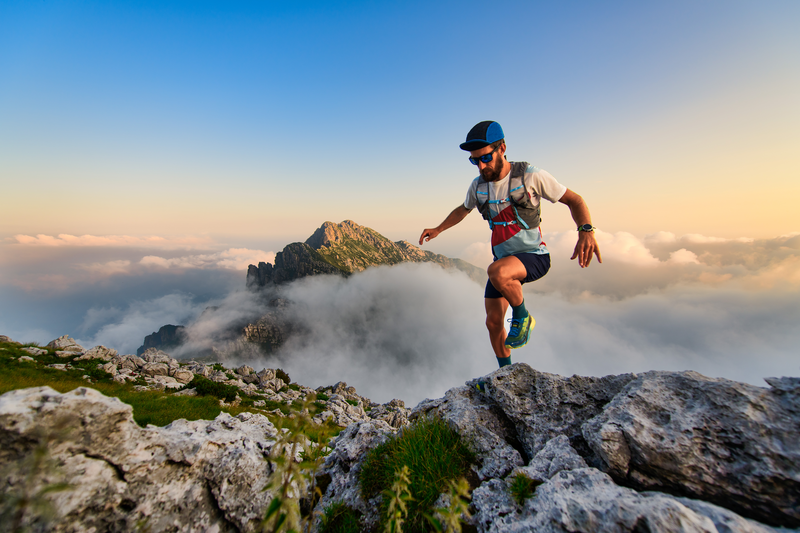
94% of researchers rate our articles as excellent or good
Learn more about the work of our research integrity team to safeguard the quality of each article we publish.
Find out more
ORIGINAL RESEARCH article
Front. Microbiol. , 21 May 2019
Sec. Microbiological Chemistry and Geomicrobiology
Volume 10 - 2019 | https://doi.org/10.3389/fmicb.2019.01111
This article is part of the Research Topic Bioleaching and Biocorrosion: Advances in Interfacial Processes View all 19 articles
The marine bacterium, Vibrio natriegens, grows quickly in a marine environment and can significantly accelerate the corrosion of steel materials. Here, we present an approach to inhibit V. natriegens-induced corrosion by biomineralization. The corrosion of steel is mitigated in seawater via the formation of a biomineralized film induced by Bacillus subtilis. The film is composed of extracellular polymeric substances (EPS) and calcite, exhibiting stable anti-corrosion activity. The microbial diversity and medium chemistry tests demonstrated that the inhibition of V. natriegens growth by B. subtilis was essential for the formation of the biomineralized film.
When steel materials are exposed to a marine environment, galvanic corrosion coupled with microbiologically influenced corrosion (MIC) will inevitably occur, resulting in tremendous economic loss and security risk (Li et al., 2015). Approaches to retard steel corrosion include cathodic protection (Bagherzadeh and Mousavinejad, 2012), coatings (Shchukin et al., 2006; Andreeva et al., 2008), and corrosion inhibitors. While these approaches are effective, they are limited by high cost, maintenance difficulties, environmental pollution, and short service time (Banerjee et al., 2012; Nkuzinna et al., 2014). In our previous study, we demonstrated that some biofilms are able to provide an environmentally friendly, stable, and lasting biomineralized film to inhibit corrosion in a marine environment (Liu et al., 2019). The film is composed of extracellular polymeric substances (EPS) and calcium carbonate with a calcite structure, exhibiting a stable and self-healing anti-corrosion activity. Bacterial metabolism produces large amounts of CO2. The precipitation of CaCO3 is mainly related to the release of CO2 from bacteria, resulting in supersaturating with respect to calcium carbonate. In this process, abundant EPS around bacteria provides nucleation site for precipitation of CaCO3.
However, the natural environment includes diverse microorganisms, may hinder this application. Specifically, bacteria that compete for growth in a natural marine environment might affect the biomineralization (Tan et al., 2018). Therefore, further investigation into biofilm-induced mineralization within a competitive bacterial medium is critical for its practical application.
The marine bacterium Vibrio natriegens is widely distributed in the ocean, which was first reported by Payne in 1958. V. natriegens grows faster than other organisms in the marine environment (Aiyar et al., 2002; Cheng et al., 2009; Mullenger and Gill, 2010; Weinstock et al., 2016; Hoffart et al., 2017). As a result, V. natriegens is typically one of the early colonizers in biofilm communities (Nadia et al., 1991). Moreover, V. natriegens is able to acquire electrons during the process of N2-fixation, which further accelerates corrosion of substrate materials under certain conditions (Angell et al., 1995; Coyer et al., 1996; Little et al., 2008; Cheng et al., 2009; Yin et al., 2009).
