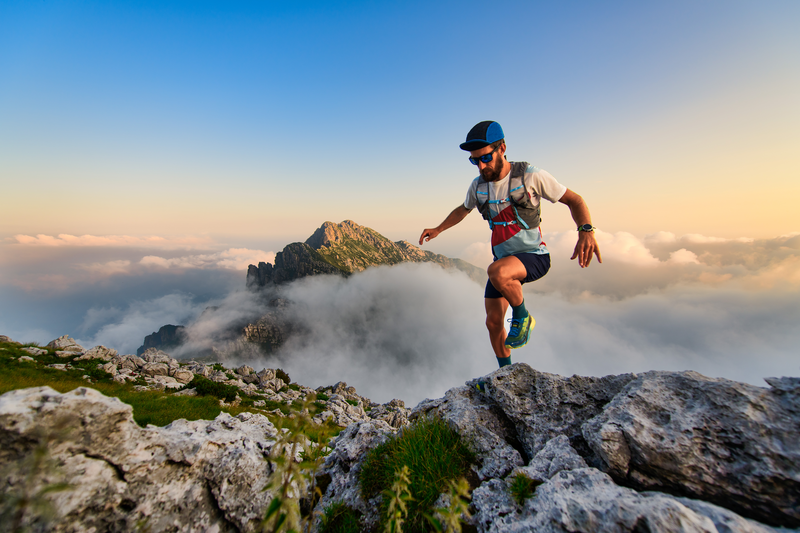
94% of researchers rate our articles as excellent or good
Learn more about the work of our research integrity team to safeguard the quality of each article we publish.
Find out more
ORIGINAL RESEARCH article
Front. Microbiol. , 10 June 2019
Sec. Food Microbiology
Volume 10 - 2019 | https://doi.org/10.3389/fmicb.2019.01275
This article is part of the Research Topic Food Safety and Foodborne Pathogen – A Global Perspective on the Diversity, Combating Multidrug Resistance and Management View all 39 articles
The pressure-assisted ultra-high temperature (PAUHT) system was built by using soybean oil as pressure-transmitting medium, and the multiple regression equation of soybean oil temperature change (ΔTP) during pressurization as a function of initial temperature (Ti) and set pressure (P) was developed: ΔTP = -13.45 + 0.46 Ti + 0.0799 P - 0.0037 - 2.83 × 10-5 P2. The fitted model indicated that the temperature of the system would achieve ≥121°C at 600 MPa when the initial temperature of soybean oil was ≥84°C. The PAUHT system could effectively inactivate spores of Bacillus subtilis 168 and Clostridium sporogenes PA3679 (less than 1 min). Treatment of 600 MPa and 121°C with no holding time resulted in a 6.75 log reductions of B. subtilis 168 spores, while treatment of 700 MPa and 121°C with pressure holding time of 20 s achieved more than 5 log reductions of C. sporogenes PA3679 spores. By comparing the PAUHT treatment with high pressure or thermal treatment alone, and also studying the effect of compression on spore inactivation during PAUHT treatment, the inactivation mechanism was further discussed and could be concluded as follows: both B. subtilis 168 and C. sporogenes PA3679 spores were triggered to germinate firstly by high pressure, which was enhanced by increased temperature, then the germinated spores were inactivated by heat.
Pressure assisted thermal sterilization (PATS), a technique combining high pressure with elevated temperature, has been used to effectively inactivate bacterial spores in low-acid food (Peleg et al., 2008; Nguyen et al., 2010; Olivier et al., 2011). Adiabatic compression caused by pressurization results in quick heating of the product to process temperatures, and the subsequent decompression results in its rapid cooling. Therefore, PATS can be conducted in a short time based on quick heating/cooling (Paidhungat et al., 2002; Ardia et al., 2004; Zhu et al., 2008; Reineke et al., 2013c). The overall treatment conditions are less severe than with conventional sterilization (Reineke et al., 2011; Doona et al., 2016a). All these attributes can lead to a better and healthier product, because unwanted reactions, like the Maillard reaction which are temperature and time dependent, might not occur or can be reduced by high pressure.
Generally, when the treatment was pressure of P = 600–800 MPa and initial temperature of Ti = 90–120°C, all spores could be inactivated (Margosch et al., 2006; Wilson et al., 2008; Lopes et al., 2018). However, studies on PATS usually use water as pressure-transmitting medium and the final temperature is usually less than 121°C after compression heating, and it still requires several minutes (> 3 min) to inactivate all spores (Margosch et al., 2004b; Ramaswamy and Balasubramaniam, 2007; Shao and Ramaswamy, 2008; Doona et al., 2017). In order to achieve higher ration of spore inactivation in shorter time, higher pressure and temperature could be a good choice for the PATS processing (Shao and Ramaswamy, 2008; Wang et al., 2017). Nowadays, studies on spore inactivation by PATS with final temperature more than 121°C are relatively limited. In order to achieve higher temperature during PATS treatment, we supposed that the soybean oil could be used as the pressure-transmitting medium because of its higher compression heating coefficient (9.2°C/100 MPa) (Patazca et al., 2007; Zhu et al., 2008). This PATS system with higher working temperature could be defined as pressure-assistant ultra-high temperature (PAUHT) system. This system is rarely built and its inactivation effect and mechanism of bacterial spores has never been investigated.
The mechanism of spore inactivation by PATS has been extensively studied (Mathys et al., 2009; Ramaswamy et al., 2013; Reddy et al., 2016). Generally, it is proposed that spores are firstly induced to germinate and lose resistance, and then inactivated by heat. However, spore germination induced by PATS is different due to different treatment conditions and species of spores. For spores of Bacillus species, the germination is different between moderate pressure (200–500 MPa) and higher pressure (> 500 MPa) (Reineke et al., 2013b). At moderate pressure, spores are induced to germinate by activating the germinant receptors (GRs) and ensuing the release of DPA (Paidhungat et al., 2002; Setlow, 2003; Vepachedu and Setlow, 2007; Doona et al., 2014). DPA release activates one of the cortex lytic enzymes (CLEs), CwlJ, and induces spore core partial hydration, which may activate another CLEs, SleB (Setlow et al., 2009; Paredes-Sabja et al., 2011). These two activated CLEs degrade the cortex, resulting in completion of spore germination (Li et al., 2013). However, the higher pressure (> 500 MPa) induced germination was triggered by directly opening the DPA channel, SpoVA, without activating the GRs (Elke and Wuytack, 2001; Vercammen et al., 2012; Reineke et al., 2013c; Sevenich et al., 2013; Kong et al., 2014; Sarker et al., 2015). Margosch and others proposed a spore inactivation mechanism by PATS that is not involved spore germination. These researchers suggest that the inactivation mechanism of B. subtilis and Bacillus licheniformis spores by PATS at temperature higher than 70°C follows a two-stage strategy: (i) DPA is released by a short HHP pulse at high temperature and (ii) then spores are thermally inactivated-independent of pressure level upon depressurization (Margosch et al., 2004a). For Clostridial spores, Doona and others reported Clostridium perfringens spores with GRs exhibit similar germination-inactivation mechanism to spores of Bacillus species when treated by PATS (Doona et al., 2016a,b). However, for Clostridium difficile spores without GRs, spores were not germinated by pressure of 150 MPa. In contrast, the pressure of 550 MPa induced spores to release DPA, but the spores did not complete germination and remained heat resistant. As for C. sporogenes spores with GRs similar to C. perfringens spores (Setlow et al., 2017), they can theoretically germinate during PATS treatment and lose heat resistance, but it has never been verified.
