- 1State Key Laboratory of Microbial Technology, Microbial Technology Institute, Shandong University, Qingdao, China
- 2Advanced Research Center for Optics, Shandong University, Qingdao, China
Salmonella genomic island 1 (SGI1) is a resistance-conferring chromosomal genomic island that contains an antibiotic resistance gene cluster. The international spread of SGI1-containing strains drew attention to the role of genomic islands in the dissemination of antibiotic resistance genes in Salmonella and other Gram-negative bacteria. In this study, five SGI1 variants conferring multidrug and heavy metal resistance were identified and characterized in Proteus mirabilis strains: SGI1-PmCAU, SGI1-PmABB, SGI1-PmJN16, SGI1-PmJN40, and SGI1-PmJN48. The genetic structures of SGI1-PmCAU and SGI1-PmABB were identical to previously reported SGI1s, while structural analysis showed that SGI1-PmJN16, SGI1-PmJN40, and SGI1-PmJN48 are new SGI1 variants. SGI1-PmJN16 is derived from SGI1-Z with the MDR region containing a new gene cassette array dfrA12-orfF-aadA2-qacEΔ1-sul1-chrA-orf1. SGI1-PmJN40 has an unprecedented structure that contains two right direct repeat sequences separated by a transcriptional regulator-rich DNA fragment, and is predicted to form two different extrachromosomal mobilizable DNA circles for dissemination. SGI1-PmJN48 lacks a common ORF S044, and its right junction region exhibits a unique genetic organization due to the reverse integration of a P. mirabilis chromosomal gene cluster and the insertion of part of a P. mirabilis plasmid, making it the largest known SGI1 to date (189.1 kb). Further mobility functional analysis suggested that these SGIs can be excised from the chromosome for transfer between bacteria, which promotes the horizontal transfer of antibiotic and heavy metal resistance genes. The identification and characterization of the new SGI1 variants in this work suggested the diversity of SGI1 structures and their significant roles in the evolution of bacteria.
Introduction
Salmonella genomic island 1 (SGI1) is a genomic island containing an antibiotic resistance gene cluster initially identified in Salmonella enterica serovar Typhimurium phage type DT104 strain (Boyd et al., 2001). The multidrug resistance (MDR) region in SGI1 is a complex In4-type class 1 integron, which contains five antibiotic resistance genes conferring resistance to ampicillin (blaPSE−1), chloramphenicol and florfenicol (floR), streptomycin and spectinomycin (aadA2), sulfonamides (sul1), and tetracycline [tet(G)] (Boyd et al., 2001). Since the identification of SGI1 in S. enterica Typhimurium DT104, SGI1 variants with high genetic diversities on the MDR regions and backbones were found in other S. enterica serovars (Levings et al., 2005), Proteus mirabilis (Ahmed et al., 2006), and Morganella morganii (Schultz et al., 2017). From a classification perspective, based on differences of antibiotic resistance gene clusters located at MDR regions, SGI1 was classified from SGI1-A to SGI1-Z (Boyd et al., 2002, 2008; Levings et al., 2007; Doublet et al., 2009; Bi et al., 2011; Le Hello et al., 2012; Lei et al., 2014, 2015). Additionally, six SGI variants (SGI1-B, SGI1-K, SGI1-P, SGI1-Q, SGI1-L, and SGI1-J) were subdivided into two or more subgroups (Levings et al., 2005, 2008; Doublet et al., 2008, 2009; Chu et al., 2012; Lei et al., 2015).
In recent years, three SGI1-related elements, SGI2, Proteus genomic island (PGI1/PGI2) and Acinetobacter genomic island 1 (AGI1), were reported in S. enterica, P. mirabilis and Acinetobacter baumannii, respectively (Levings et al., 2008; Siebor and Neuwirth, 2014; Hamidian et al., 2015; Lei et al., 2018). All these four genomic islands integrate into the 3′-end of chromosomal trmE gene and carry diverse antibiotic resistance genes in their MDR regions (Hall, 2010; Hamidian et al., 2015; Lei et al., 2018). Of particular interest, SGI1-V with extended-spectrum β-lactamase (ESBL) gene (blaVEB−6) and PGI1-PmPEL carrying carbapenemase gene (blaNDM−1) in MDR region were detected in clinical P. mirabilis strains (Siebor and Neuwirth, 2011; Girlich et al., 2015). The emergence of these strains carrying SGI1/PGI1 with ESBL gene and/or carbapenemase gene is of great concern to public health, as β-lactams and carbapenems remain the most widely used antibiotics for the treatment of bacterial infection.
It has been reported that the transcriptional activator complex AcaCD, whose coding genes are carried by IncA/C type plasmids, triggers the excision and conjugative transfer of SGI1/PGI1 (Kiss et al., 2015). SGI1/PGI1 could further be mobilized and transferred into a broad range of Enterobacteriaceae with the help of conjugative IncA/C plasmids (Siebor et al., 2016), suggesting that SGI1s/PGI1s could act as mobilizable elements for the dissemination of resistance genes. The transfer of these MDR genomic islands (GIs) increased the level of antimicrobial resistance among Enterobacteriaceae, and the GI-facilitated horizontal gene transfer contributed to the diversification and adaptation of microorganisms, therefore having an impact on the genome plasticity and evolution of bacteria (Juhas et al., 2009).
Proteus mirabilis has been recognized to be the causative agent of a variety of opportunistic nosocomial infections, and it is especially associated with urinary tract infections. It has become a potential public health concern in recent years (Cohn et al., 2003). Since the detection of a SGI1 variant in P. mirabilis in 2006, the number of reported SGI1 variants in P. mirabilis isolates from clinical, animal, or food in China and France has been increasing (such as SGI1-O, SGI1U-Z, SGI1-B2, SGI1-PmBRI, SGI1-PmCAU, and SGI1-PmABB) (Boyd et al., 2008; Bi et al., 2011; Siebor and Neuwirth, 2013; Lei et al., 2014, 2015; Girlich et al., 2015; Qin et al., 2015). These findings lead to a strong suggestion that P. mirabilis may serve as a host for mobilizable genetic elements and facilitates the dissemination of antimicrobial resistance.
In the current study, new SGI1 variants were characterized in multidrug resistant P. mirabilis strains and their genetic structures were mapped. These findings improve our understanding of the diversity of SGI1 structures and the prevalence of antibiotic resistant genomic island in P. mirabilis.
