- 1Department of Laboratory Medicine and Pathobiology, University of Toronto, Toronto, ON, Canada
- 2SAR Laboratories, Sandra Rotman Centre for Global Health, University Health Network-Toronto General Hospital, Toronto, ON, Canada
- 3Tropical Disease Unit, Division of Infectious Diseases, Department of Medicine, University of Toronto, Toronto, ON, Canada
Healthy fetal development is dependent on nutrient and oxygen transfer via the placenta. Optimal growth and function of placental vasculature is therefore essential to support in utero development. Vasculogenesis, the de novo formation of blood vessels, and angiogenesis, the branching and remodeling of existing vasculature, mediate the development and maturation of placental villi, which form the materno-fetal interface. Several lines of evidence indicate that systemic maternal infection and consequent inflammation can disrupt placental vasculogenesis and angiogenesis. The resulting alterations in placental hemodynamics impact fetal growth and contribute to poor birth outcomes including preterm delivery, small-for-gestational age (SGA), stillbirth, and low birth weight (LBW). Furthermore, pathways involved in maternal immune activation and placental vascularization parallel those involved in normal fetal development, notably neurovascular development. Therefore, immune-mediated disruption of angiogenic pathways at the materno-fetal interface may also have long-term neurological consequences for offspring. Here, we review current literature evaluating the influence of maternal infection and immune activation at the materno-fetal interface and the subsequent impact on placental vascular function and birth outcome. Immunomodulatory pathways, including chemokines and cytokines released in response to maternal infection, interact closely with the principal pathways regulating placental vascular development, including the angiopoietin-Tie-2, vascular endothelial growth factor (VEGF), and placental growth factor (PlGF) pathways. A detailed mechanistic understanding of how maternal infections impact placental and fetal development is critical to the design of effective interventions to promote placental growth and function and thereby reduce adverse birth outcomes.
Introduction
Each year an estimated 20 million infants are born low birth weight (LBW) (<2,500 g) and 14.9 million are born preterm (Lee et al., 2013). Preterm birth (PTB) is the leading direct cause of under 5 mortality, responsible for more than 1 million deaths per year (Liu et al., 2016). According to the Global Burden of Disease Study, the disability-adjusted life years attributable to PTB is 77 million, comparable to the estimates for HIV or malaria (Murray et al., 2012). While these adverse birth outcomes predominantly occur in low- and middle-income countries (Blencowe et al., 2012), rates are increasing globally, and have been consistently linked with increased risks of long-term health consequences for offspring including cardiovascular disease, diabetes, obesity, and neurodevelopmental disorders (Bilbo and Schwarz, 2009; Calkins and Devaskar, 2011). The burden of infectious diseases in pregnancy (e.g., malaria, HIV, sexually transmitted infections) is also highest in low- and middle-income countries, and a growing body of evidence indicates that these prevalent infections contribute to poor birth outcomes by inflammation-mediated disruption of placental development and function (Watson-Jones et al., 2002; Chico et al., 2017; Conroy et al., 2017; McDonald et al., 2018, 2019).
Fetal development is governed by tightly regulated processes at the materno-fetal interface. Placental vasculogenesis and angiogenesis mediate placental vascular development, which is critical to nutrient and oxygen delivery to the developing fetus. These processes are primarily regulated by mediators in the vascular endothelial growth factor (VEGF) and angiopoietin families (Geva et al., 2002). Dysregulation of these factors is associated with inadequate placental vascularization, leading to hemodynamic placental insufficiency, inadequate delivery of nutrients and oxygen to the fetus, and consequently adverse birth outcomes (Kaufmann et al., 2003). Inflammatory and angiogenic pathways are interdependent and co-regulatory, suggesting that the host response to maternal infection could dysregulate pathways essential for placental vascular development. Here, we review the impact of systemic maternal infections resulting in immune activation at the materno-fetal interface – and its subsequent impact on placental vascularization – adverse birth outcomes, and later-life neurocognitive deficits in offspring.
Placental Development: Vasculogenesis and Angiogenesis
The placenta forms the primary interface between mother and fetus, and a healthy functioning placenta is essential for a successful pregnancy. The placenta is a multi-function organ, acting as the site of materno-fetal nutrient, oxygen and waste exchange; producing hormones and growth factors critical for pregnancy progression and maintenance; and acting as a barrier to protect the fetus from maternal immune attack, toxins, and infectious pathogens (Wang and Zhao, 2010). These functions all rely on proper vascularization and perfusion of the placenta, and disruptions to placental vascular development and adaptation are associated with adverse pregnancy outcomes including preeclampsia, small-for-gestational age (SGA), PTB, and stillbirth (Kingdom, 1998; Gagnon, 2003; Wang and Zhao, 2010; Romero et al., 2011; Conroy et al., 2013; Morgan, 2016; Silver, 2018).
Placental vascular development begins early in pregnancy and undergoes adaptations across gestation. On the maternal side, uteroplacental circulation is established by the end of the first trimester (Wang and Zhao, 2010). Maternal vascular adaptation involves remodeling of the uterine spiral arteries by invasive fetal-derived extravillous trophoblasts to enable low-resistance blood flow into the intervillous space of the placenta (Wang and Zhao, 2010; Pollheimer et al., 2018). On the fetal side, primary placental villi begin to develop around day 13 post-conception, and fetoplacental vascularization of villi begins around 21 days post-conception (Kingdom et al., 2000; Kaufmann et al., 2004; Demir et al., 2006). The tertiary villi around which maternal blood flows in the intervillous space act as the functional units of the materno-fetal interface. Fetal-derived syncytiotrophoblasts are the primary mediators of exchange, protein-production, and defense at the materno-fetal interface.
Vascularization of placental villi involves the sequential processes of vasculogenesis and angiogenesis. Vasculogenesis is the de novo formation of blood vessels via differentiation of mesenchymal cells to hemangiogenic stem cells and then endothelial precursors (Demir et al., 2007). The VEGF family of ligands and receptors are heavily involved in the regulation of both vasculogenesis and angiogenesis. VEGF and its receptors (VEGFR-1 and -2) are expressed very early in placental development, and the production of VEGF by cytotrophoblasts and Hofbauer cells is thought to drive early placental vasculogenesis and subsequent angiogenesis (Kaufmann et al., 2004; Demir et al., 2006, 2007).
Angiogenesis begins at approximately 32 days post-conception (Kaufmann et al., 2004). From this point until term, the placental vascular network needed to support the rapidly growing fetus is built predominantly via branching and non-branching angiogenesis. The molecular mediation of angiogenesis requires tight temporal and spatial coordination and interaction between VEGF and angiopoietin protein family signaling (Ahmed and Perkins, 2000; Carmeliet, 2000; Yancopoulos et al., 2000; Geva et al., 2002; Charnock-Jones et al., 2004; Kaufmann et al., 2004; Benirschke et al., 2012). VEGF, placental growth factor (PlGF), and their inhibitor soluble fms-like tyrosine kinase-1 (sFlt-1) are produced by trophoblasts, and their balance is critical to healthy placental vascular development (Ahmed and Perkins, 2000; Kingdom et al., 2000; Charnock-Jones et al., 2004; Kaufmann et al., 2004). The timing and ratio of angiopoietin-1 (Ang-1) and its antagonist angiopoietin-2 (Ang-2) signaling through their receptor Tie2 is also essential for placental vascularization. Ang-1 promotes vascular maturation and stability, whereas Ang-2 allows for the destabilization and endothelial plasticity required for VEGF to drive angiogenesis and vascular remodeling (Carmeliet, 2000; Yancopoulos et al., 2000; Geva et al., 2002). These functions are reflected in the longitudinal dynamics of Ang-1 and -2 in healthy pregnancies: Ang-1 is initially low and increases across pregnancy as placental vasculature becomes more established, while Ang-2 decreases across pregnancy (Geva et al., 2002). Several groups have hypothesized that the tightly regulated longitudinal dynamics of VEGF and PlGF, as well as Ang-1 and -2, provide a molecular basis for the temporal transition from vasculogenesis to branching and then non-branching angiogenesis that underlies placental vascular development (Figure 1; Ahmed and Perkins, 2000; Geva et al., 2002; Kaufmann et al., 2004).
