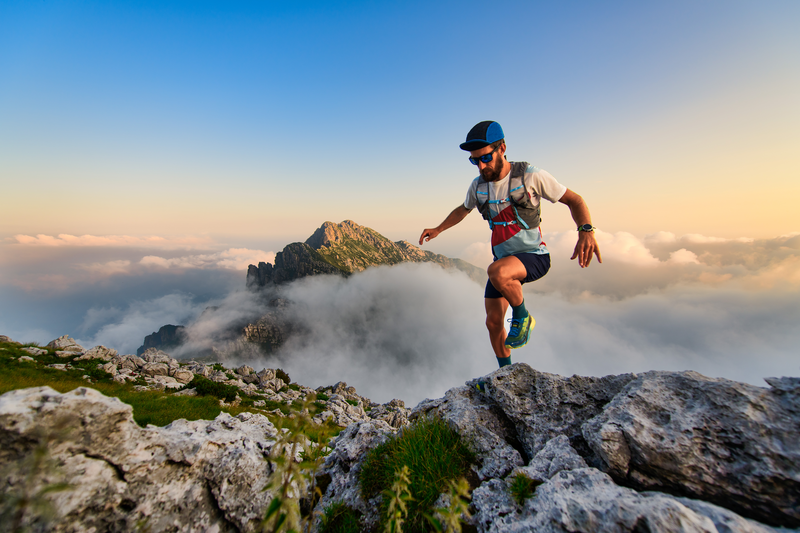
94% of researchers rate our articles as excellent or good
Learn more about the work of our research integrity team to safeguard the quality of each article we publish.
Find out more
ORIGINAL RESEARCH article
Front. Microbiol. , 05 March 2019
Sec. Virology
Volume 10 - 2019 | https://doi.org/10.3389/fmicb.2019.00420
Carbapenem-resistant Enterobacter aerogenes strains are a major clinical problem because of the lack of effective alternative antibiotics. However, viruses that lyze bacteria, called bacteriophages, have potential therapeutic applications in the control of antibiotic-resistant bacteria. In the present study, a lytic bacteriophage specific for E. aerogenes isolates, designated vB_EaeM_φEap-3, was characterized. Based on transmission electron microscopy analysis, phage vB_EaeM_φEap-3 was classified as a member of the family Myoviridae (order, Caudovirales). Host range determination revealed that vB_EaeM_φEap-3 lyzed 18 of the 28 E. aerogenes strains tested, while a one-step growth curve showed a short latent period and a moderate burst size. The stability of vB_EaeM_φEap-3 at various temperatures and pH levels was also examined. Genomic sequencing and bioinformatics analysis revealed that vB_EaeM_φEap-3 has a 175,814-bp double-stranded DNA genome that does not contain any genes considered undesirable for the development of therapeutics (e.g., antibiotic resistance genes, toxin-encoding genes, integrase). The phage genome contained 278 putative protein-coding genes and one tRNA gene, tRNA-Met (AUG). Phylogenetic analysis based on large terminase subunit and major capsid protein sequences suggested that vB_EaeM_φEap-3 belongs to novel genus “Kp15 virus” within the T4-like virus subfamily. Based on host range, genomic, and physiological parameters, we propose that phage vB_EaeM_φEap-3 is a suitable candidate for phage therapy applications.
Over the last three decades, Enterobacter aerogenes has increasingly been recognized as an important opportunistic and multidrug-resistant bacterial pathogen associated with nosocomial infections (Davin-Regli and Pages, 2015). The more frequent reports of carbapenem-resistant E. aerogenes are particularly concerning from a public health standpoint. Carbapenems are first-line drugs for the treatment of severe nosocomial infections caused by multidrug-resistant Enterobacteriaceae (Qin et al., 2014). Owing to the emergence of carbapenem-resistant strains, treatment options for patients suffering from E. aerogenes infection are limited, which can have serious consequences. As such, clinicians should be alert to carbapenem-resistant E. aerogenes infection to ensure the timely initiation of appropriate therapy (Kuai et al., 2014; Tuon et al., 2015).
