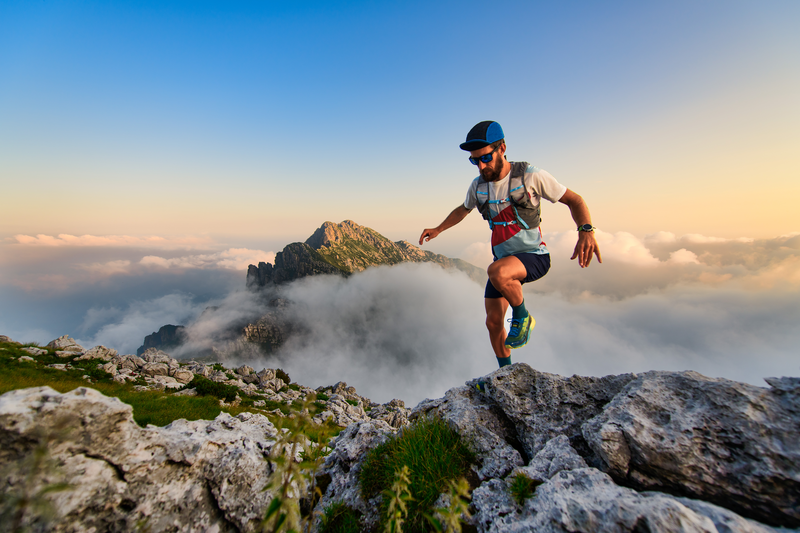
95% of researchers rate our articles as excellent or good
Learn more about the work of our research integrity team to safeguard the quality of each article we publish.
Find out more
REVIEW article
Front. Microbiol. , 09 January 2019
Sec. Fungi and Their Interactions
Volume 9 - 2018 | https://doi.org/10.3389/fmicb.2018.03273
This article is part of the Research Topic Diagnostic Approaches for Aspergillus Infections View all 20 articles
As the number of lung transplants performed worldwide each year continues to grow, the success of this procedure is threatened by the incidence of non-CMV infections such as invasive aspergillosis. Despite tremendous efforts and the availability of numerous diagnostic tests (especially in hematological malignancies) the diagnosis of invasive aspergillosis continues to be a challenge. Lung transplantation remains a unique clinical scenario, where additional host defenses are immunocompromized, making many of the available tests unsuitable. In this review we will navigate through the myriad of diagnostic tests currently available and how they apply to this unique patient population, as well as have a look into what the future holds.
Lung transplantation is an established modality for end-stage pulmonary disease. With over 4600 lung transplants performed in 2016 worldwide (Lund et al., 2017) the number of these procedures carried out annually continues to grow. One of the greatest challenges for the survival of these patients during the first year after transplant are non-CMV infections, especially invasive pulmonary aspergillosis (IPA), which portends a higher mortality than bacterial or viral infections (Mattner et al., 2007).
Several strategies are being used to prevent the development of IPA including universal and pre-emptive prophylaxis (Husain et al., 2016). However, the diagnosis of IPA in lung transplantation remains challenging. Aspergillus is a ubiquitous organism, and is often found in sputum or bronchoalveolar lavage (BAL) samples. The presence of Aspergillus in these respiratory samples does not necessarily represent a true infection, but in most cases is merely indicative of colonization. Nevertheless, colonization itself is not a risk factor to be overlooked. In the context of immunosuppression and decreased mucociliary motility, colonization and other risk factors can often lead to a true invasive infection; highlighting the importance of having precise diagnostic tools. Moreover, diagnostic tests used in other patient populations such as hematological malignancies cannot be readily applied in Lung transplant recipients (LTRs).
In this paper we will review the current state of the diagnosis of invasive aspergillosis in lung transplantation, and will comment on the currently available tests as well as diagnostic tests in development, that will available in the near future.
Invasive pulmonary aspergillosis in LTRs has several particularities that are not accounted for in The European Organisation for Research and Treatment of Cancer (EORTC) guidelines, such as colonization, tracheobronchitis and bronchial anastomotic infections. It is therefore preferred to use the International Society for Heart and Lung Transplantation (ISHLT) guidelines (Husain et al., 2011) for the diagnosis of these patients, as it includes diagnostic criteria for all these entities. For the diagnosis of ‘proven IPA’ there must be a biopsy showing histologic evidence of parenchymal invasion by fungal hyphae, or pseudohyphae, or positive culture from sterile tissue ALONE; OR with sign/symptoms AND radiological criteria AND laboratory criteria [single positive culture for mold BAL/blood OR single positive PCR for mold BAL/blood OR positive galactomannan in the BAL; OR at least TWO positive sputum cultures/PCRs of fungal organisms (excluding Candida species)]. For ‘probable IPA’ their criteria are the same as for proven, except there is negative or absent histology.
There have been immense advances in the past decade in the development of molecular and immunological tests for the diagnosis of IPA. However, these are not widely available or standardized, and culture and microscopy still play an important role in the diagnosis of IPA. Microscopic methods such as wet mounts, Gram stains, and conventional histopathology, are useful suggesting the presence of Aspergillus spp. in parenchyma biopsies or BAL samples for the diagnosis of IPA (McClenny, 2005). Aspergillus spp. grows as septate hyphae 2.5–4.5 μm in diameter and can be characterized as branching dichotomously (∼ 45°C angle) (Lass-Flörl and Mayr, 2009). Demonstration of Aspergillus spp. in tissue is the only way to prove IPA, however, a negative result cannot rule it out. For a definite identification of the species, a culture or the use of molecular methods is required, in addition to histology. Aspergillus spp. can grow from fungal media and also from sheep blood agar commonly used for bacterial culture. Culture has the advantage of speciation and ability to perform susceptibility testing. Their sensitivity nevertheless is far from ideal with a positive culture in sputum in 8 to 34% of patients with IPA and 45 to 62% in BAL (Horvath and Dummer, 1996; Hoenigl et al., 2014). Sensitivity is significantly higher in samples from lung biopsies (Lass-Flörl et al., 2007). The main caveat for these methods are the processing times, especially in immunocompromized patients where diagnosis is truly time-sensitive. Additionally in LTRs culture is unable to differentiate colonization from invasive disease, unless the culture is done directly from a tissue sample. Since Aspergillus spp. is ubiquitous, contamination of samples can also occur. Trained staff in the lab are essential for better performance of the aforementioned tests.
Serological tests are among the most widely used tests for the diagnosis of IPA. This is in part the result of the poor yield of respiratory sample cultures.