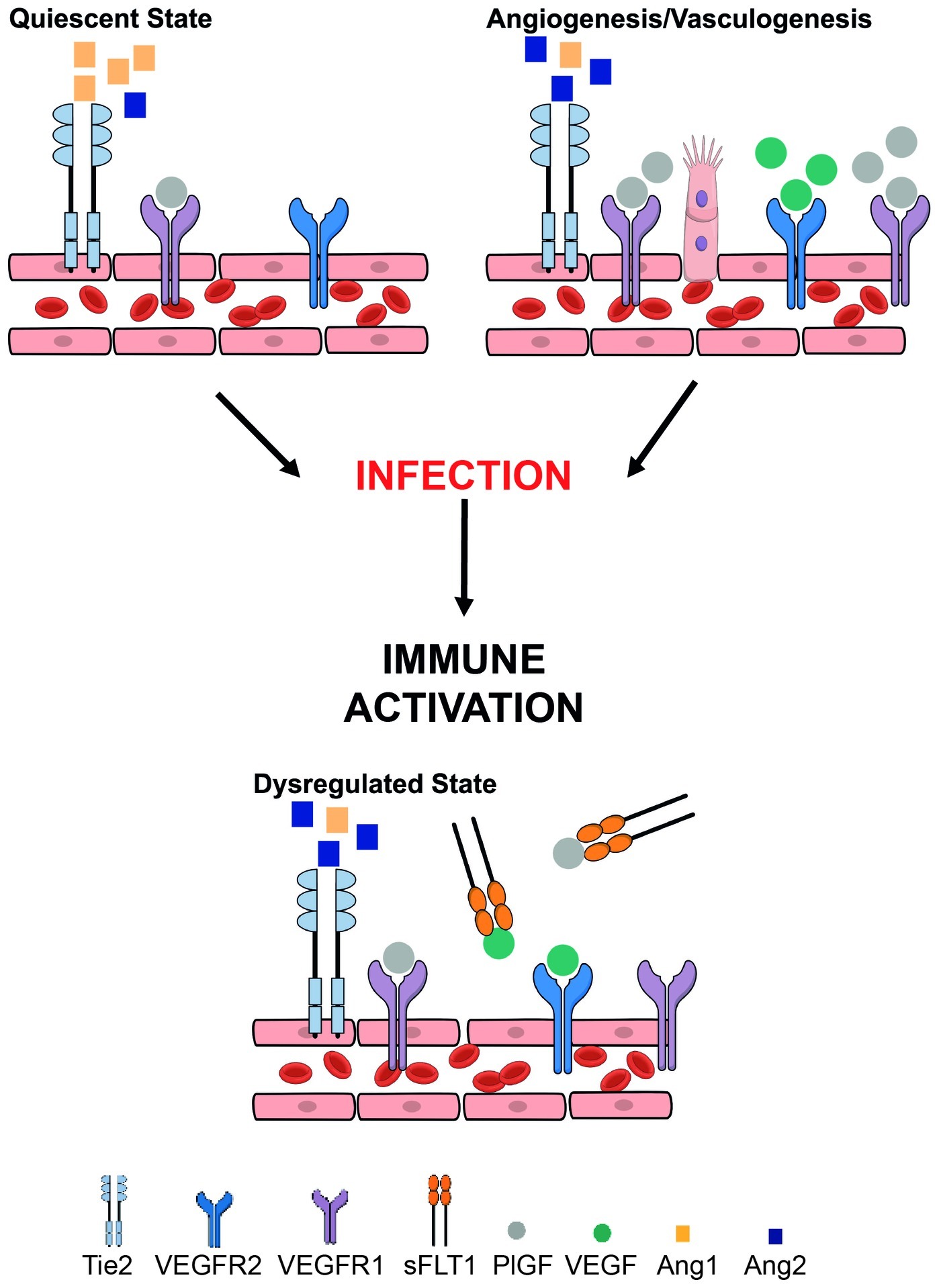
Figure 1. Overview of key angiogenic and vasculogenic factors mediating placental function and how they may be disrupted in the context of maternal infection. Placental vasculogenesis and angiogenesis are processes that are vital for placental vascular development and function. These processes depend on a fine balance between pro-angiogenic and anti-angiogenic pathways. The vascular endothelial growth factor (VEGF) family of proteins (including PlGF – placental growth factor) are pro-angiogenic mediators. VEGF and PlGF bind VEGF receptor 1 [fms-like tyrosine kinase (Flt-1)] to induce vessel proliferation and sprouting. Alternative splicing of Flt-1 results in soluble Flt-1 (sFlt-1) that is anti-angiogenic. Angiopoietin-1 (Ang-1) binds its tyrosine-kinase receptor Tie2 inducing vessel maturation, whereas angiopoietin-2 (Ang-2) promotes vessel destabilization and angiogenesis. Tight control of these pathways is essential for proper vascular development, remodeling, robust placental function, and healthy birth outcomes. Maternal infection (e.g., malaria, HIV-1) can result in immune activation and inflammation which dysregulates these tightly regulated processes, contributing to poor birth outcomes.
VEGF, PlGF, and VEGFR-1 are expressed on extravillous and villous trophoblasts, as well as Hofbauer cells in human placentas (Charnock-Jones et al., 1994; Ahmed et al., 1995; Clark et al., 1996; Khaliq et al., 1996; Vuorela et al., 1997). Their signaling has a role in trophoblast function including proliferation, differentiation, and nitric oxide (NO) production (Charnock-Jones et al., 1994; Ahmed et al., 1997; Athanassiades et al., 1998; Athanassiades and Lala, 1998; Khaliq et al., 1999). Ang-1 and -2 and their receptor Tie2 are also expressed in villous and extravillous trophoblasts in specific cell-type and temporal patterns across pregnancy, and in vitro studies reported a role for Ang/Tie2 signaling in trophoblast NO production and migration (Dunk et al., 2000; Goldman-Wohl et al., 2000; Seval et al., 2008).
Collectively, these data support critical roles for the VEGF and angiopoietin pathways in both fetoplacental vascularization (i.e., vasculogenesis and angiogenesis in the villi) and trophoblast function, as well as uteroplacental remodeling (i.e. trophoblast-mediated maternal spiral artery remodeling). With such diverse and interdependent roles for angiogenic factors across placental development, it is not surprising that their dysregulation has been associated with pathologic pregnancies and adverse birth outcomes.
Placental Development: A Role for Inflammatory Mediators
In a healthy pregnancy, the maternal immune system adapts to protect the semi-allogeneic fetus and placenta. Circulating levels of both cytokines [e.g., interferon (IFN)-γ, tumor necrosis factor (TNF), etc.] and components of the complement system (e.g., C3a, C5a, etc.) are altered across normal pregnancy (Kraus et al., 2010; Regal et al., 2015). Several cell types in the placenta including maternal and fetal-derived placental cells, as well as specialized immune cells like decidual natural killer cells (dNK), produce, express and/or secrete inflammatory cytokines and complement regulatory proteins at the materno-fetal interface in a healthy pregnancy (Bowen et al., 2002; Weckman et al., 2018). These inflammatory mediators play a dual role in immunity and processes of normal placental development including extravillous trophoblast proliferation and invasion necessary for uterine spiral artery remodeling (Albieri et al., 1999; Bowen et al., 2002; Hanna et al., 2006; Bulla et al., 2008; Pollheimer et al., 2018). Furthermore, dNKs play an important role in placental vascular development via the production of angiogenic factors including VEGF, PlGF, Ang-1, and Ang-2 (Hanna et al., 2006; Le Bouteiller, 2013). Trophoblasts also increase VEGF production in response to cytokine stimulation, and monocytes will increase production of sFlt-1 in response to complement activation (Choi et al., 2002; Girardi et al., 2006; Conroy et al., 2009). Inflammatory and angiogenic systems are interdependent and tightly regulated across pregnancy. Together, their regulation is critical to placental vascular development. Therefore, disruption of either system could lead to a cascade of downstream events with negative impacts on placental vascular development and birth outcomes.
Abnormal Placental Vascular Development Underlies Pregnancy Complications
There is abundant evidence for defective maternal spiral artery remodeling (Khong et al., 1986; Hustin et al., 1990; Pijnenborg et al., 1991; Kingdom et al., 2000; Romero et al., 2011; Fisher, 2015; Burton and Jauniaux, 2018; Pollheimer et al., 2018), abnormal villous development and vascularization (Jackson et al., 1995; Kingdom and Kaufmann, 1997; Kingdom et al., 2000; Vedmedovska et al., 2011; Burton and Jauniaux, 2018; Silver, 2018; Travaglino et al., 2019), and impaired umbilical blood flow (Trudinger et al., 1985; Salafia et al., 1997; Ferrazzi et al., 2000; Zhu et al., 2016) in a range of pregnancy outcomes with placental pathologies including preeclampsia, SGA, PTB, spontaneous abortion, and stillbirth.
Dysregulation of specific angiogenic mediators has also been associated with placental insufficiency and adverse pregnancy outcomes. The balance between angiogenic and anti-angiogenic factors, and the resulting alterations to placental vasculature, is modulated by multiple factors including oxygen homeostasis, external agents (e.g., drugs), infection, and inflammation (Khaliq et al., 1999; Kaufmann et al., 2004; Girardi et al., 2006; Conroy et al., 2013; McDonald et al., 2018; Mohammadi et al., 2018). Seminal preclinical studies established the importance of a precise angiogenic balance in utero, as both an absence and excess of VEGF and the angiopoietins were associated with abnormal embryonic development or lethality (Carmeliet et al., 1996; Suri et al., 1996; Maisonpierre et al., 1997; Miquerol et al., 2000). These findings have been extended to human studies, where multiple adverse pregnancy outcomes have been linked to dysregulation of circulating levels of angiogenic mediators critical for normal placental development including VEGF, PlGF, and sFlt-1, Ang-1 and -2, and soluble endoglin (Sharkey et al., 1996; Levine et al., 2004, 2006; Venkatesha et al., 2006; Chaiworapongsa et al., 2009; Romero et al., 2010; Conroy et al., 2013, 2017; Darling et al., 2014; McDonald et al., 2015b, 2018; Straughen et al., 2017).
As discussed above, there is a tight interrelationship between inflammatory pathways and angiogenic balance during placental development. Consequently, adverse pregnancy outcomes including spontaneous PTB and SGA have been associated with simultaneous dysregulation of both pathways (Girardi et al., 2006; Conroy et al., 2013; Darling et al., 2014; McDonald et al., 2015b). These data strongly support a role for disrupted placental development, due to inflammatory and angiogenic dysregulation at the materno-fetal interface, as a common pathway in the pathobiology of adverse birth outcomes.
Maternal Infection Disrupts Placental Vascular Development
An important contributor to disruption of the inflammatory and angiogenic environment at the materno-fetal interface is maternal infection. Infections due to the TORCH pathogens [Toxoplasma gondii, others, rubella virus, cytomegalovirus (CMV), and herpes simplex virus] may result in adverse pregnancy outcomes via vertical transmission to the fetus (Coyne and Lazear, 2016). However, even in the absence of congenital infection, maternal infections such as malaria and HIV have been linked with adverse birth outcomes including PTB, LBW, SGA, and stillbirth (Desai et al., 2007; Wedi et al., 2016; Rogerson et al., 2018). Despite increased coverage of treatment for infections in pregnancy such as malaria and HIV, rates of adverse birth outcomes remain high (Madanitsa et al., 2016; Santosa et al., 2019), and a better understanding of the pathophysiology underlying infection-induced adverse birth outcomes is needed. Increasing evidence suggests placental vascular pathology may be an important contributor to the link between maternal infection and adverse birth outcomes.
