- Department of Microbiology, Savitribai Phule Pune University, Pune, India
The acronym ESKAPE includes six nosocomial pathogens that exhibit multidrug resistance and virulence: Enterococcus faecium, Staphylococcus aureus, Klebsiella pneumoniae, Acinetobacter baumannii, Pseudomonas aeruginosa, and Enterobacter spp. Persistent use of antibiotics has provoked the emergence of multidrug resistant (MDR) and extensively drug resistant (XDR) bacteria, which render even the most effective drugs ineffective. Extended spectrum β-lactamase (ESBL) and carbapenemase producing Gram negative bacteria have emerged as an important therapeutic challenge. Development of novel therapeutics to treat drug resistant infections, especially those caused by ESKAPE pathogens is the need of the hour. Alternative therapies such as use of antibiotics in combination or with adjuvants, bacteriophages, antimicrobial peptides, nanoparticles, and photodynamic light therapy are widely reported. Many reviews published till date describe these therapies with respect to the various agents used, their dosage details and mechanism of action against MDR pathogens but very few have focused specifically on ESKAPE. The objective of this review is to describe the alternative therapies reported to treat ESKAPE infections, their advantages and limitations, potential application in vivo, and status in clinical trials. The review further highlights the importance of a combinatorial approach, wherein two or more therapies are used in combination in order to overcome their individual limitations, additional studies on which are warranted, before translating them into clinical practice. These advances could possibly give an alternate solution or extend the lifetime of current antimicrobials.
Introduction
Wonder drug penicillin started the era of antibiotics in 1928 and since then it has tremendously developed modern medicine. Persistent use of antibiotics, self-medication, and exposure to infections in hospitals has provoked the emergence of multidrug resistant (MDR) bacteria responsible for 15.5% Hospital Acquired Infection (HAIs) in the world (Rice, 2008; Allegranzi et al., 2011; Ibrahim et al., 2012; Pendleton et al., 2013). The term “ESKAPE” encompasses six such pathogens with growing multidrug resistance and virulence: Enterococcus faecium, Staphylococcus aureus, Klebsiella pneumoniae, Acinetobacter baumannii, Pseudomonas aeruginosa, and Enterobacter spp. (Rice, 2008). ESKAPE pathogens are responsible for majority of nosocomial infections and are capable of “escaping” the biocidal action of antimicrobial agents (Rice, 2008; Navidinia, 2016).
A systematic review of clinical and economic impact of antibiotic resistance reveals that, ESKAPE pathogens are associated with the highest risk of mortality thereby resulting in increased health care costs (Founou et al., 2017). World Health Organization (WHO) has also recently listed ESKAPE pathogens in the list of 12 bacteria against which new antibiotics are urgently needed (Tacconelli et al., 2018). They describe three categories of pathogens namely critical, high and medium priority, according to the urgency of need for new antibiotics. Carbapenem resistant A. baumannii and P. aeruginosa along with extended spectrum β-lactamase (ESBL) or carbapenem resistant K. pneumoniae and Enterobacter spp. are listed in the critical priority list of pathogens; whereas, vancomycin resistant E. faecium (VRE) and methicillin and vancomycin resistant S. aureus (MRSA and VRSA) are in the list of high priority group. The mechanisms of multidrug resistance exhibited by ESKAPE are broadly grouped into three categories namely, drug inactivation commonly by an irreversible cleavage catalyzed by an enzyme, modification of the target site where the antibiotic may bind, reduced accumulation of drug either due to reduced permeability or by increased efflux of the drug (Santajit and Indrawattana, 2016). They are also able to form biofilms that physically prevent the immune response cells of host as well as antibiotics to inhibit the pathogen. Moreover, biofilms protect specialized dormant cells called persister cells that are tolerant to antibiotics which cause difficult-to-treat recalcitrant infections (Lewis, 2007).
The general antimicrobial therapy to effectively treat infections involves the use of antibiotics either singly or in combination. With every passing year, the overall number of antibiotics effective against ESKAPE is declining, which is predisposing us toward a future with antibiotics that are ineffective. Analysis of the antibiotic lists recommended in the Clinical & Laboratory Standards Institute (CLSI) guidelines revealed that many antibiotics suggested against ESKAPE since 2010 have been deleted with addition of relatively few antibiotics/antibiotic combinations. Furthermore, there are incidences of resistance reported against some of these newly added antibiotics (Table 1). It is, therefore, imperative to find alternative ways to treat infections especially those caused by ESKAPE pathogens.
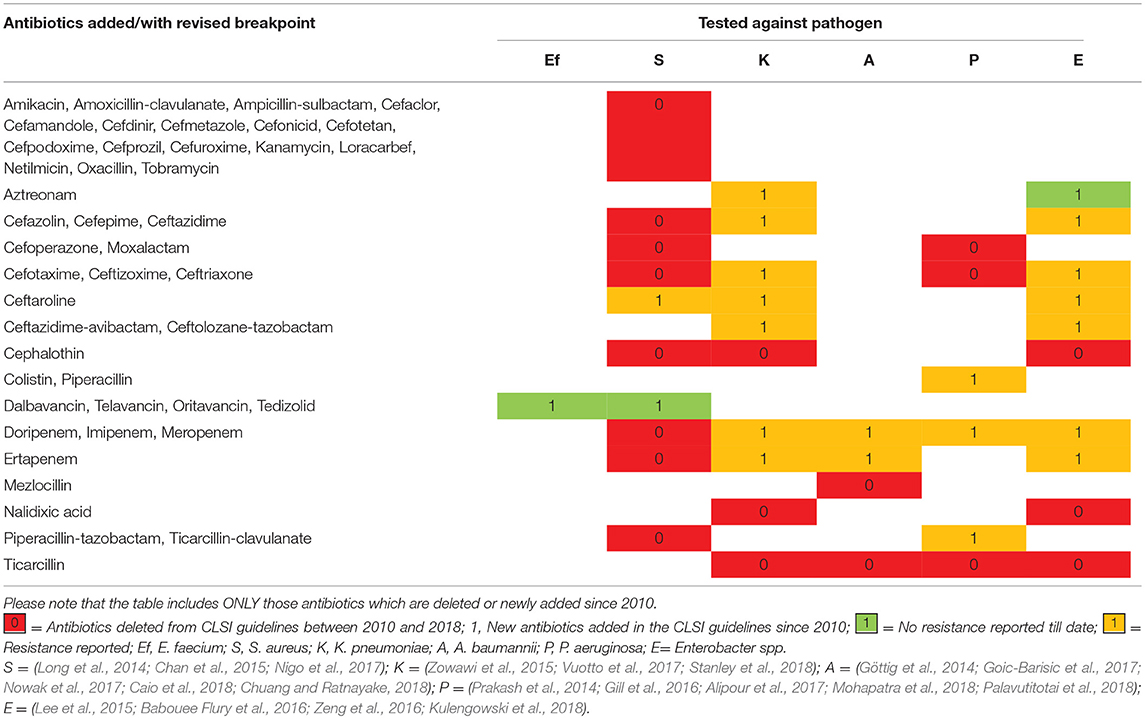
Table 1. Antibiotics added/revised and eliminated against ESKAPE from CLSI document M100 since 2010.
Alternative therapies that are currently in practice or under trial include the use of antibiotics in combination or with adjuvants, bacteriophage therapy, use of antimicrobial peptides, photodynamic therapy, antibacterial antibodies, phytochemicals and nanoparticles as antibacterial agents (Mandal et al., 2014; Kaur, 2016). There are several reviews published which describe alternative/novel therapies against MDR pathogens, however, not many have specifically focused on the ESKAPE group as a whole. These reviews describe the various therapeutic agents used with respect to their dosage, mode of action, pharmacokinetics and pharmacodynamics, stability, and toxicity. Although many alternative therapies reported have shown promising results in vitro, their efficacy when applied in vivo may not be the same due to one or several limitations. It therefore, becomes necessary to understand the action of these therapeutic agents in vivo. This review highlights therapies used to treat ESKAPE infections namely, antibiotics in combination or with adjuvants, bacteriophage therapy, antimicrobial peptides, photodynamic light therapy, and nanoparticles which have received significant attention in the last 5 years. Hereby, we have also tried to include studies especially focusing on in vivo efficacy of above mentioned therapy/therapeutic agents, their advantages and limitations. Figure 1 summarizes the major limitations of each alternative therapy which has been elaborately discussed in the following sections. To improve the efficiency and/or minimize the limitations of any therapy/therapeutic agents, combinatorial approaches are suggested. It involves use of two or more monotherapies together (co-administered, functionalized, or conjugated) which is also further described. Altogether, combinatorial approach could be a prospect for exploring novel alternate solutions against ESKAPE.
Antibiotics in Combination
Antibiotics in combination have been tested as a treatment method by a number of researchers because the possibility of a pathogen to develop resistance against a combination of two drugs is much less than that against a single drug. Similarly, the synergistic effect of combined antibiotics is more than that of the individual antibiotic. A combination of drugs also increases the spectrum of coverage (Vazquez-grande and Kumar, 2015) and has been found to be beneficial in severe infections caused by multiple pathogens (Ahmed et al., 2014). Some of these combinations tested against the ESKAPE are listed in Table 2. The problem of antibiotic resistance is so severe that it has become necessary to try combinations of the most recently synthesized antibiotics and/or last resort antibiotics to study their potential in antimicrobial therapy. The Gram positive members of the ESKAPE, E. faecium and S. aureus, have been tested against a combination of fosfomycin and daptomycin which has shown to successfully clear infection (Hall Snyder et al., 2016; Coronado-Álvarez et al., 2018). The former is a broad spectrum antibiotic that has shown promising results against Gram negative bacteria while the latter is a last resort antibiotic used to treat infections caused by E. faecium and S. aureus. Despite being a resistant pathogen there is a lack of substantial research in antibiotic combination therapy against E. faecium over the last 5 years. Most combinations tested against S. aureus in vitro include daptomycin or vancomycin with other antibiotics including ceftaroline, a newly added antibiotic to the CLSI guidelines. The effect of these and other such combinations have also been tested in various mouse models which cleared away the S. aureus infection with minimal to no toxicity. Colistin (polymyxin E) is the last resort antibiotic prescribed against Gram negative bacilli. In recent years, research has been conducted to treat infections caused by K. pneumoniae and A. baumannii using a combination of colistin or tigecycline with other antibiotics and has shown promising results in vitro and in cohort studies.
Some molecules when combined with antibiotics make an ineffective drug effective. These molecules, named “adjuvants” or “resistance breakers,” have little to no antimicrobial activity of their own (González-Bello, 2017) but inhibit the mechanism of resistance by increasing the uptake of the antibiotic through the bacterial membrane, blocking of efflux pumps, and changing the physiology of resistant cells (i.e., dispersal of biofilms to planktonic cells) (Kalan and Wright, 2011; Bernal et al., 2013). Essential oils and phenothiazines enhance the antimicrobial activity of drugs and also inhibit the transmission of resistance to other populations (Bueno, 2016).
The most popularly known adjuvants are β-lactamase inhibitors while the most recent adjuvant tested to restore meropenem activity is vaborbactam which inhibits K. pneumoniae carbapenemase activity (Jorgensen and Rybak, 2018). It has also undergone a clinical trial; registered on ClinicalTrials.gov under identifier NCT02020434; which proved the combination to be safe after testing 41 subjects (Rubino et al., 2018). However, it has a limited inhibition activity as it has been unable to do so with class B and class D β-lactamases. Other β-lactamase inhibitors include avibactam, nacubactam, and tazobactam (Monogue et al., 2018a,b). Metal chelators like EDTA, deferasirox, and deferoxamine also inhibit β-lactamases as these enzymes require metal ions for their activity (Aoki et al., 2010; Santos et al., 2012; Yoshizumi et al., 2013). These chelators have been tested in combination with antibiotics like imipenem, tobramycin, and vancomycin against S. aureus, P. aeruginosa, and E. coli in murine models with successful decrease in bacterial load. Quorum quenchers, molecules that inhibit quorum sensing thereby inhibiting biofilm formation, have also shown potential to cure infection in combination with certain antibiotics (Balamurugan et al., 2015; Chatterjee et al., 2016). 1-[(2,4-Dichlorophenethyl)amino]-3-Phenoxypropan-2-ol is so far the most promising antimicrobial agent as it has been reported to kill not only persister cells of P. aeruginosa but also non-persister cells of all the other ESKAPE members. It has also been shown to enhance killing of antibiotic resistant strains in both planktonic and biofilm forms. Its combination with a variety of antibiotics is shown to kill all of the ESKAPE making it an ideal candidate as an adjuvant (Defraine et al., 2017).
Even though there is increased activity of antibiotics when used in combination against pathogens in vitro, there are limited studies demonstrating the same in vivo and some among those have proven disadvantageous. If monotherapy selects for a narrow spectrum of resistance, a combination of two or more antibiotics selects for a broad spectrum of resistance defeating the purpose of combination therapy entirely (Vestergaard et al., 2016). Certain combinations that are meant to treat infections tend to have the opposite effect resulting in far worse damage. One antibiotic can lead to the induction of a resistance mechanism against a second antibiotic administered in combination leading to antagonistic effect (Fallah, 2018). A clinical trial conducted in Italy in which infections caused by XDR A. baumannii were treated with a combination of colistin and rifampin showed no improvement in survival rates. In fact, this combination led to increased hepatic toxicity (Durante-Mangoni et al., 2013). A similar study using a combination of colistin, tigecycline and carbapenems against A. baumannii showed futile results (López-Cortés et al., 2014). Metal chelators have shown to sequester ions not only from bacterial cells but also from host tissue cells (Yoshizumi et al., 2013).
The most recent alternative to antibiotics or their combination that shows a promising future is antibiotic hybrids which have been defined by Domalaon et al. (2018) as synthetic constructs of two or more pharmacophores belonging to an established agent known to elicit a desired antimicrobial effect. This method provides the advantage of combination therapy through the mono therapy approach, where chances of resistance are curbed, while overcoming the problem of non-complementary pharmacodynamic profiles of the individual antibiotics.
The ESKAPE tend to become resistant to either or both antibiotics used in combination with every passing year due not only to natural selection of resistant strains but also horizontal gene transfer from them to sensitive strains. This warrants testing of still new combinations. The result is a never-ending cycle from which there is no escape. It can therefore be concluded that antibiotics in combination may not always be effective and that there is a need for extensive research of alternative strategies.
Bacteriophage Therapy
Phages are century old therapeutic agents that were used for the treatment of bacterial infections. The discovery of antibiotics was an influential factor in side-lining this ambition (Mann, 2005). The focus on phage therapy has sharpened ever since antimicrobial resistance has been on a dramatic rise. Lytic phages against ESKAPE pathogens have been isolated from hospital wastewater, making them easily available therapeutic agents (Latz et al., 2016). Bacteriophages used for therapy present many advantages such as high host specificity (prevent damage to normal flora, do not infect the eukaryotic cells), low dosages for treatment, rapid proliferation inside the host bacteria, etc. that make them ideal candidates to treat bacterial infections (Domingo-Calap and Delgado-Martínez, 2018). Unlike antibiotics, the advantage of using phages is that, they develop new infectivity and regain an upper hand over bacteria as they mutate alongside their host (Pirnay et al., 2018).
