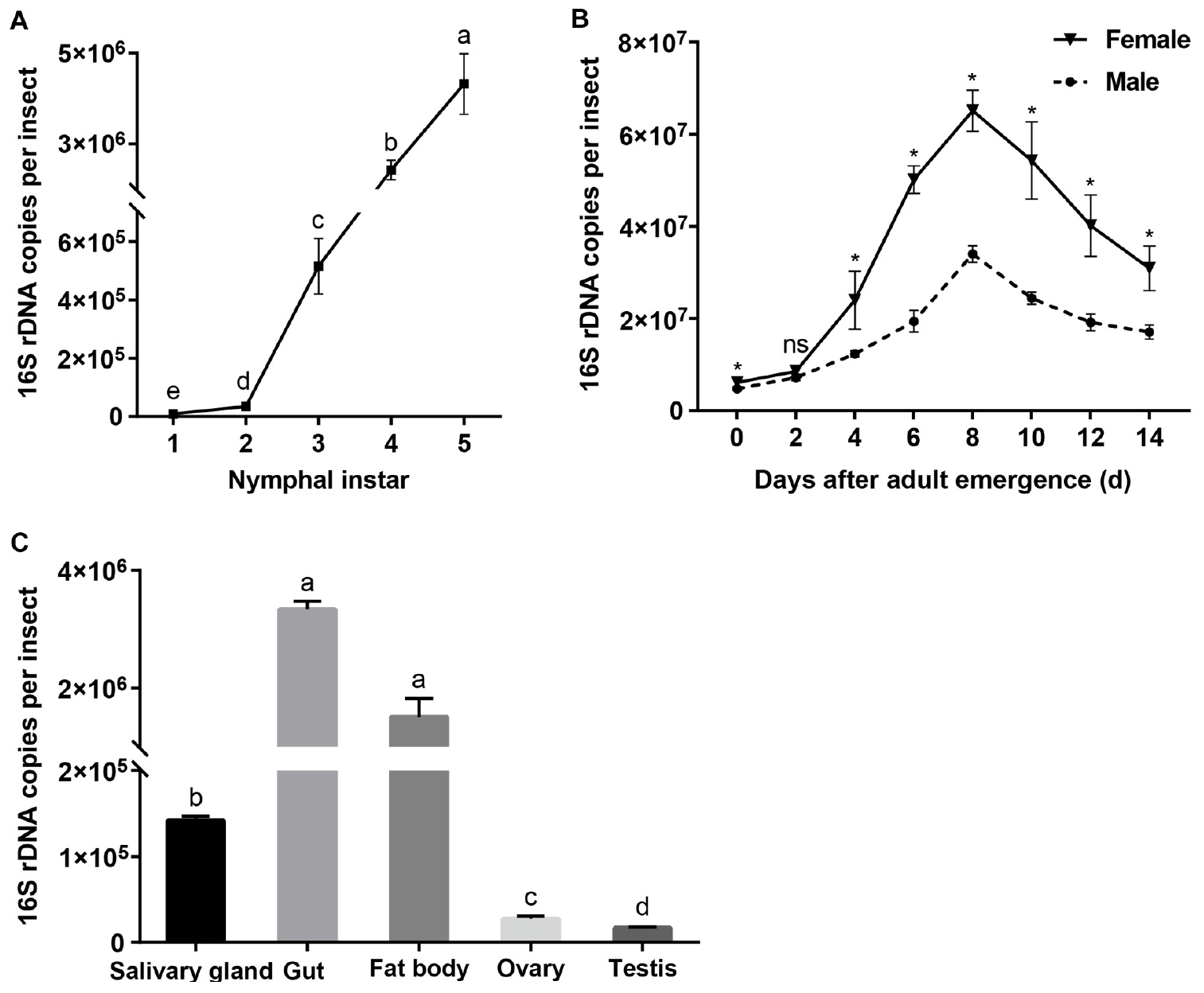
Figure 3. Asaia densities in WBPH. (A) Nymphs. (B) Adults. (C) Tissues. The data are expressed as means ± sd. Different letters in panel A and panel C indicate significant differences based on Tukey test and Games-Howell test at P < 0.05, respectively. In panel B, ∗ indicates significant difference and ns, no significant difference, between female and male of different ages according to independent sample T test at P < 0.05.