A growing body of evidence has linked maternal infection with abnormal placental pathology and altered maternal and fetal hemodynamics. Histopathological examination of human placentas have reported abnormal placental villous architecture and maternal vascular malperfusion in the context of viral, bacterial and parasitic maternal infections (Carmona-Fonseca et al., 2013; Ahmed et al., 2014; Chaikitgosiyakul et al., 2014; Kim et al., 2015; Kalk et al., 2017; Ribeiro et al., 2017; Moeller et al., 2018). Preclinical studies have also demonstrated significantly altered placental vascularization, vascular remodeling, and oxygen transport in response to maternal infection (Tabata et al., 2012; Conroy et al., 2013; Hirsch et al., 2018; McDonald et al., 2018; Phillips et al., 2018). In humans, maternal infections including influenza, Helicobacter pylori, malaria, and HIV have been associated with impaired maternal and fetal hemodynamics (e.g., high arterial resistance) (Dorman et al., 2002; Griffin et al., 2012; Hernandez-Andrade et al., 2014; McClure et al., 2014; Di Simone et al., 2017; Ome-Kaius et al., 2017). The available evidence implicates abnormalities in placental vascularization and function as a common driver behind infection-induced adverse birth outcomes, even in non-congenital infections.
Mechanistically, dysregulation of angiogenic mediators including sFlt-1, Ang-1, and -2, soluble endoglin, and PlGF and altered placental vascular structure and function has been reported in the context of maternal infections (e.g., malaria, HIV, CMV, and acute pyelonephritis) associated with adverse birth outcomes (Chaiworapongsa et al., 2010; Silver et al., 2010, 2011; Conroy et al., 2013, 2017; Ataíde et al., 2015; Gustafsson et al., 2015; McDonald et al., 2018). Inflammatory mediators including cytokines [e.g., interleukin (IL)-1, INF-γ, and TNF] and the complement system exhibit regulatory cross-talk with angiogenic factors critical to placental development (Naldini and Carraro, 2005; Fiedler and Augustin, 2006; Girardi et al., 2006; Conroy et al., 2009, 2013). There is evidence for systemic inflammation in bacterial, viral, and parasitic maternal infections (Horton et al., 2008; Conroy et al., 2013; Cérbulo-Vázquez et al., 2014; Romero et al., 2016; Fried et al., 2017; Wilkinson et al., 2017; Harjunmaa et al., 2018; McDonald et al., 2019), and statistical modeling suggests a hierarchical relationship between dysregulated inflammation, angiogenesis, and adverse birth outcomes (Conroy et al., 2013). Collectively, these studies suggest inflammation-mediated dysregulation of the tight angiogenic balance required for placental development as a shared mechanism underlying adverse birth outcomes in maternal infection (Figure 2).
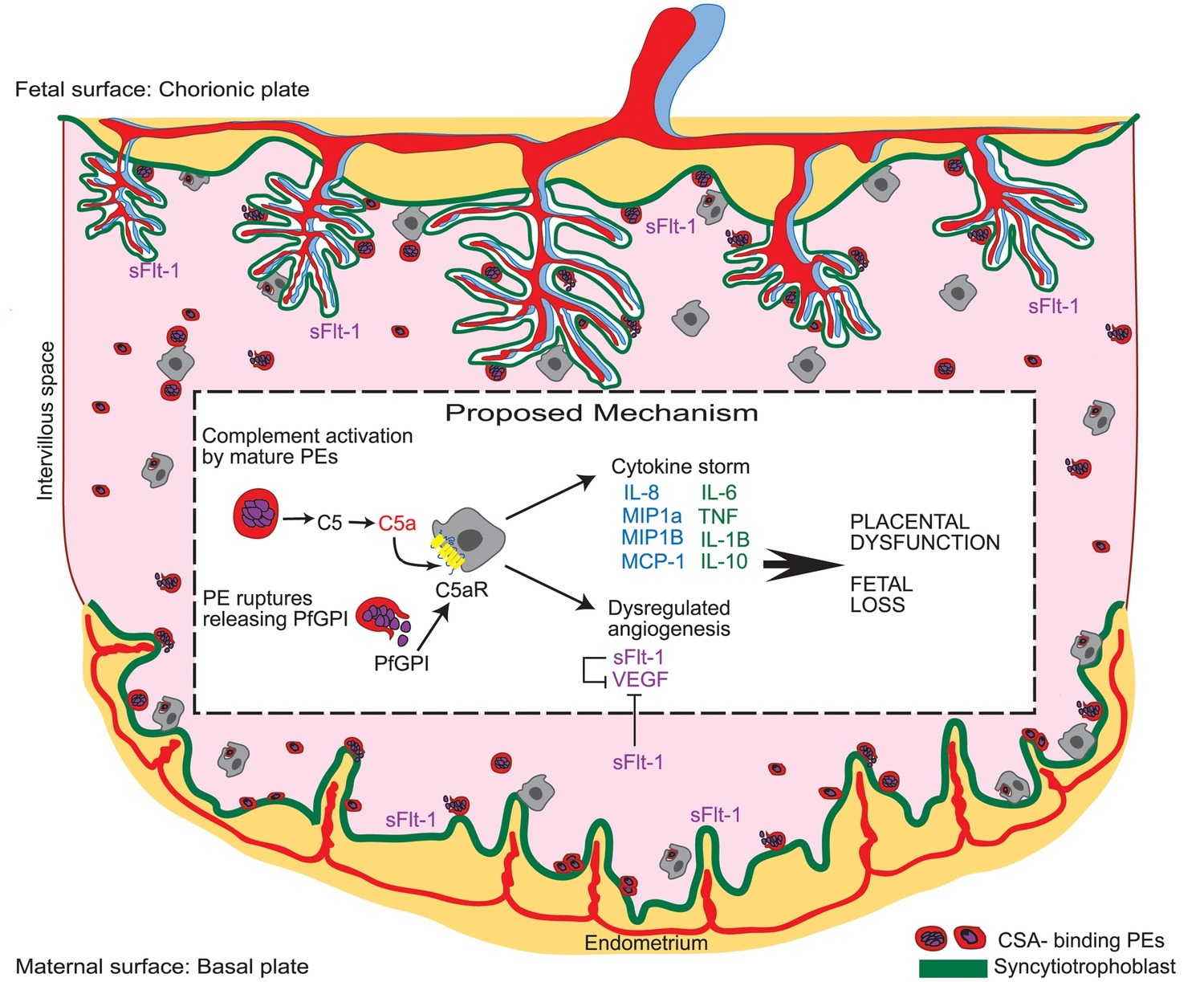
Figure 2. Malaria in pregnancy as a model for infection-driven dysregulation of placental vascular development as a common mechanism underlying adverse birth outcomes. During malaria in pregnancy, parasitized erythrocytes (PE) activate the maternal complement cascade. Complement component C5a acts synergistically with circulating parasite-associated bioactive molecules (i.e., Plasmodium falciparum glycosylphosphatidylinositol anchor molecules; PfGPI) to trigger an inflammatory response at the materno-fetal interface. This inflammatory host response dysregulates the tight balance of angiogenic factors required for placental vascular development leading to aberrant placental vascularization, placental dysfunction, and adverse birth outcomes. Existing evidence for other infections during pregnancy supports this model as a common mechanism underlying adverse birth outcomes in maternal infection [reproduced from Conroy et al., 2009].
Fetal Development is Impacted by Dysregulation of the Inflammatory-Angiogenic Axis in Maternal Infection
Many pathways critical to placental vascular development are mirrored in fetal vascular development. Normal vasculogenesis, angiogenesis, and materno-fetal hemodynamics are critical to organ growth in utero. Mounting evidence supports the hypothesis that disruption of these processes via maternal immune activation may cause defects in development of the fetal lungs, heart, and brain, with long-term consequences for offspring (Bilbo and Schwarz, 2009; McAdams et al., 2012; Burton and Jauniaux, 2018). The nature of the disruption to fetal development may depend on timing of infection. For example, studies suggest disruption to placental development and hemodynamics, especially in the first month of pregnancy, could be reflected in fetal cardiac abnormalities and congenital heart disease (Linask, 2013; Linask et al., 2014; Burton and Jauniaux, 2018). Dysregulation of inflammatory and angiogenic factors (i.e., via maternal infection) during a critical period of lung development is associated with bronchopulmonary dysplasia and increased neonatal mortality in the first 28 days of life (Thébaud and Abman, 2007; McAdams et al., 2012; Procianoy et al., 2015). Furthermore, a large and rapidly growing body of evidence has linked maternal immune activation and dysregulation of angiogenesis with impaired fetal neurodevelopment and long-lasting neurocognitive and neuropsychiatric sequelae for offspring (Knuesel et al., 2014; Estes and McAllister, 2016; Brown and Meyer, 2018).
Neurodevelopment is an intricate and strictly orchestrated process. Proper molecular signaling during critical gestational and postnatal periods is required for the establishment of effective neural networks (Stiles and Jernigan, 2010). Immune cells and cytokines such as microglia, complement, and IL-6 play an integral role in mediating these signals, and are tightly regulated at the materno-fetal interface (Billiards et al., 2006; Smith et al., 2007; Bilbo and Schwarz, 2009; Gallagher et al., 2013; McDonald et al., 2013; Graham et al., 2018; Prins et al., 2018; Rudolph et al., 2018; Spann et al., 2018; Rasmussen et al., 2019). In response to bacterial, viral, and parasitic infections, the maternal immune system is activated, exposing the fetus to cytokines and immune cells that are capable of passing through the immature fetal blood brain barrier and potentially impacting fetal neurodevelopment (Dammann and Leviton, 1997; Prins et al., 2018).
Evidence of the association between maternal immune activation and neuropsychiatric disorders, including schizophrenia (SZ), autism spectrum disorder (ASD) and bipolar disorder (BD), has accumulated in both epidemiological studies and preclinical models (Knuesel et al., 2014; Estes and McAllister, 2016; Brown and Meyer, 2018). Maternal immune activation via individual cytokines (e.g., IL-6 and IL-2), viral/bacterial mimics [e.g., polyinosinic:polycytidylic acid, poly(I:C); lipopolysaccharides, LPS], and actual infections (e.g., malaria, influenza, urinary tract infections) have been associated with long-term behavioral consequences for exposed offspring (Cai et al., 2000; Ponzio et al., 2007; Smith et al., 2007; Giovanoli et al., 2013; Knuesel et al., 2014; McDonald et al., 2015a; Choi et al., 2016; Brown and Meyer, 2018). Altered expression of proinflammatory mediators with dual roles in inflammation and neurodevelopment have been implicated in this link. Proinflammatory cytokine IL-6, which induced psychiatric behavioral outcomes in a seminal preclinical study of maternal immune activation (Smith et al., 2007), also has roles in neurogenesis, synapse formation, white matter development, and dendritic spine architecture (Wei et al., 2012; Gallagher et al., 2013; Rasmussen et al., 2019). Complement components including C5a, C3, and C1q also have well-characterized dual roles in maternal response to infection and neurodevelopment (i.e., synaptic pruning), and dysregulation of complement has been implicated in maternal infection-associated neurocognitive deficits in offspring (Stevens et al., 2007; Schafer et al., 2012; McDonald et al., 2015a; Weckman et al., 2018).