The objectives of this study were to (1) build the PAUHT system with soybean oil as pressure-transmitting medium, (2) investigate the inactivation of PAUHT on B. subtilis 168 and C. sporogenes PA3679 spores, and (3) study the inactivation mechanism of spores under the condition of PAUHT.
The bacterial strains used in the study were B. subtilis 168, FB85 (without GRs, a derivative of strain 168) (Paidhungat and Setlow, 2000) and C. sporogenes PA3679. The B. subtilis 168 and C. sporogenes PA3679 were obtained from China General Microbiology Culture Collection Center and China Center of Industrial Culture Collection, respectively. FB85 was a gift from Prof. Peter Setlow. B. subtilis 168 and FB85 strains were grown in Luria Bertani broth medium. Spores were prepared at 37°C by plating aliquots of 0.2 mL from the fresh overnight culture on the 2 × Schaeffer’s glucose medium agar plates without antibiotics as described previously (Paidhungat and Setlow, 2000; Luu et al., 2015). The spores were harvested after 2 days of incubation when over 90% of the spores were released from the mother cell as observed by phase contrast microscopy (Axio Observer. A1, Carl Zeiss, Germany). The spore suspension was cleaned by repeated centrifugation (8000 × g, 10 min, 4°C) for at least five times with cold distilled water (4°C). Then, the spores were cleaned using the histodenz gradient centrifugation and washed three times with sterilized water. Finally, the spore suspensions were stored in the dark at 4°C and were 98% free of growing or sporulating cells, germinated spores, and cell debris as shown in Supplementary Figure S1 observed by phase-contrast microscope (Setlow, 2018).
Clostridium sporogenes PA3679 was grown anaerobically in the Reinforce Clostridial Medium (RCM) broth in anaerobic jars with an Oxoid GasPak sachet (Oxoid, Basingstoke, United Kingdom) for 2 days at 37°C. An aliquot (0.2 mL) of C. sporogenes PA3679 culture was spread on the RCM plate and incubated at 37°C anaerobically for more than 5 days. The spores were harvested when over 90% of the spores were released from the mother cell observed by phase contrast microscopy. Then, the spore suspension was cleaned by centrifugation same as spores of B. subtilis strains. Finally, the spores were suspended in the sterilized water and stored at 4°C for the subsequently use. The concentration of the spore suspensions was adjusted to approximate 108 CFU/mL before treatments.
High pressure treatment was carried out with an experimental setup as show in Figure 1. The HP device (FPG 7100, Stansted Fluid Power Ltd., Essex, United Kingdom) had a 2 L cylindrical pressure chamber (70 mm in diameter and 500 mm in height) with a maximum working pressure of 900 MPa. The pressure chamber was surrounded by a heating sleeve and the maximum temperature was 110°C. A data logger connected with T-type thermocouples (Omega Engineering, CT, United States) was used to gather the in situ pressure and temperature data. The medium used for pressure transmission in the system was 30% propylene glycol.
Figure 1. Schematic diagram of the high-pressure experimental system: T1 and T2 are thermocouples in soybean oil insulator medium and high hydrostatic pressure vessel medium, respectively.
A polyoxymethylene (POM) plastic insulated chamber (18 mm inner diameter, 50 mm outer diameter, and 200 mm height) was used to hold soybean oil and the sample during the pressure treatment to provide the temperature stability as described elsewhere (Shao and Ramaswamy, 2008). The soybean oil was filled into the insulator and used as the pressurization medium to get desired temperature by compression heating.
Two mL of spore suspensions were packed in the sterilized polyamides/polyethylene plastic bags (4 cm × 4 cm) by heat sealer. The insulator and spore sample were preheated to the initial temperature prior HHP treatment. HHP treatment was carried out immediately after the spore was placed into the insulator. The compression rate was ∼350 MPa/min, and the depressurization time was less than 5 s at all pressure levels. Different pressure levels (600 and 700 MPa) combined with temperatures (121, 125, and 130°C) were set for the treatments. The treated samples were then cooled in an ice bath and immediately analyzed.
Capillary tubes (0.9 mm inner diameter, 1 mm diameter, and 100 mm length) were used for the thermal treatments at 121, 125, and 130°C in the methyl silicone oil bath by a heating circulator. Twenty μL of spore suspension was injected into the tubes by micropipette and sealed by flame as described elsewhere (Sapru et al., 1992). After heating, capillary tubes were cooled immediately in ice water, cleaned with 70% ethyl alcohol for 10 min, and washed with sterilized water. The spore suspension was flushed out with a pipette and analyzed.
After different treatments, the DPA release was determined by measuring the fluorescence of DPA with Tb3+ using a multi-well 96 fluorescence plate reader (Spark 10 M, Tecan) as described elsewhere (Yi and Setlow, 2010). After treatment, 50 μL spore suspension were mixed in the HEPES-TbCl3 solution, which consisted of 50 mM HEPES (pH 7.4) buffer and 200 μM TbCl3, the volume was made to 200 μL. Analytic grade water was used for the preparation of the buffer solution. DPA release was determined by the measurement of fluorescence emission at 545 nm with excitation at 270 nm. The maximum amount of DPA in the spore suspensions was determined by a thermal treatment for 20 min at 121°C.