Asaia Effects on WBPH Fitness
The established A+ and A– WBPH strains differed significantly in Asaia density (male: t = 314.792, df = 4, P < 0.001; female: t = 766.028, df = 4, P < 0.001), while they showed no differences in densities of YLS (male: t = 0.918, df = 4, P = 0.410; female: t = 0.302, df = 4, P = 0.778), Wolbachia (male: t = 0.133, df = 4, P = 0.900; female: t = 0.413, df = 4, P = 0.700), and Cardinium (male: t = 0.227, df = 4, P = 0.832; female: t = 0.555, df = 4, P = 0.608) (Supplementary Figure S1). With these two strains, Asaia effects on WBPH fitness were assessed in terms of nymphal duration, adult weight, fecundity, adult longevity, and feeding amount. Nymphal development of A– strain was delayed 0.41 and 0.44 d in males and females compared with that of A+ strain, respectively (male: t = 3.016, df = 58, P = 0.004; female: t = 2.817, df = 58, P = 0.007; Figure 4A). Newly emerged adults of A+ strain weighed significantly more than adults of A– strain by a difference of 0.05 mg in males (t = 3.830, df = 58, P < 0.001) and a difference of 0.13 mg in females (t = 5.324, df = 58, P < 0.001; Figure 4B). However, feeding amount represented by honeydew excretion was significantly greater in A– females (8.50 mg) than in A+ females (4.53 mg) (t = 8.961, df = 58, P < 0.001; Figure 4E). No differences were observed in adult longevity (male: t = 0.262, df = 58, P = 0.794; female: t = 0.734, df = 58, P = 0.466; Figure 4C) and fecundity (t = 0.261, df = 58, P = 0.795; Figure 4D) between A+ strain and A– strain.
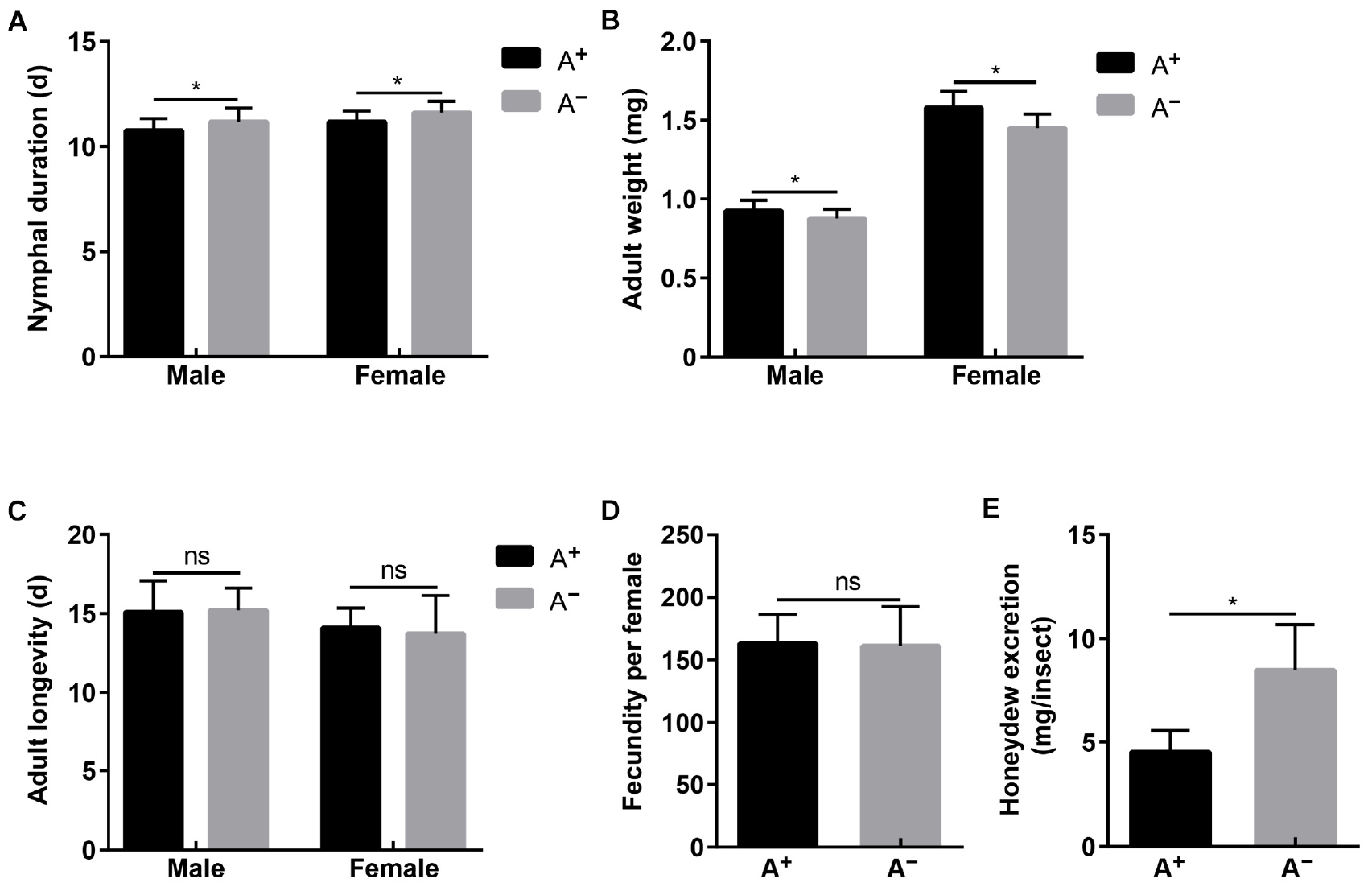
Figure 4. Fitness of A+ and A– WBPH strains that differed in Asaia densities while showed no differences in densities of YLS, Wolbachia and Cardinium. (A) Nymphal duration. (B) Adult longevity. (C) Adult weight. (D) Fecundity. (E) Honeydew excretion. Means and standard deviation are shown. Asterisk indicate significant differences based on independent sample T test at P < 0.05, while ns indicates no significant difference.
Discussion