Several studies carried out in vitro have proven phages to be effective as antibacterial agents against biofilm and planktonic cells of ESKAPE (Pallavali et al., 2017; Dvořáčková et al., 2018; Jamal et al., 2019). Table 2 gives information of phage therapy studied in various animal models as well as recent case studies and case reports of patients infected with ESKAPE pathogens. Phage therapy carried out in animal wound infection models have shown reduced mortality and enhanced wound healing. Additional studies carried out in vivo have also demonstrated efficacy and safety (non-toxic with reduced inflammatory responses) of phages used in treatment of bacterial infections.
Phage therapy, though promising, comes with some limitations. It can, however, be overcome by appropriate modifications (Wittebole et al., 2014; El-Shibiny and El-Sahhar, 2017; Domingo-Calap and Delgado-Martínez, 2018). High specificity of the phages can be considered as both advantageous and a limiting factor. Monophage therapy involves the need to check the efficacy of the phage by testing it in vitro against the disease-causing bacteria before applying it to a patient which can be a difficult process. The use of phage cocktails, comprising of a combination of phages acting against different bacterial species or strains, can avoid these problems (Chan et al., 2013). International experts believe that an ideal phage cocktail should be prepared using phages belonging to different families or groups such as having broad host range, high adsorption ability to the highly conserved cell wall structures in bacteria. Using such phage cocktails may reduce the emergence of phage resistant bacterial population. However, others advocate strategies wherein individual active phages are applied sequentially to the patient. In clinical practice, however, it appears to be difficult (Rohde et al., 2018).
Genomic characterization of phages is very important so as to predict their “safety” in therapeutic applications as demonstrated by several experts in this field. Phages can be vectors for horizontal gene transfer in bacteria, sometimes being involved in exchange of virulence or antibiotic resistance genes making a microbe more pathogenic or resistant to an antibiotic (Chen and Novick, 2009). Phages reported for therapeutic applications should not harbor virulence or antibiotic resistance genes as well as integrases, site-specific recombinases, and repressors of the lytic cycle that may accelerate the transfer/integration of these genes in the host bacterial genome. Algorithms that can be used for predicting lifestyle of a phage, and its virulent traits are available but their database needs to be updated with more genome sequences of phages (Mcnair et al., 2012). Two recent reviews excellently describe the work flow to select ideal phage candidates for therapeutic purposes (Casey et al., 2018; Philipson et al., 2018). Recent studies demonstrating in vivo efficacy of phages against ESKAPE infections have used fully characterized phages that show no virulence or antibiotic resistance genes, are considered safe as they do not exhibit any allergic or immune response, and are also reported to remain stable at varied pH and temperature which make them ideal candidates for therapy (Fish et al., 2016; Kishor et al., 2016; Wang et al., 2016; Zhou et al., 2018).
Similarly, it has also been reported that the bacterial strains used for phage production should ideally be free of functional prophages. These prophages may get induced and contaminate the phage preparation. However, a recent report discusses the risk benefit evaluation that needs to be done in highly experimental treatments of patients infected with MDR pathogens such as ESKAPE (Rohde et al., 2018).
Another limitation reported is the stability of phages and their proper administration in order to reach the site of action. Phage formulations are ingested orally, administered nasally or applied topically (Malik et al., 2017; Cooper et al., 2018). Studies have shown improved efficacy of phage when entrapped with liposomes (Singla et al., 2016; Chadha et al., 2017; Malik et al., 2017; Chhibber et al., 2018). Phages can be targeted at the infection site in the form of powdered formulations (Chang et al., 2018). Phage derived product like phage encoded lytic enzymes showing function similar to lysozyme can also be used as an antibacterial agent or can be combined with other antimicrobials like antibiotics to improve efficacy of treatment (Lin et al., 2017). A phage derived protein, “endolysin” is reported for its antibacterial and antibiofilm activity against ESKAPE (Viertel et al., 2014; Gong et al., 2016; Rios et al., 2016; Lin et al., 2017; Zhang et al., 2018). V12CBD a recombinant protein derived from bacteriophage lysine, PlyV12, was able to attenuate virulence of S. aureus, and enhance its phagocytosis in mice (Yang et al., 2018b).
Several commercial phage preparations which can be used against ESKAPE are available some of which include, “Stafal,” “Sextaphage,” “PhagoBioDerm,” and “Pyophage”. Stafal (Bohemia Pharmaceuticals, Slovakia) is an antistaphylococcal phage preparation, Sextaphage (Microgen, ImBio Nizhny Novgorod, Russia) is a cocktail against P. aeruginosa and E. coli while, Pyophage (Georgian Eliava Institute of Bacteriophage, Microbiology, and Virology) contains bacteriophages specifically eliminating causative agents of pyoinflammatory and enteric diseases. PhagoBioDerm, a polymeric bandage impregnated with cocktail of phages, ciprofloxacin, and other active ingredients ensured for sustained release of phages to treat ulcers or wound infections caused by S. aureus and P. aeruginosa (Markoishvili et al., 2002). Clinical potential of these preparations has been investigated further to determine their broad spectrum activity against other strains in vitro, in vivo efficacy in animal models as well as through several case studies or clinical trials described below.
Recent human case studies involving treatment of ESKAPE associated infections with different phages are described in Tables 2, 3. Readers may refer to previous reviews published on phage therapy which have described in numerous studies (Abedon et al., 2011; Chan et al., 2013; Górski et al., 2017; Lin et al., 2017; Sybesma et al., 2018). Most of the case studies report phage therapy given to patients on a compassionate care basis where antibiotic treatment fails.
Several case studies have demonstrated the efficacy of phage therapy in treating patients suffering from eye infections (Fadlallah et al., 2015), pancreatitis (Schooley et al., 2017), diabetic foot ulcer (Fish et al., 2016), and urinary tract infection (Ujmajuridze et al., 2018). Fadlallah et al. (2015), reported a case study of a 65 year old woman suffering from a secondary eye infection by VRSA, treated with a well-characterized commercially available lytic phage, SATA-8505 (ATCC PTA-9476) against MRSA. After 6 months of treatment, the patient was diagnosed as negative for cultures of VRSA. This case study suggests that bacteriophage eye-drops can be used as an alternative treatment of infectious keratitis by MDR pathogens. Fish et al. (2016), reported a case involving nine patients suffering from diabetic foot ulcer, who were treated with a preparation of anti-staphylococcal phages (Sb-1) on a compassionate care basis. The average healing time reported was ~7 weeks. However, one ulcer, with poor vascularity required 18 weeks of treatment. Similarly, Ujmajuridze et al. (2018), reported a preclinical pilot study for a double blind RCT registered at ClinicalTrials: NCT03140085 (Leitner et al., 2017). Pyophage were used in this study to treat nine patients suffering from UTI infections. The first phase of this study involved adaptation cycles of the commercial Pyophage preparation to increase its sensitivity toward the uropathogens. In the second phase, six of the nine patients responded to the adapted bacteriophage showing up to five log reduction in CFU of the infecting bacteria. Another study by Schooley et al. (2017) described the case of a 68 year old male diabetic patient having necrotizing pancreatitis which was complicated by an MDR A. baumannii. The patient responded to a phage therapy which consisted of a cocktail of nine phages against A. baumannii.
Few cases showing partial success of phage therapy in treating the infection are also reported (Jennes et al., 2017; Duplessis et al., 2018). Jennes et al. (2017) reported a case study of 61-year-old man diagnosed with septicaemia caused by a colistin-only-sensitive P. aeruginosa. Blood cultures turned negative immediately after BFC1 phage therapy but sores remained infected. No adverse side effects were observed during this therapy. In another study, a 2-year-old boy with a history of DiGeorge syndrome and complex congenital heart disease developed post-operative recalcitrant P. aeruginosa bacteraemia. A cocktail of two phages were used to target the infecting pathogen turning the blood cultures negative. However, the bacteraemia re-occurred after the discontinuation of phage therapy (Duplessis et al., 2018). It is however felt that commonly approved guidelines for application of phages is warranted in order to compare the efficacies of various phage treatments.
Several clinical trials have demonstrated the safety of phages and phage lytic enzymes which are in agreement with the studies carried out in animal models or as reported in numerous case studies. Although, many phase I and/or phase II clinical trials to demonstrate the efficacy of phages against ESKAPE infections have been registered in the past few years, the number of well-documented and completed trials are too low to draw meaningful conclusions (Sybesma et al., 2018). Moreover, the number of patients enrolled for the trials have severely limited the conclusions. A notable example of this is “PhagoBurn,” a multicentric randomized single blind and controlled clinical study on phage therapy to treat burn wound infections caused by P. aeruginosa and E. coli (ClinicalTrials.gov registry, NCT02116010). Twenty seven patients were enrolled for the trial which resulted in being too low from the pre-calculated 220 patients expected to give statistically significant results. Restrictive patient inclusion criteria, shorter duration of patient enrolment, and low incidence of burn wound infection during the period of study were described as possible reasons for the very few eligible patients (Servick, 2016). The 27 patients were randomly assigned to the phage treatment group and safety control group for further investigation. A cocktail of 12 phages (PP1131) with lytic activity against P. aeruginosa were added to an alginate template that was applied directly to the wound of the treated group. A diluted phage cocktail was used due to its high endotoxin content. The control group received a topical application of the standard care treatment (1% sulfadiazine silver). Few adverse events were reported both in phage treated and safety control group. The trial, however, was terminated prematurely due to insufficient efficacy of PP1131. A supporting study showed that the bacteria isolated from patients with the failed treatment were resistant to low phage doses used for this study. This, however, was the first Randomised Clinical Trial (RCT) performed following both good manufacturing practices as well as good clinical practices and was approved by three national health regulators (Belgium, France, and Switzerland). Further studies with increased phage concentration and higher number of participants are needed (Jault et al., 2019). In case of phage lytic enzymes, the first-in-human phase I clinical trial study for phage endolysin-based candidate drug SAL200, after a single intravenous administration among healthy volunteers showed no clinically significant detrimental effect (Youn Jun et al., 2017).
The other recent ongoing trial includes a randomized placebo controlled double blind clinical trial (ClinicalTrials.gov registry, NCT03140085) to study the efficacy and safety of commercially available Pyophages for treating UTI infections in patients planned for transurethral resection of prostate. Eighty-one patients were expected to enroll for this interventional study design planned between Nov 2015–Dec 2018 that may provide necessary insights into this potentially transforming alternative treatment option (Leitner et al., 2017). Similarly, another multicentric, randomized, two-parallel group, double blind controlled trial expected to enroll 60 patients was registered (ClinicalTrials.gov registry, NCT02664740) to compare efficacy of anti-staphylococcal phages verses standard treatment and placebo for diabetic foot ulcers infected by S. aureus. This study has not yet begun recruiting patients.
To further overcome limitations of phage therapy, phages can be combined with antibiotics which may show synergistic action by making either a phage or antibiotic or both to act more effectively (Table 3). Reduction in the formation of bacterial biofilms has also been reported when antibiotic treatment is used in combination with phages (Jo et al., 2016; Chaudhry et al., 2017). Endolysin produced by bacteriophages, proves to be more beneficial than lysing cell wall and facilitating entry of the antibiotic inside the bacteria (Rios et al., 2016). Phage PEV20 and ciprofloxacin exhibited a synergistic effect in vitro against P. aeruginosa (Lin Y. et al., 2018). Another interesting study reported that P. aeruginosa, while developing phage resistance when under attack by OMKO1 phage, changed the efflux pump mechanism which ultimately increased the sensitivity of P. aeruginosa to antibiotics. Such an approach creates a win-win situation causing the killing of the bacteria either through phage or by antibiotic action (Chan et al., 2016). Similarly, it has been reviewed that such changes in the surface receptors of any bacterium may also reduce its virulence (Rios et al., 2016). A case study reported OMKO1 phage in combination with ceftazidime could successfully treat a complicated postoperative P. aeruginosa infection in a patient who underwent an aortic arch replacement surgery (Chan et al., 2018). The success or failure of phage antibiotic combination therapy is still in a state of immaturity as the mechanisms involved in synergy have not been fully understood and the data of in vivo models and case reports is scarce. Further investigations are warranted in order to make any conclusive remarks.
Antimicrobial Peptides (AMPs) in Therapy
Antimicrobial peptides (AMPs) are short, positively charged, diverse host defense oligopeptides produced by all living forms including protozoa, bacteria, archaea, fungi, plants, and animals (Wang et al., 2010). They show a broad spectrum of activity against a wide range of pathogens. The capacity of AMPs to interact with bacterial cell membrane and thereby cause cell lysis makes them a potential alternative to combat MDR pathogens (Berglund et al., 2015). Furthermore, in contrast to conventional antibiotics, AMPs physically damage the bacterial cell through electrostatic interactions thereby making it difficult for bacteria to develop resistance against AMPs (Pfalzgraff et al., 2018).
Considering the critical status of ESKAPE pathogens, several attempts have been made to find out AMP based effective therapeutics. To date, there are numerous natural as well as bioengineered AMPs reported to show in vitro (Björn et al., 2016; Cappiello et al., 2016; Liu et al., 2017; Gandt et al., 2018; Irani et al., 2018; Téllez et al., 2018) as well as in vivo (Björn et al., 2016; Liu et al., 2017) antimicrobial, antibiofilm, anti-inflammatory, and wound healing abilities with minimum cytotoxicity. Histatin 5, a natural histidine rich cationic human salivary peptide, is an example. This peptide shows a strong in vitro anti-biofilm as well as potent bactericidal activity (≥70%) against ESKAPE (Du et al., 2017). Similarly, a de novo-engineered cationic peptide, WLBU-2, and a natural AMP LL-37 at 1/3X MIC has demonstrated 90% biofilm inhibition as compared to that shown by antibiotics such as tobramycin, ciprofloxacin, ceftazidime, and vancomycin at 1X MIC (Lin Q. et al., 2018). In 2017, Gaglione et al. examined human ApoB derived recombinant peptides namely r(P)ApoBL and r(P)ApoBS. Both peptides showed effective in vitro wound healing, anti-inflammatory, antimicrobial, and antibiofilm properties against MDR strains of S. aureus and P. aeruginosa.
Similar to their remarkable in vitro properties, AMPs also exhibit promising in vivo activity against ESKAPE. For example, peptide HLR1r, a structural derivative of human milk protein, lactoferrin, at very low concentration (5 mg/kg) was found to show anti-infectivity against MRSA infected wound excision model in rat along with in vitro anti-inflammatory and non-cytotoxic effects suggesting use of HLR1r in topical formulation to treat skin infections (Björn et al., 2016). PT-13 a peptide derived from seeds and leaves of Populus trichocarpa crude extract also demonstrated effective in vivo antibacterial activity in S. aureus infected G. mellonella model (Al Akeel et al., 2018). In another instance, a synthetic analog of Feleucin-K3 has shown to clear P. aeruginosa induced bacteremia in mice model with good stability and very low cytotoxicity (Xie et al., 2018). Also, a hydrogelformulation containing K-11, a hybrid peptide of melittin, cecropin A1 and magainin-2 has shown to possess wound healing ability against A. baumannii infected murine excision model proposing its possible use as a topical anti-infective therapeutic agent (Rishi et al., 2018).
Over the past decades, intense efforts taken by the scientific community and pharmaceutical industries together has made it possible to introduce certain peptides such as vancomycin, telavancin, telaprevir, teicoplanin, enfuvirtide, daptomycin, dalbavancin, bacitracin etc. for clinical use (Gomes et al., 2018). A clinical trial conducted on rabbits and humans using the peptide melamine proved it to be a stable and safe antibacterial coating for eye lenses (Dutta et al., 2014). Similarly, Pexiganan (analog of magainin), LL-37 (analog of human cathelicidin peptide), hLF1-11, and PXL-01 (derivatives of human milk protein), Novexatin (derivative of human defense peptide), Iseganan (derivative of porcine leukocytes), PAC-113 (derivative of human saliva histatin-3 peptide) etc. are few examples of AMPs which also are under clinical trials (Mahlapuu et al., 2016).