Bacillus subtilis and C. sporogenes spore counts were determined by pour-plate enumeration in duplicate, using the nutrient agar and RCM agar, respectively. One milliliter dilution of spore suspension was added into each plate. The B. subtilis plate was incubated aerobically at 37°C for 24 h, while the C. sporogenes plate was incubated anaerobically at 37°C for 36 h before enumeration. The untreated samples were used as a control for each experiment in order to obtain the initial spore counts. The logarithm of survivors [log10 (N0/Nt)] was used as the spore reduction after different treatments. N0 and Nt were the spore counts before and after treatment, respectively. The initial concentration of the spore suspensions was adjusted to approximate 108 CFU/mL before treatments.
After different treatments, the spore suspensions (OD600 = 1.0) were double stained with 0.5 μM SYTO16 (Invitrogen, Carlsbad, CA, United States) and 15 μM propidium iodide (PI) (Invitrogen, Carlsbad, CA, United States). Suspensions were vigorously mixed and incubated in the dark for 15 min prior to analysis as described previously (Reineke et al., 2013a). Both fluorescent dyes are able to stain DNA, the membrane permeant SYTO 16 acts as an indicator for spore germination, whereas the membrane impermeant PI indicates membrane damage (Mathys et al., 2009). The spores were observed by phase contrast and fluorescence microscopy (Axio Observer. A1, Carl Zeiss, Germany).
All experiments were carried out in triplicate and data were presented as mean ± standard deviation. The one-way analysis of variance was used to test the statistically significant differences (P < 0.05) between treatments using software SPSS 17 for windows (SPSS Statistical Software, Inc., Chicago, IL, United States). Software Origin 7.5 (Origin Lab, MA, United States) was used for making plots and fitting mathematical model.
Under compression during HHP treatment, the increased internal energy of the system resulted in a rapid rise in temperature (Ramaswamy and Balasubramaniam, 2007; Shao et al., 2010). In this PAUHT system, soybean oil was used as the pressure-transmitting medium to get the desired ultra-high temperature by increasing the initial temperature with compression heating. Figure 2 showed a typical temperature change of the soybean oil with different initial temperature during PAUHT treatment. When the initial temperature of soybean oil was set at 86°C, the final temperature reached 121°C with pressure of 600 MPa (Figure 2A). Under 700 MPa (Figure 2B), the final temperature of the medium reached to 130°C when the initial temperature was set at 90°C. The temperature increase (30–40°C) of soybean oil during PAUHT treatment was much more than that (11–16°C) of the 30% propylene glycol, indicating that the soybean oil has a better compression heating behavior than the 30% propylene glycol, which was consistent with the results previously reported (Houška et al., 2004; Min et al., 2010). Moreover, during the pressure holding time, the temperature of soybean oil remains almost unchanged in the insulator, indicating the POM material can be used for the insulator to remain the temperature stable. All of the results above suggested that the PAUHT constructed with soybean oil as pressure-transmitting medium has the capability to reach ultra-high temperature up to 130°C with excellent stability during the process, hence it could be used as a reliable tool for the follow-up studies.
Figure 2. Typical pressure–temperature profiles of soybean oil during PAUHT treatments: (A) 600 MPa and 121°C (1 min), and (B) 700 MPa and 130°C (1 min).
In order to predict the temperature change (ΔTP) of soybean oil during PAUHT treatment, 100 data points from the measurements were pooled to develop a multiple regression equation of ΔTP of soybean oil during pressurization as a function of initial temperature (Ti) of soybean oil and set pressure (P). It could be expressed as a quadratic function of P and Ti as follows:
(R2adj = 0.993, p < 0.05 for all items).
Where Ti = initial temperature (50°C < Ti < 90°C), P = pressure (100 MPa < P < 700 MPa), Tp = target temperature (°C), ΔTP = temperature change (°C), R2adj = adjusted R square, p = statistic parameter of significance.
When desired PAUHT conditions were given, the initial temperature of soybean oil could also be calculated by the following equation:
(R2adj = 0.993, p < 0.05 for all items).
The parameters of these equations were determined by enter regression procedure. The adjusted regression coefficient (R2adj) value was used to measure the performance of the proposed model. Eq. (2) can be used to calculate the initial temperature under different combinations of Tp and P-values.
The used conditions were listed in Table 1. The high temperature of 121°C can be achieved under PAUHT at 600 MPa commonly used in industry, as long as the initial temperature of soybean oil was 84°C. The verification experiment was done to test the proposed model. When the initial temperature was 84°C at 600 MPa, the final temperature of soybean oil can reach to 120.86°C, which was within the range of the confidence interval. As a result, this equation can be used to predict the initial temperature of soybean oil for the PAUHT treatments.
The inactivation of B. subtilis 168 and C. sporogenes PA3679 spores under different PAUHT treatments were shown in Table 2. The treatment of 600 MPa at 121°C without pressure holding time resulted in 6.75 log and 2.56 log reductions of B. subtilis 168 and C. sporogenes PA 3679 spores, respectively, indicating that spores of C. sporogenes PA 3679 were more resistant to pressure and temperature than spores of B. subtilis 168, as reported previously (Wilson et al., 2008; Ramaswamy et al., 2013). Moreover, when the temperature increased to 125°C or hold time up to 1 min, the amount of B. subtilis 168 spores was below the detection line, and the inactivation of C. sporogenes PA3679 spores was also increased. These results indicated the PAUHT treatments could effectively inactivate B. subtilis 168 and C. sporogenes PA3679 spores with less than 1 min of holding time. During pressure holding time for 1 min, the treatment of 600 MPa at 121°C resulted in 3.58 log reductions of C. sporogenes PA3679 spores. Increasing the pressure to 700 MPa, the treatment at 121°C for 1 min of holding time resulted in 6.31 log destructions of C. sporogenes PA3679 spores. Moreover, increasing the temperature to 125°C, the treatment of 600 MPa for 1 min of holding time induced 8.92 log C. sporogenes PA3679 spore inactivation. These results indicated that increase of pressure and temperature significantly accelerated the spore inactivation during pressure holding time of PAUHT treatments, which was consistent with previous report (Ahn et al., 2007). In addition, during PAUHT treatment, a compression of 120–140 s (∼5 MPa/s) was needed to arrive the final pressure of P = 600–700 MPa (Figure 2). Even without pressure holding time, 6.75 log and 2.56 log reductions of B. subtilis 168 and C. sporogenes PA3679 spores were achieved at 121°C and 600 MPa, respectively. Moreover, the treatment of 700 MPa at 121°C without pressure holding time resulted in 8.85 log (under detection line) and 4.53 log destructions of B. subtilis 168 and C. sporogenes PA3679 spores, respectively (Table 2). These results indicated that the compression played an important role in spore inactivation and should be taken into consideration during PAUHT treatments. Notably, the treatment of 700 MPa for 20 s at 121°C resulted in more than 5 log inactivation of C. sporogenes PA3679 spores. A 5 log reduction is regarded as the commercial sterilization standard for C. sporogenes PA3679 spores by food industry and regulatory agencies due to the fact that C. sporogenes spores are more heat resistant than C. botulinum spores (USFDA-FSIS, 2005; Ramaswamy et al., 2013; Reddy et al., 2016). Moreover, thermal sterilization required a process at 121.1°C for over 2.52 min to reach the goal of commercial sterilization (Theron and Irving, 1977), while using the PAUHT processing (700 MPa, 121°C) proposed here a processing time of 20 s was needed for the same effect, thus the PAUHT processing was more time efficient than thermal processing. However, although PAUHT treatment at temperature higher than 121°C could be more efficient at inactivation spores (Table 2, 600 MPa/125°C/0 s, 700 MPa/130°C/0 s), this higher temperatures would also produce unwanted side-reactions and degrades food quality (Lau and Turek, 2007). Hence, the following studies were mainly focused on the PAUHT treatment at 121°C.
