- 1Institute of Plant Protection, Jiangsu Academy of Agricultural Sciences, Nanjing, China
- 2School of the Environment and Safety Engineering, Jiangsu University, Zhengjiang, China
Rice false smut fungus (teleomorph: Villosiclava virens; anamorph: Ustilaginoidea virens) can generate chlamydospores and survive winter under field conditions. The chlamydospore is considered as an important infection source of the disease. However, little is known about the regulatory mechanism of the chlamydospore production. In this study, we identified a defective homeobox transcription factor (designated as UvHOX2) gene in a U. virens random insertional mutant B-766 that could not form chlamydospores. To confirm the regulatory function of UvHOX2, an Agrobacterium tumefaciens mediated transformation- and CRISPR/Cas9- based targeted gene replacement method was developed. The UvHox2 deletion mutants completely failed to produce chlamydospores, showed reduced conidia production and decreased virulence, and was hyper-sensitive to oxidative, osmotic, and cell wall stresses. We confirmed that UvHOX2 is located in the nuclei of U. virens, and the expression of UvHox2 was the strongest during the early stage of chlamydospore and conidium formation. Global transcription pattern of UvHOX2 was also determined by RNA-seq in this study, and several genes that might be down-stream of UvHOX2 regulation were identified. The results will better our understanding of the molecular mechanism of chlamydospore formation in U. virens as a model fungus.
Introduction
The heterothallic ascomycetous fungus Ustilaginoidea virens (Cooke) Takah (Teleomorph: Villosiclava virens) is the causal agent of rice false smut (RFS), which has become an emerging disease in China and most rice-growing areas in the world (Guo et al., 2012; Nessa et al., 2015; Yu J.J. et al., 2015). The fungus infects stamen filaments of rice at the booting stage, hijacks rice nutrients, and transforms kernels into false smut balls containing a large number of yellow or dark green-pigmented chlamydospores (Hu et al., 2014; Meng et al., 2015; Song et al., 2016; Zheng et al., 2016). Occasionally, sclerotia could form on the surface of false smut balls in late autumn when the temperature falls (Yu et al., 2016; Yong et al., 2018). Previous studies have revealed that chlamydospores could survive in nature and play an important role in the epidemiology of RFS disease between seasons (Fan et al., 2016).
In the human pathogenic yeast Candida albicans, several genes were found to be involved in chlamydospore formation, including homeobox transcription factor (TF) gene grf10 (Ghosh et al., 2015), phosphate mannose synthase encoding genes (Juchimiuk et al., 2015), mitogen-activated protein kinase gene hog1 (Eisman et al., 2006), gene encoding dolichol phosphate mannose synthase (Juchimiuk et al., 2015), chromatin remodeling complex gene isw2 (Nobile et al., 2003; Navarathna et al., 2016), MDS3, RIM101, RIM13, SCH9, and SUV3 (Nobile et al., 2003). Meanwhile, very few genes in filamentous fungi were found relative to chlamydospore formation, the limited examples include VELVET gene vel1 in biocontrol fungus Trichoderma virens (Mukherjee and Kenerley, 2010); and a group of genes in Clonostachys rosea identified from a study using RNA-seq (Sun et al., 2018). So far, the molecular mechanisms of chlamydospore formation remain elusive in filamentous fungi.
Homeobox genes encode a group of TFs, which contain a conserved homeobox domain and bind to specific DNA sequences (Gehring, 1987). In eukaryotic cells, these homeobox TFs play an important role in regulation of cell differential and development (Liu et al., 2010; Antal et al., 2012). The first reported homeobox gene in filamentous ascomycetes is pah1 in Podospora anserine (Arnaise et al., 2001). Pah1 deletion mutant showed increased production of microconidia and reduced growth rate of mycelia. In model fungus Neurospora crassa, three homeobox genes were characterized (Colot et al., 2006). Specifically, deletion of kal-1(pah1 homolog)led to defects in mycelia growth and conidiation; bek-1 was found to be essential for perithecial development whereas the third homeobox gene (Genbank accession number: NCU03070) was not described.
In recent years, several homeobox genes were systematically studied in filamentous fungi Porthe oryzea and Podospora anserine, and the results confirmed that these homeobox genes play a regulatory role in conidium and fruiting body development, as well as host infection (Kim et al., 2009; Coppin et al., 2012).
In this study, we identified a chlamydospore formation defect U. virens mutant B-766 from a random insertional mutant library that was constructed previously (Yu M.N. et al., 2015). A homeobox gene (annotated as UvHox2) was confirmed to be involved in the regulation of chlamydospore formation and pathogenicity in U. virens. A CRISPR/Cas9 system based on Agrobacterium tumefaciens mediated transformation (ATMT) was developed for targeted gene deletion. Moreover, comparative transcriptional analysis of UvHox2 deletion mutant and a wild-type strain was performed in this study. Taken together, the findings from this work will help us understand the regulatory mechanism of chlamydospore formation better.
Materials and Methods
Strains, Rice Variety, Plasmids, and Nucleotide Acids Manipulation
A virulent wild-type U. virens strain P-1 was used as starting strain in this study. A rice variety susceptible to U. virens, Liangyoupeijiu, was used in the inoculation experiments. The plasmid pCas9-tRp-gRNA was kindly provided by Dr. Jingrong Xu at Northwest A&F University (Liang et al., 2018). A. tumefaciens strain AGL-1, plasmid pmCherry-hph, pCambia-1300, pBHt2, pKHt, and pCN3EXPS were from our lab. Southern blot and thermal asymmetric interlaced PCR (TAIL-PCR) were performed as described previously (Yu M.N. et al., 2015).
Phenotypic Analysis of U. virens Strains/Mutants
The U. virens wild-type strain P-1 was routinely cultured on a potato sucrose agar medium (PSA) at 28°C for 10–15 days (Zheng et al., 2017). The transformants of P-1 were cultured on the PSA amended with 100 μg/ml hygromycin and/or 600 μg/ml geneticin 418 (G418). We used YT medium and broth to test mycelial growth rate and conidiation ability of U. virens, respectively (Tanaka et al., 2011). To determine the chlamydospore formation and the pathogenicity of U. virens strains, we inoculated rice following the method described previously (Zheng et al., 2017). Fifteen spikes were inoculated for each strain, and the number of false smut balls was counted 25 days after the inoculation. The chlamydospore formation structures on the surface of false smut balls were observed by scanning electron microscope (SEM). To stimulate chlamydospore formation in U. vires, mycelia dishes cut from the edge of fresh colonies were put on PSA medium. The cultures were incubated at 28°C under diffuse light for 2–3 months.
Ustilaginoidea virens strains were cultured on PSA medium to determine the growth rate. YT medium amended with 0.05% H2O2, 0.4 mol/l NaCl, 0.03% SDS, and 100 mg/l congo red were used to test sensitivity of stains to abiotic stresses. The cultures were incubated at 28°C for 15 days in darkness, and then the growing diameter was measured and the morphology of the colonies were characterized. Four duplicates were performed for each treatment. The sporulation capacity of the strains was determined as follows. Ten pieces of fresh mycelial dishes from each treatment were cultured in a 250 ml conical flask containing 100 ml YT liquid medium. The conical flasks were incubated at 28°C, 150 r/min for 6 days, and then the thin-wall conidia were counted with a blood cell counting chamber. To observe conidium generation structures, strains were cultured on minimal media (MM) (Gupta and Chattoo, 2008) for 10 days.
Generation of ATMT Binary Vector for Gene Deletion With CRISPR/Cas9
Generation of Gene Deletion Vector With CRISPR/Cas9
We constructed CRISPR-guideRNA(gRNA) cassettes from pCrispr-UvtR and gene replacement cassettes [upstream flank (UF)-hygromycin resistant gene(Hyg+)-downstream flank (DF)] of UvHox2 into two T-DNA regions of binary vector pCccd-dTN3, respectively. The details of vectors construction were described in Supplementary Figure S1.
Generation of Gene Deletion Mutants
Agrobacterium tumefaciens mediated transformation was performed as described previously (Yu M.N. et al., 2015). The A. tumefaciens strains AGL-1 containing pdTN3-HX2-Cas9I or pdTN3-HX2-Cas9II was employed in transformation of U. virens wild-type strain P-1. The U. virens P-1 and A. tumefaciens AGL-1 were co-cultured on nitrocellulose membrane for 3 days and then transferred onto 2% TB3 [0.3% yeast extract, 0.3% casamino acid, 1% glucose, 2% sucrose (w/v)]. To make a selective medium, 400 μg/ml cefotaxime and 150 μg/ml timentin were added into 2% TB3 medium to inhibit the growth of A. tumefaciens, and 100 μg/ml hygromycin and 600 μg/ml G418 were added into 2% TB3 medium to select transformants containing both cassettes of UF-HYG+-DF and CRISPR/Cas9-gRNA, respectively. The UvHox2 deletion mutants were screened and verified by PCR with primers P19∼P26 listed in Supplementary Table S1.
Generation of UvHox2-eGFP Constructs
The open reading frame (ORF) of UvHox2 was amplified from cDNA that was generated through reverse transcription of total RNA using the primer pair P27–P28 (Supplementary Table S1). The enhanced green florescent protein (eGFP) fragment was amplified with primer pair P29–P30 (Supplementary Table S1). UvHox2-eGFP fusion cassette was generated via double-joint PCR and ligated to BamH I-EcoR I digested pCN3EXPS to construct UvHox2-eGFP fusion vector pCN3EXPS-HX2-eGFP, in which the UvHox2-eGFP cassette was under the control of glyceraldehyde-3-phosphate dehydrogenase promoter of Cochliobolus heterostrophus. Subsequently, the vector was used to transform U. virens via ATMT protocol to generate UvHox2-eGFP over-expression mutants.
qRT-PCR Assays
Vegetative mycelia were collected from 2-day-old YT cultures that started with 1 × 106 conidia/ml. To stimulate sporulation in U. virens, mycelial dishes were cultured in YT broth by shaking for 3 days (initial stage of sporulation) or 7 days (later stage of sporulation). To collect samples undergoing chlamydospores formation, 20 rice spikes were inoculated for each strain/mutant. Rice smut balls at the initial stage [yellowish with intact membrane] and the later-stage [yellowish without membrane] of chlamydospore development were collected as described by Fan et al. (2016). PrimeScriptTM RT reagent Kit with gDNA Eraser (Takara) and SYBR® Premix Ex TaqTM II (Takara) were used to synthesize cDNA and quantitative RT-PCR (qRT-PCR). Relative expression levels of genes were calculated with the 2-ΔΔCt method. The α-tubulin gene was employed as the endogenous reference. Three biological replicates were performed to calculate the mean and the standard deviation.