Furthermore, evidence points toward a direct connection and cross-talk between pathways critical to angiogenesis and neurodevelopment; proteins including VEGF family members have important roles in both processes (Carmeliet, 2003). Interestingly, VEGF participates in signaling required for the intricate growth and patterning of nerves and blood vessels alongside one another in the developing brain (Carmeliet, 2003). This indicates that disruption of the angiogenic environment (possibly downstream of maternal infection and inflammation) at the materno-fetal interface could also impact neurocognitive development with potential long-term neurological consequences for the offspring. In support of this contention, micro-CT images of malaria-exposed murine offspring showed alterations to fetal neurovasculature that was C5a-C5aR signaling-dependent (McDonald et al., 2015a). Since C5a activation seems to be upstream of angiogenic dysregulation in malaria in pregnancy (Figure 2; Conroy et al., 2009, 2013), these preclinical data support a model whereby maternal host response to infection in pregnancy could induce disruptions to fetal neurodevelopment via both angiogenic and inflammatory mechanisms.
Abnormal Placental Development as a Driver of Infection-Induced Adverse Birth Outcomes has Implications for Treatment Strategies
An increased understanding of the impact of maternal infections on placental vascular development has implications for new intervention strategies to reduce adverse birth outcomes. For example in maternal HIV infection, despite antiretroviral treatment women exhibited dysregulation of angiogenic factors (i.e., increased circulating soluble endoglin and decreased PlGF concentrations, resulting in an anti-angiogenic state) that were associated with PTB, SGA and stillbirth (Conroy et al., 2017). Women receiving antiretrovirals and cotrimoxazole that were co-infected with HIV and malaria exhibited systemic inflammation (i.e. increased soluble TNF receptor-2) that was also associated with PTB (McDonald et al., 2019). Persistent dysregulation of inflammatory and angiogenic pathways critical to placental vascular development could explain why rates of adverse birth outcomes remain high even in the face of appropriate antimicrobial therapy and indicate that alternative strategies, including modifying host response pathways, may be necessary to reduce poor birth outcomes.
Considering the early stage at which placental vasculature is established, the timing of infection during pregnancy has important implications for prevention/treatment strategies. Increasing evidence suggests that early infection with malaria in pregnancy increases the risk of adverse birth outcomes including SGA via disruption to placental development (Griffin et al., 2012; Moeller et al., 2018). Since current malaria drug-based prevention strategies are not implemented until the second trimester, this could represent an important gap in the prevention of infection-induced dysregulation of placental development and resulting adverse birth outcomes (Huynh et al., 2015). The use of combined antiretroviral therapy (cART) in pregnancy has also been associated with dysregulated angiogenesis, compromised placental vascular development, and adverse birth outcomes, especially when initiated early in pregnancy (Mohammadi et al., 2018). In pre-clinical studies, supplementing cART-treated mice with progesterone prevented placental abnormalities, indicating that this addition to current treatment strategies might improve pregnancy outcomes by targeting dysregulated placental vascular development. In support of this contention, a study in a large cohort of women in Papua New Guinea demonstrated the ability of sulphadoxine-pyrimethamine and azithromycin to improve adverse birth outcomes by regulating inflammatory (e.g., C-reactive protein) and angiogenic factors (e.g., soluble endoglin, sFlt-1) critical to placental vascular development (Unger et al., 2019).
Conclusions
Despite Millennium and Sustainable Development Goals to improve maternal-child health, the global burden of adverse birth outcomes remains high. This is in part due to a critical knowledge gap in our understanding of the mechanisms underlying adverse birth outcomes, and consequently limited or ineffective strategies. Several lines of evidence suggest that infection-driven dysregulation of inflammation and angiogenesis at the materno-fetal interface is a common mechanism underlying inadequate placental and fetal development, and adverse birth outcomes. Placental vascular development and vascular adaptation requires tight temporal and spatial regulation of cytokines, the complement system, and angiogenic factors including the VEGF and angiopoietin families. Dysregulation of those systems in the context of maternal infection leads to aberrations in spiral artery remodeling, placental vascularization and villous architecture, and deleterious materno-fetal hemodynamics that are associated with adverse birth outcomes. Current interventions for infections in pregnancy including malaria and HIV target only the pathogen and not the host response that may drive poor birth outcomes. This may explain, at least in part, why rates of adverse birth outcomes remain high. Further, dysregulation of inflammatory and angiogenic factors at the materno-fetal interface can lead to impairments in fetal neurodevelopment including neurogenesis and neurovascular development, with long term cognitive and behavioral sequelae for offspring. Considering the importance of the relationship between infection-induced dysregulation and adverse birth outcomes, future research should focus on therapeutics that target early placental development as a strategy to reduce the global burden of adverse birth outcomes.
Author Contributions
CM and KK managed project conception and oversight. AW, MN, JW, and CM contributed to research, analysis, and writing of the manuscript. All authors contributed to manuscript revisions and approved the final manuscript.
Funding
This study was supported by Canadian Institutes of Research (CIHR) Foundation grant FDN-148439 (KK); Canada Research Chair (KK); and CIHR Doctoral Award GSD-157907 (AW).
Conflict of Interest Statement
The authors declare that the research was conducted in the absence of any commercial or financial relationships that could be construed as a potential conflict of interest.
Abbreviations
Ang-1 and -2, Angiopoietin-1 and -2; cART, Combined antiretroviral therapy; CMV, Cytomegalovirus; dNK, Decidual natural killer cells; IL, Interleukin; INF, Interferon; LBW, Low birth weight; NO, Nitric oxide; PlGF, Placental growth factor; PTB, Preterm birth; sFlt-1, Soluble fms-like tyrosine kinase-1; SGA, Small-for-gestational age; TNF, Tumor necrosis factor; VEGF, Vascular endothelial growth factor.
References
Ahmed, A., Dunk, C., Kniss, D., and Wilkes, M. (1997). Role of VEGF receptor-1 (Flt-1) in mediating calcium-dependent nitric oxide release and limiting DNA synthesis in human trophoblast cells. Lab. Investig. 76, 779–791.
Ahmed, A., Li, X. F., Dunk, C., Whittle, M. J., Rushton, D. I., and Rollason, T. (1995). Colocalisation of vascular endothelial growth factor and its Flt-1 receptor in human placenta. Growth Factors 12, 235–243.
Ahmed, A., and Perkins, J. (2000). Angiogenesis and intrauterine growth restriction. Best Pract. Res. Clin. Obstet. Gynaecol. 14, 981–998. doi: 10.1053/beog.2000.0139
Ahmed, R., Singh, N., ter Kuile, F. O., Bharti, P. K., Singh, P. P., Desai, M., et al. (2014). Placental infections with histologically confirmed Plasmodium falciparum are associated with adverse birth outcomes in India: a cross-sectional study. Malar. J. 13:232. doi: 10.1186/1475-2875-13-232
Albieri, A., Kipnis, T., and Bevilacqua, E. (1999). A possible role for activated complement component 3 in phagocytic activity exhibited by the mouse trophoblast. Am. J. Reprod. Immunol. 41, 343–352. doi: 10.1111/j.1600-0897.1999.tb00448.x
Ataíde, R., Murillo, O., Dombrowski, J. G., Souza, R. M., Lima, F. A., Lima, G. F. M. C., et al. (2015). Malaria in pregnancy interacts with and alters the angiogenic profiles of the placenta. PLoS Negl. Trop. Dis. 9:e0003824. doi: 10.1371/journal.pntd.0003824
Athanassiades, A., Hamilton, G. S., and Lala, P. K. (1998). Vascular endothelial growth factor stimulates proliferation but not migration or invasiveness in human extravillous trophoblast1. Biol. Reprod. 59, 643–654. doi: 10.1095/biolreprod59.3.643
Athanassiades, A., and Lala, P. K. (1998). Role of placenta growth factor (PIGF) in human extravillous trophoblast proliferation, migration and invasiveness. Placenta 19, 465–473. doi: 10.1016/S0143-4004(98)91039-6
Benirschke, K., Burton, G. J., and Baergen, R. N. (eds.) (2012). “Architecture of normal villous trees” in Pathology of the human placenta (Berlin, Heidelberg: Springer), 101–144.