Unfortunately, such a low number of AMPs seeking clinical approval is quite discouraging. Despite their successful in vitro and/or in vivo broad-spectrum activities, numerous AMPs have not yet crossed the hurdle of clinical trial. Amongst the few challenges that hamper the in vivo efficacy of AMPs are their cytotoxicity to mammalian cells, liability to degradation by tissue proteases, loss of activity at low salt concentrations or in presence of plasma proteins and higher production cost (Mahlapuu et al., 2016; Rios et al., 2016). The issue of peptide degradation can be solved by structural modification of AMPs such as addition of non-natural amino acids or their D-isomers, peptide cyclisation, acetylation, and amidation of N-terminus. Introduction of peptide mimetics or the use of suitable delivery system like liposome encapsulation can be done to improve their stability and reduce toxicity (Seo et al., 2012; Reinhardt and Neundorf, 2016). Additionally, efficiency of AMPs can be enhanced by combining AMPs with antibiotics (Gaglione et al., 2017; Zheng et al., 2017; Pletzer et al., 2018) or nanoparticles (Chaudhari et al., 2016; Kuo et al., 2016). Otvos et al. (2018), reported a synergestic effect of A3-APO, a proline-rich AMP, and colistin when studied in a K. pneumoniae infected bacteremia mice model. The basis for in vitro decrease in MIC of A3-APO when combined with colistin can be explained by considering the fact that colistin kills bacteria by interfering with bacterial membrane assembly and, therefore, slight reductions in bacterial membrane integrity potentiate A3-APO antibacterial action. Surprisingly, the same combination also showed a 100% survival in mice. This observation can be a direct consequence of A3-APO ability to induce immune augmentation or the deactivation of bacterial toxins. Enhanced in vitro bactericidal activity against S. aureus was also found in the case where LL-37, a human cathelicidin peptide, combined with gold nanoparticles as compared to vancomycin alone (Wang et al., 2018). In this case, gold nanoparticles increased the local density of positive charges and peptide mass and thereby enhanced the bactericidal properties of LL-37.
To summarize, owing to their in vitro and in vivo broad-spectrum antibacterial activities AMPs offer a hopeful alternative to conventional therapeutics. However, to overcome challenges in developing a safe, stable and efficient commercial product, a thorough understanding of their structure and interaction with bacterial as well as host cells is still needed. It will also be helpful to find better AMP formulation strategies to obtain maximum therapeutic actions. Overall, considering the extensive research being carried out on different AMPs against various infectious agents, the future of peptide based commercial drug formulations looks hopeful.
Photodynamic Light Therapy
Antimicrobial light therapy, either alone or combined with a photosensitizer (PS), results in a photooxidative stress response that leads to microbial death. Excitation of PS with light of an appropriate wavelength leads to formation of an excited triplet state. An excited PS can transfer electrons or energy to biomolecules or molecular oxygen, resulting in the formation of reactive oxygen species (ROS) or singlet oxygen radicals, which are toxic to cellular targets such as nucleic acids, proteins and lipids (Mai et al., 2017; Yang M.-Y. et al., 2018). Some of the most frequently used PSs include phenothiazinium derivatives (methylene blue, toluidine blue), xanthine derivatives (rose bengal), porphyrin, chlorin, or fullerene derivatives amongst many others (Abrahamse and Hamblin, 2016; Cieplik et al., 2018). Antimicrobial photodynamic therapy is widely used for treating dental, skin, and soft tissue infections. For a more detailed description of the current state and future prospects of light therapy with respect to the various photosensitisers, light sources, and methods used, mechanism of antimicrobial action or antibiofilm potential, the reader may be referred to the excellent reviews published recently (Cieplik et al., 2018; Hu et al., 2018; Tomb et al., 2018; Wozniak and Grinholc, 2018). However, none of these reviews have especially focused on in vivo studies of aPDT against ESKAPE pathogens.
There has been extensive research on designing the PSs so as to improve their pharmaceutical potential. An ideal PS used for antimicrobial therapy should have greater permeability to cross the microbial cell wall/cell membrane, selective toxicity toward the microbial cell with minimal or no damage to the host tissue and an absorption coefficient appropriate for effective penetration at the site of action. The PS chosen should not have a long half-life which causes prolonged photosensitization in the host cells even after the infection is cured. It should also not be effluxed out by the microbial efflux systems (Cieplik et al., 2018; Hu et al., 2018; Tomb et al., 2018; Wozniak and Grinholc, 2018). Efficacy of aPDT also depends on the light fluence, PS concentration and treatment time (Tomb et al., 2017; Sueoka et al., 2018; Ullah et al., 2018).
PSs chosen preferably have a large absorption coefficient in the visible spectrum, especially in the long wavelength (red near infrared) region, to allow effective penetration of light in the infected tissue (Table 2). Many researchers have attempted to improve the availability of PS by potentiating or functionalizing it with other molecules including galactose, amino acids, efflux pump inhibitors, potassium iodide, EDTA etc. A variety of PSs functionalized with addends are used to target ESKAPE pathogens. A boron-dipyrrolemethene (BODIPY)-based polygalactose, named pGEMA-I (7.3 kDa) with increased water solubility was used to demonstrate antibacterial and antibiofilm activity against P. aeruginosa, without much affecting the viability of normal cells. It was demonstrated that the selective recognition of the pathogen was due its carbohydrate binding lectin protein (LecA) which interacted with the galactose moiety of the PS (Zhao et al., 2018). C60-fullerene (LC16) bearing deca-quaternary chain and deca-tertiary-amino groups facilitates electron-transfer reactions via the photoexcited fullerene for antimicrobial effect studied in A. baumannii and S. aureus (Huang et al., 2014; Zhang et al., 2015). Another drawback of aPDT is that the ROS generation may cease after the light irradiation is turned off thus allowing un-killed bacteria to re-grow. Potentiating aPDT with potassium iodide allows the formation of iodine/tri-iodide that may remain active in the wound for a longer duration sufficient enough to prevent bacterial re-growth (Zhang et al., 2015; Wen et al., 2017).
In vitro studies have shown that blue light (aBL) has a broad spectrum antibacterial and antibiofilm activity against all six ESKAPE members (Halstead et al., 2016). In vivo data also corroborated this finding and further confirmed that using a low penetrating blue light of 415 ± 10 nm should be a preferred choice of treatment in case of topical wound infections as it causes minimal damage to the uninfected tissue cells below (Amin et al., 2016; Wang et al., 2017; Katayama et al., 2018). Some studies additionally report that an exogenous PS may not be required (Amin et al., 2016; Wang et al., 2017). Their finding was supported by experimental data showing that the endogenous porphyrins present in the bacterial cell membrane play a role in triggering the photoxidative response (Amin et al., 2016). aBL using 5-aminolevulinic acid with disodium EDTA (ALA-EDTA/2Na) had antibacterial and antibiofilm potential thus showing significant wound healing of P. aeruginosa infected cutaneous ulcers in mice model (Katayama et al., 2018). However, the role of EDTA in increasing the antibacterial action of aBL needs further investigation. The physiological mechanism behind wound healing was studied in a S. aureus infected burn model in mice revealing that the enhanced levels of factors promoting angiogenesis and epithelial regeneration (bFGF, TGFβ1, and VEGF) led to the inhibition of inflammatory factors (TNFα and IL6) in the aPDT treated group as compared to the control (Mai et al., 2017).
Sueoka et al. (2018) studied the time dependant effect of aPDT-TON 504 on P. aeruginosa and showed that a repeated exposure to light emitting diode (LED) enhanced the inhibitory effect on bacterial growth. This enhanced effect was possibly because the bacteria that survived the initial aPDT were injured by singlet oxygen generated due to excitation of remaining photosensitizer. On the contrary there are also a few reports investigating the development of resistance/tolerance to aBL. It was observed that initial exposure to low doses of aBL increased the tolerance of methicillin susceptible S. aureus (MSSA) to subsequent doses of high intensity aBL-405 nm. It is likely that increased tolerance to high intensity light may be due to up-regulation of bacterial stress responses which needs detailed investigations. However, a second set of experiment showed that repeated sub-lethal exposures of 405 nm light indicate no evidence of tolerance in S. aureus (Tomb et al., 2017).
A considerable amount of literature has been published on combined efficacy of aPDT and antibiotics demonstrated in vitro (El-Azizi and Khardori, 2016; Ronqui et al., 2016). Synergistic effects of aPDT-antibiotic combination resulted in inactivation of several virulence factors in P. aeruginosa isolates (Fila et al., 2017). aPDT when used in combination with vancomycin prolonged the survival of Galleria mellonella infected with a vancomycin resistant strain of E. faecium as compared to either of the two therapies used alone (Chibebe Junior et al., 2013). Wozniak and Grinholc (2018) in their comprehensive review analysis have, however, pointed out that most of the aPDT carried out in combination with antibiotics lack a standard methodology followed to evaluate the synergistic effect.
One of the strategies for improved PS delivery involves the use of nanoparticles which are co-administered to allow the PS entry across the membrane or for a synergistic ROS response resulting in an antimicrobial action. A combination of tetrasulfonated hydroxyl aluminum phthalocyanine (AlPcS4) and bimetallic gold/silver nanoparticles (Au/Ag-NPs) synthesized using a cell-free filtrate of Aureobasidium pullulans showed significantly higher killing as compared to the agents used individually (Maliszewska et al., 2018). Au/Ag-NPs possibly disrupted the cell membrane allowing enhanced uptake of the PS, AlPcS4. In vitro and in vivo studies demonstrated that AgNPs used in combination with blue light showed synergistic antimicrobial and antibiofilm activities against P. aeruginosa infection. Interestingly, this combination was also effective for the treatment of a chronic wound caused by mixed infection in a horse (Nour El Din et al., 2016). More recently, an in vitro study carried out using A. baumannii isolates, demonstrated that the antibacterial action of ZnO-NPs with blue light irradiation was due to their ability to damage the cytomembrane but not DNA (Yang M.-Y. et al., 2018).
Over expressing multidrug efflux pumps, commonly found in resistant pathogens, are reported to affect the intracellular concentration of PS used in aPDT, thus limiting its action (Tegos and Hamblin, 2006). Tegos et al. (2008) carried out in vitro experiments by co-incubating various combinations of PS with efflux pump inhibitors to select the best combination showing antibacterial activity. Photodynamic killing mediated by toluidine blue (TBO), when used in combination with PaβN, or INF271 (as EPIs) was most effective against P. aeruginosa and S. aureus isolates, respectively. Similarly, an in vitro study demonstrated that verapamil, an efflux pump inhibitor, when combined with aPDT, required a lower light dose for effective antibacterial, and antibiofilm action against S. aureus (de Aguiar Coletti et al., 2017). In further development of such an approach, it was recently demonstrated that INF55-(Ac)en–MB, synthetic antimicrobial hybrids designed by covalently linking a PS (methylene blue) to efflux pump inhibitors (INF55 and INF271) were more effective in treating wound infections caused by S. aureus or A. baumannii studied in mice models (Rineh et al., 2017, 2018).
It is also worth noting that synergistic effect of aPDT when used in combination with antimicrobial peptide was also demonstrated recently (de Freitas et al., 2018; Nakonieczna et al., 2018). Aurein 1.2 augmented the aPDT activity mediated by methylene blue or chlorin-e6 against strains of S. aureus, A. baumannii and more importantly against vancomycin resistant E. faecium, whereas the AMP aPDT combination with curcumin (as PS) had no effect thus revealing a PS-dependent mechanism (de Freitas et al., 2018). Later, Nakonieczna et al. (2018) carried out a similar study showing synergistic effect of rose bengal-aPDT with two synthetic AMPs, CAMEL, and pexiganan against 35 isolates of P. aeruginosa. Notably, it was also shown that this combination was non-toxic to human keratinocytes. Conjugates such as ZnPc(Lys)5 (a zinc phthalocyanine derivative coupled with pentalysine) also showed a synergistic antibacterial action which was sufficient to heal S. aureus wound infection in mice models (Ullah et al., 2018).
Overall, photodynamic therapy appears to be a promising option for treatment of infections caused due to ESKAPE pathogens, particularly effective in topical applications. aPDT co administered or conjugated with antibiotics, antimicrobial peptides, nanoparticles, or efflux pump inhibitors show a synergistic effect. However, it is difficult to compare efficacy between different combinatorial approaches due to lack of uniform methodologies. More studies on investigation of toxicity and biocompatibility of various combinations should be investigated using in vivo models for translating them into clinical practice.
Silver Nanoparticles in Therapy
Nanomedicine is one of the emerging branches for treating drug resistant pathogens. Metal nanoparticles have wide biomedical applications as antimicrobial agents due to their unique physical and chemical properties (Beyth et al., 2015; Hemeg, 2017). Amongst metal nanoparticles, silver nanoparticles (AgNPs) synthesized using physical, chemical or biological methods have shown promising antibacterial activity due to their multi-targeted approach which reduces the probability of resistance (Möhler et al., 2018; Siddiqi et al., 2018). AgNPs act by releasing Ag+ ions which results in disruption of electron transport or signal transduction pathway or leads to generation of ROS, ultimately damaging important biomolecules such as cell wall, cell membrane, cellular DNA, and/or proteins (Dakal et al., 2016; Qayyum et al., 2017).
AgNPs act by inhibiting or disrupting planktonic cells as well as biofilms of MDR pathogens. Even though earlier reports have suggested the cytotoxic effects of AgNPs (Mohanty et al., 2012), recently in vitro and in vivo studies have demonstrated the safe usage of AgNPs (Möhler et al., 2018). AgNPs synthesized using aqueous leaf extract of Corchorus capsularis exhibited antibacterial activity against S. aureus and P. aeruginosa and were found to be non-toxic to mouse fibroblast cells (Kasithevar et al., 2017). Electrochemically synthesized AgNPs showing antimicrobial activity against planktonic and biofilm forming P. aeruginosa strain were non-toxic to G. mellonella larvae model (Pompilio et al., 2018). Recently, sunlight mediated AgNPs synthesized using Capsicum annuum was tested in S. aureus infected zebra fish model which proved to be effective in inhibiting biofilm formation. Histological studies revealed that they are non-toxic and hence can be tested for efficacy in higher mammalian in vivo models (Lotha et al., 2018). A single-blind clinical trial (Clinical Trial Registration: NCT01243320 and NCT01405794) carried out in 60 healthy human volunteers, showed that commercial AgNPs when administered orally at dose of 10 and 32 ppm and monitored over 14 days were found to be non-toxic. The study revealed no significant changes in metabolic, hematologic and pro-inflammatory responses as well as no morphological changes in vital organs (Munger et al., 2014).