Comparative Transcriptional Analysis of U. virens
Total RNA of U. virens was extracted employing TRIZOL (Invitrogen). RNA integrity was determined using Bioanalyzer 2100 RNA-6000 Nano Kit (Agilent Technologies). The construction and sequencing of mRNA-seq libraries and preprocessing and mapping of Illumina reads were performed as described previously (Yu et al., 2016). The DESeq software (Anders and Huber, 2012) was used to generate base mean based on FPKM, and to evaluate significant differences in base mean between different samples. Three biological replicates were performed for each strain/mutant.
Results
Characterization of Genes Relative to Chlamydospore Formation in Mutant B-766
In a preliminary study, we identified a T-DNA insertional mutant B-766 of U. virens, which failed to form chlamydospores on false smut balls (Figure 1). To determine the copy number of T-DNA inserted in B-766, 1.4 kb hygromycin resistant cassette was employed as a probe in southern blot. The result showed that three copies of T-DNA were detected in mutant B766 (Figure 2A). T-DNA flanking regions were amplified by TAIL-PCR (Yu M.N. et al., 2015). Three copies of T-DNA were inserted into the upstream of ORFs that encode proteins KDB15727 (Genbank accession number), KDB15728, KDB14847, KDB14848, and KDB18871 (Figure 2B). We then performed qRT-PCR to screen genes relative to chlamydospore formation in mutant B-766. The expression of KDB14847 in B-766 comparing to P-1 was reduced in a higher level than other genes that might be infected by T-DNA insertion in mutant B-766 (Figure 2C). Because KDB14847 is homologous to homeobox TF MoHOX2 in Magnaporthe oryzea, we designated KDB14847 as UvHOX2.
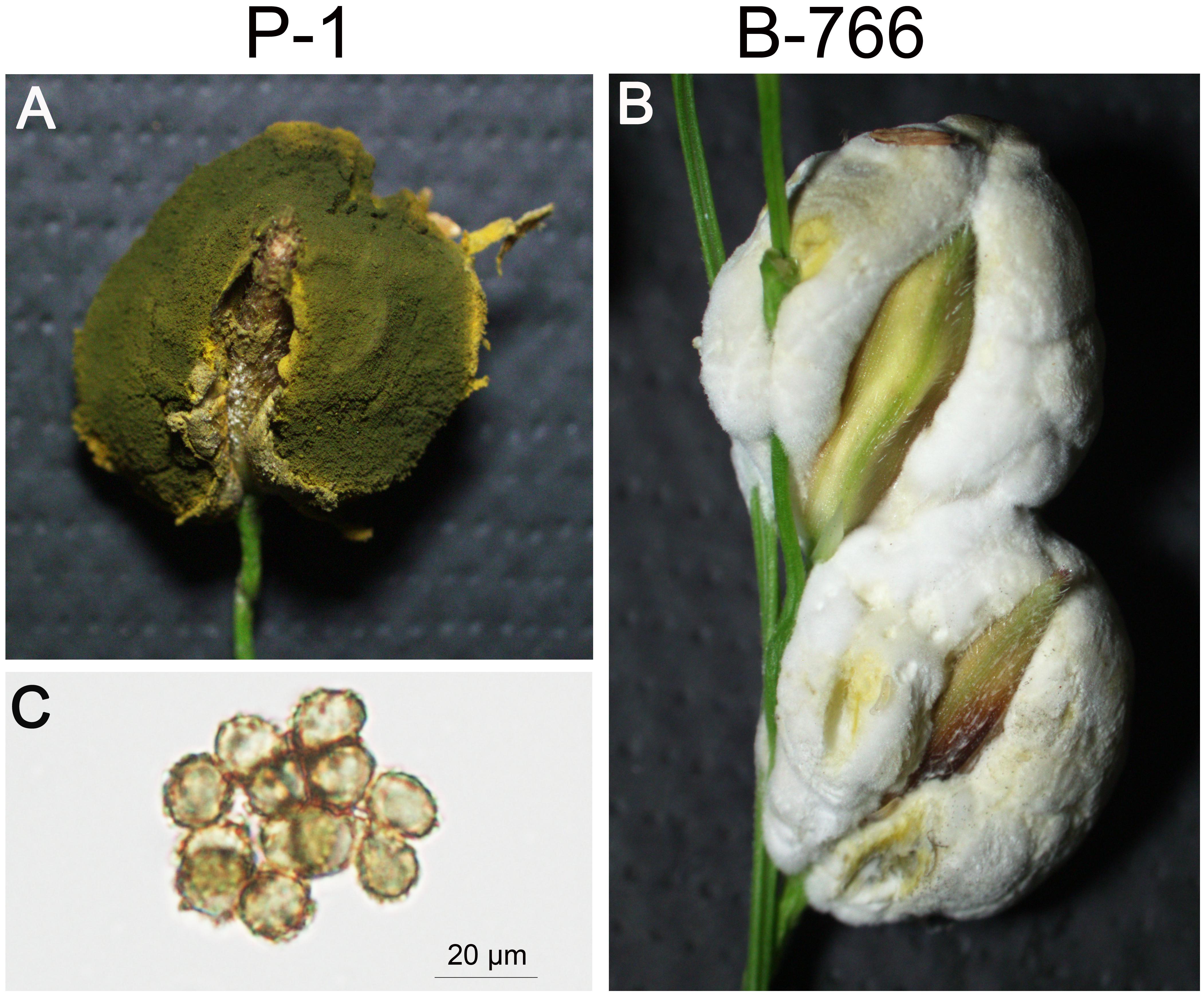
Figure 1. Rice false smut (RFS) balls of wild-type strain P-1 and T-DNA insertional mutant B-766 of U. virens. (A) RFS balls of wild-type strain P-1. (B) RFS balls of mutant B-766. (C) The chlamydospores formed on the false balls of wild-type strain P-1.
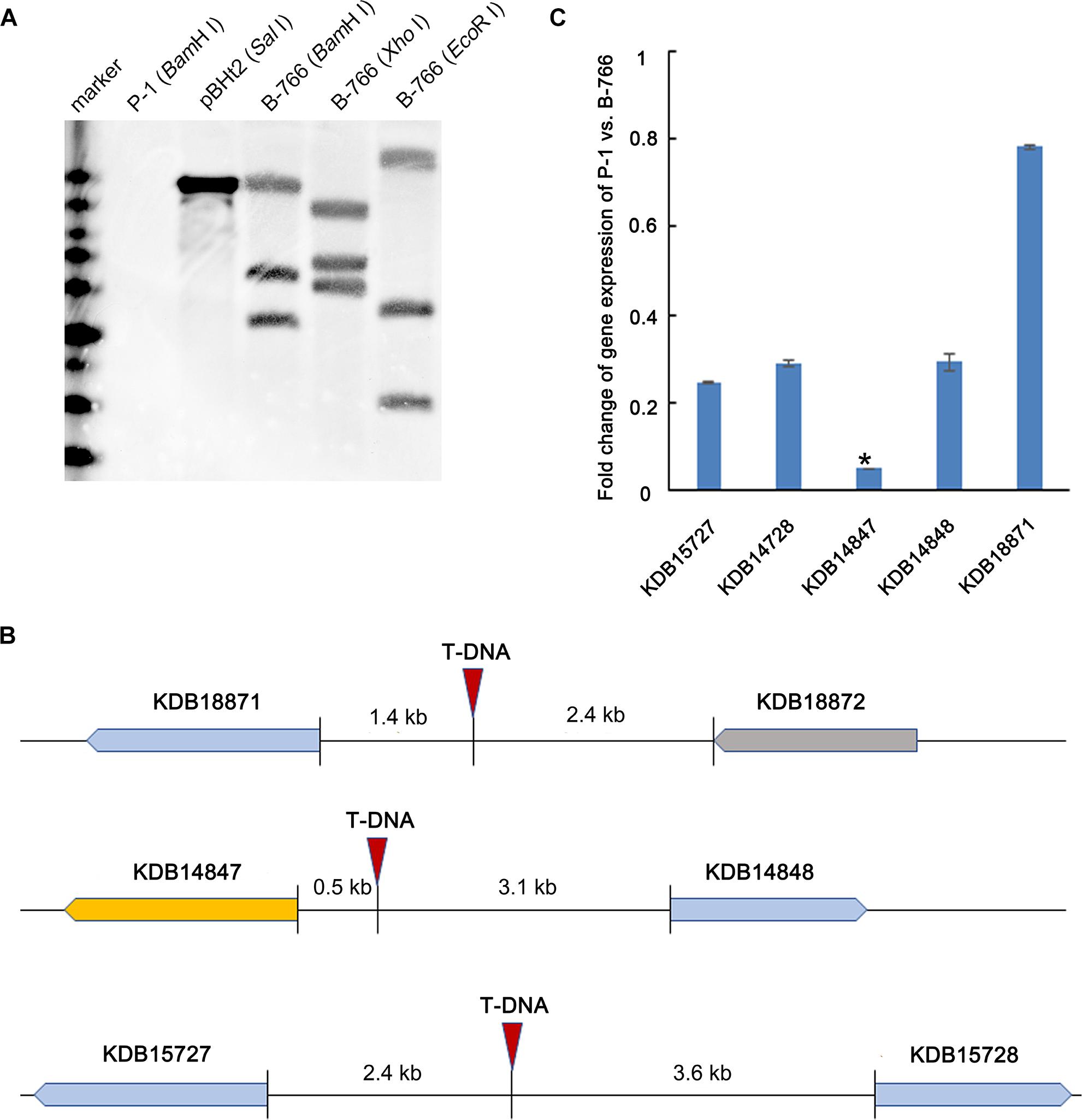
Figure 2. Characterization of T-DNA insertional mutant B-766. (A) Copy number analysis of T-DNA in B-766 by southern blot. (B) Illustration of insertion sites of T-DNA in B-766. (C) Fold change of gene expression of wild-type stain P-1 comparing to mutant B-766. The asterisk indicated that the fold change ofKDB14847 in B-766 comparing to P-1 of was significantly higher than KDB15727, KDB14728, KDB14848, and KDB18871.
Homeobox TFs in U. virens
In eukaryotic cells, homeobox TFs contain a ∼60 aa long conserved homeodomain that binds to specific DNA sequences and regulates transcription (Coppin et al., 2012). We identified seven homeobox TFs in U. virens using the InterPro term (IPR001356) and the NCBI assembly data of U. virens genome (accession number: GCA_000687475.1). The sequences of the homeobox TFs were deposited in the genbank with the accession number KDB17966, KDB17963.1, KDB17264, KDB14847, KDB13469, KDB13074, and KDB13172 (Figure 3A). The position of the homeodomain varied in the homeobox TFs in U. virens. The homeobox motif located at the N-terminus of KDB14847 (UvHOX2), KDB13469, and KDB13172, in the middle of KDB17963 and KDB13074, and at the C-terminus of KDB17966 (Figures 3A–D). Besides the homeobox domain, KDB17963 possessed a rhodanese-like domain at the C-terminus, KDB13172 harbored three zinc finger C2H2 DNA binding domains in the middle and an HTH CenpB-type DNA binding domain at the C-terminus (Figure 3A).
