- 1Department of Animal Science, McGill University, Montreal, QC, Canada
- 2College of Animal Science and Technology, Northwest A&F University, Yangling, China
- 3School of Pharmaceutical Sciences, Tsinghua University, Beijing, China
Bacitracin has been used in topical preparations with polymyxin B for bacterial infections. Colistin belongs to the polymyxin group of antibiotics and is effective against most Gram-negative bacilli. This study investigated whether colistin could affect the susceptibility of S. aureus to bacitracin. S. aureus isolates were first incubated with colistin and the susceptibility of S. aureus to bacitracin was increased. The effect of the combination of colistin and bacitracin on S. aureus was then confirmed by the checkerboard assay and the time-kill kinetics. The Triton X-100-induced autolysis was significantly increased after S. aureus was exposed to colistin. Exposure to colistin also led to a less positive charge on the cell surface and a significant leakage of Na+, Mg2, K+, Ca2+, Mn2+, Cu2+, and Zn2+. Finally, disruptions on the cell surface and an irregular morphology were observed when the bacteria were exposed to colistin and bacitracin. Bacitracin had a stronger antibacterial activity against S. aureus in the presence of colistin. This could be due to the fact that colistin damaged the bacterial membrane. This study suggests that combination of colistin with bacitracin has a potential for treating clinical S. aureus infections.
Introduction
Staphylococcus aureus is part of the commensal microorganisms in humans and approximately 20% of healthy individuals are carriers (Gordon and Lowy, 2008). Although S. aureus is not always pathogenic, it is a major cause of a wide range of human diseases, especially skin and soft-tissue infections. S. aureus infections are increasingly difficult to eradicate due to the emergence of antimicrobial resistance. In fact, infections by S. aureus were well controlled by methicillin up until the emergence of methicillin resistance S. aureus (Hiramatsu et al., 2001). Although vancomycin has been the most reliable and effective therapeutic agent against MRSA infections, an alarming emergence of vancomycin-resistant S. aureus strains have recently been reported in many countries such as in Iran, Europe, United States (Limbago et al., 2014; Askari et al., 2015; Friães et al., 2015). At the same time, the discovery and development of novel and efficient antibiotics has been significantly decreased. In the battle against rapidly emerging bacterial resistance, rational approaches to use existing antibiotics could be more efficient than discovery of new antibiotics.
Bacitracin is a polypeptide antibiotic produced by stains of Bacillus licheniformis and Bacillus subtilis and has been clinically used for staphylococcal (such as S. aureus) infections since the late 1940s (Johnson et al., 1945). Bacitracin functions as an inhibitor of cell wall biosynthesis through its binding to the undecaprenyl pyrophosphate (Stone and Strominger, 1971). The combination of bacitracin with other antibiotics has been efficient to be used as a topical agent (Werner and Russell, 1999). In the United States, ophthalmic ointments containing bacitracin, polymyxin B and neomycin have been shown to eradicate coagulase-negative staphylococci (CNS) from the human skin surface and the underlying stratum corneum as well as preventing repopulation with resident flora (Hendley and Ashe, 1991). Whether other polymyxin has similar effects like polymyxin B is unknown.
Colistin is one of the polymyxins, known as polymyxin E. Colistin is effective against most Gram-negative bacilli, and especially effective to treat infections caused by multi-drug-resistant Gram-negative bacteria (MDR-GNB) owing to its favorable properties of rapid bacterial killing, and an associated slow development of resistance (Li et al., 2006). Colistin is able to bind lipopolysaccharide (LPS) (Warren et al., 1985). Colistin kills Gram-negative bacteria mainly through disruption of outer membrane integrity of bacteria resulting from binding of the antibiotic with LPS and displacing of divalent cations (Yu et al., 2015).
Several studies have claimed that the combination therapy of colistin and other antibiotics are more efficient than a single antibiotic therapy to treat serious infections. For example, Souli et al. (2009) reported that combination of colistin and imipenem was synergistic (50% of isolates) or indifferent (50%) against colistin-susceptible blaVIM-1-type metallo-β-lactamase-producing (MBL) K. pneumoniae strains, while it was antagonistic (55.6%) and rarely synergistic (11%) against colistin-resistant strains. In another study, sub-inhibitory concentrations of meropenem (0.06–8 mg/L) and colistin (0.12–1 mg/L) were synergistic against 13 out of 51 P. aeruginosa isolates at 24 h, while the combination of meropenem (0.03–64 mg/L) and colistin (0.06–8 mg/L) showed synergy against A. baumannii isolates (Pankuch et al., 2008). Song et al. (2008) evaluated the effectiveness of the combination of CMS (colistin methanesulfonate) and rifampicin in 10 patients with ventilator-associated pneumonia caused by carbapenem-resistant (only susceptible to colistin) A. baumannii strains and found that seven out of 10 patients benefited from the combination therapy (Song et al., 2008). Recently, it has been shown that colistin potentiated the activity of drugs such as isoniazid and amikacin in vitro against Mycobacterium tuberculosis (Bax et al., 2015). Owing to its increasing use and reported efficacy in combination therapy against a variety of bacterial infections, in this study we wanted to investigate whether the antibacterial effect of bacitracin against S. aureus could be enhanced by colistin.
Materials and Methods
Bacteria Strains, Growth Conditions, and Resistance Detection
S. aureus BA01611 (MRSA, Wu et al., 2015), S. aureus BA01511 (from our laboratory), S. aureus WS1 (Li et al., 2015), S. aureus RN4220, S. aureus Mu50 (MRSA), S. aureus ATCC 29213 and S. aureus Newman were used in this study. The Mueller-Hinton (MH) medium was used for the growth of S. aureus. MICs for antimicrobial agents were determined by using an agar dilution method recommended by the Clinical and Laboratory Standards Institute (2015). The MIC was determined as the lowest concentration at which growth was completely inhibited. Determination of MICs was repeated three times.
Susceptibility Screen
The susceptibility screen assay was performed as described previously (Haaber et al., 2015). S. aureus strains were grown overnight and the cultures were adjusted to subsamples of 5 × 105 CFU/mL in the warm MH broth. Colistin sodium sulfate (Sigma) was added as an inducer at the concentrations of 1/2 MIC (64 μg/mL) for each strain. After 90 min at 37°C with shaking at 180 rpm, 10 μL aliquots of the cultures were spotted on MH agar plates containing bacitracin (Sigma) at a concentration of 1/2 MIC (64 μg/mL). The plates were incubated overnight at 37°C before checking the bacterial growth. Each experiment was repeated three times.