The major antigenic component secreted by A. fumigatus into the growth medium is galactomannan (GM) – a soluble polysaccharide that is present in the cell wall of most Aspergillus and Penicillium species (Latge et al., 1994). We can detect GM in biological fluids by a commercial sandwich enzyme-linked immunosorbent assay (ELISA) such as the PlateliaTM Aspergillus (Bio-Rad, Marnes-la-Coquette, France) (Stynen et al., 1995). Despite being used extensively, the ELISA lacks some of the advantages that cultures provide; notably speciation and sensitivities. Therefore, it is generally used in combination with cultures or histological samples. In LTRs GM has been studied both in serum and in BAL, having a better performance in the latter (Clancy et al., 2007). It’s sensitivity and specificity range from 60 to 100% and 85 to 98%, respectively, according to different studies including several meta-analysis (Pfeiffer et al., 2006; Clancy et al., 2007; Husain et al., 2007, 2008; Zou et al., 2012; see Table 1). It is to note that some of the studies were not done purely on LTR and some included other SOT and some hematological malignancies (Pfeiffer et al., 2006; Zou et al., 2012).
Table 1. Summary of human studies assessing galactomannan, BD-glucan and Aspergillus PRC in lung transplantation.
Although an optical density cut-off of 0.5 is generally used in both serum and BAL assays, several of these studies showed that increasing the optical density cut-off to 1.0 in BAL increased the specificity of the assay (Clancy et al., 2007; Husain et al., 2007, 2008). Husain et al. (2007) studied 116 LTRs, 6 with probable or proven IPA, finding sensitivity of 60% and specificity of 95%. By increasing the index cutoff value for BAL to >0.66 or = 1.0 a specificity of 98% was achieved, while maintaining a sensitivity of 60%. Similar findings were reported by Clancy et al. (2007) who noted that increasing the cut-off to ≥1 improved the specificity to 90.8%, and by Husain et al. (2008), who demonstrated a higher specificity of 96.6% with cut-off of 1.0 in a larger cohort of LTRs.
As the Aspergillus GM assay is a widely used tool, it is often used to monitor therapeutic response in LTRs. In practice, serum GM is more feasible for monitoring than BAL GM, as it does not require the repeated bronchoscopies necessary to obtain multiple BAL samples. However, GM has several limitations that need to be taken into account. Firstly, a positive BAL GM does not necessarily mean there is an invasive disease as a positive BAL GM result can also be found in colonized patients (Herrera and Husain, 2018). Secondly, both false positive and false negative results have been reported with varying frequency. Cross reactivity has been reported in patients with Paracoccidioides brasiliensis, Histoplasma capsulatum, and Cryptococcus (Xavier et al., 2009). Use of Piperacillin-tazobactam has also been associated with false positive GM in the past (Sulahian et al., 2003), as its production is the result of the fermentation product of molds of the genus Penicillium that also contain GM in their wall (Aubry et al., 2006). This issue seems to be less of a problem recently with new formulations of Piperacillin-tazobactam (Vergidis et al., 2014). In children, it has been hypothesized that GM present in some food (such as milk, rice, or protein nutriments) can possibly pass through the intestinal mucosa and in turn actuate a false positive result (Siemann et al., 1998; Gangneux et al., 2002). Finally, Plasma-Lyte an electrolyte replacement solution containing sodium gluconate, has also been identified as a cause of false positive GM in BAL samples (Muñoz et al., 2003).
In LTRs, false positivity of the GM assay was reported in 20% of the patients in a 2004 study by Husain et al. (2004) Importantly, most false-positive tests occurred in the early post-transplant period, with 79% occurring in the first 14 days following transplantation. This is a limitation of the assay as it is the time period where patients at are at higher immunosuppressive risk, and a false positive result would prompt the physicians into initiating unnecessary treatment. This phenomenon was more likely to happen in patients with the underlying diseases of cystic fibrosis (CF) or chronic obstructive pulmonary disease (COPD), as these patient populations are known to have higher rates of Aspergillus colonization. Pasqualotto et al. (2010) also found a high rate of false positivity, in a 2010 study 55.5% of the cases were associated with Aspergillus colonization when using a cut-off of 1.0. False positivity was also noted to be higher in single lung transplant recipients.
On the other hand, patients receiving anti-mold prophylaxis or treatment often have false negative GM results (Duarte et al., 2014). Other causes of false negative results include patients who have been in contact with Aspergillus before, as they may develop antibodies, which reduces the GM available for the assay to detect (Mennink-Kersten et al., 2004). Both situations are common scenarios after lung transplantation and may reduce the sensitivity of the assay. A previous study in 2013 also suggested that the GM optical index cut-off might vary according to the species of Aspergillus spp. (Xavier et al., 2013).
(1-3)-β-d-glucan is another cell wall polysaccharide that is found in most fungi, with the exception of Cryptococcus, Zygomycetes, and Blastomyces dermatitidis. Several (1-3)-β-d-glucan assays have been developed by different companies and use different cut-offs: Fungitell 60–80 pg/mL (Associates of Cape Cod, East Falmouth, MA, United States), Endosafe-PTS 10–1000 pg/mL (Charles River Laboratories, Charleston, SC), Fungitec-G 20 pg/mL (Seikagaku Biobusiness, Tokyo, Japan), beta-Glucan Test 11 pg/mL (Waco Pure Chemical Industries, Osaka, Japan), and BGSTAR β-Glucan Test 11 pg/mL (Maruha, Tokyo, Japan). It is a chromogenic kinetic assay based on the Limulus test. To summarize, β-Glucan (BG) activates factor G, a protease of Limulus amebocyte lysate (LAL), which is extracted from the amebocytes of horseshoe crab species. This triggers the activation of a coagulation cascade, and the activity of this reaction is measured (Hope et al., 2005).
The Limulus test has been used for the diagnosis of numerous invasive fungal infections, including IPA. Its performance has been shown to be best in hematological malignancies (Senn et al., 2008). In solid organ transplantation and lung transplant in particular, it has several limitations that have lead GM to be a better choice for the diagnosis of IPA. The studies performed, in both serum and BAL in LTRs have shown poor results, with sensitivities and specificities ranging from 71 to 80% and 38 to 81%, respectively (Alexander et al., 2010; Mutschlechner et al., 2015; Bhaskaran et al., 2017; Table 1). One study achieved an increased sensitivity and specificity in LTR’s by increasing the cut-off of the Fungitell assay to 524 pg/ml (Bhaskaran et al., 2017), a nearly sevenfold increase from the cut-off of 40–80 pg/ml used as standard. All studies have consistent results showing a very low specificity, which limits the use of this assay for IPA diagnosis. Conversely, it has in fact been used in other invasive fungal infections, such as Candida infections (Ahmed et al., 2017) and Pneumocystis jirovecii pneumonia, where it has an excellent performance according to a recent meta-analysis (Karageorgopoulos et al., 2013).