Bilbo, S. D., and Schwarz, J. M. (2009). Early-life programming of later-life brain and behavior: a critical role for the immune system. Front. Behav. Neurosci. 3:14. doi: 10.3389/neuro.08.014.2009
Billiards, S. S., Haynes, R. L., Folkerth, R. D., Trachtenberg, F. L., Liu, L. G., Volpe, J. J., et al. (2006). Development of microglia in the cerebral white matter of the human fetus and infant. J. Comp. Neurol. 497, 199–208. doi: 10.1002/cne.20991
Blencowe, H., Cousens, S., Oestergaard, M. Z., Chou, D., Moller, A., Narwal, R., et al. (2012). National, regional, and worldwide estimates of preterm birth rates in the year 2010 with time trends since 1990 for selected countries: a systematic analysis and implications. Lancet 379, 2162–2172. doi: 10.1016/S0140-6736(12)60820-4
Bowen, J. M., Chamley, L., Mitchell, M. D., and Keelan, J. A. (2002). Cytokines of the placenta and extra-placental membranes: biosynthesis, secretion and roles in establishment of pregnancy in women. Placenta 23, 239–256. doi: 10.1053/plac.2001.0781
Brown, A. S., and Meyer, U. (2018). Maternal immune activation and neuropsychiatric illness: a translational research perspective. Am. J. Psychiatry 175, 1073–1083. doi: 10.1176/appi.ajp.2018.17121311
Bulla, R., Agostinis, C., Bossi, F., Rizzi, L., Debeus, A., Tripodo, C., et al. (2008). Decidual endothelial cells express surface-bound C1q as a molecular bridge between endovascular trophoblast and decidual endothelium. Mol. Immunol. 45, 2629–2640. doi: 10.1016/j.molimm.2007.12.025
Burton, G. J., and Jauniaux, E. (2018). Development of the human placenta and fetal heart: synergic or independent? Front. Physiol. 9:373. doi: 10.3389/fphys.2018.00373
Cai, Z., Pan, Z. L., Pang, Y., Evans, O. B., and Rhodes, P. G. (2000). Cytokine induction in fetal rat brains and brain injury in neonatal rats after maternal lipopolysaccharide administration. Pediatr. Res. 47, 64–72. doi: 10.1203/00006450-200001000-00013
Calkins, K., and Devaskar, S. U. (2011). Fetal origins of adult disease. Curr. Probl. Pediatr. Adolesc. Health Care 41, 158–176. doi: 10.1016/j.cppeds.2011.01.001
Carmeliet, P. (2000). Mechanisms of angiogenesis and arteriogenesis. Nat. Med. 6, 389–395. doi: 10.1038/74651
Carmeliet, P. (2003). Blood vessels and nerves: common signals, pathways and diseases. Nat. Rev. Genet. 4, 710–720. doi: 10.1038/nrg1158
Carmeliet, P., Ferreira, V., Breier, G., Pollefeyt, S., Kieckens, L., Gertsenstein, M., et al. (1996). Abnormal blood vessel development and lethality in embryos lacking a single VEGF allele. Nature 380, 435–439. doi: 10.1038/380435a0
Carmona-Fonseca, J., Arango, E., and Maestre, A. (2013). Placental malaria in Colombia: histopathologic findings in Plasmodium vivax and P. falciparum infections. Am. J. Trop. Med. Hyg. 88, 1093–1101. doi: 10.4269/ajtmh.12-0363
Cérbulo-Vázquez, A., Figueroa-Damián, R., Arriaga-Pizano, L. A., Hernández-Andrade, E., Mancilla-Herrera, I., Flores-Mejía, L. A., et al. (2014). Pregnant women infected with pandemic H1N1pdm2009 influenza virus displayed overproduction of peripheral blood CD69+ lymphocytes and increased levels of serum cytokines. PLoS One 9:e107900. doi: 10.1371/journal.pone.0107900
Chaikitgosiyakul, S., Rijken, M. J., Muehlenbachs, A., Lee, S. J., Chaisri, U., Viriyavejakul, P., et al. (2014). A morphometric and histological study of placental malaria shows significant changes to villous architecture in both Plasmodium falciparum and Plasmodium vivax infection. Malar. J. 13:4. doi: 10.1186/1475-2875-13-4
Chaiworapongsa, T., Romero, R., Gotsch, F., Kusanovic, J. P., Mittal, P., Kim, S. K., et al. (2010). Acute pyelonephritis during pregnancy changes the balance of angiogenic and anti-angiogenic factors in maternal plasma. J. Matern. Fetal Neonatal Med. 23, 167–178. doi: 10.3109/14767050903067378
Chaiworapongsa, T., Romero, R., Tarca, A., Kusanovic, J. P., Mittal, P., Kim, S. K., et al. (2009). A subset of patients destined to develop spontaneous preterm labor has an abnormal angiogenic/anti-angiogenic profile in maternal plasma: evidence in support of pathophysiologic heterogeneity of preterm labor derived from a longitudinal study. J. Matern. Fetal Neonatal Med. 22, 1122–1139. doi: 10.3109/14767050902994838
Charnock-Jones, D. S., Kaufmann, P., and Mayhew, T. M. (2004). Aspects of human fetoplacental vasculogenesis and angiogenesis. I. Molecular regulation. Placenta 25, 103–113. doi: 10.1016/j.placenta.2003.10.004
Charnock-Jones, D. S., Sharkey, A. M., Boocock, C. A., Ahmed, A., Plevin, R., Ferrara, N., et al. (1994). Vascular endothelial growth factor receptor localization and activation in human trophoblast and choriocarcinoma cells. Biol. Reprod. 51, 524–530. doi: 10.1095/biolreprod51.3.524
Chico, R. M., Chaponda, E. B., Ariti, C., and Chandramohan, D. (2017). Sulfadoxine-pyrimethamine exhibits dose-response protection against adverse birth outcomes related to malaria and sexually transmitted and reproductive tract infections. Clin. Infect. Dis. 64, 1043–1051. doi: 10.1093/cid/cix026
Choi, S. J., Park, J. Y., Lee, Y. K., Choi, H. I., Lee, Y. S., Koh, C.-M., et al. (2002). Effects of cytokines on VEGF expression and secretion by human first trimester trophoblast cell line. Am. J. Reprod. Immunol. 48, 70–76. doi: 10.1034/j.1600-0897.2002.01071.x
Choi, G. B., Yim, Y. S., Wong, H., Kim, S., Kim, H., Kim, S. V., et al. (2016). The maternal interleukin-17a pathway in mice promotes autism-like phenotypes in offspring. Science 351, 933–939. doi: 10.1126/science.aad0314
Clark, D. E., Smith, S. K., Sharkey, A. M., and Charnock-Jones, D. S. (1996). Localization of VEGF and expression of its receptors flit and KDR in human placenta throughout pregnancy. Hum. Reprod. 11, 1090–1098. doi: 10.1093/oxfordjournals.humrep.a019303
Conroy, A. L., McDonald, C. R., Gamble, J. L., Olwoch, P., Natureeba, P., Cohan, D., et al. (2017). Altered angiogenesis as a common mechanism underlying preterm birth, small for gestational age, and stillbirth in women living with HIV. Am. J. Obstet. Gynecol. 217, 684.e1–684.e17. doi: 10.1016/j.ajog.2017.10.003
Conroy, A., Serghides, L., Finney, C., Owino, S. O., Kumar, S., Gowda, D. C., et al. (2009). C5a enhances dysregulated inflammatory and angiogenic responses to malaria in vitro: potential implications for placental malaria. PLoS One 4, 1–10. doi: 10.1371/journal.pone.0004953
Conroy, A. L., Silver, K. L., Zhong, K., Rennie, M., Ward, P., Sarma, J. V., et al. (2013). Complement activation and the resulting placental vascular insufficiency drives fetal growth restriction associated with placental malaria. Cell Host Microbe 13, 215–226. doi: 10.1016/j.chom.2013.01.010
Coyne, C. B., and Lazear, H. M. (2016). Zika virus – reigniting the TORCH. Nat. Rev. Microbiol. 14, 707–715. doi: 10.1038/nrmicro.2016.125
Dammann, O., and Leviton, A. (1997). Maternal intrauterine infection, cytokines, and brain damage in the preterm newborn. Pediatr. Res. 42, 1–8. doi: 10.1203/00006450-199707000-00001
Darling, A. M., McDonald, C. R., Conroy, A. L., Hayford, K. T., Liles, W. C., Wang, M., et al. (2014). Angiogenic and inflammatory biomarkers in midpregnancy and small-for-gestational-age outcomes in Tanzania. Am. J. Obstet. Gynecol. 211, 509.e1–509.e8. doi: 10.1016/j.ajog.2014.05.032
Demir, R., Kayisli, U. A., Cayli, S., and Huppertz, B. (2006). Sequential steps during vasculogenesis and angiogenesis in the very early human placenta. Placenta 27, 535–539. doi: 10.1016/j.placenta.2005.05.011
Demir, R., Seval, Y., and Huppertz, B. (2007). Vasculogenesis and angiogenesis in the early human placenta. Acta Histochem. 109, 257–265. doi: 10.1016/j.acthis.2007.02.008
Desai, M., ter Kuile, F. O., Nosten, F., McGready, R., Asamoa, K., Brabin, B., et al. (2007). Epidemiology and burden of malaria in pregnancy. Lancet Infect. Dis. 7, 93–104. doi: 10.1016/S1473-3099(07)70021-X
