- 1Institute of Technology of Agricultural Products, Hellenic Agricultural Organization “Demeter”, Lykovryssi, Greece
- 2Laboratory of Food Chemistry and Analysis, Department of Food Science and Human Nutrition, Agricultural University of Athens, Athens, Greece
- 3Department of Wine, Vine and Beverage Sciences, University of West Attica, Athens, Greece
Multi-starter wine fermentations employing non-Saccharomyces (NS) yeasts are becoming an emerging trend in winemaking. It is therefore important to determine the impacts of different NS strains in the wine phenotype and in particular the aroma outputs in different inoculation schemes and fermentation conditions. Here, two native NS yeasts, Lachancea thermotolerans LtMM7 and Hanseniaspora uvarum HuMM19, were assessed for their ability to improve the quality of Moschofilero, a Greek aromatic white wine. The NS strains were initially examined in laboratory scale fermentations in mixed inoculations with ScMM23, a native Saccharomyces cerevisiae strain. LtMM7 was selected to be further evaluated in pilot scale fermentations. Five different inoculation schemes were considered: single inoculation of ScMM23 (IS), simultaneous inoculation of ScMM23 with HuMM19 (SMH) or LtMM7 (SML), and sequential inoculation of HuMM19 (SQH) or LtMM7 (SQL) followed by ScMM23. At laboratory scale fermentations, the chemical profiles were largely affected by both the NS species and the inoculation scheme applied. The sequential inoculation using HuMM19 produced the most divergent wine phenotype. However, HuMM19 caused significant increases in acetic acid and ethyl acetate levels that impeded its use in pilot scale trials. LtMM7 significantly affected the chemical profiles of wines produced at the winery, especially in the sequential inoculation scheme. Importantly, LtMM7 significantly increased the levels of acetate esters or ethyl esters, depending on the inoculation method applied. In particular, acetate esters like isobutyl acetate, hexyl acetate, and 2-phenylethyl acetate, which all impart fruity or floral aromas, were significantly increased in SQL. On the other hand, higher levels of total ethyl esters were associated with SML. The most striking differences were observed in the levels of fruit-impair esters like ethyl decanoate, 3-methylbutyl octanoate, and isoamyl hexanoate. This is the first study to report a significant increase in the ethyl ester fraction by L. thermotolerans. Interestingly, L. thermotolerans in SQL also increased the concentrations of damascenone and geraniol, the major teprenic compound of Moschofilero, which are associated with several typical floral and fruity aromas of the variety. Present results show that L. thermotolerans may enhance the varietal character and increase the chemical complexity of Moschofilero wines.
Introduction
During winemaking, fermentation of sugars is principally conducted by Saccharomyces cerevisiae, the major wine yeast. Although overwhelmed by non-Saccharomyces (NS) yeast populations in fresh must, S. cerevisiae quickly antagonizes other species and finishes the alcoholic fermentation. Its high efficiency to convert grape sugars to ethanol and the ability to withstand the harsh conditions of fermentation has established its hegemonic role in industrial winemaking as starter yeast culture (Albergaria and Arneborg, 2016). Typically, a selected S. cerevisiae strain is inoculated at high concentration immediately after grape berry crushing. However, by these means, the indigenous yeast microbiota is suppressed and the sensory profile of the wine is largely shaped by the inoculated strain. While the dominance of the starter culture ensures fermentation stability and reproducibility, it deprives wines of the organoleptic complexity or distinctiveness that a spontaneous fermentation may offer (Rainieri and Pretorius, 2000; Mannazzu et al., 2002). Therefore, there is a steadily increasing interest for the exploitation of NS yeasts, the so-called wild yeast flora, in winemaking.
Several yeast species are naturally found on grape skins and may develop during spontaneous alcoholic fermentation, particularly at the early stage of the course. Most of them belong to the genera Hanseniaspora, Candida, Lachancea, Metschnikowia, Pichia, Torulaspora, and Zygosaccharomyces (Nisiotou et al., 2007; Fleet, 2008). Although NS yeasts initiate the fermentation, most of them are not detectable at the end of the course, either because they are ethanol intolerant or incapable to withstand the microbial antagonism. However, their role is crucial for the production of important metabolites that shape the flavor and contribute to the style of wines (Jolly et al., 2014). It has been well established that diverse yeast species or strains may confer different characteristics to wines (Petruzzi et al., 2017; Sgouros et al., 2018). NS yeasts associated with the vineyard-ecosystem are characterized by high biodiversity that can be influenced by several factors, such as the grape cultivar, the sanitary status of grape berries, the viticultural practices, and environmental factors (Nisiotou and Nychas, 2007; Bokulich et al., 2014; Banilas et al., 2016; Drumonde-Neves et al., 2016; Guillamón and Barrio, 2017). Recent studies show that distant viticultural regions maintain different microbial communities (Gayevskiy and Goddard, 2012; Bokulich et al., 2014). Most importantly, such genetic differences may coincide well with phenotypic discrepancies among yeast populations that consequently generate discrete phenotypes and regional signatures in wines (Knight et al., 2015; Banilas et al., 2016; Nisiotou et al., 2018). Thus, the use of yeasts derived from a vineyard-ecosystem may enhance the typicity and genuineness of the respective local wines, bringing the vineyard microbiome to the fore as a new scale of the terroir concept, often referred to as microbial terroir.
During the last decade, there has been an increasing interest from the wine industry in the exploitation and utilization of NS wine yeasts. Accumulating data show that different NS species/strains may have interesting enological phenotypes for the formulation of new starter cultures (Roudil et al., 2019). Some examples include the production of mannoproteins, the lowering of volatile acidity or ethanol content, the production of various aroma compounds and color stabilization. L. thermotolerans appears as a promising candidate species for use as starter culture, due to several positive physiological characteristics that enhance the flavor and improve the overall quality of wine, such as the production of glycerol and 2-phenylethanol (Gobbi et al., 2013; Benito et al., 2016; Roudil et al., 2019). It has been also shown that certain strains can increase wine total acidity through the production of lactic acid while reducing the volatile acidity (Kapsopoulou et al., 2007; Banilas et al., 2016). Other positive enological attributes refer to the relatively high alcoholic fermentation ability and the low production of acetic acid by this species (Hranilovic et al., 2018). However, studies on the flavor profile of wines produced by mixed fermentations of L. thermotolerans and S. cerevisiae are limited (Balikci et al., 2016). Hanseniaspora uvarum (anamorph Kloeckera apiculata), another wild wine yeast that is typically encountered at the early stages of fermentation, has received much less attention as candidate NS-starter in winemaking. Actually, it has long been considered undesirable in winemaking, due to the high production of ethyl acetate that diminishes wine quality (du Toit and Pretorius, 2000; Ciani et al., 2006). However, recent studies have led to the reassessment of H. uvarum as conditionally beneficial for winemaking purposes (Albertin et al., 2016). It is probably the most abundant NS yeast in fresh must, along with M. pulcherrima (Fleet, 2003; Nisiotou and Nychas, 2007), and may largely affect wine character and typicity. Factually, it has been recently shown that selected H. uvarum strains can improve the organoleptic quality of wine aroma (Tristezza et al., 2016). When co-inoculated with S. cerevisiae produces chemically distinct wines compared to pure S. cerevisiae inocula, with increased flavor diversity and thereby complexity (Martin et al., 2018).
The wine volatile profile is a critical point in the use of NS as starter cultures, since it is strongly dictated by the different winemaking conditions (i.e., grape must composition and winemaking practices), suggesting that several trial fermentations should be performed before adopting a novel starter NS culture in industrial production (Beckner Whitener et al., 2016; Whitener et al., 2017).
“Moschofilero” is a major Greek grapevine variety cultivated throughout Greece, but its origin and principal area of cultivation is “Mantinia” plateau in Peloponnese. Moschofilero is a noble pink-skinned aromatic variety used in the production of white fine dry wines with intense floral and fruity characters. Premium sparkling and dessert wines can be also be produced. The typical Moschofilero white wine has a lightweight, lemon color with green tinges. Intense aromas of rose and sweet grapey flavors paired with citrus and green fruits support its aromatic character. Currently, Moschofilero is of high demand in the market of PDO wines, appreciated for its refreshing, vibrant taste and fruity character. According to PDO production requirements, grape must be inoculated with selected starter yeast cultures that can express the aromatic typicity of Moschofilero. To this end, here we present for the first time means to produce terroir-driven wines with the use of Mantinia native yeasts. To enhance the varietal character and regional typicity of Moschofilero, H. uvarum and L. thermotolerans, two non-Saccharomyces yeast species known for high ester production, were examined in laboratory scale fermentations. L. thermotolerans was further selected for pilot scale vinification trials. Present results provide evidence for the combined use of indigenous yeasts in winemaking to fulfill the growing demand for wines with a sense of the place of origin, where historically developed (Vaudour et al., 2015).
Materials and Methods
Yeast Strains
Yeast strains Hanseniaspora uvarum HuMM19, Lachancea thermotolerans LtMM7, and Saccharomyces cerevisiae ScMM23 were isolated from spontaneously fermenting Moschofilero grape must from the Mantinia region, Peloponnese, Greece. Strains were previously selected based on positive enological characteristics such as ethanol and SO2 resistance, acetic acid production, H2S production and fermentation power (unpublished data). S. cerevisiae Zymaflore X5 (Laffort) was applied in the laboratory scale fermentations. Yeasts were identified at the species level by restriction enzyme analysis of the 5.8S-ITS rDNA region as previously described (Nisiotou et al., 2007).
Laboratory Fermentations
Fermentations were performed in triplicate in Moschofilero grape (Vitis vinifera L.) must from Mantinia region [sugars 203 g/L; pH 3.31; titratable acidity 5.6 g/L, as tartaric acid; yeast assimilable nitrogen (YAN) 240 mg/L]. Fermentations were carried out at 20°C under static conditions in 1000 mL Erlenmeyer flasks containing 750 mL of pasteurized (70°C, 10 min) grape must, supplemented with 30 ppm SO2 in the form of potassium metabisulfite. Flasks were equipped with fermentation locks containing glycerol, permitting only CO2 to escape. Yeasts inocula were cultured in grape must (26°C, 18 h, 225 rpm) and added at 6 Log CFU/mL. Different inoculation schemes were applied as follows: single inoculation of the indigenous S. cerevisiae strain ScMM23 (IS), simultaneous inoculation (SM) of ScMM23 and H. uvarum HuMM19 (SMH) or L. thermotolerans LtMM7 (SML), sequential inoculation (SQ) of HuMM19 (SQH) or LtMM7 (SQL) followed by S. cerevisiae ScMM23 after ca. 1% vol ethanol production, and single inoculation of commercial S. cerevisiae (CS). Fermentation progress was monitored by following the weight loss daily.