Other issues that limit the use of this assay include false positive results due to cross reactions with certain hemodialysis filters, beta-lactam antimicrobials, and immunoglobulins (Theel and Doern, 2013), all of them frequently used in the lung transplant population. Additionally, it is unable to differentiate colonization from invasive disease in BAL (Herrera and Husain, 2018). Its role in the management of lung transplant recipients is not yet well defined.
Many polymerase chain reaction (PCR) tests for Aspergillus spp. have been developed with great expectations as a promising tool to solve the enigma of IPA diagnosis. However, to date they have failed to live up to those expectations. Several PCRs have been developed according to primer selection (panfungal, genus specific, or species specific), and PCR formats (qualitative, quantitative, and real-time). DNA in respiratory samples of LTRs, has been studied by two standardized Aspergillus assays, Viracor (Viracor-IBT Laboratories) and MycAssay (Myconostica). One of the main caveats that this test faces is the lack of standardization in the procurement of samples. Its costs exceed those of GM or β-d-glucan, and it is not available in most centers. Furthermore, as with the aforementioned tests it is unable to differentiate colonization from invasive disease (Herrera and Husain, 2018) and unable to identify subspecies; requiring additional specific subspecies PCR and unable to provide antifungal susceptibilities. Despite these limitations and the lack of studies that have been performed in the LTR population, the few available studies have shown very good outcomes with sensitives and specificities of 100 and 88%, respectively, for diagnosis of IPA; and sensitivity and specificity for A. fumigatus-specific PCR of 85 and 96%, respectively (Luong et al., 2011; Table 1). Additional advantages include the ability to identify mutations associated with treatment resistance (Bernal-Martínez et al., 2017; Dannaoui et al., 2017) that are usually time consuming using conventional methods alone. Although AsperGenius (PathoNostics, Maastricht, Netherlands), has not been yet tested in LTRs for the identification of azole resistance in A. fumigatus, this could have a broad potential in this population, especially in colonized cystic fibrosis patients undergoing lung transplantation (Chong et al., 2016). Multiple PCR tests are being developed, but unfortunately none of these have been tested in LTRs (Salehi et al., 2016). All of these factors indicate that this test will be a very promising tool in the near future.
Table 1 summarizes the studies available in LTRs for the different serological and molecular markers available.
Use of monoclonal antibodies has changed many fields in medicine over the past decades. Some centers have attempted to develop monoclonal antibodies against Aspergillus spp. for the diagnosis of IPA.
The first of these developed was the lateral flow device, an immunochromatographic assay that uses JF5, a monoclonal antibody that binds to an extracellular glycoprotein secreted during active growth of Aspergillus spp. This assay has been tested in several populations, including SOT (Willinger et al., 2014). It seems to display a better performance in BAL, where pooled sensitivity and specificity for the proven/probable IPA was assessed in a recent meta-analysis showing 86 and 93%, respectively (Pan et al., 2015). Its main strength is high specificity, and a new version of the assays shows improved specificity compared to the older assay according to a recent study (Hoenigl et al., 2018). However, the noted sensitivity of this assay is not as good, and is further decreased in patients receiving anti-mold prophylaxis (Castillo et al., 2017). Additionally, cross-reactivity has been reported with Penicillium spp. (Heldt and Hoenigl, 2017). So far the lateral flow device has only been studied in cohorts including a mix of LTR’s, patients with hematological malignancies, or other solid organ transplants (Castillo et al., 2017). Thus, extrapolating the results for these mixed cohorts may not give an accurate indication of the sensitivity and specificity for LTR’s alone.
Most recently, a group from Baltimore United States used an anti-A. fumigatus antibody (mAb476) that rapidly detects a fungal antigen in urine (Marr et al., 2018), avoiding the need for more invasive tests. The use of this antibody test in IPA had previously been proven possible only in mice and guinea pig models (Dufresne et al., 2012). In their human study, they found that the best performance was in patients who had hematological malignancies, whereas the sensitivity from the small subgroup with other underlying diseases was lower at 63.6%. Several false negative results were found in LTRs who had non-invasive forms of the disease. Excluding these patients gave a higher sensitivity of 88.5% for the test. Specificities where high overall, at around 92%. However, false positive results were also found in patients with Histoplasmosis. The advantages offered by this test are, simplicity and the possibility of earlier diagnosis. Nevertheless, further studies are needed in LTRs as with the scarce available data, it seems more advantageous in hematological malignancies.
A very original test has been able to identify a profile of volatile organic compounds exhaled in the breath of patients with pathogenic Aspergillus. These compounds are present during the growth phase of the fungus, in patients undergoing evaluation for IPA. Detection of α-trans-bergamotene, β-trans-bergamotene, a β-vatirenene-like sesquiterpene, or trans-geranylacetone identified IPA patients with 94% sensitivity and 93% specificity (Koo et al., 2014). The study was done in a heterogeneous cohort of immunocompromized patients. In LTRs volatile organic compounds have been studied for diagnosis of chronic lung allograft dysfunction (Kuppers et al., 2018), but to the date there are no studies assessing this test for IPA diagnosis.
Other tests that have been explored by researchers to refine the diagnosis of IPA include the detection of biomarkers, such as Pentraxin-3 (PTX3) and cytokines. These tests are used in conjunction with standardized serological or other fungal diagnostic tests. One of these tests is monitoring the levels of PTX3. PTX3 is a soluble pattern recognition receptor, expressed after induction of inflammatory cytokines in response to inflammatory stimuli from endothelial cells and mononuclear phagocytes. Animal models showed PTX3 enhanced survival rate and reduced the lung fungal burden of infected rats (Lo Giudice et al., 2010). In a 2014 study in the stem cell transplant population, genetic deficiency of PTX3 was associated with increased risk of IPA in hematopoietic stem cell transplantation (HSCT) (Cunha et al., 2014). While not specific for Aspergillus spp., PTX3 has been used as diagnostic tool and for therapeutic monitoring in stem cell transplant studies (Biagi et al., 2008), where PTX3 levels were high in patients with IPA and decreased after treatment. However, only one group has explored this biomarker in the LTR population (Kabbani et al., 2017), where it was noted that PTX3 levels were significantly higher in BAL samples of LTRs with IPA. Patients with high levels of PTX3 in the BAL with positive GM or positive Aspergillus culture were 4.5 and 5.5 times more likely to have invasive pulmonary aspergillosis, respectively.