Table 2. The inactivation and DPA release of B. subtilis 168 and C. sporogenes PA 3679 spores after different treatments.
Nowadays, the discussion about the mechanism of spore inactivation by PATS is mainly focused on whether spores undergo germination during inactivation by PATS treatment (Reineke et al., 2013b). Similarly, whether spores undergo germination during PAUHT treatment is the key point of figuring out how PAUHT inactivates them. In order to understand the mechanism of spore inactivation during PAUHT treatments, the structure changes of the B. subtilis 168 and C. sporogenes PA3679 spores during the process were investigated by staining the spores with PI and SYTO 16 after treatment, followed by observation using phase contrast microscopy and fluorescence microscopy. As shown in Figure 3, after PAUHT process, both B. subtilis 168 and C. sporogenes PA3679 spores turned from bright to gray, and could be stained by PI and SYTO 16 (Figures 3C,F). As PI and SYTO 16 staining were usually considered as the indicator of the integrity of spore’s IM and cortex (Mathys et al., 2007; Kong et al., 2010), respectively, we could ensure that the PAUHT treated spores displayed damaged IM and cortex. Moreover, more than 90% DPA released after the PAUHT treatment (Table 2), further confirming that the IM was damaged (Reineke et al., 2013a). However, we noticed that PAUHT treated spores turned gray rather than dark, indicating that the cores of these spores were only partially hydrated (Katja et al., 2013). Since the cortex played a key role in maintaining the core rehydration and germinated spores would degrade their cortex and turn dark (Figures 3B,E), it could be concluded that the cortex of PAUHT treated spores were only partially damaged. From these results, we could conclude that PAUHT-inactivated spores had damaged IM and partially damaged cortex, but we couldn’t tell whether spores went through germination because (1) heat-inactivated spores had a damaged IM and partially damaged cortex similar to PAUHT-inactivated spores, but didn’t go through germination (Setlow, 2006), and (2) spores that went through stage I of germination but didn’t accomplish the stage II of germination could be inactivated by PAUHT, and also exhibited damaged IM and partially damaged cortex.
Figure 3. The phase contrast and fluorescence microscope of B. subtilis168 (A–C) and C. sporogenes PA3679 (D–F) spores: (A,D) untreated spores, (C,F) 600 MPa at 121°C for 1 min, (B) 600 MPa at ambient temperature for 10 min, (E) Incubation in 20 mM Tris buffer pH 7.4, L-alanine (100 mM), L-lactate (50 mM), and NaHCO3 at 30°C for 1 h.
Since the temperature during PAUHT was relatively high and it could play an important role in inactivation spores, we investigated the effect of heat treatment at temperatures same to PAUHT on spore inactivation. B. subtilis 168 and C. sporogenes PA3679 spores were treated at ambient pressure at 121°C for different times (10, 20, and 60 s), then the spores were stained by PI and SYTO 16 and observed by microscopy as described above. Thermal treatment of 121°C at 0.1 MPa for 1 min could induce 5.23 log and 2.51 log inactivation and DPA release (more than 90%) of B. subtilis 168 and C. sporogenes PA 3679 spores, respectively (Figure 4), indicating the temperature played an important role in spore inactivation during pressure holding time of PAUHT treatment. Moreover, compared to the untreated (Figures 5A,D) and germinated spores (Figures 5C,F), these heat treated spores were stained by both PI and SYTO 16 (Figures 5B,E), indicating the spore’s IM and cortex were damaged as previously studied (Setlow et al., 2008). Besides, the thermal treated spores turned from bright (Figures 5A,D) to gray (Figures 5B,E) rather than dark (Figures 5C,F) in the phase contrast microscopy, indicating the spore core was partially rehydrated, which is probably due to partially damage of cortex (Luu-Thi et al., 2015). These results were similar with the PAUHT treated spores, but we still could not confirm that the spores whether germinated during PAUHT treatment, because more than 90% DPA of these spores was released (Table 2), which could also be the results from germination by HP during PAUHT treatment.
Figure 4. The inactivation of B. subtilis 168 (•) and C. sporogenes PA3679 spores () by thermal treatment at 121°C for different times, and its correlating amount of released DPA for B. subtilis 168 spores and (
) for C. sporogenes PA3679 spores (
), respectively.
Figure 5. The phase contrast and fluorescence microscopy of B. subtilis 168 (A–C) and C. sporogenes PA3679 (D–F) spores: (A,D) untreated spores, (B,E) 121°C for 1 min at ambient pressure. (C) 600 MPa at ambient temperature for 10 min used as control, (F) Incubation in 20 mM Tris buffer pH 7.4, L-alanine (100 mM), L-lactate (50 mM), and NaHCO3 at 30°C for 1 h used as control.