So far, Asaia has been detected in many insects, including rice planthoppers SBPH (Li et al., 2017) and BPH (Zhang et al., 2019). Recently, an Asaia sp. was detected in WBPH populations through 16S rDNA high-throughput sequencing (Li et al., 2019). The present study further confirmed the presence of Asaia in WBPH via diagnostic PCR using the 16S rRNA gene (Table 3) and TEM observation (Figure 1). Asaia was detected in about 45% of adults of the three field collected WBPH populations (Table 3), contrasting to the 100% infection in adult wild populations of A. gambiae (Damiani et al., 2010). The reason may be that the Asaia in A. gambiae is vertically transmitted at 100% (Damiani et al., 2010), while the Asaia in WBPH is vertically transmitted at 30% (Table 3). The low vertical transmission of Asaia in WBPH is consistent with the low density of Asaia found in the ovaries and testes (Figure 3C). Nevertheless, the adult individuals and tissues of the Lab WBPH population were 100% infected with Asaia (Table 3), which was consistent with the results in the laboratory population of A. gambiae (Damiani et al., 2010). Also, Asaia is capable of intraspecies horizontal transmission in S. titanus (Gonella et al., 2012) and interspecies cross-colonizing between Ae. aegypti, A. stephensi and S. titanus (Crotti et al., 2009). The 100% infection with Asaia in the Lab WBPH population (Table 3) is probably due to high horizontal transmission of Asaia in co-feeding WBPH reared in a cage. Therefore, it can be hypothesized that low vertical transmission coupled with high horizontal transmission in co-feeding lead to the discrepancies in Asaia infection between the Lab and field collected WBPH populations. In TEM observation, the bacterial cells in WBPH showed the typical morphology of Asaia sp. with signature filamentous structures in the nucleoid region (Figure 1B) like the Asaia cells in A. aegypti and S. titanus (Crotti et al., 2009). With these results, it can be ensured that Asaia sp. exists in WBPH.
Phylogenetic analysis of 16S rRNA gene sequences clustered the symbiotic bacteria Asaia in WBPH, BPH, and N. expressus to the same branch that was distinct from the known Asaia of mosquitoes and A. krungthepensis (Figure 2). According to Tindall et al. (2010), when similarity of a microorganism 16S rRNA gene sequence to all the others is <97%, it is acceptable to classify the microorganism as a new species; and when the similarity is ≤95%, the microorganism may be classified into a new genus. The 16S rRNA gene of Asaia in WBPH exhibits 96.33% sequence similarity with that of the previously described A. krungthepensis. Therefore, the Asaia of WBPH may be a new species different from A. krungthepensis.