Identification of a specific signature of cytokines for IPA may be of tremendous help in the diagnosis of IPA. Cytokines have been studied more in depth in hematological malignancies; however, in these studies, a noted association has been made that higher levels of interleukin 10 (IL-10) were found in BAL of patients who also had IPA (Cunha et al., 2017) or worst outcomes in patients with persistently elevated IL-6 and IL-8 (Chai et al., 2010). To date, there haven’t been any studies assessing the role of cytokines in IPA in LTRs exclusively. One study that included LTRs among other solid organ transplant (SOT) recipients and hematological malignancies concluded that IL-1β, IL-6, IL-8, IL-17A, IL-23, and tumor necrosis factor alpha (TNFα) levels in BAL were significantly increased among patients with IPA, with IL-8 being the best marker (Gonçalves et al., 2017).
The incorporation of matrix-assisted laser desorption/ionization time-of-flight (MALDI-TOF) mass spectrometry to microbiology laboratories has been a revolution in the bacterial diagnosis of infections. Efforts are being made to apply this technique to other fields such as mycology. If implemented, this form of mass spectrometry will be a great advance in reducing the time of diagnosis. Yet a positive test will not be able to differentiate between colonization and disease in LTRs (Sanguinetti and Posteraro, 2014).
Triacetylfusarinine C (TAFC) is a siderophore secreted by A. fumigatus after conidiospore germination in media where iron is scarce. Its synthesis is required for fungal germination, and it is crucial for the virulence factor of A. fumigatus in a mice models of IPA (Schrettl et al., 2004). Studies performed in patients with systemic erythematous lupus have proved that TAFC can be measured in the serum of these patients (Carroll et al., 2016). In immunocompromized patients it has been tested in a small cohort of patients with hematological malignancies, where it was found to increase the sensitivity of BAL GM (Orasch et al., 2017). Limitations of this test include a lack of larger studies, and the fact that it is mainly produced by A. fumigatus and A. nidulans (Charlang et al., 1981), meaning that some other species of Aspergillus that are also known to be etiological for IPA are excluded. To date there have been no studies in LTRs.
Radiology represents one of the pillars of IPA diagnosis. Most guidelines agree that while not specific to IPA alone, the typical radiological presentations in IPA include nodules, air crescent sign, cavity and halo sign. To complicate matters, however, the radiological presentation of IPA may be different in LTRs as compared to hematological malignancies or other SOT recipients. A 2014 study showed that bilateral bronchial wall thickening and centrilobular opacities with a tree-in-bud pattern were the most common radiological findings in a cohort of LTRs (Gazzoni et al., 2014). Another study that included LTRs among other SOT patients, found that halo sign was only observed in 8% of SOT recipients, whereas peri-bronchial consolidations were observed in 31% and ground-glass opacities in 38% (Park et al., 2010).
Positron emission tomography (PET) scans have also been studied for the diagnosis of IPA (Hot et al., 2011), but have a limited utility due to false positive results in patients with post-transplant lymphoproliferative disease (PTLD) or lung cancer (Baxter et al., 2011), and therefore must be used with caution. To avoid false positive results, immunological tests combined with diagnostic imaging such as PET scans or magnetic resonance imaging (MRI) are being developed. Using the monoclonal antibody JF5 combined with PET scan, researchers have found that the antibody binds to the antigenic determinant β1,5-galactofuranose present in a diagnostic antigen that is released by the pathogen during invasive growth in the lung (Davies et al., 2017). This strategy overcomes the problems of specificity faced by a computed tomography (CT) scans of the chest or MRI alone, or with the false positive results seen with the PET scan alone. However, the availability of this developing tool will be a limiting factor for its widespread use.
Figure 1 shows a proposed algorithm for the future management and diagnosis of IPA. This algorithm includes some of the newer and developing diagnostic tools. One of the emerging factors that will likely play a large role in the future, is the detection of biomarkers related to a patient’s susceptibility to the disease, and the host’s potential to control angioinvasion. This will also be a determining factor in changing the landscape of how we manage prophylaxis of these patients, as it can help tailor a more individualized approach to treatment.
Despite the advances in transplantation and treatment of IPA, the development of an accurate diagnostic or prognostic tool for IPA continues to be a struggle.
New areas of research include identification of genetic susceptibility markers and prognostic indicators of IPA. One of those markers is Signal transducer and activator of transcription 3 (STAT3), a member of the STAT protein family and an important transcription activator has been found to have an important role in invasive fungal infections (Puel et al., 2011) as it is associated to the T-helper cell 17 (Th17) pathway (Yang et al., 2007). Inhibition of STAT3 had been shown to impair hyphal killing (Taylor et al., 2016) in animal models. Autosomal-dominant STAT3-deficient hyper-IgE syndrome is associated to susceptibility of late-onset mold infections, in particular Aspergillus (Vinh et al., 2010). STAT3 may hold the key to the timing when Aspergillus hyphae stops being a bystander, and starts to become an invasive disease.
Another set of biomarkers that can stratify the risk and prognosis of IPA is microRNA. MicroRNAs are non-coding RNAs capable of influencing gene expression through a variety of mechanisms (Lu and Rothenberg, 2013). Recent studies have examined MicroRNAs and identified those responsible for the regulation genes involved in the pulmonary immune responses following sub chronic inhalation exposure to A. fumigatus (Croston et al., 2016). Identification of microRNA’s regulation of, and impact on target genes might be able to assist in the diagnosis and treatment of IPA. Recently, zinc finger protein-77 (ZNF77) has been identified as a key player in Aspergillus colonization, by causing a loss of integrity of the bronchial epithelium and an increase in the levels of extracellular matrix proteins (Gago et al., 2018). These changes promote A. fumigatus conidial adhesion, germination and growth. However, these data have so far only been examined in limited sample sizes and need to be scrutinized in a larger cohort.
Lastly, there is an omnipresent need to perform larger studies in the LTR population. As transplant numbers continue to rise, larger studies would allow an improved comprehension of many of the tests described above which so far, have only been studied in small cohorts.
All authors contributed to the writing and revision of this manuscript.
The authors declare that the research was conducted in the absence of any commercial or financial relationships that could be construed as a potential conflict of interest.
BAL, bronchoalveolar lavage; BG, beta-glucan; CF, cystic fibrosis; CMV, cytomegalovirus; COPD, chronic obstructive pulmonary disease; CT, computed tomography; ELISA, enzyme-linked immunosorbent assay; EORTC, European Organisation for Research and Treatment of Cancer; GM, galactomannan; HSCT, hematopoietic stem cell transplantation; IL, interleukin; IPA, invasive pulmonary aspergillosis; ISHLT, international society for heart and lung transplantation; LAL, Limulus amebocyte lysate; LTRs, lung transplant recipients; MALDI-TOF, matrix-assisted laser desorption/ionization time-of-flight; MRI, magnetic resonance imaging; PCR, polymerase chain reaction; PET, positron emission tomography; PTLD, post-transplantation lymphoproliferative disease; PTX3, pentraxin-3; SOT, solid organ transplant; STAT3, signal transducer and activator of transcription 3; TAFC, triacetylfusarinine C; Th17, T-helper cell 17; TNFα, tumor necrosis factor alpha; ZNF77, zinc finger protein-77.