Herein, we find that the MIC by V. natriegens can be inhibited by the addition of Bacillus subtilis in the marine broth. Interestingly, co-culture of V. natriegens and B. subtilis resulted in a distinguishing effect on the corrosion of steel. Morphological and electrochemical analyses showed that B. subtilis provided anti-corrosion protection for steel in the presence of V. natriegens. The biomineralized film was not influenced by the competitive growth of V. natriegens. The chemical composition of the solution showed that a decrease in magnesium (Mg2+) and an increase in pH occurred during the biomineralization process induced by B. subtilis, which contributed to the inhibition of V. natriegens growth and metabolism. Therefore, this study indicated that the biomineralized film induced by B. subtilis biofilm formation is a promising approach for reducing the corrosion of steel in a complex bacterial environment.
The low-alloyed steel plates were supplied by Baosteel Inc., (China). The chemical composition of steel is: 1.52 wt% Mn, 0.70 wt% Ni, 0.04 wt% Al, 0.15 wt% Cr, 0.05 wt% C, 0.40 wt% Mo, 97.14 wt% Fe. The coupons were covered by the epoxy resin, leaving a 10 mm × 10 mm working area, and then polished from 200 to 800 grit, washed with absolute ethyl alcohol, deionized water sequentially. Lastly, they were N2-dried and sterilized by ultraviolet (UV) light.
The bacterial strains, B. subtilis and V. natriegens, were isolated from the East China Sea. The two bacterial strains were cultivated in a 2216E medium on a shaker at 120 rpm at 37°C. 2216E medium: 5 g peptone, 1 g yeast extract, and 0.01 g ferric phosphate (Sinopharm Chemical Reagent Co., Ltd., China) was added into 1 L seawater, then sterilized at 121°C for 15 min. The coupons were immersed in 1-L containers with 500 mL of 2216E medium. Two hundred microliters of an overnight culture of V. natriegens strain was used to inoculate the first container. To investigate the effect of B. subtilis on corrosion by V. natriegens, one hundred microliters of each strain was then used to inoculate the second container. The two containers were then cultured at 120 rpm for 13 days at 37°C. The relative abundance was measured using bacterial diversity analysis at Majorbio (Shanghai, China). The primers sequence was: 338F: 5′-ACTCCTACGGGAGGCAGCAG-3′, 806R: 5′- GGACTACHVGGGTWTCTAAT-3′. The V3+V4 region of the bacterial 16S rRNA gene was amplified by the above primers. PCR reaction conditions was: 95°C 3 min; 95°C 30 s, 55°C 30 s, 72°C 45 s, 30 cycles; 72°C 10 min; 10°C 10 min. PCR reaction system was: 5 × PCR buffer (with Mg2+) 4 μL; 2.5 mmol/L dNTP 2 μL; 5 μmol/L P1 (338F) 0.8 μL; 5 μmol/L P2 (806R) 0.8 μL; 5 U/μL Taq enzyme 0.4 μL; DNA template 2 μL; ddH2O 10 μL. It was then sequenced on an Illumina MiSeq PE250/PE300. The sequencing work was completed by Majorbio (Shanghai, China). Relative abundance was obtained from rank-abundance function on www.i-sanger.com.
After 2 weeks of growth, the coupons were washed gently with a phosphate buffer solution (PBS, pH = 7.4) and then immersed in 2.5% glutaraldehyde solution (Sinopharm Chemical Reagent Co., Ltd., China) for 15 min. Subsequently, the coupons with biofilms were dehydrated by gradient ethanol solution (30, 50, 60, 70, 80, 90, and 100%) sequentially for 10 min and dried with nitrogen gas. The morphology of the covered surface was characterized by a scanning electron microscope (SEM) with an energy dispersive spectrometer (JEOL JSM-7500F, Japan). The biofilm and corrosion products were removed using the NACE Recommended Method 0775-1999 protocol (NACE, 1999). The coupons were immersed sequentially in a solution of dibutyl thiourea hydrochloride, sodium bicarbonate (NaHCO3) solution (Sinopharm Chemical Reagent Co., Ltd., China), and water for 2 min each. The morphology of the coupons was observed by a 3-D optical profilometer (Bruker Contour GT, Germany).