Pilot Scale Fermentations
L. thermotolerans LtMM7 and S. cerevisiae ScMM23 were used at pilot scale fermentations. Fermentations were carried out in triplicate in a local Mantinian winery in 250 L fermentation tanks with 150 L of Moschofilero grape must (sugars 172 g/L; pH 3.48; titratable acidity 7.12 g/L, as tartaric acid; initial YAN 71.4 mg/L) at 18°C. Potassium metabisulfite was added at 30 ppm total SO2. Grape must was supplemented with nitrogen by adding 40 g/hL of inactivated yeast-product before inoculation and an inactivated-yeast product containing mineral salts after 50 g/L sugar depletion. Yeast inocula were propagated in yeast extract peptone dextrose (YPD) agar at 26°C and resuspended in 1/4 strength Ringer’s solution. L. thermotolerans LtMM7 and S. cerevisiae ScMM23 were added at 6 log CFU/mL in IS, SML, and SQL inoculation schemes as described in laboratory fermentations. Spontaneous (un-inoculated) fermentations (SP) were applied in duplicate as reference. Fermentation dynamics was followed by density measurements.
Microbiological Analysis
Must samples were taken daily, serially diluted and plated on Wallerstein laboratory nutrient agar (WL), ethanol sulfite agar (ESA), and lysine medium agar (LA) for the enumeration of total yeasts, S. cerevisiae and non-Saccharomyces species, respectively. Plates were incubated at 28°C for 2–5 days. Putative L. thermotolerans and S. cerevisiae colonies were isolated from the initial, middle and final stages of non-sterile fermentations, examined microscopically and genotyped. Genotyping of S. cerevisiae was performed by the interdelta region analysis with the primer set delta 12/delta 21 (Legras and Karst, 2003). For L. thermotolerans typing the tandem repeat-tRNA method using the primer pair TtRNASc/ISSR-MB (Barquet et al., 2012).
Chemical Analysis
Reducing sugars, total and volatile acidity, pH, and total and free SO2 determinations were performed according to the methods in the Compendium of International Methods of Analysis of Musts and Wines (OIV, 2015). YAN was assayed using the formol method (Gump et al., 2001). Organic acids (citric, tartaric, malic, succinic, lactic, acetic), sugars (glucose, fructose), glycerol and ethanol were determined by HPLC according to Nisiotou et al. (2018). The major volatile compounds [acetaldehyde, ethyl acetate, methanol, 1-propanol, 2-methyl-1-propanol (Isobutanol), 3- and 2-methyl-1-butanol] of wine fermentations were determined by direct injection of wines in a gas chromatograph as previously described (Nisiotou et al., 2018). The minor volatiles of wines were determined using a headspace SPME/GC-MS method, as described by Hjelmeland et al. (2013) with slight modifications (Nisiotou et al., 2018). Peaks were quantified relative to the internal standard using peak area of an extracted ion.
Sensory Analysis
A panel of 7 experienced assessors (3 males and 4 females, 25–55 years old, members of the Institute of Technology of Agricultural Products and of the Department of Wine, Vine and Beverage Sciences of the University of West Attica) was convened for this study. To describe the samples four aroma (tree fruits, citric fruits, floral, intensity) and seven palate (oxidation, acidity, complexity, balance, mouth aroma, persistence, after taste) terms were developed by the panel during preliminary sessions. Samples were assessed in duplicate in standard sensory analysis rooms with separate booths. Wines were presented to panelists in randomized order. The assessors scored aroma/palate attributes using a scale ranging from 0 (not perceivable) to 5 (high intensity).
Statistical Analysis
Significant differences between chemical profiles of wines from different inoculation schemes were evaluated by Analysis of Variance (ANOVA) and Tukey’s HSD test. Principal component analysis (PCA) was applied to chemical parameters to explore relationships between samples and variables. Permutational multivariate analysis of variance (PERMANOVA) was used to compare between groups of inoculation schemes. Jaccard metric was used to calculate pairwise distances and 4,999 permutations were randomly sampled to compute F-statistics. Statistical analyses were performed with the PAST software version 3.11 (Hammer et al., 2001).
Results
Kinetics and Yeast Population Dynamics in Laboratory-Scale Fermentations
Hanseniaspora uvarum HuMM19 and Lachancea thermotolerans LtMM7 were evaluated in pasteurized grape must. Equal quantities of each strain were added as inocula along with S. cerevisiae ScMM23 either simultaneously (SMH and SML inoculation schemes for HuMM19 and LtMM7, respectively, collectively called SM fermentations) or sequentially (SQH and SQL inoculation schemes, collectively called SQ fermentations). Single inoculations with the indigenous strain ScMM23 (IS) and the commercial S. cerevisiae Zymaflore X5 (CS) were also conducted as references. The fermentation kinetics of the different inoculation schemes are shown in Figure 1 and in Supplementary Figure S1. At the end of the fermentation courses, residual sugars were below the detection limit (<0.6 g/L) in all samples, except for SQL and SQH ferments, in which low levels of fructose were detected (Table 1). Profound differences were observed in the fermentation rate among different inoculation schemes, which was much lower in sequential than in simultaneous or single inoculations. The duration of fermentations lasted significantly longer (P < 0.05) in sequential additions (13.8 days for SQH and 16.5 days for SQL) than in simultaneous or single inoculations (ca. 11 days). The SML and IS schemes showed rather similar fermentation profiles, whereas the use of HuMM19 in SMH decreased the fermentation rate after day 4.5, thus causing an extension in the fermentation time by 1.2 days. A notable increase in the fermentation rate of SQH was observed at day 9.9, coupled with a rise in S. cerevisiae population by 0.6 Log CFU/mL. At this point, 72.15 g CO2 out of a total of 90.06 g was released.
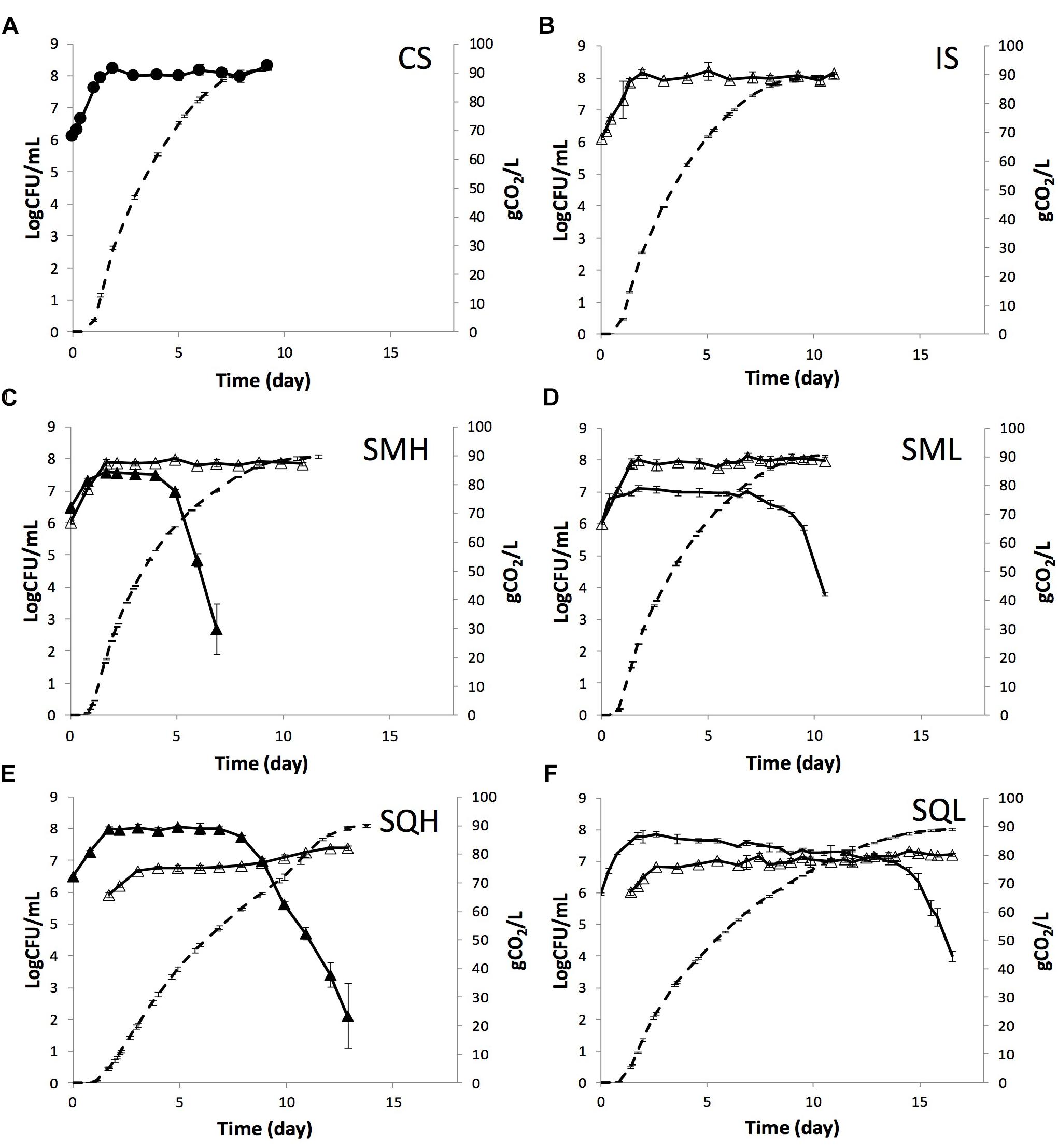
Figure 1. Kinetics (dashed line) and yeast population dynamics (continuous line) of laboratory fermentations performed by (A) commercial S. cerevisiae (●), (B) indigenous S. cerevisiae ScMM23 (△), (C) H. uvarum HuMM19 (▲) and S. cerevisiae ScMM23 (△) added simultaneously, (D) L. thermotolerans LtMM7 (−) and S. cerevisiae ScMM23 (△) added simultaneously, (E) H. uvarum HuMM19 (▲) and S. c erevisiae ScMM23 (△) added sequentially, and (F) L. thermotolerans LtMM7 (−) and S. cerevisiae ScMM23 (△) added sequentially.