Ahmed, A., Baronia, A. K., Azim, A., Marak, R. S. K., Yadav, R., Sharma, P., et al. (2017). External validation of risk prediction scores for invasive candidiasis in a medical/surgical intensive care unit: an observational study. Indian J. Crit. Care Med. 21, 514–520. doi: 10.4103/ijccm.IJCCM_33_17
Alexander, B. D., Smith, P. B., Davis, R. D., Perfect, J. R., and Reller, L. B. (2010). The (1,3){beta}-D-glucan test as an aid to early diagnosis of invasive fungal infections following lung transplantation. J. Clin. Microbiol. 48, 4083–4088. doi: 10.1128/JCM.01183-10
Aubry, A., Porcher, R., Bottero, J., Touratier, S., Leblanc, T., Brethon, B., et al. (2006). Occurrence and kinetics of false-positive Aspergillus galactomannan test results following treatment with beta-lactam antibiotics in patients with hematological disorders. J. Clin. Microbiol. 44, 389–394. doi: 10.1128/JCM.44.2.389-394.2006
Baxter, C. G., Bishop, P., Low, S. E., Baiden-Amissah, K., and Denning, D. W. (2011). Pulmonary aspergillosis: an alternative diagnosis to lung cancer after positive [18F]FDG positron emission tomography. Thorax 66, 638–640. doi: 10.1136/thx.2010.155515
Bernal-Martínez, L., Gil, H., Rivero-Menéndez, O., Gago, S., Cuenca-Estrella, M., Mellado, E., et al. (2017). Development and validation of a high-resolution melting assay to detect azole resistance in Aspergillus fumigatus. Antimicrob. Agents Chemother. 61:e01083-17. doi: 10.1128/AAC.01083-17
Bhaskaran, A., Kabbani, D., Singer, L. G., Prochnow, T., Bhimji, A., Rotstein, C., et al. (2017). (1,3) β-D-glucan in bronchoalveolar lavage of lung transplant recipients for the diagnosis of invasive pulmonary aspergillosis. Med. Mycol. 55, 173–179. doi: 10.1093/mmy/myw052
Bhimji, A., Bhaskaran, A., Singer, L. G., Kumar, D., Humar, A., Pavan, R., et al. (2018). Aspergillus galactomannan detection in exhaled breath condensate compared to bronchoalveolar lavage fluid for the diagnosis of invasive aspergillosis in immunocompromised patients. Clin. Microbiol. Infect. 24, 640–645. doi: 10.1016/j.cmi.2017.09.018
Biagi, E., Col, M., Migliavacca, M., Dell’Oro, M., Silvestri, D., Montanelli, A., et al. (2008). PTX3 as a potential novel tool for the diagnosis and monitoring of pulmonary fungal infections in immuno-compromised pediatric patients. J. Pediatr. Hematol. Oncol. 30, 881–885. doi: 10.1097/MPH.0b013e318180bc1d
Carroll, C. S., Amankwa, L. N., Pinto, L. J., Fuller, J. D., and Moore, M. M. (2016). Detection of a serum siderophore by LC-MS/MS as a potential biomarker of invasive aspergillosis. PLoS One 11:e0151260. doi: 10.1371/journal.pone.0151260
Castillo, C. G., Kauffman, C. A., Zhai, J., Jiang, H., Agozino, S. M., and Miceli, M. H. (2017). Testing the performance of a prototype lateral flow device using bronchoalveolar lavage fluid for the diagnosis of invasive pulmonary aspergillosis in high-risk patients. Mycoses 61, 4–10. doi: 10.1111/myc.12694
Chai, L. Y. A., Netea, M. G., Teerenstra, S., Earnest, A., Vonk, A. G., Schlamm, H. T., et al. (2010). Early proinflammatory cytokines and C-reactive protein trends as predictors of outcome in invasive aspergillosis. J. Infect. Dis. 202, 1454–1462. doi: 10.1086/656527
Charlang, G., Ng, B., Horowitz, N. H., and Horowitz, R. M. (1981). Cellular and extracellular siderophores of Aspergillus nidulans and Penicillium chrysogenum. Mol. Cell. Biol. 1, 94–100. doi: 10.1128/MCB.1.2.94
Chong, G. M., van der Beek, M. T., von dem Borne, P. A., Boelens, J., Steel, E., Kampinga, G. A., et al. (2016). PCR-based detection of Aspergillus fumigatus Cyp51A mutations on bronchoalveolar lavage: a multicentre validation of the AsperGenius assay®in 201 patients with haematological disease suspected for invasive aspergillosis. J. Antimicrob. Chemother. 71, 3528–3535. doi: 10.1093/JAC/DKW323
Clancy, C. J., Jaber, R. A., Leather, H. L., Wingard, J. R., Staley, B., Joseph Wheat, L., et al. (2007). Bronchoalveolar lavage galactomannan in diagnosis of invasive pulmonary aspergillosis among solid-organ transplant recipients. J. Clin. Microbiol. 45, 1759–1765. doi: 10.1128/JCM.00077-07
Croston, T. L., Nayak, A. P., Lemons, A. R., Goldsmith, W. T., Gu, J. K., Germolec, D. R., et al. (2016). Influence of Aspergillus fumigatus conidia viability on murine pulmonary microRNA and mRNA expression following subchronic inhalation exposure. Clin. Exp. Allergy 46, 1315–1327. doi: 10.1111/cea.12783
Cunha, C., Aversa, F., Lacerda, J. F., Busca, A., Kurzai, O., Grube, M., et al. (2014). Genetic PTX3 deficiency and aspergillosis in stem-cell transplantation. N. Engl. J. Med. 370, 421–432. doi: 10.1056/NEJMoa1211161
Cunha, C., Gonçalves, S. M., Duarte-Oliveira, C., Leite, L., Lagrou, K., Marques, A., et al. (2017). IL-10 overexpression predisposes to invasive aspergillosis by suppressing antifungal immunity. J. Allergy Clin. Immunol. 140, 867.e9–870.e9. doi: 10.1016/j.jaci.2017.02.034
Dannaoui, E., Gabriel, F., Gaboyard, M., Lagardere, G., Audebert, L., Quesne, G., et al. (2017). Molecular diagnosis of invasive aspergillosis and detection of azole resistance by a newly commercialized PCR kit. J. Clin. Microbiol. 55, 3210–3218. doi: 10.1128/JCM.01032-17
Davies, G., Rolle, A.-M., Maurer, A., Spycher, P. R., Schillinger, C., Solouk-Saran, D., et al. (2017). Towards translational immunopet/mr imaging of invasive pulmonary aspergillosis: the humanised monoclonal antibody JF5 detects Aspergillus lung infections in vivo. Theranostics 7, 3398–3414. doi: 10.7150/thno.20919
Duarte, R. F., Sánchez-Ortega, I., Cuesta, I., Arnan, M., Patiño, B., Fernández de Sevilla, A., et al. (2014). Serum galactomannan-based early detection of invasive aspergillosis in hematology patients receiving effective antimold prophylaxis. Clin. Infect. Dis. 59, 1696–1702. doi: 10.1093/cid/ciu673
Dufresne, S. F., Datta, K., Li, X., Dadachova, E., Staab, J. F., Patterson, T. F., et al. (2012). Detection of urinary excreted fungal galactomannan-like antigens for diagnosis of invasive aspergillosis. PLoS One 7:e42736. doi: 10.1371/journal.pone.0042736