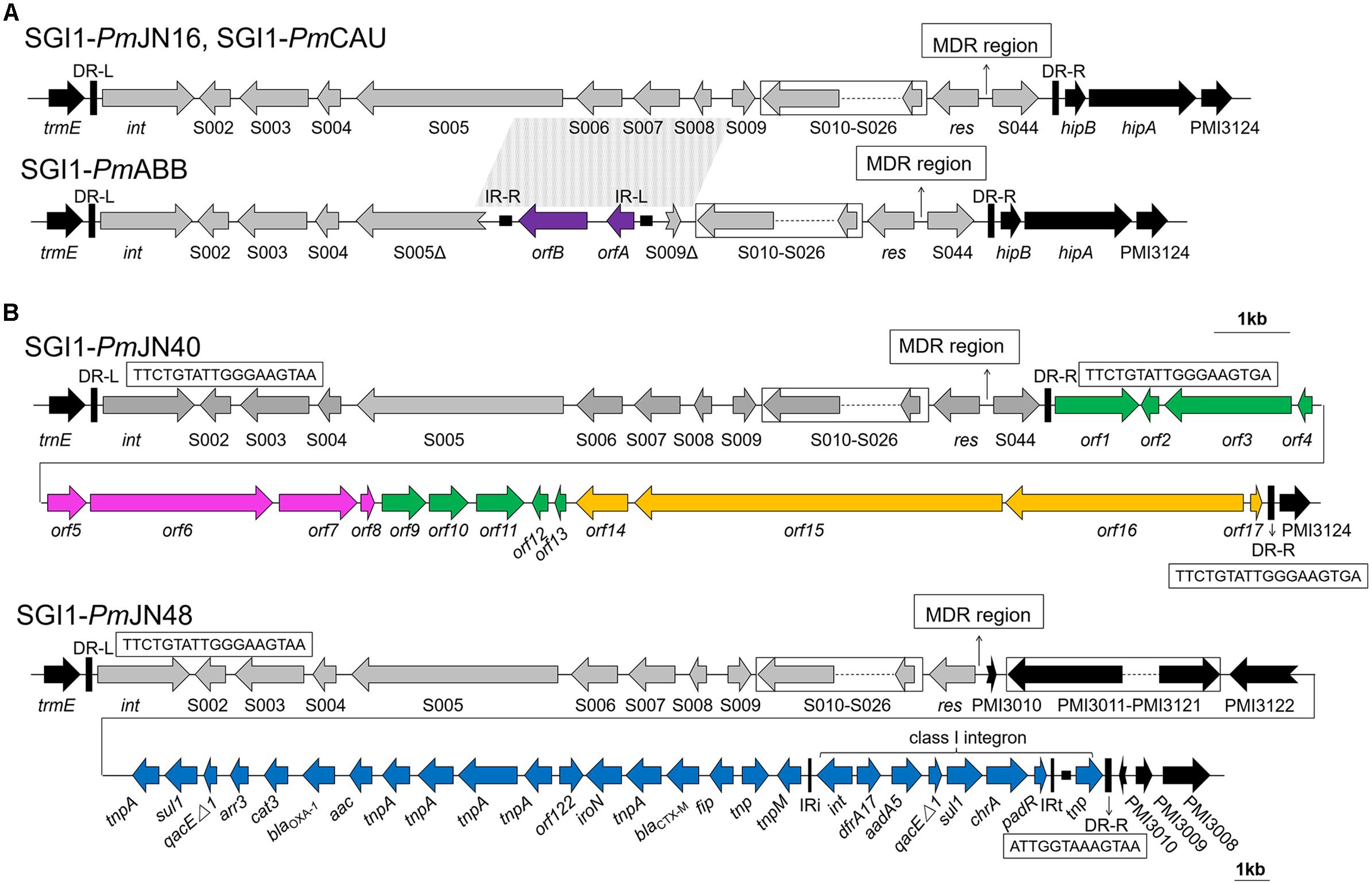
Figure 1. Backbone structures of SGI1s identified in this work. (A) Backbone structures of SGI1-PmCAU, SGI1-PmABB, and SGI1-PmJN16. (B) Backbone structures of SGI1-PmJN40 and SGI1-PmJN48. SGI1 backbones are shown with different colored arrows based on their origins: SGI1 conserved backbone (gray), IS1356 (purple), chromosomal DNA of Vibrio parahaemolyticus (green), chromosomal DNA of Shewanella bicestrii (pink), chromosomal DNA of Photobacterium damselae (orange), pPm14C18 (blue), and chromosomal DNA of Proteus mirabilis (black). The direct repeats of SGI1 (DR-L/DR-R) and inverted repeats of class I integron (IRi/IRt) are indicated by thick vertical bars and thin vertical bars, respectively. The small filled squares represent inverted repeats of the IS. The position of the MDR regions is indicated by thin arrows. Truncated genes are shown by swallowtail arrows.
Materials and Methods
Sampling and Bacterial Isolation
During the time period from June to September 2013, fifty-seven antibiotic-resistant P. mirabilis strains were isolated from a commercial broiler slaughter plant in Shandong Province, China. Sterile, moistened swabs were used to wipe 2 cm2 areas from the surfaces and insides of 107 eviscerated broiler chicken carcasses by the method of Wu et al. (2015). Swabs were agitated in 10 mL of sterile saline (0.9% NaCl), and the suspensions were serially diluted. A 100 μL aliquot of each dilution was spread onto eosin methylene blue (EMB) plates (AOBOX, Beijing, China) that were supplemented with one of the following six antimicrobial agents. Antibiotics used for screening these strains included amoxicillin–clavulanic acid (32/16 mg/L), ciprofloxacin (4 mg/L), amikacin (32 mg/L), doxycycline (16 mg/L), sulbactam–cefoperazone (64/64 mg/L), and sulfamethoxazole–trimethoprim (4/76 mg/L). The isolated strains were resistant to at least one of the antibiotics used. Genomic DNA was extracted from the bacteria using bacteria genome extraction kit (GeneRay, Shanghai, China).
Plasmid
The IncA/C type pR55 plasmid capable of mediating conjugative transfer of SGI1s is a kind gift from Prof. Hongning Wang from Sichuan University (Douard et al., 2010; Doublet et al., 2012). The plasmid is harbored by an Escherichia coli C600 strain.
Antimicrobial Susceptibility Assay
The antimicrobial sensitivity phenotypes of P. mirabilis strains were determined by the disk diffusion method using Müller–Hinton agar plates following CLSI guidelines M100-S26 (CLSI, 2016). The following antimicrobial agents were used: ceftazidime (CAZ, 30 mg/L), ampicillin (AMP, 10 mg/L), cefotaxime (CTX, 30 mg/L), nalidixic acid (NAL, 30 mg/L), streptomycin (STR, 10 mg/L), kanamycin (KAN, 30 mg/L), tetracycline (TET, 30 mg/L), trimethoprim (TMP, 5 mg/L), chloramphenicol (CHL, 30 mg/L), sulfisoxazole (SFX, 250 mg/L), erythromycin (ERY, 15 mg/L), rifampin (RIF, 5 mg/L), and imipenem (IPM, 10 mg/L). E. coli ATCC 25922 was used as a quality control.
