- 1Department of Microbiology, Center of Sciences Biological, Universidade Estadual de Londrina, Londrina, Brazil
- 2Institut Armand-Frappier, Institut National de la Recherche Scientifique, Laval, QC, Canada
- 3Avian Health Laboratory & Technical Innovation, Institute of Veterinary Research Desiderio Finamor (IPVDF), Eldorado do Sul, Rio Grande do Sul, Brazil
Pathogenic Escherichia coli found in humans and poultry carcasses harbor similar virulence and resistance genes. The present study aimed to analyze the distribution of extraintestinal pathogenic E. coli (ExPEC) virulence factors (VF), blaCTX−M groups, fosA3, and mcr-1 genes in E. coli isolated from commercialized chicken carcasses in southern Brazil and to evaluate their pathogenic risk. A total of 409 E. coli strains were isolated and characterized for genes encoding virulence factors described in ExPEC. Results of antimicrobial susceptibility testing confirmed that the strains were resistant to β-lactams, fosfomycin, colistin, and others resistance groups. The highest prevalence of VFs was observed in isolates belonging to the CTX-M groups, especially the CTX-M-2 group, when compared to those in other susceptible strains or strains with different mechanisms of resistance. Furthermore, ESBL strains were found to be 1.40 times more likely to contain three to five ExPEC virulence genes than non-ESBL strains. Our findings revealed the successful conjugation between ESBL-producing E. coli isolated from chicken carcass and the E. coli recipient strain J53, which suggested that genetic determinants encoding CTX-M enzymes may have originated from animals and could be transmitted to humans via food chain. In summary, chicken meat is a potential reservoir of MDR E. coli strains harboring resistance and virulence genes that could pose serious risks to human public health.
Introduction
Humans and warm-blooded animals naturally harbor bacteria in their intestines, such as Escherichia coli, which is usually a non-pathogenic commensal bacterium. However, E. coli could cause extraintestinal diseases, including urinary tract infection, septicemia and meningitis in humans or even colibacillosis in poultry, which is attributed to the acquisition of virulence factors (VFs) (Müller et al., 2016).
Extraintestinal pathogenic E. coli (ExPEC) strains are characterized by several VF, including adhesins, invasins, protectins, and toxins, as well as several uptake systems for essential nutrients, such as iron (iron-uptake systems) (Johnson et al., 2008b). Commensal and pathogenic E. coli can be classified under different phylogenetic groups, since the VF found in each of the varieties are distributed differently (Clermont et al., 2000). Most commensal strains belong to phylogenetic group A or B1, and ExPEC strains, which harbor more VFs than commensal strains, are assigned to phylogenetic group B2 or D (Tenaillon et al., 2010; Cyoia et al., 2015).
In addition to VFs, the spread of resistance elements among human pathogens may be related to the Enterobacteriaceae family, in which E. coli belongs. Among the Gram-negative bacteria that are resistant to antibiotics, those that produce CTX-M-type ESBLs represent a serious public health concern worldwide (Xie et al., 2016). In particular, most commonly detected CTX-M groups include CTX-M-1, CTX-M-2, CTX-M-8, CTX-M-9, and CTXM-25 (Saravanan et al., 2018).
The detection of plasmidial genes that are mainly related to antimicrobial resistance to fosfomycin and colistin represents another major health concern (Sato et al., 2013; McGann et al., 2016). Fosfomycin is used to treat urinary tract infections (UTI) that are mostly caused by Gram-negative and Gram-positive bacteria, which are highly prevalent in North America (Giancola et al., 2017), and have recently received research attention because of the rapid spread of multidrug-resistance. This resistance is related to a novel gene called fosA3, which has been reported in E. coli and Klebsiella pneumonia and is often detected in blaCTX−M -producing and multidrug-resistant E. coli both in animals and in clinical isolates (Ho et al., 2013). Colistin is prescribed for the treatment of UTI and has been associated with many cases of resistance worldwide. Furthermore, renewed attention has been paid to the mcr-1 gene because it has been detected not only in clinical isolates but also in animal, food, and environmental samples (Fernandes et al., 2016; McGann et al., 2016; Rapoport et al., 2016; Skov and Monnet, 2016; Zeng et al., 2016).
Pathogenic E. coli found in humans and poultry carcass were found to harbor similar virulence and resistance genes in the plasmids (Stromberg et al., 2017). These findings raise the possibility that E. coli present in the intestinal tract of healthy individuals could acquire those genes from E. coli derived from chicken meat, which could act as a reservoir for bacteria harboring resistance genes (Manges and Johnson, 2012). Therefore, present study aimed to analyze the distribution of ExPEC VFs, blaCTX−M groups, and the fosA3 and mcr-1 genes in E. coli isolated from chicken carcasses commercialized in southern Brazil (States of Paraná-PR, Santa Catarina-SC, and Rio Grande do Sul-RS).
Materials and Methods
Bacterial Isolates
Escherichia coli strains were isolated in the Basic and Applied Bacteriology Laboratory at Londrina State University (Biosafety level 2) from 98 commercial refrigerated chicken carcass (35 chicken carcasses from PR, 23 chicken carcasses from SC, and 40 chicken carcasses from RS), sold in southern Brazil from 2013 to 2014. Each chicken carcass was rinsed into the sterile packaging with 100 mL of Brain Heart Infusion (Himedia Laboratories Pvt. Ltd., Mumbai, India). After homogenization, 0.1 mL of the mixture was smeared onto MacConkey agar (Neogen Corporation Lansing, Michigan) and Violet Red Bile Lactose agar (Oxoid Ltd., Basingstoke, Hants, UK) by the pour plate method. Colonies suspected to be E. coli were confirmed by biochemical testing using EPM-MILi and Simmons Citrate agar (PROBAC, Brazil). After biochemical confirmation, one to five strains were collected from each chicken carcass and subsequently analyzed for the genotypic characteristics of ExPEC virulence factors and phenotypic resistance. Only strains that showed difference in those characteristics were selected for further analysis.