One of the widely explored applications of AgNPs is their use in the form of composite dressings or hydrogels for treatment of topical wound infections. AgNPs incorporated in chitosan composite dressings offer sustained release of Ag+ ions at low dosage which are non-toxic to fibroblast cells. Studies in mice models suggested that AgNPs/chitosan composite dressings and low molecular weight chitosan-coated silver nanoparticles were effective in reducing bacterial load, were non-toxic and biocompatible, had low absorption in body and promoted better wound healing against S. aureus and P. aeruginosa (Liang et al., 2016; Peng et al., 2017). Similarly, studies using three other polymer dressings made of chitosan, nylon, and collagen incorporated with AgNPs exhibited in vitro antibacterial activities against ESKAPE pathogens (Radulescu et al., 2016; Rath et al., 2016; Ding et al., 2017). These dressings did not exhibit inflammatory responses, showed re-epithelization of cells and better wound contraction leading to accelerated wound healing in mice models. Sodium carboxymethyl cellulose hydrogel loaded with polyethylene glycol coated AgNPs showed antibacterial activity, re-epithelization, and wound healing in MRSA infected mice model (Mekkawy et al., 2017). Similarly, topical application of nanosilvernanohydrogels in combination with Aloe vera accelerated wound contraction and enhanced wound healing due to the moist environment provided by Aloe vera (Anjum et al., 2016). In yet another formulation, AgNPs coated on to MCM-41 type mesoporous silica nanoparticles prevented their aggregation and allowed sustained release of Ag+ ions displaying a long-term antibacterial activity against S. aureus. These antibacterial nanofibrous membranes could reduce inflammatory response and accelerate wound healing in wistar rats (Dong et al., 2016). A randomized clinical trial was carried out to test the antibacterial effect of two silver dressings and their healing time in burn patients. It was demonstrated that the hydrofiber silver dressing (AquacelR) was preferred over the nanocrystalline silver dressing (ActicoatTM) due to reduction of bioburden, quick wound healing, ease of using, comfort to the patients, and low cost (Verbelen et al., 2014). On the other hand ActicoatTM showed complete wound healing within 12 weeks in 64% of the patients with leg ulcers as compared to those who were treated with Iodosorb dressings (cadexomer iodine) (Miller et al., 2010).
Polymer-based nanomaterials and metal NPs are used in antimicrobial coatings on surface of medical devices, such as catheters and implants for prevention of infections. AgNPs when embedded in electrospun hyaluronic acid/polycaprolactonenanofibrous membranes coated on flexor tendon animal models prevented bacterial infection during the early postsurgical period (Chen et al., 2015; Shalumon et al., 2018). Likewise, implants coated with nanocomposite layer of polysaccharide 1-deoxylactit-1-yl chitosan and AgNPs in a mini-pig animal model showed good biocompatibility with the bone tissue (Marsich et al., 2013). All these studies demonstrated that the entrapped AgNPs allowed controlled release of Ag+ ions displaying prolonged antibacterial and antibiofilm action as well as reduced inflammatory responses. A randomized clinical trial demonstrated the efficacy of triple-lumen central venous catheters impregnated with AgNPs (AgTive) which showed reduced bacterial colonization as compared to conventional catheters in intensive care unit patients (Antonelli et al., 2012).
Repeated exposure of AgNPs at sub inhibitory concentrations may lead to resistance in bacterial pathogens. To overcome this limitation, a combination of AgNPs with antibiotics has been suggested in order to increase the therapeutic efficacy of either, resulting in reduction of dose and hence toxicity. In vitro studies demonstrating AgNPs co-incubated with different antibiotics showed synergistic antibacterial activities against ESKAPE (Ghosh et al., 2012; Panáček et al., 2015; Golińska et al., 2016; Habash et al., 2017; Singh et al., 2018; Wypij et al., 2018). Synergistic antibacterial activity of AgNPs in combination with polymyxin B was demonstrated in A. baumannii infected mouse model with 60% survival rate as compared to the controls treated with antibiotic or AgNPs alone (Wan et al., 2016).
Despite the use of AgNPs as a potential therapeutic agent, literature survey indicates a paucity of data obtained from in vivo studies carried out to test the toxicity, efficacy, pharmacokinetic, and immuno-modulatory response of the AgNPs. Further investigations through well-defined studies and clinical trials will lead to applications of AgNPs in wound dressings or medical devices.
Concluding Remarks
There is an urgent need to restock our armamentarium of antimicrobials in order to stay ahead of the ever rising drug resistant ESKAPE pathogens. There is an insufficiency of effective antibiotic combinations in addition to the dry pipeline of new drugs. Huge efforts have been taken to use antibiotics in combination with adjuvants targeting important metabolic mechanisms/pathways contributing to drug resistance (permeablisers, lactamase inhibitors, efflux pump inhibitors, quorum sensing inhibitors, toxin inhibitors etc.) The modest success received to date with such antibiotic-adjuvant combinations has paved way to explore other alternative strategies to combat drug resistance. There is a significant rise in the interest shown by the scientific community to use novel therapeutic agents such as phages, antimicrobial peptides, metal nanoparticles, and photodynamic light which, although, have some limitations as discussed above. Some of the commonly described limitations of these therapies include stability and toxicity of the therapeutic agent, its targeted delivery at the site of infection, or immune response developed by the host against the therapeutic agent. Ongoing research has therefore led to further develop or modify these novel therapeutic agents or therapies so as to surmount the limitations as well as to overcome the barriers of bacterial resistance.
This review summarizes studies that demonstrate potential alternative therapies using in vivo models some of which have extended further to the level of clinical trials. The interest in phage therapy to treat bacterial infections is fast growing leading to development of commercial preparations such as “Stafal,” “Sextaphage,” “PhagoBioDerm,” and “Pyophage” against MDR pathogens. Similarly, use of silver nanoparticles as antibiofilm coatings in surgical implants, antimicrobial agents in topical applications or as formulations in wound dressings has shown promising activities in animal models. Clinical trials using commercially available nanosilver coated dressings (ActicoatTM, AquacelR) or catheters (AgTive) is another noteworthy advancement. AMPs have received great attention due to their broad spectrum activity; however, they have shown limited pharmaceutical potential due to their toxicity, stability, and production costs. Photodynamic light therapy which is widely used for cancer therapy has also been demonstrated to be an effective strategy for clearing wound infections. However, additional studies demonstrating the efficacy and safety of these therapeutic agents against ESKAPE infections are desired. Similarly, randomized clinical trials would enable these therapeutic agents to cross the regulatory hurdles and find application in clinical practice.
It was observed that, majority of these studies have used animal models infected by S. aureus, A. baumannii, and P. aeruginosa to test the efficacy of the therapeutic agent. The probable reason for this could be that these pathogens mostly cause topical infections (wound, burn and abscess) and because majority of the limitations (targeted delivery, stability, immune response, toxicity etc.) described for each therapy can be minimized though not avoided in such models. It would be important to study the effect of these therapeutic agents against systemic infections caused by ESKAPE members. It was also observed that, the methods used for estimating efficacy of any therapeutic agent were not uniform. Table 2 reveals that, the in vivo efficacy of various therapies is given either in terms of log or percent reduction of microbial load or as percent survival of the infected host (animal model). The methods followed to estimate the reduced pathogen loads as well as dosages used for treatment also vary. It is therefore not appropriate to compare these studies to identify the best therapeutic agent/therapy against any ESKAPE member.
In addition to the growing concern in searching and evaluating the clinical potential of the above discussed alternative therapies, research on combinatorial approach, based on the synergistic action of two or more therapies is also gaining attention. Most commonly studied combinations involve use of a therapeutic agent/ therapy (phage, aPDT, AMP, or AgNP) in combination with antibiotic/s or in some cases with an efflux pump inhibitor or quorum sensing inhibitor. Another interesting option used was the combination of the therapeutic agents in a conjugate or hybrid (antibiotic-antibiotic, antibiotic-EPI, PS-AMP, PS-EPI etc.) for an increased efficacy against the pathogen. Most of these studies demonstrated that the combinatorial approach helped overcome the limitation caused by individual therapeutic agent. For example, an antibiotic combined with an efflux pump inhibitor or a photosensitizer conjugated with an AMP improved their entry and retention into the target pathogen for enhanced antimicrobial action. Similarly, combinations of antibiotics with nanoparticles or AMPS reduced the toxicity caused by these agents which were required at high dosages when used alone. The synergistic action allows for an increased bioavailability of the drug or therapeutic agent, broad antimicrobial spectrum, reduced toxicity, and decreased chances of development of resistance. However, Table 3 shows that most of the studies demonstrating potential of combinatorial approach are currently based on in vitro evaluation only. Data supporting the potential of a combinatorial therapy with respect to the mechanism of synergy, its in vivo efficacy, toxicity, and immune response is scarce and needs further investigation.
Finally, cost effectiveness of the above described therapeutic agents/therapy over the conventional antimicrobial agents also play a crucial role in their clinical application. Production cost for any therapeutic agent will strongly depend on the various regulatory hurdles they pass to come into clinical practice. An agent or therapy which is too expensive may not be a preferred choice for treatment in under developed countries or weaker economies thus leading to over-use of conventional antimicrobials thus contributing to the growing drug resistance.
To conclude, a uniform research methodology used to test the efficacy of these therapeutic agents in accordance with well-defined standards will make it possible to reliably compare the data presented by various research groups. Well-performed clinical trials of these therapeutic agents used as monotherapy or as a combinatorial approach will allow us to derive the real potential of these therapeutic combinations for being translated into clinical practice.
Author Contributions
KP conceived the concept and edited. MM, EK, SK, and MT wrote and edited the manuscript and agreed for submission.
Funding
MT is a Project Assistant under UPE-Phase II (UGC-262-A-2); EK is a recipient of CSIR-SRF; SK is a Project Assistant DST-PURSE (GOI-A-670).
Conflict of Interest Statement
The authors declare that the research was conducted in the absence of any commercial or financial relationships that could be construed as a potential conflict of interest.
The reviewer RP declared a shared affiliation, with no collaboration, with the authors to the handling editor at the time of the review.
References
Abedon, S. T., Kuhl, S. J., Blasdel, B. G., and Kutter, E. M. (2011). Phage treatment of human infections. Bacteriophage 1, 66–85. doi: 10.4161/bact.1.2.15845
Abrahamse, H., and Hamblin, M. R. (2016). New photosensitizers for photodynamic therapy. Biochem. J. 473, 347–364. doi: 10.1042/BJ20150942
Ahmed, A., Azim, A., Gurjar, M., and Baronia, A. K. (2014). Current concepts in combination antibiotic therapy for critically ill patients. Indian J. Crit. Care Med. 18, 310–314. doi: 10.4103/0972-5229.132495
Akram, F. E., El-Tayeb, T., Abou-Aisha, K., and El-Azizi, M. (2016). A combination of silver nanoparticles and visible blue light enhances the antibacterial efficacy of ineffective antibiotics against methicillin-resistant Staphylococcus aureus (MRSA). Ann. Clin. Microbiol. Antimicrob. 15, 48. doi: 10.1186/s12941-016-0164-y
Al Akeel, R., Mateen, A., Syed, R., Alqahtani, M. S., and Alqahtani, A. S. (2018). Alanine rich peptide from Populus trichocarpa inhibit growth of Staphylococcus aureus via targetting its extracellular domain of Sensor Histidine Kinase YycGex protein. Microb. Pathog. 121, 115–122. doi: 10.1016/j.micpath.2018.05.010
Alipour, N., Karagoz, A., Taner, A., Gaeini, N., Alipour, N., Zeytin, H., et al. (2017). Outbreak of hospital infection from biofilm-embedded pan drug-resistant Pseudomonas aeroginosa, due to a contaminated bronchoscope. J. Prev. Med. 2:1. doi: 10.21767/2572-5483.100014
Allegranzi, B., Nejad, S. B., Combescure, C., Graafmans, W., Attar, H., Donaldson, L., et al. (2011). Burden of endemic health-care-associated infection in developing countries: systematic review and meta-analysis. Lancet 377, 228–241. doi: 10.1016/S0140-6736(10)61458-4
Amin, R. M., Bhayana, B., Hamblin, M. R., and Dai, T. (2016). Antimicrobial blue light inactivation of Pseudomonas aeruginosa by photo-excitation of endogenous porphyrins: in vitro and in vivo studies. Lasers Surg. Med. 48, 562–568. doi: 10.1002/lsm.22474
Anjum, S., Gupta, A., Sharma, D., Gautam, D., Bhan, S., Sharma, A., et al. (2016). Development of novel wound care systems based on nanosilver nanohydrogels of polymethacrylic acid with Aloe vera and curcumin. Mater. Sci. Eng. C. Mater. Biol. Appl. 64, 157–166. doi: 10.1016/j.msec.2016.03.069
Antonelli, M., De Pascale, G., Ranieri, V. M., Pelaia, P., Tufano, R., Piazza, O., et al. (2012). Comparison of triple-lumen central venous catheters impregnated with silver nanoparticles (AgTive®) vs. conventional catheters in intensive care unit patients. J. Hosp. Infect. 82, 101–107. doi: 10.1016/j.jhin.2012.07.010
Aoki, N., Ishii, Y., Tateda, K., Saga, T., Kimura, S., Kikuchi, Y., et al. (2010). Efficacy of calcium-EDTA as an inhibitor for metallo-β-lactamase in a mouse model of Pseudomonas aeruginosa pneumonia. Antimicrob. Agents Chemother. 54, 4582–4588. doi: 10.1128/AAC.00511-10
Babouee Flury, B., Ellington, M. J., Hopkins, K. L., Turton, J. F., Doumith, M., Loy, R., et al. (2016). Association of novel nonsynonymous single nucleotide polymorphisms in ampD with cephalosporin resistance and phylogenetic variations in ampC, ampR, ompF, and ompC in Enterobacter cloacae isolates that are highly resistant to carbapenems. Antimicrob. Agents Chemother. 60, 2383–2390. doi: 10.1128/AAC.02835-15
Balamurugan, P., Hema, M., Kaur, G., Sridharan, V., Prabu, P. C., Sumana, M. N., et al. (2015). Development of a biofilm inhibitor molecule against multidrug resistant Staphylococcus aureus associated with gestational urinary tract infections. Front. Microbiol. 6:832. doi: 10.3389/fmicb.2015.00832
Berglund, N. A., Piggot, T. J., Jefferies, D., Sessions, R. B., Bond, P. J., and Khalid, S. (2015). Interaction of the antimicrobial peptide polymyxin B1 with both membranes of E. coli: a molecular dynamics study. PLoS Comput. Biol. 11:e1004180. doi: 10.1371/journal.pcbi.1004180
Bernal, P., Molina-Santiago, C., Daddaoua, A., and Llamas, M. A. (2013). Highlight Antibiotic adjuvants: identification and clinical use. Microb. Biotechnol. 6, 445–449. doi: 10.1111/1751-7915.12044
Beyth, N., Houri-Haddad, Y., Domb, A., Khan, W., and Hazan, R. (2015). Alternative antimicrobial approach: nano-antimicrobial materials. Evid. Based Complement. Altern. Med. 2015:246012. doi: 10.1155/2015/246012
Biswas, B., Adhya, S., Washart, P., Paul, B., Trostel, A. N., Powell, B., et al. (2002). Bacteriophage therapy rescues mice bacteremic from a clinical isolate of vancomycin-resistant Enterococcus faecium. Infect. Immun. 70, 204–210. doi: 10.1128/IAI.70.1.204-210.2002
Björn, C., Mahlapuu, M., Mattsby-Baltzer, I., and Håkansson, J. (2016). Anti-infective efficacy of the lactoferrin-derived antimicrobial peptide HLR1r. Peptides 81, 21–28. doi: 10.1016/j.peptides.2016.04.005
Bueno, J. (2016). Antimicrobial adjuvants drug discovery, the challenge of avoid the resistance and recover the susceptibility of multidrug-resistant strains. J. Microb. Biochem. Technol. 8, 169–176. doi: 10.4172/1948-5948.1000281
Cai, Y., Yang, D., Wang, J., and Wang, R. (2018). Activity of colistin alone or in combination with rifampicin or meropenem in a carbapenem-resistant bioluminescent Pseudomonas aeruginosa intraperitoneal murine infection model. J. Antimicrob. Chemother. 73, 456–461. doi: 10.1093/jac/dkx399
Caio, C., Maugeri, G., Zingali, T., Gona, F., Stefani, S., and Mezzatesta, M. L. (2018). Extensively drug-resistant ArmA-producing Acinetobacter baumannii in an Italian intensive care unit. N. Microbiol. 41, 159–161.