Detection and Sequencing of SGI1s
Salmonella genomic island 1 and its chromosomal location were detected using primers targeting the left junction (primer pairs PmLJ1/LJ-R1), right junction (primer pairs 104-RJ/PmRJ1), and ORF S026 (primer pairs S026-F/S026-R) in the chromosome. The structures of SGI1s were analyzed by PCR-mapping and sequencing DNA fragments using the corresponding primers described in Supplementary Table S1.
The special right junction regions of SGI1-PmJN40 and SGI1-PmJN48 were further obtained by genome sequencing of SGI1-containing P. mirabilis strains using Illumina MiSeq (Illumina Inc., San Diego, CA, United States) sequencing platform with a 400-bp paired-end library, as well as SOAPdevono v2.04 and GapCloser v1.12 software to construct de novo assemblies (Luo et al., 2012). Gaps between scaffolds were closed by PCR followed by sequencing.
Bioinformatics
The complete nucleotide sequences of SGI1s were analyzed using the BLAST algorithm1. The GenBank accession numbers for SGI1-PmCAU, SGI1-PmABB, SGI1-PmJN40, SGI1-PmJN16, and SGI1-PmJN48 are JX089581.1 (Siebor and Neuwirth, 2013), JX121638.1 (Siebor and Neuwirth, 2013), MF576128, MF576129, and MF576130, respectively.
Chromate Resistance Assays
Chromate resistance was measured by the comparison of growth rates in LB media. Overnight cultures were diluted 2000-fold into 50-mL flasks containing 25 mL of fresh medium supplemented with different chromate (K2CrO4) concentrations. The bacterial suspensions were incubated at 37°C with shaking at 170 rpm for 12 h, and the value of OD600 was measured (Alvarez et al., 1999; Branco et al., 2008).
Stability Test for SGI1
Ten microliter cultures of all the five SGI1-positive P. mirabilis strains were serially transferred into 3 mL LB medium (300 × dilution per passage), grown at 37°C under vigorous shaking without antibiotic selection. A total of 24 passages over 192 h were performed for stability test. Cultures from the final passage were plated on LB agar plates without antibiotic selection, and one hundred clones were picked from each strain for the detection of presence/absence of SGI1 by PCR reactions.
Conjugative Transfer Test for SGI1
The pR55 helper plasmid harbored by E. coli C600 was transferred to SGI1-containing P. mirabilis via conjugation prior to testing the transferability of SGI1s identified in this work. Briefly, pR55-containing E. coli C600 was grown to late-log phase, and mixed together with the recipient SGI1-carrying P. mirabilis strains at a ratio of 4:1. The mixture was subsequently incubated at 37°C overnight without shaking, diluted 10-fold, and plated on selective MacConkey agar plates containing chloramphenicol (30 mg/L) and trimethoprim (30 mg/L).
To test whether SGI1s found in this work can be mobilized to another bacterium via conjugative transfer, we mixed pR55+SGI1-containing P. mirabilis donor strains with the sodium azide-resistant E. coli recipient strain J53 following procedures described above. Sodium azide (200 mg/L) and trimethoprim (30 mg/L)/streptomycin (30 mg/L) were used to select transconjugants. The SGI1 frequency of transfer was determined by dividing the number of E. coli SGI1 transconjugants by the number of P. mirabilis donor cells, as previously described (Douard et al., 2010).
The transconjugants were examined for the presence of SGI1-specific genes with PCR reactions, in order to confirm the conjugative transfer of SGI1s from respective P. mirabilis donor strains to the recipient E. coli J53 strain. The primers used for screening SGI1-positive transconjugants are listed in Supplementary Table S1.
Results
Detection of SGI1s in Multidrug Resistant P. mirabilis
The general structure and mechanism of site-specific integration for SGI1 in P. mirabilis can be depicted in a model shown in Supplementary Figure S1. The left junction region of SGI1s as well as ORF S026 were detected in 5 out of 57 multidrug resistant P. mirabilis strains (JN16, JN29, JN40, JN47, and JN48) isolated from a commercial broiler slaughterhouse. Interestingly, P. mirabilis JN40 and JN48 were negative for the right junction with primers 104-RJ (S044 specific) and PmRJ1 (hipB specific) (Supplementary Figure S2). The right junction regions of these two SGI1s were then further analyzed by PCR with primer pairs 104-RJ/hipA-R1 (hipA specific) and 104-RJ/MP-R1 (specific for the membrane protein PMI3124-encoding gene) that respectively, target the two genes downstream of hipB on the chromosome of P. mirabilis HI4320 (Siebor and Neuwirth, 2013). However, the right junction regions were still undetected in P. mirabilis JN40 and JN48 strains using the above primers (Supplementary Figure S2). These results suggested a different right junction structure of SGI1s in P. mirabilis JN40 and JN48 in comparison with the conventional right junction region.
Sequencing of SGI1 Variants
The complete sequences of SGI1s in P. mirabilis JN16, JN29, and JN47 were obtained by PCR mapping and sequencing of the backbones and MDR regions using primers listed in Supplementary Table S1. For SGI1s containing unconventional right junction structures, whole genome sequencing of their host strains (P. mirabilis JN40 and JN48) was performed to obtain their full length sequences. Complete nucleotide sequences of SGI1s were analyzed using the BLAST algorism1. The genetic organization of SGI1 in P. mirabilis JN29 and JN47 was identical to the previously reported SGI1-PmCAU and SGI1-PmABB, respectively (Siebor and Neuwirth, 2013), while SGI1s identified in P. mirabilis JN16, JN40, and JN48 were not previously observed (Table 1). According to the nomenclature system of SGI1 (Mulvey et al., 2006), these three new SGI1s were subsequently denominated SGI1-PmJN16, SGI1-PmJN40, and SGI1-PmJN48.
Characterization of the SGI1 Backbones
The structures of the SGI1 backbones are displayed in Figure 1. Sequence analysis of SGI1-PmCAU, SGI1-PmABB, SGI1- PmJN16, and SGI1-PmJN40 showed that their left direct repeat (DR-L) was nearly identical to the right direct repeat (DR-R). As shown in Figure 1A, SGI1-PmCAU and SGI1-PmJN16 contain the conventional backbone regions (S001–S027 and S044) as previously reported (Lei et al., 2015). In SGI1-PmABB, the region spanning from ORF S005 to ORF S009 was deleted (2780 bp), and replaced by an insertion of IS1359 of the IS3 family initially described in Vibrio cholera (GenBank accession number EU664602) (Doublet et al., 2009). SGI1-PmCAU, SGI1-PmABB and SGI1-PmJN16 integrated between the chromosomal genes trmE and hipB/hipA, but not for SGI1-PmJN40 and SGI1PmJN48.