In order to further investigate whether spores underwent germination during PAUHT treatment, we investigated the effect of pressure treatment alone on spore germination. The pressure treatment was performed at 600 MPa at ambient temperature for 1 min of holding time. The DPA release was determined after high pressure treatment and used as the indicator for spore germination as other reports (Yi and Setlow, 2010). As shown in Figure 6A, pressure at 600 MPa for 1 min could not directly inactivate the B. subtilis 168 and FB 85 spores (data was not shown), but it could induce more than 25% DPA to release, similar as previous studies (Vercammen et al., 2012; Sarker et al., 2015), indicating spores were induced to germinate by HP of 600 MPa. Since FB85 spores has no GRs (Paidhungat et al., 2002), thus germination under pressure of 600 MPa were likely triggered by opening the DPA channel without activating the GRs, as reported previously (Black et al., 2005; Doona et al., 2014). For C. sporogenes PA3679 spores. Pressure at 600 MPa for 1 min couldn’t induce spores to release DPA (Figure 6A), indicating C. sporogenes PA3679 spores could not germinate at this pressure. However, in the presence of SpoVA channels, the treatment of 600 MPa can theoretically induce C. sporogenes PA3679 spores to germinate similar to the C. perfringens spores (Paredes-Sabja et al., 2011; Doona et al., 2016b). It has been reported that the HP of 550 MPa triggered the C. perfringens spores to release their DPA and germinate, which happened after the cortex hydrolysis caused by activating the CLEs, SleC (Paredes-Sabja et al., 2009; Doona et al., 2016b). The C. sporogenes spores does not have the CSP and SleC (Paredes-Sabja et al., 2011; Doona et al., 2014), which are nececery for the cortex degradation of C. perfringens spores. Hence, the lack of CSP and SleC was probably the reason why HP at ambient temperature could not induce C. sporogenes PA 3679 spores to release DPA and germinate. In addition, previous reports indiccated that spore germination could be potentiated or activated by heat activation (Luu et al., 2015), hence we further investegate the effect of heat activation on spore germination triggered by HP (Figure 6A). Both B. subtilis 168 and C. sporogenes PA3679 spores were heat activated at 75°C for 15 min prior HP treatment of 600 MPa at atmosphere for 1 min. for B. subtilis 168 spores, heat activated spores released DPA (68%) more than unactivated spores (26%) (Figure 6A), indicating HP triggered germination was enhanced by heat activation as previous reports (Luu et al., 2015; Doona et al., 2016b). Notably, for C. sporogenes PA3679 spores, after the heat activation (75°C, 15 min), more than 20% DPA was released (Figure 6A), indicating the C. sporogenes PA3679 spores germinated after the HP of 600 MPa for 1 min. The effect of heat activation on C. sporogenes PA3679 spore germination by HP was surprising, since this has not been reported previously.
Figure 6. The DPA release or inactivation of unactivated and heat activated (75°C, 15 min) spores after treatment of 600 MPa at ambient temperature for 1 min (A) or PAUHT treatment of 600 MPa at 121°C without pressure holding time (B).
Considering the spore germination triggered by HP could be enhanced by heat activation, we further investigate the effect of heat activation on spore inactivation during PAUHT treatment. Both B. subtilis 168 and C. sporogenes PA3679 spores were heat activated at 75°C for 15 min prior PAUHT treatment of 600 MPa at 121°C without pressure holding time. After PAUHT treatment, the counts of spore inactivation were determined, as shown in Figure 6B. For B. subtilis 168 after heat activation, 6.83 log spores were inactivated by the compression during PAUHT treatment of 600 MPa at 121°C, which was comparative to unactivated spores (6.75 log inactivation), indicating that the heat activation has no effect on inactivation of B. subtilis 168 spores by PAUHT treatment. It could be attributed to the lack of GRs in B. subtilis 168 spores, since heat activation acted primarily on GRs (Luu et al., 2015). However, for C. sporogenes PA3679, 3.56 log of heat activated spores were inactivated by the same treatment, which was higher than unactivated spores (2.56 log inactivation), indicating that heat activation could significantly enhance the spore inactivation during compression of PAUHT treatment. These observations suggested that heat activation play different role on inactivation of different spores during compression. For C. sporogenes PA3679 spores, since the heat activation could not inactivate spores, but could enhance the spore germination by HP. Therefore, more heat activated spores were inactivated during compression, possibly because the HP triggered germination was enhanced by heat activation. Hence, the C. sporogenes PA3679 spores were probably triggered to germinate and then inactivated during compression of PAUHT treatment.
In order to further investigate whether spores undergo germination during compression of PAUHT treatment, the B. subtilis 168 and C. sporogenes PA3679 spores were treated with various conditions of Ti = 65–84°C and high pressure P = 500–600 MPa during compression (hold time = 0 s). At the completion of the compression (100–120 s), the pressure was released, samples were withdrawn from the HPP chamber, cooled on ice, and then recovered on LB and RCM agar. As shown in Table 3. During compression of the PAUHT treatment of 600 MPa at 121°C (Ti = 84 and P = 600 MPa), no increase of B. subtilis 168 and C. sporogenes PA3679 counts was discernible as above noted (Table 2), indicating the spores were not activated during compression of PAUHT treatment. Similar results were obtained for B. subtilis 168 spores with Ti = 65–75°C and P = 500–600 MPa. However, for the C. sporogenes PA3679 spores, under the conditions of Ti = 65°C and P = 600 MPa, the viable counts increased after the compression (98% increase), indicating the C. sporogenes PA3679 spores were activated after compression. At Ti = 75°C and P = 600 MPa, a slight decrease (27%) in spore counts after the compression suggested that inactivation was preceded by activation. Hence, during compression of the PAUHT treatment (Ti = 84°C and P = 600 MPa), the C. sporogenes PA3679 spores may also pass through the process of activation and inactivation.
Table 3. Activation and germination and of B. subtilis 168 and C. sporogenes PA3679 spores during compression.
The effect of compression (Ti = 65–84°C, P = 500–600 MPa) on the germination of B. subtilis 168 and C. sporogenes PA3679 spores were further investigated. After the completion of compression, spores were treated by wet heat (80°C, 20 min) then enumerated. As shown in Table 3. At Ti = 75°C and P = 500 MPa or Ti = 65°C and P = 600 MPa, for B. subtilis 168, more than 90% spores were inactivated by subsequent wet heat treatment, indicating the spores were induced to germinate by compression. At Ti = 75°C and P = 600 MPa, the rate of germination decreased to 53% after compression, indicating some population of germinated spores were inactivated during compression. Similar results were obtained for C. sporogenes PA3679 spores. Hence, during compression of PAUHT treatment (Ti = 84°C and P = 600 MPa), both B. subtilis 168 and C. sporogenes PA3679 spores possibly underwent the process of germination and inactivation.
As a consequence, during the PAUHT treatment, the compression and pressure holding process played important role in spore inactivation. The mechanism of spore inactivation during PAUHT treatment could be concluded as follows: for B. subtilis 168, spores were firstly induced to germinate under HP, which was enhanced by increased temperature. Then the germinated spores were inactivated by heat as previous reports (Luu et al., 2015; Doona et al., 2016a, 2017). For C. sporogenes PA3679, spores were likely activated during the compression time of PAUHT, and then the activated spores were induced to germination by HP, followed by inactivation by heat. In addition, the PAUHT inactivated spores showed gray rather than dark observed by phase contrast microscopy, indicating that these spores only undergo the first stage of germination (DPA was released and spores lost partial resistance) and the cortex degradation was blocked, which was possibly due to the inactivation of CLEs by heat or pressure (Reineke et al., 2011).