Recently, there has been increased interest in the use of obligate lytic phages as a possible alternative or supplement to traditional antibiotics for the treatment of antibiotic-resistant pathogens (Lu and Koeris, 2011). The advantages of phage therapy over currently available antibiotics include rapid self-proliferation, minimal impact on normal flora, ability to control biofilms, and low intrinsic toxicity (Kim et al., 2015). Before clinical application, potential therapeutic phages must be comprehensively examined to ensure safety and efficacy (Lin et al., 2017; Philipson et al., 2018). As yet, E. aerogenes bacteriophages have not been extensively investigated. Currently, there are only four reported fully-sequenced E. aerogenes phages: F20 (JN672684; Mishra et al., 2012), vB_EaeM_φEap-2 (NC_028695; Li et al., 2016), vB_EaeM_φEap-1 (NC_028772), and UZ1 (unclassified; Verthe et al., 2004). F20 was classified as belonging to the Siphoviridae family of T1-like viruses (Mishra et al., 2012), vB_EaeM_φEap-2 also belongs to the family Siphoviridae and is related to Salmonella phage FSL SP-031 (KC139518; Li et al., 2016), and vB_EaeM_φEap-1 (NC_028772) is a member of the family Podoviridae. In the current study, we focused on E. aerogenes phage vB_EaeM_φEap-3, a T4-like bacteriophage belonging to the genus “Kp15 virus” within the family Myoviridae.
Enterobacter aerogenes clinical strain 3-SP is a generous gift from Dr. Dongsheng Zhou, State Key Laboratory of Pathogen and Biosecurity, Beijing Institute of Microbiology and Epidemiology, Beijing, China, which is isolated from a human case of pneumonia at a Chinese teaching hospital (Chen et al., 2015). The isolate was originally obtained as part of routine patient care. Approval was obtained for this original procedure. Informed Oral consent was obtained, and this was sufficient for the ethics committee. Approval was not needed for this retrospective study, as approval had been obtained for the original study. Strain 3-SP was used as a host for the isolation and proliferation of phage vB_EaeM_φEap-3 and contains a pNDM-BJ01-like conjugative plasmid named p3SP-NDM that confers carbapenem resistance (Chen et al., 2015).
Bacteriophage vB_EaeM_φEap-3 was isolated from a sewage wastewater sample from the Navy General Hospital, Beijing, China, using the double-layer overlay technique and E. aerogenes 3-SP as the indicator strain, as previously described (Wommack et al., 2009). Briefly, 0.22 μm filtrates of sewage samples were mixed with E. aerogenes 3-SP culture to enrich the phage at 37°C. The culture was centrifuged and the supernatant was filtered through a 0.22 μm pore-size membrane to remove the residual bacterial cells. Aliquots of the diluted filtrate were mixed with E. aerogenes culture; 3 mL of molten top soft nutrient agar (0.4% agar) was added and mixed, and overlaid on the solidified base nutrient agar (1.5% agar). Following incubation overnight at 37°C, clear phage plaques were picked from the plate. A pure phage suspension was obtained by three rounds of single-plaque purification and reinfection of the exponentially growing 3-SP strain, as reported previously (Kropinski et al., 2009b). Phage titers are expressed in plaque-forming units (PFUs)/mL and were measured using a soft agar overlay method (Kropinski et al., 2009a).
The phage particle preparation was centrifuged at 20,000 ×g for 2 h and the resulting pellet resuspended in SM buffer (10 mM Tris-HCl, pH 7.5; 100 mM NaCl; 10 mM MgSO4) to a concentration of ∼109 PFU/mL. Samples were processed by negative staining with 2% (wt/vol) uranyl acetate for 30 s and examined using a Tecnai Spirit 120-kV transmission electron microscope (FEI Company, Hillsboro, OR, United States) at different magnitudes to determine the phage morphology.
The host range of phage vB_EaeM_φEap-3 was determined via the spot test method using 28 E. aerogenes strains, 16 enterobacterial isolates (Enterobacter cloacae, n = 2; Enterobacter sakazakii, n = 2; Serratia marcescens, n = 5; Klebsiella pneumoniae, n = 1; Leclercia adecarboxylata, n = 1; Raoultella ornithinolytica, n = 1; Citrobacter freundii, n = 1; Shigella sonnei, n = 1; Vibrio parahaemolyticus, n = 1, and Escherichia coli, n = 1), three Gram-negative non-fermenters (Pseudomonas aeruginosa, n = 1; Acinetobacter baumannii, n = 1; and Achromobacter xylosoxidans, n = 1), and one Gram-positive bacterium (Stenotrophomonas maltophilia, n = 1). Each strain was grown in 5 mL of Luria–Bertani (LB) broth at 37°C to an optical density at 600 nm (OD600) of 0.5 before being centrifuged at 4000 × g for 5 min and resuspended in 3 mL of SM buffer. A 0.2-mL aliquot of bacterial suspension was then mixed with 3 mL of molten soft-agar and poured onto an LB agar plate. After the agar had solidified, 0.01 mL of phage suspension was spotted onto the overlay. Sensitivity of the bacterium to vB_EaeM_φEap-3 infection was assessed following overnight incubation as described previously (Kutter, 2009).