X-ray diffraction (XRD; X’Pert PRO XRD, PANalytical, Almelo, Netherlands) was applied to investigate the phase identification of the film on the coupons (40 kV, 10 mA, Cu-Kα radiation source, 0.26°/s scanning rate, 2𝜃 degree of 20–90°). Furthermore, the steel coupons covered with biofilms were analyzed by X-ray photoelectron spectroscopy (XPS; Kratos, AXIS Ultra DLD, England; 200 μm spot size, 0–1200 eV, 45° emission angle). High-resolution spectra of C 1s and O 1s scans were obtained. Experiments were performed with three random tests on the different scanning areas, and only representative images were shown.
The electrochemical methods, such as open-circuit potential (OCP), linear polarization (LPR), and electrochemical impedance spectroscopy (EIS), were carried out using an electrochemical workstation (Ivium, Eindhoven, Netherlands). The steel coupons were working electrodes. A saturated calomel electrode (SCE) and a platinum (Pt) sheet were the reference electrode and the counter electrode, respectively. Prior to the measurements, the OCP of the steel coupons was monitored for at least 60 min in order to reach a steady state. The LPR measurement was obtained at a scan rate of 0.125 mV s-1 in the range from -5 to 5 mV versus the OCP, with a sampling frequency of 1 Hz (Xu et al., 2018). The EIS measurement was measured with a frequency range between 1 × 10-2 and 1 × 105 Hz and ± 10 mV amplitude. The impedance data were analyzed by ZSimpWin. The corrosion rate was obtained from weight loss tests using the following equation:
In addition, we used a local electrochemical impedance spectroscopy (LEIS) workstation (Bio-logic M470, Seyssins, France) to study the local impedance map on the coupon surface. A carbon rod was used as the counter electrode with a SCE as the reference electrode. The surface of coupon was the working electrode. There was a 100-μm distance between the working electrode and the Pt probe tip. The LEIS maps were measured with 10 μA current amplitude and 10 Hz frequency. We chose the 10 Hz single frequency because the curve of impedance vs. frequency was at the logarithm frequency interval of 10 Hz frequency, which is beneficial to the stable testing and resistance to the disturbance (Liu J. et al., 2018; Ma et al., 2018).
The content of Ca2+, Mg2+, and NH4+ was measured to investigate N2-fixation of V. natriegens in two containers. pH was also obtained in this process. The Ca and Mg concentration in the seawater was investigated by ICP in first 5 days. NH4+ in medium was determined by ion chromatography (Thomas et al., 2002). Three replicates were measured in all cases, and the background correction was obtained using blank samples.
Scanning electron microscope was used to investigate the surface morphology of the coupons from co-cultures of B. subtilis and V. natriegens in marine broth after 13 days. The control culture contained only the V. natriegens strain. Figures 1a,b illustrate the rough, cracked, and heterogeneous corrosion products that formed on the steel surface in the control medium. The iron oxide (Fe3O4) formed in a petal-like manner on the surface of the steel coupons. In contrast, only triangular-shaped minerals mineral precipitation was present on the steel in the medium containing B. subtilis and V. natriegens at day 13 (Figures 1c,d). The biomineralized film was homogeneous and compact, demonstrating a potential to protect steel coupons. Obviously, the biomineralized film that formed on the coupons was not influenced by the competitive growth of V. natriegens. The energy dispersive spectrometer measured the film composition on day 13. As shown in Supplementary Figure S1a, the amount of C, O, Mg, and Ca on the steel coupons in the control medium was 3.4, 24.1, 0.3, and 5.3 wt%, respectively. In contrast, the amount of C, O, Mg, and Ca on the steel coupons in the presence of both B. subtilis and V. natriegens was 13.3, 36.5, 4.0, and 44.5 wt%, respectively. These results indicate that the biomineralized film formed on the steel in the competitive bacterial environment.
Figure 1. Scanning electron microscope views of the morphology of the biofilm of (a,b) only V. natriegens after 13 days and (c,d) co-cultures of B. subtilis and V. natriegens. Image of two different magnitudes are shown in the (a,c) left and (b,d) right panel.
Cross-sectional SEM images were analyzed in order to investigate the thickness of the biomineralized film and the film-substrate interface. The average thickness of the corrosion product film on the steel coupon in the control culture was approximately 29 μm, as shown in Figure 2a. Figure 2b shows that the thickness of the biomineralization film, which formed on the steel in the co-culture, was approximately 44 μm. The biomineralization film and steel interface was compact in the presence of B. subtilis and V. natriegens. In contrast, in the presence of only V. natriegens, the corrosion product film was relatively loose with obvious cracks, which are unable to resist the penetration of chloride ions to the steel.