Asaia abundance in WBPH nymphs experienced an exponential increase with the progress of instars (Figure 3A). In Diaphorina citri nymphs, Wolbachia also showed a similar increase pattern in relation to nymph instars (Dossi et al., 2014). In the adults, Asaia abundance underwent a unimodal change, peaking in 8 days old adults (Figure 3B), indicating Asaia proliferation was correlated with the reproductively active stage of WBPH. Similar results have been reported for Buchnera density in adult aphids (Baumann, 2005; Sakurai et al., 2005). Asaia density decreased in the old WBPH adults (Figure 3B); a similar symbiont drop has also been observed in Acyrthosiphon pisum (Nishikori et al., 2009) and Camponotus floridanus (Ratzka et al., 2013). Interestingly, Asaia densities in the newly emerged WBPH adults were not different from those in the old nymphs (Figures 3A,B). Asaia densities also responded to the sex, being higher in WBPH females than in males (Figure 3B). Female-biased symbiont densities have also been reported for Wolbachia and Cardinium in WBPH (Noda et al., 2001; Nakamura et al., 2012; Zhang et al., 2012a). It can be expected that the higher density in female adults may benefit from vertical transmission of symbionts, as suggested for Portiera in Bemisia tabaci (Bing et al., 2013). In horizontal transmission through-feeding, symbionts gained from diet first reach the gut lumen, after crossing numerous physical and biochemical barriers in the haemocoel, and can finally colonize different tissues (Vallet-Gely et al., 2008). Quantitative PCR data showed that Asaia in WBPH largely colonized the salivary glands, guts and fat bodies, and a small amount of Asaia was found in the testes and ovaries (Figure 3C), confirming our results suggesting tissue localization of Asaia in WBPH (Table 3). A similar tissue specific Asaia abundance was reported in Anopheles mosquitoes, where Asaia acquired through feeding numerously colonized the gut and then spread to other tissues through hemolymph (Favia et al., 2007; Rami et al., 2018). Abundance differences of Asaia among/between tissues, sexes, nymphal and adult stages may relate to the biological functions for WBPH, which need to be determined in further studies.
Asaia effects on WBPH fitness were assessed using A+ and A– WBPH strains. Asaia promoted nymphal development (Figure 4A) and increased adult weight (Figure 4B), which corresponds to the rapid increase in Asaia density in progressing nymphal instars (Figure 3A), indicating that Asaia may play a role in improving WBPH fitness, as Buchnera providing essential amino acids for aphids (Baumann, 2005; Sakurai et al., 2005). Our results agree with previous studies in Anopheles mosquitoes, where Asaia accelerated development of A. stephensi (Chouaia et al., 2012) and A. gambiae (Mitraka et al., 2013), and increased nymphal weight (Mitraka et al., 2013). In phloem sap feeding Hemiptera insects, the amount of honeydew is used to represent food intake (Pathak et al., 1982). The amount of honeydew secreted by the A– strain almost doubled that of the A+ strain (Figure 4E), similar to the result reported in Aphis fabae in the absence of secondary symbionts (Schillewaert et al., 2017). The phloem sap of rice is characterized by an imbalance of nutrient components and cannot satisfy the normal growth and developmental needs for planthoppers (Xue et al., 2014). Therefore, we assume that Asaia plays a role in supplementing the nutritional needs of WBPH. Thereby, when WBPH are deprived of Asaia, the insects compensate lack of nutrients through increasing feeding. Surprisingly, the differences in adult longevity (Figure 4C) and fecundity (Figure 4D) between A+ and A– strains were not significant. These results show that, even though Asaia is not essential for WBPH survival, it affects the host fitness to some extent probably through involvement in host nutrient supply. Further studies using metagenomics and proteomics techniques are needed to investigate the fundamental mechanism underlying Asaia effects on WBPH fitness, especially its nutritional role for the host. It is worth noting that feeding WBPH on tetracycline hydrochloride-treated rice seedlings resulted in A– insects at about 80%, which indicates that Asaia may be more susceptible to tetracycline hydrochloride than the other symbionts. Asaia is mostly harbored in the alimentary organs, such as the guts and salivary glands, thus making it easily exposed to the antibiotics.
