- 1Departamento de Microbiología e Inmunología, Facultad de Ciencias Biológicas, Universidad Autónoma de Nuevo León, San Nicolás de los Garza, Mexico
- 2Departamento de Ciencias Químico Biológicas, División de Ciencias e Ingeniería, Universidad de Sonora, Navojoa, Mexico
Endophytes constitute plant-colonizing microorganisms in a mutualistic symbiosis relationship. They are found in most ecosystems reducing plant crops’ biotic and abiotic stressors by stimulating immune responses, excluding plant pathogens by niche competition, and participating in antioxidant activities and phenylpropanoid metabolism, whose activation produces plant defense, structural support, and survival molecules. In fact, metabolomic studies have demonstrated that endophyte genes associated to specific metabolites are involved in plant growth promotion (PGP) by stimulating plant hormones production such as auxins and gibberellins or as plant protective agents against microbial pathogens, cancer, and insect pests, but eco-friendly and eco-safe. A number of metabolites of Gram-positive endophytes isolated from agriculture, forest, mangrove, and medicinal plants, mainly related to the Firmicutes phyla, possess distinctive biocontrol and plant growth-promoting activities. In general, Actinobacteria and Bacillus endophytes produce aromatic compounds, lipopeptides, plant hormones, polysaccharides, and several enzymes linked to phenylpropanoid metabolism, thus representing high potential for PGP and crop management strategies. Furthermore, Actinobacteria have been shown to produce metabolites with antimicrobial and antitumor activities, useful in agriculture, medicine, and veterinary areas. The great endophytes diversity, their metabolites production, and their adaptation to stress conditions make them a suitable and unlimited source of novel metabolites, whose application could reduce agrochemicals usage in food and drugs production.
Introduction to Endophytes
Endophytes are facultative or obligate symbiotic microorganisms, mainly bacterial and fungal species, that live in apparently healthy internal plant tissues, without causing disease (Schulz and Boyle, 2006). The most studied ones are bacterial and fungal species.
The purpose of this minireview is to highlight the importance of previously reported endophytic Gram-positive bacteria bioactive products. The International Union for Conservation of Nature and Natural Resources estimates that there are about 297,326 species of plants (Monocotyledons, Dicotyledons, Gymnosperms, Ferns and allies and Mosses), but only a few of them have been studied for their endophyte microbiota (Strobel and Daisy, 2003; Aitken, 2004). Endophytic microorganisms are known to influence plant physiology and development, among which, Gram-positive bacteria are important in such activities as bioremediation, biocontrol, plant growth, symbiotic-mutualistic, commensalistic, trophobiotic interactions, control of soil-borne pathogens, and support of host plant defense against environmental stress (Ryan et al., 2008). An endophytic community is complex and several factors may affect its structure, such as plant-microbe and microbe-microbe interactions and environmental conditions (Ryan et al., 2008). For bacterial endophytes diversity analysis, cultivation-based and culture-independent methods are used. In regard to cultivation studies, a great number of bacteria, mostly Proteobacteria, have been reported as endophytes, being the most frequent from Actinobacteria, Bacteroidetes, and Firmicutes phyla (Rosenblueth and Martínez-Romero, 2006).
The most abundant metabolite producing Gram-positive bacteria endophytes found within diverse environments are Bacillus and Streptomyces species (Reinhold-Hurek and Hurek, 2011; Frank et al., 2017; Figures 1–3).
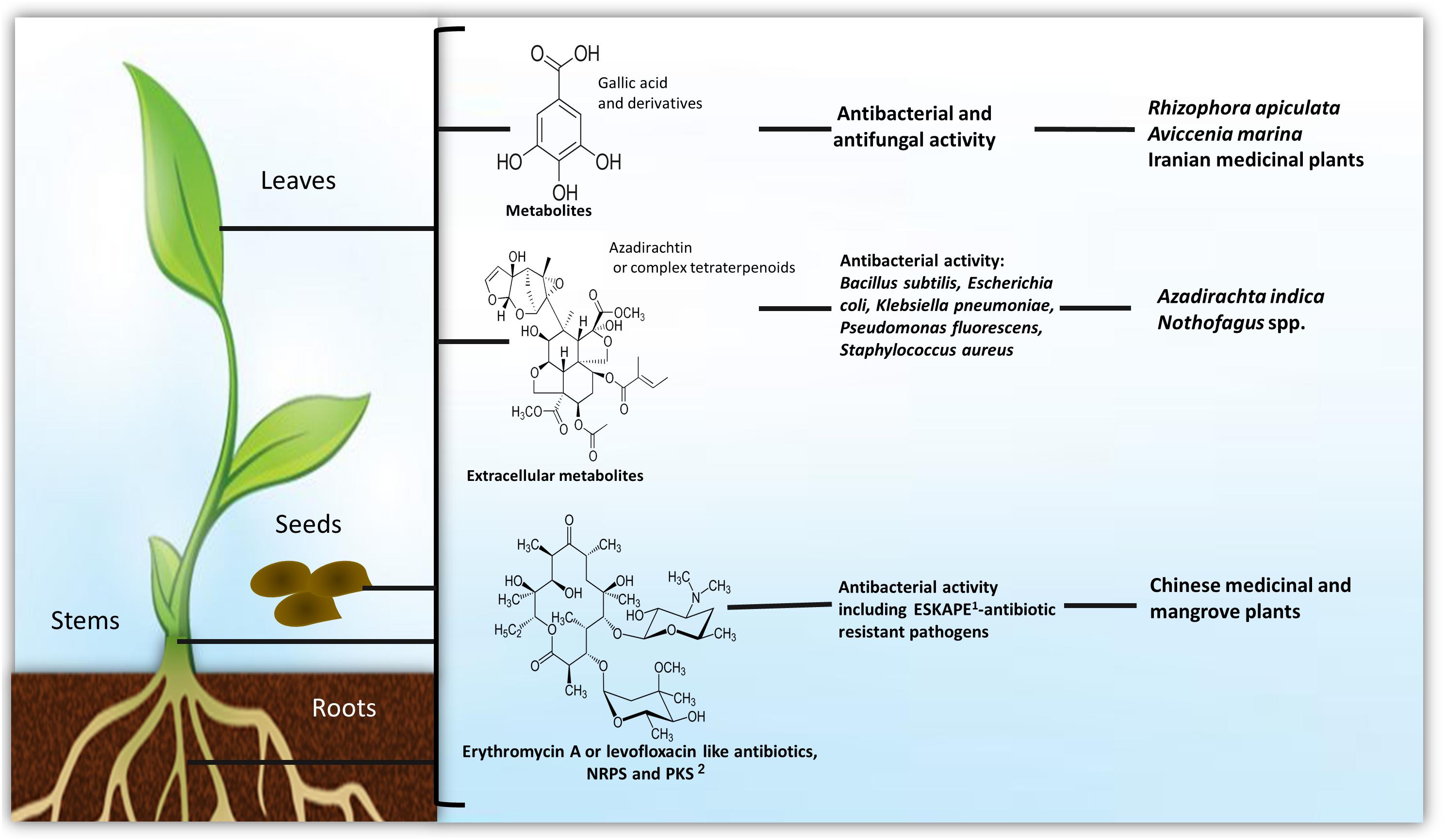
Figure 1. Production of metabolites by endophytes among plant tissues. 1The “ESKAPE” bacterial pathogens (Acinetobacter baumannii, Enterobacter spp., Enterococcus faecium, Klebsiella pneumoniae, Pseudomonas aeruginosa, and Staphylococcus aureus) are the leading nosocomial infectious agents throughout the world. 2Metabolites synthesized by non-ribosomal peptide synthetases (NRPS) or polyketide synthase (PKS). Detailed information on antimicrobial metabolites against animal/human pathogens are in Supplementary Table S1, against plant pathogens and insect pests in Supplementary Table S2, as plant growth stimulant in Supplementary Table S3 and as anti-cancer agent in Supplementary Table S4.
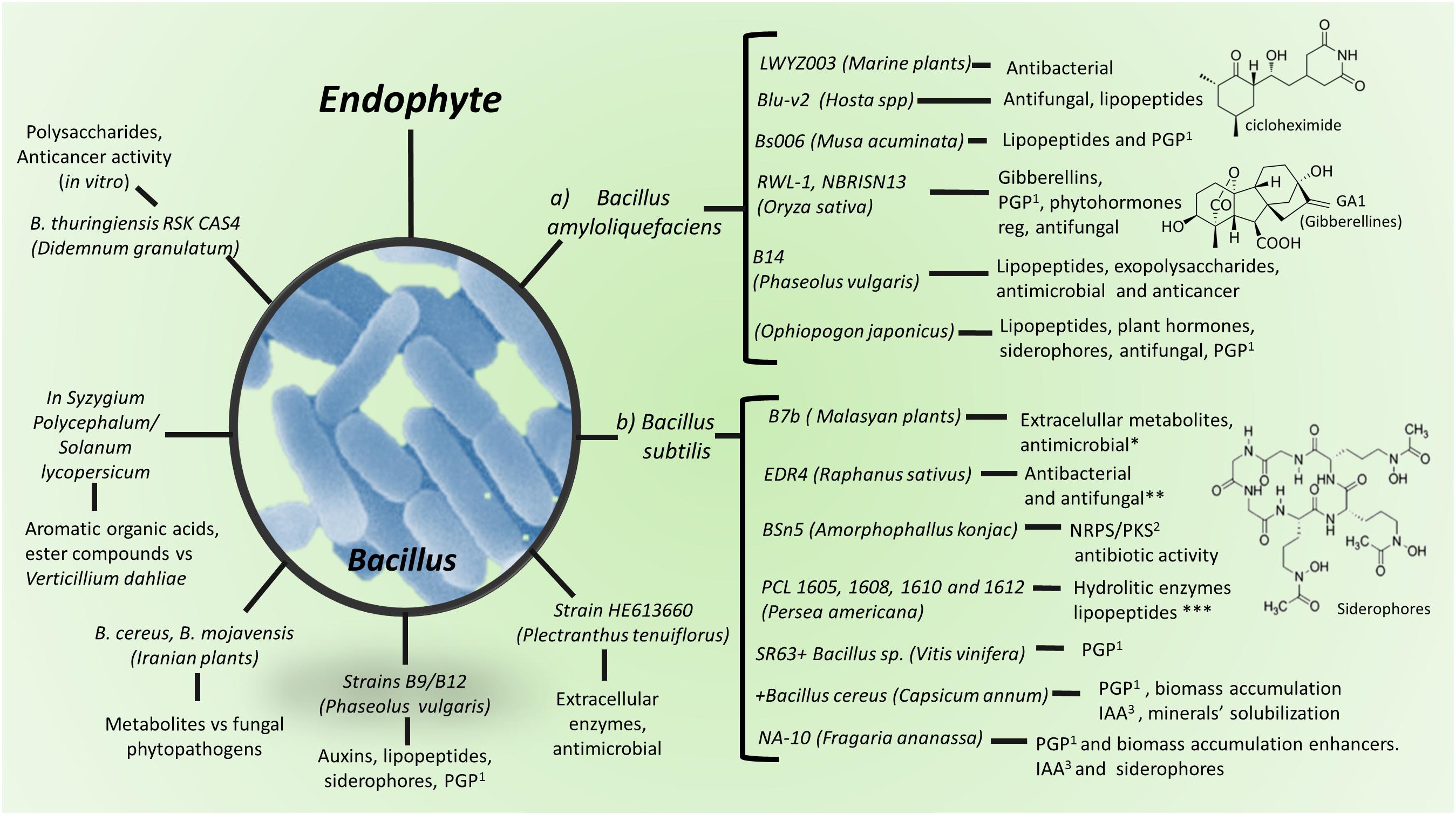
Figure 2. Production of metabolites by Bacillus spp. and strains as plant endophytes. (a) Bacillus amyloliquefaciens; (b) Bacillus subtilis. 1PGP, plant growth promoting. 2Metabolites synthesized by NRPS or PKS. 3IAA, indol acetic acid. Detailed information on antimicrobial metabolites against animal/human pathogens are in Supplementary Table S1, against plant pathogens and insect pests in Supplementary Table S2, as plant growth stimulant in Supplementary Table S3 and as anti-cancer agent in Supplementary Table S4.