The latency period and burst size of vB_EaeM_φEap-3 were determined by monitoring dynamic changes in the number of phage particles during a replicative cycle. Briefly, host strain 3-SP was grown at 37°C to mid-exponential phase (OD600 = 0.4–0.5) before being centrifuged at 4000 ×g for 10 min at 4°C. The cell pellet was then resuspended in a 0.1-volume of SM buffer. A 0.1-mL aliquot of phage suspension was then added to 0.9 mL of the bacterial suspension to achieve a multiplicity of infection of 0.01. Phages were allowed to absorb for 5 min at 37°C, and then the mixture was centrifuged twice at 16,000 ×g for 2 min to remove the unabsorbed phages. The mixtures were then resuspended in 10 mL of LB broth and incubated at 37°C. Samples were collected at 10-min intervals for 80 min with or without 1% chloroform and immediately diluted and plated for phage titer assays (Pajunen et al., 2000). Results are reported as the average phage titer, while the burst size was calculated by dividing the average PFU/mL of the latent period by the average PFU/mL of the last three time points of the experiment (Buttimer et al., 2018). Results are the mean of three replicates ± standard deviation.
The stability of vB_EaeM_φEap-3 at different pH levels was evaluated by suspending phages at approximately 1.2 × 107 PFU/mL in 1 mL of SM buffer previously adjusted with 1 M NaOH or 1 M HCl to yield a pH range from 1.0–14.0. Phage preparations were incubated at room temperature for 60 min. The stability of vB_EaeM_φEap-3 at different temperatures was determined by incubating phage preparations (∼1.2 × 107 PFU/mL) at 4, 25, 37, 50, 60, 70, or 80°C for 15, 30, 45, or 60 min. After treatment, tubes were cooled and serial dilutions of each sample were tested against strain 3-SP in a double-layer agar assay to measure the lytic activity of the phage. Results are expressed as PFU/mL. Each assay was performed in triplicate and the results are the means of the three replicates.
Cell debris from 500 mL of E. aerogenes strain 3-SP culture infected with vB_EaeM_φEap-3 was collected by low-speed centrifugation (9000 ×g, 10 min, 4°C). Prior to DNA extraction, DNase (1 μg/mL) and RNase (1 μg/mL) were added to the phage lysate, which was then incubated at 37°C for 30 min. Following incubation, phage particles were precipitated with 1 M NaCl and 10% (w/v) polyethylene glycol (PEG) 8000 and incubated on ice for 1 h. The mixture was then centrifuged at 9000 ×g for 10 min at 4°C and the pellet was resuspended in 8 mL of SM buffer. An equal volume of chloroform was added to extract the PEG and cell debris, and then centrifuged at 4000 ×g for 15 min. The aqueous phase containing the bacteriophage particles was recovered and transferred to a new tube and DNA was extracted with phenol-chloroform (24:1, vol/vol) and precipitated with 100% ethanol. Finally, DNA samples were dissolved in 0.5 mL of sterile ddH2O and stored at 4°C.
The genome of vB_EaeM_φEap-3 was sequenced using the Illumina HiSeq 2500 system (Illumina, United States). The reads were assembled using SSAKE (v3.8) assembly software. The final assembled sequences were searched against the protein and nucleotide databases available via the National Center for Biotechnology Information website1 using Basic Local Alignment Search Tool (BLAST) software (Altschul et al., 1997). BLASTP2 analyses were used to identify putative homologies with predicted phage proteins. Potential open reading frames (ORFs) were identified using PHASTER3 (Arndt et al., 2016). The annotation was numbered with reference to the “Kp15 virus” genus. Potential tRNAs were identified using tRNAscan-SE Search Server4 (Lowe and Chan, 2016). Computer-based predictions were checked manually. Multiple sequence alignment of the chromosomes of related bacteriophages was carried out using Mauve software5 (Darling et al., 2004). Phylogenetic analyses were performed using the large subunit terminase or major capsid protein sequences of bacteriophages reported by the International Committee on Taxonomy of Viruses (ICTV)6. Analyses were conducted using the neighbor-joining method and 1000 bootstrap replicates by ClustalW. A genome map was generated using the CLC Main Workbench, version 6.1.1 (CLC bio, Aarhus, Denmark).