Gago, S., Overton, N. L. D., Ben-Ghazzi, N., Novak-Frazer, L., Read, N. D., Denning, D. W., et al. (2018). Lung colonization by Aspergillus fumigatus is controlled by ZNF77. Nat. Commun. 9:3835. doi: 10.1038/s41467-018-06148-7
Gangneux, J. P., Lavarde, D., Bretagne, S., Guiguen, C., and Gandemer, V. (2002). Transient aspergillus antigenaemia: think of milk. Lancet 359:1251. doi: 10.1016/S0140-6736(02)08238-7
Gazzoni, F. F., Hochhegger, B., Severo, L. C., Marchiori, E., Pasqualotto, A., Sartori, A. P., et al. (2014). High-resolution computed tomographic findings of aspergillus infection in lung transplant patients. Eur. J. Radiol. 83, 79–83. doi: 10.1016/j.ejrad.2013.03.026
Gonçalves, S. M., Lagrou, K., Rodrigues, C. S., Campos, C. F., Bernal-Martínez, L., Rodrigues, F., et al. (2017). Evaluation of bronchoalveolar lavage fluid cytokines as biomarkers for invasive pulmonary aspergillosis in at-risk patients. Front. Microbiol. 8:2362. doi: 10.3389/fmicb.2017.02362
Heldt, S., and Hoenigl, M. (2017). Lateral flow assays for the diagnosis of invasive aspergillosis: current status. Curr. Fungal Infect. Rep. 11, 45–51. doi: 10.1007/s12281-017-0275-8
Herrera, S., and Husain, S. (2018). Early diagnosis of fungal infections in lung transplant recipients, colonization versus invasive disease? Curr. Opin. Organ. Transplant. 23, 381–387. doi: 10.1097/MOT.0000000000000543
Hoenigl, M., Eigl, S., Heldt, S., Duettmann, W., Thornton, C., and Prattes, J. (2018). Clinical evaluation of the newly formatted lateral-flow device for invasive pulmonary aspergillosis. Mycoses 61, 40–43. doi: 10.1111/myc.12704
Hoenigl, M., Prattes, J., Spiess, B., Wagner, J., Prueller, F., Raggam, R. B., et al. (2014). Performance of galactomannan, beta-d-glucan, Aspergillus lateral-flow device, conventional culture, and PCR tests with bronchoalveolar lavage fluid for diagnosis of invasive pulmonary aspergillosis. J. Clin. Microbiol. 52, 2039–2045. doi: 10.1128/JCM.00467-14
Hope, W. W., Walsh, T. J., and Denning, D. W. (2005). Laboratory diagnosis of invasive aspergillosis. Lancet Infect. Dis. 5, 609–622. doi: 10.1016/S1473-3099(05)70238-3
Horvath, J. A., and Dummer, S. (1996). The use of respiratory-tract cultures in the diagnosis of invasive pulmonary aspergillosis. Am. J. Med. 100, 171–178. doi: 10.1016/S0002-9343(97)89455-7
Hot, A., Maunoury, C., Poiree, S., Lanternier, F., Viard, J. P., Loulergue, P., et al. (2011). Diagnostic contribution of positron emission tomography with [18F]fluorodeoxyglucose for invasive fungal infections. Clin. Microbiol. Infect. 17, 409–417. doi: 10.1111/j.1469-0691.2010.03301.x
Husain, S., Clancy, C. J., Nguyen, M. H., Swartzentruber, S., Leather, H., LeMonte, A. M., et al. (2008). Performance characteristics of the platelia Aspergillus enzyme immunoassay for detection of Aspergillus galactomannan antigen in bronchoalveolar lavage fluid. Clin. Vaccine Immunol. 15, 1760–1763. doi: 10.1128/CVI.00226-08
Husain, S., Kwak, E. J., Obman, A., Wagener, M. M., Kusne, S., Stout, J. E., et al. (2004). Prospective assessment of platelia aspergillus galactomannan antigen for the diagnosis of invasive aspergillosis in lung transplant recipients. Am. J. Transplant. 4, 796–802. doi: 10.1111/j.1600-6143.2004.00415.x
Husain, S., Mooney, M. L., Danziger-Isakov, L., Mattner, F., Singh, N., Avery, R., et al. (2011). A 2010 working formulation for the standardization of definitions of infections in cardiothoracic transplant recipients. J. Heart Lung Transplant. 30, 361–374. doi: 10.1016/j.healun.2011.01.701
Husain, S., Paterson, D. L., Studer, S. M., Crespo, M., Pilewski, J., Durkin, M., et al. (2007). Aspergillus galactomannan antigen in the bronchoalveolar lavage fluid for the diagnosis of invasive aspergillosis in lung transplant recipients. Transplantation 83, 1330–1336. doi: 10.1097/01.tp.0000263992.41003.33
Husain, S., Sole, A., Alexander, B. D., Aslam, S., Avery, R., Benden, C., et al. (2016). The 2015 international society for heart and lung transplantation guidelines for the management of fungal infections in mechanical circulatory support and cardiothoracic organ transplant recipients: executive summary. J. Heart Lung Transplant. 35, 261–282. doi: 10.1016/j.healun.2016.01.007
Kabbani, D., Bhaskaran, A., Singer, L. G., Bhimji, A., Rotstein, C., Keshavjee, S., et al. (2017). Pentraxin 3 levels in bronchoalveolar lavage fluid of lung transplant recipients with invasive aspergillosis. J. Heart Lung Transplant. 36, 973–979. doi: 10.1016/j.healun.2017.04.007
Karageorgopoulos, D. E., Qu, J.-M., Korbila, I. P., Zhu, Y. G., Vasileiou, V. A., and Falagas, M. E. (2013). Accuracy of β-D-glucan for the diagnosis of Pneumocystis jirovecii pneumonia: a meta-analysis. Clin. Microbiol. Infect. 19, 39–49. doi: 10.1111/j.1469-0691.2011.03760.x
Koo, S., Thomas, H. R., Daniels, S. D., Lynch, R. C., Fortier, S. M., Shea, M. M., et al. (2014). A breath fungal secondary metabolite signature to diagnose invasive aspergillosis. Clin. Infect. Dis. 59, 1733–1740. doi: 10.1093/cid/ciu725
Kuppers, L., Holz, O., Schuchardt, S., Gottlieb, J., Fuge, J., Greer, M., et al. (2018). Breath volatile organic compounds of lung transplant recipients with and without chronic lung allograft dysfunction. J. Breath Res. 12:036023. doi: 10.1088/1752-7163/aac5af
Lass-Flörl, C., Follett, S. A., Moody, A., and Denning, D. W. (2011). Detection of Aspergillus in lung and other tissue samples using the MycAssay Aspergillus real-time PCR kit. Can. J. Microbiol. 57, 765–768. doi: 10.1139/w11-064
Lass-Flörl, C., and Mayr, A. (2009). Diagnosing invasive fungal diseases – limitations of microbiological diagnostic methods. Expert Opin. Med. Diagn. 3, 461–470. doi: 10.1517/17530050902878031
Lass-Flörl, C., Resch, G., Nachbaur, D., Mayr, A., Gastl, G., Auberger, J., et al. (2007). The value of computed tomography-guided percutaneous lung biopsy for diagnosis of invasive fungal infection in immunocompromised patients. Clin. Infect. Dis. 45:e101-4. doi: 10.1086/521245
Latge, J. P., Kobayashi, H., Debeaupuis, J. P., Diaquin, M., Sarfati, J., Wieruszeski, J. M., et al. (1994). Chemical and immunological characterization of the extracellular galactomannan of Aspergillus fumigatus. Infect. Immun. 62, 5424–5433.