SGI1-PmJN40 found in P. mirabilis JN40 displays a unique and unprecedented backbone structure that contain one DR-L and two identical DR-Rs (Figure 1B). Present between the two DR-Rs is a 23.7 kb fragment (from orf1 to orf17) that is a combination of V. parahaemolyticus (GenBank accession number CP026041.1), Shewanella bicestrii (GenBank accession number CP022358.1), and Photobacterium damselae (GenBank accession number CP021151.1) genomic DNA (Figure 1B). This DNA fragment contains genes encoding transcriptional regulators (orf4 and orf12), DNA binding proteins (orf2 and orf7) and toxin-antitoxin (orf5 and orf8). The transcriptional regulators encoded by orf4 and orf12 belong to AlpA family phage regulatory protein and XRE family transcriptional regulator, respectively. It has been reported that AlpA-type regulators are positive regulatory factors of integrase in the P4-like prophage (Kirby et al., 1994). In addition, The XRE-type regulators were shown to function as a repressor of conjugative transfer and participate in regulation of excision and transfer of genomic island (López-Fuentes et al., 2015). The two DNA binding proteins encoded by SGI1-PmJN40 may function in binding to promoter regions for transcriptional regulation, although they cannot be immediately classified as transcriptional regulators. Furthermore, TA systems contribute to the maintenance of genetic elements by reducing growth, inhibiting growth or killing a subpopulation of cells (Hernández-Arriaga et al., 2014; Marsan et al., 2017). Therefore, it can be inferred that these functional genes may play a potential regulatory role. The presence of two identical DR-Rs in SGI1-PmJN40 suggests its potential to form two different mobilizable DNA species in the form of free circles, respectively, denominated SGI1-PmJN40-S (from int to S044) and SGI1-PmJN40-L (from int to orf17). Considering the potential regulatory role of the genes between two DR-Rs (from orf1 to orf17), the larger circle (SGI1-PmJN40-L) may have a regulatory function.
SGI1-PmJN48 is a new SGI1 variant with a special genetic organization in its right junction region. ORF S044 was absent in SGI1-PmJN48 and replaced by the insertion of a large gene fragment containing P. mirabilis chromosomal DNA (PMI3010-PMI3122), followed by a gene cluster identical to part of a P. mirabilis plasmid pPm14C18 (GenBank accession number KU605240) (Figure 1B). Considering the existence of an imperfect direct repeat (ATTGGTAAAGTAA) in the downstream of the insertion (Figure 1B), we propose that the insertion from PMI3010 to padR/tnp was part of SGI1-PmJN48. The size of SGI1-PmJN48 is 189.1 kb, which is the longest SGI1 identified to date.
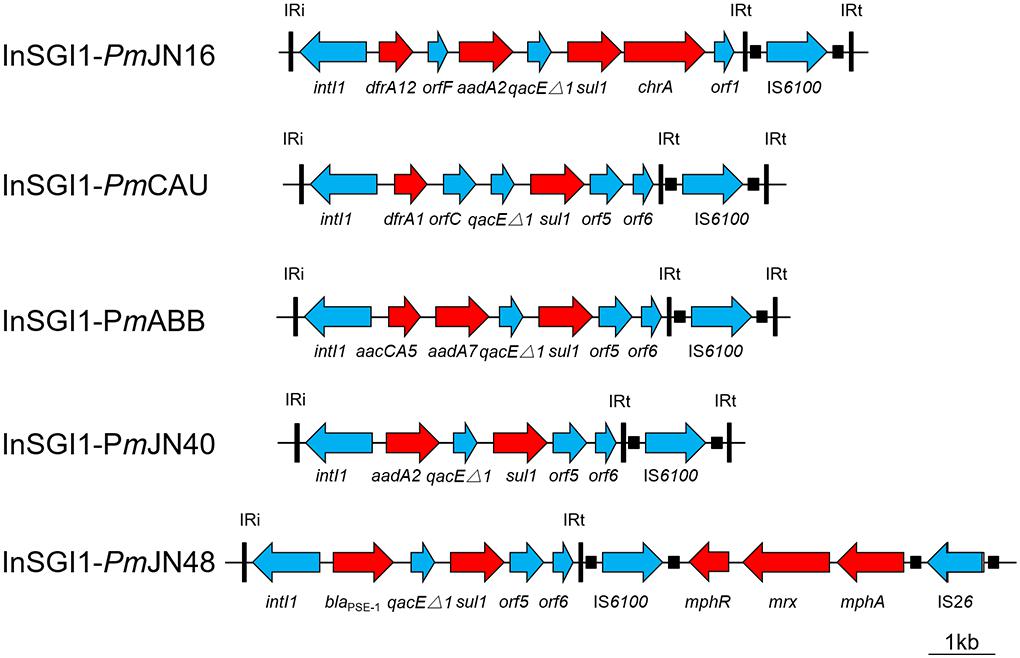
Figure 2. Schematic view of the MDR region within the SGI1 of P. mirabilis. Antibiotic resistance genes are indicated by red arrows. Thin vertical bars represent the inverted repeats of class I integron (IRi/IRt). Small filled squares represent the inverted repeats of the IS.
Characterization of the MDR Regions
The complex genetic structures of MDR regions in five SGI1s were determined. All the MDR regions include a class 1 integron belonging to the In4 family as previously reported (Boyd et al., 2001; Ahmed et al., 2006). InSGI1-PmCAU, InSGI1-PmABB and InSGI1-PmJN40 contain the dfrA1-orfC, aacCA5-aadA7 and aadA2 gene cassettes in their variable regions, respectively (Figure 2). The resistance phenotypes mediated by these drug resistance genes of the SGI1-containing bacteria are shown in Table 1.
InSGI1-PmJN16 has a unique MDR structure that has not been observed previously in SGI1. It contains a dfrA12-orfF-aadA2-qacEΔ1-sul1-chrA-orf1 gene cassette. The gene cassette array dfrA12-orfF-aadA2-qacEΔ1-sul1 was similar with the MDR region of SGI1-Z identified in P. mirabilis (Qin et al., 2015). However, the sul1 gene in InSGI1-PmJN16 was followed by the chrA-orf1 gene cassette developing a new structure of SGI1 MDR. It is noteworthy that the chrA gene is expected to confer chromate resistance by encoding a chromate transport protein (Alvarez et al., 1999). In order to confirm this resistance, we tested the chromate resistance of SGI1-PmJN16-positive P. mirabilis JN16 using the chrA-negative P. mirabilis JN49 as the negative control (Supplementary Figure S3). The JN16 strain showed significantly higher chromate tolerance than that in the JN49 strain. This result indicated that chrA can indeed encode for chromate resistance.