NDM-1 carbapenemase-producing E. aerogenes strain 3-SP, originally isolated from a human case of pneumonia at a Chinese teaching hospital (Chen et al., 2015), was used as a host to investigate the presence of phages in a wastewater sample from the Navy General Hospital in Beijing. Double-layer overlay plates resulted in a significant number of small plaques (diameter < 1 mm) with a similar morphology, indicating the presence of a single lytic phage (Figure 1). A single plaque was selected and used for phage proliferation and purification. Using TEM, the morphology of the phage was determined. The head of the phage is prolate, with two icosahedral ends and a cylindrical mid-section measuring ∼115 nm. The head is connected by a neck with an apparent collar to a tail tube (∼110 nm long) surrounded by a contractile sheath, a baseplate, and a complex system of tail fibers and spikes (Figure 2). On the basis of morphology and according to Ackermann’s classification (Ackermann, 2009a,b), the phage was classified as belonging to the family Myoviridae, which comprises a quarter of tailed bacteriophages and includes the E. coli phage T4. The phage was named vB_EaeM_φEap-3 according to the proposed naming system, where vB = bacterial virus; Eae = abbreviation of the host species; M = myovirus; φEap-3 = name of phage (Kropinski et al., 2009b; Adriaenssens and Brister, 2017).
Figure 2. Morphology of phage vB_EaeM_φEap-3. Transmission electron micrograph of negatively stained phage vB_EaeM_φEap-3 at × 150,000 magnification. The bar indicates 100 nm.
A total of 48 clinical isolates (28 E. aerogenes, 19 non-E. aerogenes Gram-negative bacteria, and one Gram-positive bacterium) were used to evaluate the host range of vB_EaeM_φEap-3 (Table 1). Results demonstrated that vB_EaeM_φEap-3 had lytic activity specific to E. aerogenes strains (n = 18), with none of the other strains susceptible to infection. vB_EaeM_φEap-3 has a broader host range than the previous reported Enterobacter phage vB_EaeM_φEap-2 (Li et al., 2016, 2017).
Results from one-step growth experiments showed that vB_EaeM_φEap-3 was characterized by a relatively short latent period (approximately 10 min), followed by a rise period of 20 min. A growth plateau was reached within 40 min (Figure 3). The burst size of vB_EaeM_φEap-3 was calculated to be approximately 109 phage particles per infected bacterial cell.
Figure 3. One-step growth curve of phage vB_EaeM_φEap-3. Phage vB_EaeM_φEap-3 was grown in an exponential phase culture of Enterobacter aerogenes strain 3-SP. Data points indicate the PFU/mL at different time points. Each data point represents the mean of three independent experiments.
Results obtained from temperature stability assays demonstrated that vB_EaeM_φEap-3 remained stable at temperatures ranging from 4–37°C. Decreases in infectivity were observed following incubation at 60 or 70°C for 15 min, while the phage was completely inactivated by incubation at 50°C for 60 min or 80°C for 15 min (Figure 4). Results of pH stability testing revealed that phage viability was mainly unaffected following incubation in buffer at pH values ranging from 6–7, while reductions of approximately 30 and 60% were detected at pH 3 and pH 11. vB_EaeM_φEap-3 was completely inactivated at pH 1–2 and pH 12–14 (Figure 5).
Figure 4. pH stability of phage vB_EaeM_φEap-3. Each data point represents the mean of three independent experiments. Standard deviations are shown as vertical lines. Results are expressed as PFU/mL.
Figure 5. Thermal stability of phage vB_EaeM_φEap-3. Each data point represents the mean of three independent experiments. Results were expressed as PFU/mL.