Lo Giudice, P., Campo, S., Verdoliva, A., Rivieccio, V., Borsini, F., De Santis, R., et al. (2010). Efficacy of PTX3 in a rat model of invasive aspergillosis. Antimicrob. Agents Chemother. 54, 4513–4515. doi: 10.1128/AAC.00674-10
Lu, T. X., and Rothenberg, M. E. (2013). Diagnostic, functional, and therapeutic roles of microRNA in allergic diseases. J. Allergy Clin. Immunol. 132, 3–13. doi: 10.1016/j.jaci.2013.04.039
Lund, L. H., Khush, K. K., Cherikh, W. S., Goldfarb, S., Kucheryavaya, A. Y., Levvey, B. J., et al. (2017). The registry of the international society for heart and lung transplantation: thirty-fourth adult heart transplantation report—2017; focus theme: allograft ischemic time. J. Heart Lung Transplant. 36, 1037–1046. doi: 10.1016/j.healun.2017.07.019
Luong, M.-L., Clancy, C. J., Vadnerkar, A., Kwak, E. J., Silveira, F. P., Wissel, M. C., et al. (2011). Comparison of an Aspergillus real-time polymerase chain reaction assay with galactomannan testing of bronchoalvelolar lavage fluid for the diagnosis of invasive pulmonary aspergillosis in lung transplant recipients. Clin. Infect. Dis. 52, 1218–1226. doi: 10.1093/cid/cir185
Marr, K. A., Datta, K., Mehta, S., Ostrander, D. B., Rock, M., Francis, J., et al. (2018). Urine antigen detection as an aid to diagnose invasive aspergillosis. Clin. Infect. Dis. 67, 1705–1711. doi: 10.1093/cid/ciy326
Mattner, F., Fischer, S., Weissbrodt, H., Chaberny, I. F., Sohr, D., Gottlieb, J., et al. (2007). Post-operative nosocomial infections after lung and heart transplantation. J. Heart Lung Transplant. 26, 241–249. doi: 10.1016/j.healun.2006.12.009
McClenny, N. (2005). Laboratory detection and identification of Aspergillus species by microscopic observation and culture: the traditional approach. Med. Mycol. 43(Suppl. 1), S125–S128. doi: 10.1080/13693780500052222
Mennink-Kersten, M. A., Donnelly, J. P., and Verweij, P. E. (2004). Detection of circulating galactomannan for the diagnosis and management of invasive aspergillosis. Lancet Infect. Dis. 4, 349–357. doi: 10.1016/S1473-3099(04)01045-X
Muñoz, P., Alcalá, L., Sánchez Conde, M., Palomo, J., Yáñ J., Pelaez, T., et al. (2003). The isolation of Aspergillus fumigatus from respiratory tract specimens in heart transplant recipients is highly predictive of invasive aspergillosis. Transplantation 75, 326–329. doi: 10.1097/01.TP.0000044358.99414.B8
Mutschlechner, W., Risslegger, B., Willinger, B., Hoenigl, M., Bucher, B., Eschertzhuber, S., et al. (2015). Bronchoalveolar lavage fluid (1,3)β-D-glucan for the diagnosis of invasive fungal infections in solid organ transplantation. Transplantation 99:e140-4. doi: 10.1097/TP.0000000000000635
Orasch, T., Prattes, J., Faserl, K., Eigl, S., Düttmann, W., Lindner, H., et al. (2017). Bronchoalveolar lavage triacetylfusarinine C (TAFC) determination for diagnosis of invasive pulmonary aspergillosis in patients with hematological malignancies. J. Infect. 75, 370–373. doi: 10.1016/j.jinf.2017.05.014
Pan, Z., Fu, M., Zhang, J., Zhou, H., Fu, Y., and Zhou, J. (2015). Diagnostic accuracy of a novel lateral-flow device in invasive aspergillosis: a meta-analysis. J. Med. Microbiol. 64, 702–707. doi: 10.1099/jmm.0.000092
Park, S. Y., Kim, S.-H., Choi, S.-H., Sung, H., Kim, M. N., Woo, J. H., et al. (2010). Clinical and radiological features of invasive pulmonary aspergillosis in transplant recipients and neutropenic patients. Transpl. Infect. Dis. 12, 309–315. doi: 10.1111/j.1399-3062.2010.00499.x
Pasqualotto, A. C., Xavier, M. O., Sánchez, L. B., de Oliveira Costa, C. D., Schio, S. M., Camargo, S. M., et al. (2010). Diagnosis of invasive aspergillosis in lung transplant recipients by detection of galactomannan in the bronchoalveolar lavage fluid. Transplantation 90, 306–311. doi: 10.1097/TP.0b013e3181e49bc1
Pfeiffer, C. D., Fine, J. P., and Safdar, N. (2006). Diagnosis of invasive aspergillosis using a galactomannan assay: a meta-analysis. Clin. Infect. Dis. 42, 1417–1427. doi: 10.1086/503427
Puel, A., Cypowyj, S., Bustamante, J., Wright, J. F., Liu, L., Lim, H. K., et al. (2011). Chronic mucocutaneous candidiasis in humans with inborn errors of interleukin-17 immunity. Science 332, 65–68. doi: 10.1126/science.1200439
Salehi, E., Hedayati, M. T., Zoll, J., Rafati, H., Ghasemi, M., Doroudinia, A., et al. (2016). Discrimination of aspergillosis, mucormycosis, fusariosis, and scedosporiosis in formalin-fixed paraffin-embedded tissue specimens by use of multiple real-time quantitative PCR assays. J. Clin. Microbiol. 54, 2798–2803. doi: 10.1128/JCM.01185-16
Sanguinetti, M., and Posteraro, B. (2014). MALDI-TOF mass spectrometry: any use for aspergilli? Mycopathologia 178, 417–426. doi: 10.1007/s11046-014-9757-1
Schrettl, M., Bignell, E., Kragl, C., Joechl, C., Rogers, T., Arst, H. N. Jr., et al. (2004). Siderophore biosynthesis but not reductive iron assimilation is essential for Aspergillus fumigatus virulence. J. Exp. Med. 200, 1213–1219. doi: 10.1084/jem.20041242