Determination of the in vitro Effects of Combinations of Bacitracin and Colistin
The antimicrobial combination assays were conducted with bacitracin plus colistin by using the broth microdilution checkerboard technique (Mataraci and Dosler, 2012). The test was performed using 96-well microtiter plates containing colistin and bacitracin in twofold serial concentrations. Bacterial suspensions were prepared to yield a final inocula of ∼5 × 105 CFU/mL. Plates were read after overnight incubation at 37°C. Fractional Inhibitory Concentration (FIC) Index was calculated according to the formulas: FICbacitracin = MICbacitracin+colistin/MICbacitracin, FICcolistin = MICbacitracin+colistin/ MICcolistin, FIC Index = FICbacitracin+ FICcolistin. FIC Index values were interpreted according to Mun et al. (2013): synergy (FIC Index ≤ 0.5); partial synergy (FIC Index > 0.5 to ≤ 0.75); additivity (FIC Index > 0.75 to ≤ 1); no interaction (indifference) (FIC Index > 1 to ≤ 4) and antagonism (FIC Index > 4.0) (Mun et al., 2013). Each experiment was repeated three times.
The Time-Kill Assay
The time-killing curve assays were performed in triplicate to study the effect of the combination of bacitracin and colistin on S. aureus BA01611 growth as previously described by Mun et al. (2013) with minor modifications (Mun et al., 2013). A single bacterial colony was added to 2 mL of the MHB and grown overnight at 37°C with shaking at 180 rpm. The overnight culture was diluted with pre-warmed MHB to obtain a starting inoculum of approximately 5 × 105 CFU/mL. The S. aureus BA01611 strain was exposed to colistin at the concentrations of 0 or 1/2 MIC (64 μg/mL) in the presence or absence of 1/2 MIC (64 μg/mL, except 8 μg/mL for S. aureus BA01511) bacitracin. Samples were taken at 0, 2, 4, 6, 8, 16, and 24 h, serially diluted, spread on drug-free plates, and incubated at 37°C for 24 h before counting the colonies. Each experiment was repeated three times.
Autolysis Assay
The autolysis assay was performed as described previously (Meehl et al., 2007). S. aureus BA01611 isolate was grown overnight and diluted in the pre-warmed MH broth and grown to the mid-exponential phase. The cells were exposed to 64 μg/mL colistin while the control was not exposed to colistin. After 1 h of exposure, the cultured cells were washed twice in cold sterile distilled water and re-suspended in the same volume of 0.05 M Tris-HCl, pH 7.2, containing 0.05% Triton X-100. Cells were incubated at 30°C, and the OD600 was measured every 30 min. Data are expressed as the percent loss of OD600 at the indicated times compared to findings at time zero. Each experiment was repeated three times.
Determination of Whole-Cell Surface Charges
The determination of whole-cell surface charges was performed as described previously (Meehl et al., 2007). After culturing overnight, S. aureus BA01611 was diluted in the pre-warmed MH broth to ensure continued exponential growth during treatment. Cells were treated with and without colistin for 1, 2, and 4 h before harvesting at the mid-exponential phase. After washing twice by centrifugation in 20 mM MOPS buffer, pH 7, the cells were re-suspended in the neutral pH MOPS buffer to a final OD600 of 0.6 before adding the positively charged cytochrome c (Sigma) at a concentration of 0.125 mg/mL. The mixture was incubated at room temperature for 10 min before centrifugation and the amount of unbound cationic cytochrome c in the supernatant was quantified photometrically at 530 nm. Each experiment was repeated three times.
Ion Leakage
The leakage of ions was performed as described previously (Miksusanti et al., 2008). Bacterial cells (S. aureus BA01611) were incubated for 24 h and then diluted in pre-warmed MH broth to ensure continued exponential growth during treatment. Cells were exposed to colistin (64 μg/mL) for 1 and 2 h. The bacterial cells without colistin exposure were used as a control. Bacterial cells were harvested by centrifugation at 12,000 × g for 15 min and washed twice with cold deionized water. The cells were then adjusted to the same OD600 and the dry weight was determined followed by digestion in a HNO3/H2O2 solution at 100°C for 24 h. After dissolution, the cells were centrifuged and the supernatant were recovered and assayed for total mineral content of cells (K+, Na+, Ca2+, Mg2+, Zn2+, Cu2+, and Mn2+) by ICP Mass Spectrometers (Varian 810/820-MS). Each experiment was repeated three times.
Scanning Electron Microscope (SEM)
SEM were performed as described previously (You et al., 2013). S. aureus BA01611 cells were treated with 1/2 MIC (64 μg/mL) colistin and/or 1/2 MIC (64 μg/mL, except 8 μg/mL for S. aureus BA01511) bacitracin for 1 h and 2 h. Untreated controls were also prepared. The bacterial cells were collected via centrifugation at 10,000 × g and then the pellet formed was washed with PBS for three times. Fixation was done by suspending the bacterial cells into 0.25% of glutaraldehyde solution (in PBS, pH 7.0) and then incubated at room temperature for 1 h before collecting the fixed bacterial pellet. Dehydration of the bacterial cells was done by washing the pellets with ethanol at different concentrations up to 100%. After the critical-point drying, the bacterial cells were observed with a field-emission scanning electron microscopy (FE-SEM; FEI Inspect F50).
Results
Existence of the Effect of the Combination of Colistin and Bacitracin Against S. aureus
In order to determine whether colistin would induce S. aureus susceptibility to bacitracin, seven S. aureus strains were screened. S. aureus strains were first treated with colistin at a concentration of 1/2 MIC (64 μg/mL), then 10 μL aliquots of the cultures were spotted on freshly prepared MH agar plates containing bacitracin at a concentration of 1/2 MIC (64 μg/mL, except 8 μg/mL for S. aureus BA01511). As shown in Figure 1, such treatments inhibited the growth of all seven strains, suggesting that colistin could induce S. aureus susceptibility to bacitracin.