The strain ScMM23 showed similar kinetics in IS and simultaneously-inoculated (SMH or SML) fermentations (Figure 1). ScMM23 peaked within 45 h after inoculation and then maintained high levels till the end of the course. A rather small (ca. 0.17 Log CFU/mL) albeit persistent decrease in the maximum population of ScMM23 was observed in SMH compared to IS. ScMM23 showed completely different kinetic behavior when inoculated sequentially with either HuMM19 or LtMM7. The population densities of ScMM23 in sequential fermentations were significantly lower (ca. 1 Log CFU/mL) compared to single or simultaneous inoculations. In SQH, a first plateau was reached within 34 h of inoculation (Figure 1E). At about day 8, the population started to gradually increase and retained these levels till the end of the fermentation. A rather small but gradual increase in the population of ScMM23 was also observed in SQL after day 11.8 till the end of the course. Notably, in both SQH and SQL fermentations, the increase in ScMM23 population coincided well with the drop of NS yeast counts. The presence of HuMM19 caused a rather small (0.2 Log CFU/mL) but continual decrease in the population of ScMM23 up to day 8, as was also observed in SMH.
As opposed to S. cerevisiae, the NS strains reached higher population levels in SQ than in SM fermentations. The maximum population density recorded for strain HuMM19 was by 0.47 Log CFU/mL higher in SQH than in SMH. Similarly, strain LtMM7 achieved higher density by 0.75 Log CFU/mL in SQL compared to SML. Differences were further observed in the length of the stationary phase between the different fermentation schemes. The stationary phase for both strains HuMM19 and LtMM7 lasted longer in SQ compared to SM fermentations, i.e., 7 days in SQH vs. 4 days in SMH and 15 days in SQL vs. 9 days in SML. The subsequent death rate of HuMM19 was faster in SMH than in SQH ferment, while no respective differences were observed for LtMM7 in SQL and SML ferments. HuMM19 achieved higher population densities than LtMM7 in simultaneous and sequential inoculations by 0.47 and 0.20 Log CFU/mL, respectively. Irrespective of the inoculation scheme applied, the populations of both HuMM19 and LtMM7 declined upon the release of ca. 60 g CO2 (corresponding to 8.0% vol ethanol) and 88 g CO2 (11.7% vol), respectively.
Kinetics and Yeast Population Dynamics in Pilot Scale Fermentations
Strains ScMM23 and LtMM7, which showed desirable analytical profiles in laboratory fermentations, were used in pilot scale fermentations of naturally-processed grape must at the premises of a commercial winery. The fermentation dynamics under different inoculation schemes are shown in Figure 2 and Supplementary Figure S2. IS and SM ferments showed similar fermentation kinetics, characterized by higher fermentation rates compared to SQ and spontaneous (SP) fermentations and faster completion of the course by approximately 1 day. The SP fermentation exhibited the longest lag phase followed by a sharp decline in the grape must density after day 4. Differences were observed in the growth kinetics of yeasts among the various inoculation schemes. S. cerevisiae ScMM23 followed similar kinetics in IS and SM fermentations, with maximum population densities of 8.10 ± 0.20 and 8.03 ± 0.07 Log CFU/mL, respectively (Figures 2A,B). The respective levels were lower in SQ (7.61 ± 0.08 Log CFU/mL) and SP (7.81 ± 0.07 Log CFU/mL) ferments (Figures 2C,D) compared to both IS and SM ferments. ScPK7 dominated in both IS and SM fermentations at percentages (93–100%). Lower percentages (14–38%) were observed in SQ fermentation while it could not be detected in SP ferment.
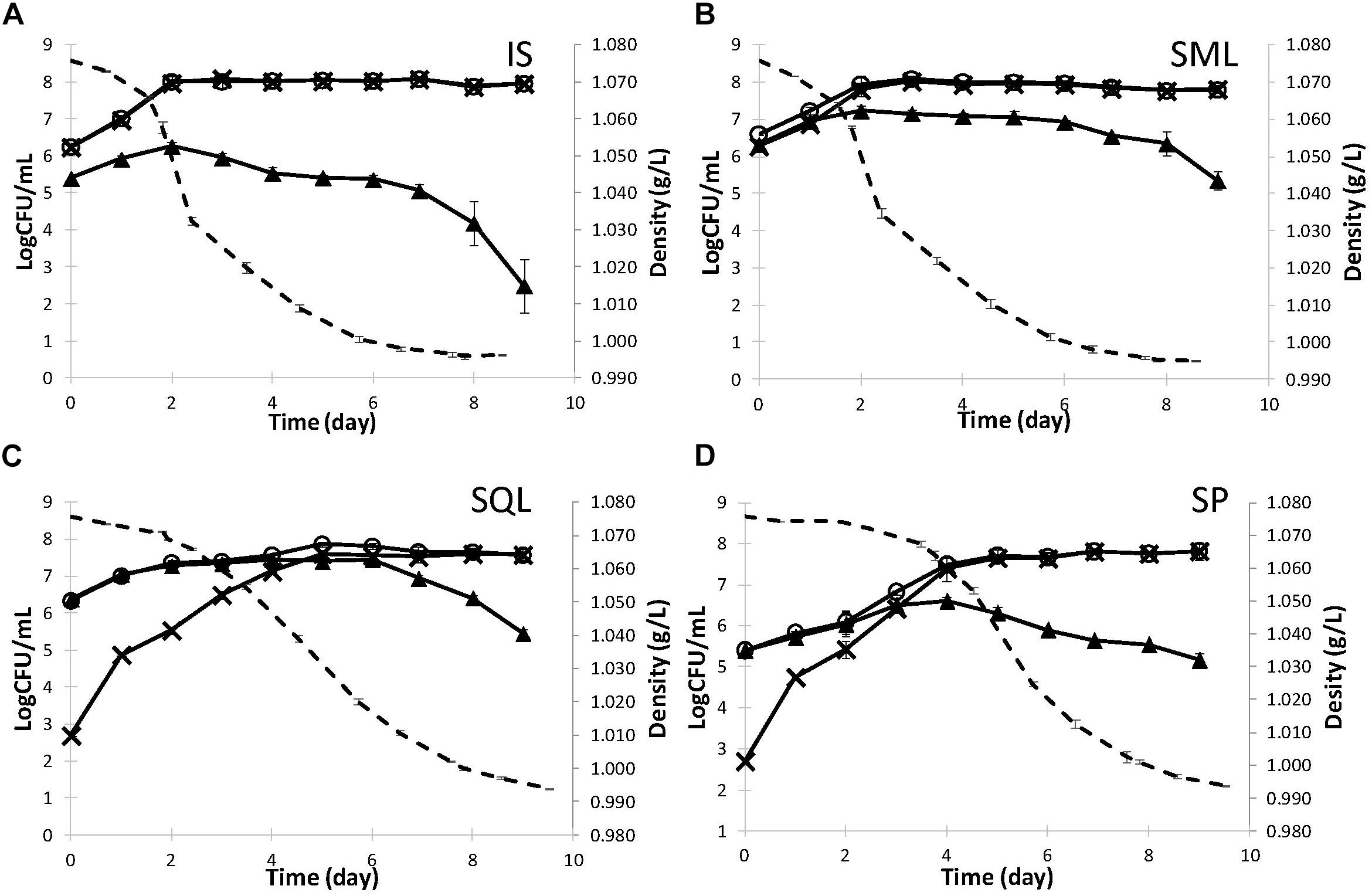
Figure 2. Kinetics (dashed line) and yeast population dynamics (continuous line) of pilot-scale fermentations inoculated with S. cerevisiae ScMM23 (A), L. thermotolerans LtMM7/S. cerevisiae ScMM23 added simultaneously (B), L. thermotolerans LtMM7/S. cerevisiae ScMM23 added sequentially (C). Spontaneous fermentation was also conducted as reference (D). WL agar (○) was used for enuxmeration of total yeast population, ESA for S. cerevisiae (×), and LA (▲) for non-Saccharomyces yeasts.
The addition of LtMM7 in either SM or SQ ferments significantly altered the kinetic profile of the non-Saccharomyces (NS) yeast fraction of the fermentative microbiota. While the indigenous NS yeasts did not exceed 6.60 ± 0.11 Log CFU/mL or 6.26 ± 0.11 Log CFU/mL in either SP or IS fermentations, significantly higher counts were observed in SQL (7.44 ± 0.18 Log CFU/mL) and SML (7.23 ± 0.15 Log CFU/mL) inoculation schemes (Figure 2). In both SM and SQ fermentations, NS populations peaked by day 2. Differences were thereafter observed, as the NS population started to gradually decline in SM, whereas in SQ it was retained at high density up to day 6. At the end of alcoholic fermentation strain LtMM7 was isolated at frequencies of 63 and 67% in SML and SQL ferment, respectively. In mixed inoculations, NS counts started to decrease at 1.021 g/L density (ca. 11% vol).
The Effect of Different Inoculation Schemes on the Wine Chemical Profiles in Laboratory-Scale Fermentations
The chemical characteristics and the major volatiles (Tables 1, 2) of laboratory wines were analyzed by Permutational Multivariate Analysis of Variance (PERMANOVA). As it was shown, the inoculation scheme applied significantly affected the chemical profile of wine (F = 1095, P < 0.01). Pairwise PERMANOVA was applied to reveal the level of discrimination among the four types of inoculation schemes (Supplementary Table S1) considering F-values as an indicator of discrimination between samples. The chemical profile of wine was largely affected by the non-Saccharomyces species applied. The wine produced by the sequential inoculation using the HuMM19 strain (SQH) was the most divergent among all ferments, with F-values ranging from 1,368 to 2,521 followed by SMH (F-values 161–1,368). The time of S. cerevisiae addition, either simultaneously or sequentially to the NS strain, also affected the chemical profile of wines. It was shown that, irrespectively of the NS strain used, SQ-inoculated fermentations exhibited higher level of differentiation from IS as compared to SM ferments (Supplementary Table S1). The chemical profiles of IS, CS and SML schemes were more similar to each other than to other ferments.
The chemical profiles of the different ferments were analyzed by Principal Component Analysis (PCA) (Figure 3). The first two principal components accounted for 65.5% (43.4 and 22.1% for PC1 and PC2, respectively) of total variability. IS and SML were closely located to each other, sharing high values of malic acid which loaded negatively on PC1. On the opposite side of PC2 axis, SQH formed a distantly separated cluster showing highly positive scores on PC1 for acetic acid, ethyl acetate, isobutanol and volatile acidity. SQL was also well separated on the opposite quadrant to SQH along the PC1 axis. SMH showed high values on PCY for characteristics such as ethanol, glycerol and succinic acid.