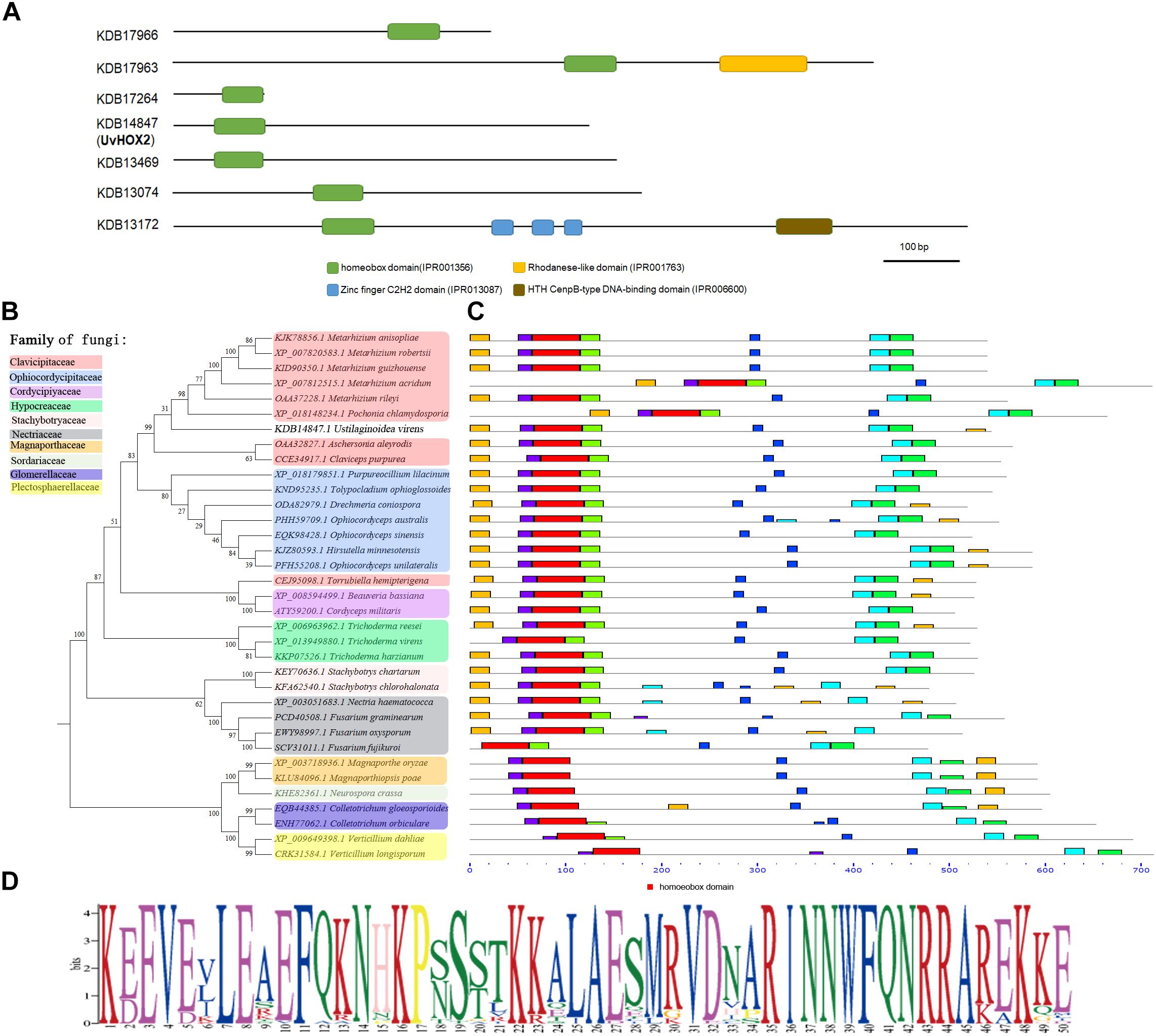
Figure 3. Phylogenetic analysis of homeobox transcription factors (TFs) in U. virens. (A) Positions of homeobox domain in homeobox TFs in U. virens. (B) Phylogenetic analysis of homeobox TFs that homologous to UvHOX2 in filamentous fungi. (C) Positions of homeobox domain (red) in UvHOX2 homologs. (D) Sequences of homeobox domain in filamentous fungi.
Generation of UvHOX2 Deletion Mutants
To delete UvHox2 in the U. virens wild-type strain P-1, we constructed two binary vectors pdTN3-HX2-Cas9I or pdTN3-HX2-Cas9II containing two T-DNA regions, respectively. The first T-DNA inserted with UF-HYG+-RF cassette, and the other one harbors CRIPSR/Cas9-gRNA (gRNA1 or gRNA2) and NEO+ cassettes. The ATMT method was used to co-transfer the two T-DNAs into the U. virens wild-type strain P-1. The transformants resistant to hygromycin B and G418 were picked from the selective medium after about 7–10 days culture at 28°C in darkness. For pdTN3-HX2-Cas9I, 9 out of 33 transformants were confirmed as UvHox2 deletion mutants. For pdTN3- HX2-Cas9II, 10 out of 45 transformants were confirmed as UvHox2 deletion mutants (Figure 4). The homologous gene replacement frequency was 27.3 and 22.2% for pdTN3-HX2-Cas9I and pdTN3-HX2-Cas9II, respectively. Potential off-target sites were checked using protocol described by Liang et al. (2018), and no off-target event was detected in all transformants.
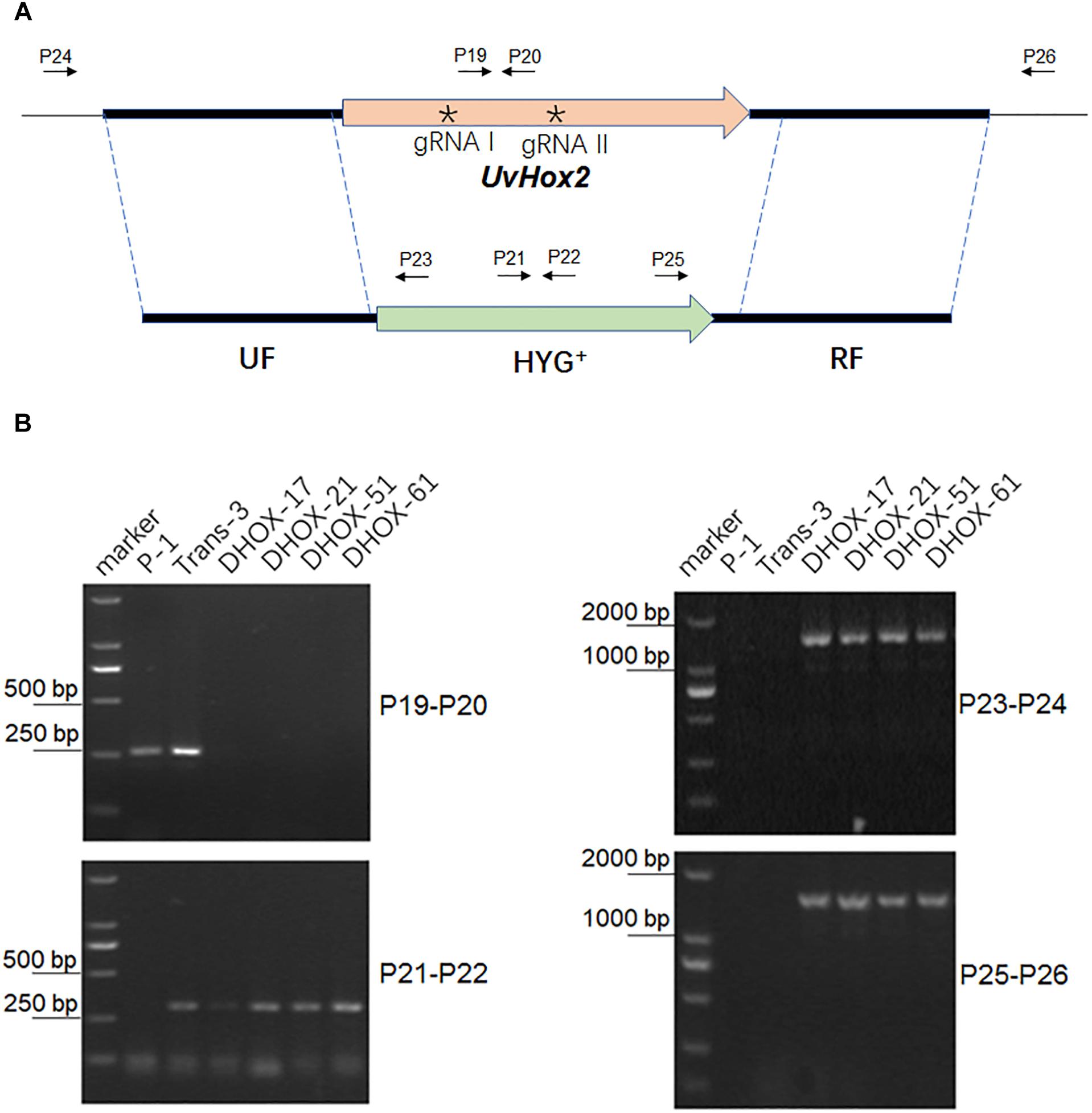
Figure 4. Deletion of the UvHOX2 gene in U. virens. (A) Illustration of targeted deletion of UvHOX2 utilizing CRISPR/Cas9 and homologous replacement. (B) PCR analysis of UvHOX2 deletion mutants DHOX-17, DHOX-21, DHOX-51, and DHOX-61. The wild-type strain P-1 and transformant Trans-3 with ectopic inserted UF-HYG+-RF cassette were included as controls. The asterisks indicates the positions of gRNA spacers (gRNA I and gRNA II) designed for CRISPR/Cas9.