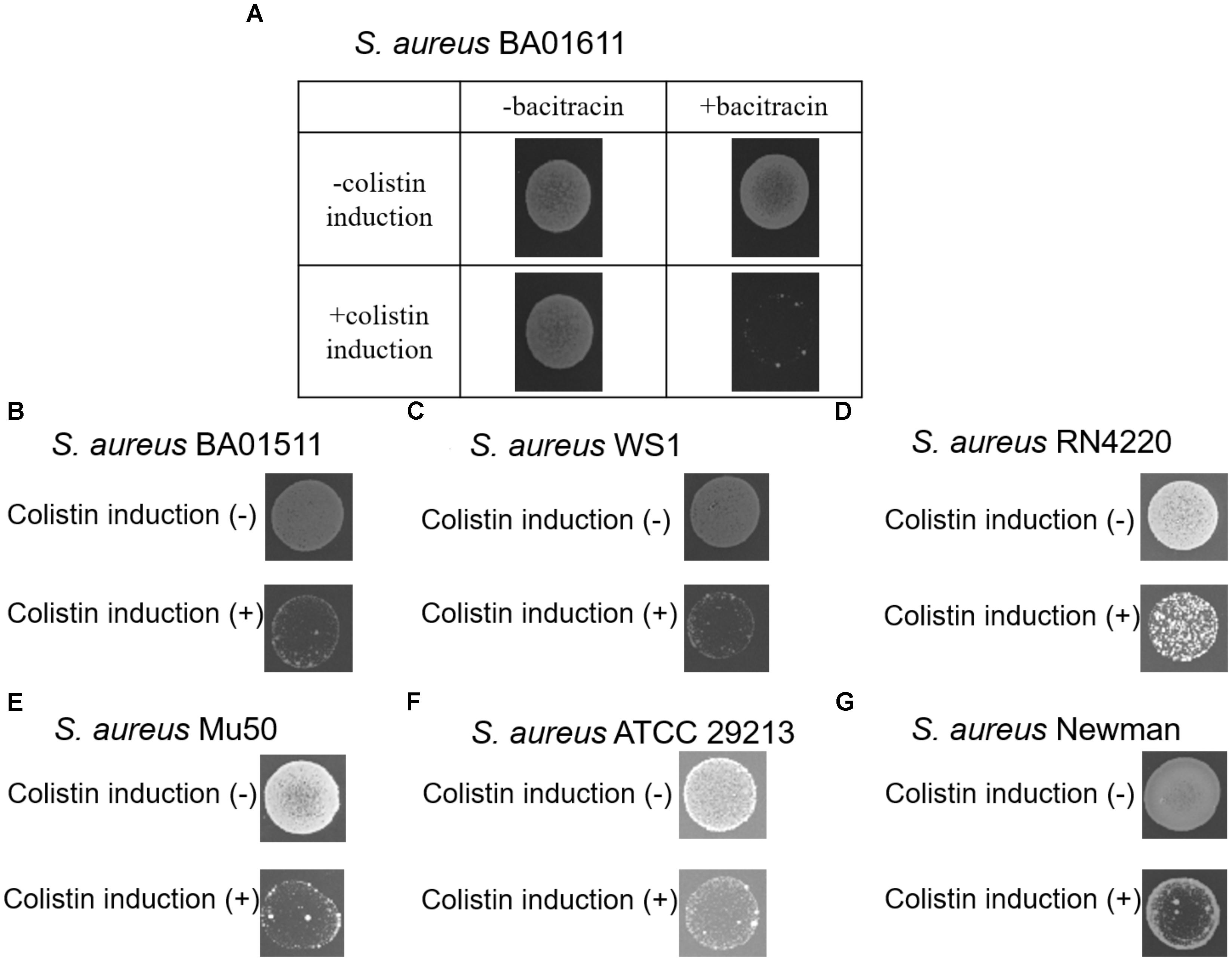
FIGURE 1. The ability of seven S. aureus stains to form colonies at a concentration of 1/2 MIC (64 μg/mL, except 8 μg/mL for S. aureus BA01511) bacitracin was assayed after pre-exposure to colistin for 90 min. (A) S. aureus BA01611 isolate was also incubated on MHA without bacitracin, (B) S. aureus BA01511, (C) S. aureus WS1, (D) S. aureus RN4220, (E) S. aureus Mu50, (F) S. aureus ATCC 29213, and (G) S. aureus Newman.
Next, a checkerboard assay was performed for each S. aureus strain, using several concentrations ranging from below the MIC to 2x MIC. As shown in Figure 2. synergy as indicated by FIC index ≤ 0.5 was obvious in six out of seven strains, while S. aureus WS1 showed a partial synergy with the FIC index equal to 0.53125.
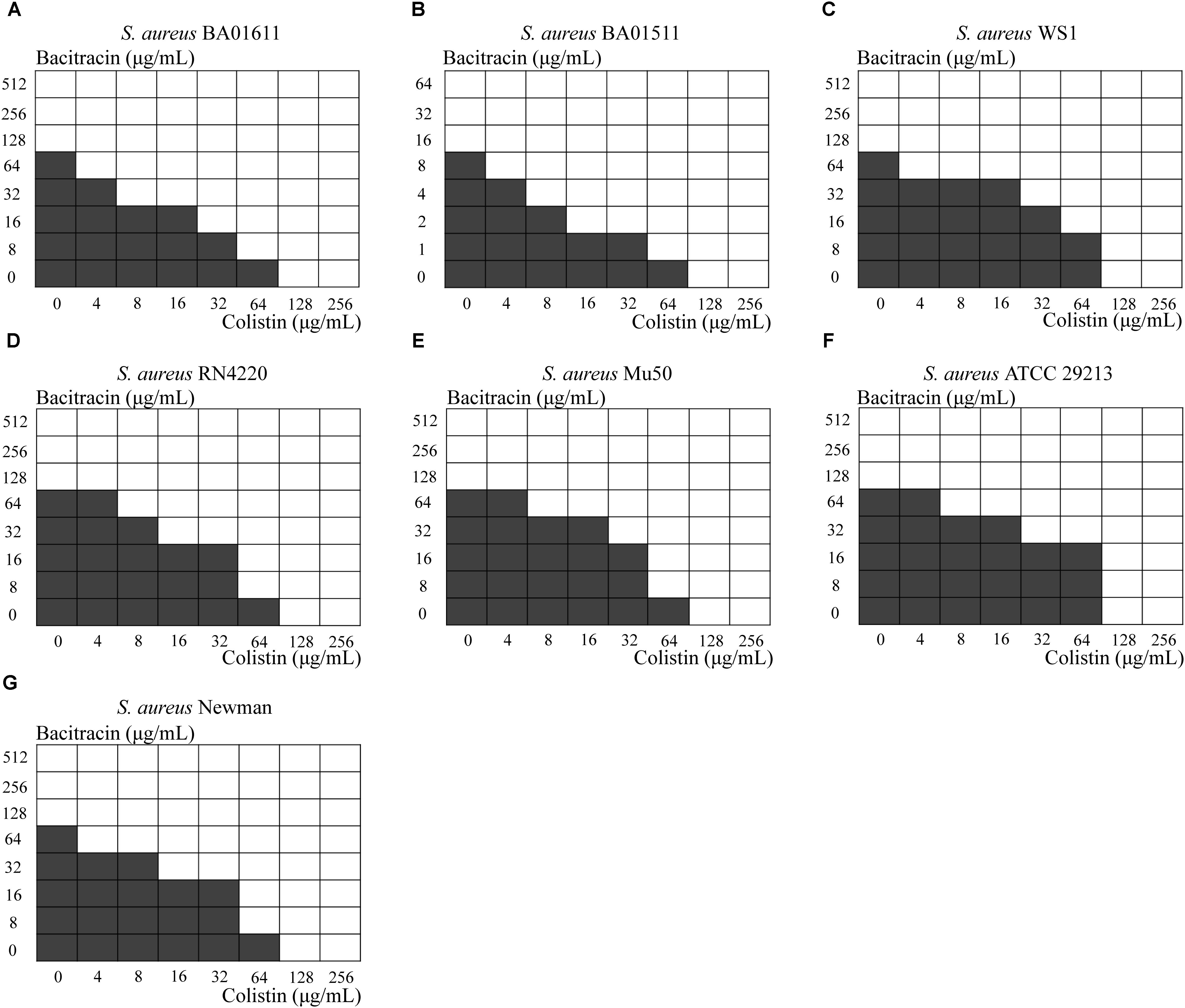
FIGURE 2. Results from the checkerboard test. Shading indicates visible growth. The Fractional Inhibitory Concentration (FIC) was calculated. (A) S. aureus BA01611 (FIC index = 0.3125), (B) S. aureus BA01511 (FIC index = 0.2500), (C) S. aureus WS1 (FIC index = 0.53125), (D) S. aureus RN4220 (FIC index = 0.375), (E) S. aureus Mu50 (FIC index = 0.5000), (F) S. aureus ATCC 29213 (FIC index = 0.3750) and (G) S. aureus Newman (FIC index = 0.3750).