In summary, we detected the presence of Asaia in WBPH through TEM observation and diagnostic PCR, characterized its phylogenies, density dynamics, and effects on host fitness. Asaia of WBPH, BPH, and N. expressus are phylogenetically in a single clade that is distinct from the known Asaia of mosquitoes and A. krungthepensis. Asaia densities increase with WBPH nymphal development, and are greater in gut, fat body and salivary gland, and greater in females than in males. Asaia in WBPH functions to accelerate nymphal development and increase adult weight, while reduce feeding. A better understanding of the interactions between host insects and symbionts will provide ample information on the potential of manipulating microbiota to control insect pests. Further studies are needed to explore the roles of Asaia at the nutritional and molecular level, and the details of its horizontal transmission in WBPH.
Data Availability Statement
The datasets generated for this study can be found in the MK814862, MK811206, MK811207, and MK598732.
Author Contributions
FL, HH, and MH conceived and designed the experiments. FL performed the experiments. FL and MH analyzed the data. FL, AA, and MH wrote the manuscript. All the authors read and approved the final version of the manuscript.
Funding
This study was financially supported by the National Key R&D Program of China (2018YFD0200300 and 2016YFD0300700).
Conflict of Interest
The authors declare that the research was conducted in the absence of any commercial or financial relationships that could be construed as a potential conflict of interest.
Acknowledgments
The authors thank Qi Cai and Haiyan Zhang for assistance in the experiments. The authors are grateful to Baoli Qiu from the South China Agricultural University for comments of an early draft of the manuscript.
Supplementary Material
The Supplementary Material for this article can be found online at: https://www.frontiersin.org/articles/10.3389/fmicb.2019.02179/full#supplementary-material
Footnotes
References
Baumann, P. (2005). Biology of bacteriocyte-associated endosymbionts of plant sap-sucking insects. Annu. Rev. Microbiol. 59, 155–189. doi: 10.1146/annurev.micro.59.030804.121041
Bing, X. L., Yang, J., Zchori-Fein, E., Wang, X. W., and Liu, S. S. (2013). Characterization of a newly discovered symbiont of the whitefly Bemisia tabaci (Hemiptera: Aleyrodidae). Appl. Environ. Microbiol. 79, 569–575. doi: 10.1128/AEM.03030-12
Cao, W., Ma, Z., Chen, Y. H., and Yu, X. (2015). Pichia anomala, a new species of yeast-like endosymbionts and its variation in small brown planthopper (Laodelphax striatellus). J. Biosci. Bioeng. 119, 669–673. doi: 10.1016/j.jbiosc.2014.11.007
Capone, A., Ricci, I., Damiani, C., Mosca, M., Rossi, P., Scuppa, P., et al. (2013). Interactions between Asaia, Plasmodium and Anopheles: new insights into mosquito symbiosis and implications in malaria symbiotic control. Parasit. Vectors. 6:182. doi: 10.1186/1756-3305-6-182
Chouaia, B., Rossi, P., Epis, S., Mosca, M., Ricci, I., Damiani, C., et al. (2012). Delayed larval development in Anopheles mosquitoes deprived of Asaia bacterial symbionts. BMC Microbiol. 12:S2. doi: 10.1186/1471-2180-12-S1-S2
Crotti, E., Damiani, C., Pajoro, M., Gonella, E., Rizzi, A., Ricci, I., et al. (2009). Asaia, a versatile acetic acid bacterial symbiont, capable of cross–colonizing insects of phylogenetically distant genera and orders. Environ. Microbiol. 11, 3252–3264. doi: 10.1111/j.1462-2920.2009.02048.x
Crotti, E., Rizzi, A., Chouaia, B., Ricci, I., Favia, G., Alma, A., et al. (2010). Acetic acid bacteria, newly emerging symbionts of insects. Appl. Environ. Microbiol. 76, 6963–6970. doi: 10.1128/AEM.01336-10