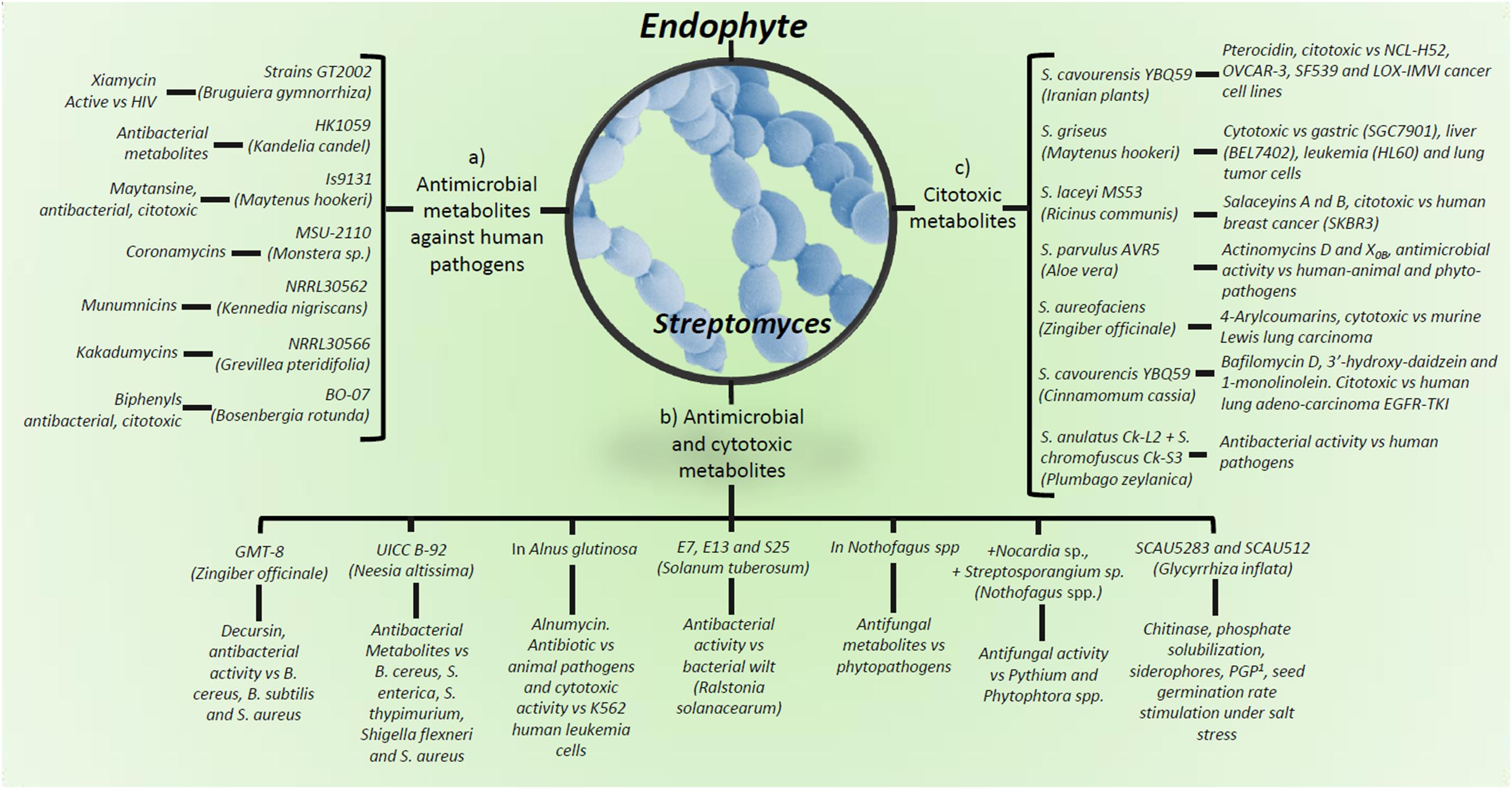
Figure 3. Production of metabolites by Streptomyces spp. and strains as plant endophytes. (a) Antimicrobial metabolites against human pathogens; (b) metabolites showing antimicrobial and cytotoxic activity; (c) cytotoxic metabolites against several tumor cell lines. 1PGP, plant growth promote. Detailed information on antimicrobial metabolites against animal/human pathogens are in Supplementary Table S1, against plant pathogens and insect pests in Supplementary Table S2, as plant growth stimulant in Supplementary Table S3 and as anti-cancer agent in Supplementary Table S4.
Endophytes are found in plants of most ecosystems and are of agricultural importance since they help to improve crops yields, by stimulating plants growth and immune response, excluding plant pathogens by niche competition, as well as actively participating in phenylpropanoid metabolism and antioxidant activities (Pandey et al., 2018). Among plant microbiota, endophytic bacteria can be found in most plant species and be recovered from roots, leaves, stems, and a few from flowers, fruits, and seeds (Lodewyckx et al., 2002); they have the potential to produce a variety of secondary metabolites with application in agriculture and pharmaceutical and industrial biotechnology (Lodewyckx et al., 2002; Strobel and Daisy, 2003; Ryan et al., 2008). Bacterial endophytes live within cell walls and xylem vessels intercellular regions and they may colonize seeds (Cankar et al., 2005; Johnston-Monje and Raizada, 2011), fruits (de Melo-Pereira et al., 2012), and flowers (Compant et al., 2011), among other tissues. It is known that endophytic bacteria are located in the apoplast (Koskimäki et al., 2015), and plant roots are proposed to be the entry point (Paungfoo-Lonhienne et al., 2010). It is also suggested that they are transmitted using an alternative vertical strategy due to their presence in flowers and seeds (Tamosiune et al., 2017). The potential explanation for their ubiquitous presence into plant tissues is the diversity of positive effects on plant growth and fitness they have shown, by stimulating the host phenylpropanoid pathway or by producing several linked-metabolites to the plants’ metabolism (Brader et al., 2014; Haidar et al., 2016; Lòpez-Fernàndez et al., 2016; Alaimo et al., 2018). Many reports indicate that bacterial endophytes help to provide nutrients as plant growth-promoters, and induce tolerance/resistance against biotic and abiotic stress conditions (Ryan et al., 2008).
In addition, several metabolites produced by microbial endophytes act as antimicrobial agents against human, animal, and plant pathogens. Whereas the antimicrobial effect against phytopathogens will have the positive effect on the host plant, the efficacies of endophyte metabolites may show a great clinical potential for medical and veterinary treatments. Indeed, nature-occurring antibiotics are low-molecular-weight products made by microbes that inhibit the growth or kill phytopathogens, bacteria, fungi, viruses, and protozoans, that cause human and animal diseases (Demain, 1981; Jakubiec-Krzesniak et al., 2018; Tripathi et al., 2018). Some important antibiotics producers have been recently found as endophytes in different plant species (Eljounaidi et al., 2016).
It is known that immunocompromised individuals (AIDS, cancer, and organ transplant patients) are at high risk for developing opportunistic microbial infections by Aspergillus sp., Candida albicans, Clostridium difficile, Coccidioides immitis, Cryptococcus neoformans, Cryptosporidium, Mycobacterium avium complex, M. tuberculosis, Pneumocystis jirovecii, Pseudomonas aeruginosa, Salmonella sp., Staphylococcus aureus, Streptococcus pneumoniae, and S. pyogenes, as well as parasitic infections caused by Cryptosporidium spp., Encephalitozoon spp., Isospora belli, Leishmania spp., Plasmodium falciparum, Toxoplasma gondii, and Trypanosoma cruzi. The urgent need for human diseases prevention and treatment, has promoted the discovery and development of novel and efficient therapeutic agents to which resistance has not been produced (Strobel and Daisy, 2003; Chinedum, 2005). For instance, drug resistance is a recognized phenomenon that disease-causing microbial agents develop against pharmaceutical therapy. Infectious diseases and cancer share similarities in the mechanisms of resistance to drugs, such as drug efflux, which is evolutionarily conserved (Housman et al., 2014). Therefore, in this review, the antibacterial, antifungal, antiviral, and antitumor activities of metabolites produced by specific Gram-positive bacteria endophytes are highlighted, as well as their potential use as plant growth promoters (PGP).
Gram-Positive Endophytes Against Human/Animal Pathogens
Among Actinobacteria, Actinomycetes are Gram-positive, filamentous bacteria with great potential as biocontrol agents, which produce approximately two-thirds of natural antibiotics, where 75% derived from Streptomyces species (de Lima Procópio et al., 2012; Figures 1, 3). Actinomycetes, including the Actinomyces, Actinoplanes, Amycolatopsis, Micromonospora, Saccharopolyspora, and Streptomyces genera, are recognized as bioactive secondary metabolites producers, not only showing antimicrobial, but also insecticidal and antitumoral activities (Renu et al., 2008; Kekuda et al., 2010).