UvHox2 Is Essential for Chlamydospore Formation and Contributes to Pathogenicity
UvHox2 deletion mutants DHOX17 and DHOX21 generated by pdTN3-HX2-Cas9I and DHOX51 and DHOX61 generated by pdTN3-HX2-Cas9II were then used to inoculate rice at booting stage on 15 spikes. Disease incidence of RFS was detected 25 days after inoculation. The rice smut balls were observed once again 60 days after innoculation to check the chlamydospore formation. The results showed that the virulence of the UvHox2 deletion mutants was significantly reduced compared to the wild-type strain P-1. The number of RFS balls on the spikes inoculated with the mutants DHOX-17, DHOX-21, DHOX-51, and DHOX-61 were 3.8 ± 1.6, 4.7 ± 2.6, 4.3 ± 1.6, and 4.3 ± 1.8, respectively; meanwhile, the number of smut balls on spikes inoculated with the wild-type strain P-1 was 21 ± 3.5 (Figure 5A and Table 1). The false smut ball samples were fixed with 2.5% glutaraldehyde in phosphate buffer pH 7.4 and subsequently observed under a SEM. The pictures showed that the chlamydospore formation structure was normal on the surface of false smut balls on the wild-type strain inoculated spikes. Short peg-like branches (sterigmata) were observed on the parallel arranged sporangiophore-like mycelia, and chlamydospores formed on these sterigmata in the wild-type strain P-1. In contrast, no special structure of hypha was observed on the false smut balls of UvHox2 deletion mutants, and the hyphae were abnormally curved and twined (Figure 5B). To test whether conidia of U. virens wild-type strain (WT)/mutants could occasionally be converted into chlamydospores in the absence of water, conidia of U. virens WT/mutants were placed on glass slides and kept in the 9 mm-diameter petri dishes. After 10-days of incubation at 28°C in darkness, we found a small portion of conidia wild-type strain P-1 and UvHox2 deletion mutants could both convert into chlamydospores (Figure 5C). The finding implied that UvHOX2 plays a key regulatory role in formation of chlamydospores rather than conversion from specific cells into chlamydospores.
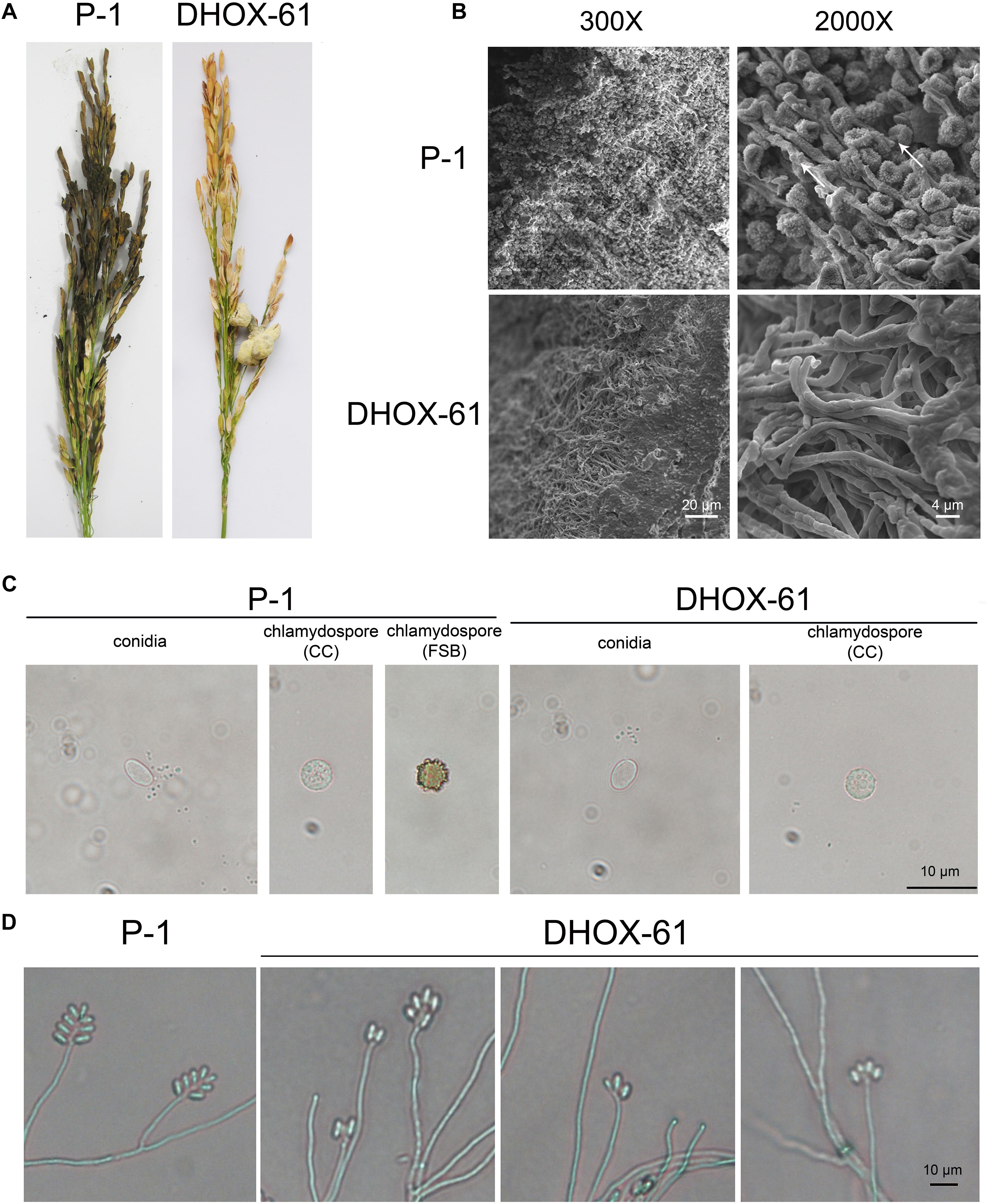
Figure 5. Rice false balls of wild-type strain P-1 and UvHOX-2 deletion mutant DHOX-61. (A) Rice spikes inoculated with P-1 or DHOX-61. (B) Chlamydospores on the surface of rice false ball of P-1 or DHOX-61 observed under a scanning electron microscope with 300× and 2000× amplification, respectively. The arrows indicate the short peg-like branches (sterigmata) were observed on the parallel arranged sporangiophore-like mycelia. (C) Chlamysopores of P-1 or DHPX-61 converted from conidia (CC) or formed on RFS balls (FSB). (D) Sporulation structures of P-1 or DHOX-61.
UvHOX2 Regulates Conidiogenesis
The conidial production of UvHox2 deletion mutants in YT broth was significantly reduced in comparison to that of the wild-type strain P-1 (Table 2). To observe the intact conidial sporulating structures, we cultured these strains (WT/mutants) on MM media. Wild-type strains produced typical conidial sporulating structures, but 86% of the UvHox2 deletion mutants produced abnormal sporulating structures (Figure 5D). Whereas, the conidia produced by the wild-type strain and the UvHox2 deletion mutant were the same in appearance.
UvHOX2 Is Not Critical for Mycelia Growth and Conidium Germination
In addition, mycelium growth rate and conidium germination rate of the UvHox2 deletion mutants was detected on YT media. The mycelium growth of UvHox2 deletion mutants were slightly reduced comparing to wild-type strains. And no significant differences in the germination of conidia were observed between the UvHox2 deletion mutant DHOX-61 and the wild-type strain P-1 (Table 2). This indicates that UvHOX2 is not critical in the regulation of mycelium growth and conidium germination in U. virens.
UvHox2 Deletion Mutants Exhibited Increased Sensitivity to Oxidative, Osmotic, and Cell Wall Stresses
When cultured on YT media amended with 0.05% H2O2 and 0.4 mol/l NaCl, the colony diameter of the UvHox2 deletion mutant DHOX-61 was significantly smaller than that of the wild-type strain P-1. When cultured on YT amended with 0.03% SDS and 100 mg/l congo red, the colony diameter of DHOX-61 was equivalent to that of P-1. However, the colony of DHOX-61 cultured on 100 mg/l congo red had shrinks and less aerial mycelia than P-1 (Table 3 and Figure 6). These findings showed that the UvHox2 deletion mutants were more sensitive to oxidative, osmotic and cell wall stresses than the wild-type strains. It suggests that UvHOX2 is also involved in responses to oxidative, osmotic, and cell wall stresses.
Subcellular Location and Expression Patterns of UvHOX2
The UvHOX2-eGFP construct was transformed into wild-type strain P-1. The mutant HOX-GFP-2 was picked from a batch of transformants for its strong signal of the over-expressed eGFP fusion protein. The eGFP signal in the HOX-GFP-2 mycelia located in the nuclei (Figure 7A). 4′,6-diamidino-2-phenylindole (DAPI) was used to dye the mycelia and show the location of the nuclei in the cells.
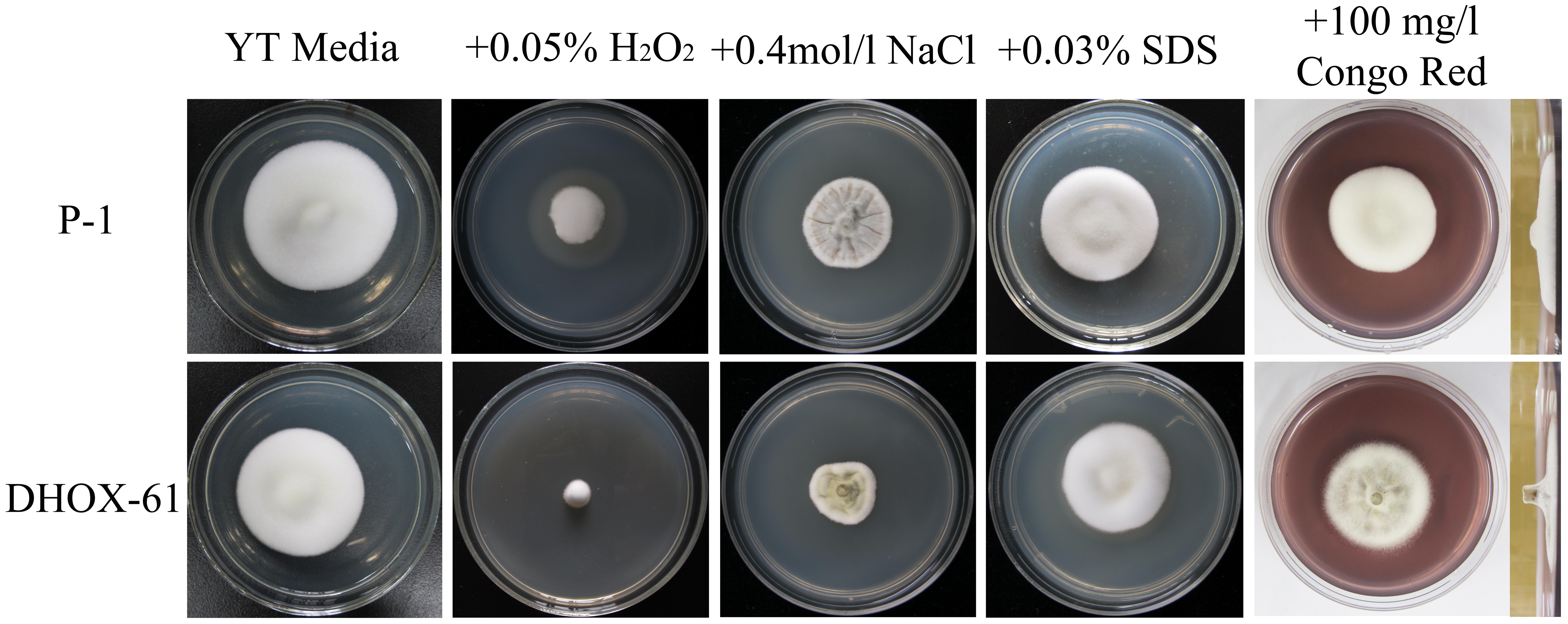
Figure 6. Growth of the UvHOX2 deletion mutant in the presence of different biotic stresses. The wild-type strain P-1, UvHOX2 deletion mutant DHOX-61 were cultured on YT medium or amended with 0.05% H2O2, 0.4 mol/l NaCl, 0.03% SDS and 100 mg/l congo red. Photographs were taken after incubation at 28°C for 15 days.