Cappiello, F., Di Grazia, A., Segev-Zarko, L.-A., Scali, S., Ferrera, L., Galietta, L., et al. (2016). Esculentin-1a-derived peptides promote clearance of Pseudomonas aeruginosa internalized in bronchial cells of cystic fibrosis patients and lung cell migration: biochemical properties and a plausible mode of action. Antimicrob. Agents Chemother. 60, 7252–7262. doi: 10.1128/AAC.00904-16
Casey, E., van Sinderen, D., and Mahony, J. (2018). In vitro characteristics of phages to guide ‘real life' phage therapy suitability. Viruses 10:E163. doi: 10.3390/v10040163
Chadha, P., Katare, O. P., and Chhibber, S. (2017). Liposome loaded phage cocktail: enhanced therapeutic potential in resolving Klebsiella pneumoniae mediated burn wound infections. Burns 43, 1532–1543. doi: 10.1016/j.burns.2017.03.029
Chan, B. K., Abedon, S. T., and Loc-Carrillo, C. (2013). Phage cocktails and the future of phage therapy. Future Microbiol. 8, 769–783. doi: 10.2217/fmb.13.47
Chan, B. K., Sistrom, M., Wertz, J. E., Kortright, K. E., Narayan, D., and Turner, P. E. (2016). Phage selection restores antibiotic sensitivity in MDR Pseudomonas aeruginosa. Sci. Rep. 6:26717. doi: 10.1038/srep26717
Chan, B. K., Turner, P. E., Kim, S., Mojibian, H. R., Elefteriades, J. A., and Narayan, D. (2018). Phage treatment of an aortic graft infected with Pseudomonas aeruginosa. Evol. Med. Public Heal. 2018, 60–66. doi: 10.1093/emph/eoy005
Chan, L. C., Basuino, L., Diep, B., Hamilton, S., Chatterjee, S. S., and Chambers, H. F. (2015). Ceftobiprole- and ceftaroline-resistant methicillin-resistant Staphylococcus aureus. Antimicrob. Agents Chemother. 59, 2960–2963. doi: 10.1128/AAC.05004-14
Chang, R. Y. K., Chen, K., Wang, J., Wallin, M., Britton, W., Morales, S., et al. (2018). Proof-of-principle study in a murine lung infection model of antipseudomonal activity of phage PEV20 in a dry-powder formulation. Antimicrob. Agents Chemother. 62:e01714-17. doi: 10.1128/AAC.01714-17
Chatterjee, M., Anju, C. P., Biswas, L., Anil Kumar, V., Gopi Mohan, C., and Biswas, R. (2016). Antibiotic resistance in Pseudomonas aeruginosa and alternative therapeutic options. Int. J. Med. Microbiol. 306, 48–58. doi: 10.1016/j.ijmm.2015.11.004
Chaudhari, A. A., Ashmore, D., Nath, S., deb Kate, K., Dennis, V., Singh, S. R., et al. (2016). A novel covalent approach to bio-conjugate silver coated single walled carbon nanotubes with antimicrobial peptide. J. Nanobiotechnol. 14:58. doi: 10.1186/s12951-016-0211-z
Chaudhry, W. N., Concepcion-Acevedo, J., Park, T., Andleeb, S., Bull, J. J., and Levin, B. R. (2017). Synergy and order effects of antibiotics and phages in killing Pseudomonas aeruginosa biofilms. PLoS ONE 12:e0168615. doi: 10.1371/journal.pone.0168615
Chen, C., Mangoni, M. L., and Di, Y. P. (2017). In vivo therapeutic efficacy of frog skin-derived peptides against Pseudomonas aeruginosa-induced pulmonary infection. Sci. Rep. 7:8548. doi: 10.1038/s41598-017-08361-8
Chen, C. H., Chen, S. H., Shalumon, K. T., and Chen, J. P. (2015). Dual functional core–sheath electrospun hyaluronic acid/polycaprolactone nanofibrous membranes embedded with silver nanoparticles for prevention of peritendinous adhesion. Acta Biomater. 26, 225–235. doi: 10.1016/j.actbio.2015.07.041
Chen, H. L., Su, P. Y., Kuo, S. C., Lauderdale, T. L. Y., and Shih, C. (2018). Adding a C-terminal cysteine (CTC) can enhance the bactericidal activity of three different antimicrobial peptides. Front. Microbiol. 9:1440. doi: 10.3389/fmicb.2018.01440
Chen, J., and Novick, R. P. (2009). Phage-mediated intergeneric transfer of toxin genes. Science 323, 139–141. doi: 10.1126/science.1164783
Chhibber, S., Kaur, J., and Kaur, S. (2018). Liposome entrapment of bacteriophages improves wound healing in a diabetic mouse MRSA infection. Front. Microbiol. 9:561. doi: 10.3389/fmicb.2018.00561
Chibebe Junior, J., Fuchs, B. B., Sabino, C. P., Junqueira, J. C., Jorge, A. O. C., Ribeiro, M. S., et al. (2013). Photodynamic and antibiotic therapy impair the pathogenesis of Enterococcus faecium in a whole animal insect model. PLoS ONE 8:e55926. doi: 10.1371/journal.pone.0055926
Chuang, L., and Ratnayake, L. (2018). Overcoming challenges of treating extensively drug-resistant Acinetobacter baumannii bacteraemic urinary tract infection. Int. J. Antimicrob. Agents. 52, 521–522. doi: 10.1016/j.ijantimicag.2018.07.016
Cieplik, F., Deng, D., Crielaard, W., Buchalla, W., Hellwig, E., Al-Ahmad, A., et al. (2018). Antimicrobial photodynamic therapy – what we know and what we don't. Crit. Rev. Microbiol. 44, 571–589. doi: 10.1080/1040841X.2018.1467876
Ciociola, T., Giovati, L., Giovannelli, A., Conti, S., Castagnola, M., and Vitali, A. (2018). The activity of a mammalian proline-rich peptide against Gram-negative bacteria, including drug-resistant strains, relies on a nonmembranolytic mode of action. Infect. Drug Resist. 11, 969–979. doi: 10.2147/IDR.S165179
Cooper, C. J., Koonjan, S., and Nilsson, A. S. (2018). Enhancing whole phage therapy and their derived antimicrobial enzymes through complex formulation. Pharmaceuticals 11:E34. doi: 10.3390/ph11020034
Corbett, D., Wise, A., Langley, T., Skinner, K., Trimby, E., Birchall, S., et al. (2017). Potentiation of antibiotic activity by a novel cationic peptide: potency and spectrum of activity of SPR741. Antimicrob. Agents Chemother. 61, e00200–e00217. doi: 10.1128/AAC.00200-17
Coronado-Álvarez, N. M., Parra, D., and Parra-Ruiz, J. (2018). Clinical efficacy of fosfomycin combinations against a variety of gram-positive cocci. Enferm. Infecc. Microbiol. Clin. 37, 4–10. doi: 10.1016/j.eimc.2018.05.009
Costley, D., Nesbitt, H., Ternan, N., Dooley, J., Huang, Y. Y., Hamblin, M. R., et al. (2017). Sonodynamic inactivation of gram-positive and gram-negative bacteria using a rose bengal-antimicrobial peptide conjugate. Int. J. Antimicrob. Agents 49, 31–36. doi: 10.1016/j.ijantimicag.2016.09.034
Dakal, T. C., Kumar, A., Majumdar, R. S., and Yadav, V. (2016). Mechanistic basis of antimicrobial actions of silver nanoparticles. Front. Microbiol. 7:1831. doi: 10.3389/fmicb.2016.01831
Danis-Wlodarczyk, K., Vandenheuvel, D., Jang, H. B., Briers, Y., Olszak, T., Arabski, M., et al. (2016). A proposed integrated approach for the preclinical evaluation of phage therapy in Pseudomonas infections. Sci. Rep. 6:28115. doi: 10.1038/srep28115
de Aguiar Coletti, T. M. S. F., de Freitas, L. M., Almeida, A. M. F., and Fontana, C. R. (2017). Optimization of antimicrobial photodynamic therapy in biofilms by inhibiting efflux pump. Photomed. Laser Surg. 35, 378–385. doi: 10.1089/pho.2016.4246
de Breij, A., Riool, M., Cordfunke, R. A., Malanovic, N., de Boer, L., Koning, R. I., et al. (2018). The antimicrobial peptide SAAP-148 combats drug-resistant bacteria and biofilms. Sci. Transl. Med. 10:eaan4044. doi: 10.1126/scitranslmed.aan4044
de Freitas, L. M., Lorenzón, E. N., Santos-Filho, N. A., Zago, L. H. P., Uliana, M. P., de Oliveira, K. T., et al. (2018). Antimicrobial photodynamic therapy enhanced by the peptide aurein 1.2. Sci. Rep. 8:4212. doi: 10.1038/s41598-018-22687-x
Defraine, V., Verstraete, L., Van Bambeke, F., Anantharajah, A., Townsend, E. M., Ramage, G., et al. (2017). Antibacterial activity of 1-[(2,4-Dichlorophenethyl)amino]-3-Phenoxypropan-2-ol against antibiotic-resistant strains of diverse bacterial pathogens, biofilms and in pre-clinical infection models. Front. Microbiol. 8:2585. doi: 10.3389/fmicb.2017.02585
Ding, L., Shan, X., Zhao, X., Zha, H., Chen, X., Wang, J., et al. (2017). Spongy bilayer dressing composed of chitosan-Ag nanoparticles and chitosan-Bletilla striata polysaccharide for wound healing applications. Carbohydr. Polym. 157, 1538–1547. doi: 10.1016/j.carbpol.2016.11.040
Domalaon, R., Idowu, T., Zhanel, G. G., and Schweizer, F. (2018). Antibiotic hybrids: the next generation of agents and adjuvants against gram-negative pathogens? Clin. Microbiol. Rev. 31, e00077–e00017. doi: 10.1128/CMR.00077-17
Domingo-Calap, P., and Delgado-Martínez, J. (2018). Bacteriophages: protagonists of a post-antibiotic era. Antibiotics 7:66. doi: 10.3390/antibiotics7030066
Dong, R. H., Jia, Y. X., Qin, C. C., Zhan, L., Yan, X., Cui, L., et al. (2016). In situ deposition of a personalized nanofibrous dressing via a handy electrospinning device for skin wound care. Nanoscale 8, 3482–3488. doi: 10.1039/C5NR08367B
Du, H., Puri, S., McCall, A., Norris, H. L., Russo, T., and Edgerton, M. (2017). Human salivary protein histatin 5 has potent bactericidal activity against ESKAPE pathogens. Front. Cell. Infect. Microbiol. 7:41. doi: 10.3389/fcimb.2017.00041
Duplessis, C., Biswas, B., Hanisch, B., Perkins, M., Henry, M., Quinones, J., et al. (2018). Refractory Pseudomonas bacteremia in a 2-year-old sterilized by bacteriophage therapy. J. Pediatric Infect. Dis. Soc. 7, 253–256. doi: 10.1093/jpids/pix056
Durante-Mangoni, E., Signoriello, G., Andini, R., Mattei, A., De Cristoforo, M., Murino, P., et al. (2013). Colistin and rifampicin compared with colistin alone for the treatment of serious infections due to extensively drug-resistant Acinetobacter baumannii: a multicenter, randomized clinical trial. Clin. Infect. Dis. 57, 349–358. doi: 10.1093/cid/cit253
Dutta, D., Ozkan, J., and Willcox, M. D. P. (2014). Biocompatibility of antimicrobial melimine lenses. Optom. Vis. Sci. 91, 570–581. doi: 10.1097/OPX.0000000000000232
Dvořáčková, M., Růžička, F., Benešík, M., Pantůček, R., and Dvoráková-Heroldová, M. (2018). Antimicrobial effect of commercial phage preparation Stafal® on biofilm and planktonic forms of methicillin-resistant Staphylococcus aureus. Folia Microbiol. 64, 121–126. doi: 10.1007/s12223-018-0622-3
El-Azizi, M., and Khardori, N. (2016). Efficacy of ultraviolet C light at sublethal dose in combination with antistaphylococcal antibiotics to disinfect catheter biofilms of methicillin-susceptible and methicillin-resistant Staphylococcus aureus and Staphylococcus epidermidis in vitro. Infect. Drug Resist. Volume 9, 181–189. doi: 10.2147/IDR.S109343
El-Shibiny, A., and El-Sahhar, S. (2017). Bacteriophages: the possible solution to treat infections caused by pathogenic bacteria. Can. J. Microbiol. 63, 865–879. doi: 10.1139/cjm-2017-0030
Fadlallah, A., Chelala, E., and Legeais, J. M. (2015). Corneal infection therapy with topical bacteriophage administration. Open Ophthalmol. J. 9, 167–168. doi: 10.2174/1874364101509010167
Fallah, K. N. (2018). The Effects of Combination Antibiotic Therapy on Methicillin-Resistant Staphylococcus aureus. Doctoral dissertation.