Senn, L., Robinson, J. O., Schmidt, S., Knaup, M., Asahi, N., Satomura, S., et al. (2008). 1,3-Beta-D-glucan antigenemia for early diagnosis of invasive fungal infections in neutropenic patients with acute leukemia. Clin. Infect. Dis. 46, 878–885. doi: 10.1086/527382
Siemann, M., Koch-Dörfler, M., and Gaude, M. (1998). False-positive results in premature infants with the Platelia®Aspergillus sandwich enzyme-linked immunosorbent assay. Mycoses 41, 373–377. doi: 10.1111/j.1439-0507.1998.tb00356.x
Stynen, D., Goris, A., Sarfati, J., and Latgé, J. P. (1995). A new sensitive sandwich enzyme-linked immunosorbent assay to detect galactofuran in patients with invasive aspergillosis. J. Clin. Microbiol. 33, 497–500.
Sulahian, A., Touratier, S., and Ribaud, P. (2003). False positive test for aspergillus antigenemia related to concomitant administration of piperacillin and tazobactam. N. Engl. J. Med. 349, 2366–2367. doi: 10.1056/NEJM200312113492424
Taylor, P. R., Roy, S., Meszaros, E. C., Sun, Y., Howell, S. J., Malemud, C. J., et al. (2016). JAK/STAT regulation of Aspergillus fumigatus corneal infections and IL-6/23-stimulated neutrophil, IL-17, elastase, and MMP9 activity. J. Leukoc. Biol. 100, 213–222. doi: 10.1189/jlb.4A1015-483R
Theel, E. S., and Doern, C. D. (2013). Point-counterpoint: β-d-Glucan testing is important for diagnosis of invasive fungal infections. J. Clin. Microbiol. 51, 3478–3483. doi: 10.1128/JCM.01737-13
Vergidis, P., Razonable, R. R., Wheat, L. J., Estes, L., Caliendo, A. M., Baden, L. R., et al. (2014). Reduction in false-positive aspergillus serum galactomannan enzyme immunoassay results associated with use of piperacillin-tazobactam in the United States. J. Clin. Microbiol. 52, 2199–2201. doi: 10.1128/JCM.00285-14
Vinh, D. C., Sugui, J. A., Hsu, A. P., Freeman, A. F., and Holland, S. M. (2010). Invasive fungal disease in autosomal-dominant hyper-IgE syndrome. J. Allergy Clin. Immunol. 125, 1389–1390. doi: 10.1016/j.jaci.2010.01.047
Willinger, B., Lackner, M., Lass-Flörl, C., Prattes, J., Posch, V., Selitsch, B., et al. (2014). Bronchoalveolar lavage lateral-flow device test for invasive pulmonary aspergillosis in solid organ transplant patients: a semiprospective multicenter study. Transplantation 98, 898–902. doi: 10.1097/TP.0000000000000153
Xavier, M. O., Araujo, J. S., Aquino, V. R., Severo, C. B., Guazzelli, L. S., Severo, L. C., et al. (2013). Variability in galactomannan detection by platelia Aspergillus EIATM according to the Aspergillus species. Rev. Inst. Med. Trop. Sao Paulo 55:S0036-46652013000300145. doi: 10.1590/S0036-46652013000300001
Xavier, M. O., Pasqualotto, A. C., Cardoso, I. C. E., and Severo, L. C. (2009). Cross-reactivity of Paracoccidioides brasiliensis, Histoplasma capsulatum, and Cryptococcus species in the commercial platelia Aspergillus enzyme immunoassay. Clin. Vaccine Immunol. 16, 132–133. doi: 10.1128/CVI.00310-08
Yang, X. O., Panopoulos, A. D., Nurieva, R., Chang, S. H., Wang, D., Watowich, S. S., et al. (2007). STAT3 regulates cytokine-mediated generation of inflammatory helper T cells. J. Biol. Chem. 282, 9358–9363. doi: 10.1074/jbc.C600321200
Keywords: lung transplant, invasive aspergillosis, PCR, Aspergillus, immunocompromized, galactomannan, BDglucan, cytokines
Citation: Herrera S and Husain S (2019) Current State of the Diagnosis of Invasive Pulmonary Aspergillosis in Lung Transplantation. Front. Microbiol. 9:3273. doi: 10.3389/fmicb.2018.03273
Received: 04 October 2018; Accepted: 17 December 2018;
Published: 09 January 2019.
Edited by:
Martin Hoenigl, University of California, San Diego, United StatesReviewed by:
Jeffrey Jenks, University of California, San Diego, United StatesCopyright © 2019 Herrera and Husain. This is an open-access article distributed under the terms of the Creative Commons Attribution License (CC BY). The use, distribution or reproduction in other forums is permitted, provided the original author(s) and the copyright owner(s) are credited and that the original publication in this journal is cited, in accordance with accepted academic practice. No use, distribution or reproduction is permitted which does not comply with these terms.
*Correspondence: Sabina Herrera, c2FiaW5haGVycmVyYW1kQGdtYWlsLmNvbQ== Shahid Husain, c2hhaGlkLmh1c2FpbkB1aG4uY2E=
Disclaimer: All claims expressed in this article are solely those of the authors and do not necessarily represent those of their affiliated organizations, or those of the publisher, the editors and the reviewers. Any product that may be evaluated in this article or claim that may be made by its manufacturer is not guaranteed or endorsed by the publisher.
Research integrity at Frontiers
Learn more about the work of our research integrity team to safeguard the quality of each article we publish.