Damiani, C., Ricci, I., Crotti, E., Rossi, P., Rizzi, A., Scuppa, P., et al. (2008). Paternal transmission of symbiotic bacteria in malaria vectors. Curr. Biol. 18, R1087–R1088. doi: 10.1016/j.cub.2008.10.040
Damiani, C., Ricci, I., Crotti, E., Rossi, P., Rizzi, A., Scuppa, P., et al. (2010). Mosquito-bacteria symbiosis: the case of Anopheles gambiae and Asaia. Microb. Ecol. 60, 644–654. doi: 10.1007/s00248-010-9704-8
Dillon, R. J., and Dillon, V. M. (2004). The gut bacteria of insects: nonpathogenic interactions. Annu. Rev. Entomol. 49, 71–92. doi: 10.1146/annurev.ento.49.061802.123416
Dossi, F. C., da Silva, E. P., and Cônsoli, F. L. (2014). Population dynamics and growth rates of endosymbionts during Diaphorina citri (Hemiptera, Liviidae) ontogeny. Microb. Ecol. 68, 881–889. doi: 10.1007/s00248-014-0463-9
Dunbar, H. E., Wilson, A. C. C., Ferguson, N. R., and Moran, N. A. (2007). Aphid thermal tolerance is governed by a point mutation in bacterial symbionts. PLoS Biol. 5:1006–1015. doi: 10.1371/journal.pbio.0050096
Favia, G., Ricci, I., Damiani, C., Raddadi, N., Crotti, E., Marzorati, M., et al. (2007). Bacteria of the genus Asaia stably associate with Anopheles stephensi, an Asian malarial mosquito vector. Proc. Natl. Acad. Sci. U.S.A. 104, 9047–9051. doi: 10.1073/pnas.0610451104
Frago, E., Dicke, M., and Godfray, H. C. J. (2012). Insect symbionts as hidden players in insect-plant interactions. Trends Ecol. Evol. 27, 705–711. doi: 10.1016/j.tree.2012.08.013
Gonella, E., Crotti, E., Rizzi, A., Mandrioli, M., Favia, G., Daffonchio, D., et al. (2012). Horizontal transmission of the symbiotic bacterium Asaia sp. in the leafhopper Scaphoideus titanus Ball (Hemiptera: Cicadellidae). BMC Microbiol. 12:S4. doi: 10.1186/1471-2180-12-S1-S4
Good, A. P., Gauthier, M. P. L., Vannette, R. L., and Fukami, T. (2014). Honey bees avoid nectar colonized by three bacterial species, but not by a yeast species, isolated from the bee gut. PLoS One 9:e86494. doi: 10.1371/journal.pone.0086494
Hou, Y., Ma, Z., Dong, S., Chen, Y. H., and Yu, X. (2013). Analysis of yeast-like symbiote diversity in the brown planthopper (BPH), Nilaparvata lugens Stål, using a novel nested PCR-DGGE protocol. Curr. Microbiol. 67, 263–270. doi: 10.1007/s00284-013-0356-z
Kautz, S., Rubin, B. E., Russell, J. A., and Moreau, C. S. (2013). Surveying the microbiome of ants: comparing 454 pyrosequencing with traditional methods to uncover bacterial diversity. Appl. Environm. Microbiol. 79, 525–534. doi: 10.1128/AEM.03107-12
Li, F., Li, P., Hua, H., Hou, M., and Wang, F. (2019). Diversity, tissue localization and infection pattern of bacterial symbionts in the white-backed planthopper, Sogatella furcifera (Hemiptera: Delphacidae). Microb. Ecol. doi: 10.1007/s00248-019-01433-4
Li, S., Zhou, C., Chen, G., and Zhou, Y. (2017). Bacterial microbiota in small brown planthopper populations with different rice viruses. J. Basic Microbiol. 57, 590–596. doi: 10.1002/jobm.201700004
Mitraka, E., Stathopoulos, S., Siden-Kiamos, I., Christophides, G. K., and Louis, C. (2013). Asaia accelerates larval development of Anopheles gambiae. Pathog. Glob. Health. 107, 305–311. doi: 10.1179/2047773213Y.0000000106
Moran, N. A. (2001). The coevolution of bacterial endosymbionts and phloem-feeding insects. Ann. Missouri. Bot. Gard. 88, 35–44. doi: 10.2307/2666130
Moran, N. A., McCutcheon, J. P., and Nakabachi, A. (2008). Genomics and evolution of heritable bacterial symbionts. Annu. Rev. Genet. 42, 165–190. doi: 10.1146/annurev.genet.41.110306.130119