Fila, G., Kawiak, A., and Grinholc, M. S. (2017). Blue light treatment of Pseudomonas aeruginosa: strong bactericidal activity, synergism with antibiotics and inactivation of virulence factors. Virulence 8, 938–958. doi: 10.1080/21505594.2016.1250995
Fish, R., Kutter, E., Wheat, G., Blasdel, B., Kutateladze, M., and Kuhl, S. (2016). Bacteriophage treatment of intransigent diabetic toe ulcers: a case series. J. Wound Care 25, S27–S33. doi: 10.12968/jowc.2016.25.Sup7.S27
Founou, R. C., Founou, L. L., and Essack, S. Y. (2017). Clinical and economic impact of antibiotic resistance in developing countries: a systematic review and meta-analysis. PLoS ONE 12:e0189621. doi: 10.1371/journal.pone.0189621
Gaglione, R., Dell'Olmo, E., Bosso, A., Chino, M., Pane, K., Ascione, F., et al. (2017). Novel human bioactive peptides identified in Apolipoprotein B: evaluation of their therapeutic potential. Biochem. Pharmacol. 130, 34–50. doi: 10.1016/j.bcp.2017.01.009
Gandt, A., Griffith, E. C., Lister, I. M., Billings, L. L., Han, A., Tangallapally, R., et al. (2018). In vivo and in vitro effects of a ClpP-activating antibiotic against vancomycin-resistant Enterococci. Antimicrob. Agents Chemother. 62, e00424–e00418. doi: 10.1128/AAC.00424-18
Ghosh, S., Patil, S., Ahire, M., Kitture, R., Kale, S., Pardesi, K., et al. (2012). Synthesis of silver nanoparticles using Dioscorea bulbifera tuber extract and evaluation of its synergistic potential in combination with antimicrobial agents. Int. J. Nanomed. 7, 483–96. doi: 10.2147/IJN.S24793
Gill, J. S., Arora, S., Khanna, S. P., and Kumar, K. H. (2016). Prevalence of multidrug-resistant, extensively drug-resistant, and pandrug-resistant Pseudomonas aeruginosa from a tertiary level intensive care unit. J. Glob. Infect. Dis. 8, 155–159. doi: 10.4103/0974-777X.192962
Goic-Barisic, I., Seruga Music, M., Kovacic, A., Tonkic, M., and Hrenovic, J. (2017). Pan drug-resistant environmental isolate of Acinetobacter baumannii from croatia. Microb. Drug Resist. 23, 494–496. doi: 10.1089/mdr.2016.0229
Golińska, P., Wypij, M., Rathod, D., Tikar, S., Dahm, H., and Rai, M. (2016). Synthesis of silver nanoparticles from two acidophilic strains of Pilimelia columellifera subsp. pallida and their antibacterial activities. J. Basic Microbiol. 56, 541–556. doi: 10.1002/jobm.201500516
Gomes, B., Augusto, M. T., Felício, M. R., Hollmann, A., Franco, O. L., Gonçalves, S., et al. (2018). Designing improved active peptides for therapeutic approaches against infectious diseases. Biotechnol. Adv. 36, 415–429. doi: 10.1016/j.biotechadv.2018.01.004
Gong, P., Cheng, M., Li, X., Jiang, H., Yu, C., Kahaer, N., et al. (2016). Characterization of Enterococcus faecium bacteriophage IME-EFm5 and its endolysin LysEFm5. Virology 492, 11–20. doi: 10.1016/j.virol.2016.02.006
González-Bello, C. (2017). Antibiotic adjuvants – a strategy to unlock bacterial resistance to antibiotics. Bioorg. Med. Chem. Lett. 27, 4221–4228. doi: 10.1016/j.bmcl.2017.08.027
Górski, A., Jończyk-Matysiak, E., Łusiak-Szelachowska, M., Miȩdzybrodzki, R., Weber-Dąbrowska, B., and Borysowski, J. (2017). The potential of phage therapy in sepsis. Front. Immunol. 8:1783. doi: 10.3389/fimmu.2017.01783
Göttig, S., Gruber, T. M., Higgins, P. G., Wachsmuth, M., Seifert, H., and Kempf, V. A. J. (2014). Detection of pan drug-resistant Acinetobacter baumannii in Germany. J. Antimicrob. Chemother. 69, 2578–2579. doi: 10.1093/jac/dku170
Greimel, F., Scheuerer, C., Gessner, A., Simon, M., Kalteis, T., Grifka, J., et al. (2017). Efficacy of antibiotic treatment of implant-associated Staphylococcus aureus infections with moxifloxacin, flucloxacillin, rifampin, and combination therapy: an animal study. Drug Des. Devel. Ther. 11, 1729–1736. doi: 10.2147/DDDT.S138888
Habash, M. B., Goodyear, M. C., Park, A. J., Surette, M. D., Vis, E. C., Harris, R. J., et al. (2017). Potentiation of tobramycin by silver nanoparticles against Pseudomonas aeruginosa Biofilms. Antimicrob. Agents Chemother. 61:e00415-17. doi: 10.1128/AAC.00415-17
Hall Snyder, A. D., Werth, B. J., Nonejuie, P., McRoberts, J. P., Pogliano, J., Sakoulas, G., et al. (2016). Fosfomycin enhances the activity of daptomycin against vancomycin-resistant Enterococci in an in vitro pharmacokinetic-pharmacodynamic model. Antimicrob. Agents Chemother. 60, 5716–5723. doi: 10.1128/AAC.00687-16
Halstead, F. D., Thwaite, J. E., Burt, R., Laws, T. R., Raguse, M., Moeller, R., et al. (2016). Antibacterial activity of blue light against nosocomial wound pathogens growing planktonically and as mature biofilms. Appl. Environ. Microbiol. 82, 4006–4016. doi: 10.1128/AEM.00756-16
Hemeg, H. (2017). Nanomaterials for alternative antibacterial therapy. Int. J. Nanomed. Vol. 12, 8211–8225. doi: 10.2147/IJN.S132163
Hu, X., Huang, Y. Y., Wang, Y., Wang, X., and Hamblin, M. R. (2018). Antimicrobial photodynamic therapy to control clinically relevant biofilm infections. Front. Microbiol. 9, 1–24. doi: 10.3389/fmicb.2018.01299
Huang, L., Wang, M., Dai, T., Sperandio, F. F., Huang, Y. Y., Xuan, Y., et al. (2014). Antimicrobial photodynamic therapy with decacationic monoadducts and bisadducts of [70]fullerene: in vitro and in vivo studies. Nanomedicine 9, 253–266. doi: 10.2217/nnm.13.22
Ibrahim, M. E., Bilal, N. E., and Hamid, M. E. (2012). Increased multi-drug resistant Escherichia coli from hospitals in Khartoum state, Sudan. Afr. Health Sci. 12, 368–375. doi: 10.4314/ahs.v12i3.19
Irani, N., Basardeh, E., Samiee, F., Fateh, A., Shooraj, F., Rahimi, A., et al. (2018). The inhibitory effect of the combination of two new peptides on biofilm formation by Acinetobacter baumannii. Microb. Pathog. 121, 310–317. doi: 10.1016/j.micpath.2018.05.051
Jamal, M., Andleeb, S., Jalil, F., Imran, M., Nawaz, M. A., Hussain, T., et al. (2019). Isolation, characterization and efficacy of phage MJ2 against biofilm forming multi-drug resistant Enterobacter cloacae. Folia Microbiol. 64, 101–111. doi: 10.1007/s12223-018-0636-x
Jault, P., Leclerc, T., Jennes, S., Pirnay, J. P., Que, Y. A., Resch, G., et al. (2019). Efficacy and tolerability of a cocktail of bacteriophages to treat burn wounds infected by Pseudomonas aeruginosa (PhagoBurn): a randomised, controlled, double-blind phase 1/2 trial. Lancet Infect. Dis. 19, 35–45. doi: 10.1016/S1473-3099(18)30482-1
Jennes, S., Merabishvili, M., Soentjens, P., Pang, K. W., Rose, T., Keersebilck, E., et al. (2017). Use of bacteriophages in the treatment of colistin-only-sensitive Pseudomonas aeruginosa septicaemia in a patient with acute kidney injury-a case report. Crit. Care 21:129. doi: 10.1186/s13054-017-1709-y
Jo, A., Ding, T., and Ahn, J. (2016). Synergistic antimicrobial activity of bacteriophages and antibiotics against Staphylococcus aureus. Food Sci. Biotechnol. 25, 935–940. doi: 10.1007/s10068-016-0153-0
Jorgensen, S. C. J., and Rybak, M. J. (2018). Meropenem and vaborbactam: stepping up the battle against carbapenem-resistant Enterobacteriaceae. Pharmacotherapy 38, 444–461. doi: 10.1002/phar.2092
Kalan, L., and Wright, G. D. (2011). Antibiotic adjuvants: multicomponent anti-infective strategies. Expert Rev. Mol. Med. 13:e5. doi: 10.1017/S1462399410001766
Kasithevar, M., Periakaruppan, P., Muthupandian, S., and Mohan, M. (2017). Antibacterial efficacy of silver nanoparticles against multi-drug resistant clinical isolates from post-surgical wound infections. Microb. Pathog. 107, 327–334. doi: 10.1016/j.micpath.2017.04.013
Katayama, B., Ozawa, T., Morimoto, K., Awazu, K., Ito, N., Honda, N., et al. (2018). Enhanced sterilization and healing of cutaneous pseudomonas infection using 5-aminolevulinic acid as a photosensitizer with 410-nm LED light. J. Dermatol. Sci. 90, 323–331. doi: 10.1016/j.jdermsci.2018.03.001
Kaur, I. (2016). Novel strategies to combat antimicrobial resistance. J. Infect. Dis. Ther. 4:292. doi: 10.4172/2332-0877.100029
Kishor, C., Mishra, R. R., Saraf, S. K., Kumar, M., Srivastav, A. K., and Nath, G. (2016). Phage therapy of staphylococcal chronic osteomyelitis in experimental animal model. Indian J. Med. Res. 143, 87–94. doi: 10.4103/0971-5916.178615
Kulengowski, B., Rutter, W. C., Campion, J. J., Lee, G. C., Feola, D. J., and Burgess, D. S. (2018). Effect of increasing meropenem MIC on the killing activity of meropenem in combination with amikacin or polymyxin B against MBL- and KPC-producing Enterobacter cloacae. Diagn. Microbiol. Infect. Dis. 92, 262–266. doi: 10.1016/j.diagmicrobio.2018.06.013
Kuo, Y. L., Wang, S. G., Wu, C. Y., Lee, K. C., Jao, C. J., Chou, S. H., et al. (2016). Functional gold nanoparticle-based antibacterial agents for nosocomial and antibiotic-resistant bacteria. Nanomedicine 11, 2497–2510. doi: 10.2217/nnm-2016-0232
Latz, S., Wahida, A., Arif, A., Häfner, H., Hoß, M., Ritter, K., et al. (2016). Preliminary survey of local bacteriophages with lytic activity against multi-drug resistant bacteria. J. Basic Microbiol. 56, 1117–1123. doi: 10.1002/jobm.201600108
LaVergne, S., Hamilton, T., Biswas, B., Kumaraswamy, M., Schooley, R. T., and Wooten, D. (2018). Phage therapy for a multidrug-resistant Acinetobacter baumannii craniectomy site infection. Open Forum Infect. Dis. 5:ofy064. doi: 10.1093/ofid/ofy064
Lee, N. Y., Lee, C. C., Li, C. W., Li, M. C., Chen, P. L., Chang, C. M., et al. (2015). Cefepime therapy for monomicrobial Enterobacter cloacae bacteremia: unfavorable outcomes in patients infected by cefepime-susceptible dose-dependent isolates. Antimicrob. Agents Chemother. 59, 7558–7563. doi: 10.1128/AAC.01477-15
Leitner, L., Sybesma, W., Chanishvili, N., Goderdzishvili, M., Chkhotua, A., Ujmajuridze, A., et al. (2017). Bacteriophages for treating urinary tract infections in patients undergoing transurethral resection of the prostate: a randomized, placebo-controlled, double-blind clinical trial. BMC Urol. 17:90. doi: 10.1186/s12894-017-0283-6
Lewis, K. (2007). Persister cells, dormancy and infectious disease. 5, 48–56. doi: 10.1038/nrmicro1557
Liang, D., Lu, Z., Yang, H., Gao, J., and Chen, R. (2016). Novel asymmetric wettable AgNPs/Chitosan wound dressing: in vitro and in vivo evaluation. ACS Appl. Mater. Interfaces 8, 3958–3968. doi: 10.1021/acsami.5b11160
Lin, D., Koskella, B., and Lin, H. C. (2017). Phage therapy: An alternative to antibiotics in the age of multi-drug resistance. World J. Gastrointest. Pharmacol. Ther. 8, 162–173. doi: 10.4292/wjgpt.v8.i3.162
Lin, Q., Deslouches, B., Montelaro, R. C., and Di, Y. P. (2018). Prevention of ESKAPE pathogen biofilm formation by antimicrobial peptides WLBU2 and LL37. Int. J. Antimicrob. Agents. 52, 667–672. doi: 10.1016/j.ijantimicag.2018.04.019
Lin, Y., Chang, R. Y. K., Britton, W. J., Morales, S., Kutter, E., and Chan, H.-K. (2018). Synergy of nebulized phage PEV20 and ciprofloxacin combination against Pseudomonas aeruginosa. Int. J. Pharm. 551, 158–165. doi: 10.1016/j.ijpharm.2018.09.024
Liu, S., Long, Q., Xu, Y., Wang, J., Xu, Z., Wang, L., et al. (2017). Assessment of antimicrobial and wound healing effects of Brevinin-2Ta against the bacterium Klebsiella pneumoniae in dermally-wounded rats. Oncotarget 8, 111369–111385. doi: 10.18632/oncotarget.22797
Long, S. W., Olsen, R. J., Mehta, S. C., Palzkill, T., Cernoch, P. L., Perez, K. K., et al. (2014). PBP2a mutations causing high-level Ceftaroline resistance in clinical methicillin-resistant Staphylococcus aureus isolates. Antimicrob. Agents Chemother. 58, 6668–6674. doi: 10.1128/AAC.03622-14
López-Cortés, L. E., Cisneros, J. M., Fernández-Cuenca, F., Bou, G., Tomás, M., Garnacho-Montero, J., et al. (2014). Monotherapy versus combination therapy for sepsis due to multidrug-resistant Acinetobacter baumannii: analysis of a multicentre prospective cohort. J. Antimicrob. Chemother. 69, 3119–3126. doi: 10.1093/jac/dku233
Lotha, R., Shamprasad, B. R., Sundaramoorthy, N. S., Ganapathy, R., Nagarajan, S., and Sivasubramanian, A. (2018). Zero valent silver nanoparticles capped with capsaicinoids containing Capsicum annuum extract, exert potent anti-biofilm effect on food borne pathogen Staphylococcus aureus and curtail planktonic growth on a zebrafish infection model. Microb. Pathog. 124, 291–300. doi: 10.1016/j.micpath.2018.08.053
Mahlapuu, M., Håkansson, J., Ringstad, L., and Björn, C. (2016). Antimicrobial peptides: an emerging category of therapeutic agents. Front. Cell. Infect. Microbiol. 6:194. doi: 10.3389/fcimb.2016.00194
Mai, B., Gao, Y., Li, M., Wang, X., Zhang, K., Liu, Q., et al. (2017). Photodynamic antimicrobial chemotherapy for Staphylococcus aureus and multidrug-resistant bacterial burn infection in vitro and in vivo. Int. J. Nanomed. 12, 5915–5931. doi: 10.2147/IJN.S138185
Malik, D. J., Sokolov, I. J., Vinner, G. K., Mancuso, F., Cinquerrui, S., Vladisavljevic, G. T., et al. (2017). Formulation, stabilisation and encapsulation of bacteriophage for phage therapy. Adv. Colloid Interface Sci. 249, 100–133. doi: 10.1016/j.cis.2017.05.014
Maliszewska, I., Kałas, W., Wysokiéska, E., Tylus, W., Pietrzyk, N., Popko, K., et al. (2018). Enhancement of photo-bactericidal effect of tetrasulfonated hydroxyaluminum phthalocyanine on Pseudomonas aeruginosa. Lasers Med. Sci. 33, 79–88. doi: 10.1007/s10103-017-2337-0