Lipopeptides are among the most important classes of secondary metabolites produced by endophytic bacteria, which are formed by cyclic or short linear peptides linked to a lipid tail or lipophilic molecules. Lipopeptides may show antimicrobial, cytotoxic and surfactant activities; they are synthesized by non-ribosomal peptide synthetases (NRPS), or polyketide synthase (PKS) and have great structural diversity based on a hydrophobic fatty acid acyl chain of 13 to 17 carbons, linked to a hydrophilic peptide of 7–25 aminoacids. Lipopeptides are important for both, their antibiotic activity and for inducing plant defense mechanisms (Stein, 2005; Raaijmakers et al., 2010). One bacterial strain may synthesize several polypeptide isoforms. Bacillus and Paenibacillus-related lipopeptides are the most studied ones (Villarreal-Delgado et al., 2018), whereas several Bacillus amyloliquefaciens strains have been recognized as higher lipopeptides producers (Figure 2A; Ongena and Jacques, 2008). In addition to lipopeptides, B. subtilis produces NRPS lantibiotics (lanthionine-containing antibiotics) (Stein, 2005); lipopeptides are responsible for biofilm and swarming development, whereas lantibiotics play as pheromones in quorum-sensing (Stein, 2005). B. subtilis also produces compounds such as polyketides, an aminosugar, and a phospholipid; polyketides include bacillomycin, fengycin, iturin, lichenysin, mycosubtilin, plipastatin, pumilacidin, and surfactin (Figure 2B); whereas Paenibacillus polymyxa synthesizes polymyxins (cyclic cationic lipopeptides) (Stein, 2005; Grady et al., 2016; Supplementary Tables S1–S4).
In addition to biologically active secondary metabolites, bacterial endophytes also produce important antimicrobial enzymes, mainly by Bacilli class members (Figure 2). In a study looking for highly producing enzymes endophytes, in the mangrove in Thailand, Khianngam et al. (2013) found that Gram-positive bacteria showed more hydrolytic activity compared with that of Gram-negative ones. Testing endophytes in hosts from the Rhizophoraceae family, results showed amylase, cellulase, and lipase activity by B. infantis and B. granadenis; and amylase, cellulase, lipase, lipolytic and proteinase activity by B. safensis. Similarly, cellulase, lipase, and proteinase activities by Paenibacillus sp. and S. warneri were detected in Acanthaceae family endophytes. Endophytes isolated from Brazilian mangrove plants showed high enzymatic activity; among these isolates, Bacillus sp. (MCR2.56) was reported to show particularly high amylase and esterasic activities; six Bacillus isolates (MCR2.51, MCA2.42, MCA2.51, MBR2.4, MBA2.33, and MBA2.4) high endocellulolytic activity, whereas the actinobacteria Microbacterium sp. (MCA2.54) and Curtobacterium sp. (MBR2.20) showed high endoglucanase and protease activity, respectively (Castro et al., 2014; Figure 2 and Supplementary Tables S1–S3).
Bioactive endophytic Streptomycetes can be isolated from plants worldwide (Castillo et al., 2007; Figure 3). Medicinal plants have been used for centuries as an alternative therapy for disease treatment. Interestingly, Chinese medicinal plants-endophytic actinomycetes were reported to have antibacterial activity against E. coli and S. aureus (Zhao et al., 2011; Figure 1). Recently, endophytic actinomycetes were reported in several Chinese mangrove plants to exhibit antibacterial activity against Acinetobacter baumannii, Enterococcus faecalis, E. coli, Klebsiella pneumoniae, P. aeruginosa, and S. aureus, some of which are resistant to the vancomycin, methicillin, and carbapenem antibiotics (Jiang et al., 2018; Figures 1, 3). Several metabolites from endophytic Streptomyces species have been characterized and associated with antibiotic activity, including kakadumycins, munumbicins, p-aminoacetophenonic acids, and xiamycins (Castillo et al., 2002, 2003; Guan et al., 2005); antimalarial (coronamycin, munumbicin D) (Castillo et al., 2002; Ezra et al., 2004); and antifungal (munumbicin D) (Shimizu et al., 2000; Ezra et al., 2004) activities (Figure 3 and Supplementary Table S1). Similarly, Iranian medicinal plants-endophytic actinomyctes exhibited antimicrobial activity against the pathogenic bacteria B. cereus, B. subtilis, E. coli, Citrobacter freundii, K. pneumoniae, Proteus mirabilis, Shigella flexneri, and S. aureus (Beiranvand et al., 2017; Figures 1, 3), whereas Malaysian plants-endophytic B. subtilis possessed antibacterial activity against S. aureus, methicillin resistant S. aureus, and P. aeruginosa (Fikri et al., 2018; Figure 2B). The African Combretum molle-endophytic Bacillus and Lysinibacillus species exhibited antibacterial activity against B. cereus, E. coli, P. aeruginosa, and S. aureus (Diale et al., 2018; Supplementary Table S1).
Kennedia nigriscans-endophytic Streptomyces sp. strain NRRL 30562 produces munumbicins A, B, C, and D, active against growth of B. anthracis, E. faecalis, vancomycin-resistant E. faecalis, multiple-drug-resistant (MDR) M. tuberculosis, S. pneumoniae, S. aureus, and methicillin-resistant S. aureus; furthermore, munumbicins have been shown to be more effective than chloroquine to kill the malaria-causing agent P. falciparum (Castillo et al., 2002, 2006; Christina et al., 2013; Figure 3A). Similarly, kakadumycins have antibacterial and antimalarial activities comparable with those of munumbicins (Waring and Wakelin, 1974; Katagiri et al., 1975; Castillo et al., 2003; Figure 3A and Supplementary Table S1).
Streptomyces sp. strain SUK06, isolated from the Malasian medicinal plant Thottea grandiflora, commonly used as an alternative mean to heal wounds and treat skin infections and fever, produces secondary antimicrobial metabolites against B. cereus, B. subtilis, Plesiomonas shigelloides, P. aeruginosa, and methicillin-resistant S. aureus (Ghadin et al., 2008). Similarly, metabolites and cell wall-degrading enzymes from Panax ginseng- and Plectranthus tenuiflorus-endophytic Bacillus sp., Micrococcus sp., and P. polymyxa, were reported to possess antibacterial activity against E. coli, K. pneumoniae, P. mirabilis, Salmonella enterica subsp. enterica serovar Typhi, S. aureus, and S. agalactiae (El-Deeb et al., 2013; Figure 2 and Supplementary Table S1).
Secondary metabolites spinosyn A and D, are produced by the soil actinomycete Saccharopolyspora spinosa, highly effective against lepidopteran and dipteran pests, among others, which commercial product named spinosad, has been commercialized for ∼250 countries and adopted in integrated pest management programs worldwide. Furthermore, there are reports of endophytic Saccharopolyspora species, although their potential as bioinsecticide has not yet been elucidated (Qin et al., 2011).
There are many medical and agricultural applications for the Azadirachta indica (known as neem) produced compounds (Figure 1). Macrococcus caseolyticus (ALS-1), a member of the Firmicutes, has been reported to produce free radical scavenging compounds. This strain was isolated from Aloe vera in an effort to cultivate bacterial endophytes that could be related to this plant curative and therapeutic uses (Akinsanya et al., 2015). In fact, 80% of the A. vera bacterial endophytes produced 1,1-diphenyl-2-picrylhydrazyl, showing over 75% scavenging properties. The Raphanus sativus (young radish) leaf and root endophytic B. subtilis, Sphingobacterium siyangensis, and P. polymyxa were shown to inhibit the growth of B. cereus, E. coli, P. aeruginosa, and S. aureus, in addition to Salmonella, Shigella, and Listeria species (Liu et al., 2010). Zingiber officinale roots-endophytic Streptomyces sp. was shown to possess antimicrobial activity against B. cereus, B. subtilis, and S. aureus (Taechowisan et al., 2013; Figure 3B and Supplementary Table S1).
Gram-Positive Endophytes Against Plant Pathogens
Endophytes found in grapevine (Vitis vinifera) may represent one interesting example of a widely studied crop system. Metatranscriptoma analysis of vineyards prokaryotic microbiome confirmed that two out of three main bacterial phyla detected (Actinobacteria and Firmicutes) belonged to the Gram-positive group, thus reflecting bacterial metabolic assessments to become symbionts (either epiphytes or endophytes) and be distributed along plant tissues. This study demonstrated that the abundance and richness balance between beneficial microorganisms was critical for phytopathogens biocontrol and grapevine management, where the microbiota stability relied on environmental physicochemical conditions; being the soil type, geography and climate crucial factors to favor this crop. Moreover, detection of a specific strain reflected its ability to be established under the host microclimatic conditions, where Bacillus spp. were widely spread in flowers, leaves and grapes (Alaimo et al., 2018). In addition, Haidar et al. (2016) found that B. pumilus conferred systemic resistance against this pathogen, after studying the antagonistic bacteria modes of action for the phytopathogen Phaeomoniella chlamydospora biocontrol in grapevine.
The biological control of plant phytopathogens by endophytes was reported in late 50s where, a Micromonospora isolate from tomato showed antagonistic activity against Fusarium oxysporum f.sp. lycopersici (Manikprabhu and Li, 2016). As previously stated, the most abundant Gram-positive bacterial endophytes found within diverse environments are Bacillus and Streptomyces species (Reinhold-Hurek and Hurek, 2011; Frank et al., 2017), both exhibiting secondary metabolites showing antimicrobial activity also against plant pathogens. In fact, there have been proposed Bacillus spp. endophytes for crop management (Aloo et al., 2018; Figure 2). Similarly, Streptomyces spp. endophytes are widely reported as phytopathogens biocontrol agents (Figures 1, 3). For example, K. nigriscans-endophytic Streptomyces sp. strain NRRL 30562 were recently reported to produce antibiotics as munumbicins A, B, C, and D, active against plant pathogenic bacteria and fungi (Castillo et al., 2002). Leguminose plants-endophytic Streptomyces caeruleatus was reported to be effective against the soybean pathogen X. campestris pv. glycine (Mingma et al., 2014; Figure 3B); whereas metabolites from A. indica- and Nothofagus spp.-endophytic actinomyctes inhibited the plant pathogenic fungi Mycosphaerella fijiensis, Sclerotinia sclerotiorum, and Rhizoctonia solani, and Pythium and Phytophthora species (Castillo et al., 2007; Verma et al., 2009; Figures 1, 3B). Indeed, Castillo et al. (2007) reported Streptomyces spp. endophytic of Nothofagus spp. in southern Patagonia, where the same strain characterized as Streptomyces seoulensis (based on molecular sequenciation and biological activity) was isolated from two different plants (strains coded C2 and C4, respectively); their antifungal activity was then proposed to elucidate the native plants survival mechanisms against plant pathogens within that area (Figures 1, 3B). Similarly, metabolites produced by roots’ endophytic actinomycetes previously described (Matsumoto and Takahashi, 2017), inhibited Kocuria rhizophila strain KB-212, Mucor racemosus strain KF-223, and Xanthomonas campestris pv. oryzae strain KB-88 growth (Figure 1 and Supplementary Table S2).