Di Simone, N., Tersigni, C., Cardaropoli, S., Franceschi, F., Di Nicuolo, F., Castellani, R., et al. (2017). Helicobacter pylori infection contributes to placental impairment in preeclampsia: basic and clinical evidences. Helicobacter 22:e12347. doi: 10.1111/hel.12347
Dorman, E. K., Shulman, C. E., Kingdom, J., Bulmer, J. N., Mwendwa, J., Peshu, N., et al. (2002). Impaired uteroplacental blood flow in pregnancies complicated by falciparum malaria. Ultrasound Obstet. Gynecol. 19, 165–170. doi: 10.1046/j.0960-7692.2001.00545.x
Dunk, C., Shams, M., Nijjar, S., Rhaman, M., Qiu, Y., Bussolati, B., et al. (2000). Angiopoietin-1 and Angiopoietin-2 activate trophoblast Tie-2 to promote growth and migration during placental development. Am. J. Pathol. 156, 2185–2199. doi: 10.1016/S0002-9440(10)65089-4
Estes, M. L., and McAllister, A. K. (2016). Maternal immune activation: implications for neuropsychiatric disorders. Science 353, 772–777. doi: 10.1126/science.aag3194
Ferrazzi, E., Rigano, S., Bozzo, M., Bellotti, M., Giovannini, N., Galan, H., et al. (2000). Umbilical vein blood flow in growth-restricted fetuses. Ultrasound Obstet. Gynecol. 16, 432–438. doi: 10.1046/j.1469-0705.2000.00208.x
Fiedler, U., and Augustin, H. G. (2006). Angiopoietins: a link between angiogenesis and inflammation. Trends Immunol. 27, 552–558. doi: 10.1016/j.it.2006.10.004
Fisher, S. J. (2015). Why is placentation abnormal in preeclampsia? Am. J. Obstet. Gynecol. 213, S115–S122. doi: 10.1016/j.ajog.2015.08.042
Fried, M., Kurtis, J. D., Swihart, B., Pond-Tor, S., Barry, A., Sidibe, Y., et al. (2017). Systemic inflammatory response to malaria during pregnancy is associated with pregnancy loss and preterm delivery. Clin. Infect. Dis. 65, 1729–1735. doi: 10.1093/cid/cix623
Gagnon, R. (2003). Placental insufficiency and its consequences. Eur. J. Obstet. Gynecol. Reprod. Biol. 110, S99–S107. doi: 10.1016/S0301-2115(03)00179-9
Gallagher, D., Norman, A. A., Woodard, C. L., Yang, G., Fujitani, M., Vessey, J. P., et al. (2013). Transient maternal IL-6 mediates long-lasting changes in neural stem cell pools by deregulating an endogenous self-renewal pathway. Cell Stem Cell 13, 564–576. doi: 10.1016/j.stem.2013.10.002
Geva, E., Ginzinger, D. G., Zaloudek, C. J., Moore, D. H., Byrne, A., and Jaffe, R. B. (2002). Human placental vascular development: vasculogenic and angiogenic (branching and nonbranching) transformation is regulated by vascular endothelial growth factor-A, angiopoietin-1, and angiopoietin-2. J. Clin. Endocrinol. Metab. 87, 4213–4224. doi: 10.1210/jc.2002-020195
Giovanoli, S., Engler, H., Engler, A., Richetto, J., Voget, M., Willi, R., et al. (2013). Stress in puberty unmasks latent neuropathological consequences of prenatal immune activation in mice. Science 339, 1095–1099. doi: 10.1126/science.1228261
Girardi, G., Yarilin, D., Thurman, J. M., Holers, V. M., and Salmon, J. E. (2006). Complement activation induces dysregulation of angiogenic factors and causes fetal rejection and growth restriction. J. Exp. Med. 203, 2165–2175. doi: 10.1084/jem.20061022
Goldman-Wohl, D. S., Ariel, I., Greenfield, C., Lavy, Y., and Yagel, S. (2000). Tie-2 and angiopoietin-2 expression at the fetal-maternal interface: a receptor ligand model for vascular remodelling. Mol. Hum. Reprod. 6, 81–87. doi: 10.1093/molehr/6.1.81
Graham, A. M., Rasmussen, J. M., Rudolph, M. D., Heim, C. M., Gilmore, J. H., Styner, M., et al. (2018). Maternal systemic interleukin-6 during pregnancy is associated with newborn amygdala phenotypes and subsequent behavior at 2 years of age. Biol. Psychiatry 83, 109–119. doi: 10.1016/j.biopsych.2017.05.027
Griffin, J. B., Lokomba, V., Landis, S. H., Thorp, J. M., Herring, A. H., Tshefu, A. K., et al. (2012). Plasmodium falciparum parasitaemia in the first half of pregnancy, uterine and umbilical artery blood flow, and foetal growth: a longitudinal Doppler ultrasound study. Malar. J. 11:319. doi: 10.1186/1475-2875-11-319
Gustafsson, R. K. L., Jeffery, H. C., Yaiw, K.-C., Wilhelmi, V., Kostopoulou, O. N., Davoudi, B., et al. (2015). Direct infection of primary endothelial cells with human cytomegalovirus prevents angiogenesis and migration. J. Gen. Virol. 96, 3598–3612. doi: 10.1099/jgv.0.000301
Hanna, J., Goldman-Wohl, D., Hamani, Y., Avraham, I., Greenfield, C., Natanson-Yaron, S., et al. (2006). Decidual NK cells regulate key developmental processes at the human fetal-maternal interface. Nat. Med. 12, 1065–1074. doi: 10.1038/nm1452
Harjunmaa, U., Doyle, R., Järnstedt, J., Kamiza, S., Jorgensen, J., Stewart, C., et al. (2018). Periapical infection may affect birth outcomes via systemic inflammation. Oral Dis. 24, 847–855. doi: 10.1111/odi.12817
Hernandez-Andrade, E., Figueroa, R., Cerbulo-Vazquez, A., Benavides-Serralde, J. A., Borbón, G. P., and Ramírez, J. M. (2014). Fetal hemodynamic changes in pregnant women with influenza AH1N1 infection and reduced arterial partial pressure of oxygen. Ultrasound Obstet. Gynecol. 44, 492–494. doi: 10.1002/uog.13417
Hirsch, A. J., Roberts, V. H. J., Grigsby, P. L., Haese, N., Schabel, M. C., Wang, X., et al. (2018). Zika virus infection in pregnant rhesus macaques causes placental dysfunction and immunopathology. Nat. Commun. 9:263. doi: 10.1038/s41467-017-02499-9
Horton, A. L., Boggess, K. A., Moss, K. L., Jared, H. L., Beck, J., and Offenbacher, S. (2008). Periodontal disease early in pregnancy is associated with maternal systemic inflammation among African American women. J. Periodontol. 79, 1127–1132. doi: 10.1902/jop.2008.070655
Hustin, J., Jauniaux, E., and Schaaps, J. P. (1990). Histological study of the materno-embryonic interface in spontaneous abortion. Placenta 11, 477–486. doi: 10.1016/S0143-4004(05)80193-6
Huynh, B.-T., Cottrell, G., Cot, M., and Briand, V. (2015). Burden of malaria in early pregnancy: a neglected problem? Clin. Infect. Dis. 60, 598–604. doi: 10.1093/cid/ciu848
Jackson, M. R., Walsh, A. J., Morrow, R. J., Mullen, J. B., Lye, S. J., and Ritchie, J. W. (1995). Reduced placental villous tree elaboration in small-for-gestational-age pregnancies: relationship with umbilical artery Doppler waveforms. Am. J. Obstet. Gynecol. 172, 518–525. doi: 10.1016/0002-9378(95)90566-9
Kalk, E., Schubert, P., Bettinger, J. A., Cotton, M. F., Esser, M., Slogrove, A., et al. (2017). Placental pathology in HIV infection at term: a comparison with HIV-uninfected women. Tropical Med. Int. Health 22, 604–613. doi: 10.1111/tmi.12858
Kaufmann, P., Black, S., and Huppertz, B. (2003). Endovascular trophoblast invasion: implications for the pathogenesis of intrauterine growth retardation and preeclampsia. Biol. Reprod. 69, 1–7. doi: 10.1095/biolreprod.102.014977
Kaufmann, P., Mayhew, T. M., and Charnock-Jones, D. S. (2004). Aspects of human fetoplacental vasculogenesis and angiogenesis. II. Changes during normal pregnancy. Placenta 25, 114–126. doi: 10.1016/j.placenta.2003.10.009
Khaliq, A., Dunk, C., Jiang, J., Shams, M., Li, X. F., Acevedo, C., et al. (1999). Hypoxia down-regulates placenta growth factor, whereas fetal growth restriction up-regulates placenta growth factor expression: molecular evidence for “placental hyperoxia” in intrauterine growth restriction. Lab. Investig. 79, 151–170.
Khaliq, A., Li, X. F., Shams, M., Sisi, P., Acevedo, C. A., Whittle, M. J., et al. (1996). Localisation of placenta growth factor (PIGF) in human term placenta. Growth Factors 13, 243–250.
Khong, T. Y., De Wolf, F., Robertson, W. B., and Brosens, I. (1986). Inadequate maternal vascular response to placentation in pregnancies complicated by pre-eclampsia and by small-for-gestational age infants. Br. J. Obstet. Gynaecol. 93, 1049–1059.