Mandal, S. M., Roy, A., Ghosh, A. K., Hazra, T. K., Basak, A., and Franco, O. L. (2014). Challenges and future prospects of antibiotic therapy: from peptides to phages utilization. Front. Pharmacol. 5:105. doi: 10.3389/fphar.2014.00105
Manohar, P., Nachimuthu, R., and Lopes, B. S. (2018). The therapeutic potential of bacteriophages targeting gram-negative bacteria using Galleria mellonella infection model. BMC Microbiol. 18:97. doi: 10.1186/s12866-018-1234-4
Markoishvili, K., Tsitlanadze, G., Katsarava, R., Morris, J. G., and Sulakvelidze, A. (2002). A novel sustained-release matrix based on biodegradable poly(ester amide)s and impregnated with bacteriophages and an antibiotic shows promise in management of infected venous stasis ulcers and other poorly healing wounds. Int. J. Dermatol. 41, 453–458. doi: 10.1046/j.1365-4362.2002.01451.x
Marsich, E., Travan, A., Donati, I., Turco, G., Kulkova, J., Moritz, N., et al. (2013). Biological responses of silver-coated thermosets: an in vitro and in vivo study. Acta Biomater. 9, 5088–5099. doi: 10.1016/j.actbio.2012.10.002
Mcnair, K., Bailey, B. A., and Edwards, R. A. (2012). PHACTS, a computational approach to classifying the lifestyle of phages. Bioinformatics 28, 614–618. doi: 10.1093/bioinformatics/bts014
Mekkawy, A. I., El-Mokhtar, M. A., Nafady, N. A., Yousef, N., Hamad, M. A., El-Shanawany, S. M., et al. (2017). In vitro and in vivo evaluation of biologically synthesized silver nanoparticles for topical applications: effect of surface coating and loading into hydrogels. Int. J. Nanomedicine 12, 759–777. doi: 10.2147/IJN.S124294
Miller, C. N., Newall, N., Kapp, S. E., Lewin, G., Karimi, L., Carville, K., et al. (2010). A randomized-controlled trial comparing cadexomer iodine and nanocrystalline silver on the healing of leg ulcers. Wound Repair Regen. 18, 359–367. doi: 10.1111/j.1524-475X.2010.00603.x
Mohanty, S., Mishra, S., Jena, P., Jacob, B., Sarkar, B., and Sonawane, A. (2012). An investigation on the antibacterial, cytotoxic, and antibiofilm efficacy of starch-stabilized silver nanoparticles. Nanomedicine 8, 916–924. doi: 10.1016/j.nano.2011.11.007
Mohapatra, D. P., Debata, N. K., and Singh, S. K. (2018). Extensively drug-resistant and pan-drug resistant Gram-negative bacteria in a tertiary-care hospital in Eastern India: A 4 year retrospective study. J. Glob. Antimicrob. Resist. 15, 246–249. doi: 10.1016/j.jgar.2018.08.010
Möhler, J. S., Sim, W., Blaskovich, M. A. T., Cooper, M. A., and Ziora, Z. M. (2018). Silver bullets: a new lustre on an old antimicrobial agent. Biotechnol. Adv. 36, 1391–1411. doi: 10.1016/j.biotechadv.2018.05.004
Monogue, M. L., Giovagnoli, S., Bissantz, C., Zampaloni, C., and Nicolau, D. P. (2018a). In vivo efficacy of meropenem, with a novel non-β-lactam β-lactamase inhibitor, nacubactam, against gram-negative organisms exhibiting various resistance mechanisms in a murine complicated urinary tract infection model. Antimicrob. Agents Chemother. 62:e02596-17. doi: 10.1128/AAC.02596-17
Monogue, M. L., Sakoulas, G., Nizet, V., and Nicolau, D. P. (2018b). Humanized exposures of a β-lactam-β-lactamase inhibitor, tazobactam, versus non-β-lactam-β-lactamase inhibitor, avibactam, with or without colistin, against Acinetobacter baumannii in murine thigh and lung infection models. Pharmacology 101, 255–261. doi: 10.1159/000486445
Moon, S. H., Zhang, X., Zheng, G., Meeker, D. G., Smeltzer, M. S., and Huang, E. (2017). Novel linear lipopeptide paenipeptins with potential for eradicating biofilms and sensitizing gram-negative bacteria to rifampicin and clarithromycin. J. Med. Chem. 60, 9630–9640. doi: 10.1021/acs.jmedchem.7b01064
Morimoto, K., Ozawa, T., Awazu, K., Ito, N., Honda, N., Matsumoto, S., et al. (2014). Photodynamic therapy using systemic administration of 5-aminolevulinic acid and a 410-nm wavelength light-emitting diode for methicillin-resistant Staphylococcus aureus-infected ulcers in mice. PLoS ONE 9:e105173. doi: 10.1371/journal.pone.0105173
Munger, M. A., Radwanski, P., Hadlock, G. C., Stoddard, G., Shaaban, A., Falconer, J., et al. (2014). In vivo human time-exposure study of orally dosed commercial silver nanoparticles. Nanomed. Nanotechnol. Biol. Med. 10, 1–9. doi: 10.1016/j.nano.2013.06.010
Nakonieczna, J., Wolnikowska, K., Ogonowska, P., Neubauer, D., Bernat, A., and Kamysz, W. (2018). Rose bengal-mediated photoinactivation of multidrug resistant Pseudomonas aeruginosa is enhanced in the presence of antimicrobial peptides. Front. Microbiol. 9:1949. doi: 10.3389/fmicb.2018.01949
Navidinia, M. (2016). The clinical importance of emerging ESKAPE pathogens in nosocomial infections. J. Paramed. Sci. 7, 2008–4978. doi: 10.22037/jps.v7i3.12584
Nigo, M., Diaz, L., Carvajal, L. P., Tran, T. T., Rios, R., Panesso, D., et al. (2017). Ceftaroline-resistant, daptomycin-tolerant, and heterogeneous vancomycin-intermediate methicillin-resistant Staphylococcus aureus causing infective endocarditis. Antimicrob. Agents Chemother. 61:e01235-16. doi: 10.1128/AAC.01235-16
Nour El Din, S., El-Tayeb, T. A., Abou-Aisha, K., and El-Azizi, M. (2016). In vitro and in vivo antimicrobial activity of combined therapy of silver nanoparticles and visible blue light against Pseudomonas aeruginosa. Int. J. Nanomed. 11, 1749–1758. doi: 10.2147/IJN.S102398
Nowak, J., Zander, E., Stefanik, D., Higgins, P. G., Roca, I., Vila, J., et al. (2017). High incidence of pandrug-resistant Acinetobacter baumannii isolates collected from patients with ventilator-associated pneumonia in Greece, Italy and Spain as part of the magicbullet clinical trial. J. Antimicrob. Chemother. 72, 3277–3282. doi: 10.1093/jac/dkx322
Ostorhazi, E., Hoffmann, R., Herth, N., Wade, J. D., Kraus, C. N., and Otvos, L. (2018). Advantage of a narrow spectrum host defense (Antimicrobial) peptide over a broad spectrum analog in preclinical drug development. Front. Chem. 6:359. doi: 10.3389/fchem.2018.00359
Otvos, L., Ostorhazi, E., Szabo, D., Zumbrun, S. D., Miller, L. L., Halasohoris, S. A., et al. (2018). Synergy between proline-rich antimicrobial peptides and small molecule antibiotics against selected gram-negative pathogens in vitro and in vivo. Front. Chem. 6:309. doi: 10.3389/fchem.2018.00309
Palavutitotai, N., Jitmuang, A., Tongsai, S., Kiratisin, P., and Angkasekwinai, N. (2018). Epidemiology and risk factors of extensively drug-resistant Pseudomonas aeruginosa infections. PLoS ONE 13:e0193431. doi: 10.1371/journal.pone.0193431
Pallavali, R. R., Degati, V. L., Lomada, D., Reddy, M. C., and Durbaka, V. R. P. (2017). Isolation and in vitro evaluation of bacteriophages against MDR-bacterial isolates from septic wound infections. PLoS ONE 12:e0179245. doi: 10.1371/journal.pone.0179245
Panáček, A., Smékalová, M., Kilianová, M., Prucek, R., Bogdanová, K., Večeřová, R., et al. (2015). Strong and nonspecific synergistic antibacterial efficiency of antibiotics combined with silver nanoparticles at very low concentrations showing no cytotoxic effect. Molecules 21:26. doi: 10.3390/molecules21010026
Pendleton, J. N., Gorman, S. P., and Gilmore, B. F. (2013). Clinical relevance of the ESKAPE pathogens. Expert Rev. Anti. Infect. Ther. 11, 297–308. doi: 10.1586/eri.13.12
Peng, Y., Song, C., Yang, C., Guo, Q., and Yao, M. (2017). Low molecular weight chitosan-coated silver nanoparticles are effective for the treatment of MRSA-infected wounds. Int. J. Nanomed. 12, 295–304. doi: 10.2147/IJN.S122357
Pfalzgraff, A., Brandenburg, K., and Weindl, G. (2018). Antimicrobial peptides and their therapeutic potential for bacterial skin infections and wounds. Front. Pharmacol. 9:281. doi: 10.3389/fphar.2018.00281
Philipson, C., Voegtly, L., Lueder, M., Long, K., Rice, G., Frey, K., et al. (2018). Characterizing phage genomes for therapeutic applications. Viruses 10:188. doi: 10.3390/v10040188
Pirnay, J. P., Verbeken, G., Ceyssens, P. J., Huys, I., De Vos, D., Ameloot, C., et al. (2018). The magistral phage. Viruses 10:64. doi: 10.3390/v10020064
Pletzer, D., Mansour, S. C., and Hancock, R. E. W. (2018). Synergy between conventional antibiotics and anti-biofilm peptides in a murine, sub-cutaneous abscess model caused by recalcitrant ESKAPE pathogens. PLoS Pathog. 14:e1007084. doi: 10.1371/journal.ppat.1007084
Pompilio, A., Geminiani, C., Bosco, D., Rana, R., Aceto, A., Bucciarelli, T., et al. (2018). Electrochemically synthesized silver nanoparticles are active against planktonic and biofilm cells of Pseudomonas aeruginosa and other cystic fibrosis-associated bacterial pathogens. Front. Microbiol. 9:1349. doi: 10.3389/fmicb.2018.01349
Prakash, V., Mishra, P., Premi, H., Walia, A., Dhawan, S., and Kumar, A. (2014). Increasing incidence of multidrug resistant Pseudomonas aeruginosa in inpatients of a tertiary care hospital. Int. J. Res. Med. Sci. 2:1302. doi: 10.5455/2320-6012.ijrms20141111
Qayyum, S., Oves, M., and Khan, A. U. (2017). Obliteration of bacterial growth and biofilm through ROS generation by facilely synthesized green silver nanoparticles. PLoS ONE 12:0181363. doi: 10.1371/journal.pone.0181363
Radulescu, M., Andronescu, E., Dolete, G., Popescu, R. C., Fufă, O., Chifiriuc, M. C., et al. (2016). Silver nanocoatings for reducing the exogenous microbial colonization of wound dressings. Materials 9:345. doi: 10.3390/ma9050345
Rath, G., Hussain, T., Chauhan, G., Garg, T., and Goyal, A. K. (2016). Collagen nanofiber containing silver nanoparticles for improved wound-healing applications. J. Drug Target. 24, 520–529. doi: 10.3109/1061186X.2015.1095922
Regeimbal, J. M., Jacobs, A. C., Corey, B. W., Henry, M. S., Thompson, M. G., Pavlicek, R. L., et al. (2016). Personalized therapeutic cocktail of wild environmental phages rescues mice from Acinetobacter baumannii wound infections. Antimicrob. Agents Chemother. 60, 5806–5816. doi: 10.1128/AAC.02877-15
Reinhardt, A., and Neundorf, I. (2016). Design and application of antimicrobial peptide conjugates. Int. J. Mol. Sci. 17:E701. doi: 10.3390/ijms17050701
Rice, L. B. (2008). Federal funding for the study of antimicrobial resistance in nosocomial pathogens: no ESKAPE. J. Infect. Dis. 197, 1079–1081. doi: 10.1086/533452
Rineh, A., Bremner, J. B., Hamblin, M. R., Ball, A. R., Tegos, G. P., and Kelso, M. J. (2018). Attaching NorA efflux pump inhibitors to methylene blue enhances antimicrobial photodynamic inactivation of Escherichia coli and Acinetobacter baumannii in vitro and in vivo. Bioorg. Med. Chem. Lett. 28, 2736–2740. doi: 10.1016/j.bmcl.2018.02.041
Rineh, A., Dolla, N. K., Ball, A. R., Magana, M., Bremner, J. B., Hamblin, M. R., et al. (2017). Attaching the NorA efflux pump inhibitor INF55 to methylene blue enhances antimicrobial photodynamic inactivation of methicillin-resistant Staphylococcus aureus in vitro and in vivo. ACS Infect. Dis. 3, 756–766. doi: 10.1021/acsinfecdis.7b00095
Rios, A. C., Moutinho, C. G., Pinto, F. C., Del Fiol, F. S., Jozala, A., Chaud, M. V., et al. (2016). Alternatives to overcoming bacterial resistances: state-of-the-art. Microbiol. Res. 191, 51–80. doi: 10.1016/j.micres.2016.04.008
Rishi, N. P., Vashist, T., Sharma, A., Kaur, A., Kaur, A., Kaur, N., et al. (2018). Efficacy of designer K11 antimicrobial peptide (a hybrid of melittin, cecropin A1 and magainin 2) against Acinetobacter baumannii infected wounds. Pathog. Dis. 76:fty072. doi: 10.1093/femspd/fty072
Rohde, C., Resch, G., Pirnay, J. P., Blasdel, B., Debarbieux, L., Gelman, D., et al. (2018). Expert opinion on three phage therapy related topics: bacterial phage resistance, phage training and prophages in bacterial production strains. Viruses 10:178. doi: 10.3390/v10040178
Ronqui, M. R., de Aguiar Coletti, T. M. S. F., de Freitas, L. M., Miranda, E. T., and Fontana, C. R. (2016). Synergistic antimicrobial effect of photodynamic therapy and ciprofloxacin. J. Photochem. Photobiol. B Biol. 158, 122–129. doi: 10.1016/j.jphotobiol.2016.02.036
Rubino, C. M., Bhavnani, S. M., Loutit, J. S., Lohse, B., Dudley, M. N., and Griffith, D. C. (2018). Single-dose pharmacokinetics and safety of meropenem-vaborbactam in subjects with chronic renal impairment. Antimicrob. Agents Chemother. 62:e02103-17. doi: 10.1128/AAC.02103-17
Sangaonkar, G. M., and Pawar, K. D. (2018). Garcinia indica mediated biogenic synthesis of silver nanoparticles with antibacterial and antioxidant activities. Colloids Surf. B. Biointerfaces 164, 210–217. doi: 10.1016/j.colsurfb.2018.01.044
Santajit, S., and Indrawattana, N. (2016). Mechanisms of antimicrobial resistance in ESKAPE pathogens. Biomed Res. Int. 2016:2475067. doi: 10.1155/2016/2475067
Santos, A. L., Sodre, C. L., Valle, R. S., Silva, B. A., Souza-Goncalves, A. L., Sangenito, L. S., et al. (2012). Antimicrobial action of chelating agents: repercussions on the microorganism development, virulence and pathogenesis. Curr. Med. Chem. 19, 2715–2737. doi: 10.2174/092986712800609788
Schooley, R. T., Biswas, B., Gill, J. J., Hernandez-Morales, A., Lancaster, J., Lessor, L., et al. (2017). Development and use of personalized bacteriophage-based therapeutic cocktails to treat a patient with a disseminated resistant Acinetobacter baumannii Infection. Antimicrob. Agents Chemother. 61:e00954-17. doi: 10.1128/AAC.00954-17
Seo, M. D., Won, H. S., Kim, J. H., Mishig-Ochir, T., Lee, B. J., Seo, M. D., et al. (2012). Antimicrobial peptides for therapeutic applications: a review. Molecules 17, 12276–12286. doi: 10.3390/molecules171012276