Zea mays seeds-endophytic B. amyloliquefaciens and B. subtilis were observed to inhibit F. moniliforme fungus growth by producing lipopeptides (Gond et al., 2015); similarly, Bruguiera gymnorrhiza (L.) Lam-endophytic B. amyloliquefaciens was shown to be antagonistic to various bacterial (Ralstonia solanacearum, P. syringae, and X. campestris), and fungal (Colletotrichum musae, F. oxysporum, Phytophthora capsici, and R. solani) pathogens of plants and to be effective in the biocontrol of Capsicum bacterial wilt in pot and field trials (Hu et al., 2010).
Oryza sativa-endophytic B. cereus and B. mojavensis were observed to exhibit antimicrobial activity against the fungal rice pathogens F. fujikuroi, F. proliferum, F. verticillioides, Magnaporthe grisea, and M. salvinii (Etesami and Alikhani, 2017; Supplementary Table S2).
Young radish-endophytic B. subtilis, Brachybacterium, and P. polymyxa were reported to possess antifungal activity against F. oxysporum, Pythium ultimum, Phytophthora capsici, and R. solani (Seo et al., 2010). An antifungal protein from the wheat-endophytic B. subtilis strain EDR4 inhibited B. cinerea, F. graminearum, F. oxysporum f.sp. vasinfectum, G. graminis var. tritici, Macrophoma kuwatsukai, and R. cerealis growth (Liu et al., 2010; Supplementary Table S2).
Metabolites From Gram-Positive Endophytes as Plant Growth-Promoters
Endophytic bacteria use to protect crops from microbial diseases is relevant, for their potential to promote host growth and antimicrobial activity (Safiyazov et al., 1995; Berg et al., 2005). Plant growth promotion (PGP) and, in most cases, abiotic stress tolerance and disease protection properties induction, are associated with endophytic bacteria potential to produce different compounds. Plants acclimate to environmental stresses by altering their physiology to be able to overcome stress factors such as dehydration, mechanical injury, nutrient deficiency, high solar radiation, or biotic/abiotic factors. It has been observed that plant inoculation with endophytic bacteria leads to accumulation of “protective” compounds, such as proline, carbohydrates, and antioxidants, in addition to antibiotics and fungal cell-wall lytic enzymes, which can inhibit growth of plant pathogens (Brader et al., 2014) or prime plant response to pathogens by induced systemic resistance (ISR) mechanisms (Pieterse et al., 2014).
Bacterial endophytes PGP potential is explained through several proposed mechanisms. Several of which help to increase accessibility to nutrients, e.g., nitrogen and phosphorus or metals, or produce metabolites that could regulate plant growth, development and defense responses, such as the well-known phytohormones abscisic acid, auxins, brassinosteroids, cytokinins, ethylene, gibberellins, jasmonates, and strigolactones (Reinhold-Hurek and Hurek, 2011; Brader et al., 2014; Santoyo et al., 2016; Shahzad et al., 2016; Figures 1–3).
Some examples of Gram-positive PGP bacterial endophytes are B. pumilus strain E2S2, whose treatment increased roots and shoots length and fresh and dry biomass, as compared with untreated sorghum plants, and helped to augment cadmium uptake (Luo et al., 2012). B. amyloliquefaciens strain NBRI-SN13 (SN13) isolated from an alkaline soil of Banthara, Lucknow, India, showed several PGP attributes and to induce solubilization of tricalcium phosphate more efficiently, when inoculated as endophyte (Nautiyal et al., 2013; Figure 2A). Plants treated with B. atrophaeus strain EY6, B. sphaericus GC subgroup B EY30, B. subtilis strain EY2, S. kloosii strain EY37 and K. erythromyxa strain EY43 as endophytes, have been shown to increase strawberry fruit growth and yield (Karlidag et al., 2011; Figure 2).
Interestingly, C. botulinum strain 2301 has been shown to have a significant PGP effect on clover in field experiments (Zeiller et al., 2015); whereas Exiguobacterium acetylicum 1P strain MTCC 8707, a cold tolerant bacterial strain from the Uttarakhand Himalayas, promotes wheat seedlings growth (Selvakumar et al., 2010; Supplementary Table S3).
Brevibacillus brevis strain SVC(II)14 exerted beneficial PGP on cotton crop (Nehra et al., 2016). Bacillus spp. strains CPMO6 and BM17, actinobacteria isolates ACT01 and ACT07, and lactic acid bacteria strain BL06 induce phosphate solubilization more efficiently when present as endophytes in citrus (Giassi et al., 2016).
In recent years, it has also been demonstrated that the entomopathogenic bacteria B. thuringiensis can have PGP attributes. Armada et al. (2016) tested an autochthonous isolate in interaction with native arbuscular mycorrhizal fungi (single or mixture) and found stimulating plant growth, nutrition and drought tolerance responses.
Siderophores production by endophytes improves plant growth by binding to available iron, competing for this element with phytopathogens and protecting the host plant from their infection (Figures 2, 3; Sabaté et al., 2018). B. subtilis strain B26 has been shown to induce drought tolerance in Brachypodium distachyon grass. This was correlated to augmentation of starch, fructose, glucose and total soluble carbohydrates content. However, increase of raffinose-related family carbohydrates (well-known stress response metabolites) was not observed in control and treated plants (Gagné-Bourque et al., 2015). A proline accumulation stimulating effect by endophytic strains of the actinobacteria Arthrobacter sp. and the Firmicutes Bacillus spp. were reported in pepper (Capsicum annuum L., Solanales: Solanaceae) plants in vitro, where their synthesis was related to osmotic stress responses (Sziderics et al., 2007). In addition, plants inoculated with bacterial endophytes, could tolerate abiotic stresses by increasing enzymatic activity. B. cereus strain CSR-B-1, B. marisflavi strain CSR-G-4, B. pumilus strain CSR-B-2, B. saffensis strain CSR-G-5, B. subtilis strain CSR-G-1, and B. thuringiensis strain CSRB-3, induced increment of superoxide dismutase, phenylalanine lyase, catalase, and peroxidase enzymes activity in gladiolus plants under sodium high concentration conditions (Damodaran et al., 2014; Supplementary Table S3).
Tolerance to low temperatures and growth promotion by endophytic activity has been reported as well. Verma et al. (2015), found Bacillus and Bacillus derived genera as wheat (Triticum aestivum) endophytes from the northern hills zone of India, among others. Phosphate and potassium are major essential macronutrients, but soluble phosphate and potassium concentrations in soil for plant intake are usually very low. Plants need zinc at low concentration since it is toxic at high concentration, thus zinc solubilization by endophytes dosifies the plant intake amount in response to plant and microbial nutritional requests. The most efficient phosphate solubilizing Gram-positive bacteria (PSB) belong to the genera Bacillus. Besides, it has been reported that B. amyloliquefasciens, B. megaterium, and Bacillus sp., exhibit phosphorus, potassium, and zinc solubilization (Figure 2; Verma et al., 2015).
Anticancer Activity of Gram-Positive Endophytic Bacteria
Cancer prevails as one of the leading causes of death worldwide, in spite of therapy advances (Global Cancer Observatory [GLOBOCAN], 2008; Chen et al., 2013). Conventional chemotherapy and radiotherapy have important disadvantages including drug resistance and serious side effects, which has prompted the search for new antitumor agents with high therapeutic efficacy and marginal or null detrimental effects.
Many endophytic actinomycete compounds were isolated and have found application not only as antimicrobial agents but also as cytotoxic agents against tumor cells (Figures 1, 3). Some members of the Gram-positive bacteria group have been recently found as endophytes in different plant species (Eljounaidi et al., 2016). Endophyte extracts have demonstrated to be a better choice versus chemotherapy agents due to their antitumor activity efficacy and lower side-effects, since they are less toxic to normal cells and more effective against several drug resistant microorganisms. As a consequence, the natural endophyte-derived metabolites have attracted peculiar attention with the purpose of being human cancer-chemopreventive compounds and anticancer chemotherapeutic drugs (Cardoso-Filho, 2018; Figures 3B,C). Endophytic Gram-positive bacterial natural products have emerged as one of the most reliable alternative treatment sources (Gutierrez et al., 2012; Chen et al., 2013), including antitumor agents such as anthracyclines, anthraquinones, aureolic acids, β-glucans, carzinophilin, coumarins, enediynes, flavonoids, glycopeptides, macrotetrolides, mitomycins, naphthoquinones, polysaccharides, and quinoxalines (Waring and Wakelin, 1974; Igarashi et al., 2007; Taechowisan et al., 2007; Chen et al., 2013; Cardoso-Filho, 2018; Figures 3B,C and Supplementary Table S4).
Actinomycetes, including the genera Actinomyces, Actinoplanes, Amycolatopsis, Micromonospora, Saccharopo-lyspora, and Streptomyces are recognized as producers of bioactive metabolites with not only antimicrobial, but also antitumor potential (Renu et al., 2008; Kekuda et al., 2010; Figure 3C and Supplementary Table S4). In this concern, Ophiopogon japonicus-endophytic B. amyloliquefaciens sp. exopolysaccharides were reported to possess antitumor activity against the human gastric carcinoma cell lines MC-4 and SGC-7901 (Chen et al., 2013; Figure 2A). Furthermore, Maytenus hookeri-maytansine-producing endophytic Streptomyces sp. strain Is9131 inhibited human SGC7901 gastric, HL60 leukemia, BEL7402 liver, and A-549 lung tumor cell lines growth (Lu and Shen, 2003; Zhao et al., 2005; Figure 3B and Supplementary Table S4). Alnus glutinosa-endophytic Streptomyces alnumycin was reported to inhibit the growth of K562 human leukemia cells (Bieber et al., 1998; Figure 3C), whereas Ricinus communis-endophytic salaceyins-producing Streptomyces laceyi strain MS53 was observed to be cytotoxic against the human breast cancer cell line SKBR3 (Kim et al., 2006; Figure 3B). In addition, herbaceous and arbor plants-pterocidin-producing endophytic Streptomyces hygroscopicus strain TP-A0451 was reported to inhibit human cancer cell lines NCI-H522, OVCAR-3, SF539, and LOX-IMVI growth (Igarashi et al., 2006; Qin et al., 2011), and Z. officinale 4-arylcoumarins-producing-endophytic Streptomyces aureofaciens strain CMUAc130 was shown to be cytotoxic against murine Lewis lung carcinoma (Taechowisan et al., 2007; Qin et al., 2011; Figure 3C and Supplementary Table S4).