The genome of vB_EaeM_φEap-3 is composed of a double-stranded DNA molecule of 175,814 bp with a GC content of 42%. A total of 278 putative coding sequences (CDSs) were detected (Supplementary Table S1). A tRNA gene, tRNA-Met (AUG), was detected. Approximately half of the predicted CDSs (n = 149, 53.6%) were present on the same strand. The shortest CDS encodes a putative protein of 26 amino acid residues (orf223), while the longest encodes a putative protein of 1394 residues (orf266). A specific function (e.g., DNA metabolism, structural proteins, enzymes involved in cell lysis) could be assigned to 113 of the 278 predicted proteins (40.6%), with all sequences showing high identity to proteins from phages belonging to the “Kp15 virus” genus of the Tevenvirinae subfamily. No specific function was assigned to the remaining 165 CDSs (59.4%; Table 2). No sequences with significant similarity to known antibiotic resistance, virulence, or toxin proteins, or to elements associated with lysogeny (i.e., integrase), were identified. The presence of the ndd gene (orf276), the deduced amino acid sequence of which shared 100% identity with the nucleoid disruption protein of Klebsiella phage KP15, suggested a lytic lifestyle for vB_EaeM_φEap-3 (Kesik-Szeloch et al., 2013). Comparative analysis of the whole genome sequence of vB_EaeM_φEap-3 against those of phages retrieved from the NCBI databases revealed that vB_EaeM_φEap-3 is most closely related to coliphages RB16 (NC_014467; Petrov et al., 2006) and RB43 (NC_007023; Petrov et al., 2006), Klebsiella phages KP15 (GU295964; Kesik-Szeloch et al., 2013), KP27 (HQ918180; Kesik-Szeloch et al., 2013), Matisse (KT001918; Provasek et al., 2015), and Miro (KT001919; Mijalis et al., 2015), Cronobacter phage vB_CsaM_GAP161 (JN882287; Abbasifar et al., 2012), and members of the myoviral subfamily Tevenvirinae, belonging to a genus of T4-like viruses (Adriaenssens and Brister, 2017; Figure 6). A large percentage of the putative proteins that could be assigned a metabolic function were devoted to DNA metabolism and, replication (Figure 7). Seven proteins making up the basic replisome, which acts as a biological machine that can move the replication fork through model templates at in vivo speeds (Miller et al., 2003), were also identified in the genome of vB_EaeM_φEap-3 (Table 2). The vB_EaeM_φEap-3 genome-packaging proteins showed a high degree of similarity to those of KP15 and KP27 viruses. Phage structural proteins identified in vB_EaeM_φEap-3 genome included head proteins, whisker/neck proteins, tail proteins, baseplate proteins, and tail fiber proteins (Table 2). As a member of the “Kp15 virus” genus, the lysis system of vB_EaeM_φEap-3 is composed of four proteins (endolysin, holin, antiholin, and spanin; Table 2). vB_EaeM_φEap-3 holin (orf268), with one transmembrane domain, was identified as a class III holin, and belongs to the holin T superfamily group (Maciejewska et al., 2017). The lysis genes of vB_EaeM_φEap-3 show the same organization and >99% predicted amino acid sequence similarity to the same regions of phages KP15 and KP27 (Maciejewska et al., 2017).
Figure 6. Multiple alignment of the chromosomes of phage vB_EaeM_φEap-3, coliphages RB16 and RB43, Klebsiella phages KP15 and KP27 obtained using Mauve software http://asap.ahabs.wisc.edu/mauve/. The height of the similarity profile corresponds to the average level of conservation in that region of the genome sequence. Completely white regions represent fragments that are not aligned or contain a particular genome-specific sequence element.
Figure 7. Genome map of phage vB_EaeM_φEap-3. The genome map was generated using the CLC Main Workbench, version 6.1.1 (CLC bio, Aarhus, Denmark). Predicted open reading frames are indicated by arrows, with the direction of the arrows representing the direction of transcription.
A phylogenetic tree based on the predicted large terminase subunit amino acid sequences revealed that vB_EaeM_φEap-3 belongs to the genus “Kp15 virus” of the subfamily Tevenvirinae, family Myoviridae (Figure 8A). This classification was confirmed based on the analysis of the major capsid proteins (Figure 8B).
Figure 8. Phylogenetic trees generated based on the (A) large terminase subunit and (B) major capsid proteins of vB_EaeM_φEap-3 and homolog proteins from other members of the Tevenvirinae subfamily. Amino acid sequences were compared using ClustalW, and phylogenetic trees were generated using the neighbor-joining method.