Servick, K. (2016). Beleaguered phage therapy trial presses on. Science 352, 1506–1506. doi: 10.1126/science.352.6293.1506
Shalumon, K. T., Sheu, C., Chen, C. H., Chen, S. H., Jose, G., Kuo, C. Y., et al. (2018). Multi-functional electrospun antibacterial core-shell nanofibrous membranes for prolonged prevention of post-surgical tendon adhesion and inflammation. Acta Biomater. 72, 121–136. doi: 10.1016/j.actbio.2018.03.044
Shivaswamy, V. C., Kalasuramath, S. B., Sadanand, C. K., Basavaraju, A. K., Ginnavaram, V., Bille, S., et al. (2015). Ability of bacteriophage in resolving wound infection caused by multidrug-resistant Acinetobacter baumannii in uncontrolled diabetic rats. Microb. Drug Resist. 21, 171–177. doi: 10.1089/mdr.2014.0120
Siddiqi, K. S., Husen, A., and Rao, R. A. K. (2018). A review on biosynthesis of silver nanoparticles and their biocidal properties. J. Nanobiotechnol. 16:14. doi: 10.1186/s12951-018-0334-5
Singh, R., Vora, J., Nadhe, S. B., Wadhwani, S. A., Shedbalkar, U. U., and Chopade, B. A. (2018). Antibacterial activities of bacteriagenic silver nanoparticles against nosocomial Acinetobacter baumannii. J. Nanosci. Nanotechnol. 18, 3806–3815. doi: 10.1166/jnn.2018.15013
Singla, S., Harjai, K., Katare, O. P., and Chhibber, S. (2016). Encapsulation of bacteriophage in liposome accentuates its entry in to macrophage and shields it from neutralizing antibodies. PLoS ONE 11:e0153777. doi: 10.1371/journal.pone.0153777
Skinner, K., Sandoe, J. A. T., Rajendran, R., Ramage, G., and Lang, S. (2017). Efficacy of rifampicin combination therapy for the treatment of enterococcal infections assessed in vivo using a Galleria mellonella infection model. Int. J. Antimicrob. Agents 49, 507–511. doi: 10.1016/j.ijantimicag.2016.12.006
Soothill, J. S. (1992). Treatment of experimental infections of mice with bacteriophages. J. Med. Microbiol. 37, 258–261. doi: 10.1099/00222615-37-4-258
Stanley, I. J., Kajumbula, H., Bazira, J., Kansiime, C., Rwego, I. B., and Asiimwe, B. B. (2018). Multidrug resistance among Escherichia coli and Klebsiella pneumoniae carried in the gut of out-patients from pastoralist communities of Kasese district, Uganda. PLoS ONE 13:e0200093. doi: 10.1371/journal.pone.0200093
Sueoka, K., Chikama, T., Latief, M. A., Ko, J. A., Kiuchi, Y., Sakaguchi, T., et al. (2018). Time-dependent antimicrobial effect of photodynamic therapy with TONS 504 on Pseudomonas aeruginosa. Lasers Med. Sci. 33, 1455–1460. doi: 10.1007/s10103-018-2490-0
Sybesma, W., Rohde, C., Bardy, P., Pirnay, J. P., Cooper, I., Caplin, J., et al. (2018). Silk route to the acceptance and re-implementation of bacteriophage therapy—part II. Antibiotics 7:35. doi: 10.3390/antibiotics7020035
Tacconelli, E., Carrara, E., Savoldi, A., Harbarth, S., Mendelson, M., Monnet, D. L., et al. (2018). Discovery, research, and development of new antibiotics: the WHO priority list of antibiotic-resistant bacteria and tuberculosis. Lancet Infect. Dis. 18, 318–327. doi: 10.1016/S1473-3099(17)30753-3
Tegos, G. P., and Hamblin, M. R. (2006). Phenothiazinium antimicrobial photosensitizers are substrates of bacterial multidrug resistance pumps. Antimicrob. Agents Chemother. 50, 196–203. doi: 10.1128/AAC.50.1.196-203.2006
Tegos, G. P., Masago, K., Aziz, F., Higginbotham, A., Stermitz, F. R., and Hamblin, M. R. (2008). Inhibitors of bacterial multidrug efflux pumps potentiate antimicrobial photoinactivation. Antimicrob. Agents Chemother. 52, 3202–3209. doi: 10.1128/AAC.00006-08
Téllez, G. A., Zapata, J. A., Toro, L. J., Henao, D. C., Bedoya, J. P., Rivera, J. D., et al. (2018). Identification, characterization, immunolocalization, and biological activity of lucilin peptide. Acta Trop. 185, 318–326. doi: 10.1016/j.actatropica.2018.06.003
Tomb, R. M., Maclean, M., Coia, J. E., MacGregor, S. J., and Anderson, J. G. (2017). Assessment of the potential for resistance to antimicrobial violet-blue light in Staphylococcus aureus. Antimicrob. Resist. Infect. Control 6:100. doi: 10.1186/s13756-017-0261-5
Tomb, R. M., White, T. A., Coia, J. E., Anderson, J. G., MacGregor, S. J., and Maclean, M. (2018). Review of the comparative susceptibility of microbial species to photoinactivation using 380–480 nm violet-blue light. Photochem. Photobiol. 94, 445–458. doi: 10.1111/php.12883
Ujmajuridze, A., Chanishvili, N., Goderdzishvili, M., Leitner, L., Mehnert, U., Chkhotua, A., et al. (2018). Adapted bacteriophages for treating urinary tract infections. Front. Microbiol. 9:1832. doi: 10.3389/fmicb.2018.01832
Ullah, A., Zhang, Y., Iqbal, Z., Zhang, Y., Wang, D., Chen, J., et al. (2018). Household light source for potent photo-dynamic antimicrobial effect and wound healing in an infective animal model. Biomed. Opt. Express 9, 1006–1019. doi: 10.1364/BOE.9.001006
Vazquez-grande, G., and Kumar, A. (2015). Optimizing antimicrobial therapy of sepsis and septic shock : focus on antibiotic combination therapy. Semin Respir Crit Care Med. 1, 154–166. doi: 10.1055/s-0034-1398742
Verbelen, J., Hoeksema, H., Heyneman, A., Pirayesh, A., and Monstrey, S. (2014). Aquacel® Ag dressing versus ActicoatTM dressing in partial thickness burns: a prospective, randomized, controlled study in 100 patients. Part 1: burn wound healing. Burns 40, 416–427. doi: 10.1016/j.burns.2013.07.008
Vestergaard, M., Paulander, W., Marvig, R. L., Clasen, J., Jochumsen, N., Molin, S., et al. (2016). Antibiotic combination therapy can select for broad-spectrum multidrug resistance in Pseudomonas aeruginosa. Int. J. Antimicrob. Agents 47, 48–55. doi: 10.1016/j.ijantimicag.2015.09.014
Viertel, T. M., Ritter, K., and Horz, H. P. (2014). Viruses versus bacteria-novel approaches to phage therapy as a tool against multidrug-resistant pathogens. J. Antimicrob. Chemother. 69, 2326–2336. doi: 10.1093/jac/dku173
Vuotto, C., Longo, F., Pascolini, C., Donelli, G., Balice, M. P., Libori, M. F., et al. (2017). Biofilm formation and antibiotic resistance in Klebsiella pneumoniae urinary strains. J. Appl. Microbiol. 123, 1003–1018. doi: 10.1111/jam.13533
Wan, G., Ruan, L., Yin, Y., Yang, T., Ge, M., and Cheng, X. (2016). Effects of silver nanoparticles in combination with antibiotics on the resistant bacteria Acinetobacter baumannii. Int. J. Nanomed. Vol. 11, 3789–3800. doi: 10.2147/IJN.S104166
Wang, G., Li, X., and Zasloff, M. (2010). “A database view of naturally occurring antimicrobial peptides: nomenclature, classification and amino acid sequence analysis,” in Antimicrobial Peptides: Discovery, Design and Novel Therapeutic Strategies (Oxfordshire: CABI), 1–21. doi: 10.1079/9781845936570.0001
Wang, S., Yan, C., Zhang, X., Shi, D., Chi, L., Luo, G., et al. (2018). Antimicrobial peptide modification enhances the gene delivery and bactericidal efficiency of gold nanoparticles for accelerating diabetic wound healing. Biomater. Sci. 6, 2757–2772. doi: 10.1039/C8BM00807H
Wang, Y., Harrington, O. D., Wang, Y., Murray, C. K., Hamblin, M. R., and Dai, T. (2017). In vivo investigation of antimicrobial blue light therapy for multidrug- resistant Acinetobacter baumannii burn infections using bioluminescence imaging. J. Vis. Exp. 122:e54997. doi: 10.3791/54997
Wang, Z., Zheng, P., Ji, W., Fu, Q., Wang, H., Yan, Y., et al. (2016). SLPW: a virulent bacteriophage targeting methicillin-resistant Staphylococcus aureus in vitro and in vivo. Front. Microbiol. 7:934. doi: 10.3389/fmicb.2016.00934
Wen, X., Zhang, X., Szewczyk, G., El-Hussein, A., Huang, Y.-Y., Sarna, T., et al. (2017). Potassium iodide potentiates antimicrobial photodynamic inactivation mediated by rose bengal in in vitro and in vivo studies. Antimicrob. Agents Chemother. 61:e00467-17. doi: 10.1128/AAC.00467-17
Wittebole, X., De Roock, S., and Opal, S. M. (2014). A historical overview of bacteriophage therapy as an alternative to antibiotics for the treatment of bacterial pathogens. Virulence 5, 226–235. doi: 10.4161/viru.25991
Wozniak, A., and Grinholc, M. (2018). Combined antimicrobial activity of photodynamic inactivation and antimicrobials-state of the art. Front. Microbiol. 9:930. doi: 10.3389/fmicb.2018.00930
Wypij, M., Świecimska, M., Czarnecka, J., Dahm, H., Rai, M., and Golinska, P. (2018). Antimicrobial and cytotoxic activity of silver nanoparticles synthesized from two haloalkaliphilic actinobacterial strains alone and in combination with antibiotics. J. Appl. Microbiol. 124, 1411–1424. doi: 10.1111/jam.13723
Xie, J., Li, Y., Li, J., Yan, Z., Wang, D., Guo, X., et al. (2018). Potent effects of amino acid scanned antimicrobial peptide Feleucin-K3 analogs against both multidrug-resistant strains and biofilms of Pseudomonas aeruginosa. Amino Acids 50, 1471–1483. doi: 10.1007/s00726-018-2625-4
Yadav, R., Bulitta, J. B., Wang, J., Nation, R. L., and Landersdorfer, C. B. (2017). Evaluation of pharmacokinetic/ pharmacodynamic model-based optimized combination regimens against multidrug-resistant Pseudomonas aeruginosa in a murine thigh infection model by using humanized dosing schemes. Antimicrob. Agents Chemother. 61:e01268-17. doi: 10.1128/AAC.01268-17
Yang, H., Chen, G., Hu, L., Liu, Y., Cheng, J., Ye, Y., et al. (2018a). Enhanced efficacy of imipenem-colistin combination therapy against multiple-drug-resistant Enterobacter cloacae: in vitro activity and a Galleria mellonella model. J. Microbiol. Immunol. Infect. 51, 70–75. doi: 10.1016/j.jmii.2016.01.003
Yang, H., Xu, J., Li, W., Wang, S., Li, J., Yu, J., et al. (2018b). Staphylococcus aureus virulence attenuation and immune clearance mediated by a phage lysin-derived protein. EMBO J. 37:e98045. doi: 10.15252/embj.201798045
Yang, M. Y., Chang, K. C., Chen, L. Y., Wang, P. C., Chou, C. C., Wu, Z. B., et al. (2018). Blue light irradiation triggers the antimicrobial potential of ZnO nanoparticles on drug-resistant Acinetobacter baumannii. J. Photochem. Photobiol. B. 180, 235–242. doi: 10.1016/j.jphotobiol.2018.02.003
Yoshizumi, A., Ishii, Y., Livermore, D. M., Woodford, N., Kimura, S., Saga, T., et al. (2013). Efficacies of calcium-EDTA in combination with imipenem in a murine model of sepsis caused by Escherichia coli with NDM-1 β-lactamase. J. Infect. Chemother. 19, 992–995. doi: 10.1007/s10156-012-0528-y
Youn Jun, S., Jin Jang, I., Yoon, S., Jang, K., Yu, K. S., Youn Cho, J., et al. (2017). Pharmacokinetics and tolerance of the phage endolysin-based candidate drug SAL200 after a single intravenous administration among healthy volunteers. Antimicrob. Agents Chemother. 61:e02629-16. doi: 10.1128/AAC.02629-16
Zeng, K. J., Doi, Y., Patil, S., Huang, X., and Tian, G. B. (2016). Emergence of the plasmid-mediated mcr-1 gene in colistin-resistant Enterobacter aerogenes and Enterobacter cloacae. Antimicrob. Agents Chemother. 60, 3862–3863. doi: 10.1128/AAC.00345-16
Zhang, J., Xu, L. L., Gan, D., and Zhang, X. (2018). In vitro study of bacteriophage AB3 endolysin LysAB3 activity against Acinetobacter baumannii biofilm and biofilm-bound A. baumannii. Clin. Lab. 64, 1021–1030. doi: 10.7754/Clin.Lab.2018.180342
Zhang, Y., Dai, T., Wang, M., Vecchio, D., Chiang, L. Y., and Hamblin, M. R. (2015). Potentiation of antimicrobial photodynamic inactivation mediated by a cationic fullerene by added iodide: in vitro and in vivo studies. Nanomedicine 10, 603–614. doi: 10.2217/nnm.14.131
Zhao, Y., Lu, Z., Dai, X., Wei, X., Yu, Y., Chen, X., et al. (2018). Glycomimetic-conjugated photosensitizer for specific Pseudomonas aeruginosa recognition and targeted photodynamic therapy. Bioconjug. Chem. 29, 3222–3230. doi: 10.1021/acs.bioconjchem.8b00600
Zheng, Z., Tharmalingam, N., Liu, Q., Jayamani, E., Kim, W., Fuchs, B. B., et al. (2017). Synergistic efficacy of Aedes aegypti antimicrobial peptide cecropin A2 and tetracycline against Pseudomonas aeruginosa. Antimicrob. Agents Chemother. 61:e00686-17. doi: 10.1128/AAC.00686-17
Zhou, W., Feng, Y., and Zong, Z. (2018). Two new lytic bacteriophages of the myoviridae family against carbapenem-resistant Acinetobacter baumannii. Front. Microbiol. 9:850. doi: 10.3389/fmicb.2018.00850
Keywords: ESKAPE, multidrug resistance, alternative therapy, combination therapy, phage therapy, antimicrobial peptides, silver nanoparticles, photodynamic light therapy
Citation: Mulani MS, Kamble EE, Kumkar SN, Tawre MS and Pardesi KR (2019) Emerging Strategies to Combat ESKAPE Pathogens in the Era of Antimicrobial Resistance: A Review. Front. Microbiol. 10:539. doi: 10.3389/fmicb.2019.00539
Received: 31 May 2018; Accepted: 01 March 2019;
Published: 01 April 2019.
Edited by:
Rebecca Thombre, Modern College of Arts, Science and Commerce, Pune University, IndiaReviewed by:
Vishvanath Tiwari, Central University of Rajasthan, IndiaRajashree Bhalchandra Patwardhan, Savitribai Phule Pune University, India
Copyright © 2019 Mulani, Kamble, Kumkar, Tawre and Pardesi. This is an open-access article distributed under the terms of the Creative Commons Attribution License (CC BY). The use, distribution or reproduction in other forums is permitted, provided the original author(s) and the copyright owner(s) are credited and that the original publication in this journal is cited, in accordance with accepted academic practice. No use, distribution or reproduction is permitted which does not comply with these terms.
*Correspondence: Karishma R. Pardesi, karishma@unipune.ac.in