Many plant growth promoter compounds have shown cytotoxicity against tumor cells. Nodules of Lupinus angustifolius-endophytic anthraquinones-producing Micromo-nospora sp. actinomycete significantly inhibited invasion of murine colon 26-L5 carcinoma cells (Igarashi et al., 2007; Qin et al., 2011). Recently, Taechowisan et al. (2017) reported anticancer activity of Boesenbergia rotunda-endophytic biphenyls-producing Streptomyces sp. strain BO-07 against human HepG2 and Huh7 liver, and HeLa cervical tumor cell lines (Figure 3B). Furthermore, Cinnamomum cassia-endophytic Streptomyces cavourensis strain YBQ59 was shown to inhibit human lung adenocarcinoma EGFR-TKI-resistant cells A549 and H1299 growth (Vu et al., 2018; Figure 3C and Supplementary Table S4).
Conclusion and Perspectives
Since the first reports of the industrial potential use of secondary metabolites produced by endophytes, there is more evidence that endophytic Gram-positive bacteria are one of the most important sources of novel compounds that have proven potential for either agriculture, medical and/or pharmaceutical application, thanks to their PGP, antimicrobial and anticancer activities. Indeed, endophytic Gram-positive bacteria help plants to better survive under biotic and abiotic stress conditions. It is not random that many endophytic Gram-positive have been isolated from medicinal plants from all over the world. In fact, many reports of endophytic Gram-positive bacteria showing such activities have been isolated from mangrove and under extreme environment conditions growing plants, like crops from salty soils and crops and trees from cold areas, where phylogenic analysis of native strains demonstrate they contain genes to produce different metabolites that, all together, are helping the host plant, not just to survive, but also to improve the plant adaptation to these extreme environmental conditions. In general, Bacillus class endophytes have been reported to produce aromatic compounds, lipopeptides, plant hormones, polysaccharides, and several enzymes, thus representing higher potential in agriculture for PGP and crop management strategies (Villarreal-Delgado et al., 2018). Similarly, Actinobacteria class endophytes are being found to produce antimicrobial- and antitumor-like activity metabolites, thus representing high potential for agriculture, medical, and veterinary application. In this minireview, we presented sources and specific isolated strains information, with the aim to provide current research highlights and perspectives for their future applications. These fascinating microorganisms are diverse and greatly adaptable to extreme stress conditions, making them excellent novel metabolites sources, whose application would be important for environmentally friendly and sustainable food and drugs production.
Author Contributions
All authors listed have made a substantial, direct and intellectual contribution to the work, and approved it for publication.
Funding
This work was supported by Consejo Nacional de Ciencia y Tecnología, Grant No. 5109 to PTG and by Laboratorio de Inmunología y Virología del Departamento de Microbiología e Inmunologia (LIV-DEMI) de la FCB-UANL.
Conflict of Interest Statement
The authors declare that the research was conducted in the absence of any commercial or financial relationships that could be construed as a potential conflict of interest.
Supplementary Material
The Supplementary Material for this article can be found online at: https://www.frontiersin.org/articles/10.3389/fmicb.2019.00463/full#supplementary-material
References
Aitken, G. (2004). A New Approach to Conservation: The Importance of the Individual through Wildlife Rehabilitation. London: Routledge.
Akinsanya, M. A., Goh, J. K., Lim, S. P., and Ting, A. S. Y. (2015). Diversity, antimicrobial and antioxidant activities of culturable bacterial endophyte communities in Aloe vera. FEMS Microbiol. Lett. 362:fnv184. doi: 10.1093/femsle/fnv184
Alaimo, S., Marceca, G. P., Giugno, R., Ferro, A., and Pulvirenti, A. (2018). Current knowledge and computational techniques for grapevine meta-omics analysis. Front. Plant Sci. 8:2241. doi: 10.3389/fpls.2017.02241
Aloo, B. N., Makumba, B. A., and Mbega, E. R. (2018). The potential of bacilli rhizobacteria for sustainable crop production and environmental sustainability. Microbiol. Res. 219, 26–39. doi: 10.1016/j.micres.2018.10.011
Armada, E., Probanza, A., Roldán, A., and Azcón, R. (2016). Native plant growth promoting bacteria Bacillus thuringiensis and mixed or individual mycorrhizal species improved drought tolerance and oxidative metabolism in Lavandula dentata plants. J. Plant Physiol. 192, 1–12. doi: 10.1016/j.jplph.2015.11.007
Baldan, E., Nigris, S., Populin, F., Zottini, M., Squartini, A., and Baldan, B. (2014). Identification of culturable bacterial endophyte community isolated from tissues of Vitis vinifera “Glera”. Plant Biosys. 148, 508–516. doi: 10.1080/11263504.2014.916364
Beck, H. C., Hansen, A. M., and Lauritsen, F. R. (2003). Novel pyrazine metabolites found in polymyxin biosynthesis by Paenibacillus polymyxa. FEMS Microbiol. Lett. 220, 67–73. doi: 10.1016/S0378-1097(03)00054-5
Beiranvand, M., Amin, M., Hashemi-Shahraki, A., Romani, B., Yaghoubi, S., and Sadeghi, P. (2017). Antimicrobial activity of endophytic bacterial populations isolated from medical plants of Iran. Iran. J. Microbiol. 9, 11–18.
Berg, G., Krechel, A., Ditz, M., Sikora, R. A., Ulrich, A., and Hallmann, J. (2005). Endophytic and ectophytic potato-associated bacterial communities differ in structure and antagonistic function against plant pathogenic fungi. FEMS Microbiol. Ecol. 51, 215–229. doi: 10.1016/j.femsec.2004.08.006
Bieber, B., Nüske, J., Ritzau, M., and Gräfe, U. (1998). Alnumycin a new naphthoquinone antibiotic produced by an endophytic Streptomyces sp. J. Antibiot. 51, 381–382. doi: 10.7164/antibiotics.51.381
Brader, G., Compant, S., Mitter, B., Trognitz, F., and Sessitsch, A. (2014). Metabolic potential of endophytic bacteria. Curr. Opin. Biotechnol. 27, 30–37. doi: 10.1016/j.copbio.2013.09.012
Cai, X. C., Liu, C. H., Wang, B. T., and Xue, Y. R. (2017). Genomic and metabolic traits endow bacillus velezensis CC09 with a potential biocontrol agent in control of wheat powdery mildew disease. Microbiol. Res. 196, 89–94. doi: 10.1016/j.micres.2016.12.007
Cankar, K., Kraigher, H., Ravnikar, M., and Rupnik, M. (2005). Bacterial endophytes from seeds of Norway spruce (Picea abies L. Karst). FEMS Microbiol. Lett. 244, 341–345. doi: 10.1016/j.femsle.2005.02.008
Cardoso-Filho, J. A. (2018). “Endophytic microbes as a novel source for producing anticancer compounds as multidrug resistance modulators,” in Anticancer Plants: Natural Products and Biotechnological Implements, eds M. Akhtar and M. Swamy (Singapore: Springer), 343–381.
Castillo, U., Harper, J. K., Strobel, G. A., Sears, J., Alesi, K., Ford, E., et al. (2003). Kakadumycins, novel antibiotics from Streptomyces sp. NRRL 30566, an endophyte of Grevillea pteridifolia. FEMS Microbiol. Lett. 224, 183–190. doi: 10.1016/S0378-1097(03)00426-9
Castillo, U. F., Browne, L., Strobel, G. A., Hess, W. M., Ezra, S., Pacheco, G., et al. (2007). Biologically active endophytic streptomycetes from Nothofagus spp. and other plants in Patagonia. Microb. Ecol. 53, 12–19. doi: 10.1007/s00248-006-9129-6
Castillo, U. F., Strobel, G. A., Ford, E. J., Hess, W. M., Porter, H., Jensen, J. B., et al. (2002). Munumbicins, wide-spectrum antibiotics produced by Streptomyces NRRL30562, endophytic on Kennedia nigriscans. Microbiology 148, 2675–2685. doi: 10.1099/00221287-148-9-2675
Castillo, U. F., Strobel, G. A., Mullenberg, K., Condron, M. M., Teplow, D. B., Folgiano, V., et al. (2006). Munumbicins E-4 and E-5: novel broad-spectrum antibiotics from Streptomyces NRRL 3052. FEMS Microbiol. Lett. 255, 296–300. doi: 10.1111/j.1574-6968.2005.00080.x
Castro, R. A., Quecine, M. C., Lacava, P. T., Batista, B. D., Luvizotto, D. M., Marcon, J., et al. (2014). Isolation and enzyme bioprospection of endophytic bacteria associated with plants of Brazilian mangrove ecosystem. SpringerPlus 3:382. doi: 10.1186/2193-1801-3-382
Cazorla, F. M., Romero, D., Pérez-García, A., Lugtenberg, B. J. J., Vicente, A. D., and Bloemberg, G. (2007). Isolation and characterization of antagonistic Bacillus subtilis strains from the avocado rhizoplane displaying biocontrol activity. J. Appl. Microbiol. 103, 1950–1959. doi: 10.1111/j.1365-2672.2007.03433.x
Chandrakar, S., and Gupta, A. K. (2017). Antibiotic potential of endophytic actinomycetes of medicinal herbs against human pathogenic bacteria. Proc. Natl. Acad. Sci. India Biol. Sci. 87, 905–915.
Chandrakar, S., and Gupta, A. K. (2018). Actinomycin-producing endophytic Streptomyces parvulus associated with root of aloe vera and optimization of conditions for antibiotic production. Probiotics Antimicrob. Prot. doi: 10.1007/s12602-018-9451-6 [Epub ahead of print].