Figure 2. Cross-section views of the biofilm of (a) only V. natriegens and (b) co-cultures of B. subtilis and V. natriegens after 13 days.
Figure 3 shows the XRD patterns of the steel surfaces after 13 days. In the medium containing B. subtilis and V. natriegens, calcium and magnesium carbonate were the main components of the biomineralization film, which was consistent with our previous study (Liu T. et al., 2018). Fe3O4 was the main component of the corrosion products film in the medium with V. natriegens. High-resolution XPS of C 1s and O 1s from the coupons is shown in Figure 4. In the C 1s spectrum, the peaks at 284.6, 285.4, and 288.4 eV are attributed to the binding energies of C-C, C-O-C, and CO32- bonds, respectively (Figures 4A,B). According to the XRD analysis, the carbonate was calcium magnesium carbonate, while the C-C and C-O-C signals were attributable to the biofilm on the coupon (Liu et al., 2016). Table 1 shows that the film had a higher carbonate content (41%) in the presence of B. subtilis and V. natriegens, whereas the amount of carbonate was only 10% in the medium with V. natriegens, which was consistent with the XRD results. In the O 1s spectrum (Figures 4C,D), the peaks of 529.8, 531.6, and 533.6 eV were caused by the combined energy of the metal oxide (Fe3O4), C-O, and C=O bonds, respectively (Gauzzi et al., 1990). The data in Table 1 indicate that the amount of metal oxide (529.8 eV) was 52 and 16% in the control medium and the co-cultures medium, respectively, suggesting that the corrosion products (Fe3O4) decreased due to the formation of a biomineralized film.
Figure 3. X-ray diffraction spectrum measured on the coupon immersed in medium with only V. natriegens (black line), and in the co-cultures of B. subtilis and V. natriegens (red line), respectively.
Figure 4. X-ray photoelectron spectroscopy spectra of C 1s for biofilm of (A) only V. natriegens and (B) co-cultures of B. subtilis and V. natriegens; O 1s for biofilm of (C) only V. natriegens and (D) co-cultures of V. natriegens and B. subtilis.
In our previous study, we found that the biomineralized film induced by B. subtilis exhibited excellent corrosion resistance. However, the experimental medium only contained the B. subtilis strain, and the protection of the biomineralized film in a competitive bacterial environment was not explored. Figure 5 shows the corrosion rates of the steel coupons in the co-cultures medium and control medium. The corrosion rate in the presence of V. natriegens was 0.32 mm/a corrosion, which was 32-fold higher than the corrosion rate in the presence of B. subtilis and V. natriegens (0.01 mm/a). These results indicate that B. subtilis effectively inhibited the uniform V. natriegens-induced corrosion of steel via biomineralization.
Figure 5. Corrosion rate of coupons after 30 days of exposure in different media. The errors shown for each data is the standard deviation obtained from five sets of measurements.
Figure 6a shows the time dependence of the OCP of the steel coupons for 13 days. In the medium with only V. natriegens, the OCP of the steel coupon fluctuated at approximately -0.69 V (SCE) during the entire immersion period. However, in the presence of V. natriegens and B. subtilis, the OCP moved positively to -0.39 V (SCE) on day 13, indicating the inhibition of an anodic reaction by the biomineralized film. LPR was utilized to evaluate the Rp value corresponding to the corrosion rate based on a small overpotential. Figure 6b shows that the Rp value of the steel coupon in the co-cultures medium was relatively higher than the value in the control medium, consistent with the results of the weight loss test.
Additionally, EIS was applied to evaluate the electrochemical process of the interface of the medium, film, and steel. Figure 7A shows that two time constants appeared on the Nyquist diagram in the marine broth with V. natriegens at day 1. This could be attributed to the rapid formation of the corrosion product film when the steel coupons were immersed in the medium. The equivalent circuit, i.e., Rs(Qf(Rf(RctQdl))), is shown in Supplementary Figure S2a. The fitted data (Table 2) indicated that the charge transfer resistance (Rct) increased from 262.4 to 758.3 Ω cm2 and then decreased to 318.3 Ω cm2 on day 7. On day 13, the Rct value increased to 1149.0 Ω cm2. The irregular changes of the Rct value with the immersion time resulted from the shedding and deposition of corrosion products. However, in the presence of both V. natriegens and B. subtilis, Nyquist diagrams exhibited rather different shapes during the immersion time. During the early stage (before day 7), one loop, which corresponded to one time constant in the Nyquist diagram, indicated that the mineral deposits were not well-formed (Supplementary Figure S2b). At day 9, two time constants were observed, indicating the formation of a compact biomineralized film on the steel coupons. The equivalent circuit, i.e., Rs(Qf(Rf(RctQdl))), is shown in Supplementary Figure S2c. The Rf value that was attributed to the barrier effect of the biomineralized film increased from 1031 to 2.37 × 105 Ω cm2. These results demonstrate that B. subtilis effectively protected the steel coupons from V. natriegens-induced corrosion.