Kim, C. J., Romero, R., Chaemsaithong, P., and Kim, J.-S. (2015). Chronic inflammation of the placenta: definition, classification, pathogenesis, and clinical significance. Am. J. Obstet. Gynecol. 213, S53–S69. doi: 10.1016/j.ajog.2015.08.041
Kingdom, J. (1998). Adriana and Luisa Castellucci Award Lecture 1997. Placental pathology in obstetrics: adaptation or failure of the villous tree? Placenta 19, 347–351. doi: 10.1016/S0143-4004(98)90073-X
Kingdom, J., Huppertz, B., Seaward, G., and Kaufmann, P. (2000). Development of the placental villous tree and its consequences for fetal growth. Eur. J. Obstet. Gynecol. Reprod. Biol. 92, 35–43. doi: 10.1016/S0301-2115(00)00423-1
Kingdom, J. C., and Kaufmann, P. (1997). Oxygen and placental villous development: origins of fetal hypoxia. Placenta 18, 613–621; discussion 623–626. doi: 10.1016/S0143-4004(97)90000-X
Knuesel, I., Chicha, L., Britschgi, M., Schobel, S. A., Bodmer, M., Hellings, J. A., et al. (2014). Maternal immune activation and abnormal brain development across CNS disorders. Nat. Rev. Neurol. 10, 643–660. doi: 10.1038/nrneurol.2014.187
Kraus, T. A., Sperling, R. S., Engel, S. M., Lo, Y., Kellerman, L., Singh, T., et al. (2010). Peripheral blood cytokine profiling during pregnancy and post-partum periods. Am. J. Reprod. Immunol. 64, 411–426. doi: 10.1111/j.1600-0897.2010.00889.x
Le Bouteiller, P. (2013). Human decidual NK cells: unique and tightly regulated effector functions in healthy and pathogen-infected pregnancies. Front. Immunol. 4:404. doi: 10.3389/fimmu.2013.00404
Lee, A. C., Katz, J., Blencowe, H., Cousens, S., Kozuki, N., Vogel, J. P., et al. (2013). National and regional estimates of term and preterm babies born small for gestational age in 138 low-income and middle-income countries in 2010. Lancet Glob. Health 1, e26–e36. doi: 10.1016/S2214-109X(13)70006-8
Levine, R. J., Lam, C., Qian, C., Yu, K. F., Maynard, S. E., Sachs, B. P., et al. (2006). Soluble endoglin and other circulating antiangiogenic factors in preeclampsia. N. Engl. J. Med. 355, 992–1005. doi: 10.1056/NEJMoa055352
Levine, R. J., Maynard, S. E., Qian, C., Lim, K.-H., England, L. J., Yu, K. F., et al. (2004). Circulating angiogenic factors and the risk of preeclampsia. N. Engl. J. Med. 350, 672–683. doi: 10.1056/NEJMoa031884
Linask, K. K. (2013). The heart-placenta axis in the first month of pregnancy: induction and prevention of cardiovascular birth defects. J. Pregnancy 2013:320413. doi: 10.1155/2013/320413
Linask, K. K., Han, M., and Bravo-Valenzuela, N. J. M. (2014). Changes in vitelline and utero-placental hemodynamics: implications for cardiovascular development. Front. Physiol. 5. doi: 10.3389/fphys.2014.00390
Liu, L., Oza, S., Hogan, D., Chu, Y., Perin, J., Zhu, J., et al. (2016). Global, regional, and national causes of under-5 mortality in 2000-15: an updated systematic analysis with implications for the sustainable development goals. Lancet 388, 3027–3035. doi: 10.1016/S0140-6736(16)31593-8
Madanitsa, M., Kalilani, L., Mwapasa, V., van Eijk, A. M., Khairallah, C., Ali, D., et al. (2016). Scheduled intermittent screening with rapid diagnostic tests and treatment with dihydroartemisinin-piperaquine versus intermittent preventive therapy with sulfadoxine-pyrimethamine for malaria in pregnancy in Malawi: an open-label randomized controlled trial. PLoS Med. 13:e1002124. doi: 10.1371/journal.pmed.1002124
Maisonpierre, P. C., Suri, C., Jones, P. F., Bartunkova, S., Wiegand, S. J., Radziejewski, C., et al. (1997). Angiopoietin-2, a natural antagonist for Tie2 that disrupts in vivo angiogenesis. Science 277, 55–60. doi: 10.1126/science.277.5322.55
McAdams, R. M., Vanderhoeven, J., Beyer, R. P., Bammler, T. K., Farin, F. M., Liggitt, H. D., et al. (2012). Choriodecidual infection downregulates angiogenesis and morphogenesis pathways in fetal lungs from Macaca nemestrina. PLoS One 7:e46863. doi: 10.1371/journal.pone.0046863
McClure, E. M., Meshnick, S. R., Lazebnik, N., Mungai, P., King, C. L., Hudgens, M., et al. (2014). A cohort study of Plasmodium falciparum malaria in pregnancy and associations with uteroplacental blood flow and fetal anthropometrics in Kenya. Int. J. Gynecol. Obstet. 126, 78–82. doi: 10.1016/j.ijgo.2014.01.016
McDonald, C. R., Cahill, L. S., Gamble, J. L., Elphinstone, R., Gazdzinski, L. M., Zhong, K. J. Y., et al. (2018). Malaria in pregnancy alters l-arginine bioavailability and placental vascular development. Sci. Transl. Med. 10:eaan6007. doi: 10.1126/scitranslmed.aan6007
McDonald, C. R., Cahill, L. S., Ho, K. T., Yang, J., Kim, H., Silver, K. L., et al. (2015a). Experimental malaria in pregnancy induces neurocognitive injury in uninfected offspring via a C5a-C5a receptor dependent pathway. PLoS Pathog. 11:e1005140. doi: 10.1371/journal.ppat.1005140
McDonald, C. R., Darling, A. M., Conroy, A. L., Tran, V., Cabrera, A., Liles, W. C., et al. (2015b). Inflammatory and angiogenic factors at mid-pregnancy are associated with spontaneous preterm birth in a cohort of Tanzanian women. PLoS One 10:e0134619. doi: 10.1371/journal.pone.0134619
McDonald, C. R., Elphinstone, R. E., and Kain, K. C. (2013). The impact of placental malaria on neurodevelopment of exposed infants: a role for the complement system? Trends Parasitol. 29, 213–219. doi: 10.1016/j.pt.2013.03.005
McDonald, C. R., Weckman, A. M., Conroy, A. L., Olwoch, P., Natureeba, P., Kamya, M. R., et al. (2019). Systemic inflammation is associated with malaria and preterm birth in women living with HIV on antiretrovirals and co-trimoxazole. Sci. Rep. 9:6758. doi: 10.1038/s41598-019-43191-w
Miquerol, L., Langille, B. L., and Nagy, A. (2000). Embryonic development is disrupted by modest increases in vascular endothelial growth factor gene expression. Development 127, 3941–3946.
Moeller, S. L., Nyengaard, J. R., Larsen, L. G., Nielsen, K., Bygbjerg, I. C., Msemo, O. A., et al. (2018). Malaria in early pregnancy and the development of the placental vasculature. J. Infect. Dis. jiy735. doi: 10.1093/infdis/jiy735
Mohammadi, H., Papp, E., Cahill, L., Rennie, M., Banko, N., Pinnaduwage, L., et al. (2018). HIV antiretroviral exposure in pregnancy induces detrimental placenta vascular changes that are rescued by progesterone supplementation. Sci. Rep. 8:6552. doi: 10.1038/s41598-018-24680-w
Morgan, T. K. (2016). Role of the placenta in preterm birth: a review. Am. J. Perinatol. 33, 258–266. doi: 10.1055/s-0035-1570379
Murray, C. J. L., Vos, T., Lozano, R., Naghavi, M., Flaxman, A. D., Michaud, C., et al. (2012). Disability-adjusted life years (DALYs) for 291 diseases and injuries in 21 regions, 1990-2010: a systematic analysis for the global burden of disease study 2010. Lancet 380, 2197–2223. doi: 10.1016/S0140-6736(12)61689-4
Naldini, A., and Carraro, F. (2005). Role of inflammatory mediators in angiogenesis. Curr. Drug Targets Inflamm. Allergy 4, 3–8. doi: 10.2174/1568010053622830
Ome-Kaius, M., Karl, S., Wangnapi, R. A., Bolnga, J. W., Mola, G., Walker, J., et al. (2017). Effects of Plasmodium falciparum infection on umbilical artery resistance and intrafetal blood flow distribution: a Doppler ultrasound study from Papua New Guinea. Malar. J. 16:35. doi: 10.1186/s12936-017-1689-z
Phillips, P., Brown, M. B., Progulske-Fox, A., Wu, X.-J., and Reyes, L. (2018). Porphyromonas gingivalis strain-dependent inhibition of uterine spiral artery remodeling in the pregnant rat. Biol. Reprod. 99, 1045–1056. doi: 10.1093/biolre/ioy119
Pijnenborg, R., Anthony, J., Davey, D. A., Rees, A., Tiltman, A., Vercruysse, L., et al. (1991). Placental bed spiral arteries in the hypertensive disorders of pregnancy. Br. J. Obstet. Gynaecol. 98, 648–655.
Pollheimer, J., Vondra, S., Baltayeva, J., Beristain, A. G., and Knöfler, M. (2018). Regulation of placental extravillous trophoblasts by the maternal uterine environment. Front. Immunol. 9:2597. doi: 10.3389/fimmu.2018.02597
Ponzio, N. M., Servatius, R., Beck, K., Marzouk, A., and Kreider, T. (2007). Cytokine levels during pregnancy influence immunological profiles and neurobehavioral patterns of the offspring. Ann. N. Y. Acad. Sci. 1107, 118–128. doi: 10.1196/annals.1381.013
Prins, J. R., Eskandar, S., Eggen, B. J. L., and Scherjon, S. A. (2018). Microglia, the missing link in maternal immune activation and fetal neurodevelopment; and a possible link in preeclampsia and disturbed neurodevelopment? J. Reprod. Immunol. 126, 18–22. doi: 10.1016/j.jri.2018.01.004
Procianoy, R., Hentges, C., and Silveira, R. (2015). Vascular endothelial growth factor/placental growth factor heterodimer levels in preterm infants with bronchopulmonary dysplasia. Am. J. Perinatol. 33, 480–485. doi: 10.1055/s-0035-1566294
Rasmussen, J. M., Graham, A. M., Entringer, S., Gilmore, J. H., Styner, M., Fair, D. A., et al. (2019). Maternal interleukin-6 concentration during pregnancy is associated with variation in frontolimbic white matter and cognitive development in early life. NeuroImage 185, 825–835. doi: 10.1016/j.neuroimage.2018.04.020
Regal, J. F., Gilbert, J. S., and Burwick, R. M. (2015). The complement system and adverse pregnancy outcomes. Mol. Immunol. 67, 56–70. doi: 10.1016/j.molimm.2015.02.030
Ribeiro, C. F., Lopes, V. G. S., Brasil, P., Pires, A. R. C., Rohloff, R., and Nogueira, R. M. R. (2017). Dengue infection in pregnancy and its impact on the placenta. Int. J. Infect. Dis. 55, 109–112. doi: 10.1016/j.ijid.2017.01.002
Rogerson, S. J., Desai, M., Mayor, A., Sicuri, E., Taylor, S. M., and van Eijk, A. M. (2018). Burden, pathology, and costs of malaria in pregnancy: new developments for an old problem. Lancet Infect. Dis. 18, e107–e118. doi: 10.1016/S1473-3099(18)30066-5