Antimicrobial Susceptibility Test
Antimicrobial susceptibility testing was performed using the standard disk diffusion method recommended by the Clinical and Laboratory Standards Institute (CLSI, 2015). The following antimicrobial agents were used in the study: 5 μ g of ciprofloxacin; 10 μg of each of ampicillin, gentamicin, norfloxacin, and enrofloxacin; 30 μg of each of cefazolin, cefotaxime, cefoxitin, ceftazidime, tetracycline, nalidixic acid, and chloramphenicol; 300 μg of nitrofurantoin; 1.25/23.75μg of trimethoprim-sulfamethoxazole; 200 μg of fosfomycin; and 20/10 μg of amoxicillin-clavulanic acid (Oxoid Ltd., Basingstoke, Hants, UK). Strains resistant to third-generation cephalosporins were confirmed for ESBL production by double-disk diffusion testing between amoxicillin/clavulanate and cefotaxime or ceftazidime (Jacoby and Han, 1996) or by conducting a combination disc test using cefotaxime, cefotaxime + clavulanic acid (Becton Dickinson, Sparks, MD), ceftazidime, and ceftazidime + clavulanic acid (Becton Dickinson, Sparks, MD), following the CLSI recommendations. The positive strains in the phenotypic tests to ESBL production were screened for ESBL genes, and the strains resistant to fosfomycin were screened for the fosA3 gene. The E. coli isolate ATCC 25922 was used as a quality control during antimicrobial susceptibility testing. Results were interpreted based on the CLSI criteria.
Detection of Antimicrobial Resistance Genes
ESBL-producing E. coli was characterized for ESBL genes encoding CTX-M (groups 1, 2, 8, 9, and 25), TEM, and SHV by Polymerase Chain Reaction (PCR) (Arlet and Philippon, 1991; Bedenić et al., 2001; Woodford et al., 2006). The presence of acquired fosfomycin resistance genes such as fosA3 was determined by PCR using specific primer sets (Sato et al., 2013). The strains were additionally tested for the presence of colistin resistance gene mcr-1 by PCR (Liu et al., 2016). PCR amplicons were visualized on 2.0% agarose gels stained with GelRed (Biotium, Hayward, CA, USA). After gel electrophoresis, the images were captured using Image Capture Systems (LPixImageHE).
Conjugation Experiments
To verify whether the plasmid harboring blaCTX−M resistance genes could be transferred between E. coli strains, the horizontal-transfer efficiencies of the blaCTX−M genes were assessed by performing conjugation experiments between three selected strains harboring blaCTX−M resistance genes. Volumes of cultures of each donor (ESBL-producing E. coli isolated from chicken carcass) and azide-resistant E. coli J53, recipient strain grown in Luria-Bertani broth (Difco Laboratories, Detroit, Mich) were mixed and incubated for 18–24 h at 37°C. Transconjugants were then selected on MacConkey agar containing 2 μg/mL cefotaxime (Sigma Chemical Co., St. Louis, MO) and 100 μg/mL sodium azide (Sigma Chemical Co., St. Louis, MO) and subsequently used for phylogenetic analysis and testing for the presence of blaCTX−M genes (Xie et al., 2016).
Phylogenetic Classification
E. coli strains were assigned to phylogenetic groups (A, B1, B2, or D) by PCR (Clermont et al., 2000). Each PCR reaction contained 1.25 U of Taq DNA polymerase (Life technologies, Rockville, MD) in 1 × PCR buffer (Life Technologies, Rockville, MD), 0.2 mM each dNTP, 2.5 mM MgCl2, and 1 μM each primer. PCR amplicons were visualized on 2.0% agarose gels stained with GelRed (Biotium, Hayward, CA, USA). After gel electrophoresis, the images were captured using Image Capture Systems (LPixImageHE).
Virulence Genes
We surveyed five VF genes that are normally studied in ExPEC strains. The selected genes included: iutA (aerobactin siderophore receptor gene), hlyF (putative avian hemolysin), iss (episomal increased serum survival gene), iroN (salmochelin siderophore receptor gene), and ompT (episomal outer membrane protease gene) (Johnson et al., 2008a). Each PCR reaction contained 1.25 U of Taq DNA polymerase (Life Technologies, Rockville, MD) in 1 × PCR buffer (Life Technologies, Rockville, MD), 0.2 mM each dNTP, 2.5 mM MgCl2, and 1 μM each primer. PCR amplicons were visualized on 2.0% agarose gels stained with GelRed (Biotium, Hayward, CA, USA). After gel electrophoresis, the images were captured using Image Capture Systems (LPixImageHE).
Statistical Analysis
Frequencies of ExPEC virulence genes in ESBL-producing and non-ESBL-producing strains were compared by Fisher's exact test and Pearson's Chi-square test. The risk of ESBL-producing E. coli harboring more ExPEC genes than non-ESBL-producing E. coli at 95% confidence interval (95% CI) was determined by calculating the relative risk (RR). Statistically significant differences were considered at p < 0.05. The test was performed using the statistical software R version 3.5.1.
Results
Antimicrobial Resistance of E. coli From Poultry Carcasses
A total of 409 E. coli isolates from chicken carcasses from southern Brazil were tested. Among these, 121, 135, and 153 were isolated from carcasses from the PR, SC, and RS states. Results of the antimicrobial susceptibility test indicated that strains from chicken carcasses showed a high frequency of antimicrobial resistance, in total 66% of the isolates were resistant to antibiotics. We identified multidrug-resistant E. coli strains from chicken carcasses from PR, SC and RS (82, 53, and 80%, respectively). The most common antimicrobial agents for which strains were found to be resistant included tetracycline (68.77%), nalidixic acid (67.61%), and ampicillin (68.77%). The ESBL phenotype was confirmed for 119 isolates (~32% of PR, 31% of SC, and 35% of RS) of the 409 strains isolated from commercial refrigerated chicken carcasses, which represents 29.1% of all isolates. Furthermore, ESBL-producing E. coli were found to be more resistant to a higher number of antimicrobials (p < 0.05) compared to non-ESBL-producing E. coli (Figure 1). Of the 409 E. coli strains tested, 99.3% were classified as susceptible to fosfomycin, whereas none showed intermediate resistance and three strains (0.70%) showed resistance to fosfomycin.