Furthermore, a time-kill analysis was carried out with one MRSA strain S. aureus BA01611. As shown in Figure 3, colistin alone or bacitracin alone did not lead to cell growth inhibition after 24 h incubation compared to the control. The combination of colistin and bacitracin resulted in a > 2log10 decrease compared to the control after the 24 h incubation period, indicating that the efficiency of the combination of colistin and bacitracin against S. aureus strains.
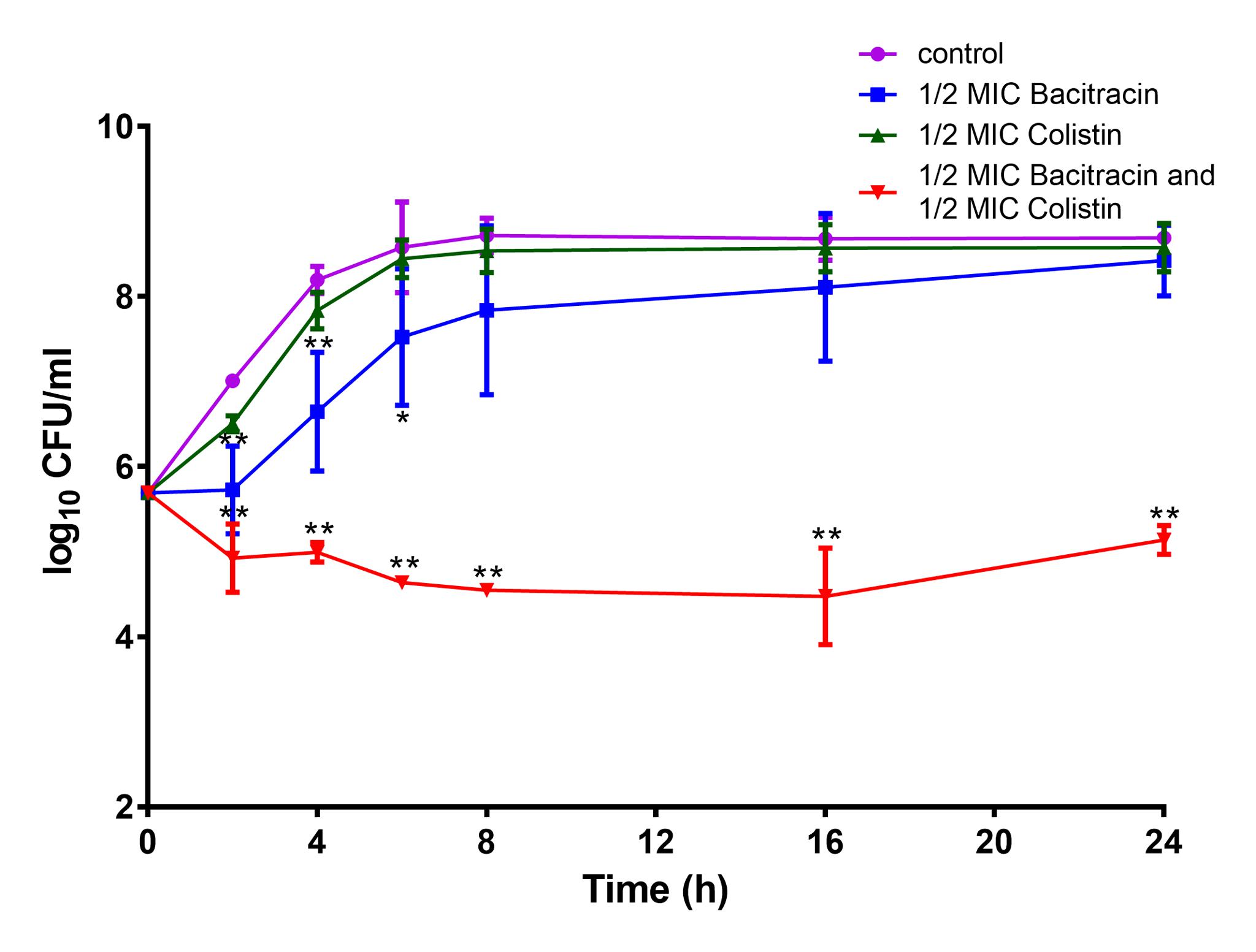
FIGURE 3. Time-kill curves of colistin and/or bacitracin against S. aureus BA01611. Data are presented as means ± standard deviations. The statistical analysis was performed using a one-way analysis of variance (ANOVA) with the least significant difference (LSD) test. ∗P < 0.05, ∗∗P < 0.01 in comparison with the control group.
Autolytic Activity Is Increased in S. aureus Treated With Colistin
To investigate how colistin induced S. aureus susceptibility to bacitracin, a Triton X-100-induced autolytic activity of the BA01611 strain was first measured after exposure to colistin. As shown in Figure 4, exposure of S. aureus BA01611 to colistin at a concentration of 64 μg/mL increased autolysis in comparison with that of the unexposed strain. Triton lysis profiles differed significantly after 150 min exposure to colistin (0.468 ± 0.0196 vs. 0.403 ± 0.0142 after 150 min: 0.527 ± 0.0073 vs. 0.460 ± 0.0117 after 180 min). The results indicated that the susceptibility to bacitracin after colistin induction could be due to the damaged cell structure.