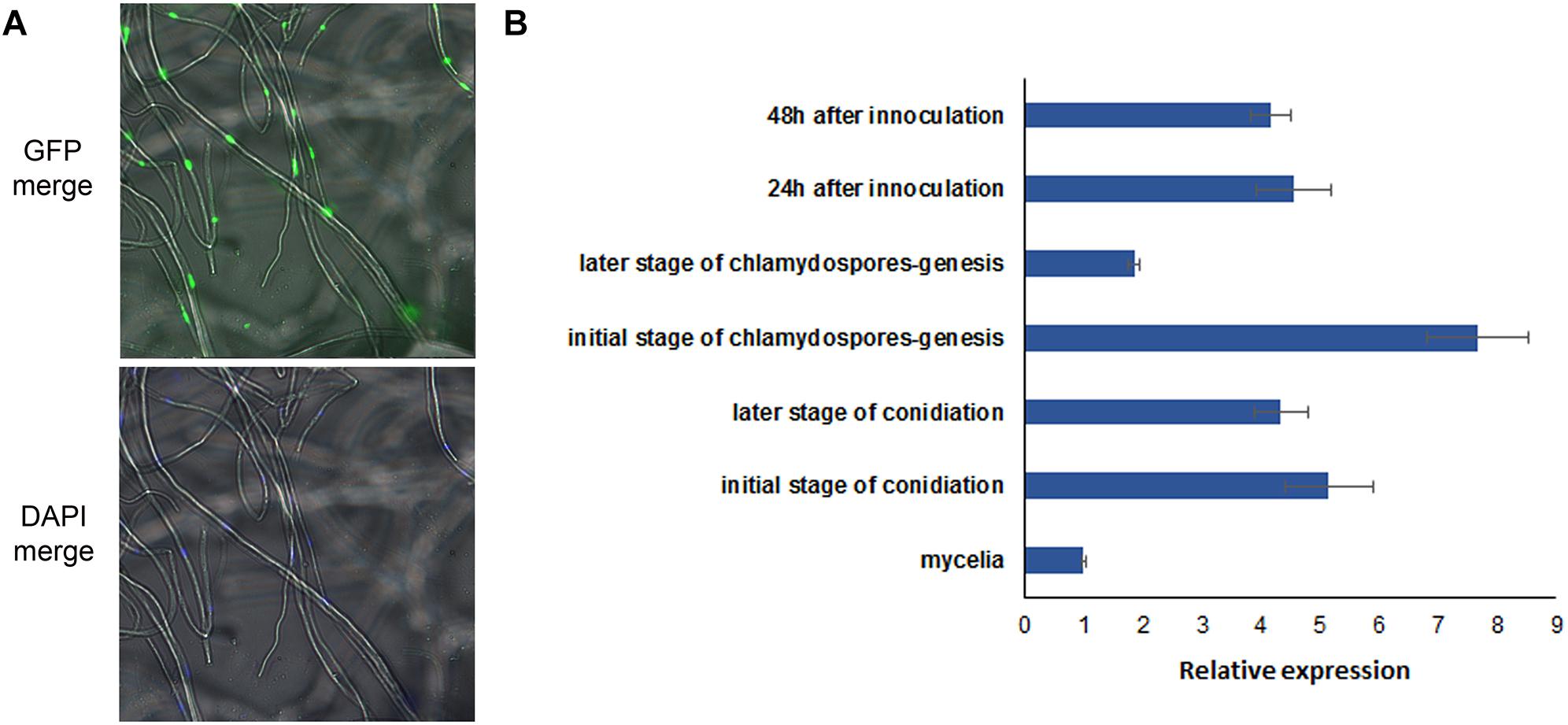
Figure 7. Location and expression pattern of UvHOX2 in U. virens. (A) UvHOX2-eGFP was activated and located in nuclei of mycelia. 4′,6-diamidino-2-phenylindole (DAPI) was used to stain nuclei in cells. (B) Expression pattern of UvHOX2 was determined by qRT-PCR. House-keeping gene elongation factor 1-α (EF1-α) was employed as a reference gene.
The expression levels of UvHox2 at the stages of mycelium growth, conidium generation, and chlamydospore formation were determined by qRT-PCR. The results showed that the expression of UvHox2 was the highest during the early stage of chlamydospore formation and decreased at the later stage of chlamydospore formation. Meanwhile, the expression of UvHox2 was also high at the sporulating stage and the early stage of infection on rice. In contrast, the expression of UvHox2 was low during vegetative growth. (Figure 7B).
The Global Transcription Pattern of UvHox2 Deletion Mutant Differs From That of the Wild Type at the Early Stage of Chlamydospore Development
To identify putative regulated targets of the homeobox TF UvHOX2 during the formation of the chlamydospore generation structure, we compared the global gene transcription patterns in the UvHox2 deletion mutant DHOX-61 with that in the wild-type strain P-1 by RNA-seq analysis. We inoculate P-1 (WTC) and DHOX-61 (DH) on rice as described above and collected rice false balls after 21 days. For false smut ball generated by P-1 (WTC), the membrane-like structure covering the false smut balls were intact then, and chlamydospore formation was at the early stage (Figure 8A).
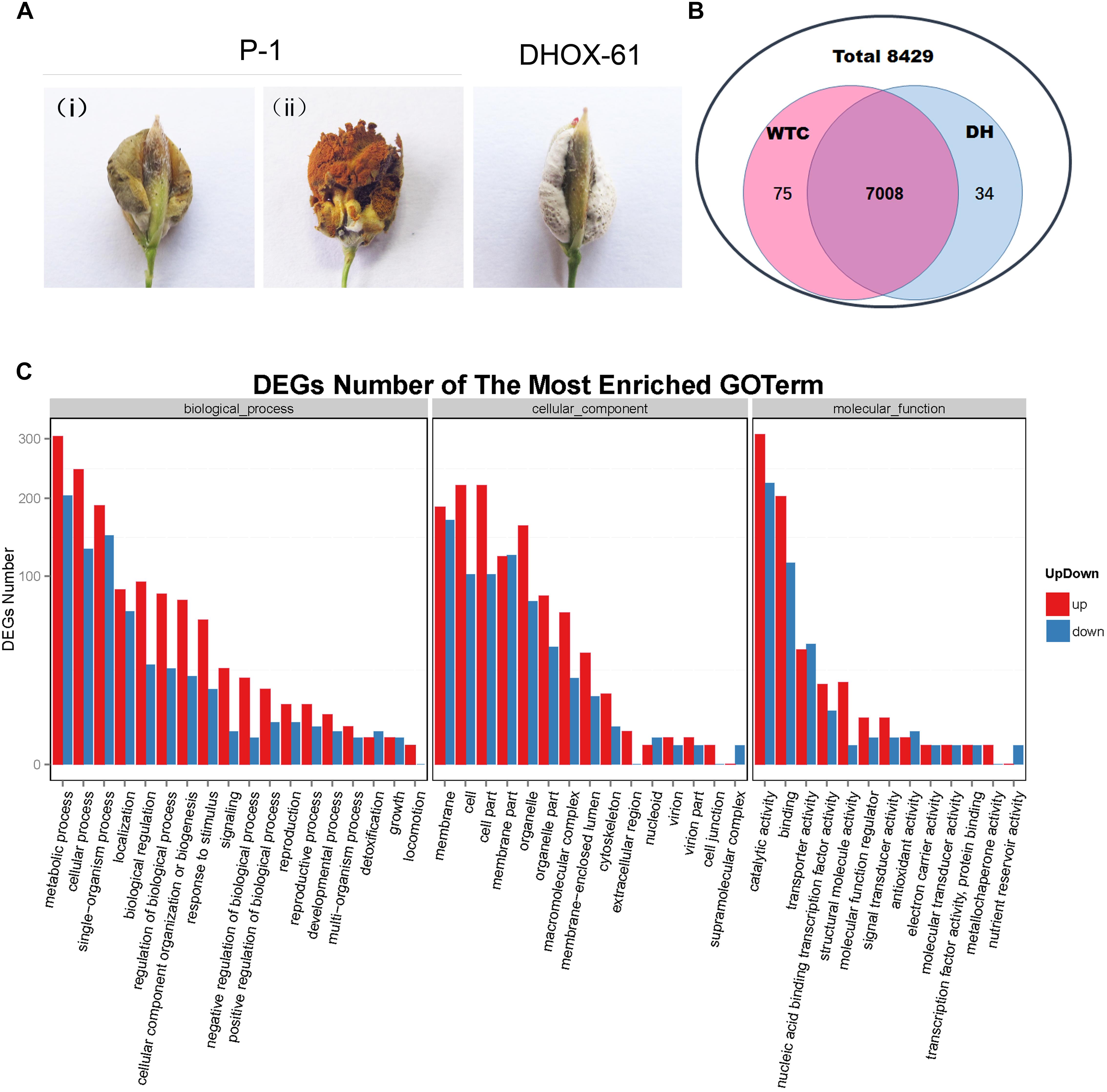
Figure 8. Comparative transcriptional analysis of genes regulated by UvHOX2 during chlamydospore development. (A) False smut ball samples were collected to perform RNA-seq and qRT-PCR assay. P-1 (wild-type strain of U. virens): (i) false smut balls at the initial stage of chlamydospore generation (WTC sample); (ii) false smut balls at the later stage of chlamydospore generation; DHOX-61 (UvHox2 deletion mutant of U. virens): false smut balls of DHOX-61 at the initial stage of chlamydospore formation. (B) Mapping and assembly statistics for WTC and DH samples. (C) Gene Ontology (GO) term of differentially expressed genes in DH vs. WTC. The most enriched GO terms were biological processes, cellular components, and molecular function.