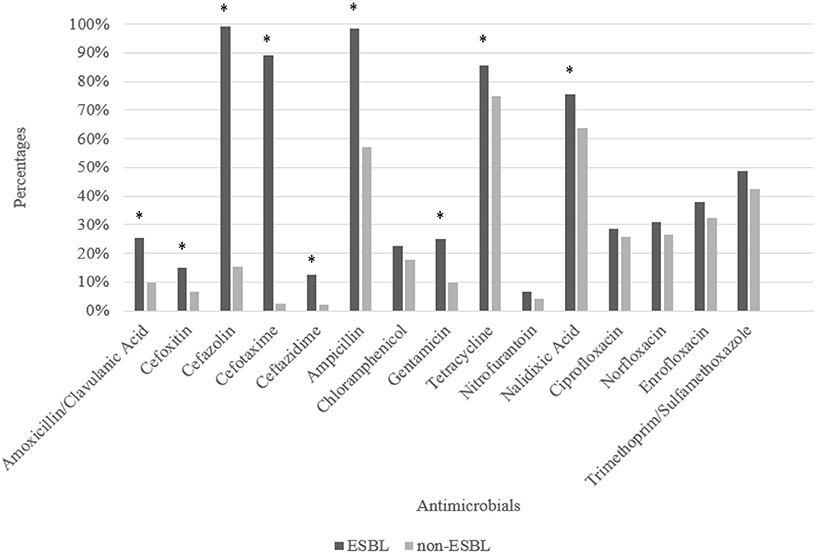
Figure 1. Percentage resistance exhibited by ESBL-producing E. coli strains and non-ESBL-producing E. coli strains isolated from commercial chicken carcasses in southern Brazil from 2013 to 2014. *p < 0.05 by Pearson's Chi-square test.
Detection of Antimicrobial Resistance Genes
The majority of ESBL-producing E. coli isolates (32.23%) were collected from the PR state, while the RS state showed the lowest number of ESBL-producing E. coli isolates (27.45%). Out of the 119 ESBL strains, 97 harbored the blaCTX−M gene, six harbored CTX-M-1 group, 61 harbored CTX-M-2 group, and 30 harbored CTX-M-8 group (Table 1 and Figure 2). The CTX-M-9 group and CTX-M-25 group were not detected in the strains (Figure 2). The remaining E. coli strains harbored the blaSHV (7.56%) and blaTEM (10.08%) genes (Figure 2).
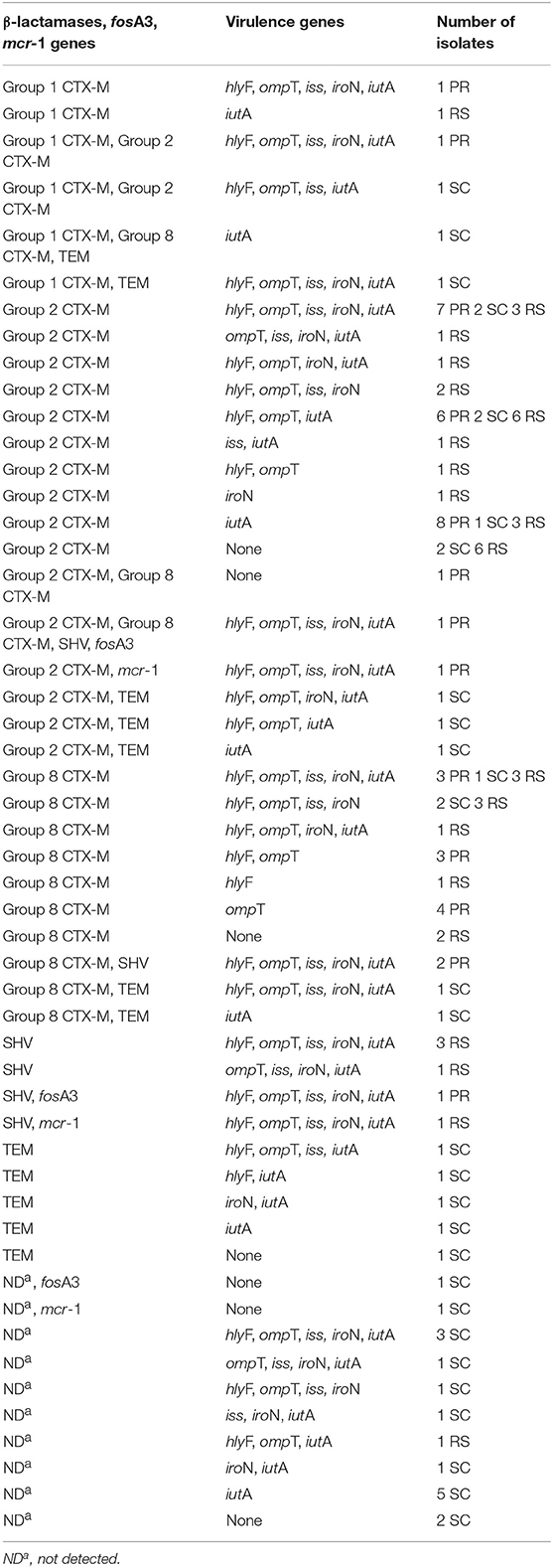
Table 1. Distribution of resistance and virulence genes among 119 ESBL-producing E. coli strains isolated from chicken carcasses commercialized in Brazil.
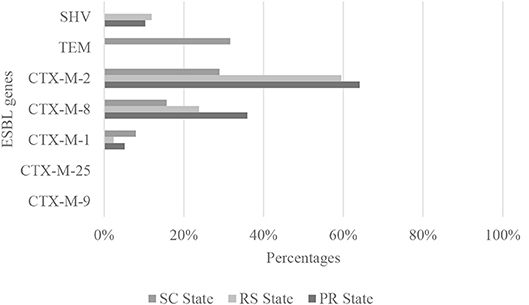
Figure 2. Distribution of ESBL genes encoding CTX-Ms, TEM, and SHV detected in E. coli strains isolated from commercial chicken carcasses in southern Brazil from 2013 to 2014.
Fosfomycin resistance was identified based on phenotypic tests and subsequently confirmed by PCR. The three fosfomycin-resistant strains that harbored the fosA3 gene were found to be blaCTX−M positive (3.33%). PCR analysis of the 119 ESBL-producing E. coli isolates revealed that 2.50% of the isolates harbored genes encoding resistance to colistin, corresponding to one resistant strain from each state (PR, SC, and RS). Furthermore, these strains were ESBL-producing E. coli, and two of these strains harbored five ExPEC virulence genes tested in the present study (iss, iroN, iutA, hlyF, and ompT) (Table 1) and were assigned to different phylogenetic groups (A, B2, and B1).