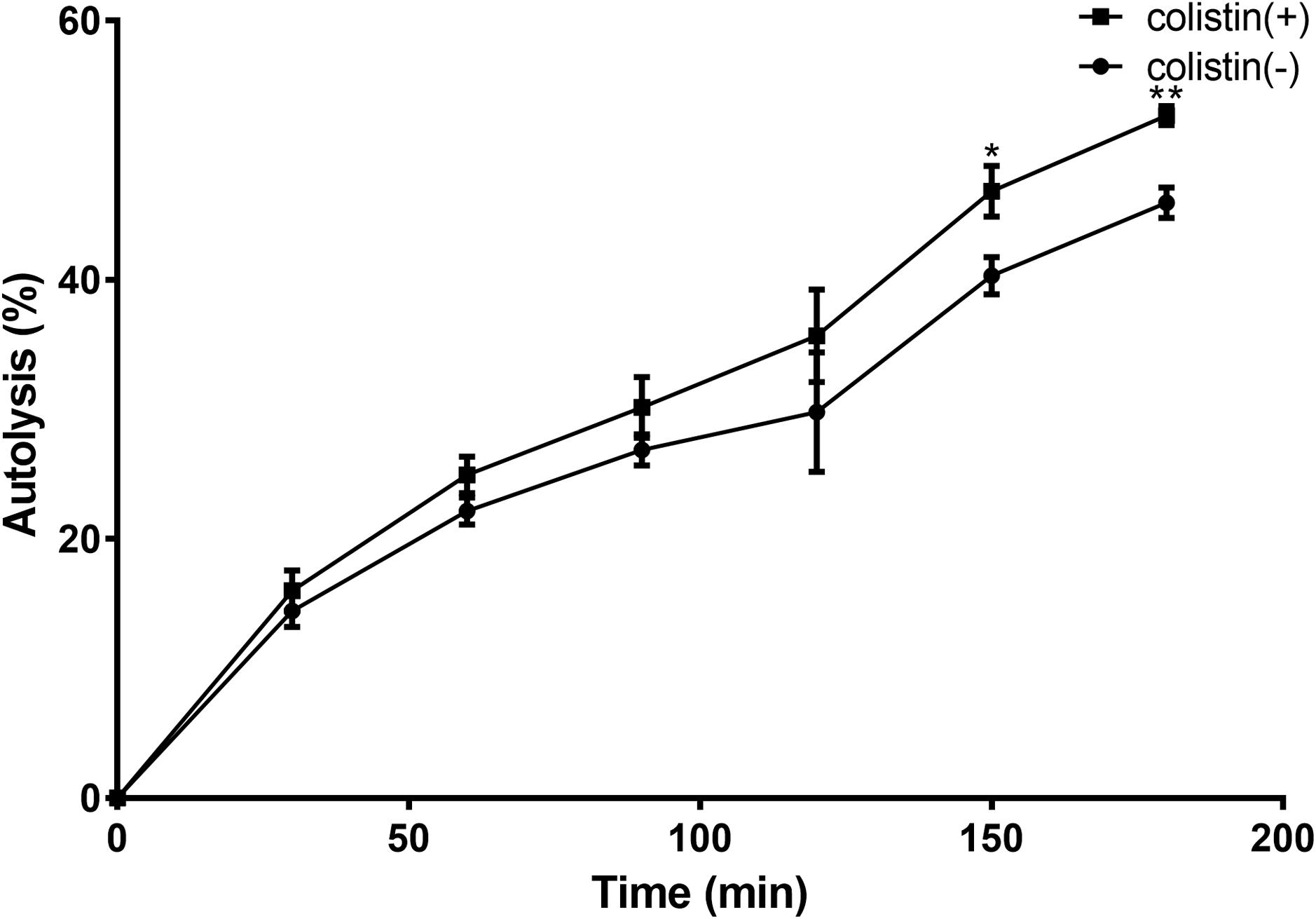
FIGURE 4. Triton X-100 autolysis assay of S. aureus BA01611. Cells were grown with or without colistin for 1 h before being washed and resuspended in buffer containing the detergent. Absorbance at 600 nm was determined for 3 h. Autolysis was determined based on the decrease in the optical density over time relative to that at time zero for each sample. Data are presented as means ± standard deviations. The statistical analysis was performed using a one-way analysis of variance (ANOVA) with the least significant difference (LSD) test. ∗P < 0.05, ∗∗P < 0.01 in comparison with the control group.
Whole-Cell Surface Positive Charge Decreased in S. aureus Treated With Colistin
As colistin is known as an important cationic antimicrobial peptide which could displace calcium and magnesium, whether the electrostatic nature of S. aureus BA01611 surface was affected by colistin was then determined by adding cationic cytochrome c. As shown in Figure 5, bacterial cells treated with colistin had a significantly greater quantity of bound cytochrome c than non-exposed strains. These results implied that colistin might render the bacterial cell membranes less positively charged and make the bacterial cells more susceptible to other antibiotics.