The raw data of the RNA-seq was deposited in GenBank (accession number: SUB4385058). There was high Pearson correlation among duplicates. We found that the global transcription patterns of DH and WTC at the early stage of chlamydospore formation differed considerably. In a total of 8429 genes identified in U. virens previously (Zhang et al., 2014), 75 genes excluding UvHox2 were only expressed in WTC, 34 genes were barely expressed in DH, and 7008 overlapping genes were expressed in both WTC and DH (Figure 8B and Supplementary Table S2). Furthermore, we identified 1877 genes that have differential expression by at least twofold between WTC and DH, including 1185 genes being up-regulated and 692 genes being down-regulated in DH vs. WTC. These genes constituted approximately one-half of the differentially expressed genes, and they could be assigned to three major functional groups: biological process, cellular component, and molecular function (Figure 8C and Supplementary Table S2). In the biological process group, the top three subgroups of differentially regulated genes in DH vs. WTC were “metabolic process,” “cellular process,” and “single-organism process.” In the cellular component group, the top three subgroups of differentially expressed genes in DH vs. WTC were “membrane,” “cell,” and “cell part.” In the molecular function group, the top three subgroups of differentially expressed genes in DH vs. WTC were “catalytic activity,” “binding,” and “transporter activity.”
To validate the RNA-seq data, quantitative real-time polymerase chain reaction (qRT-PCR) was performed to confirm the differential expression of six selected genes. The qRT-PCR data for these genes were consistent with those obtained from RNA-seq (Supplementary Figure S2).
Genes Involved in Signal-Transduction Pathway
Several differentially expressed genes were detected in WTC, which were regarded as components in signal recognition and transduction system (Table 4). We also found that 43 genes encoding TFs were up-regulated during chlamydospore formation (Supplementary Table S2). And 19 of these genes were up-regulated beyond four folds (Table 4).
Genes Involved in Cell Wall Synthesis
Several differentially expressed genes were found to be closely linked to cell wall integrity. A gene encoding chitin deacetylase (KDB11455) were specially expressed in WTC but not in DH. Meanwhile, a chitin synthase (KDB11224) gene was up-regulated in WTC compared to DH. Chlamydospores of U. virens have thick cell walls. Chitin is an important component in cell wall, and fungi might mask chitin by deacetylating it into chitosan (Cord-Landwehr et al., 2016). These chitin synthases and deacetylase may play a key role in the thin cell wall synthesis in chlamydospores.
Genes Involved in Ubiquitination and Autophagy
Autophagy is a kind of intracellular recycling system that degrade cytoplasmic materials in lysosome/vacuole during development and in response to cell stresses in eukaryotic cells (Liu et al., 2017). During chlamydospore formation, a lot of cytoplasmic materials may be degraded and reutilized. Here we identified three genes involved in autophagy that were differentially expressed in DH vs. WTC (Table 4).
Genes Involved in Osmotic Response and Cell Membrane Integrity
We found ten osmotic stress responsive genes were differentially expressed in DH vs. WTC. Nine of these genes encoding components for osmolarity two-component response system were up-regulated in DH vs. WTC. Meanwhile, several genes encoding cell membrane components were found to be up-regulated in WTC (Table 4). Most of them are transporter genes, which suggested that U. virens cells need to exchange substances with the environment more frequently during chlamydospore formation.
Generation of Chlamydospores and Conidia Might Share a BrlA-AbaA-WetA Regulatory Pathway
In Aspergillus nidulans, Myb-like DNA-binding protein FlbD is required for early conidiophore development (Wieser and Adams, 1995; Dong et al., 2015; Matheis et al., 2017). FluG regulates FlbD via repressing SfgA, a negative regulator of FlbD. FlbD delivers signals to the down-stream regulatory component FlbB to activate conidiogenesis regulatory cascade BrlA-AbaA-WetA (Wu et al., 2018). In U. virens, we found that homologs of FluG (KDB12888), FlbD (KDB18803), BrlA (KDB11753), AbaA (KDB11305), and WetA (KDB15008) were expressed at a higher level at the initial stage of chlamydospore and conidim formation than that at the vegetative growth stage. Homologs of FluG and FlbD were up-regulated, while homologs of BrlA, AbaA, and WetA were down-regulated in both initial sporulation mycelia and false smut ball at the initial stage of chlamydospore formation in DH compared to WTC (Figure 9). This suggested that the generation of chlamydospores and conidia may share the BrlA-AbaA-WetA regulatory pathway, and BrlA-AbaA-WetA signal cascade was downstream the UvHOX2 regulation.
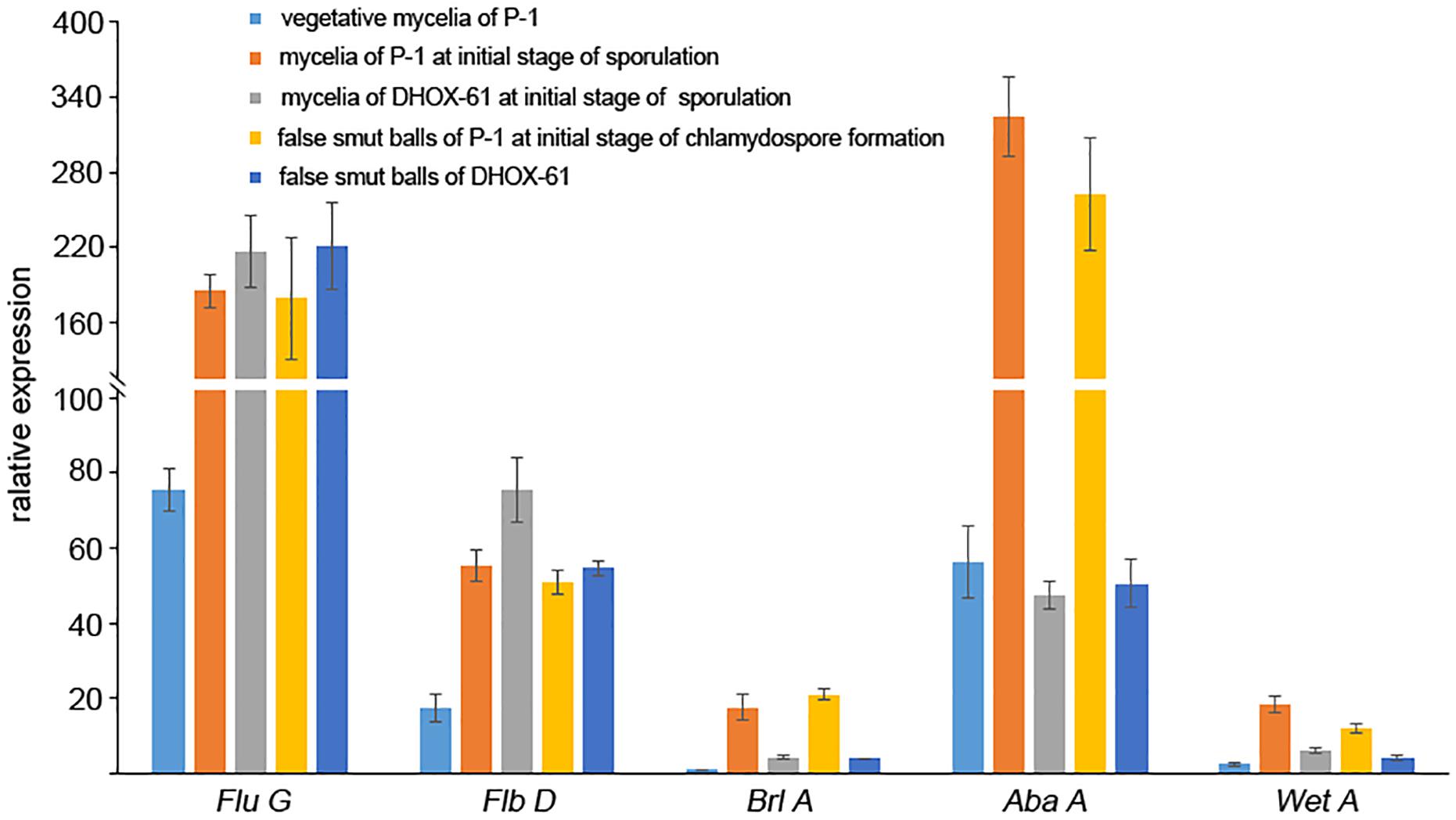
Figure 9. Expression of regulatory factors during chlamydospore and conidium formation in U. virens wild-type strain P-1 and UvHOX2 deletion mutant DHOX-61. The relative expression level of Flu D, Flb D, Brl A, Aba A, and Wet A at vegetative mycelia of P-1, mycelia of P-1 at initial stage of sporulation, mycelia of DHOX-61 at initial stage of sporulation stage, false smut balls of P-1 at initial stage of chlamydospores formation of U. virens, and false smut balls of DHOX-61.
Discussion
Chlamydospores are a type of asexual spores that allow fungi to survive unfavorable conditions. These thick-wall spores play important roles in epidemic of pathogenic fungi as asexual resting spores and/or infection resources. Famous chlamydospore producing plant pathogenic fungi include U. virens (Zhang et al., 2014), Fusarium oxysporum (Klein et al., 2011), F. sporotrichioides, and F. graminearum (Goh et al., 2009). Some well-studied fungal biocontrol agents in livestocks, for example, Trichoderma spp. (Li et al., 2005), Metarhizium anisopliae (Ment et al., 2010), Pochonia chlamydosporia (Wang et al., 2005), and Clonostachys rosea (Ahmed et al., 2014), also produce chlamydospores. The development of chlamydospore is generally controlled by regulatory networks stimulated by the environment. Here, we report a homeobox TF UvHOX2 that is essential for chlamydospore formation and also contributes to pathogenicity in U. virens. Additionally, we identified a group of genes that may participate in the downstream regulatory network of UvHOX2 during chlamydospore formation.
Homeobox domain-containing proteins play a critical role in the regulatory network of fungal development and pathogenicity as downstream elements in plant pathogenic fungi, but the number of homeobox genes varies (Kim et al., 2009). Since the homolog of the transcription motif of STE12 in fungi is distinct from the typical homeodomain, we did not take account of the homolog of STE12 (KDB11415) as a homeobox gene. As a result, we identified seven genes encoding homeobox containing proteins in U. virens. Two of them contain multiple DNA-binding motifs besides homeobox motif. Only one homeobox DNA-binding motif was identified in the N-terminus of UvHOX2. In ascomycetous fungi, orthologs of UvHOX2 may play conserved roles in the development of specific structures during sporulation. In Verticillium dahliae, deletion of vhb1 (homolog of UvHox2) reduced sporulation rates in liquid medium (Sarmiento-Villamil et al., 2018). In Fusarium species, the deletion mutants failed to form conventional phialides and had obstructions in generating microconidia, but it could still produce macroconidia which was formed from hyphae through a budding-like mechanism (Zheng et al., 2012). In plant pathogen Magnaporthe oryzea, the Mohtf1 gene (homolog of UvHox2) is essential for conidiation but not for hypha growth and pathogenicity. The Mohtf1 deletion mutants generated more conidiophores, which failed to develop into sterigmata-like structures (Kim et al., 2009; Liu et al., 2010). Accordingly, deletion of the homolog of UvHox2 caused obstructions in conidiophore-genesis and completely abolished the generation structure of chlamydospores.