The spread of antibiotic-resistance among bacterial pathogens poses a serious problem in a clinical setting because of the lack of available treatment options. In particular, carbapenem resistance and its growing association with a multidrug-resistant phenotype in Enterobacteriaceae, including E. aerogenes, has become a major clinical challenge. As an important opportunistic pathogen, E. aerogenes can cause nosocomial outbreaks and invasive infections such as septicemia (Kuai et al., 2014). In this work, we describe a lytic bacteriophage characterized by its specific lytic activity toward E. aerogenes. Morphological characterization performed by TEM showed that vB_EaeM_φEap-3 is a member of the family Myoviridae, while phylogenetic analysis using previously verified markers (Ackermann et al., 2011; Cheepudom et al., 2015) suggested that it belongs to the novel genus “Kp15 virus” of the Tevenvirinae subfamily. Physiological characterization showed that vB_EaeM_φEap-3 is characterized by a relatively short latent period and a burst size of 109 phage particles per infected bacterial cell. Results of temperature and pH stability testing also expand our knowledge of this novel phage. These features, together with the host specificity, the close genetic relatedness to the strictly lytic genus “Kp15 virus” phages, and the absence of genes associated with lysogeny, make vB_EaeM_φEap-3 an excellent candidate for potential clinical applications, such as decontamination or therapy. Finally, the results confirmed that vB_EaeM_φEap-3 is a promising candidate to hinder the colonization of E. aerogenes.
The complete genome sequence of phage vB_EaeM_φEap-3 is available in GenBank under accession number KT321315.
JZ and ZZ did the experiments and contributed equally to this study as joint first authors. CT, XC, LH, XW, HL, WL, and AJ analyzed the data. RF, ZY, and JY provided the bacterial strains. XZ managed the project and designed the experiments. JZ and XZ wrote the article.
This work received support from the National Natural Science Foundation of China (Grant No. 31670174).
The authors declare that the research was conducted in the absence of any commercial or financial relationships that could be construed as a potential conflict of interest.
We would like to thank the Center for Biological Imaging (CBI), Institute of Biophysics, Chinese Academy of Sciences for our electron microscopy work and we would be grateful to Deyin Fan for his help of making EM samples. We also thank Tamsin Sheen, Ph.D., from Liwen Bianji, Edanz Editing China7, for editing the English text of a draft of this manuscript.
The Supplementary Material for this article can be found online at: https://www.frontiersin.org/articles/10.3389/fmicb.2019.00420/full#supplementary-material
Abbasifar, R., Kropinski, A. M., Sabour, P. M., Ackermann, H. W., Lingohr, E. J., and Griffiths, M. W. (2012). Complete genome sequence of Cronobacter sakazakii bacteriophage vB_CsaM_GAP161. J. Virol. 86, 13806–13807. doi: 10.1128/JVI.02546-12
Ackermann, H. W. (2009b). Phage Classification and Characterization. Methods Mol. Biol. 501, 127–140.
Ackermann, H. W., Krisch, H. M., and Comeau, A. M. (2011). Morphology and genome sequence of phage varphi1402: a dwarf myovirus of the predatory bacterium Bdellovibrio bacteriovorus. Bacteriophage 1,138–142.
Adriaenssens, E., and Brister, J. R. (2017). How to name and classify your phage: an informal guide. Viruses 9:E70. doi: 10.3390/v9040070
Altschul, S. F., Madden, T. L., Schaffer, A. A., Zhang, J., Zhang, Z., Miller, W., et al. (1997). Gapped BLAST and PSI-BLAST: a new generation of protein database search programs. Nucleic Acids Res. 25, 3389–3402.
Arndt, D., Grant, J. R., Marcu, A., Sajed, T., Pon, A., Liang, Y., et al. (2016). PHASTER: a better, faster version of the PHAST phage search tool. Nucleic Acids Res. 44, W16–W21. doi: 10.1093/nar/gkw387
Buttimer, C., Lucid, A., Neve, H., Franz, C., O’mahony, J., Turner, D., et al. (2018). Pectobacterium atrosepticum Phage vB_PatP_CB5: a Member of the Proposed Genus ’Phimunavirus’. Viruses 10:E394. doi: 10.3390/v10080394
Cheepudom, J., Lee, C. C., Cai, B., and Meng, M. (2015). Isolation, characterization, and complete genome analysis of P1312, a thermostable bacteriophage that infects Thermobifida fusca. Front. Microbiol. 6:959. doi: 10.3389/fmicb.2015.00959
Chen, Z., Li, H., Feng, J., Li, Y., Chen, X., Guo, X., et al. (2015). NDM-1 encoded by a pNDM-BJ01-like plasmid p3SP-NDM in clinical Enterobacter aerogenes. Front. Microbiol. 6:294. doi: 10.3389/fmicb.2015.00294
Darling, A. C., Mau, B., Blattner, F. R., and Perna, N. T. (2004). Mauve: multiple alignment of conserved genomic sequence with rearrangements. Genome Res. 14, 1394–1403.