Figure 7. Nyquist and Bode diagrams measured on the coupons immersed in medium (A,B) with only V. natriegens, and (C,D) the co-cultures of B. subtilis and V. natriegens, respectively.
Table 2. Electrochemical impedance parameters fitted from the measured impedance plots in Figure 7.
Local electrochemical impedance spectroscopy measurements were carried out at a single frequency, which has been widely used for monitoring pitting corrosion or evaluating the homogeneity of coating/film due to its localized sensitivity. Figure 8 shows that the highest impedance value of the biomineralized film was approximately 1.1 × 105 Ω cm2, whereas the value in the medium with only V. natriegens was approximately 1.4 × 104 Ω cm2. The impedance value gradient on the biomineralized film was much smaller than the corrosion products film, suggesting that the biomineralized film with calcite structure formed uniformly on the steel coupons.
Figure 8. Local electrochemical impedance spectroscopy maps measured on the coupons immersed in medium with (A) only V. natriegens, and (B) co-cultures of B. subtilis and V. natriegens after 13 days.
Figure 9 shows the pit morphology of the steel coupons after removing the films. In the presence of V. natriegens and B. subtilis, the pits on the coupon were smaller and fewer than that in the medium with only V. natriegens. After analysis, the average depth of the pits was 36 μm, and the average diameter of the pits was 91 μm in the presence of V. natriegens. Both the number and the size of the pits decreased in the medium with the biomineralized film, with the pits measuring at 19 and 49 μm in depth and diameter.
Figure 9. Scanning electron microscope and the optical profilometry images of the pit morphology on the coupon immersed in medium with (a,c) only V. natriegens, and (b,d) co-cultures of B. subtilis and V. natriegens, (e) and the derived topography.
A microbial diversity test was utilized to investigate the relative content of the two bacteria as a function of time. Figure 10 shows that approximately 70% relative abundance was occupied by B. subtilis on day 2. However, at day 13, B. subtilis occupied the main content (96% relative abundance) of the medium, with only 4% relative abundance being identified as V. natriegens. This indicated that the growth of V. natriegens was inhibited by B. subtilis.
In Figure 11, in the medium with single V. natriegens, only 10.1 and 14.2% of the initial soluble Ca and Mg was removed after 5 days. The concentrations of NH4+ increased from 0.11to 23.2 mmol/L. The value of pH increased from 7.37 to 7.90. While, in co-cultures of B. subtilis and V. natriegens, 30.4 and 61.4% of the initial soluble Ca and Mg was removed after first day, respectively (Figure 11b). After 5 days, 91.8 and 68.5% of the initial soluble Ca and Mg was removed. The concentrations of NH4+ increased from 0.12 to 43.4 mmol/L. The value of pH increased from 7.25 to 8.52.
Figure 11. Time curses of pH (gray), soluble Ca (black), soluble Mg (green) and NH4+ (light magenta) in medium with (A) only V. natriegens, and (B) co-cultures of B. subtilis and V. natriegens.