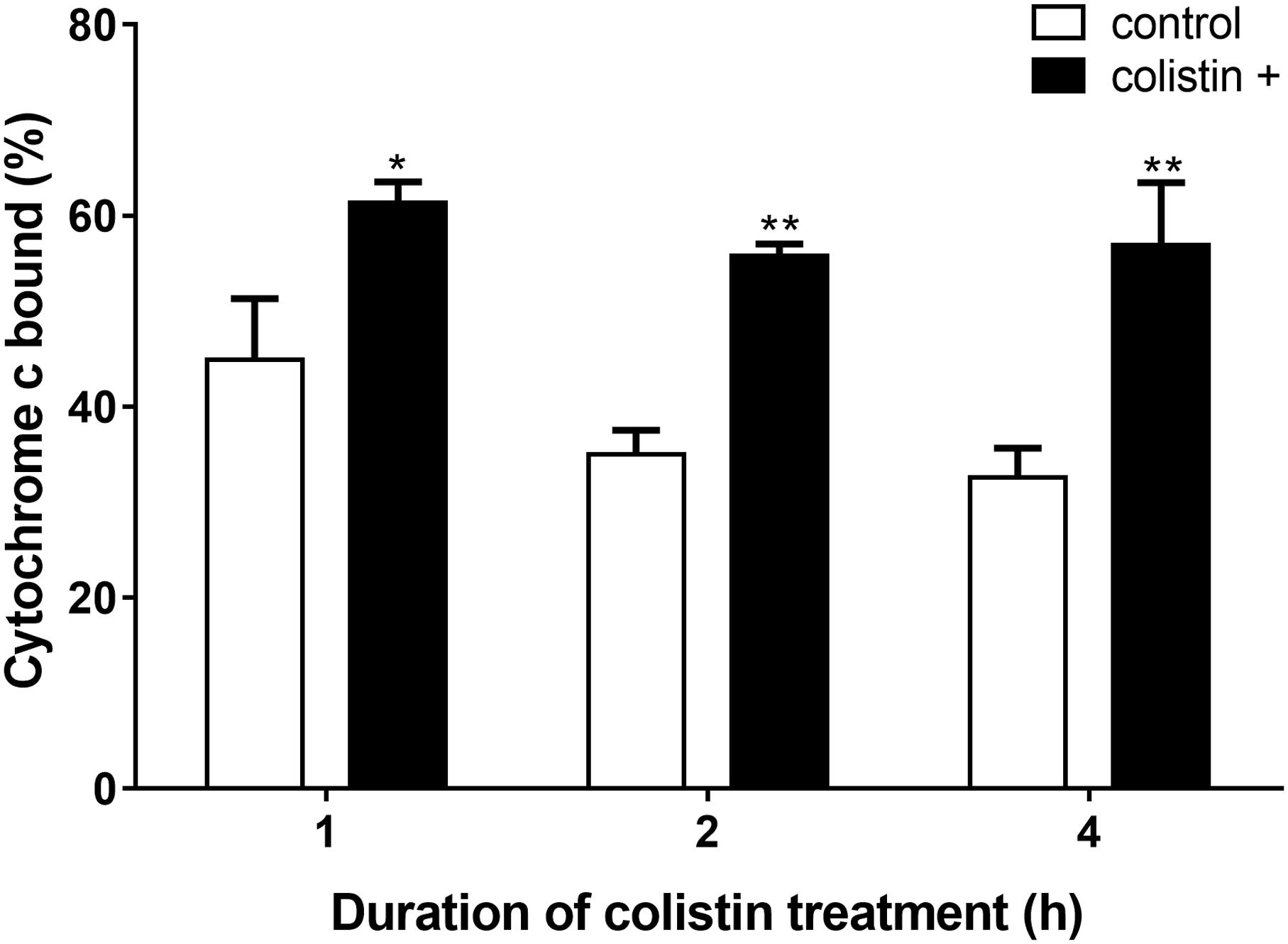
FIGURE 5. Detection of cationic cytochrome c binding to whole S. aureus BA01611 cells in the presence or absence of colistin. The figure shows the percentage of bound cytochrome c after incubating S. aureus BA01611 with colistin for 1, 2, or 4 h. Data are presented as means ± standard deviations. The statistical analysis was performed using a one-way analysis of variance (ANOVA) with the least significant difference (LSD) test. ∗P < 0.05, ∗∗P < 0.01 in comparison with the control group.
Effects of Colistin on Ions Leakage of S. aureus
To further investigate whether S. aureus ions leakage occurred upon colistin exposure, total mineral content of cells (K+, Na+, Ca2+, Mg2+, Zn2+, Cu2+, and Mn2+) of S. aureus BA01611 was determined by ICP Mass Spectrometers. While exposure to colistin for 1 h did not affect the concentration of the majority of ions, exposure to colistin for 2 h led to significant decrease in concentration of ions: Na+ by 63.0%; 86.9% for Mg2+; 86.1% for K+; 61.1% for Ca2+; 85.7% for Mn2+; 65.0% for Cu2+ and 80.7% for Zn2+ (Figure 6).
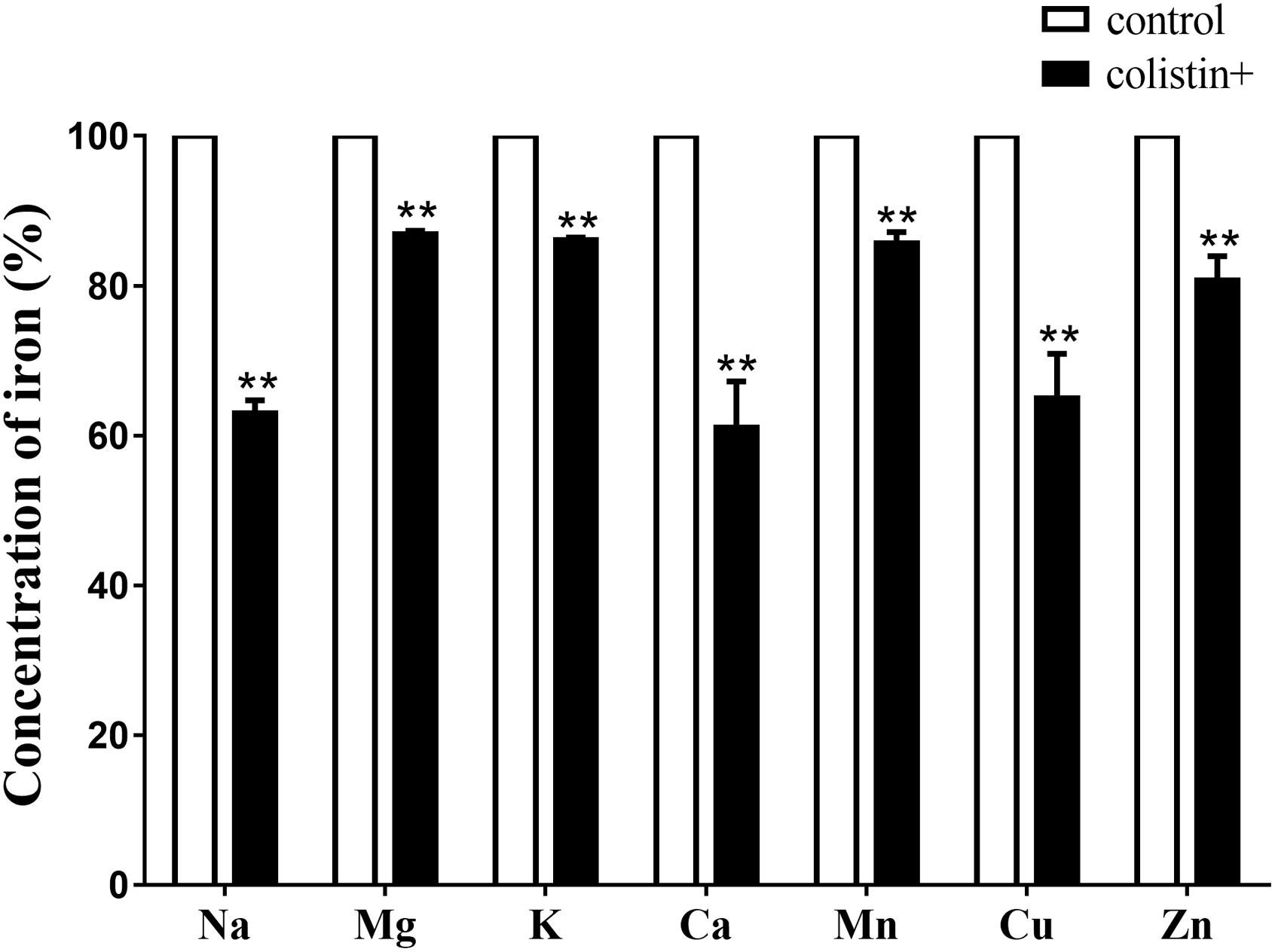
FIGURE 6. Ion leakages when S. aureus BA01611 was exposed to colistin for 2 h. The samples were digested in a HNO3/H2O2 solution of 6 mol/L. The total mineral contents of the bacteria were measured by a Varian 810/820-MS ICP Mass Spectrometer. Data are presented as means ± standard deviations. The statistical analysis was performed using a one-way analysis of variance (ANOVA) with the least significant difference (LSD) test. ∗P < 0.05, ∗∗P < 0.01 in comparison with the control group.