In this work, PAUHT system was established by using soybean oil as adiabatic medium, and a regression model was developed to predict the initial temperature of soybean oil for PAUHT. The B. subtilis 168 and C. sporogenes PA3679 spores were inactivated during PAUHT treatment in short time (< 1 min). The inactivation mechanism of B. subtilis 168 and C. sporogenes PA 3679 spores by PAUHT treatment could be supposed as follows: during PAUHT treatment, spores were firstly triggered to germinate by HP, with completing stage I of germination, and then the germinated spores were inactivated by heat.
The inactivation of bacterial spores in the low-acid canned food remains the most serious problem in food sterilization because of the high resistance of spores to high pressure and thermal processing. In this work, we showed that the proposed PAUHT system with soybean oil as adiabatic medium was effective for inactivating bacterial spores in short time (<1 min), therefore it has the potential to be a promising alternative technique for food sterilization. Further understanding of spore inactivation mechanism by PAUHT treatment will help to make this technique available for studies in pilot and production scale.
DL carried out the experiments and wrote the manuscript. LZ, XW, and PW gave the advice and assistance during the experiments. XL and FC reviewed the manuscript and gave the advice on the manuscript. XMW revised the manuscript. XH designed the experiments and reviewed the manuscript.
This work was supported by Key Project of National Natural Science Foundation of China (NSFC) (No. 31530058).
The authors declare that the research was conducted in the absence of any commercial or financial relationships that could be construed as a potential conflict of interest.
The Supplementary Material for this article can be found online at: https://www.frontiersin.org/articles/10.3389/fmicb.2019.01275/full#supplementary-material
Ahn, J., Balasubramaniam, V. M., and Yousef, A. E. (2007). Inactivation kinetics of selected aerobic and anaerobic bacterial spores by pressure-assisted thermal processing. Int. J. Food Microbiol. 113, 321–329. doi: 10.1016/j.ijfoodmicro.2006.08.012
Ardia, A., Knorr, D., and Heinz, V. (2004). Adiabatic heat modelling for pressure build-up during high-pressure treatment in liquid-food processing. Food Bioprod. Process. 82, 89–95. doi: 10.1205/096030804322985362
Black, E. P., Koziol-Dube, K., Guan, D., Wei, J., Setlow, B., Cortezzo, D. E., et al. (2005). Factors influencing germination of Bacillus subtilis spores via activation of nutrient receptors by high pressure. Appl. Environ. Microbiol. 71, 5879–5887. doi: 10.1128/AEM.71.10.5879-5887.2005
Doona, C. J., Feeherry, F. E., Kustin, K., Chen, H., Huang, R., Philip Ye, X., et al. (2017). A quasi-chemical model for bacterial spore germination kinetics by high pressure. Food Eng. Rev. 9, 122–142. doi: 10.1007/s12393-016-9155-1
Doona, C. J., Feeherry, F. E., Ross, E. W., and Kustin, K. (2016a). Chemical kinetics for the microbial safety of foods treated with high pressure processing or hurdles. Food Eng. Rev. 8, 272–291. doi: 10.1007/s12393-015-9138-7
Doona, C. J., Feeherry, F. E., Setlow, B., Wang, S., Li, W., Nichols, F. C., et al. (2016b). Effects of high-pressure treatment on spores of Clostridium species. Appl. Environ. Microbiol. 82, 5287–5297. doi: 10.1128/AEM.01363-16
Doona, C. J., Ghosh, S., Feeherry, F. F., Ramirez-Peralta, A., Huang, Y., Chen, H., et al. (2014). High pressure germination of Bacillus subtilis spores with alterations in levels and types of germination proteins. J. Appl. Microbiol. 117, 711–720. doi: 10.1111/jam.12557
Elke, Y., and Wuytack, C. W. M. (2001). A study on the effects of high pressure and heat on Bacillus subtilis spores at low pH. Int. J. Food Microbiol. 64, 333–341. doi: 10.1016/S0168-1605(00)00478-5
Houška, M., Kubásek, M., Strohalm, J., Landfeld, A., and Kamarád, J. (2004). Warming of olive oil processed by high hydrostatic pressure. High Pressure Res. 24, 303–308. doi: 10.1080/08957950410001721173
Katja, N., Peter, S., Li, Y. Q., and Moeller, R. (2013). High salinity alters the germination behavior of Bacillus subtilis spores with nutrient and nonnutrient germinants. Appl. Environ. Microbiol. 80, 1314–1321. doi: 10.1128/AEM.03293-13
Kong, L., Zhang, P., Yu, J., and Setlow, P. (2014). Monitoring rates and heterogeneity of high-pressure germination of Bacillus spores by phase-contrast microscopy of individual spores. Appl. Environ. Microbiol. 80, 345–353. doi: 10.1128/AEM.03043-13
Kong, L., Zhang, P., Yu, J., Setlow, P., and Li, Y. Q. (2010). Monitoring the kinetics of uptake of a nucleic acid dye during the germination of single spores of Bacillus species. Anal. Chem. 82, 8717–8724. doi: 10.1021/ac1022327
Lau, M. H., and Turek, E. J. (2007). “Determination of quality differences in low-acid foods sterilized by high pressure versus retorting,” in High Pressure Processing of Food, Chap. 9, eds C. J. Doona and F. E. Feeherry (Hoboken, NJ: IFT. Press-Blackwell Publishing), 195–219. doi: 10.1002/9780470376409.ch9
Li, Y., Butzin, X. Y., Davis, A., Setlow, B., Korza, G., Ustok, F. I., et al. (2013). Activity and regulation of various forms of CwlJ, SleB, and YpeB proteins in degrading cortex peptidoglycan of spores of Bacillus species in vitro and during spore germination. J. Bacteriol. 195, 2530–2540. doi: 10.1128/JB.00259-13
Lopes, R. P., Mota, M. J., Gomes, A. M., Delgadillo, I., and Saraiva, J. A. (2018). Application of high pressure with homogenization, temperature, carbon dioxide, and cold plasma for the inactivation of Bacterial spores: a review. Compr. Rev. Food Sci. Food Saf. 17, 532–555. doi: 10.1111/1541-4337.12311