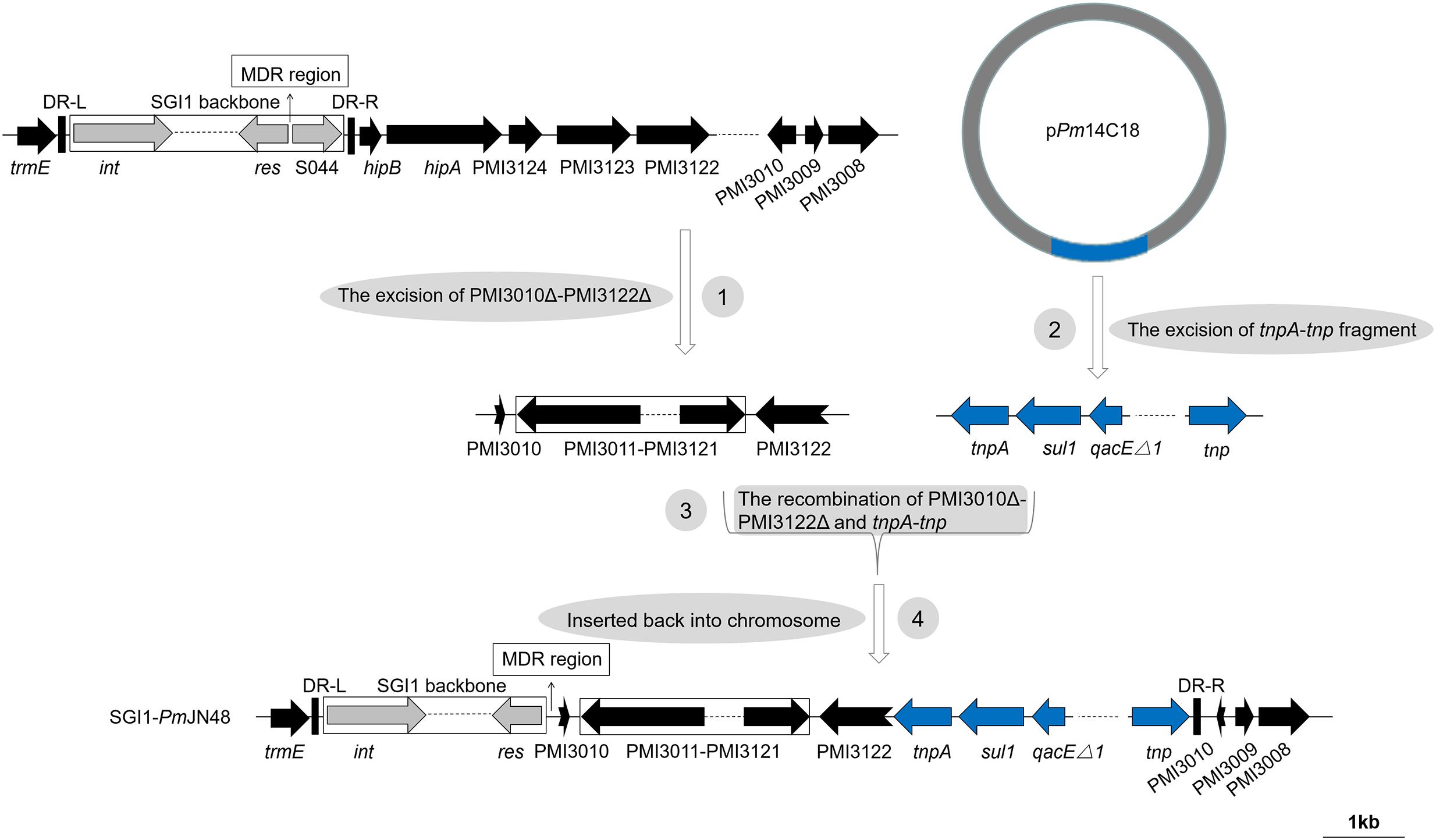
Figure 3. The four-step pathway for the generation of SGI1-PmJN48. SGI1-PmJN48 backbone was shown with different colored arrows: conserved SGI1 backbone (gray), chromosomal DNA of P. mirabilis (black), tnpA-tnp fragment of plasmid pPm14C18 (blue). The direct repeats of SGI1 (DR-L/DR-R) and the position of the MDR regions are indicated by thick vertical bars and thin arrows, respectively. Truncated genes are shown by swallowtail arrows.
SGI1PmJN48 carries two MDR clusters in its conventional MDR region, including blaPSE−1-qacEΔ1-sul1 and mphA-mrx-mphR (Figure 2). The blaPSE−1-qacEΔ1-sul1 gene cassette is part of SGI1-B, while the macrolide inactivation gene cluster mphA-mrx-mphR conferring resistance to erythromycin has been identified in SGI1-B2 (Hall, 2010; Lei et al., 2015). Therefore, the MDR region of SGI1-PmJN48 might derive from the recombination and rearrangement of SGI1-B and SGI1-B2. In addition to these two MDR clusters, other resistance gene clusters were found in SGI1-PmJN48 that were originally part of the plasmid pPm14C18 (GenBank accession number KU605240): arr3-cat3-blaOXA−1-aac, blaCTX−M and part of a class 1 integron (intI1-dfrA17-aadA5-qacEΔ1-sul1-chrA) (Figure 1B). These genes could confer resistance to rifampin, chloramphenicol, β-lactams, aminoglycosides, sulfonamides, quaternary ammonium compound, and chromate, respectively (Alvarez et al., 1999; Partridge et al., 2009). The chromate resistance phenotype of P. mirabilis JN48 that harbors SGI1PmJN48 was described in Supplementary Figure S3, confirming the functionality of the chrA gene.
Mobility and Stability of SGI1
Salmonella genomic island 1s can excise from the chromosome, forming a free circle that could be transferred with the help of IncA/C plasmid (Doublet et al., 2005; Douard et al., 2010). The free circular form of SGI1 has been previously identified in S. enterica and P. mirabilis. The formation of free circle implied the correct excision of SGI1 and the capacity of SGI1 to be transferred by the helper plasmid (Kiss et al., 2015; Lei et al., 2015). In this study, the circular extrachromosomal forms (attP) of SGI1-PmCAU, SGI1-PmJN40-S, SGI1-PmABB and SGI1-PmJN16 were detected by PCR using the circ1/circ2 primers (Supplementary Figures S1, S2), suggesting the mobility of SGI1s.
In order to further confirm the mobility of SGI1s, we performed conjugative transfer tests for SGI1-containing P. mirabilis strains that contain the IncA/C-type pR55 helper plasmid. Primers were designed to detect SGI1s in transconjugants (targeted genes: xis and S044 for all SGI1s; orf4, orf16, and orf17 for SGI1-PmJN40; PMI3015, PMI3046, and chrA for SGI1-PmJN48). We were able to detect the conjugative transfer of SGI1-PmCAU, SGI1-PmJN40-L (large circular form of SGI1-PmJN40), SGI1-PmABB and SGI1-PmJN48 from the donor strains to recipient E. coli J53 strain at frequencies between 10−5 and 10−6, suggesting that these SGI1s are functional and can be transferred between bacterial cells with the help of IncA/C plasmids (Table 2 and Supplementary Figure S4). Unfortunately, we were unable to transfer pR55 to P. mirabilis JN16 that harbors SGI1-PmJN16, therefore weren’t able to further characterize the conjugative transferability of SGI1-PmJN16. Nevertheless, the capability of SGI1-PmJN16 to form circular forms suggests its potential to be transferred to another cell.