Romero, R., Chaemsaithong, P., Docheva, N., Korzeniewski, S. J., Tarca, A. L., Bhatti, G., et al. (2016). Clinical chorioamnionitis at term IV: the maternal plasma cytokine profile. J. Perinat. Med. 44, 77–98. doi: 10.1515/jpm-2015-0103
Romero, R., Chaiworapongsa, T., Erez, O., Tarca, A. L., Gervasi, M. T., Kusanovic, J. P., et al. (2010). An imbalance between angiogenic and anti-angiogenic factors precedes fetal death in a subset of patients: results of a longitudinal study. J. Matern. Fetal Neonatal Med. 23, 1384–1399. doi: 10.3109/14767051003681121
Romero, R., Kusanovic, J. P., Chaiworapongsa, T., and Hassan, S. S. (2011). Placental bed disorders in preterm labor, preterm PROM, spontaneous abortion and abruptio placentae. Best Pract. Res. Clin. Obstet. Gynaecol. 25, 313–327. doi: 10.1016/j.bpobgyn.2011.02.006
Rudolph, M. D., Graham, A. M., Feczko, E., Miranda-Dominguez, O., Rasmussen, J. M., Nardos, R., et al. (2018). Maternal IL-6 during pregnancy can be estimated from newborn brain connectivity and predicts future working memory in offspring. Nat. Neurosci. 21, 765–772. doi: 10.1038/s41593-018-0128-y
Salafia, C., Pezzullo, J., Minior, V., and Divon, M. (1997). Placental pathology of absent and reversed end-diastolic flow in growth-restricted fetuses. Obstet. Gynecol. 90, 830–836. doi: 10.1016/S0029-7844(97)00473-0
Santosa, W. B., Staines-Urias, E., Tshivuila-Matala, C. O. O., Norris, S. A., and Hemelaar, J. (2019). Perinatal outcomes associated with maternal HIV and antiretroviral therapy in pregnancies with accurate gestational age in South Africa. AIDS 33, 1623–1633. doi: 10.1097/QAD.0000000000002222
Schafer, D. P., Lehrman, E. K., Kautzman, A. G., Koyama, R., Mardinly, A. R., Yamasaki, R., et al. (2012). Microglia sculpt postnatal neural circuits in an activity and complement-dependent manner. Neuron 74, 691–705. doi: 10.1016/j.neuron.2012.03.026
Seval, Y., Sati, L., Celik-Ozenci, C., Taskin, O., and Demir, R. (2008). The distribution of angiopoietin-1, angiopoietin-2 and their receptors tie-1 and tie-2 in the very early human placenta. Placenta 29, 809–815. doi: 10.1016/j.placenta.2008.06.009
Sharkey, A. M., Cooper, J. C., Balmforth, J. R., McLaren, J., Clark, D. E., Charnock-Jones, D. S., et al. (1996). Maternal plasma levels of vascular endothelial growth factor in normotensive pregnancies and in pregnancies complicated by pre-eclampsia. Eur. J. Clin. Investig. 26, 1182–1185. doi: 10.1046/j.1365-2362.1996.830605.x
Silver, R. M. (2018). Examining the link between placental pathology, growth restriction, and stillbirth. Best Pract. Res. Clin. Obstet. Gynaecol. 49, 89–102. doi: 10.1016/j.bpobgyn.2018.03.004
Silver, K. L., Conroy, A. L., Leke, R. G. F., Leke, R. J. I., Gwanmesia, P., Molyneux, M. E., et al. (2011). Circulating soluble endoglin levels in pregnant women in Cameroon and Malawi – associations with placental malaria and fetal growth restriction. PLoS One 6:e24985. doi: 10.1371/annotation/e9946f72-821d-45ea-bcd4-3fa3ff89a5fe
Silver, K. L., Zhong, K., Leke, R. G. F., Taylor, D. W., and Kain, K. C. (2010). Dysregulation of angiopoietins is associated with placental malaria and low birth weight. PLoS One 5:e9481. doi: 10.1371/journal.pone.0009481
Smith, S. E. P., Li, J., Garbett, K., Mirnics, K., and Patterson, P. H. (2007). Maternal immune activation alters fetal brain development through interleukin-6. J. Neurosci. 27, 10695–10702. doi: 10.1523/JNEUROSCI.2178-07.2007
Spann, M. N., Monk, C., Scheinost, D., and Peterson, B. S. (2018). Maternal immune activation during the third trimester is associated with neonatal functional connectivity of the salience network and fetal to toddler behavior. J. Neurosci. 38, 2877–2886. doi: 10.1523/JNEUROSCI.2272-17.2018
Stevens, B., Allen, N. J., Vazquez, L. E., Howell, G. R., Christopherson, K. S., Nouri, N., et al. (2007). The classical complement cascade mediates CNS synapse elimination. Cell 131, 1164–1178. doi: 10.1016/j.cell.2007.10.036
Stiles, J., and Jernigan, T. L. (2010). The basics of brain development. Neuropsychol. Rev. 20, 327–348. doi: 10.1007/s11065-010-9148-4
Straughen, J. K., Misra, D. P., Helmkamp, L., and Misra, V. K. (2017). Preterm delivery as a unique pathophysiologic state characterized by maternal soluble FMS-like tyrosine kinase 1 and uterine artery resistance during pregnancy: a longitudinal cohort study. Reprod. Sci. 24, 1583–1589. doi: 10.1177/1933719117698574
Suri, C., Jones, P. F., Patan, S., Bartunkova, S., Maisonpierre, P. C., Davis, S., et al. (1996). Requisite role of angiopoietin-1, a ligand for the TIE2 receptor, during embryonic angiogenesis. Cell 87, 1171–1180. doi: 10.1016/S0092-8674(00)81813-9
Tabata, T., Petitt, M., Fang-Hoover, J., Rivera, J., Nozawa, N., Shiboski, S., et al. (2012). Cytomegalovirus impairs cytotrophoblast-induced lymphangiogenesis and vascular remodeling in an in vivo human placentation model. Am. J. Pathol. 181, 1540–1559. doi: 10.1016/j.ajpath.2012.08.003
Thébaud, B., and Abman, S. H. (2007). Bronchopulmonary dysplasia: where have all the vessels gone? Roles of angiogenic growth factors in chronic lung disease. Am. J. Respir. Crit. Care Med. 175, 978–985. doi: 10.1164/rccm.200611-1660PP
Travaglino, A., Raffone, A., Saccone, G., Migliorini, S., Maruotti, G. M., Esposito, G., et al. (2019). Placental morphology, apoptosis, angiogenesis and epithelial mechanisms in early-onset preeclampsia. Eur. J. Obstet. Gynecol. Reprod. Biol. 234, 200–206. doi: 10.1016/j.ejogrb.2018.12.039
Trudinger, B. J., Giles, W. B., Cook, C. M., Bombardieri, J., and Collins, L. (1985). Fetal umbilical artery flow velocity waveforms and placental resistance: clinical significance. Br. J. Obstet. Gynaecol. 92, 23–30.
Unger, H. W., Hansa, A. P., Buffet, C., Hasang, W., Teo, A., Randall, L., et al. (2019). Sulphadoxine-pyrimethamine plus azithromycin may improve birth outcomes through impacts on inflammation and placental angiogenesis independent of malarial infection. Sci. Rep. 9:2260. doi: 10.1038/s41598-019-38821-2
Vedmedovska, N., Rezeberga, D., Teibe, U., Melderis, I., and Donders, G. G. G. (2011). Placental pathology in fetal growth restriction. Eur. J. Obstet. Gynecol. Reprod. Biol. 155, 36–40. doi: 10.1016/j.ejogrb.2010.11.017
Venkatesha, S., Toporsian, M., Lam, C., Hanai, J., Mammoto, T., Kim, Y. M., et al. (2006). Soluble endoglin contributes to the pathogenesis of preeclampsia. Nat. Med. 12, 642–649. doi: 10.1038/nm1429
Vuorela, P., Hatva, E., Lymboussaki, A., Kaipainen, A., Joukov, V., Persico, M. G., et al. (1997). Expression of vascular endothelial growth factor and placenta growth factor in human placenta1. Biol. Reprod. 56, 489–494. doi: 10.1095/biolreprod56.2.489
Wang, Y., and Zhao, S. (2010). Vascular biology of the placenta. Morgan & Claypool Life Sciences. Available at: http://www.ncbi.nlm.nih.gov/pubmed/21452443 (Accessed January 16, 2019).
Watson-Jones, D., Changalucha, J., Gumodoka, B., Weiss, H., Rusizoka, M., Ndeki, L., et al. (2002). Syphilis in pregnancy in Tanzania. I. Impact of maternal syphilis on outcome of pregnancy. J. Infect. Dis. 186, 940–947. doi: 10.1086/342952
Weckman, A., Tran, V., and Kain, K. C. (2018). “Complement and malaria in pregnancy” in Complement activation in malaria immunity and pathogenesis. ed. J. A. Stoute (Springer, Cham).
Wedi, C. O. O., Kirtley, S., Hopewell, S., Corrigan, R., Kennedy, S. H., and Hemelaar, J. (2016). Perinatal outcomes associated with maternal HIV infection: a systematic review and meta-analysis. Lancet HIV 3, e33–e48. doi: 10.1016/S2352-3018(15)00207-6
Wei, H., Chadman, K. K., McCloskey, D. P., Sheikh, A. M., Malik, M., Brown, W. T., et al. (2012). Brain IL-6 elevation causes neuronal circuitry imbalances and mediates autism-like behaviors. Biochim. Biophys. Acta 1822, 831–842. doi: 10.1016/j.bbadis.2012.01.011
Wilkinson, A. L., Pedersen, S. H., Urassa, M., Michael, D., Andreasen, A., Todd, J., et al. (2017). Maternal systemic or cord blood inflammation is associated with birth anthropometry in a Tanzanian prospective cohort. Tropical Med. Int. Health 22, 52–62. doi: 10.1111/tmi.12799
Yancopoulos, G. D., Davis, S., Gale, N. W., Rudge, J. S., Wiegand, S. J., and Holash, J. (2000). Vascular-specific growth factors and blood vessel formation. Nature 407, 242–248. doi: 10.1038/35025215
Keywords: infection, pregnancy, placenta, vascular development, adverse birth outcomes
Citation: Weckman AM, Ngai M, Wright J, McDonald CR and Kain KC (2019) The Impact of Infection in Pregnancy on Placental Vascular Development and Adverse Birth Outcomes. Front. Microbiol. 10:1924. doi: 10.3389/fmicb.2019.01924
Edited by:
Demba Sarr, University of Georgia, United StatesReviewed by:
Claudio Romero Farias Marinho, University of São Paulo, BrazilDavid W. Walker, RMIT University, Australia
Ricardo Ataide, Burnet Institute, Australia
Copyright © 2019 Weckman, Ngai, Wright, McDonald and Kain. This is an open-access article distributed under the terms of the Creative Commons Attribution License (CC BY). The use, distribution or reproduction in other forums is permitted, provided the original author(s) and the copyright owner(s) are credited and that the original publication in this journal is cited, in accordance with accepted academic practice. No use, distribution or reproduction is permitted which does not comply with these terms.
*Correspondence: Kevin C. Kain, kevin.kain@uhn.ca
†These authors share co-first authorship