Luu, S., Cruz-Mora, J., Setlow, B., Feeherry, F. E., Doona, C. J., and Setlow, P. (2015). The effects of heat activation on Bacillus spore germination, with nutrients or under high pressure, with or without various germination proteins. Appl. Environ. Microbiol. 81, 2927–2938. doi: 10.1128/AEM.00193-15
Luu-Thi, H., Corthouts, J., Passaris, I., Grauwet, T., Aertsen, A., Hendrickx, M., et al. (2015). Carvacrol suppresses high pressure high temperature inactivation of Bacillus cereus spores. Int. J. Food Microbiol. 197, 45–52. doi: 10.1016/j.ijfoodmicro.2014.12.016
Margosch, D., Ehrmann, M. A., Buckow, R., Heinz, V., Vogel, R. F., and Ganzle, M. G. (2006). High-pressure-mediated survival of Clostridium botulinum and Bacillus amyloliquefaciens endospores at high temperature. Appl. Environ. Microbiol. 72, 3476–3481. doi: 10.1128/AEM.72.5.3476-3481.2006
Margosch, D., Ehrmann, M. A., Gänzle, M. G., and Vogel, R. F. (2004a). Comparison of pressure and heat resistance of Clostridium botulinum and other endospores in mashed carrots. J. Food Prot. 67, 2530–2537. doi: 10.4315/0362-028X-67.11.2530
Margosch, D., Ganzle, M. G., Ehrmann, M. A., and Vogel, R. F. (2004b). Pressure inactivation of Bacillus endospores. Appl. Environ. Microbiol. 70, 7321–7328. doi: 10.1128/AEM.70.12.7321-7328.2004
Mathys, A., Chapman, B., Bull, M., Heinz, V., and Knorr, D. (2007). Flow cytometric assessment of Bacillus spore response to high pressure and heat. Innov. Food Sci. Emerg. Technol. 8, 519–527. doi: 10.1016/j.ifset.2007.06.010
Mathys, A., Reineke, K., Heinz, V., and Knorr, D. (2009). High pressure thermal sterilization – development and application of temperature controlled spore inactivation studies. High Pressure Res. 29, 3–7. doi: 10.1080/08957950802526469
Min, S., Sastry, S. K., and Balasubramaniam, V. M. (2010). Compressibility and density of select liquid and solid foods under pressures up to 700MPa. J. Food Eng. 96, 568–574. doi: 10.1016/j.jfoodeng.2009.09.003
Nguyen, L. T., Tay, A., Balasubramaniam, V., Legan, J., Turek, E. J., and Gupta, R. (2010). Evaluating the impact of thermal and pressure treatment in preserving textural quality of selected foods. LWT-Food Sci. Technol. 43, 525–534. doi: 10.1016/j.lwt.2009.09.022
Olivier, S. A., Bull, M. K., Stone, G., van Diepenbeek, R. J., Kormelink, F., Jacops, L., et al. (2011). Strong and consistently synergistic inactivation of spores of spoilage-associated Bacillus and Geobacillus spp. by high pressure and heat compared with inactivation by heat alone. Appl. Environ. Microbiol. 77, 2317–2324. doi: 10.1128/AEM.01957-10
Paidhungat, M., Setlow, B., Daniels, W. B., Hoover, D., Papafragkou, E., and Setlow, P. (2002). Mechanisms of induction of germination of Bacillus subtilis spores by high pressure. Appl. Environ. Microbiol. 68, 3172–3175. doi: 10.1128/aem.68.6.3172-3175.2002
Paidhungat, M., and Setlow, P. (2000). Role of Ger proteins in nutrient and nonnutrient triggering of spore germination in Bacillus subtilis. J. Bacteriol. 182, 2513–2519. doi: 10.1128/JB.182.9.2513-2519.2000
Paredes-Sabja, D., Setlow, P., and Sarker, M. R. (2009). SleC is essential for cortex peptidoglycan hydrolysis during germination of spores of the pathogenic bacterium Clostridium perfringens. J. Bacteriol. 191, 2711–2720. doi: 10.1128/JB.01832-08
Paredes-Sabja, D., Setlow, P., and Sarker, M. R. (2011). Germination of spores of Bacillales and Clostridiales species: mechanisms and proteins involved. Trends Microbiol. 19, 85–94. doi: 10.1016/j.tim.2010.10.004
Patazca, E., Koutchma, T., and Balasubramaniam, V. (2007). Quasi-adiabatic temperature increase during high pressure processing of selected foods. J. Food Eng. 80, 199–205. doi: 10.1016/j.jfoodeng.2006.05.014
Peleg, M., Normand, M. D., Corradini, M. G., Van Asselt, A. J., De Jong, P., and Ter Steeg, P. F. (2008). Estimating the heat resistance parameters of bacterial spores from their survival ratios at the end of UHT and other heat treatments. Crit. Rev. Food Sci. Nutr. 48, 634–648. doi: 10.1080/10408390701724371
Ramaswamy, H., Shao, Y., Bussey, J., and Austin, J. (2013). Screening of twelve Clostridium botulinum (group I) spores for high-pressure resistance at elevated-temperatures. Food Bioprod. Process. 91, 403–412. doi: 10.1016/j.fbp.2013.02.009
Ramaswamy, R., and Balasubramaniam, V. (2007). Effect of polarity and molecular structure of selected liquids on their heat of compression during high pressure processing. High Pressure Res. 27, 299–307. doi: 10.1080/08957950701385926
Reddy, N. R., Patazca, E., Morrissey, T. R., Skinner, G. E., Loeza, V., Schill, K. M., et al. (2016). Thermal and pressure-assisted thermal destruction kinetics for spores of type A Clostridium botulinum and Clostridium sporogenes PA3679. J. Food Prot. 79, 253–262. doi: 10.4315/0362-028X.JFP-15-310
Reineke, K., Ellinger, N., Berger, D., Baier, D., Mathys, A., Setlow, P., et al. (2013a). Structural analysis of high pressure treated Bacillus subtilis spores. Innov. Food Sci. Emerg. Technol. 17, 43–53. doi: 10.1016/j.ifset.2012.10.009
Reineke, K., Mathys, A., Heinz, V., and Knorr, D. (2013b). Mechanisms of endospore inactivation under high pressure. Trends Microbiol. 21, 296–304. doi: 10.1016/j.tim.2013.03.001
Reineke, K., Schlumbach, K., Baier, D., Mathys, A., and Knorr, D. (2013c). The release of dipicolinic acid-the rate-limiting step of Bacillus endospore inactivation during the high pressure thermal sterilization process. Int. J. Food Microbiol. 162, 55–63. doi: 10.1016/j.ijfoodmicro.2012.12.010