Conjugation Experiments
Among the blaCTX−M positive E. coli isolates tested that belonged to phylogenetic group B1, all strains successfully transferred their cefotaxime resistance phenotypes to the E. coli recipient strain J53 via conjugation.
Phylogenetic Classification
Phylogenetic analysis revealed that most of the E. coli strains belonged to group B1 (36.6%), followed by groups A (31.7%), D (28.1%), and B2 (3.40%) (Table 2). The determination of E. coli phylogenetic groups showed that the majority of the 119 ESBL-producing E. coli belonged to phylogenetic group D (36.06%), followed by a nearly even distribution of the remaining three phylogenetic groups, namely, B1 (31.97%), A (27.63%), and B2 (4.22%) (Table 2).
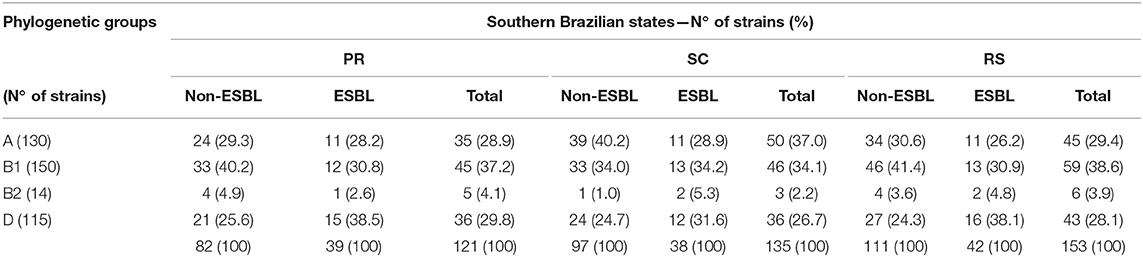
Table 2. Phylogenetic distribution of 290 non-ESBL-producing E. coli strains and 119 ESBL-producing E. coli strains isolated from chicken carcasses from different southern Brazilian states.
Virulence Genes
ExPEC VFs were identified in the various E. coli strains. Among the 409 E. coli strains analyzed, the prevalence of individual ExPEC VF genes ranged from 33.3% (iss, an episomal increased serum survival gene) to 51.6% (iutA, an aerobactin siderophore receptor gene). Results indicated that 58% of ESBL-producing E. coli harbored three to five ExPEC virulence genes (Table 1).
The highest prevalence of ExPEC VFs was observed in strains harboring CTX-M resistance relative to other susceptible strains or even strains with different mechanisms of resistance (p < 0.01). The relative risk for ESBL strains that did not contain any ExPEC genes was 0.35 (95 % CI, 0.21–0.57; p < 0.01). On the other hand, the RR for ESBL strains harboring three or more ExPEC genes was 1.40 (95 % CI, 1.13–1.73; p < 0.01) (Table 3). For each non-ESBL strain harboring three or more ExPEC virulence genes (Supplementary Material), there are 1.40 ESBL strains harboring three or more ExPEC virulence genes (RR>1). For example, in the PR state, the iutA gene was present in 54% of the E. coli isolates, and present in 80% of the blaCTX−M producing E. coli. Similar results were observed in the other two states for all five virulence genes.
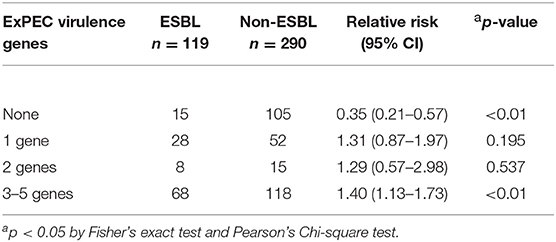
Table 3. Risk factor analysis indicating that ESBL-producing E. coli harbor more virulence genes than non-ESBL-producing E. coli.
Discussion
In the present study, we analyzed a total of 409 E. coli strains from commercial chicken carcasses in Brazil isolated from 2013 to 2014. About 71% of isolates were MDR (Magiorakos et al., 2012), which demonstrate the high antimicrobial resistance. Our current findings are consistent with reports from other countries, which detected MDR in Gram negative bacteria from chicken meat in Italy (66.9% resistant) and India (79.6% resistant) (Ghodousi et al., 2015; Shrestha et al., 2017). In the states of PR and RS, approximately 80% of carcasses were found to be contaminated with E. coli that were resistant to three or more antimicrobial groups, whereas the rates of resistance in the state of SC were slightly lower (53%). The higher rates of antimicrobial resistance and MDR in strains could be due to environmental contamination with antibiotic residues in aviculture industries and/or selective pressure caused by the indiscriminate use of antimicrobial compounds as a result of poor monitoring by regulatory bodies (Koga et al., 2015). Importantly, some growth promoters, such as poultry feeds, have been prohibited in animal production in several countries, like in Brazil since 1998 (Brasil Ministério da Agricultura, 2003, 2009).
Almost 30% of the isolates analyzed in the present study were found to be resistant to β-lactams and thus represent a potential health concern. The resistant E. coli harbored genes encoding ESBL enzymes that hydrolyze penicillins, cephalosporins, and monobactams and were inhibited by treatment with “classical” β-lactamase inhibitors such as clavulanic acid, sulbactam, and tazobactam (Bevan et al., 2017; Saravanan et al., 2018). Notably, ESBL-producing E. coli showed stronger resistance to others antimicrobials, such as aminoglycosides, quinolones, and tetracyclines, when compared to non-ESBL-producing E. coli (p < 0.05), further promoting the health risks due to consumption of undercooked meat or the handling or preparation of uncooked poultry products contaminated with resistant strains (Shrestha et al., 2017; Saravanan et al., 2018). CTX-M ß-lactamases are the most widespread type of ESBL and have been identified since the mid-2000s and were specifically detected in clinical isolates of E. coli (Bush, 2018). ESBL-producing bacteria have been increasingly detected in meat from food-producing animals such as, poultry (Ghodousi et al., 2015; Shrestha et al., 2017; Poirel et al., 2018). Our findings have raised significant concerns, since the 30% prevalence of ESBL-producing samples in chicken carcasses in southern Brazil was higher than those reported in other regions, as in USA (27%), in India (21%) and in other samples from Brazil (7%) (Freeman et al., 2009; Datta et al., 2014; Gonçalves et al., 2016). Among all ESBL strains, we found 97% classified as blaCTX−M and the majority belonged to CTX-M-2 group, although the rates varied depending on the region worldwide. Recent studies reported the presence of the CTX-M-1 resistance genes in E. coli strains from poultry meat from Sweden (54–58%), Belgium (62%), Canada (66.2%), Italy (8.9%), and Japan (34%) (Smet et al., 2010; Denisuik et al., 2013; Brolund et al., 2014; Ghodousi et al., 2015; Nahar et al., 2018). However, CTX-M-9 represented the most prevalent group in reports of ESBL E. coli from Spain (Garrido et al., 2014), Portugal (Fernandes et al., 2014), Japan (Nahar et al., 2018), and Italy (Ghodousi et al., 2015).