The stability of SGI1 in the chromosome of S. enterica and P. mirabilis has been previously described (Kiss et al., 2012; Lei et al., 2015). In order to test the stability of SGI1s found in this study, all five SGI1-containing strains were propagated, lasting for 24 passages and 192 h in the absence of antimicrobial pressure. No SGI1-negative clone was detected from the 500 clones picked (100 clones were picked from each strain) after the final passage, suggesting that SGI1s found in this work are stable in P. mirabilis.
The mobility and stability tests performed on the five SGI1s found in this work suggest that they are stable, that they can excise from the chromosome, and that they can transfer between cells. During the transfer of SGI1s, they can serve as vectors of resistance genes and play a significant role in the dissemination of antibiotic and heavy metal resistance.
Discussion
Five SGI1s were identified and characterized in multidrug resistant P. mirabilis strains, and different antibiotic and heavy metal resistant gene clusters were identified in their MDR regions, suggesting their roles in the conferment and dissemination of resistance. Among the five SGI1s, two SGI1s (SGI1-PmCAU and SGI1-PmABB) are identical to previously identified counterparts, while all remaining three SGI1s (SGI1-PmJN16, SGI1-PmJN40, and SGI1-PmJN48) showed new structures and functions.
SGI1-PmJN16 has a conventional backbone structure and a new MDR region structure (Figures 1, 2). The integron variable region gene cassette array of SGI1-PmJN16 is dfrA12-orfF-aadA2-qacEΔ1-sul1-chrA-orf1 that has not been reported in other SGI1s. This new gene cassette array is a variant of its counterpart in SGI1-Z (dfrA12-orfF-aadA2-qacEΔ1-sul1). Therefore, we propose that SGI1-PmJN16 is derived from previously reported SGI1-Z (Qin et al., 2015) by adding new functional chromate resistance gene chrA, which adds additional resistance feature for this SGI1.
SGI1-PmJN40 is a unique SGI1 that contains two identical DR-Rs. This unprecedented structure suggests its capability to form two mobilizable circular forms via integrase-mediated recombination. The larger SGI1 species (SGI1-PmJN40-L) contains a hybrid DNA fragment of V. parahaemolyticus, S. bicestrii, and P. damselae chromosomal DNA that is rich in regulator-coding genes (orf2, orf4, orf5, orf7, orf8, and orf12) and potentially functions in regulation. While only the smaller circular form of SGI1-PmJN40 (SGI1-PmJN40-S) was directly observed, we were able to detect the conjugative transfer of the large circular form of SGI1-PmJN40 (SGI1-PmJN40-L). Therefore, we hypothesize that both forms are concurrently present and functional in P. mirabilis.
For the S044-lacking SGI1-PmJN48, a large insertion containing P. mirabilis chromosomal DNA (PMI3010-PMI3122 in the inverse orientation) and plasmid-borne gene cluster from pPm14C18 is present (Figure 1B). As shown in Figure 3, a complex four-step event likely took place for the generation of SGI1-PmJN48: PMI3010Δ-PMI3122Δ was excised from the chromosome, recombined with the tnpA-tnp fragment excised from the plasmid pPm14C18, and inserted back into P. mirabilis chromosome by replacing S044-PMI3122Δ. SGI1-PmJN48 contains multiple MDR regions (blaPSE−1-qacEΔ1-sul1, mphA-mrx-mphR, arr3-cat3-blaOXA−1-aac, blaCTX−M, and dfrA17-aadA5-qacEΔ1-sul1-chrA) that confer resistance to a series of antibiotics and heavy metal including erythromycin, rifampin, chloramphenicol, ampicillin, trimethoprim, streptomycin/kanamycin, sulfisoxazole, and chromate (Table 1 and Supplementary Figure S3), making it a strong disseminator of MDR. It is noteworthy that these gene clusters are surrounded by different transposase genes of insertion sequences (Figure 1B), leading to the proposal that the insertion of these resistance genes is mediated by various transposons and ISs. Experimental evidence in this work showed that this large SGI1 can be mobilized and transferred between cells via conjugative transfer, suggesting it is the largest functional SGI1 that has a unique structure, to the best of our knowledge.
The newly identified SGIs in this work give us a better understanding of the genetic diversity of SGI1s: SGI1-PmJN40 is a new type SGI1 that can potentially form two circular species for dissemination; SGI1-PmJN48 contains a very long DNA insert that can potentially form a MDR disseminator with the size of over 150 kb. These new structures are drastically different from previously identified SGI1s, and encourage us to expand our search for more unconventional mobile genetic elements.
Author Contributions
LB and MF performed the experiments. LB, ZL, MW, and HX analyzed the data. LB, MW, and HX wrote the manuscript. ZL critically revised the manuscript. MW and HX conceived of the study. LB, MF, ZL, MW, and HX approved the final manuscript.
Funding
This work was supported by the National Key Research and Development Program of China (2017YFD0400301), the National Natural Science Foundation of China (31770042 and 31770043), Shandong Province Natural Science Foundation (ZR2015EM052), Shandong Province Key Research and Development Program (2016GSF121040 and 2018GSF118008), the Fundamental Research Funds of Shandong University (2017JC028, 2018JC013, and 2018JC027), the State Key Laboratory of Microbial Technology Open Project Funds, Shandong University (M2017-07 and M2018-07), and Jinan Cultural Industry Development Fund.
Conflict of Interest Statement
The authors declare that the research was conducted in the absence of any commercial or financial relationships that could be construed as a potential conflict of interest.
Acknowledgments
The authors wish to thank Novogene Technology Co., Ltd., Beijing, China, for high throughput sequencing. The authors also wish to thank Professor Hongning Wang from Sichuan University for generously donating pR55-containing E. coli C600 strain.
Supplementary Material
The Supplementary Material for this article can be found online at: https://www.frontiersin.org/articles/10.3389/fmicb.2018.03172/full#supplementary-material
Footnotes
References
Ahmed, A. M., Hussein, A. I. A., and Shimamoto, T. (2006). Proteus mirabilis clinical isolate harbouring a new variant of Salmonella genomic island 1 containing the multiple antibiotic resistance region. J. Antimicrob. Chemother. 59, 184–190. doi: 10.1093/jac/dkl471
Alvarez, A. H., Moreno-Sánchez, R., and Cervantes, C. (1999). Chromate efflux by means of the ChrA chromate resistance protein from Pseudomonas aeruginosa. J. Bacteriol. 181, 7398–7400.