Nakamura, Y., Yukuhiro, F., Matsumura, M., and Noda, H. (2012). Cytoplasmic incompatibility involving Cardinium and Wolbachia in the white-backed planthopper Sogatella furcifera (Hemiptera Delphacidae). Appl. Entomol. Zool. 47, 273–283. doi: 10.1007/s13355-012-0120-z
Nishikori, K., Morioka, K., Kubo, T., and Morioka, M. (2009). Age-and morph-dependent activation of the lysosomal system and Buchnera degradation in aphid endosymbiosis. J. Insect Physiol. 55, 351–357. doi: 10.1016/j.jinsphys.2009.01.001
Noda, H., and Koizumi, Y. (2003). Sterol biosynthesis by: cytochrome P450 sterol C-22 desaturase genes from yeast-like symbiotes of rice planthoppers and anobiid beetles. Insect Biochem. Mol. Biol. 33, 649–658. doi: 10.1016/s0965-1748(03)00056-0
Noda, H., Koizumi, Y., Zhang, Q., and Deng, K. (2001). Infection density of Wolbachia and incompatibility level in two planthopper species, Laodelphax striatellus and Sogatella furcifera. Insect Biochem. Mol. Biol. 31, 727–737. doi: 10.1016/S0965-1748(00)00180-6
Noda, H., Nakashima, N., and Koizumi, M. (1995). Phylogenetic position of yeast-like symbiotes of rice planthoppers based on partial 18S rDNA sequences. Insect Biochem. Mol. Biol. 25, 639–646. doi: 10.1016/0965-1748(94)00107-s
Pais, R., Lohs, C., Wu, Y., Wang, J., and Aksoy, S. (2008). The obligate mutualist Wigglesworthia glossinidia influences reproduction, digestion, and immunity processes of its host, the tsetse fly. Appl. Environ. Microbiol. 74, 5965–5974. doi: 10.1128/AEM.00741-08
Pathak, P. K., Saxena, R. C., and Heinrichs, E. A. (1982). Heinrichs EA. parafilm sachet for measuring honeydew excretion by Nilaparvata lugens on rice. J. Econ. Entomol. 75, 194–195. doi: 10.1093/jee/75.2.194
Rami, A., Raz, A., Zakeri, S., and Djadid, N. D. (2018). Isolation and identification of Asaia sp. in Anopheles spp. mosquitoes collected from Iranian malaria settings: steps toward applying paratransgenic tools against malaria. Parasit. Vectors. 11:367. doi: 10.1186/s13071-018-2955-9
Ratzka, C., Gross, R., and Feldhaar, H. (2013). Gene expression analysis of the endosymbiont-bearing midgut tissue during ontogeny of the carpenter ant Camponotus floridanus. J. Insect Physiol. 59, 611–623. doi: 10.1016/j.jinsphys.2013.03.011
Robinson, C. J., Schloss, P., Ramos, Y., Raffa, K., and Handelsman, J. (2010). Robustness of the bacterial community in the cabbage white butterfly larval midgut. Microb. Ecol. 59, 199–211. doi: 10.1007/s00248-009-9595-8
Sakamoto, J. M., Feinstein, J., and Rasgon, J. L. (2006). Wolbachia infections in the cimicidae: museum specimens as an untapped resource for endosymbiont surveys. Appl. Environ. Microbiol. 72, 3161–3167. doi: 10.1128/AEM.72.5.3161-3167.2006
Sakurai, M., Koga, R., Tsuchida, T., Meng, X. Y., and Fukatsu, T. (2005). Rickettsia symbiont in the pea aphid Acyrthosiphon pisum: novel cellular tropism, effect on host fitness, and interaction with the essential symbiont Buchnera. Appl. Environ. Microbiol. 71, 4069–4075. doi: 10.1128/AEM.71.7.4069-4075.2005
Scarborough, C. L., Ferrari, J., and Godfray, H. C. J. (2005). Aphid protected from pathogen by endosymbiont. Science 310, 1781–1781. doi: 10.1126/science.1120180
Schillewaert, S., Parmentier, T., Vantaux, A., Van den Ende, W., Vorburger, C., and Wenseleers, T. (2017). The influence of facultative endosymbionts on honeydew carbohydrate and amino acid composition of the black bean aphid Aphis fabae. Physiol. Entomol. 42, 125–133. doi: 10.1111/phen.12181
Sloan, D. B., and Moran, N. A. (2012). Endosymbiotic bacteria as a source of carotenoids in whiteflies. Biol. Lett. 8, 986–989. doi: 10.1098/rsbl.2012.0664