One important finding from the current study is the successful conjugation between ESBL-producing E. coli isolated from chicken carcass to the E. coli recipient strain J53, which suggest that genetic determinants encoding CTX-M enzymes could be conjugative. According to Xie et al. (2016), commensal B1 strains isolated from food-producing animals could act as reservoirs of ESBL genes, which could be disseminated to human bacteria via the food chain, thereby raising a significant public health concern (Leverstein-van Hall et al., 2011; Xie et al., 2016; Poirel et al., 2018). Furthermore, resistance conferred by ESBLs is often associated with resistance to other classes of antibiotics, such as trimethoprim-sulfamethoxazole, aminoglycosides, and fluoroquinolone (Coque et al., 2008; Zeng and Lin, 2017). Therefore, the transfer of CTX-M mobile plasmids are likely to be accompanied by acquisition of other resistance genes. Some studies reported that plasmid-mediated fosfomycin resistance is frequently detected among CTX-M-producing E. coli isolated from food-producing animals (Sato et al., 2013; Xie et al., 2016). During sample collection in 2013, fosfomycin was not commonly used in animal production because of its high cost; nevertheless, 3% of the strains tested positive for the presence of the fosA3 gene.
The use of polymyxins (colistin) in food-producing animals, especially in feed additives, represents another health concern. One colistin-resistant E. coli strain harboring five ExPEC virulence genes was detected in each of the southern Brazilian states. Several recent studies have also suggested the possibility of transfer of the mcr-1 gene to humans via the food chain (Carnevali et al., 2016; Wang et al., 2017). Although the current results indicated a very low presence of the mcr-1 gene, other studies indicated that the higher prevalence of colistin resistance could be attributed to the widespread use of colistin in food production in recent years (Huang et al., 2017). Thus, the use of fosfomycin and colistin in food production, such as in poultry, could lead to a public health concern, considering that these antimicrobials are used for the treatment of extraintestinal infections in humans. Therefore, similar to colistin, fosfomycin should also be banned from animal production in many countries.
Current evidence indicates that E. coli isolated from chickens and human ExPECs, harbor highly similar virulence genes, thereby suggesting a potential risk to cause diseases in humans (Manges and Johnson, 2012). A higher number of virulence factors present in ExPEC indicates a link to pathogenicity (Pitout, 2012). Furthermore, studies demonstrated an association between ExPEC virulence factors and phylogenetic groups. Intestinal E. coli isolates belonging to groups A and B1 harbor fewer ExPEC virulence genes, and ExPECs strains belonging to groups B2 and D contain a higher number of virulence genes (Koga et al., 2015; Müller et al., 2016; Pavlickova et al., 2017). Consistent with previous studies, most E. coli strains isolated from chicken carcasses harbor three to five ExPEC virulence genes (33–51%, varying between the five genes) and belonged to phylogenetic group B1 (36%), which represents a group of more multi-resistant commensal strains (Koga et al., 2015; Müller et al., 2016). Among these strains, 58% of ESBL-producing E. coli harbored three to five ExPEC virulence genes. Most of these strains were associated with phylogenetic group D, unlike non-ESBL-producing E. coli, which were associated with group B1. These rates are high compared to 28% of ExPEC isolated from patients mostly with UTIs in southern Brazil (Cyoia et al., 2015) or very similar to those reported in APEC strains (Mohamed et al., 2018), thereby indicating that some ESBL-producing E. coli strains from poultry meat are potentially pathogenic.
Importantly, blaCTX−M ESBL-producing E. coli strains were found to harbor a higher number of ExPEC virulence genes relative to other susceptible strains or even strains that were resistant to other groups of antimicrobials (p < 0.01). In addition, ESBL strains are 1.40 times more likely to contain three to five ExPEC virulence genes than non-ESBL strains, which in turn increases their risk for pathogenic potential (RR = 1.40, 95% CI, 1.13–1.73; p < 0.01). The above findings suggest that E. coli present in chicken meat, which could act as a reservoir for these antimicrobial resistance and virulence genes could be a potential risk for colonization and/or transfer of this resistance to bacteria in the intestinal tracts of humans.
Despite the importance of identifying ESBL-producing E. coli belonging to phylogenetic group D, which is commonly associated with strains found in hospitals and ambulatory patients (Pietsch et al., 2017), the detection of commensal strains from group B1 is also notable. Although transferable isolates belonging to phylogenetic group B1 do not comprise the most virulent phylogenetic group (such as B2 or D), these strains still harbor both virulence and resistance genes. Therefore, chicken meat could serve as an important reservoir for resistance genes and could be responsible for the spread of MDR bacteria via the food chain.
Conclusion
Our results highlight the high prevalence of ExPEC virulence genes and antimicrobial resistance genes associated with chicken meat. Brazil is the largest exporter of chicken meat and the second largest producer of chicken meat worldwide. These findings further represent a public health concern, considering that chicken meat could serve as a reservoir for the spread of plasmids harboring resistance and virulence genes through the food chain. Future studies should investigate whether both, resistance and virulence genes are transferred together to other bacteria and determine whether they are present in the same plasmid.
Author Contributions
PC contributed to the development of experimental research, data analysis, and preparation of the article. VK, BB, and KB contributed to the development of experimental research. EN contributed to the statistical analysis. RK, GN, KB, BB, SH, and CD contributed to and assisted in the design of the work, assisted in critical data interpretation, and in preparation of the article. All authors have participated in this study and commented on the manuscript.