Bi, S., Yan, H., Chen, M., Zhang, Z., Shi, L., and Wang, H. (2011). New variant Salmonella genomic island 1-U in Proteus mirabilis clinical and food isolates from South China. J. Antimicrob. Chemother. 66, 1178–1179. doi: 10.1093/jac/dkr030
Boyd, D. A., Cloeckaert, A., Chaslus-Dancla, E., and Mulvey, M. R. (2002). Characterization of variant Salmonella genomic island 1 multidrug resistance regions from serovars typhimurium DT104 and Agona. Antimicrob. Agents Chemother. 46, 1714–1722. doi: 10.1128/AAC.46.6.1714-1722.2002
Boyd, D. A., Peters, G. A., Cloeckaert, A., Boumedine, K. S., Chaslus-Dancla, E., Imberechts, H., et al. (2001). Complete nucleotide sequence of a 43-kilobase genomic island associated with the multidrug resistance region of Salmonella enterica serovar Typhimurium DT104 and its identification in phage type DT120 and serovar Agona. J. Bacteriol. 183, 5725–5732. doi: 10.1128/JB.183.19.5725-5732.2001
Boyd, D. A., Shi, X., Hu, Q., Ng, L. K., Doublet, B., Cloeckaert, A., et al. (2008). Salmonella Genomic Island 1 (SGI1), variant SGI1-I, and new variant SGI1-O in Proteus mirabilis clinical and food isolates from China. Antimicrob. Agents Chemother. 52, 340–344. doi: 10.1128/AAC.00902-07
Branco, R., Chung, A. P., Johnston, T., Gurel, V., Morais, P., and Zhitkovich, A. (2008). The chromate-inducible chrBACF operon from the transposable element TnOtChr confers resistance to chromium (VI) and superoxide. J. Bacteriol. 190, 6996–7003. doi: 10.1128/JB.00289-08
Chu, C., Doublet, B., Lee, Y. L., Cloeckaert, A., Chiou, C. S., Chen, S. W., et al. (2012). Salmonella genomic island 1-J variants associated with change in the antibiotic resistance gene cluster in multidrug-resistant Salmonella enterica serovar Virchow isolated from humans, Taiwan, 2004-2006. Clin. Microbiol. Infect. 18, 47–53. doi: 10.1111/j.1469-0691.2011.03464.x
CLSI. (2016). Performance Standards for Antimicrobial Susceptibility Testing,” in CLSI Supplement M100, 26th Edn. Wayne, PA: Clinical and Laboratory Standards Institute.
Cohn, L. A., Gary, A. T., Fales, W. H., and Madsen, R. W. (2003). Trends in fluoroquinolone resistance of bacteria isolated from canine urinary tracts. J. Vet. Diagn. Invest. 15, 338–343. doi: 10.1177/104063870301500406
Douard, G., Praud, K., Cloeckaert, A., and Doublet, B. (2010). The Salmonella genomic island 1 is specifically mobilized in trans by the IncA/C multidrug resistance plasmid family. PLoS One 5:e15302. doi: 10.1371/journal.pone.0015302
Doublet, B., Boyd, D. A., Douard, G., Praud, K., Cloeckaert, A., and Mulvey, M. R. (2012). Complete nucleotide sequence of the multidrug resistance IncA/C plasmid pR55 from Klebsiella pneumoniae isolated in 1969. J. Antimicrob. Chemother. 67, 2354–2360. doi: 10.1093/jac/dks251
Doublet, B., Boyd, D. A., Mulvey, M. R., and Cloeckaert, A. (2005). The Salmonella genomic island 1 is an integrative mobilizable element. Mol. Microbiol. 55, 1911–1924. doi: 10.1111/j.1365-2958.2005.04520.x
Doublet, B., Praud, K., Bertrand, S., Collard, J. M., Weill, F. X., and Cloeckaert, A. (2008). Novel insertion sequence-and transposon-mediated genetic rearrangements in genomic island SGI1 of Salmonella enterica serovar Kentucky. Antimicrob. Agents Chemother. 52, 3745–3754. doi: 10.1128/AAC.00525-08
Doublet, B., Praud, K., Weill, F. X., and Cloeckaert, A. (2009). Association of IS26-composite transposons and complex In4-type integrons generates novel multidrug resistance loci in Salmonella genomic island 1. J. Antimicrob. Chemother. 63, 282–289. doi: 10.1093/jac/dkn500
Girlich, D., Dortet, L., Poirel, L., and Nordmann, P. (2015). Integration of the blaNDM-1 carbapenemase gene into Proteus genomic island 1 (PGI1-PmPEL) in a Proteus mirabilis clinical isolate. J. Antimicrob. Chemother. 70, 98–102. doi: 10.1093/jac/dku371
Hall, R. M. (2010). Salmonella genomic islands and antibiotic resistance in Salmonella enterica. Future Microbiol. 5, 1525–1538. doi: 10.2217/fmb.10.122
Hamidian, M., Holt, K. E., and Hall, R. M. (2015). Genomic resistance island AGI1 carrying a complex class 1 integron in a multiply antibiotic-resistant ST25 Acinetobacter baumannii isolate. J. Antimicrob. Chemother. 70, 2519–2523. doi: 10.1093/jac/dkv137
Hernández-Arriaga, A. M., Chan, W. T., Espinosa, M., and Díaz-Orejas, R. (2014). Conditional activation of toxin-antitoxin systems: postsegregational killing and beyond. Microbiol. Spectr. 2:PLAS-0009-2013. doi: 10.1128/microbiolspec.PLAS-0009-2013
Juhas, M., van der Meer, J. R., Gaillard, M., Harding, R. M., Hood, D. W., and Crook, D. W. (2009). Genomic islands: tools of bacterial horizontal gene transfer and evolution. FEMS Microbiol. Rev. 33, 376–393. doi: 10.1111/j.1574-6976.2008.00136.x
Kirby, J. E., Trempy, J. E., and Gottesman, S. (1994). Excision of a P4-like cryptic prophage leads to Alp protease expression in Escherichia coli. J. Bacteriol. 176, 2068–2081. doi: 10.1128/jb.176.7.2068-2081.1994
Kiss, J., Nagy, B., and Olasz, F. (2012). Stability, entrapment and variant formation of Salmonella genomic island 1. PLoS One 7:e32497. doi: 10.1371/journal.pone.0032497
Kiss, J., Papp, P. P., Szabó, M., Farkas, T., Murányi, G., Szakállas, E., et al. (2015). The master regulator of IncA/C plasmids is recognized by the Salmonella genomic island SGI1 as a signal for excision and conjugal transfer. Nucleic Acids Res. 43, 8735–8745. doi: 10.1093/nar/gkv758
Le Hello, S., Weill, F. X., Guibert, V., Praud, K., Cloeckaert, A., and Doublet, B. (2012). Early strains of multidrug-resistant Salmonella enterica serovar Kentucky sequence type 198 from Southeast Asia harbor Salmonella genomic island 1-J variants with a novel insertion sequence. Antimicrob. Agents Chemother. 56, 5096–5102. doi: 10.1128/AAC.00732-12