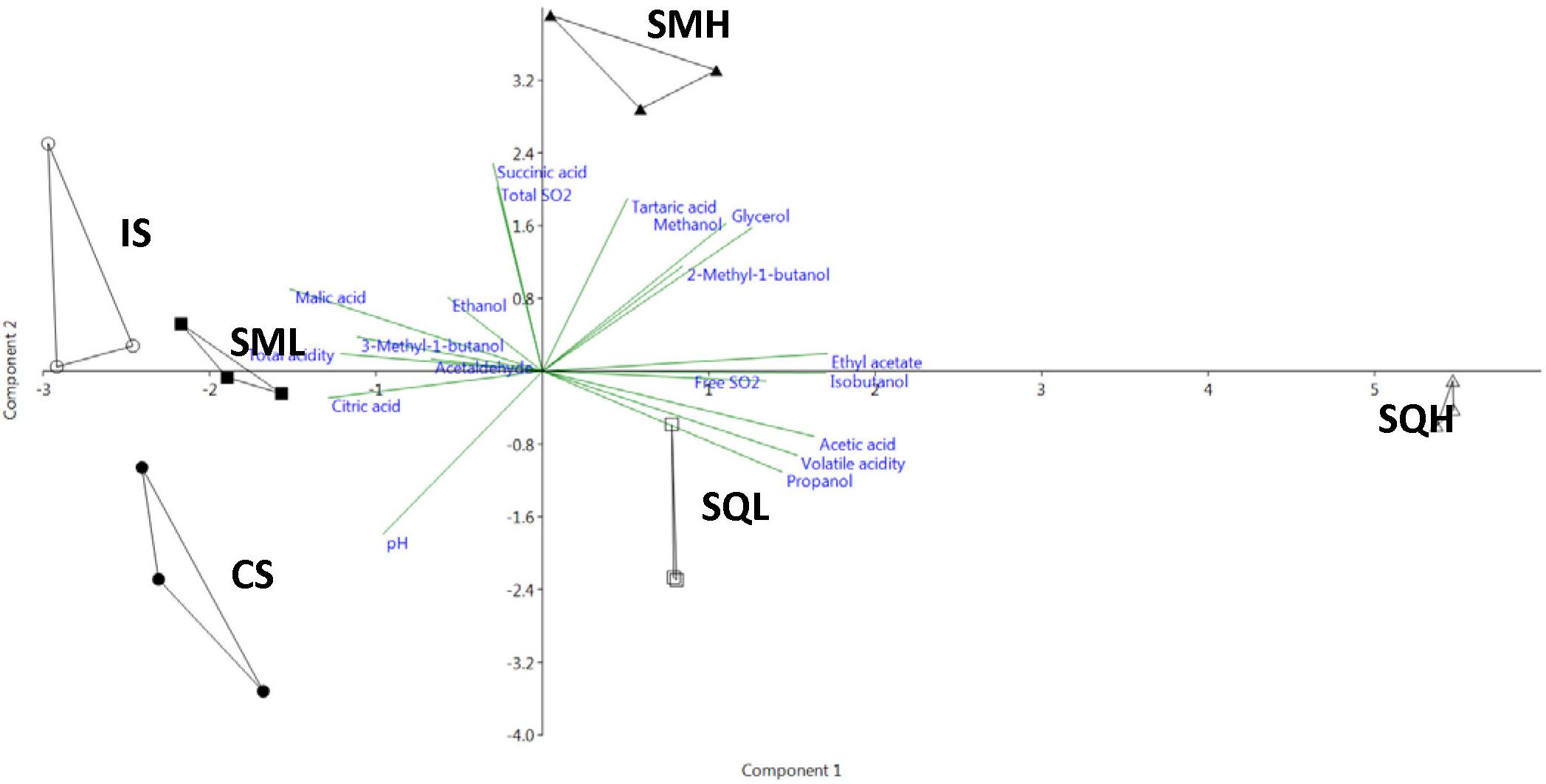
Figure 3. Principal Component Analysis (PCA) of the chemical attributes of the laboratory scale produced wines. PC1 and PC2 correspond to 43.4 and 22.1% of the total variation, respectively. CS, commercial S. cerevisiae; IS, S. cerevisiae ScMM23; SMH, H. uvarum HuMM19, and S. cerevisiae ScMM23 added simultaneously; SQH, H. uvarum HuMM19, and S. cerevisiae ScMM23 added sequentially; SML, L. thermotolerans LtMM7, and S. cerevisiae ScMM23 added simultaneously; SQL, L. thermotolerans LtMM7, and S. cerevisiae ScMM23 added sequentially.
Chemical parameters were individually subjected to ANOVA to evaluate their contribution to the differentiation of chemical profiles (Table 1). The total acidity (TA) was significantly increased in IS and SQL ferments compared to other inoculation schemes. As opposed, SQH exhibited lower TA values by more than 2 g/L. The SQ fermentations produced elevated levels of volatile acidity compared to other schemes, with SQH showing the highest amount. Residual fructose could only be detected only in SQL and SQH, whereas it was below the detection limit (<0.6 g/L) in all other ferments. A considerable amount of lactic acid was recorded in SQL (2.4 ± 0.1 g/L). The use of HuMM19 significantly enhanced the glycerol content in the respective ferments. ScMM23 yielded higher amounts of glycerol and malic acid when compared to the commercial yeast starter. The inoculation scheme was also found to affect the major volatile profile of the ferments (Table 2). The most profound difference was detected in the concentration of ethyl acetate, which was drastically increased in SQH, followed by SMH. LtMM7 caused a significant rise in ethyl acetate only in SQL; yet the level was lower than the one detected in SQH or SMH. The addition of LtMM7 caused considerable accumulations of acetaldehyde. Sequential inoculations yielded significant higher levels of ethyl acetate than simultaneous additions. Between the two NS yeasts applied, HuMM7 was strongly associated with increased production of ethyl acetate, since both SMH and SQH ferments contained significantly higher amounts than the other ferments. The addition of NS yeasts increased the levels of propanol and isobutanol. The effect was more evident in SQ than in SM ferments.
The Effect of Different Inoculation Schemes on the Wine Chemical Profiles in Pilot-Scale Fermentations
The chemical compositions of wines produced at a commercial winery with the strains ScMM23 and LtMM7 under different inoculation schemes are shown in Tables 3–5. By applying PERMANOVA it was shown that the inoculation scheme significantly affected the chemical profiles of wines (F = 5.4, P < 0.01). As shown by pairwise PERMANOVA (Supplementary Table S1), the chemical profile of the SQL-produced wine was the most divergent among other ferments, followed by SML (mean F-values of 13.0 and 12.5, respectively). IS and SP ferments displayed lower mean F-values at 4.4 and 3.8, respectively.
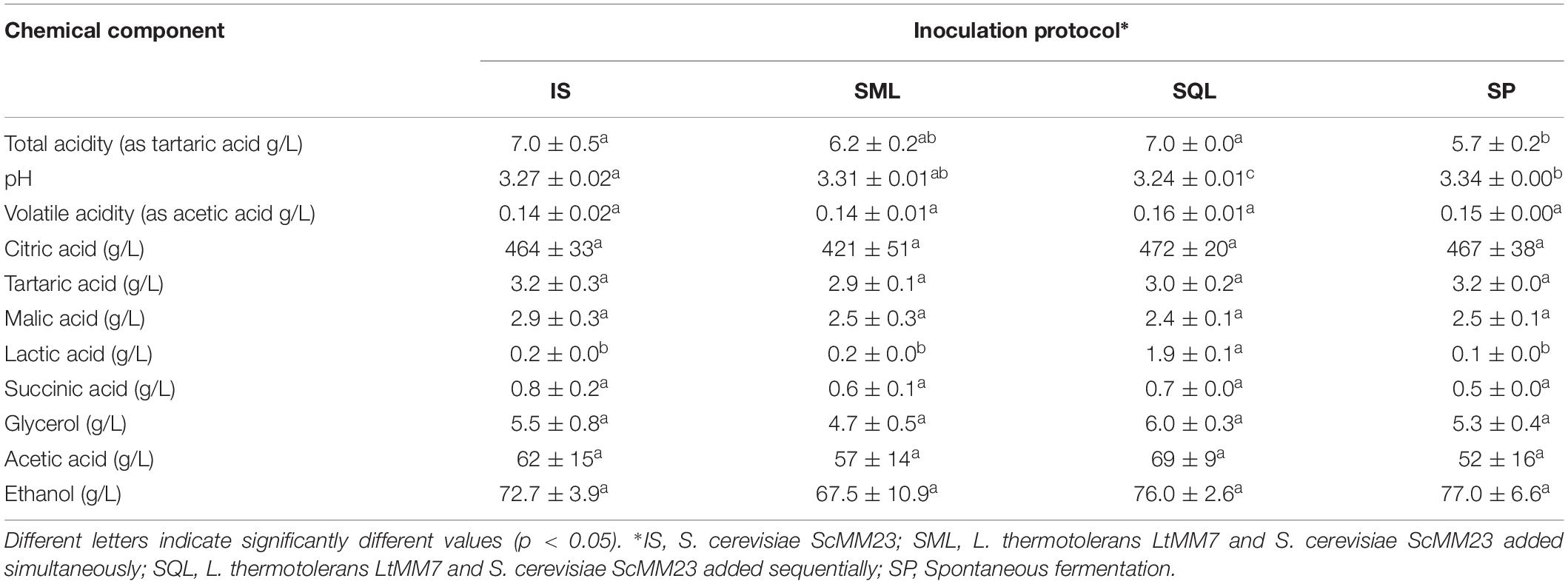
Table 3. Chemical characteristics of wines produced in pilot-plant fermentations (mean ± SD, n = 3 or n = 2 in SP).