Tamura, K., Stecher, G., Peterson, D., Filipski, A., and Kumar, S. (2013). MEGA6: molecular evolutionary genetics analysis version 6.0. Mol. Biol. Evol. 30, 2725–2729. doi: 10.1093/molbev/mst197
Tindall, B. J., Rosselló-Móra, R., Busse, H. J., Ludwig, W., and Kämpfer, P. (2010). Notes on the characterization of prokaryote strains for taxonomic purposes. Int. J. Syst. Evol. Microbiol. 60, 249–266. doi: 10.1099/ijs.0.016949-0
Tsuchida, T., Koga, R., Matsumoto, S., and Fukatsu, T. (2011). Interspecific symbiont transfection confers a novel ecological trait to the recipient insect. Biol. Lett. 7, 245–248. doi: 10.1098/rsbl.2010.0699
Vallet-Gely, I., Lemaitre, B., and Boccard, F. (2008). Bacterial strategies to overcome insect defences. Nat. Rev. Microbiol. 6, 302–313. doi: 10.1038/nrmicro1870
Weeks, A. R., Velten, R., and Stouthamer, R. (2003). Incidence of a new sex ratio-distorting endosymbiotic bacterium among arthropods. Proc. Biol. Sci. 270, 1857–1865. doi: 10.1098/rspb.2003.2425
Weisburg, W. G., Barns, S. M., Pelletier, D. A., and Lane, D. J. (1991). 16S ribosomal DNA amplification for phylogenetic study. J. Bacteriol. 173, 697–703. doi: 10.1128/jb.173.2.697-703.1991
Werren, J. H., Baldo, L., and Clark, M. E. (2008). Wolbachia: master manipulators of invertebrate biology. Nat. Rev. Microbiol. 6, 741–751. doi: 10.1038/nrmicro1969
Xue, J., Zhou, X., Zhang, C. X., Yu, L. L., Fan, H. W., Wang, Z., et al. (2014). Genomes of the rice pest brown planthopper and its endosymbionts reveal complex complementary contributions for host adaptation. Genom. Biol. 15:521. doi: 10.1186/s13059-014-0521-0
Yamada, Y., Katsura, K., Kawasaki, H., Widyastuti, Y., Saono, S., Seki, T., et al. (2000). Asaia bogorensis gen. nov., sp. nov., an unusual acetic acid bacterium in the alpha-proteobacteria. Int. J. Syst. Evol. Microbiol. 50, 823–829. doi: 10.1099/00207713-50-2-823
Zhang, J. H., Yu, N., Xu, X. X., and Liu, Z. W. (2019). Community structure, dispersal ability and functional profiling of microbiome existing in fat body and ovary of the brown planthopper. Nilaparvata lugens. Insect Sci. 26, 683–694. doi: 10.1111/1744-7917.12575
Zhang, X. F., Zhao, D. X., and Hong, X. Y. (2012a). Caridinium–the leading factor of cytomplasmic incompatibility in the planthopper Sogatella furcifera doubly infected with Wolbachia and Cardinium. Environ. Entomol. 41, 833–840. doi: 10.1603/EN12078
Zhang, X. F., Zhao, D. X., Li, H. S., and Hong, X. Y. (2012b). Expression of cytoplasmic incompatibility and host fitness effects in field populations of Sogatella furcifera infected with Cardinium. J. Econ. Entomol. 105, 2161–2166. doi: 10.1603/ec12268
Keywords: Sogatella furcifera, bacterial symbiont, Asaia, infection, host fitness
Citation: Li F, Hua H, Ali A and Hou M (2019) Characterization of a Bacterial Symbiont Asaia sp. in the White-Backed Planthopper, Sogatella furcifera, and Its Effects on Host Fitness. Front. Microbiol. 10:2179. doi: 10.3389/fmicb.2019.02179
Received: 11 June 2019; Accepted: 05 September 2019;
Published: 18 September 2019.
Edited by:
Mariana Mateos, Texas A&M University, United StatesCopyright © 2019 Li, Hua, Ali and Hou. This is an open-access article distributed under the terms of the Creative Commons Attribution License (CC BY). The use, distribution or reproduction in other forums is permitted, provided the original author(s) and the copyright owner(s) are credited and that the original publication in this journal is cited, in accordance with accepted academic practice. No use, distribution or reproduction is permitted which does not comply with these terms.
*Correspondence: Maolin Hou, bWFvbGluaG91X2lwcEAxNjMuY29t; bWxob3VAaXBwY2Fhcy5jbg==