Chatterjee, P., Samaddar, S., Niinemets,Ü., and Sa, T. M. (2018). Brevibacterium linens RS16 confers salt tolerance to Oryza sativa genotypes by regulating antioxidant defense and H+ ATPase activity. Microbiol. Res. 215, 89–101. doi: 10.1016/j.micres.2018.06.007
Chen, Y. T., Yuan, Q., Shan, L. T., Lin, M. A., Cheng, D. Q., and Li, C. Y. (2013). Antitumor activity of bacterial exopolysaccharides from the endophyte Bacillus amyloliquefaciens sp. Isolated from Ophiopogon japonicus. Oncol. Lett. 5, 1787–1792. doi: 10.3892/ol.2013.1284
Christina, A., Christapher, V., and Bhore, S. J. (2013). Endophytic bacteria as a source of novel antibiotics: an overview. Pharmacog. Rev. 7, 11–16. doi: 10.4103/0973-7847.112833
Compant, S., Mitter, B., Colli-Mull, J. G., Gangl, H., and Sessitsch, A. (2011). Endophytes of grapevine flowers, berries, and seeds: identification of cultivable bacteria, comparison with other plant parts, and visualization of niches of colonization. Microb. Ecol. 62, 188–197. doi: 10.1007/s00248-011-9883-y
Damodaran, T., Rai, R. B., Jha, S. K., Kannan, R., Pandey, B. K., Sah, V., et al. (2014). Rhizosphere and endophytic bacteria for induction of salt tolerance in gladiolus grown in sodic soils. J. Plant Interact. 9, 577–584. doi: 10.1080/17429145.2013.873958
de Lima Procópio, R. E., da Silva, I. R., Martins, M. K., de Azevedo, J. L., and de Araújo, J. M. (2012). Antibiotics produced by streptomyces. Braz. J. Infect. Dis. 16, 466–471. doi: 10.1016/j.bjid.2012.08.014
de Melo-Pereira, G. V., Magalhães, K. T., Lorenzetii, E. R., Souza, T. P., and Schwan, R. F. (2012). A multiphasic approach for the identification of endophytic bacterial in strawberry fruit and their potential for plant growth promotion. Microb. Ecol. 63, 405–417. doi: 10.1007/s00248-011-9919-3
Deng, Y., Zhu, Y., Wang, P., Zhu, L., Zheng, J., Li, R., et al. (2011). Complete genome sequence of Bacillus subtilis BSn5, an endophytic bacterium of Amorphophallus konjac with antimicrobial activity for the plant pathogen Erwinia carotovora subsp. Carotovora. J. Bacteriol. 193, 2070–2071. doi: 10.1128/JB.00129-11
Diale, M. O., Ubomba-Jaswa, E., and Serepa-Dlamini, M. H. (2018). The antibacterial activity of bacterial endophytes isolated from Combretum molle. Afr. J. Biotechnol. 17, 255–262. doi: 10.5897/AJB2017.16349
Ding, L., Maier, A., Fiebig, H. H., Lin, W. H., and Hertweck, C. (2011). A family of multicyclic indolosesquiterpenes from a bacterial endophyte. Organ. Biomol. Chem. 9, 4029–4031. doi: 10.1039/c1ob05283g
Ding, L., Münch, J., Goerls, H., Maier, A., Fiebig, H. H., Lin, W. H., et al. (2010). Xiamycin, a pentacyclic indolosesquiterpene with selective anti-HIV activity from a bacterial mangrove endophyte. Bioorg. Med. Chem. Lett. 20, 6685–6687. doi: 10.1016/j.bmcl.2010.09.010
El-Deeb, B., Fayez, K., and Gherbawy, Y. (2013). Isolation and characterization of endophytic bacteria from Plectranthus tenuiflorus medicinal plant in Saudi Arabia desert and their antimicrobial activities. J. Plant Interact. 8, 56–64. doi: 10.1080/17429145.2012.680077
Eljounaidi, K., Lee, S. K., and Bae, H. (2016). Bacterial endophytes as potential biocontrol agents of vascular wilt diseases – Review and future prospects. Biol. Control 103, 62–68. doi: 10.1016/j.biocontrol.2016.07.013
Etesami, H., and Alikhani, H. A. (2017). Evaluation of gram-positive rhizosphere and endophytic bacteria for biological control of fungal rice (Oryzia sativa L.) pathogens. Eur. J. Plant Pathol. 147, 7–14. doi: 10.1007/s10658-016-0981-z
Ezra, D., Castillo, U. F., Strobel, G. A., Hess, W. M., Porter, H., Jensen, J. B., et al. (2004). Coronamycins, peptide antibiotics produced by a verticillate Streptomyces sp. (MSU-2110) endophytic on Monstera sp. Microbiology 150, 785–793. doi: 10.1099/mic.0.26645-0
Ferrigo, D., Causin, R., and Raiola, A. (2017). Effect of potential biocontrol agents selected among grapevine endophytes and commercial products on crown gall disease. BioControl 62, 821–833. doi: 10.1007/s10526-017-9847-3
Fikri, A. S. I., Rahman, I. A., Nor, N. S. M., and Hamzah, A. (2018). “Isolation and identification of local bacteria endophyte and screening of its antimicrobial property against pathogenic bacteria and fungi,” in Proceedings of AIP Conference, Vol. 1940, (Melville, NY: AIP Publishing), 020072. doi: 10.1063/1.5027987
Frank, A., Saldierna-Guzmán, J., and Shay, J. (2017). Transmission of bacterial endophytes. Microorganisms 5:E70. doi: 10.3390/microorganisms5040070
Gagné-Bourque, F., Mayer, B. B. F., Charron, J.-B. B., Vali, H., Bertrand, A., and Jabaji, S. (2015). Accelerated growth rate and increased drought stress resilience of the model grass Brachypodium distachyon colonized by Bacillus subtilis B26. PLoS One 10:e130456. doi: 10.1371/journal.pone.0130456
Gamez, R., Cardinale, M., Montes, M., Ramirez, S., Schnell, S., and Rodriguez, F. (2019). Screening, plant growth promotion and root colonization pattern of two rhizobacteria (Pseudomonas fluorescens Ps006 and Bacillus amyloliquefaciens Bs006) on banana cv. Williams (Musa acuminata Colla). Microbiol. Res. 220, 12–20. doi: 10.1016/j.micres.2018.11.006
Gayathri, P., and Muralikrishnan, V. (2013). Isolation and characterization of endophytic actinomycetes from mangrove plant for antimicrobial activity. Int. J. Curr. Microbiol. Appl. Sci. 2, 78–89.
Ghadin, N., Zin, N. M., Sabaratnam, V., Badya, N., Basri, D. F., Lian, H. H., et al. (2008). Isolation and characterization of a novel endophytic Streptomyces SUK 06 with antimicrobial activity from Malaysian plant. Asian J. Plant Sci. 7, 189–194. doi: 10.3923/ajps.2008.189.194
Giassi, V., Kiritani, C., and Kupper, K. C. (2016). Bacteria as growth-promoting agents for citrus rootstocks. Microbiol. Res. 190, 46–54. doi: 10.1016/j.micres.2015.12.006
Global Cancer Observatory [GLOBOCAN] (2008). Section of Cancer Information WHO. Available at: http://www.who.int/mediacentre/factsheets/fs297/en/ [accessed August 05, 2018].
Gond, S. K., Bergen, M. S., and Torres, M. S. (2015). Endophytic bacillus spp. Produce antifungal lipopeptides and induce host defence gene expression in maize. Microbiol. Res. 172, 79–87. doi: 10.1016/j.micres.2014.11.004
Gowtham, H. G., Murali, M., Singh, S. B., Lakshmeesha, T. R., Murthy, K. N., Amruthesh, K. N., et al. (2018). Plant growth promoting rhizobacteria Bacillus amyloliquefaciens improves plant growth and induces resistance in chilli against anthracnose disease. Biol. Control 126, 209–217. doi: 10.1016/j.biocontrol.2018.05.022
Grady, E. N., MacDonald, J., Liu, L., Richman, A., and Yuan, Z. C. (2016). Current knowledge and perspectives of paenibacillus: a review. . Microb. Cell Fact 15:203. doi: 10.1186/s12934-016-0603-7
Guan, S. H., Sattler, I., Lin, W. H., Guo, D. A., and Grabley, S. (2005). p-Aminoacetophenonic acids produced by a mangrove endophyte: Streptomyces griseus subspecies. J. Nat. Prod. 68, 1198–1200. doi: 10.1021/np0500777
Gutierrez, R. M., Gonzalez, A. M., and Ramirez, A. M. (2012). Compounds derived from endophytes: a review of phytochemistry and pharmacology. Curr. Med. Chem. 19, 2992–3030. doi: 10.2174/092986712800672111
Haidar, R., Roudet, J., Bonnard, O., Dufour, M. C., Corio-Costet, M. F., Fert, M., et al. (2016). Screening and modes of action of antagonistic bacteria to control the fungal pathogen Phaeomoniella chlamydospora involved in grapevine trunk diseases. Microbiol. Res. 192, 172–184. doi: 10.1016/j.micres.2016.07.003
Housman, G., Byler, S., Heerboth, S., Lapinska, K., Longacre, M., Snyder, N., et al. (2014). Drug resistance in cancer: an overview. Cancers 6, 1769–1792. doi: 10.3390/cancers6031769
Hu, H. Q., Li, X. S., and He, H. (2010). Characterization of an antimicrobial material from a newly isolated Bacillus amyloliquefaciens from mangrove for biocontrol of Capsicum bacterial wilt. Biol. Control 54, 359–365. doi: 10.1016/j.biocontrol.2010.06.015
Igarashi, Y., Miura, S. S., Fujita, T., and Furumai, T. (2006). Pterocidin, a cytotoxic compound from the endophytic Streptomyces hygroscopicus. J. Antibiot. 59, 193–195. doi: 10.1038/ja.2006.28
Igarashi, Y., Trujillo, M. E., Martínez-Molina, E., Yanase, S., Miyanaga, S., Obata, T., et al. (2007). Antitumor anthraquinones from an endophytic actinomycete Micromonospora lupine sp, nov. Bioorg. Med. Chem. Lett. 17, 3702–3705. doi: 10.1016/j.bmcl.2007.04.039
Inahashi, Y., Iwatsuki, M., Ishiyama, A., Namatame, M., Nishihara-Tsukashima, A., Matsumoto, A., et al. (2011a). Spoxazomicins A-C, novel antitrypanosomal alkaloids produced by an endophytic actinomycete. Streptosporangium oxazolinicum K07-0460T. J. Antibiot. 64, 303–307. doi: 10.1038/ja.2011.16
Inahashi, Y., Matsumoto, A., Mura, S., and Takahashi, Y. (2011b). Streptosporangium oxazolinicum sp. nov., a novel endophytic actinomycete producing new antitrypanosomal antibiotics, spoxazomicins. J. Antibiot. 64, 297–302. doi: 10.1038/ja.2011.18
Indrawati, I., Rossiana, N., and Hidayat, T. R. (2018). “Antibacterial activity of bacterial endophytes from Kupa plant (Syzygium polycephalum Miq. (Merr & Perry) against pathogenic bacteria,” in Proceedings of the IOP Conference Series: Earth and Environmental Science, Vol. 166, (Bristol: IOP Publishing), 012013. doi: 10.1088/1755-1315/166/1/012013
Jakubiec-Krzesniak, K., Rajnisz-Mateusiak, A., Guspiel, A., Ziemska, J., and Solecka, J. (2018). Secondary metabolites of actinomycetes and their antibacterial, antifungal and antiviral properties. Pol. J. Microbiol. 67, 259–272. doi: 10.21307/pjm-2018-048
Jiang, Z., Tuo, L., Huang, D., Osterman, I. A., Tyurin, A., Liu, S., et al. (2018). Diversity, novelty and antimicrobial activity of endophytic actinobacteria from mangrove plants in Beilun estuary national nature reserve of Guangxi. China. Front. Microbiol. 9:868. doi: 10.3389/fmicb.2018.00868
Johnston-Monje, D., and Raizada, M. N. (2011). Conservation and diversity of seed associated endophytes in Zea across boundaries of evolution, ethnography and ecology. PLoS One 6:e20396. doi: 10.1371/journal.pone.0020396
Karlidag, H., Esitken, A., Yildirim, E., Donmez, M. F., and Turan, M. (2011). Effects of plant growth promoting bacteria on yield, growth, leaf water content, membrane permeability, and ionic composition of strawberry under saline conditions. J. Plant Nutr. 34, 34–45. doi: 10.1080/01904167.2011.531357
Katagiri, K., Yoshida, T., and Sato, K. (1975). “Quinoxaline antibiotics,” in Antibiotics Mechanism of Action of Antimicrobial and Antitumour Agents, eds J. W. Corcoran and F. E. Hahn (Heidelberg: Springer-Verlag), 234–251.