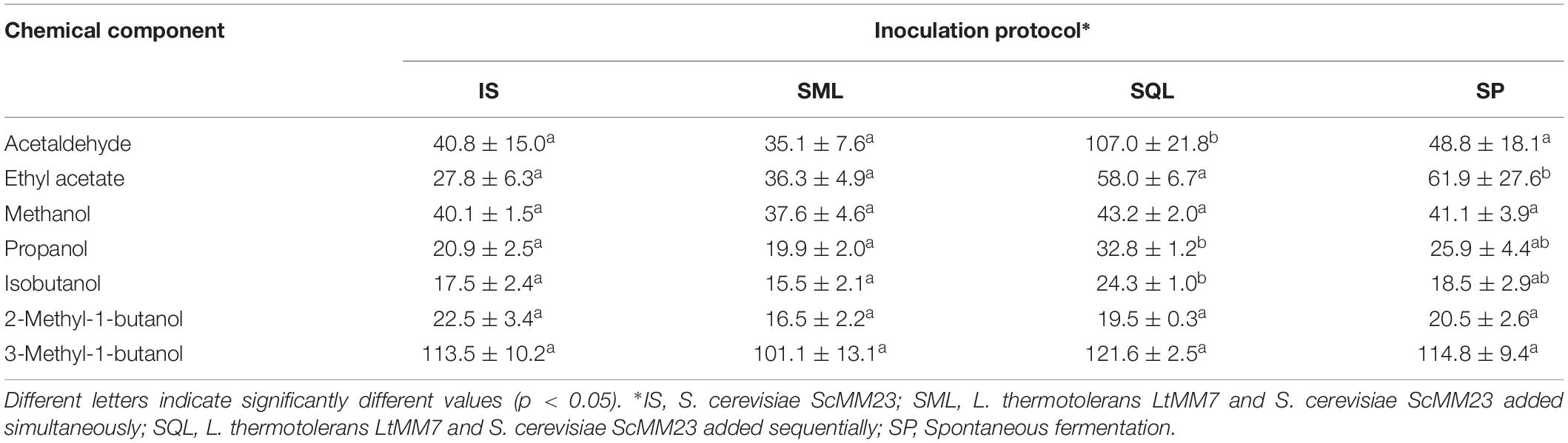
Table 4. Major volatiles (mg/L) of wines produced in pilot scale fermentations (mean ± SD, n = 3 or n = 2 in SP).
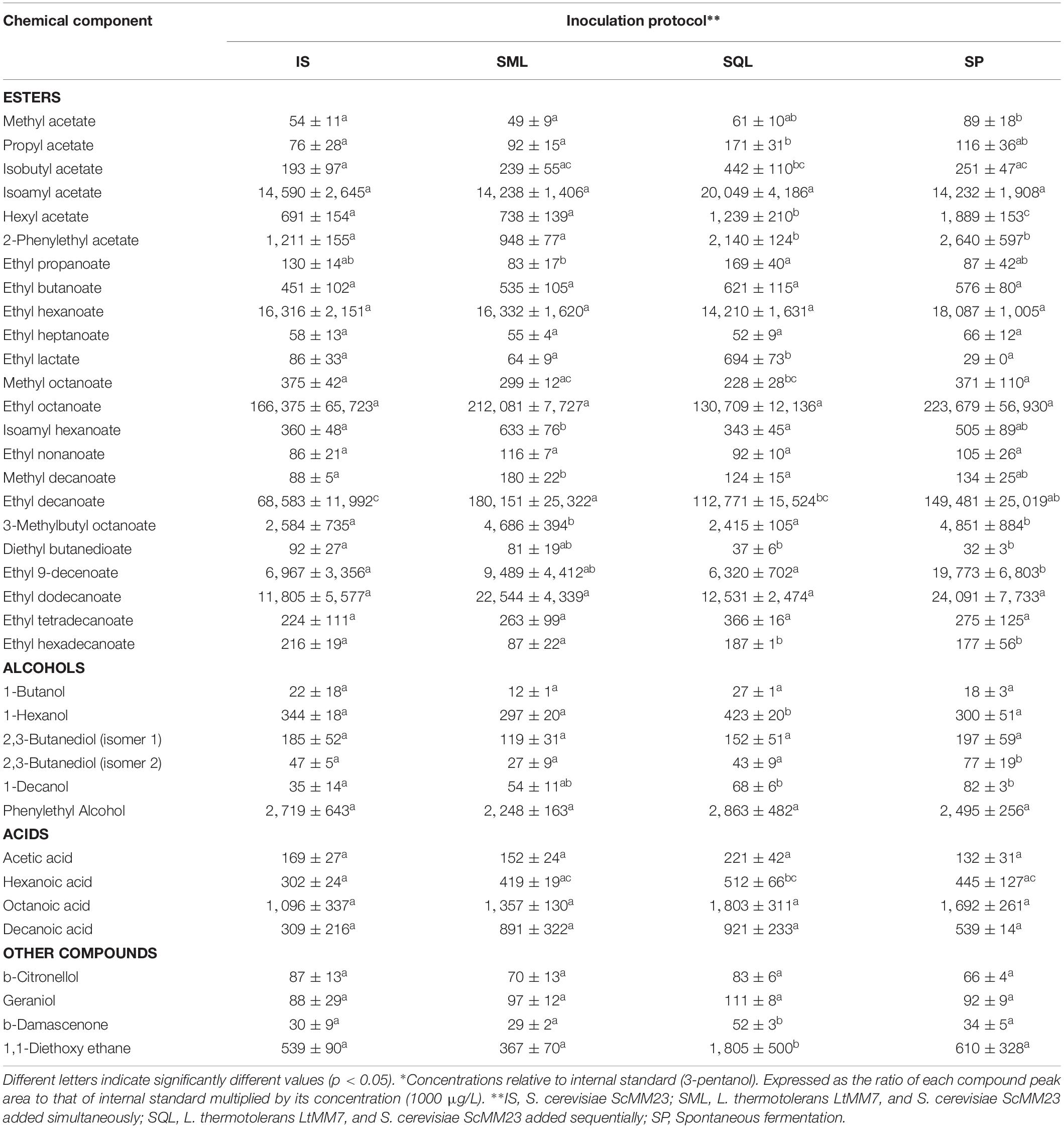
Table 5. Minor volatiles (μg/L)* of wines produced in pilot scale fermentations (mean ± SD, n = 3 or n = 2 in SP).
The chemical profiles of different ferments were compared by PCA (Figure 4). The cumulative variability for the first two components was at 58.1% (35.7 and 22.4% for PC2). The profiles of SQL ferments were the most distantly located on the PCA plot, exhibiting highly positive scores on PC1 for numerous characteristics, such as acetic acid, damascenone, isoamyl acetate and phenylethyl alcohol. IS and SML ferments were separated from SQL along the PC2 direction. IS was valued negatively on PC2, mainly due to the presence of malic acid, succinic acid and 2-methyl-1-butanol. The SML ferment exhibited high values of ethyl esters that loaded negatively on the PC1, such as ethyl octanoate, isoamyl hexanoate, methyl decanoate, ethyl decanoate and 3-methylbutyl octanoate. SP ferments were most closely located to SML, due to the high values of certain compounds on PC2, such as ethyl esters (Hexyl acetate, Ethyl hexanoate, Ethyl 9-decenoate) or alcohols (1-Decanol and 2,3-Butanediol).
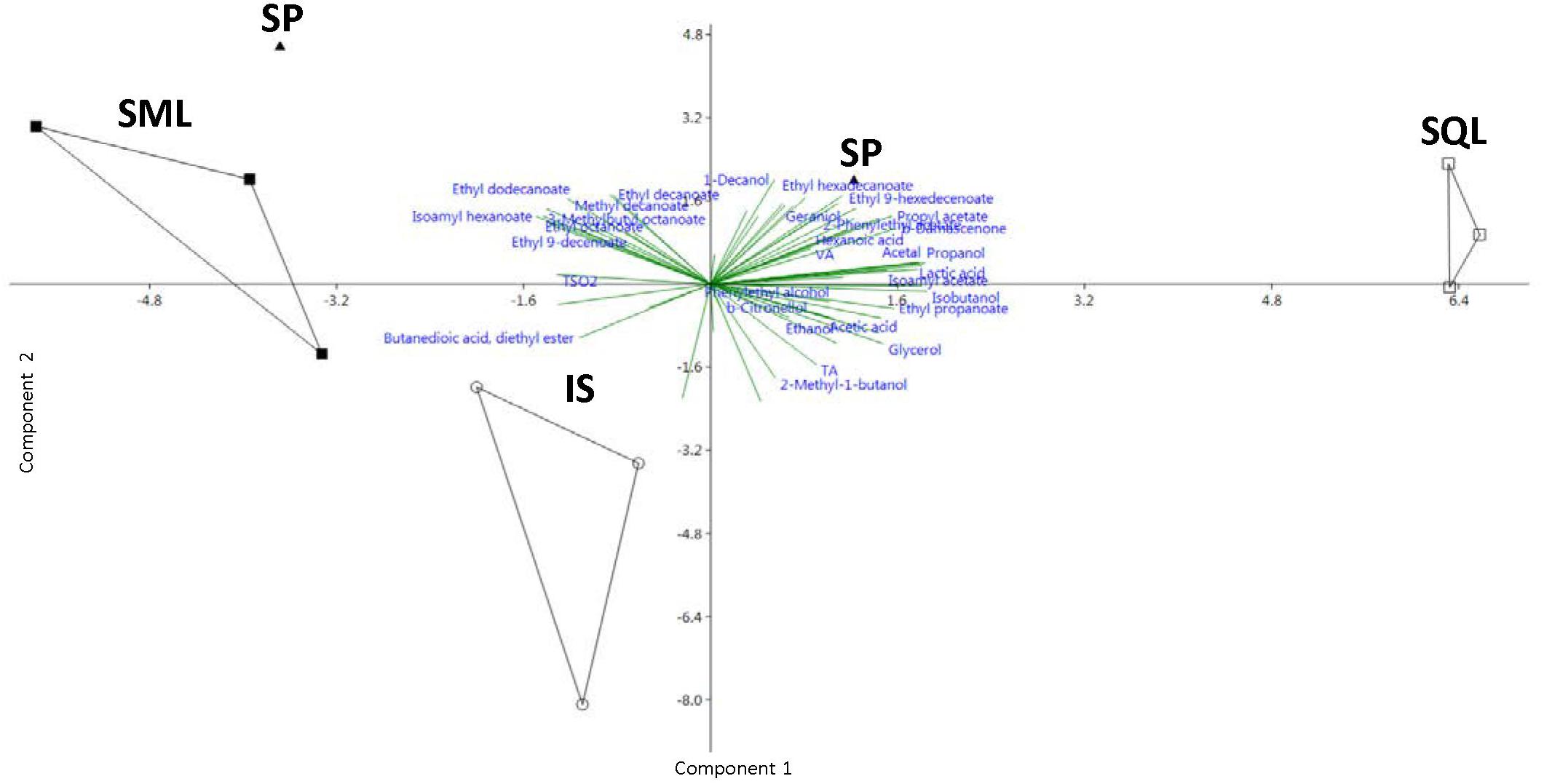
Figure 4. Principal Component Analysis (PCA) of the chemical attributes of the pilot scale produced wines. PC1 and PC2 correspond to 35.7 and 22.4% of the total variation, respectively. IS, S. cerevisiae ScMM23; SML, L. thermotolerans LtMM7, and S. cerevisiae ScMM23 added simultaneously; SQL, L. thermotolerans LtMM7, and S. cerevisiae ScMM23 added sequentially; SP, Spontaneous fermentation.