Numerous studies have shown that corrosion of steel can be accelerated by the facultative anaerobic bacterium V. natriegens, which has the capability of N2-fixation. The process of biological N2-fixation is the reduction of N2 to ammonium:
where the N2-fixation is a complex process that requires Mg2+, nitrogenases, and electron carriers. The hydrolysis of two Mg-ATP is accompanied by electron transfer. It has been shown that V. natriegens can accelerate the corrosion of steel by electron transfer and the nitrogenases associated with N2-fixation. Here, we present evidence that B. subtilis can inhibit V. natriegens-induced corrosion via biomineralization. Our previous work showed that B. subtilis could induce calcium carbonate precipitation on the surface of steel, decreasing the concentration of Ca2+ and Mg2+ in the medium. Furthermore, the pH in the medium inoculated with V. natriegens and B. subtilis was approximately 8.5, which is higher than the pH in the presence of only V. natriegens (approximately 7.9) (Figure 11). The increase in pH is attributed to the process by which microorganisms decompose nitrogenous organic compounds to produce ammonia via bacterial ammonification (Dannenmann et al., 2009). Microbial decomposition of organic nitrogen-containing compounds is the hydrolysis of macromolecules into small molecules by hydrolases secreted in vitro under aerobic or anaerobic conditions (Pan et al., 2018). As a microorganism with the capacity for ammonification, B. subtilis can decompose proteins and various nitrogen-containing organic substances to ammonium ions (NH4+) and bicarbonate (HCO3-), effectively, increasing the pH of the medium (Lipman, 1909; Hoffmann et al., 1998). Therefore, the N2-fixation process would be inhibited in the environment, which has a low concentration of Mg2+ and high pH value. In addition, the biodiversity experiment demonstrated that B. subtilis has a growth advantage in a competitive bacterial medium, even though V. natriegens was reported to have the shortest doubling time (9.8 min at 37°C). As a result, the initial biofilm structure is composed primarily of B. subtilis and not V. natriegens. Thus, calcium carbonate precipitation can be induced, thereby impeding the electron transfer between the anode and cathode on the steel surface. Consistent with these results, we found that the pitting corrosion was improved by the addition of B. subtilis via biomineralized film formation on the steel surface. The compact and hierarchical organic/inorganic film strongly contributed to the reduction of Cl- permeation and electron transfer.
For the real application in the field, this method may be used in two approaches. First, we can use this method in a closed environment, e.g., ballast tank and pipeline, where have the relatively simple species of bacteria. When the corrosion occurs, the B. subtilis provides the anticorrosion activity for the steel as a corrosion inhibitor. Second, biomineralization can be used as a pretreatment method, leading to a high corrosion-resistance for the steel. Therefore, the culturing conditions that can be extended to the large scale treatment should be investigated carefully in the future.
In this study, we evaluated the application of a biomineralized film in a competitive bacterial environment containing the corrosion-inducing bacterium. B. subtilis provided significant anti-corrosion protection for steel coupons in the presence of the corrosion-inducing bacteria, V. natriegens. Biomineralized film induced by B. subtilis was well formed on the steel surface in the co-cultures medium. The growth of V. natriegens was inhibited by B. subtilis due to the decrease in magnesium and the increase in pH within the medium as a result of biomineralization. Therefore, the results from this study show that B. subtilis-induced biomineralized film has the potential to be used in a real marine environment.
No datasets were generated or analyzed for this study.
ZG, SP, QZ, YW, NG, XC, YD, and YY performed experiments and analyzed data. ToL provided software. ZG and TaL designed the experiments, analyzed data, and wrote the manuscript.
This work was supported by National Key R&D Program of China (Nos. 2016YFB0300700, 2016YFB0300702, and 2016YFB0300704) and China Postdoctoral Science Foundation (2018M641980).
The authors declare that the research was conducted in the absence of any commercial or financial relationships that could be construed as a potential conflict of interest.
We thank LetPub (www.letpub.com) for its linguistic assistance during the preparation of this manuscript.