Lei, C., Chen, Y., Kong, L., Zeng, J., Wang, Y., Zhang, A., et al. (2018). PGI2, a novel SGI1-relative multidrug-resistant genomic island characterized in Proteus mirabilis. Antimicrob. Agents Chemother. 62:e0019-18. doi: 10.1128/AAC.00019-18
Lei, C., Zhang, A., Liu, B., Wang, H., Guan, Z., Xu, C., et al. (2014). Molecular characteristics of Salmonella genomic Island 1 in Proteus mirabilis isolates from poultry farms in China. Antimicrob. Agents Chemother. 58, 7570–7572. doi: 10.1128/AAC.03992-14
Lei, C., Zhang, A., Liu, B., Wang, H., Yang, L., Guan, Z., et al. (2015). Two novel Salmonella genomic island 1 variants in Proteus mirabilis isolates from swine farms in China. Antimicrob. Agents Chemother. 59, 4336–4338. doi: 10.1128/AAC.00120-15
Levings, R. S., Djordjevic, S. P., and Hall, R. M. (2008). SGI2, a relative of Salmonella genomic island SGI1 with an independent origin. Antimicrob. Agents Chemother. 52, 2529–2537. doi: 10.1128/AAC.00189-08
Levings, R. S., Lightfoot, D., Partridge, S. R., Hall, R. M., and Djordjevic, S. P. (2005). The genomic island SGI1, containing the multiple antibiotic resistance region of Salmonella enterica serovar Typhimurium DT104 or variants of it, is widely distributed in other S. enterica serovars. J. Bacteriol. 187, 4401–4409. doi: 10.1128/JB.187.13.4401-4409
Levings, R. S., Partridge, S. R., Djordjevic, S. P., and Hall, R. M. (2007). SGI1-K, a variant of the SGI1 genomic island carrying a mercury resistance region, in Salmonella enterica serovar Kentucky. Antimicrob. Agents Chemother. 51, 317–323. doi: 10.1128/AAC.01229-06
López-Fuentes, E., Torres-Tejerizo, G., Cervantes, L., and Brom, S. (2015). Genes encoding conserved hypothetical proteins localized in the conjugative transfer region of plasmid pRet42a from Rhizobium etli CFN42 participate in modulating transfer and affect conjugation from different donors. Front. Microbiol. 5:793. doi: 10.3389/fmicb.2014.00793
Luo, R., Liu, B., Xie, Y., Li, Z., Huang, W., Yuan, J., et al. (2012). SOAPdenovo2: an empirically improved memory-efficient short-read de novo assembler. Gigascience 1:18. doi: 10.1186/2047-217X-1-18
Marsan, D., Place, A., Fucich, D., and Chen, F. (2017). Toxin-antitoxin systems in estuarine Synechococcus strain CB0101 and their transcriptomic responses to environmental stressors. Front. Microbiol. 8:1213. doi: 10.3389/fmicb.2017.01213
Mulvey, M. R., Boyd, D. A., Olson, A. B., Doublet, B., and Cloeckaert, A. (2006). The genetics of Salmonella genomic island 1. Microbes Infect. 8, 1915–1922. doi: 10.1016/j.micinf.2005.12.028
Partridge, S. R., Tsafnat, G., Coiera, E., and Iredell, J. R. (2009). Gene cassettes and cassette arrays in mobile resistance integrons. FEMS Microbiol. Rev. 33, 757–784. doi: 10.1111/j.1574-6976.2009.00175.x
Qin, S., Qi, H., Zhang, Q., Zhao, D., Liu, Z., Tian, H., et al. (2015). Emergence of extensively drug-resistant Proteus mirabilis harboring a conjugative NDM-1 plasmid and a novel Salmonella genomic island 1 variant, SGI1-Z. Antimicrob. Agents Chemother. 59, 6601–6604. doi: 10.1128/AAC.00292-15
Schultz, E., Barraud, O., Madec, J.-Y., Haenni, M., Cloeckaert, A., Ploy, M.-C., et al. (2017). Multidrug resistance Salmonella genomic island 1 in a Morganella morganii subsp. morganii human clinical isolate from France. mSphere 2:e00118-17. doi: 10.1128/mSphere.00118-17
Siebor, E., de Curraize, C., Amoureux, L., and Neuwirth, C. (2016). Mobilization of the Salmonella genomic island SGI1 and the proteus genomic island PGI1 by the A/C2 plasmid carrying blaTEM-24 harboured by various clinical species of Enterobacteriaceae. J. Antimicrob. Chemother. 71, 2167–2170. doi: 10.1093/jac/dkw151
Siebor, E., and Neuwirth, C. (2011). The new variant of Salmonella genomic island 1 (SGI1-V) from a Proteus mirabilis French clinical isolate harbours blaVEB-6 and qnrA1 in themultiple antibiotic resistance region. J. Antimicrob. Chemother. 66, 2513–2520. doi: 10.1093/jac/dkr335
Siebor, E., and Neuwirth, C. (2013). Emergence of Salmonella genomic island 1 (SGI1) among Proteus mirabilis clinical isolates in Dijon, France. J. Antimicrob. Chemother. 68, 1750–1756. doi: 10.1093/jac/dkt100
Siebor, E., and Neuwirth, C. (2014). Proteus genomic island 1 (PGI1), a new resistance genomic island from two Proteus mirabilis French clinical isolates. J. Antimicrob. Chemother. 69, 3216–3220. doi: 10.1093/jac/dku314
Keywords: Proteus mirabilis, Salmonella genomic island 1, antibiotic resistance, heavy metal resistance, horizontal gene transfer, mobile genetic element
Citation: Bie L, Fang M, Li Z, Wang M and Xu H (2018) Identification and Characterization of New Resistance-Conferring SGI1s (Salmonella Genomic Island 1) in Proteus mirabilis. Front. Microbiol. 9:3172. doi: 10.3389/fmicb.2018.03172
Received: 30 July 2018; Accepted: 07 December 2018;
Published: 19 December 2018.
Edited by:
Axel Cloeckaert, Institut National de la Recherche Agronomique (INRA), FranceReviewed by:
Liang Li, Los Angeles Biomedical Research Institute, United StatesFerenc Olasz, Agricultural Biotechnology Institute, Hungary
Copyright © 2018 Bie, Fang, Li, Wang and Xu. This is an open-access article distributed under the terms of the Creative Commons Attribution License (CC BY). The use, distribution or reproduction in other forums is permitted, provided the original author(s) and the copyright owner(s) are credited and that the original publication in this journal is cited, in accordance with accepted academic practice. No use, distribution or reproduction is permitted which does not comply with these terms.
*Correspondence: Mingyu Wang, wangmingyu@sdu.edu.cn Hai Xu, haixu@sdu.edu.cn