Davin-Regli, A., and Pages, J. M. (2015). Enterobacter aerogenes and Enterobacter cloacae; versatile bacterial pathogens confronting antibiotic treatment. Front. Microbiol. 6:392. doi: 10.3389/fmicb.2015.00392
Kesik-Szeloch, A., Drulis-Kawa, Z., Weber-Dabrowska, B., Kassner, J., Majkowska-Skrobek, G., Augustyniak, D., et al. (2013). Characterising the biology of novel lytic bacteriophages infecting multidrug resistant Klebsiella pneumoniae. Virol. J. 10:100. doi: 10.1186/1743-422X-10-100
Kim, M. S., Kim, Y. D., Hong, S. S., Park, K., Ko, K. S., and Myung, H. (2015). Phage-encoded colanic acid-degrading enzyme permits lytic phage infection of a capsule-forming resistant mutant Escherichia coli strain. Appl. Environ. Microbiol. 81, 900–909. doi: 10.1128/AEM.02606-14
Kropinski, A. M., Mazzocco, A., Waddell, T. E., Lingohr, E., and Johnson, R. P. (2009a). Enumeration of bacteriophages by double agar overlay plaque assay. Methods Mol. Biol. 501, 69–76. doi: 10.1007/978-1-60327-164-6_7
Kropinski, A. M., Prangishvili, D., and Lavigne, R. (2009b). Position paper: the creation of a rational scheme for the nomenclature of viruses of Bacteria and Archaea. Environ. Microbiol. 11, 2775–2777.
Kuai, S., Shao, H., Huang, L., Pei, H., Lu, Z., Wang, W., et al. (2014). KPC-2 carbapenemase and DHA-1 AmpC determinants carried on the same plasmid in Enterobacter aerogenes. J. Med. Microbiol. 63, 367–370. doi: 10.1099/jmm.0.054627-0
Li, E., Wei, X., Ma, Y., Yin, Z., Li, H., Lin, W., et al. (2016). Isolation and characterization of a bacteriophage phiEap-2 infecting multidrug resistant Enterobacter aerogenes. Sci. Rep. 6:28338. doi: 10.1038/srep28338
Li, E., Wei, X., Ma, Y., Yin, Z., Li, H., Lin, W., et al. (2017). Corrigendum: isolation and characterization of a bacteriophage phiEap-2 infecting multidrug resistant Enterobacter aerogenes. Sci. Rep. 7:46805.
Lin, D. M., Koskella, B., and Lin, H. C. (2017). Phage therapy: an alternative to antibiotics in the age of multi-drug resistance. World J. Gastrointest Pharmacol. Ther. 8, 162–173. doi: 10.4292/wjgpt.v8.i3.162
Lowe, T. M., and Chan, P. P. (2016). tRNAscan-SE On-line: integrating search and context for analysis of transfer RNA genes. Nucleic Acids Res. 44, W54–W57. doi: 10.1093/nar/gkw413
Lu, T. K., and Koeris, M. S. (2011). The next generation of bacteriophage therapy. Curr. Opin. Microbiol. 14, 524–531. doi: 10.1016/j.mib.2011.07.028
Maciejewska, B., Roszniowski, B., Espaillat, A., Kesik-Szeloch, A., Majkowska-Skrobek, G., Kropinski, A. M., et al. (2017). Klebsiella phages representing a novel clade of viruses with an unknown DNA modification and biotechnologically interesting enzymes. Appl. Microbiol. Biotechnol. 101, 673–684. doi: 10.1007/s00253-016-7928-3
Mijalis, E. M., Lessor, L. E., Cahill, J. L., Rasche, E. S., and Kuty Everett, G. F. (2015). Complete Genome Sequence of Klebsiella pneumoniae Carbapenemase-Producing K. pneumoniae Myophage Miro. Genome Announc. 3:e994-15. doi: 10.1128/genomeA.01137-15
Miller, E. S., Kutter, E., Mosig, G., Arisaka, F., Kunisawa, T., and Ruger, W. (2003). Bacteriophage T4 genome. Microbiol. Mol. Biol. Rev. 67, 86–156.