The Supplementary Material for this article can be found online at: https://www.frontiersin.org/articles/10.3389/fmicb.2019.01111/full#supplementary-material
Aiyar, S. E., Gaal, T., and Gourse, R. L. (2002). rRNA promoter activity in the fast-growing bacterium Vibrio natriegens. J. Bacteriol. 184, 1349–1358. doi: 10.1128/jb.184.5.1349-1358.2002
Andreeva, D. V., Fix, D., Mohwald, H., and Shchukin, D. G. (2008). Self-healing anticorrosion coatings based on pH-sensitive polyelectrolyte/inhibitor sandwichlike nanostructures. Adv. Mater. 20, 2789–2794. doi: 10.1002/adma.200800705
Angell, P., Luo, J. S., and White, D. C. (1995). Microbially sustained pitting corrosion of 304 stainless steel in anaerobic seawater. Corros. Sci. 37, 1085–1096. doi: 10.1016/0010-938X(95)00016-D
Bagherzadeh, M. R., and Mousavinejad, T. (2012). Preparation and investigation of anticorrosion properties of the water-based epoxy-clay nanocoating modified by Na+-MMT and Cloisite 30B. Prog. Org. Coat. 74, 589–595. doi: 10.1016/j.porgcoat.2012.02.006
Banerjee, S., Srivastava, V., and Singh, M. M. (2012). Chemically modified natural polysaccharide as green corrosion inhibitor for mild steel in acidic medium. Corros. Sci. 59, 35–41. doi: 10.1016/j.corsci.2012.02.009
Cheng, S., Tian, J., Chen, S., Lei, Y., Chang, X., Liu, T., et al. (2009). Microbially influenced corrosion of stainless steel by marine bacterium Vibrio natriegens: (I) Corrosion behavior. Mater. Sci. Eng. C 29, 751–755. doi: 10.1016/j.msec.2008.11.013
Coyer, J. A., Cabello-Pasini, A., Swift, H., and Alberte, R. S. (1996). N2 fixation in marine heterotrophic bacteria: dynamics of environmental and molecular regulation. Proc. Natl. Acad. Sci. U.S.A. 93, 3575–3580. doi: 10.1073/pnas.93.8.3575
Dannenmann, M., Simon, J., Gasche, R., Holst, J., Naumann, P. S., and Papen, H. (2009). Tree girdling provides insight on the role of labile carbon in nitrogen partitioning between soil microorganisms and adult European beech. Soil Biol. Biochem. 41, 1622–1631. doi: 10.1016/j.soilbio.2009.04.024
Gauzzi, A., Mathieu, H. J., James, J. H., and Kellett, B. (1990). AES, XPS and SIMS characterization of YBa2Cu3O7 superconducting high Tc thin films. Vacuum 41, 870–874.
Hoffart, E., Grenz, S., Lange, J., Nitschel, R., and Muller, F. (2017). High substrate uptake rates empower Vibrio natriegens as production host for industrial biotechnology. Appl. Environ. Microbiol. 83:e01614-17. doi: 10.1128/AEM.01614-17
Hoffmann, T., Frankenberg, N., Marino, M., and Jahn, D. (1998). Ammonification in Bacillus subtilis utilizing dissimilatory nitrite reductase is dependent on resDE. J. Bacteriol. 180, 186–189.
Li, X. G., Zhang, D. W., Liu, Z. Y., Li, Z., Du, C. W., and Dong, C. F. (2015). Share corrosion data. Nature 527, 441–442. doi: 10.1007/s10584-015-1507-y
Lipman, C. B. (1909). Toxic and antagonistic effects of salts as related to ammonification by Bacillus subtilis. Bot. Gaz. 48, 105–125. doi: 10.1086/329972
Little, B. J., Lee, J. S., and Ray, R. I. (2008). The influence of marine biofilms on corrosion: a concise review. Electrochim. Acta 54, 2–7. doi: 10.1016/j.electacta.2008.02.071
Liu, H., Gu, T., Zhang, G., Cheng, Y., Wang, H., and Liu, H. (2016). The effect of magneticfield on biomineralization and corrosion behavior of carbon steel induced by iron-oxidizing bacteria. Corros. Sci. 102, 93–102. doi: 10.1016/j.corsci.2015.09.023
Liu, J., Liu, T., Guo, Z., Guo, N., Lei, Y., Chang, X., et al. (2018). Promoting barrier performance and cathodic protection of zinc-rich epoxy primer via single-layer graphene. Polymers 10, 591–594. doi: 10.3390/polym10060591
Liu, T., Guo, Z., Zeng, Z., Guo, N., Lei, Y., Liu, T., et al. (2018). Marine bacteria provide lasting anticorrosion activity for steel via biofilm-induced mineralization. ACS Appl. Mater. Interfaces 10, 40317–40327. doi: 10.1021/acsami.8b14991
Liu, T., Wang, Y., Pan, S., Zhao, Q., Zhang, C., Gao, S., et al. (2019). The addition of copper accelerates the corrosion of steel via impeding biomineralized film formation of Bacillus subtilis in seawater. Corros. Sci. 149, 153–163. doi: 10.1016/j.corsci.2019.01.010
Ma, H., Gu, Y., Gao, H., Jiao, X., Che, J., and Zeng, Q. (2018). Microstructure, chemical composition and local corrosion behavior of a friction stud welding joint. J. Mater. Eng. Perform. 27, 666–676. doi: 10.1007/s11665-018-3182-4
Mullenger, L., and Gill, N. R. (2010). Vibrio natriegens: a rapidly growing micro-organism ideally suited for class experiments. J. Biol. Educ. 7, 33–39. doi: 10.1080/00219266.1973.9653881
NACE (1999). Preparation, Installation, Analysis, and Interpretation of Corrosion Coupons in Oilfield Operations NACE Standard RP0775-1999-R2017. Houston, TX: NACE.