Conidia and chlamydospores are asexual spores produced by U. virens. In A. nidulans, regulatory factors, BrlA, AbaA, and WetA, were considered as cell developmental regulators that were critical for the development of conidiophore and phialide, as well as spore maturation (Cary et al., 2017; Wu et al., 2018). Here, we provided a clue that BrlA-AbaA-WetA cascade may also participate in the regulation of chlamydospore formation in U. virens. Deletion of UvHox2 reduced the expression of BrlA, AbaA, and WetA, but the deletion did not affect the upstream regulatory factors FluG and FlbD. This suggested that UvHOX2 and FlbD regulatory pathways could coordinate to regulate the downstream BrlA-AbaA-WetA cascade during sporulation and chlamydospore formation in U. virens. Although deletion of UvHox2 did not completely block the BrlA-AbaA-WetA signal cascade, the UvHox2 deletion mutant lost the ability to form special structures for chlamydospore generation. UvHOX2 must control other regulatory pathways, which are critical for generating special structures during chlamydospore formation. Moreover, because conidia generated by UvHox2 deletion mutant could convert into chlamydospore as wild-type strains do, UvHOX2 may not be critical for maturation of chlamydospores in U. virens.
The two-component signaling proteins responsive to osmotic stress play an important role in cell tolerance under adverse environmental conditions. Our results revealed that Sln1 and Skn7, which were differentially expressed in DH vs. WTC, may function downstream of the UvHOX2 signaling pathway. Although the histidine kinase protein Sln1 and TF Skn7 play important roles in responses to osmotic, oxidative, and cell wall stresses (Zhang et al., 2010; Tang et al., 2017), their exact functions vary among fungi. The infection process of U. virens is unique compared to most phytopathogenic fungal pathogens (do not penetrate or destroy the host cells after infection) and the morphology of this fungi vary (Tang et al., 2013; Fan et al., 2014; Zhang et al., 2014; Yu J.J. et al., 2015; Song et al., 2016). The exact roles of these responsive proteins in cell development and pathogenesis need to be uncovered in the future.
The low frequency of homologous gene replacement in U. virens had limited its genetic study at the molecular level in the past years. Recently, a successful gene-deletion system based on CRISPR-Cas9 has been developed by Liang et al. (2018). It makes it convenient and efficient to perform gene deletion in U. virens. Using the optimized CRISPR-Cas9 cassettes, we developed a gene deletion system based on ATMT transformation and CRISPR-Cas9. This gene-deletion system shortens culture-selection-period by 7–10 days and provides a valuable tool for molecular genetic study in U. virens.
Author Contributions
YL and JY conceived and designed the experiments. JY, MYu, ZQ, MYo, RZ, and XY performed the experiments. JY, TS, HC, XP, and YD analyzed the data. YL contributed to reagents, materials, and analysis tools. JY and YL wrote the manuscript.
Funding
This work was supported by the National Natural Science Foundation of China (Grant numbers: 31301624 and 31571961), the Natural Science Foundation of Jiangsu Province (Grant number: BK20151368), and China Postdoctoral Science Foundation (Grant number: 2018M632257).
Conflict of Interest Statement
The authors declare that the research was conducted in the absence of any commercial or financial relationships that could be construed as a potential conflict of interest.
Acknowledgments
We would like to thank Dr. Xu Jin-Rong for providing pCas9-tRp-gRNA vector.
Supplementary Material
The Supplementary Material for this article can be found online at: https://www.frontiersin.org/articles/10.3389/fmicb.2019.01071/full#supplementary-material
References
Ahmed, M., Laing, M., and Nsahlai, I. (2014). A new control strategy for nematodes of sheep using chlamydospores of a fungus, Clonostachys rosea f. rosea, and an ethanolic extract of a plant, Ananas comosus. Biocontrol Sci. Tech. 24, 860–871.
Anders, S., and Huber, W. (2012). Differential Expression of RNA-Seq Data at the Gene Level–the DESeq Package. Heidelberg: European Molecular Biology Laboratory (EMBL).
Antal, Z., Rascle, C., Cimerman, A., Viaud, M., Billon-Grand, G., Choquer, M., et al. (2012). The homeobox BcHOX8 gene in Botrytis cinerea regulates vegetative growth and morphology. PLoS One 7:e48134. doi: 10.1371/journal.pone.0048134
Arazoe, T., Miyoshi, K., Yamato, T., Ogawa, T., Ohsato, S., Arie, T., et al. (2015). Tailor-made CRISPR/Cas system for highly efficient targeted gene replacement in the rice blast fungus. Biotechnol. Bioeng. 112, 2543–2549.
Arnaise, S., Zickler, D., Poisier, C., and Debuchy, R. (2001). pah1: a homeobox gene involved in hyphal morphology and microconidiogenesis in the filamentous ascomycete Podospora anserina. Mol. Microbiol. 39, 54–64.
Cary, J. W., Harris-Coward, P., Scharfenstein, L., Mack, B. M., Chang, P. K., Wei, Q. J., et al. (2017). The Aspergillus flavus homeobox gene, hbx1, is required for development and aflatoxin production. Toxins 9:315. doi: 10.3390/toxins9100315
Casanova, M., Gonzalez, I., Sprissler, C., Zalila, H., Dacher, M., Basmaciyan, L., et al. (2015). Implication of different domains of the Leishmania major metacaspase in cell death and autophagy. Cell Death Dis. 6:e1933. doi: 10.1038/cddis.2015.288
Colot, H. V., Park, G., Turner, G. E., Ringelberg, C., Crew, C. M., Litvinkova, L., et al. (2006). A high-throughput gene knockout procedure for Neurospora reveals functions for multiple transcription factors. Proc. Natl. Acad. Sci. U.S.A. 103, 10352–10357. doi: 10.1073/pnas.0601456103
Coppin, E., Berteaux-Lecellier, V., Bidard, F., Brun, S., Ruprich-Robert, G., Espagne, E., et al. (2012). Systematic deletion of homeobox genes in Podospora anserina uncovers their roles in shaping the fruiting body. PLoS One 7:e37488. doi: 10.1371/journal.pone.0037488
Cord-Landwehr, S., Melcher, R. L., Kolkenbrock, S., and Moerschbacher, B. M. (2016). A chitin deacetylase from the endophytic fungus Pestalotiopsis sp. efficiently inactivates the elicitor activity of chitin oligomers in rice cells. Sci. Rep. 6:38018. doi: 10.1038/srep38018
Dong, Y., Zhao, Q., Liu, X., Zhang, X., Qi, Z., Zhang, H., et al. (2015). MoMyb1 is required for asexual development and tissue-specific infection in the rice blast fungus Magnaporthe oryzae. BMC Microbiol. 15:37. doi: 10.1186/s12866-015-0375-y
Eisman, B., Alonso-Monge, R., Roman, E., Arana, D., Nombela, C., and Pla, J. (2006). The Cek1 and Hog1 mitogen-activated protein kinases play complementary roles in cell wall biogenesis and chlamydospore formation in the fungal pathogen Candida albicans. Eukaryot. Cell 5, 347–358.
Engler, C., and Marillonnet, S. (2014). “Golden gate cloning,” in DNA Cloning and Assembly Methods. eds V. Svein and L. Rahmi (Berlin: Springer) 119–131.
Fan, J., Guo, X. Y., Huang, F., Li, Y., Liu, Y. F., Li, L., et al. (2014). Epiphytic colonization of Ustilaginoidea virens on biotic and abiotic surfaces implies the widespread presence of primary inoculum for rice false smut disease. Plant Pathol. 63, 937–945.
Fan, J., Yang, J., Wang, Y. Q., Li, G. B., Li, Y., Huang, F., et al. (2016). Current understanding on Villosiclava virens, a unique flower-infecting fungus causing rice false smut disease. Mol. Plant Pathol. 17, 1321–1330. doi: 10.1111/mpp.12362
Ghosh, A. K., Wangsanut, T., Fonzi, W. A., and Rolfes, R. J. (2015). The GRF10 homeobox gene regulates filamentous growth in the human fungal pathogen Candida albicans. Fems Yeast. Res. 15:fov093. doi: 10.1093/femsyr/fov093
Goh, Y. K., Daida, P., and Vujanovic, V. (2009). Effects of abiotic factors and biocontrol agents on chlamydospore formation in Fusarium graminearum and Fusarium sporotrichioides. Biocontrol Sci. Tech. 19, 151–167.
Guo, X., Li, Y., Fan, J., Li, L., Huang, F., and Wang, W. (2012). Progress in the study of false smut disease in rice. J. Agri. Sci. Tech. 2, 1211–1217.
Gupta, A., and Chattoo, B. B. (2008). Functional analysis of a novel ABC transporter ABC4 from Magnaporthe grisea. FEMS Microbiol. Lett. 278, 22–28.
Hu, M. L., Luo, L. X., Wang, S., Liu, Y. F., and Li, J. Q. (2014). Infection processes of Ustilaginoidea virens during artificial inoculation of rice panicles. Eur. J. Plant Pathol. 139, 67–77.
Inoue, Y., and Klionsky, D. J. (2010). “Regulation of macroautophagy in Saccharomyces cerevisiae,” in Seminars in Cell & Developmental Biology, ed. J. Davey (Amsterdam: Elsevier), 664–670.
Juchimiuk, M., Kruszewska, J., and Palamarczyk, G. (2015). Dolichol phosphate mannose synthase from the pathogenic yeast Candida albicans is a multimeric enzyme. Biochim. Biophys. Acta Gen. Subj. 1850, 2265–2275. doi: 10.1016/j.bbagen.2015.08.012
Kim, S., Park, S. Y., Kim, K. S., Rho, H. S., Chi, M. H., Choi, J., et al. (2009). Homeobox transcription factors are required for conidiation and appressorium development in the rice blast fungus Magnaporthe oryzae. PLoS Genet. 5:e1000757. doi: 10.1371/journal.pgen.1000757
Klein, E., Katan, J., and Gamliel, A. (2011). Soil suppressiveness to Fusarium disease following organic amendments and solarization. Plant Dis. 95, 1116–1123. doi: 10.1094/PDIS-01-11-0065
Kwon, M. H., Callaway, H., Zhong, J., and Yedvobnick, B. (2013). A targeted genetic modifier screen links the SWI2/SNF2 protein domino to growth and autophagy genes in Drosophila melanogaster. G3 3, 815–825. doi: 10.1534/g3.112.005496
Li, L., Qu, Q., Tian, B., and Zhang, K. (2005). Induction of chlamydospores in Trichoderma harzianum and Gliocladium roseum by antifungal compounds produced by Bacillus subtilis C2. J. Phytopathol. 153, 686–693.