Each of the chemical parameters was subjected to ANOVA to investigate its contribution to the chemical profile of wines. Total acidity was significantly lower (P < 0.05) in SP compared to IS and SQL ferments (Table 3). The use of LtMM7 in SQL fermentation caused the decline of pH (P < 0.05) probably due to the production of considerable amounts of lactic acid. Significant raises were also observed in acetaldehyde, propanol and isobutanol levels in SQL ferments. Differences were further observed in the concentration of minor volatiles, mostly associated with the use of LtMM7 in the SQL inoculation scheme (Table 5). LtMM7 notably increased the levels of acetate esters, especially in SQL ferment in which their concentration was twofold higher compared to IS. Propyl acetate, isobutyl acetate, hexyl acetate and 2-phenylethyl acetate were significantly higher in SQL than in IS or SML. LtMM7 was also associated with significantly higher levels of ethyl esters when used in SML inoculation. The most striking differences were observed in the levels of ethyl decanoate, 3- methylbutyl octanoate and isoamyl hexanoate. The concentration of total acids and terpenes were also significantly affected by the addition of LtMM7, with SQL ferment exhibiting the highest values.
Sensory Analysis
Figure 5 shows the mean scores of sensory characteristics of Moschofilero wines as evaluated by the sensory assessors’ panel. According to ANOVA, significant differences (p < 0.05) among samples were detected for three descriptors, i.e., floral aroma, balance, and after-taste. In particular, SQL wine was characterized by the highest intensity of floral aroma and after-taste, followed by SML, while SML was the most balanced among all the wines. Considering multiple pairwise comparisons, SP wine showed significantly higher intensity of tree fruit aroma than the other wines. SP was also characterized by the highest overall aroma intensity (significantly different from IS). SQL wines were found to have the most intense citric fruit aroma and complexity, which were significantly different from IS wine. In addition, SQL wines had increased acidity and palate complexity (significantly higher compared to IS), but a lower balance as compared to other wines.
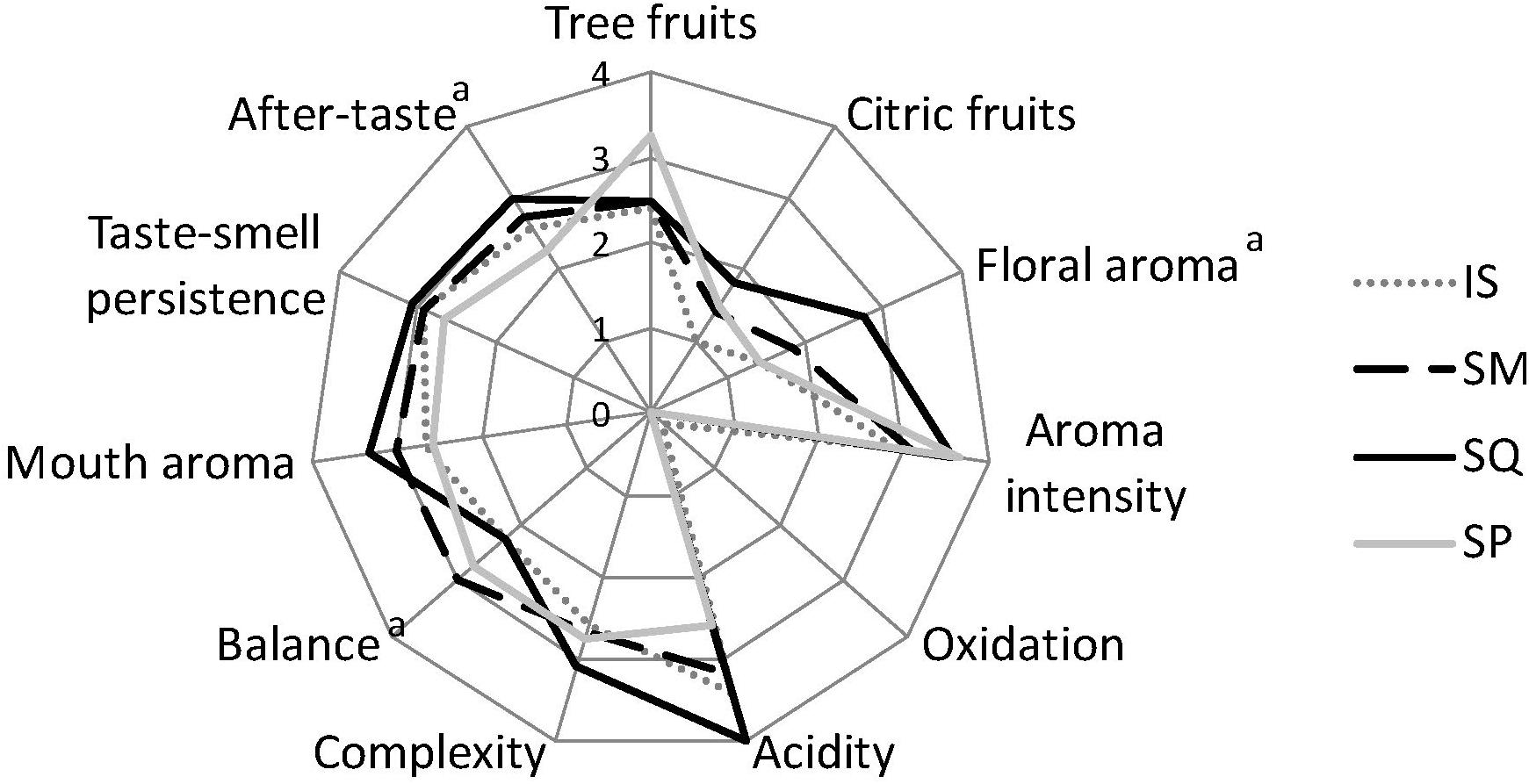
Figure 5. Means of the sensory attributes for the pilot scale produced wines. IS, S. cerevisiae ScMM23; SML, L. thermotolerans LtMM7, and S. cerevisiae ScMM23 added simultaneously; SQL, L. thermotolerans LtMM7, and S. cerevisiae ScMM23 added sequentially; SP, spontaneous fermentation. Significant differences among samples are indicated by superscript (p < 0.05).
Discussion
“Moschofilero” is a native Greek grape variety traditionally cultivated in the “Mantinia” plateau in Peloponnese, Greece, since ancient times. It is used in the production of “Mantinia” PDO blanc de gris white wines with a characteristic fruity and floral aromatic profile (rose, lemon, and jasmine) that has been associated with its place of origin. To enhance the connection of Moschofilero wines with its original area of cultivation, the introduction of the notion of “microbial terroir” was considered in the present study, by applying autochthonous yeasts as starters in the fermentation of grape must. Besides, high ester producing species were considered for their potential to enhance the floral aromas and fruity bouquet of wines.
Here, two autochthonous H. uvarum and L. thermotolerans strains were investigated for their ability to contribute the aromatic features associated with the wild yeast flora, along with a S. cerevisiae strain, also isolated from the Mantinia vineyard, to complete the fermentation course. The fermentation kinetics in sterile must was affected by both the inoculation scheme and the NS yeast species applied. When simultaneously inoculated, S. cerevisiae was highly antagonistic and reduced the population of both NS yeasts as compared to SQ inoculations. This is commonly attributed to higher sugar fermentation capacity and nitrogen uptake of S. cerevisiae compared to several NS wine yeasts including H. uvarum (Andorrà et al., 2012; Albergaria and Arneborg, 2016). The population reduction was larger for L. thermotolerans than H. uvarum, showing that the former species might be more susceptible to S. cerevisiae activity. L. thermotolerans has been shown to immediately diminish upon addition of S. cerevisiae possibly through a concerted action of cell-to-cell contact and antimicrobial peptides (Benito et al., 2015, 2016; Kemsawasd et al., 2015). In some other studies, though, L. thermotolerans showed increased persistence in mixed fermentations, especially in SQ inoculations (Comitini et al., 2011; Gobbi et al., 2013). These results together suggest that the viability of NS yeasts in mixed-culture or spontaneous fermentations may not be solely defined at the species level but is also highly strain-dependent (Wang et al., 2015). It is important thus that strain compatibilities should be considered upon designing of mixed inocula in wine fermentations.
The wine chemical profile in sterile must fermentations was highly differentiated by applying the sequential inoculation scheme. The strongest differentiation was associated with the use of H. uvarum, despite the fact that it showed much lower persistence than L. thermotolerans. However, its presence was strongly correlated with significant increase of acetic acid and ethyl acetate. H. uvarum often generates elevated levels of acetate and ethyl acetate (du Toit and Pretorius, 2000; Ciani et al., 2006). Nevertheless, it is an important biotic component of wine fermentation as a high producer of fruity esters, while its low frequency has been associated with reduced aroma complexity of wine (Rementeria et al., 2003). Nevertheless, there is great genetic and phenotypic variability among vineyard-associated H. uvarum isolates. For instance, the use of a selected H. uvarum isolate increased medium chain fatty acid (MCFA) ethyl ester content without raising the acetate concentration beyond the acceptable limit (Hu et al., 2018). In the present study, although H. uvarum strain HuMM19 produced relatively low levels of acetic acid (according to the optimal concentration range of 0.2–0.7 g/L) (Lambrechts and Pretorius, 2000), it strongly increased the concentration of ethyl acetate far above the acceptable limits (100 mg/L), responsible for solvent/nail polish-like odor (Sumby et al., 2010). On the other hand, the use of L. thermotolerans was associated with several positive enological characteristics but not any obvious defects, and was thus selected to perform further fermentations at pilot scale trials.