Conflict of Interest Statement
The authors declare that the research was conducted in the absence of any commercial or financial relationships that could be construed as a potential conflict of interest.
Acknowledgments
This study was supported by grants from Coordenação de Aperfeiçoamento do Pessoal de Ensino Superior (CAPES). Thanks are also due to Fundação Araucária for the use of financial facilities (Chamada Pública CP 09/2016—Protocol 10748).
Supplementary Material
The Supplementary Material for this article can be found online at: https://www.frontiersin.org/articles/10.3389/fmicb.2018.03254/full#supplementary-material
References
Arlet, G., and Philippon, G. (1991). Construction by polymerase chain reaction and use of intragenic DNA probes for three main types of transferable beta-lactamase (TEM, SHV, CARB) [corrected]. FEMS Microbiol Lett. 66, 19–25.
Bedenić, B., Randegger, C., Stobberingh, E., and Hachler, H. (2001). Molecular epidemiology of extended-spectrum β-lactamases from Klebsiella pneumoniae strains isolated in Zagreb, Croatia. Eur. J. Clin. Microbiol Infect. Dis. 20, 505–508. doi: 10.1007/PL00011293
Bevan, E. R., Jones, A. M., and Hawkey, P. M. (2017). Global epidemiology of CTX-M β-lactamases: temporal and geographical shifts in genotype. J. Antimicrob. Chemother. 72, 2145–2155. doi: 10.1093/jac/dkx146
Brasil Ministério da Agricultura (2003). “Pecuária e abastecimento,” in 27 De Junho de 2003. Proíbe a Fabricacão, a Manipulacão, o Fracionamento, a Comercializacão, a Importacão e o Uso dos Princípios Ativos Cloranfenicol e Nitrofuranos e os Produtos que Contenham estes Princípios Ativos, Para uso Veterinário e Susceptível de Emprego na Alimentacão de Todos os Animais e Insetos. Instrucão Normativa (Portuguese). Available online at: http://www.agricultura.gov.br/arqeditor/file/CRC/IN%2009-2003%20%20Proibe%20uso%20de%20cloranfenicol%20e%20nitrofuranos
Brasil Ministério da Agricultura (2009). “Pecuaria e abastecimento,” in Regulamento Tecnico Para a Fabricacao, o Controle de Qualidade, a Comercializacao e o Emprego de Produtos Antimicrobianos de Uso Veterinario. Instrucao Normativa 26, Ministerio da Agricultura, Pecuaria e Abastecimento Brasilia (Portuguese). Available online at: http://sistemasweb.agricultura.gov.br/sislegis/action/detalhaAto.do?method=visualizarAtoPortalMapa&chave=1984822284
Brolund, A., Edquist, P. J., Makitalo, B., Olsson-Liljequist, B., Soderblom, T., Wisell, K. T., et al. (2014). Epidemiology of extended-spectrum beta-lactamase producing Escherichia coli in Sweden 2007–2011. Clin. Microbiol. Infect. 20, 344–352. doi: 10.1111/1469-0691.12413
Bush, K. (2018). Past and present perspectives on β-lactamases. Antimicrob. Agents Chemother. 62, e01076–e01018. doi: 10.1128/AAC.01076-18
Carnevali, C., Morganti, M., Scaltriti, E., Bolzoni, L., Pongolini, S., and Casadei, G. (2016). Occurrence of mcr-1 in colistin-resistant Salmonella enterica isolates recovered from humans and animals in Italy, 2012 to 2015. Antimicrob. Agents Chemother. 60, 7532–7534. doi: 10.1128/AAC.01803-16
Clermont, O., Bonacorsi, S., and Bingen, E. (2000). Rapid and simple determination of the Escherichia coli phylogenetic group. Appl. Environ. Microbiol. 66, 4555–4558. doi: 10.1128/AEM.66.10.4555-4558.2000
CLSI (2015). Performance Standards for Antimicrobial Susceptibility Testing. Twenty-Fifth Informational Supplement CLSI Document M100-S25. Wayne, PA: Clinical and Laboratory Standards Institute.
Coque, T. M., Baquero, F., and Canton, R. (2008). Increasing prevalence of ESBL producing Enterobacteriaceae in Europe. Euro Surveill. 13:19051.
Cyoia, P. S., Rodrigues, G. R., Nishio, E. K., Medeiros, L. P., Koga, V. L., Pereira, A. P., et al. (2015). Presence of virulence genes and pathogenicity islands in extraintestinal pathogenic Escherichia coli isolates from Brazil. J. Infect. Dev. Ctries. 9, 1068–1075. doi: 10.3855/jidc.6683
Datta, P., Gupta, V., Sidhu, S., and Chander, J. (2014). Community Urinary Tract Infection due to ESBL producing E. coli: epidemiology and susceptibility to oral antimicrobials including Mecillinam. Nepal J. Med. Sci. 3, 5–7. doi: 10.3126/njms.v3i1.10341
Denisuik, A. J., Lagace-Wiens, P. R., Pitout, J. D., Mulvey, M. R., Simner, P. J., Tailor, F., et al. (2013). Molecular epidemiology of extended-spectrum betalactamase., AmpC beta-lactamase- and carbapenemase-producing Escherichia coli and Klebsiella pneumoniae isolated from Canadian hospitals over a 5 year period: CANWARD 2007–11. J. Antimicrob. Chemother. 68, i57–65. doi: 10.1093/jac/dkt027
Fernandes, M. R., Moura, Q., Sartori, L., Silva, K. C., Cunha, M. P., Esposito, F., et al. (2016). Silent dissemination of colistin resistant Escherichia coli in South America could contribute to the global spread of the mcr-1 gene. Euro Surveill. 21:30214. doi: 10.2807/1560-7917.ES.2016.21.17.30214
Fernandes, R., Amador, P., Oliveira, C., and Prudencio, C. (2014). Molecular characterization of ESBL-producing Enterobacteriaceae in northern Portugal. Sci. World J. 2014:782897. doi: 10.1155/2014/782897
Freeman, J. T., Sexton, D. J., and Anderson, D. J. (2009). Emergence of extended-Spectrum b-lactamase–producing Escherichia coli in community hospitals throughout North Carolina: a harbinger of a wider problem in the United States?. Clin. Infect. Dis. 49, 30–32. doi: 10.1086/600046