Cell Surface Was Damaged When S. aureus Co-treated With Colistin and Bacitracin
In order to further confirm that the affected cell surface was involved in the effect of colistin to increase susceptibility to bacitracin, S. aureus BA01611 was exposed to 1/2 MIC (64 μg/mL) colistin in the presence or absence of 1/2 MIC (64 μg/mL, except 8 μg/mL for S. aureus BA01511) bacitracin for 1 and 2 h and the structure of the cell surfaces was visualized by SEM. As shown in Figure 7, the surfaces of the untreated cells were relatively smooth and continuous with good structural integrity (Figure 7A). With the treatment of colistin or bacitracin, grape-like cell clusters were shown and the cell boundaries were faint and unclear (Figures 7B,C). In the combined treatment of colistin and bacitracin, the morphology of S. aureus changed significantly: many ghost cells with irregular morphology, and irregular and distorted outlines of the cell packets. In addition, the cells and clumps were larger than those with or without the treatment of colistin or bacitracin alone (Figures 7D,E).
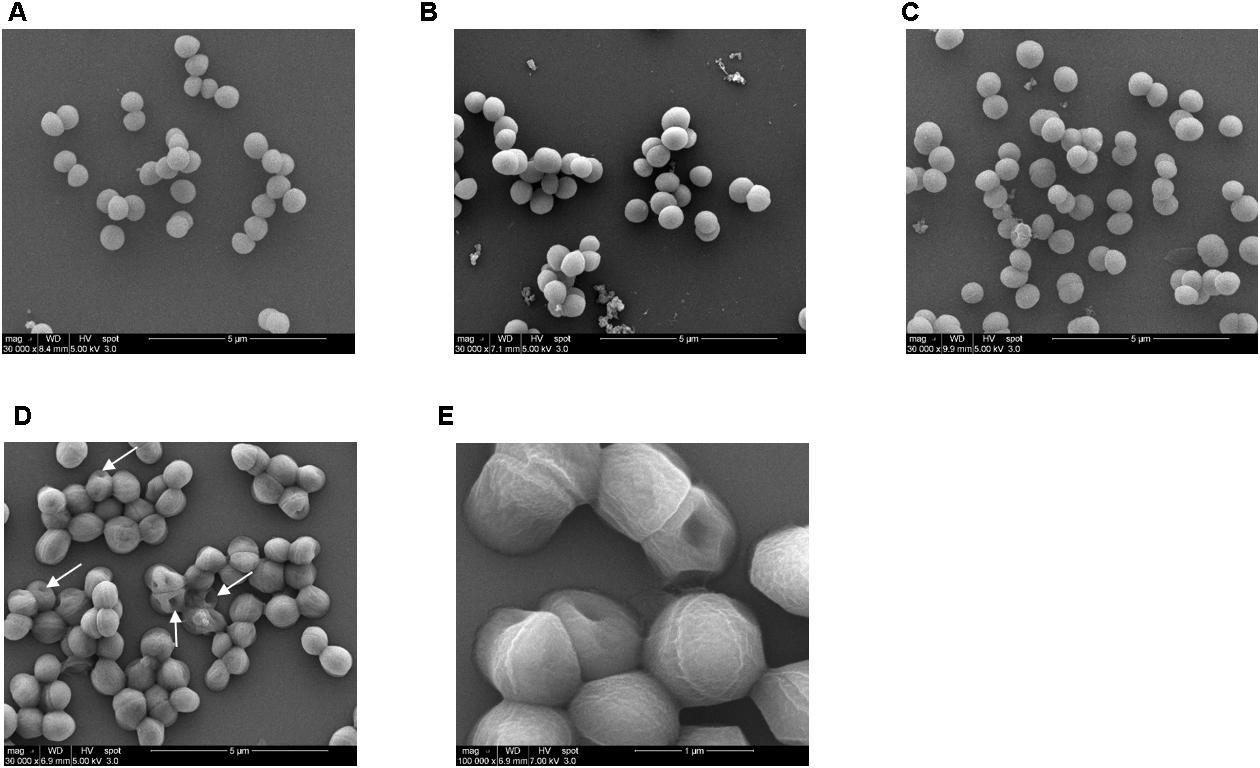
FIGURE 7. SEM images of S. aureus BA01611 cells. (A) Untreated S. aureus BA01611 (control); (B) S. aureus BA01611 treated with colistin; (C) S. aureus BA01611 treated with bacitracin; (D) S. aureus BA01611 treated with both colistin and bacitracin. Arrows indicated partially damaged surface; (E) high magnification of S. aureus BA01611 treated with both colistin and bacitracin. The cell surface was damaged in multiple locations.
Discussion
The rise of antimicrobial resistance in S. aureus is a major challenge in both hospitals and community settings (Ventola, 2015). Currently, 60–90% of S. aureus infections are reported to be methicillin-resistant (MRSA), and people with MRSA are estimated to be 64% more likely to die than people with a non-resistant form of the infection (WHO, 2014). The limited availability of effective therapies against S. aureus has increased the quest to discover new treatment options including the development of new antibiotics as well the combination of antibiotics currently in use in clinical practice. In this study, we found that the antibacterial effects of bacitracin against S. aureus was enhanced by colistin.