Ester production is an important quality attribute of yeast activity, contributing significantly to the aroma of wines (Ugliano and Henschke, 2009). The total amount as well as the profile of ester production shows high variability depending on the yeast species or strains implicated, shaping thereby wine style and character (Beckner Whitener et al., 2016). Although L. thermotolerans was one of the first NS yeasts to be released commercially as starter culture for winemaking, the profile of ester production was only recently explored in more detail (Beckner Whitener et al., 2016; Whitener et al., 2017), while very few studies have investigated its performance in industrial scale fermentations (Gobbi et al., 2013). It has been generally accepted that L. thermotolerans affects the aroma profile of wines by producing several acetate esters rather than ethyl esters (Morales et al., 2017; Morata et al., 2018). When compared to S. cerevisiae, it generally produces significantly lower levels of acetate esters or total esters, excluding ethyl lactate and ethyl acetate (Gobbi et al., 2013; Balikci et al., 2016; Beckner Whitener et al., 2016; Whitener et al., 2017). Here the strain LtMM7 significantly increased the levels of both acetate esters and ethyl esters. It is also important to note that the inoculation protocol applied significantly affected the relative production of acetate esters and ethyl esters. In particular, acetate esters such as isobutyl acetate, hexyl acetate and 2-phenylethyl acetate, which impart fruity or floral aromas, were significantly increased in the SQL ferment. Importantly, the level of 2-phenylethyl acetate, which confers floral, rosy and honey-like with fruity nuance odors, all typical varietal aromas of Moschofilero, was doubled in SQL compared to IS. In line with that, the floral aroma intensity was significantly higher in SQL wine compared to other wines. On the other hand, higher levels of total ethyl esters were associated with SML (eight different odorant active compounds). The most striking differences were observed in the levels of ethyl decanoate (soapy, floral), 3-methylbutyl octanoate (fruit) and isoamyl hexanoate (anise, fruit, spice). The increase in the concentration of ethyl esters positively correlates with the fruity aroma of wine (Hu et al., 2018) and the respective wines were characterized by higher overall aroma intensity and mouth aroma than IS. Several fermentation factors have been evaluated to stimulate ethyl ester production, such as the addition of MCFA precursors (Saerens et al., 2008), nitrogen additions (Rollero et al., 2015) or mixed fermentations with NS yeasts. With respect to the later, accumulated data show that ethyl ester content can be enhanced by the use of NS yeasts in a strain-specific way (Hu et al., 2018; Nisiotou et al., 2018).
Varietal aromatic precursors, such as terpenes and C13-norisoprenoids, are predominantly found in grapes in their glycosylated odorless form. They can then be hydrolyzed by glucosidases to free aromatic derivatives during fermentation. Moschofilero is an aromatic (floral) variety rich in total terpenes, a large portion of which is in bound form (Metafa and Economou, 2013). Geraniol constitutes the highest fraction of bound terpenes also accounting for about half of the total free terpenic content in wine (Metafa and Economou, 2013). It seems that a fermentation protocol which can liberate bound aromatic compounds could enhance the varietal character of Moschofilero. Contrary to previous beliefs (Comitini et al., 2011), current studies show that certain strains of L. thermotolerans may exhibit high β-glucosidase activity (Cordero-Bueso et al., 2012). Recently, L. thermotolerans was found to increase the free terpenic content (farnesol, geraniol, α-ionene, and cosmene) in Sauvignon blanc wines (Beckner Whitener et al., 2016). In another study, L. thermotolerans was shown to produce the highest relative concentration of linalool than other yeast species (Whitener et al., 2017). In the present study, the use of L. thermotolerans in SQL fermentation was shown to increase the concentrations of geraniol and damascenone. Geraniol, a major odor compound of Moschofilero, has a low odor threshold (30 μg/l) (Guth, 1997) and is associated with several typical aromas of Moschofilero, such as floral, sweet, rosy, fruity and a citrus nuance. β-damascenone is a key odor in grapes with low odor threshold (4–7 μg/L in wine matrix) (Pineau et al., 2007). The contribution of damascenone in wine flavor is important either directly by conferring floral and exotic fruit notes (Guth, 1997) or indirectly by strengthening the fruit aromas of other compounds (Pineau et al., 2007).
Conclusion
In conclusion, the present results show that the use of an indigenous L. thermotolerans strain as a NS yeast starter along with a selected S. cerevisiae strain may enhance the typical floral and fruity aromas of Moschofilero, one of the most important Greek white wines. This is the first study to show a significant increase in ethyl ester fraction by L. thermotolerans. Importantly, the inoculation scheme significantly affected the relative production of acetate esters and ethyl esters. As opposed, a selected indigenous H. uvarum strain did not show a prominent enological potential to be used as a fermentation starter, coinciding with previous studies showing that this NS species may generate elevated levels of ethyl acetate. Taken together, well-selected indigenous NS strains may be used in multispecies fermentations to enhance the organoleptic properties and the typicity of local wines by introducing the so-called microbial terroir effect.
Data Availability
All datasets generated for this study are included in the manuscript and/or the Supplementary Files.
Author Contributions
AN designed the study, performed the experiments, analyzed the data, and wrote the manuscript. AM conducted the experiments and analyzed the data. CT contributed to data analysis and writing the manuscript. GB analyzed the data and contributed to writing the manuscript.
Funding
This work was partially supported by the European Regional Development Fund (ERDF) and National Resources, under the Operational Program Competitiveness and Entrepreneurship (EPAN II), Action “COOPERATION 2011” (Grant No. 11SYN_2_704).
Conflict of Interest Statement
The authors declare that the research was conducted in the absence of any commercial or financial relationships that could be construed as a potential conflict of interest.
Acknowledgments
The authors would like to thank “DOMAIN SPYROPOULOS” winery for their assistance in the pilot scale fermentations. Dimitra Dourou and George Sgouros are gratefully acknowledged for technical assistance.
Supplementary Material
The Supplementary Material for this article can be found online at: https://www.frontiersin.org/articles/10.3389/fmicb.2019.01712/full#supplementary-material
References
Albergaria, H., and Arneborg, N. (2016). Dominance of Saccharomyces cerevisiae in alcoholic fermentation processes: role of physiological fitness and microbial interactions. Appl. Microbiol. Biotechnol. 100, 2035–2046. doi: 10.1007/s00253-015-7255-0
Albertin, W., Miot-Sertier, C., Bely, M., Mostert, T. T., Colonna-Ceccaldi, B., Coulon, J., et al. (2016). Hanseniaspora uvarum from winemaking environments show spatial and temporal genetic clustering. Front. Microbiol. 6:1569. doi: 10.3389/fmicb.2015.01569
Andorrà, I., Berradre, M., Mas, A., Esteve-Zarzoso, B., and Guillamón, J. M. (2012). Effect of mixed culture fermentations on yeast populations and aroma profile. LWT Food Sci. Technol. 49, 8–13. doi: 10.1016/j.lwt.2012.04.008
Balikci, E. K., Tanguler, H., Jolly, N. P., and Erten, H. (2016). Influence of Lachancea thermotolerans on cv. Emir wine fermentation. Yeast 33, 313–321. doi: 10.1002/yea.3166
Banilas, G., Sgouros, G., and Nisiotou, A. (2016). Development of microsatellite markers for Lachancea thermotolerans typing and population structure of wine-associated isolates. Microbiol. Res. 193, 1–10. doi: 10.1016/j.micres.2016.08.010
Barquet, M., Martin, V., Medina, K., Perez, G., Carrau, F., and Gaggero, C. (2012). Tandem repeat-tRNA (TRtRNA) PCR method for the molecular typing of non-Saccharomyces subspecies. Appl. Microbiol. Biotechnol. 93, 807–814. doi: 10.1007/s00253-011-3714-4
Beckner Whitener, M. E., Stanstrup, J., Panzeri, V., Carlin, S., Divol, B., Du Toit, M., et al. (2016). Untangling the wine metabolome by combining untargeted SPME–GCxGC-TOF-MS and sensory analysis to profile Sauvignon blanc co-fermented with seven different yeasts. Metabolomics 12:53. doi: 10.1007/s11306-016-0962-964
Benito, A., Calderón, F., Palomero, F., and Benito, S. (2015). Combine use of selected Schizosaccharomyces pombe and Lachancea thermotolerans yeast strains as an alternative to the traditional malolactic fermentation in red wine production. Molecules 20, 9510–9523. doi: 10.3390/molecules20069510
Benito, A., Calderón, F., Palomero, F., and Benito, S. (2016). Quality and composition of Airén wines fermented by sequential inoculation of Lachancea thermotolerans and Saccharomyces cerevisiae. Food Technol. Biotechnol. 54, 135–144.
Bokulich, N. A., Thorngate, J. H., Richardson, P. M., and Mills, D. A. (2014). Microbial biogeography of wine grapes is conditioned by cultivar, vintage, and climate. PNAS 111, E139–E148. doi: 10.1073/pnas.1317377110
Ciani, M., Beco, L., and Comitini, F. (2006). Fermentation behaviour and metabolic interactions of multistarter wine yeast fermentations. Int. J. Food Microbiol. 108, 239–245. doi: 10.1016/j.ijfoodmicro.2005.11.012
Comitini, F., Gobbi, M., Domizio, P., Romani, C., Lencioni, L., Mannazzu, I., et al. (2011). Selected non-Saccharomyces wine yeasts in controlled multistarter fermentations with Saccharomyces cerevisiae. Food Microbiol. 28, 873–882. doi: 10.1016/j.fm.2010.12.001
Cordero-Bueso, G., Esteve-Zarzoso, B., Cabellos, J. M., Gil-Diaz, M., and Arroyo, T. (2012). Biotechnological potential of non-Saccharomyces yeasts isolated during spontaneous fermentations of Malvar (Vitis vinifera cv. L.). Eur. Food Res. Technol. 236, 193–207. doi: 10.1007/s00217-012-1874-9
Drumonde-Neves, J., Franco-Duarte, R., Lima, T., Schuller, D., and Pais, C. (2016). Yeast biodiversity in vineyard environments is increased by human intervention. PLoS One 11:e0160579. doi: 10.1371/journal.pone.0160579
du Toit, M., and Pretorius, I. (2000). Microbial spoilage and preservation of wine: using weapons from Nature’s own arsenal-A review. S. Afr. J. Enol. Vitic. 21, 74–96.
Fleet, G. H. (2003). Yeast interactions and wine flavour. Int. J. Food Microbiol. 86, 11–22. doi: 10.1016/s0168-1605(03)00245-9
Fleet, G. H. (2008). Wine yeasts for the future. FEMS Yeast Res. 8, 979–995. doi: 10.1111/j.1567-1364.2008.00427.x
Gayevskiy, V., and Goddard, M. R. (2012). Geographic delineations of yeast communities and populations associated with vines and wines in New Zealand. ISME J. 6, 1281–1290. doi: 10.1038/ismej.2011.195
Gobbi, M., Comitini, F., Domizio, P., Romani, C., Lencioni, L., Mannazzu, I., et al. (2013). Lachancea thermotolerans and Saccharomyces cerevisiae in simultaneous and sequential co-fermentation: a strategy to enhance acidity and improve the overall quality of wine. Food Microbiol. 33, 271–281. doi: 10.1016/j.fm.2012.10.004
Guillamón, J. M., and Barrio, E. (2017). Genetic polymorphism in wine yeasts: mechanisms and methods for its detection. Front. Microbiol. 8:806. doi: 10.3389/fmicb.2017.00806
Gump, B. H., Zoecklein, B. W., and Fugelsang, K. C. (2001). “Food Microbiology Protocols,” in Methods in Biotechnology, eds J. F. T. Spencer and A. L. Ragout de Spencer (Totowa, NY: Humana Press), 283–296.