Mishra, C. K., Choi, T. J., and Kang, S. C. (2012). Isolation and characterization of a bacteriophage F20 virulent to Enterobacter aerogenes. J. Gen. Virol. 93, 2310–2314. doi: 10.1099/vir.0.043562-0
Pajunen, M., Kiljunen, S., and Skurnik, M. (2000). Bacteriophage phiYeO3-12, specific for Yersinia enterocolitica serotype O:3, is related to coliphages T3 and T7. J. Bacteriol. 182, 5114–5120.
Petrov, V. M., Nolan, J. M., Bertrand, C., Levy, D., Desplats, C., Krisch, H. M., et al. (2006). Plasticity of the gene functions for DNA replication in the T4-like phages. J. Mol. Biol. 361, 46–68.
Philipson, C. W., Voegtly, L. J., Lueder, M. R., Long, K. A., Rice, G. K., Frey, K. G., et al. (2018). Characterizing phage genomes for therapeutic applications. Viruses 10:E188. doi: 10.3390/v10040188
Provasek, V. E., Lessor, L. E., Cahill, J. L., Rasche, E. S., and Kuty Everett, G. F. (2015). Complete genome sequence of carbapenemase-producing Klebsiella pneumoniae myophage matisse. Genome Announc. 3, e1136-15. doi: 10.1128/genomeA.01136-15
Qin, X., Yang, Y., Hu, F., and Zhu, D. (2014). Hospital clonal dissemination of Enterobacter aerogenes producing carbapenemase KPC-2 in a Chinese teaching hospital. J. Med. Microbiol. 63, 222–228. doi: 10.1099/jmm.0.064865-0
Tuon, F. F., Scharf, C., Rocha, J. L., Cieslinsk, J., Becker, G. N., and Arend, L. N. (2015). KPC-producing Enterobacter aerogenes infection. Braz. J. Infect. Dis. 19, 324–327. doi: 10.1016/j.bjid.2015.01.003
Verthe, K., Possemiers, S., Boon, N., Vaneechoutte, M., and Verstraete, W. (2004). Stability and activity of an Enterobacter aerogenes-specific bacteriophage under simulated gastro-intestinal conditions. Appl. Microbiol. Biotechnol. 65, 465–472. doi: 10.1007/s00253-004-1585-7
Keywords: E. aerogenes, bacteriophage, vB_EaeM_φEap-3, genome sequencing, Myoviridae
Citation: Zhao J, Zhang Z, Tian C, Chen X, Hu L, Wei X, Li H, Lin W, Jiang A, Feng R, Yuan J, Yin Z and Zhao X (2019) Characterizing the Biology of Lytic Bacteriophage vB_EaeM_φEap-3 Infecting Multidrug-Resistant Enterobacter aerogenes. Front. Microbiol. 10:420. doi: 10.3389/fmicb.2019.00420
Received: 07 November 2018; Accepted: 18 February 2019;
Published: 05 March 2019.
Edited by:
Robert Czajkowski, University of Gdańsk, PolandReviewed by:
Alexander P. Hynes, McMaster University, CanadaCopyright © 2019 Zhao, Zhang, Tian, Chen, Hu, Wei, Li, Lin, Jiang, Feng, Yuan, Yin and Zhao. This is an open-access article distributed under the terms of the Creative Commons Attribution License (CC BY). The use, distribution or reproduction in other forums is permitted, provided the original author(s) and the copyright owner(s) are credited and that the original publication in this journal is cited, in accordance with accepted academic practice. No use, distribution or reproduction is permitted which does not comply with these terms.
*Correspondence: Zhe Yin, amVycnk5eWluQDE2My5jb20= Xiangna Zhao, eG5hemhhb0AxNjMuY29t
†These authors have contributed equally to this work as joint first authors
Disclaimer: All claims expressed in this article are solely those of the authors and do not necessarily represent those of their affiliated organizations, or those of the publisher, the editors and the reviewers. Any product that may be evaluated in this article or claim that may be made by its manufacturer is not guaranteed or endorsed by the publisher.
Research integrity at Frontiers
Learn more about the work of our research integrity team to safeguard the quality of each article we publish.