Kekuda, T. P., Shobha, K. S., and Onkarappa, R. (2010). Fascinating diversity and potent biological activities of actinomycete metabolites. J. Pharm. Res. 3, 250–256.
Khianngam, S., Techakriengkrai, T., Raksasiri, B. V., Kanjanamaneesathian, M., and Tanasupawat, S. (2013). “Isolation and screening of endophytic bacteria for hydrolytic enzymes from plant in mangrove forest at Pranburi, Prachuap Khiri Khan, Thailand,” in Proceedings of the 5th International Symposium on Plant Protection and Plant Health in Europe: Endophytes for plant protection: the state of the art, (Berlin: Humboldt University Berlin).
Kim, N., Shin, J. C., Kim, W., Hwang, B. Y., Kim, B. S., Hong, Y. S., et al. (2006). Cytotoxic 6-alkylsalicylic acids from the endophytic Streptomyces laceyi. J. Antibiot. 59, 797–800. doi: 10.1038/ja.2006.105
Koskimäki, J. J., Pirttilä, A. M., Ihantola, E. L., Halonen, O., and Frank, A. C. (2015). The intracellular scots pine shoot symbiont Methylobacterium extorquens DSM13060 aggregates around the host nucleus and encodes eukaryote-like proteins. mBio 6:e39–15. doi: 10.1128/mBio.00039-15
Li, H., Soares, M. A., Torres, M. S., and Bergen, M. (2015). Endophytic bacterium, Bacillus amyloliquefaciens, enhances ornamental hosta resistance to diseases and insect pests. J. Plant Interact. 10, 224–229. doi: 10.1080/17429145.2015.1056261
Liu, B., Huang, L., Buchenauer, H., and Kang, Z. (2010). Isolation and partial characterization of an antifungal protein from the endophytic Bacillus subtilis strain EDR4. Pesticide Biochem. Physiol. 98, 305–311. doi: 10.1016/j.pestbp.2010.07.001
Liu, Z., Wang, Y., Jia, X., and Lu, W. (2018). Isolation of secondary metabolites with antimicrobial activities from Bacillus amyloliquefaciens LWYZ003. Trans. Tianjin Univ. 25, 38–44. doi: 10.1016/j.jgar.2017.08.008
Lodewyckx, C., Vangronsveld, J., Porteous, F., Moore, E. R. B., Taghavi, S., Mezgeay, M., et al. (2002). Endophytic bacteria and their potential applications. Crit. Rev. Plant Sci. 21, 583–606. doi: 10.1080/0735-260291044377
Lòpez-Fernàndez, S., Compant, S., Vrhovsek, U., Bianchedi, P. L., Sessitsch, A., Pertot, I., et al. (2016). Grapevine colonization by endophytic bacteria shifts secondary metabolism and suggests activation of defense pathways. Plant Soil 405, 155–175. doi: 10.1007/s11104-015-2631-1
Lu, C., and Shen, Y. (2003). A new macrolide antibiotic with antitumor activity produced by Streptomyces sp. CS, a commensal microbe of Maytenus hookeri. J. Antibiot. 56, 415–418. doi: 10.7164/antibiotics.56.415
Luo, S., Xu, T., Chen, L., Chen, J., Rao, C., Xiao, X., et al. (2012). Endophyte-assisted promotion of biomass production and metal-uptake of energy crop sweet sorghum by plant-growth-promoting endophyte Bacillus sp. SLS18. Appl. Microbiol. Biotechnol. 93, 1745–1753. doi: 10.1007/s00253-011-3483-0
Manikprabhu, D., and Li, W. J. (2016). “Antimicrobial agents from actinomycetes Chemistry and Applications,” in Antimicrobials Synthetic and Natural Compounds, eds D. Dhanasekaran, N. Thajuddin, and A. Panneerselvam (Milton Park: Taylor & Francis Group), 99–115.
Matsumoto, A., and Takahashi, Y. (2017). Endophytic actinomycetes: promising source of novel bioactive compounds. J. Antibiot. 70, 514–519. doi: 10.1038/ja.2017.20
Mingma, R., Pathom-aree, W., Trakulnaleamsai, S., Thamchaipenet, A., and Duangmal, K. (2014). Isolation of rhizospheric and roots endophytic actinomycetes from leguminosae plant and their activities to inhibit soybean pathogen. Xanthomonas campestris pv. glycine. World J. Microbiol. Biotechnol. 30, 271–280. doi: 10.1007/s11274-013-1451-9
Mohamad, O. A., Li, L., Ma, J. B., Hatab, S., Xu, L., Guo, J. W., et al. (2018). Evaluation of the antimicrobial activity of endophytic bacterial populations from Chinese traditional medicinal plant licorice and characterization of the bioactive secondary metabolites produced by Bacillus atrophaeus against Verticillium dahliae. Front. Microbiol. 9:924. doi: 10.3389/fmicb.2018.00924
Nautiyal, C. S., Srivastava, S., Chauhan, P. S., Seem, K., Mishra, A., and Sopory, S. K. (2013). Plant growth-promoting bacteria Bacillus amyloliquefaciens NBRISN13 modulates gene expression profile of leaf and rhizosphere community in rice during salt stress. Plant Physiol. Biochem. 66, 1–9. doi: 10.1016/j.plaphy.2013.01.020
Nehra, V., Saharan, B. S., and Choudhary, M. (2016). Evaluation of Brevibacillus brevis as a potential plant growth promoting rhizobacteria for cotton (Gossypium hirsutum) crop. SpringerPlus 5:948. doi: 10.1186/s40064-016-2584-8
Ongena, M., and Jacques, P. (2008). Bacillus lipopeptides: versatile weapons for plant disease biocontrol. Trends Microbiol. 16, 115–125. doi: 10.1016/j.tim.2007.12.009
Pandey, P. K., Singh, S., Singh, M. C., Singh, A. K., Yadav, S. K., Pandey, A. K., et al. (2018). “Diversity, ecology, and conservation of fungal and bacterial endophytes,” in Microbial Resource Conservation, eds S. K. Sharma and A. Varma (New York, NY: Springer International Publishing), 393–430.
Paungfoo-Lonhienne, C., Rentsch, D., Robatzek, S., Webb, R. I., Sagulenko, E., Näsholm, T., et al. (2010). Turning the table: plants consume microbes as a source of nutrients. PLoS One 5:e11915. doi: 10.1371/journal.pone.0011915
Peña-Yam, L. P., Ruíz-Sánchez, E., Barboza-Corona, J. E., and Reyes-Ramírez, A. (2016). Isolation of mexican bacillus species and their effects in promoting growth of chili pepper (Capsicum annuum L. cv Jalapeño). Indian J. Microbiol. 56, 375–378. doi: 10.1007/s12088-016-0582-8
Pieterse, C. M. J., Zamioudis, C., Berendsen, R. L., Weller, D. M., Van Wees, S. C. M., and Bakker, P. A. H. M. (2014). Induced systemic resistance by beneficial microbes. Ann. Rev. Phytopathol. 52, 347–375. doi: 10.1146/annurev-phyto-082712-102340
Posada, L. F., Álvarez, J. C., Romero-Tabarez, M., de-Bashan, L., and Villegas-Escobar, V. (2018). Enhanced molecular visualization of root colonization and growth promotion by bacillus subtilis EA-CB0575 in different growth systems. Microbiol. Res. 217, 69–80. doi: 10.1016/j.micres.2018.08.017
Pratiwi, R. H., Hanafi, M., Artanti, N., and Pratiwi, R. D. (2018). Bioactivity of antibacterial compounds produced by endophytic actinomycetes from Neesia altissima. J. Trop. Life Sci. 8, 37–42. doi: 10.11594/jtls.08.01.07
Qin, S., Xing, K., Jiang, J. H., Xu, L. H., and Li, W. J. (2011). Biodiversity, bioactive natural products and biotechnological potential of plant-associated endophytic actinobacteria. Appl. Microbiol. Biotechnol. 89, 457–473. doi: 10.1007/s00253-010-2923-6
Raaijmakers, J. M., de Bruijn, I., Nybroe, O., and Ongena, M. (2010). Natural functions of lipopeptides from Bacillus and Pseudomonas: more than surfactants and antibiotics. FEMS Microbiol. Rev. 34, 1037–1062. doi: 10.1111/j.1574-6976.2010.00221.x
Rado, R., Andrianarisoa, B., Ravelomanantsoa, S., Rakotoarimanga, N., Rahetlah, V., Fienena, F. R., et al. (2015). Biocontrol of potato wilt by selective rhizospheric and endophytic bacteria associated with potato plant. Afr. J. Food Agric. Nutr. Dev. 15, 9762–9776.