Guth, H. (1997). Quantitation and sensory studies of character impact odorants of different white wine varieties. J. Agric. Food Chem. 45, 3027–3032. doi: 10.1021/jf970280a
Hammer, Ø., Harper, D. A. T., and Ryan, P. D. (2001). PAST: paleontological statistics software package for education and data analysis. Palaeontol. Electronica 4, 1–9.
Hjelmeland, A. K., King, E. S., Ebeler, S. E., and Heymann, H. (2013). Characterizing the chemical and sensory profiles of United States Cabernet Sauvignon wines and blends. Am. J. Enol. Vitic. 64, 169–179. doi: 10.5344/ajev.2012.12107
Hranilovic, A. M., Gambetta, J., Schmidtke, L., Boss, P. R., Grbin, P., Masneuf-Pomarede, I., et al. (2018). Oenological traits of Lachancea thermotolerans show signs of domestication and allopatric differentiation. Sci. Rep. 8:14812. doi: 10.1038/s41598-018-33105-7
Hu, K., Jin, G.-J., Mei, W. C., Li, T., and Tao, Y.-S. (2018). Increase of medium-chain fatty acid ethyl ester content in mixed H. uvarum/S. cerevisiae fermentation leads to wine fruity aroma enhancement. Food Chem. 239, 495–501. doi: 10.1016/j.foodchem.2017.06.151
Jolly, N. P., Varela, C., and Pretorius, I. S. (2014). Not your ordinary yeast: non Saccharomyces yeasts in wine production uncovered. FEMS Yeast Res. 14, 215–237. doi: 10.1111/1567-1364.12111
Kapsopoulou, K., Mourtzini, A., Anthoulas, M., and Nerantzis, E. (2007). Biological acidification during grape must fermentation using mixed cultures of Kluyveromyces thermotolerans and Saccharomyces cerevisiae. World J. Microbiol. Biotechnol. 23, 735–739. doi: 10.1007/s11274-006-9283-5
Kemsawasd, V., Branco, P., Almeida, M. G., Caldeira, J., Albergaria, H., and Arneborg, N. (2015). Cell-to-cell contact and antimicrobial peptides play a combined role in the death of Lachanchea thermotolerans during mixed-culture alcoholic fermentation with Saccharomyces cerevisiae. FEMS Microbiol. Lett. 362:fnv103. doi: 10.1093/femsle/fnv103
Knight, S., Klaere, S., Fedrizzi, B., and Goddard, M. R. (2015). Regional microbial signatures positively correlate with differential wine phenotypes: evidence for a microbial aspect to terroir. Sci. Rep. 5:14233. doi: 10.1038/srep14233
Lambrechts, M. G., and Pretorius, I. S. (2000). Yeast and its importance to wine aroma - A review. S. Afr. J. Enol. Vitic. 21, 97–129.
Legras, J. L., and Karst, F. (2003). Optimisation of interdelta analysis for Saccharomyces cerevisiae strain characterization. FEMS Microbiol. Lett. 221, 249–255. doi: 10.1016/s0378-1097(03)00205-2
Mannazzu, I., Clementi, F., and Ciani, M. (2002). “Strategies and criteria for the isolation and selection of autochthonous starters,” in Biodiversity and Biotechnology of Wine Yeasts, ed. M. Ciani (Trivandrum: Research Signpost), 19–34.
Martin, V., Valera, M. J., Medina, K., Boido, E., and Carrau, F. (2018). Oenological Impact of the Hanseniaspora/Kloeckera yeast genus on wines—a review. Fermentation 4:76. doi: 10.3390/fermentation4030076
Metafa, M., and Economou, A. (2013). Chemometrical development and comprehensive validation of a solid phase microextraction/gas chromatography–mass spectrometry methodology for the determination of important free and bound primary aromatics in Greek wines. J. Chromatogr. A 1305, 244–258. doi: 10.1016/j.chroma.2013.07.005
Morales, M. L., Fierro-Risco, J., Callejoìn, R. M., and Paneque, P. (2017). Monitoring volatile compounds production throughout fermentation by Saccharomyces and non-Saccharomyces strains using headspace sorptive extraction. J. Food Sci. Technol. 54, 538–557. doi: 10.1007/s13197-017-2499-6
Morata, A., Loira, I., Tesfaye, W., Bañuelos, M. A., González, C., and Suárez-Lepé, J. A. (2018). Lachancea thermotolerans applications in wine technology. Fermentation 4:53. doi: 10.3390/fermentation4030053
Nisiotou, A. A., and Nychas, G.-J. E. (2007). Yeast populations residing on healthy or Botrytis-infected grapes from a vineyard in Attica, Greece. Appl. Environ. Microbiol. 73, 2765–2768. doi: 10.1128/aem.01864-06
Nisiotou, A. A., Sgouros, G., Mallouchos, A., Nisiotis, C. S., Michaelidis, C., Tassou, C., et al. (2018). The use of indigenous Saccharomyces cerevisiae and Starmerella bacillaris strains as a tool to create chemical complexity in local wines. Food Res. Int. 111, 498–508. doi: 10.1016/j.foodres.2018.05.035
Nisiotou, A. A., Spiropoulos, A. E., and Nychas, G. J. E. (2007). Yeast community structures and dynamics in healthy and Botrytis-affected grape must fermentations. Appl. Environ. Microbiol. 73, 6705–6713. doi: 10.1128/aem.01279-07
OIV (2015). Compendium of International Methods of Wine and Must Analysis. Paris: France International Organisation of Vine and Wine (OIV).
Petruzzi, L., Capozzi, V., Berbegal, C., Corbo, M. R., Bevilacqua, A., Spano, G., et al. (2017). Microbial resources and enological significance: opportunities and benefits. Front. Microbiol. 8:995. doi: 10.3389/fmicb.2017.00995
Pineau, B., Barbe, J. C., Van Leeuwen, C., and Dubourdieu, D. (2007). Which impact for beta-damascenone on red wines aroma? J. Agric. Food Chem. 55, 4103–4108. doi: 10.1021/jf070120r
Rainieri, S., and Pretorius, I. S. (2000). Selection and improvement of wine yeasts. Ann. Microbiol. 50, 15–31.
Rementeria, A., Rodriguez, J. A., Cadaval, A., Amenabar, R., Muguruza, J. R., Hernando, F. L., et al. (2003). Yeast associated with spontaneous fermentations of white wines from the “Txakoli de Bizkaia” region (Basque Country, North Spain). Int. J. Food Microbiol. 86, 201–207. doi: 10.1016/s0168-1605(03)00289-7
Rollero, S., Bloem, A., Camarasa, C., Sanchez, I., Ortiz-Julien, A., Sablayrolles, J.-M., et al. (2015). Combined effects of nutrients and temperature on the production of fermentative aromas by Saccharomyces cerevisiae during wine fermentation. Appl. Microbiol. Biotechnol. 99, 2291–2304. doi: 10.1007/s00253-014-6210-9
Roudil, L., Russo, P., Berbegal, C., Albertin, W., Spano, G., and Capozzi, V. (2019). Non-Saccharomyces commercial starter cultures: scientific trends, recent patents and innovation in the wine sector. Recent Pat. Food Nutr. Agric. 10, 1–13. doi: 10.2174/2212798410666190131103713
Saerens, S. M. G., Delvaux, F., Verstrepen, K. J., Van Dijck, P., Thevelein, J. M., and Delvaux, F. R. (2008). Parameters affecting ethyl ester production by Saccharomyces cerevisiae during fermentation. Appl. Environ.Microbiol. 74, 454–461. doi: 10.1128/aem.01616-07
Sgouros, G., Chalvantzi, I., Mallouchos, A., Paraskevopoulos, Y., Banilas, G., and Nisiotou, A. (2018). Biodiversity and enological potential of non-Saccharomyces yeasts from Nemean vineyards. Fermentation 4:32. doi: 10.3390/fermentation4020032
Sumby, K. M., Grbin, P. R., and Jiranek, V. (2010). Microbial modulation of aromatic esters in wine: current knowledge and future prospects. Food Chem. 121, 1–16. doi: 10.1016/j.foodchem.2009.12.004
Tristezza, M., Tufariello, M., Capozzi, V., Spano, G., Mita, G., and Grieco, F. (2016). The oenological potential of Hanseniaspora uvarum in simultaneous and sequential co-fermentation with Saccharomyces cerevisiae for industrial wine production. Front. Microbiol. 7:670. doi: 10.3389/fmicb.2016.00670
Ugliano, M., and Henschke, P. A. (2009). “Yeasts and Wine Flavour,” in Wine Chemistry and Biochemistry, eds M. V. Moreno-Arribas and M. C. Polo (New York, NY: Springer-Verlag), 313–392. doi: 10.1007/978-0-387-74118-5_17
Vaudour, E., Costantini, E., Jones, G. V., and Mocali, S. (2015). An overview of the recent approaches to terroir functional modelling, footprinting and zoning. Soil 1, 287–312. doi: 10.5194/soil-1-287-2015
Wang, C., Mas, A., and Esteve-Zarzoso, B. (2015). Interaction between Hanseniaspora uvarum and Saccharomyces cerevisiae during alcoholic fermentation. Int. J. Food Microbiol. 206, 67–74. doi: 10.1016/j.ijfoodmicro.2015.04.022
Keywords: non-Saccharomyces, wine fermentation, yeast starter cultures, Hanseniaspora uvarum, Lachancea thermotolerans, wine chemical profile, volatiles
Citation: Nisiotou A, Mallouchos A, Tassou C and Banilas G (2019) Indigenous Yeast Interactions in Dual-Starter Fermentations May Improve the Varietal Expression of Moschofilero Wine. Front. Microbiol. 10:1712. doi: 10.3389/fmicb.2019.01712
Received: 12 April 2019; Accepted: 11 July 2019;
Published: 26 July 2019.
Edited by:
Matthias Sipiczki, University of Debrecen, HungaryReviewed by:
Gustavo Cordero-Bueso, University of Cádiz, SpainAngela Capece, University of Basilicata, Italy
Ilaria Mannazzu, University of Sassari, Italy
Francisco Carrau, Universidad de la República, Uruguay
Copyright © 2019 Nisiotou, Mallouchos, Tassou and Banilas. This is an open-access article distributed under the terms of the Creative Commons Attribution License (CC BY). The use, distribution or reproduction in other forums is permitted, provided the original author(s) and the copyright owner(s) are credited and that the original publication in this journal is cited, in accordance with accepted academic practice. No use, distribution or reproduction is permitted which does not comply with these terms.
*Correspondence: Aspasia Nisiotou, anisiotou.wi@nagref.gr