Nadia, D. B. R., Conte, M., Guezennec, J., and Prieur, D. (1991). Monitoring of a Vibrio natriegens and Desulfovibrio vulgaris marine aerobic biofilm on a stainless steel surface in a laboratory tubular flow system. J. Appl. Microbiol. 71, 244–251. doi: 10.1111/j.1365-2672.1991.tb04455.x
Nkuzinna, O. C., Menkiti, M. C., Onukwuli, O. D., Mbah, G. O., Okolo, B. I., Egbujor, M. C., et al. (2014). Application of factorial design of experiment for optimization of inhibition effect of acid extract of Gnetum africana on copper corrosion. Nat. Resour. 5, 299–307. doi: 10.4236/nr.2014.57028
Pan, J., Wei, C., Fu, B., Ma, J., Preis, S., Wu, H., et al. (2018). Simultaneous nitrite and ammonium production in an autotrophic partial denitrification and ammonification of wastewaters containing thiocyanate. Bioresour. Technol. 252, 20–27. doi: 10.1016/j.biortech.2017.12.059
Shchukin, D. G., Zheludkevich, M., Yasakau, K., Lamaka, S., Ferreira, M. G. S., and Möhwald, H. (2006). Layer-by-layer assembled nanocontainers for self-healing corrosion protection. Adv. Mater. 18, 1672–1678. doi: 10.1002/adma.200502053
Tan, F., Wu, J., Zhang, D., Li, E., Sun, Y., and Gao, J. (2018). Corrosion of Q235 carbon steel influenced by the introduction of aerogenic and aerobic bacteria. Mater. Corros. 69, 1196–1204. doi: 10.1002/maco.201810086
Thomas, D. H., Rey, M., and Jackson, P. E. (2002). Determination of inorganic cations and ammonium in environmental waters by ion chromatography with a high-capacity cation-exchange column. J. Chromatogr. A 956, 181–186. doi: 10.1016/S0021-9673(02)00141-3
Weinstock, M. T., Hesek, E. D., Wilson, C. M., and Gibson, D. G. (2016). Vibrio natriegens as a fast-growing host for molecular biology. Nat. Methods 13, 849–851. doi: 10.1038/nmeth.3970
Xu, D., Zhou, E., Zhao, Y., Li, H., Liu, Z., Zhang, D., et al. (2018). Enhanced resistance of 2205 Cu-bearing duplex stainless steel towards microbiologically influenced corrosion by marine aerobic Pseudomonas aeruginosa biofilms. J. Mater. Sci. Technol. 34, 1325–1336. doi: 10.1016/j.jmst.2017.11.025
Keywords: low-alloyed steel, Bacillus subtilis, Vibrio natriegens, seawater, corrosion inhibition, biomineralization
Citation: Guo Z, Pan S, Liu T, Zhao Q, Wang Y, Guo N, Chang X, Liu T, Dong Y and Yin Y (2019) Bacillus subtilis Inhibits Vibrio natriegens-Induced Corrosion via Biomineralization in Seawater. Front. Microbiol. 10:1111. doi: 10.3389/fmicb.2019.01111
Received: 21 February 2019; Accepted: 01 May 2019;
Published: 21 May 2019.
Edited by:
Dake Xu, Northeastern University, ChinaReviewed by:
Yingchao Li, China University of Petroleum, ChinaCopyright © 2019 Guo, Pan, Liu, Zhao, Wang, Guo, Chang, Liu, Dong and Yin. This is an open-access article distributed under the terms of the Creative Commons Attribution License (CC BY). The use, distribution or reproduction in other forums is permitted, provided the original author(s) and the copyright owner(s) are credited and that the original publication in this journal is cited, in accordance with accepted academic practice. No use, distribution or reproduction is permitted which does not comply with these terms.
*Correspondence: Tao Liu, bGl1dGFvQHNobXR1LmVkdS5jbg== Tong Liu, bGl1dG9uZ0BxdXN0LmVkdS5jbg==
Disclaimer: All claims expressed in this article are solely those of the authors and do not necessarily represent those of their affiliated organizations, or those of the publisher, the editors and the reviewers. Any product that may be evaluated in this article or claim that may be made by its manufacturer is not guaranteed or endorsed by the publisher.
Research integrity at Frontiers
Learn more about the work of our research integrity team to safeguard the quality of each article we publish.