Garrido, A., Seral, C., Gude, M. J., Casado, C., Gonzalez-Dominguez, M., Saenz, Y., et al. (2014). Characterization of plasmid-mediated beta-lactamases in fecal colonizing patients in the hospital and community setting in Spain. Microb. Drug Resist. 20, 301–304. doi: 10.1089/mdr.2013.0109
Ghodousi, A., Bonura, C., Di Noto, A. M., and Mammina, C. (2015). Extended-Spectrum ß-lactamase, AmpC-producing and fluoroquinolone-resistant E. coli in retail broiler chicken meat, Italy. Food Pathog. Dis. 12:619. doi: 10.1089/fpd.2015.1936
Giancola, S. E., Mahoney, M. V., Hogan, M. D., Raux, B. R., McCoy, C., and Hirsch, E. B. (2017). Assessment of Fosfomycin for complicated or multidrug-resistant urinary tract infection: patient characteristics and outcomes. Chemotherapy 62, 100–104. doi: 10.1159/000449422
Gonçalves, L. F., Martins-Junior, P. O., Melo, A. B. F., Silva, R. C. R. M., Martins, V. P., Pitondo-Silva, A., et al. (2016). Multidrug resistance dissemination by extended-spectrum beta-lactamase-producing Escherichia coli causing community acquired urinary tract infection in the Central-Western Region, Brazil. J. Global Antimicrob. Resist. 6, 1–4. doi: 10.1016/j.jgar.2016.02.003
Ho, P. L., Chan, J., Lo, W. U., Law, P. Y., Li, Z., Lai, E. L., et al. (2013). Dissemination of plasmid-mediated fosfomycin resistance fosA3 among multidrug-resistant Escherichia coli from livestock and other animals. J. Appl. Microbiol. 114, 695–702. doi: 10.1111/jam.12099
Huang, X., Yu, L., Chen, X., Zhi, C., Yao, X., Liu, Y., et al. (2017). High prevalence of colistin resistance and mcr-1 gene in Escherichia coli isolated from food animals in China. Front. Microbiol. 8:562. doi: 10.3389/fmicb.2017.00562
Jacoby, G. A., and Han, P. (1996). Detection of extended-spectrum β-lactamases in clinical isolates of Klebsiella pneumoniae and Escherichia coli. J. Clin. Microbiol. 34, 908–911.
Johnson, T. J., Wannemuehler, Y., Doetkott, C., Johnson, S. J., Rosenberger, S. C., and Nolan, L. K. (2008a). Identification of minimal predictors of avian pathogenic Escherichia coli virulence for use as a rapid diagnostic tool. J. Clin. Microbiol. 46, 3987–3996. doi: 10.1128/JCM.00816-08
Johnson, T. J., Wannemuehler, Y., Johnson, S. J., Stell, A. L., Doetkott, C., et al. (2008b). Comparison of extraintestinal pathogenic Escherichia coli strains from human and avian sources reveals a mixed subset representing potential zoonotic pathogens. Appl. Environ. Microbiol. 74, 7043–7050. doi: 10.1128/AEM.01395-08
Koga, V. L., Scandorieiro, S., Vespero, E. C., Oba, A., de Brito, B. G., de Brito, K. C. T., et al. (2015). Comparison of antibiotic resistance and virulence factors among Escherichia coli isolated from conventional and free-range poultry. Biomed Res. Int. 2015:618752. doi: 10.1155/2015/618752
Leverstein-van Hall, M. A., Dierikx, C. M., Cohen Stuart, J., Voets, G. M., van den Munckhof, M. P., van Essen-Zandbergen, A., et al. (2011). Dutch patients, retail chicken meat and poultry share the same ESBL genes, plasmids and strains. Clin. Microbiol. Infect. 17, 873–880. doi: 10.1111/j.1469-0691.2011.03497.x
Liu, Y. Y., Wang, Y., Walsh, T. R., Yi, L. X., Zhang, R., Spencer, J., et al. (2016). Emergence of plasmid-mediated colistin resistance mechanism MCR-1 in animals and human beings in China: a microbiological and molecular biological study. Lancet Infect. Dis.16, 161–168. doi: 10.1016/S1473-3099(15)00424-7
Magiorakos, A. P., Srinivasan, A., Carey, R. B., Carmeli, Y., Falagas, M. E., Giske, C. G., et al. (2012). Multidrug-resistant, extensively drug-resistant and pandrug-resistant bacteria: an international expert proposal for interim standard definitions for acquired resistance. Clin. Microbiol. Infect. 18, 268–281. doi: 10.1111/j.1469-0691.2011.03570.x
Manges, A. R., and Johnson, J. R. (2012). Food-borne origins of Escherichia coli causing extraintestinal infections. Clin. Infect. Dis. 55, 712–719. doi: 10.1093/cid/cis502
McGann, P., Snesrud, E., Maybank, R., Corey, B., Ong, A. C., Clifford, R., et al. (2016). Escherichia coli harboring mcr-1 and blaCTX-M on a novel IncF plasmid: first report of mcr-1 in the United States. Antimicrob. Agents Chemother. 60, 4420–4421. doi: 10.1128/AAC.01103-16
Mohamed, L., Zhao, G., Li, Y., Gao, Y., Kaidi, R., Oumouna, M., et al. (2018). Virulence traits of avian pathogenic (APEC) and fecal (AFEC) E. coli isolated from broiler chickens in Algeria. Trop. Anim. Health Prod. 50, 547–553. doi: 10.1007/s11250-017-1467-5
Müller, A., Stephan, R., and Nüesch-Inderbinen, M. (2016). Distribution of virulence factors in ESBL-producing Escherichia coli isolated from the environment, livestock, food and humans. Sci. Tot. Environ. 541, 667–672. doi: 10.1016/j.scitotenv.2015.09.135
Nahar, A., Awasthi, S. P., Hatanaka, N., Okuno, K., Hoang, P. H., Hassan, J., et al. (2018). Prevalence and characteristics of extended-spectrum B-lactamase-producing Escherichia coli in domestic and imported chicken meats in Japan. J. Vet. Med. Sci. 80, 510–517. doi: 10.1292/jvms.17-0708