Reineke, K., Mathys, A., and Knorr, D. (2011). The impact of high pressure and temperature on bacterial spores: inactivation mechanisms of Bacillus subtilis above 500 MPa. J. Food Sci. 76, M189–M197. doi: 10.1111/j.1750-3841.2011.02066.x
Sapru, V., Teixeire, A. A., Smerage, G. H., and Lindsay, J. A. (1992). Predicting thermophilic spore population dynamics for UHT sterilization processes. J. Food Sci. 57, 1248–1253. doi: 10.1111/j.1365-2621.1992.tb11310.x
Sarker, M. R., Akhtar, S., Torres, J. A., and Paredes-Sabja, D. (2015). High hydrostatic pressure-induced inactivation of bacterial spores. Crit. Rev. Microbiol. 41, 18–26. doi: 10.3109/1040841X.2013.788475
Setlow, B., Peng, L., Loshon, C. A., Li, Y. Q., Christie, G., and Setlow, P. (2009). Characterization of the germination of Bacillus megaterium spores lacking enzymes that degrade the spore cortex. J. Appl. Microbiol. 107, 318–328. doi: 10.1111/j.1365-2672.2009.04210.x
Setlow, B., Wahome, P. G., and Setlow, P. (2008). Release of small molecules during germination of spores of Bacillus Species. J. Bacteriol. 190, 4759–4763. doi: 10.1128/JB.00399-08
Setlow, P. (2003). Spore germination. Curr. Opin. Microbiol. 6, 550–556. doi: 10.1016/j.mib.2003.10.001
Setlow, P. (2006). Spores of Bacillus subtilis: their resistance to and killing by radiation, heat and chemicals. J. Appl. Microbiol. 101, 514–525. doi: 10.1111/j.1365-2672.2005.02736.x
Setlow, P. (2018). Observations on research with spores of Bacillales and Clostridiales species. J. Appl. Microbiol. 126, 348–358. doi: 10.1111/jam.14067
Setlow, P., Wang, S., and Li, Y.-Q. (2017). Germination of spores of the orders Bacillales and Clostridiales. Annu. Rev. Microbiol. 71, 459–477. doi: 10.1146/annurev-micro-090816-093558
Sevenich, R., Bark, F., Crews, C., Anderson, W., Pye, C., Riddellova, K., et al. (2013). Effect of high pressure thermal sterilization on the formation of food processing contaminants. Innov. Food Sci. Emerg. Technol. 20, 42–50. doi: 10.1016/j.ifset.2013.07.006
Shao, Y., and Ramaswamy, H. S. (2008). Clostridium sporogenes-ATCC 7955 spore destruction kinetics in milk under high pressure and elevated temperature treatment conditions. Food Bioprocess Technol. 4, 458–468. doi: 10.1007/s11947-008-0165-8
Shao, Y., Zhu, S., Ramaswamy, H., and Marcotte, M. (2010). Compression heating and temperature control for high-pressure destruction of bacterial spores: an experimental method for kinetics evaluation. Food Bioprocess Technol. 3, 71–78. doi: 10.1007/s11947-008-0057-y
Theron, E. O., and Irving, J. P. (1977). Thermal destruction of Clostridium botulinum spores suspended in tomato juice in aluminum thermal death time tubes. Appl. Environ. Microbiol. 34, 23–29.
Vepachedu, V. R., and Setlow, P. (2007). Role of SpoVA proteins in release of dipicolinic acid during germination of Bacillus subtilis spores triggered by dodecylamine or lysozyme. J. Bacteriol. 189, 1565–1572. doi: 10.1128/JB.01613-06
Vercammen, A., Vivijs, B., Lurquin, I., and Michiels, C. W. (2012). Germination and inactivation of Bacillus coagulans and Alicyclobacillus acidoterrestris spores by high hydrostatic pressure treatment in buffer and tomato sauce. Int. J. Food Microbiol. 152, 162–167. doi: 10.1016/j.ijfoodmicro.2011.02.019
Wang, S., Brunt, J., Peck, M. W., Setlow, P., and Li, Y. Q. (2017). Analysis of the germination of individual Clostridium sporogenes spores with and without germinant receptors and cortex-lytic enzymes. Front. Microbiol. 8:2047. doi: 10.3389/fmicb.2017.02047
Wilson, D. R., Dabrowski, L., Stringer, S., Moezelaar, R., and Brocklehurst, T. F. (2008). High pressure in combination with elevated temperature as a method for the sterilisation of food. Trends Food Sci. Technol. 19, 289–299. doi: 10.1016/j.tifs.2008.01.005
Yi, X., and Setlow, P. (2010). Studies of the commitment step in the germination of spores of bacillus species. J. Bacteriol. 192, 3424–3433. doi: 10.1128/JB.00326-10
Keywords: pressure-assisted ultra-high temperature, mathematical model, bacterial spores, sterilization, inactivation mechanism
Citation: Liang D, Zhang L, Wang X, Wang P, Liao X, Wu X, Chen F and Hu X (2019) Building of Pressure-Assisted Ultra-High Temperature System and Its Inactivation of Bacterial Spores. Front. Microbiol. 10:1275. doi: 10.3389/fmicb.2019.01275
Received: 28 August 2018; Accepted: 22 May 2019;
Published: 10 June 2019.
Edited by:
Om V. Singh, TSG Consulting, a Science Group Company, United StatesReviewed by:
Hui Li, Chinese Academy of Agricultural Sciences, ChinaCopyright © 2019 Liang, Zhang, Wang, Wang, Liao, Wu, Chen and Hu. This is an open-access article distributed under the terms of the Creative Commons Attribution License (CC BY). The use, distribution or reproduction in other forums is permitted, provided the original author(s) and the copyright owner(s) are credited and that the original publication in this journal is cited, in accordance with accepted academic practice. No use, distribution or reproduction is permitted which does not comply with these terms.
*Correspondence: Xiaosong Hu, aHV4aWFvczEyM0AxMjYuY29t
Disclaimer: All claims expressed in this article are solely those of the authors and do not necessarily represent those of their affiliated organizations, or those of the publisher, the editors and the reviewers. Any product that may be evaluated in this article or claim that may be made by its manufacturer is not guaranteed or endorsed by the publisher.
Research integrity at Frontiers
Learn more about the work of our research integrity team to safeguard the quality of each article we publish.