Liang, Y., Han, Y., Wang, C., Jiang, C., and Xu, J.-R. (2018). Targeted deletion of the USTA and UvSLT2 genes efficiently in Ustilaginoidea virens with the CRISPR-Cas9 system. Front. Plant Sci. 9:699. doi: 10.3389/fpls.2018.00699
Liu, W. D., Xie, S. Y., Zhao, X. H., Chen, X., Zheng, W. H., Lu, G. D., et al. (2010). A homeobox gene is essential for conidiogenesis of the rice blast fungus Magnaporthe oryzae. Mol. Plant Microbe Interact. 23, 366–375. doi: 10.1094/MPMI-23-4-0366
Liu, X.-H., Zhao, Y.-H., Zhu, X.-M., Zeng, X.-Q., Huang, L.-Y., Dong, B., et al. (2017). Autophagy-related protein MoAtg14 is involved in differentiation, development and pathogenicity in the rice blast fungus Magnaporthe oryzae. Sci. Rep. 7:40018. doi: 10.1038/srep40018
Matheis, S., Yemelin, A., Scheps, D., Andresen, K., Jacob, S., Thines, E., et al. (2017). Functions of the Magnaporthe oryzae Flb3p and Flb4p transcription factors in the regulation of conidiation. Microbiol. Res. 196, 106–117. doi: 10.1016/j.micres.2016.12.010
McEwan, D. G., and Dikic, I. (2014). Cullins keep autophagy under control. Dev. Cell 31, 675–676. doi: 10.1016/j.devcel.2014.12.010
Meng, J., Sun, W., Mao, Z., Xu, D., Wang, X., Lu, S., et al. (2015). Main ustilaginoidins and their distribution in rice false smut balls. Toxins 7, 4023–4034. doi: 10.3390/toxins7104023
Ment, D., Gindin, G., Glazer, I., Perl, S., Elad, D., and Samish, M. (2010). The effect of temperature and relative humidity on the formation of Metarhizium anisopliae chlamydospores in tick eggs. Fungal Biol. 114, 49–56. doi: 10.1016/j.mycres.2009.10.005
Mukherjee, P. K., and Kenerley, C. M. (2010). Regulation of morphogenesis and biocontrol properties in Trichoderma virens by a VELVET protein. vel1. Appl. Environ Microbiol. 76, 2345–2352. doi: 10.1128/AEM.02391-09
Mullins, E. D., Chen, X., Romaine, P., Raina, R., Geiser, D. M., and Kang, S. (2001). Agrobacterium-mediated transformation of Fusarium oxysporum: an efficient tool for insertional mutagenesis and gene transfer. Phytopathology 91, 173–180. doi: 10.1094/PHYTO.2001.91.2.173
Navarathna, D. H. M. L. P., Pathirana, R. U., Lionakis, M. S., Nickerson, K. W., and Roberts, D. D. (2016). Candida albicans ISW2 regulates chlamydospore suspensor cell formation and virulence in vivo in a mouse model of disseminated candidiasis. PLoS One 11:0164449. doi: 10.1371/journal.pone.0164449
Nessa, B., Salam, M. U., Haque, A. M., Biswas, J. K., Kabir, M. S., Macleod, W. J., et al. (2015). Spatial pattern of natural spread of rice false smut (Ustilaginoidea virens) disease in fields. Am. J. Agric. Biol. Sci. 10:63.
Nobile, C. J., Bruno, V. M., Richard, M. L., Davis, D. A., and Mitchell, A. P. (2003). Genetic control of chlamydospore formation in Candida albicans. Microbiology 149, 3629–3637.
Sarmiento-Villamil, J. L., Prieto, P., Klosterman, S. J., and Garcia-Pedrajas, M. D. (2018). Characterization of two homeodomain transcription factors with critical but distinct roles in virulence in the vascular pathogen Verticillium dahliae. Mol. Plant Pathol. 19, 986–1004. doi: 10.1111/mpp.12584
Song, J. H., Wei, W., Lv, B., Lin, Y., Yin, W. X., Peng, Y. L., et al. (2016). Rice false smut fungus hijacks the rice nutrients supply by blocking and mimicking the fertilization of rice ovary. Environ. Microbiol. 18, 3840–3849. doi: 10.1111/1462-2920.13343
Sun, Z. B., Zhang, J., Sun, M. H., and Li, S. D. (2018). Identification of genes related to chlamydospore formation in Clonostachys rosea. MicrobiologyOpen 8:e00624. doi: 10.1002/mbo3.624
Tanaka, E., Kumagawa, T., Tanaka, C., and Koga, H. (2011). Simple transformation of the rice false smut fungus Villosiclava virens by electroporation of intact conidia. Mycoscience 52, 344–348.
Tang, C., Xiong, D., Fang, Y., Tian, C., and Wang, Y. (2017). The two-component response regulator VdSkn7 plays key roles in microsclerotial development, stress resistance and virulence of Verticillium dahliae. Fungal Genet. Biol. 108, 26–35. doi: 10.1016/j.fgb.2017.09.002
Tang, Y. X., Jin, J., Hu, D. W., Yong, M. L., Xu, Y., and He, L. P. (2013). Elucidation of the infection process of Ustilaginoidea virens (teleomorph: Villosiclava virens) in rice spikelets. Plant Pathol. 62, 1–8.
Wang, K., Riggs, R. D., and Crippen, D. (2005). Isolation, selection, and efficacy of Pochonia chlamydosporia for control of Rotylenchulus reniformis on cotton. Phytopathology 95, 890–893. doi: 10.1094/PHYTO-95-0890
Wieser, J., and Adams, T. H. (1995). flbD encodes a Myb-like DNA-binding protein that coordinates initiation of Aspergillus nidulans conidiophore development. Genes Dev. 9, 491–502.
Wu, M.-Y., Mead, M. E., Lee, M.-K., Loss, E. M. O., Kim, S.-C., Rokas, A., et al. (2018). Systematic dissection of the evolutionarily conserved WetA developmental regulator across a genus of filamentous fungi. mBio 9:e1130-18. doi: 10.1128/mBio.01130-18
Yong, M. L., Liu, Y. J., Chen, T. Q., Fan, L. L., Wang, Z. Y., and Hu, D. W. (2018). Cytological studies on the infection of rice root by Ustilaginoidea virens. Microsc. Res. Tech. 81, 389–396. doi: 10.1002/jemt.22990
Yu, J.-H., Hamari, Z., Han, K.-H., Seo, J.-A., Reyes-Domínguez, Y., and Scazzocchio, C. (2004). Double-joint PCR: a PCR-based molecular tool for gene manipulations in filamentous fungi. Fungal Genet. Biol. 41, 973–981.
Yu, J. J., Sun, W. X., Yu, M. N., Yin, X. L., Meng, X. K., Zhao, J., et al. (2015). Characterization of mating-type loci in rice false smut fungus Villosiclava virens. Fems Microbiol. Lett. 362:fnv014. doi: 10.1093/femsle/fnv014
Yu, M. N., Yu, J. J., Hu, J. K., Huang, L., Wang, Y. H., Yin, X. L., et al. (2015). Identification of pathogenicity-related genes in the rice pathogen Ustilaginoidea virens through random insertional mutagenesis. Fungal Genet. Biol. 76, 10–19. doi: 10.1016/j.fgb.2015.01.004
Yu, J. J., Yu, M. N., Nie, Y. F., Sun, W. X., Yin, X. L., Zhao, J., et al. (2016). Comparative transcriptome analysis of fruiting body and sporulating mycelia of Villosiclava virens reveals genes with putative functions in sexual reproduction. Curr. Genet. 62, 575–584. doi: 10.1007/s00294-015-0563-1
Zhang, H., Liu, K., Zhang, X., Song, W., Zhao, Q., Dong, Y., et al. (2010). A two-component histidine kinase, MoSLN1, is required for cell wall integrity and pathogenicity of the rice blast fungus, Magnaporthe oryzae. Curr. Genet. 56, 517–528. doi: 10.1007/s00294-010-0319-x
Zhang, Y., Zhang, K., Fang, A., Han, Y., Yang, J., Xue, M., et al. (2014). Specific adaptation of Ustilaginoidea virens in occupying host florets revealed by comparative and functional genomics. Nat. Commun. 5:3849. doi: 10.1038/ncomms4849
Zheng, D. W., Wang, Y., Han, Y., Xu, J. R., and Wang, C. F. (2016). UvHOG1 is important for hyphal growth and stress responses in the rice false smut fungus Ustilaginoidea virens. Sci. Rep. 6:24824. doi: 10.1038/srep24824
Zheng, M. T., Ding, H., Huang, L., Wang, Y. H., Yu, M. N., Zheng, R., et al. (2017). Low-affinity iron transport protein Uvt3277 is important for pathogenesis in the rice false smut fungus Ustilaginoidea virens. Curr. Genet. 63, 131–144. doi: 10.1007/s00294-016-0620-4
Keywords: Villosiclava virens, chlamydospore, conidiation, pathogenicity, homeobox transcription factor
Citation: Yu J, Yu M, Song T, Cao H, Pan X, Yong M, Qi Z, Du Y, Zhang R, Yin X and Liu Y (2019) A Homeobox Transcription Factor UvHOX2 Regulates Chlamydospore Formation, Conidiogenesis, and Pathogenicity in Ustilaginoidea virens. Front. Microbiol. 10:1071. doi: 10.3389/fmicb.2019.01071
Received: 28 December 2018; Accepted: 29 April 2019;
Published: 20 June 2019.
Edited by:
Gustavo Henrique Goldman, University of São Paulo, BrazilReviewed by:
Jing Fan, Sichuan Agricultural University, ChinaLu Zheng, Huazhong Agricultural University, China
Copyright © 2019 Yu, Yu, Song, Cao, Pan, Yong, Qi, Du, Zhang, Yin and Liu. This is an open-access article distributed under the terms of the Creative Commons Attribution License (CC BY). The use, distribution or reproduction in other forums is permitted, provided the original author(s) and the copyright owner(s) are credited and that the original publication in this journal is cited, in accordance with accepted academic practice. No use, distribution or reproduction is permitted which does not comply with these terms.
*Correspondence: Yongfeng Liu, liuyf@jaas.ac.cn