Pavlickova, S., Klancnik, A., Dolezalova, M., Mozina, S. S., and Holko, I. (2017). Antibiotic resistance, virulence factors and biofilm formation ability in Escherichia coli strains isolated from chicken meat and wildlife in the Czech Republic. J. Environ. Sci. Health 52, 570–576. doi: 10.1080/03601234.2017.1318637
Pietsch, M., Eller, C., Wendt, C., Holfelder, M., Falgenhauer, L., Fruth, A., et al. (2017). Molecular characterisation of extended-spectrum β-lactamase (ESBL)-producing Escherichia coli isolates from hospital and ambulatory patients in Germany. Vet. Microb. 200, 130–137. doi: 10.1016/j.vetmic.2015.11.028
Pitout, J. D. (2012). Extraintestinal pathogenic Escherichia coli: a combination of virulence with antibiotic resistance. Front. Microbiol. 3:9. doi: 10.3389/fmicb.2012.00009
Poirel, L., Madec, J.-Y., Lupo, A., Schink, A.-K., Kieffer, N., Nordmann, P., et al. (2018). Antimicrobial resistance in Escherichia coli. Microbiol Spectrum. 6:ARBA-0026-2017. doi: 10.1128/microbiolspec.ARBA-0026-2017
Rapoport, M., Faccone, D., Pasteran, F., Ceriana, P., Albornoz, E., and Petroni, A. (2016). First Description of mcr-1-mediated colistin resistance in human infections caused by Escherichia coli: first description in Latin America. Antimicrob. Agents Chemother. 60, 4412–4413. doi: 10.1128/AAC.00573-16
Saravanan, M., Ramachandran, B., and Barabadi, H. (2018). The prevalence and drug resistance pattern of extended spectrum β-lactamases (ESBLs) producing Enterobacteriaceae in Africa. Microb. Pathog. 114, 180–192. doi: 10.1016/j.micpath.2017.11.061
Sato, N., Kawamura, K., Nakane, K., Wachino, J., and Arakawa, Y. (2013). First detection of fosfomycin resistance gene fosA3 in CTX-M-producing Escherichia coli isolates from healthy individuals in Japan. Microb. Drug Resist. 19, 477–482. doi: 10.1089/mdr.2013.0061
Shrestha, A., Bajracharya, A. M., Subedi, H., Turha, R. S., Kafle, S., Sharma, S., et al. (2017). Multi-drug resistance and extended spectrum beta lactamase producing Gram negative bacteria from chicken meat in Bharatpur Metropolitan, Nepal. BMC Res. Notes 10:574. doi: 10.1186/s13104-017-2917-x
Skov, R. L., and Monnet, D. L. (2016). Plasmid-mediated colistin resistance (mcr-1 gene): three months later, the story unfolds. Euro Surveill. 21:30155. doi: 10.2807/1560-7917.ES.2016.21.9.30155
Smet, A., Martel, A., Persoons, D., Dewulf, J., Heyndrickx, M., Claeys, G., et al. (2010). Characterization of extended-spectrum beta-lactamases produced by Escherichia coli isolated from hospitalized and nonhospitalized patients: emergence of CTX-M-15-producing strains causing urinary tract infections. Microb. Drug Resist. 16, 129–134. doi: 10.1089/mdr.2009.0132
Stromberg, Z. R., Johnson, J. R., Fairbrother, J. M., Kilbourne, J., Van Goor, A., Curtiss, R., et al. (2017). Evaluation of Escherichia coli isolates from healthy chickens to determine their potential risk to poultry and human health. PLoS ONE 12:0180599. doi: 10.1371/journal.pone.0180599
Tenaillon, O., Skurnik, D., Picard, B., and Denamur, E. (2010). The population genetics of commensal Escherichia coli. Nat. Rev. Microbiol. 8, 207–217. doi: 10.1038/nrmicro2298
Wang, Y., Zhang, R., Li, J., Wu, Z., Yin, W., Schwarz, S., et al. (2017). Comprehensive resistome analysis reveals the prevalence of NDM and MCR-1 in Chinese poultry production. Nat. Microbiol. 2:16260. doi: 10.1038/nmicrobiol.2016.260
Woodford, N., Fagan, E. J., and Ellington, M. J. (2006). Multiplex PCR for rapid detection of genes encoding CTX-M extended-spectrum β-lactamases. J. Antimicrob. Chemother. 57, 154–155. doi: 10.1093/jac/dki412
Xie, M., Lin, D., Chen, K., Chan, E. W. C., Yao, W., and Chen, S. (2016). Molecular characterization of Escherichia coli strains isolated from retail meat that harbor blaCTX-M and fosA3 Genes. Antimicrob. Agents Chemother. 60, 2450–2455. doi: 10.1128/AAC.03101-15
Zeng, K. J., Doi, Y., Patil, S., Huang, X., and Tian, G. B. (2016). Emergence of plasmid-mediated mcr-1 gene in colistin-resistant Enterobacter aerogenes and Enterobacter cloacae. Antimicrob. Agents Chemother. 60, 3862–3863. doi: 10.1128/AAC.00345-16
Keywords: ESBL, multidrug-resistance, phylogenetic groups, CTX-M, fosfomycin
Citation: Cyoia PS, Koga VL, Nishio EK, Houle S, Dozois CM, Brito KCT, Brito BG, Nakazato G and Kobayashi RKT (2019) Distribution of ExPEC Virulence Factors, blaCTX-M, fosA3, and mcr-1 in Escherichia coli Isolated From Commercialized Chicken Carcasses. Front. Microbiol. 9:3254. doi: 10.3389/fmicb.2018.03254
Received: 01 August 2018; Accepted: 14 December 2018;
Published: 14 January 2019.
Edited by:
Gilberto Igrejas, University of Trás-os-Montes and Alto Douro, PortugalReviewed by:
Alain Hartmann, Institut National de la Recherche Agronomique (INRA), FranceMagaly Toro, Universidad de Chile, Chile
Copyright © 2019 Cyoia, Koga, Nishio, Houle, Dozois, Brito, Brito, Nakazato and Kobayashi. This is an open-access article distributed under the terms of the Creative Commons Attribution License (CC BY). The use, distribution or reproduction in other forums is permitted, provided the original author(s) and the copyright owner(s) are credited and that the original publication in this journal is cited, in accordance with accepted academic practice. No use, distribution or reproduction is permitted which does not comply with these terms.
*Correspondence: Renata Katsuko Takayama Kobayashi, kobayashirkt@uel.br