Ramamoorthy, S., Gnanakan, A., Lakshmana, S. S., Meivelu, M., and Jeganathan, A. (2018). Structural characterization and anticancer activity of extracellular polysaccharides from ascidian symbiotic bacterium Bacillus thuringiensis. Carbohydrate Pol. 190, 113–120. doi: 10.1016/j.carbpol.2018.02.047
Reinhold-Hurek, B., and Hurek, T. (2011). Living inside plants: bacterial endophytes. Curr. Opin. Plant Biol. 14, 435–443. doi: 10.1016/j.pbi.2011.04.004
Renu, S., Monisha, K., and Lal, R. (2008). Bioactive compounds from marine actinomycetes. Indian J. Microb. 48, 410–431. doi: 10.1007/s12088-008-0052-z
Rosenblueth, M., and Martínez-Romero, E. (2006). Bacterial endophytes and their interactions with hosts. Mol. Plant Microbe Interact. 19, 827–837. doi: 10.1094/MPMI-19-0827
Ryan, R. P., Germaine, K., Franks, A., Ryan, D. J., and Dowling, D. N. (2008). Bacterial endophytes: recent developments and applications. FEMS Microbiol. Lett. 278, 1–9. doi: 10.1111/j.1574-6968.2007.00918.x
Sabaté, D. C., Brandan, C. P., Petroselli, G., Erra-Balsells, R., and Audisio, M. C. (2018). Biocontrol of Sclerotinia sclerotiorum (Lib.) de Bary on common bean by native lipopeptide-producer Bacillus strains. Microbiol. Res. 211, 21–30. doi: 10.1016/j.micres.2018.04.003
Safiyazov, J. S., Mannanov, R. N., and Sattarova, R. K. (1995). The use of bacterial antagonists for the control of cotton disease. Field Crops Res. 43, 51–54. doi: 10.1016/0378-4290(95)00038-R
Santoyo, G., Moreno-Hagelsieb, G., del Orozco-Mosqueda, M. C., and Glick, B. R. (2016). Plant growth-promoting bacterial endophytes. Microbiol. Res. 183, 92–99. doi: 10.1016/j.micres.2015.11.008
Schulz, B., and Boyle, C. (2006). What are endophytes? Soil Biol. 9, 1–14. doi: 10.1007/3-540-33526-9_1
Selvakumar, G., Kundu, S., Joshi, P., Nazim, S., Gupta, A. D., and Gupta, H. S. (2010). Growth promotion of wheat seedlings by exiguobacterium acetylicum 1P (MTCC 8707) a cold tolerant bacterial strain from the uttarakhand himalayas. Indian J. Microbiol. 50, 50–56. doi: 10.1007/s12088-009-0024-y
Seo, W. T., Lim, W. J., Kim, E. J., Yun, H. D., Lee, Y. H., and Cho, K. M. (2010). Endophytic bacterial diversity in the young radish and their antimicrobial activity against pathogens. J. Korean Soc. Appl. Biol. Chem. 53, 493–503. doi: 10.3839/jksabc.2010.075
Shahzad, R., Waqas, M., Khan, A. L., Asaf, S., Khan, M. A., Kang, S. M., et al. (2016). Seed-borne endophytic Bacillus amyloliquefaciens RWL-1 produces gibberellins and regulates endogenous phytohormones of Oryza sativa. Plant Physiol. Biochem. 106, 236–243. doi: 10.1016/j.plaphy.2016.05.006
Shimizu, M., Nakagawa, Y., Yukio, S. A. T. O., Furumai, T., Igarashi, Y., Onaka, H., et al. (2000). Studies on endophytic actinomycetes (I) Streptomyces sp. Isolated from rhododendron and its antifungal activity. J. Gen. Plant Pathol. 66, 360–366. doi: 10.1007/PL00012978
Stein, T. (2005). Bacillus subtilis antibiotics: structures, syntheses and specific functions. Mol. Microbiol. 56, 845–857. doi: 10.1111/j.1365-2958.2005.04587.x
Strobel, G. A., and Daisy, B. (2003). Bioprospecting for microbial endophytes and their natural products. Microbiol. Mol. Biol. Rev. 67, 491–502. doi: 10.1128/MMBR.67.4.491-502.2003
Sziderics, A. H., Rasche, F., Trognitz, F., Sessitsch, A., and Wilhelm, E. (2007). Bacterial endophytes contribute to abiotic stress adaptation in pepper plants (Capsicum annuum L.). Can. J. Microbiol. 53, 1195–1202. doi: 10.1139/W07-082
Taechowisan, T., Chaisaeng, S., and Phutdhawong, W. S. (2017). Antibacterial, antioxidant and anticancer activities of biphenyls from Streptomyces sp. BO-07: an endophyte in Boesenbergia rotunda (L.) Mansf A. Food Agric. Immunol. 28, 1330–1346. doi: 10.1080/09540105.2017.1339669
Taechowisan, T., Chanaphat, S., Ruensamran, W., and Phutdhawong, W. S. (2013). Antibacterial activity of decursin from streptomyces sp. GMT-8; an endophyte in zingiber officinale rosc. J. Appl. Pharm. Sci. 3, 74–78.
Taechowisan, T. C., Lu, C. H., Shen, Y. M., and Lumyong, S. (2007). Antitumor activity of 4-arylcoumarins from endophytic Streptomyces aureofaciens CMUAc130. J. Cancer Res. Ther. 3, 86–91. doi: 10.4103/0973-1482.34685
Tamosiune, I., Baniulis, D., and Stanys, V. (2017). “Role of endophytic bacteria in stress tolerance of agricultural plants: diversity of microorganisms and molecular mechanisms,” in Probiotics in Agroecosystem, eds V. Kumar, M. Kumar, S. Sharma, and R. Prasad (Singapore: Springer).
Tian, B., Zhang, C., Ye, Y., Wen, J., Wu, Y., Wang, H., et al. (2017). Beneficial traits of bacterial endophytes belonging to the core communities of the tomato root microbiome. Agric. Ecosys. Environ. 247, 149–156. doi: 10.1016/j.agee.2017.06.041
Tripathi, V. C., Satish, S., Horam, S., Raj, S., Arockiaraj, J., Pasupuleti, M., et al. (2018). Natural products from polar organisms: structural diversity, bioactivities and potential pharmaceutical applications. Polar Sci. 18, 147–166. doi: 10.1016/j.polar.2018.04.006
Tsurumaru, H., Okubo, T., Okazaki, K., Hashimoto, M., Kakizaki, K., Hanzawa, E., et al. (2015). Metagenomic analysis of the bacterial community associated with the taproot of sugar beet. Microbes Environ. 30, 63–69. doi: 10.1264/jsme2.ME14109
Verma, P., Yadav, A. N., Khannam, K. S., Panjiar, N., Kumar, S., Saxena, A. K., et al. (2015). Assessment of genetic diversity and plant growth promoting attributes of psychrotolerant bacteria allied with wheat (Triticum aestivum) from the northern hills zone of India. Ann. Microbiol. 65, 1885–1899. doi: 10.1007/s13213-014-1027-4
Verma, V. C., Gond, S. K., Kumar, A., Mishra, A., Kharwar, R. N., and Gange, A. C. (2009). Endophytic actinomycetes from Azadirachta indica A. Juss.: isolation, diversity, and anti-microbial activity. Microb. Ecol. 57, 749–756. doi: 10.1007/s00248-008-9450-3
Villarreal-Delgado, M. F., Villa-Rodríguez, E. D., Cira-Chávez, L. A., Estrada-Alvarado, M. I, Parra-Cota, F. I., and de los Santos-Villalobos, S. (2018). The genus bacillus as a biological control agent and its implications in the agricultural biosecurity. Mex. J. Phytopathol. 36, 95–130.
Vu, H. N. T., Nguyen, D. T., Nguyen, H. Q., Chu, H. H., Chu, S. K., Van Chau, M., et al. (2018). Antimicrobial and cytotoxic properties of bioactive metabolites produced by Streptomyces cavourensis YBQ59 isolated from cinnamomum cassia prels in yen bai province of vietnam. Curr. Microbiol. 75, 1247–1255. doi: 10.1007/s00284-018-1517-x
Waring, M. J., and Wakelin, L. P. (1974). Echinomycin: abifunctional intercalating antibiotic. Nature 252, 653–657. doi: 10.1038/252653a0
Wei, W., Zhou, Y., Chen, F., Yan, X., Lai, Y., Wei, C., et al. (2018). Isolation, diversity, and antimicrobial and immunomodulatory activities of endophytic actinobacteria from tea cultivars Zijuan and Yunkang-10 (Camellia sinensis var. assamica). Front. Microbiol. 9:1304. doi: 10.3389/fmicb.2018.01304
Xia, Y., DeBolt, S., Dreyer, J., Scott, D., and Williams, M. A. (2015). Characterization of culturable bacterial endophytes and their capacity to promote plant growth from plants grown using organic or conventional practices. Front. Plant Sci. 6:490. doi: 10.3389/fpls.2015.00490
Yuan, J., Wu, Y., Zhao, M., Wen, T., Huang, Q., and Shen, Q. (2018). Effect of phenolic acids from banana root exudates on root colonization and pathogen suppressive properties of Bacillus amyloliquefaciens NJN-6. Biol. Control 125, 131–137. doi: 10.1016/j.biocontrol.2018.05.016
Zeiller, M., Rothballer, M., Iwobi, A. N., Böhnel, H., Gessler, F., Hartmann, A., et al. (2015). Systemic colonization of clover (Trifolium repens) by clostridium botulinum strain 2301. Front. Microbiol. 6:1207. doi: 10.3389/fmicb.2015.01207
Zhao, K., Li, J., Zhang, X., Chen, Q., Liu, M., Ao, X., et al. (2018). Actinobacteria associated with Glycyrrhiza inflata Bat. Are diverse and have plant growth promoting and antimicrobial activity. Sci. Rep. 8:13661. doi: 10.1038/s41598-018-32097-8
Zhao, K., Penttinen, P., Guan, T., Xiao, J., Chen, Q., Xu, J., et al. (2011). The diversity and anti-microbial activity of endophytic actinomycetes isolated from medicinal plants in Panxi Plateau. Chin. Curr. Microbiol. 62, 182–190. doi: 10.1007/s00284-010-9685-3
Keywords: metabolites, amylases, chitinases, endoglucanases, esterases, proteases, plant hormones, toxins
Citation: Ek-Ramos MJ, Gomez-Flores R, Orozco-Flores AA, Rodríguez-Padilla C, González-Ochoa G and Tamez-Guerra P (2019) Bioactive Products From Plant-Endophytic Gram-Positive Bacteria. Front. Microbiol. 10:463. doi: 10.3389/fmicb.2019.00463
Received: 29 October 2018; Accepted: 21 February 2019;
Published: 29 March 2019.
Edited by:
José E. Barboza-Corona, Universidad de Guanajuato, MexicoReviewed by:
Ashutosh Sharma, Tecnologico de Monterrey, MexicoTapan Kumar Adhya, KIIT University, India
Copyright © 2019 Ek-Ramos, Gomez-Flores, Orozco-Flores, Rodríguez-Padilla, González-Ochoa and Tamez-Guerra. This is an open-access article distributed under the terms of the Creative Commons Attribution License (CC BY). The use, distribution or reproduction in other forums is permitted, provided the original author(s) and the copyright owner(s) are credited and that the original publication in this journal is cited, in accordance with accepted academic practice. No use, distribution or reproduction is permitted which does not comply with these terms.
*Correspondence: Patricia Tamez-Guerra, cGF0YW1lekBob3RtYWlsLmNvbQ==; cGF0cmljaWEudGFtZXpnckB1YW5sLmVkdS5teA==