Most studies on combination therapy involving colistin have focused on Gram-negative bacteria. For example, colistin combined with nisin A or pediocin PA-1/AcH synergistically inhibited the enterohemorrhagic bacterium E. coli O157:H7 (Naghmouchi et al., 2013). Similarly, several antibiotics such as rifampicin, imipenem, doxycycline, azithromycin, and ciprofloxacin worked synergistically with colistin against P. aeruginosa (Biswas et al., 2012). However, Haaber et al. (2015) discovered that susceptibility of S. aureus to vancomycin was reduced by concurrent exposure to colistin. Bacitracin is proposed as one of the most popular topical antibiotics and usually combined with polymyxin B in a number of commercially available ointments and creams that are used on minor cuts and abrasions as well as postsurgical wounds (Gette et al., 1992; Spann et al., 2009). Additionally, bacitracin was effective against methicillin-resistant S. aureus in vitro (Chapnick et al., 1996) and in vivo (Soto et al., 1999). Therefore, combination of bacitracin and colistin could be an alternative for the treatment of S. aureus infections. This study explored that possibility in vitro and our results showed for the first time that colistin enhanced the inhibitory effect of bacitracin against S. aureus. Seven S. aureus strains used in this study were resistant to colistin with high MIC values. Generally speaking, Gram-positive bacteria are generally less sensitive to polymyxins than Gram-negative bacteria (Storm et al., 1977). The increased susceptibility of S. aureus to bacitracin by colistin was confirmed by several assays: the spotted assay, the checkerboard assay and time kill kinetics. Moreover, the damaged and irregular surface of S. aureus when co-exposure to colistin and bacitracin confirmed the effectiveness of this combination treatment.
How colistin increased susceptibility of bacitracin against S. aureus remains unknown. Because colistin kills Gram-negative bacteria by damaging the bacterial cell membrane and in addition to the synergy observed with other antibiotics against Gram-negative bacteria that has been reportedly linked to this function (Biswas et al., 2012), the effects of colistin on S. aureus cell surface were explored to explain the increased susceptibility to bacitracin. Based on our results, two potential mechanisms were proposed: disruption of the cell surface of S. aureus by colistin to increase entry of bacitracin into the cells and enhancement of leakage of divalent ions by colistin to enhance bacitracin antibacterial ability.
Destruction of S. aureus surface by colistin was supported by our results. In this study, S. aureus BA01611 exposed to colistin had an increased autolysis. In addition, colistin decreased positive charges on S. aureus cell surface. This could lead to unstable bacterial cells, as networks of metal cations could influence the rigidity and porosity of the cell wall (Silhavy et al., 2010). Furthermore, observed ion leakage from the bacterial cells after colistin exposure suggested increased S. aureus membrane permeability. As reviewed by Karaiskos et al. (2017), permeabilization of the bacterial membrane by colistin may allow the access of a second drug through the outer membrane into the periplasmic space and the cytoplasm, consequently improving the bactericidal activity against Gram-negative bacteria (Karaiskos et al., 2017). Electron microscopic study has also shown the cytoplasmic membrane of Gram-negative bacteria appeared to be damaged and part of the cytoplasmic material released in fibrous forms through the cracks when exposed to colistin (Koike et al., 1969). Thus, it is plausible that colistin can also modify cell structure of S. aureus and allow more bacitracin to get into the cytoplasm. Such a possibility needs further studies to be confirmed.
The increased susceptibility to bacitracin by colistin may also be due to the rearrangement of electric charges on cell surface, leading to the enhanced efficacy of bacitracin. As described above, S. aureus showed a higher negative charge of the cells and reduced cellular concentrations of Ca2+, Mn2+, Zn2+, and Mg2+ after being exposed to colistin. Bacitracin affects the biosynthesis of the peptidoglycan of bacterial cell walls by binding very tightly to the long-chain C55-isoprenol pyrophosphate and preventing its recycling (Stone and Strominger, 1973). These processes need a divalent metal ion and the antimicrobial activity of bacitracin could be stimulated by various metal ions such as Cu2+, Mn2+ or Zn2+ (Stone and Strominger, 1971; Ming and Epperson, 2002). In Gram-negative bacteria, the polycationic ring of colistin binds to LPS by displacement, in a competitive fashion, of the calcium (Ca2+) and magnesium (Mg2+) ions from the phosphate groups of LPS, leading to disruption of the outer membrane and loss of cellular contents and consequently killing the bacterium (Bialvaei and Samadi, 2015). We hypothesize that colistin may be capable of modulating the teichoic acid net charge by displacing the divalent cations from the phosphate groups of teichoic acids in the cell surface of S. aureus and thus enhancing the inhibitory effect of bacitracin on S. aureus.
Conclusion
Our study showed the combination of colistin and bacitracin could be feasible for treating S. aureus infections. While colistin has attracted considerable interest as an antibiotic for use against multidrug-resistant Gram-negative bacteria, its potential for Gram-positive bacteria such as S. aureus should be further explored.
Author Contributions
WS, VU, and XZ designed the research. WS and LW performed the research. WS, LW, and XZ analyzed the data. WS and XZ wrote the paper.
Funding
This research was financially supported by Agriculture and Agri-Food Canada, The Natural Sciences and Engineering Research Council of Canada (Ottawa, ON, Canada) and Dairy Farmers of Canada (Ottawa, ON, Canada).
Conflict of Interest Statement
The authors declare that the research was conducted in the absence of any commercial or financial relationships that could be construed as a potential conflict of interest.
Acknowledgments
We would like to thank Shoaib Ashraf for technical assistance.
References
Askari, E., Zarifian, A., Pourmand, M., and Naderi-Nasab, M. (2015). High-level vancomycin-resistant Staphylococcus aureus (VRSA) in iran: a systematic review. J. Med. Microbiol. 1, 53–61.
Bax, H. I., de Steenwinkel, J. E., ten Kate, M. T., van der Meijden, A., Verbon, A., and Bakker Woudenberg, I. A. (2015). Colistin as a potentiator of anti-TB drug activity against Mycobacterium tuberculosis. J. Antimicrob. Chemother. 70, 2828–2837. doi: 10.1093/jac/dkv194
Bialvaei, A. Z., and Samadi, K. H. (2015). Colistin, mechanisms and prevalence of resistance. Curr. Med. Res. Opin. 31, 707–721. doi: 10.1185/03007995.2015.1018989
Biswas, S., Brunel, J. M., Dubus, J. C., Reynaud-Gaubert, M., and Rolain, J. M. (2012). Colistin: an update on the antibiotic of the 21st century. Expert. Rev. Anti. Infect. Ther. 10, 917–934. doi: 10.1586/eri.12.78
Chapnick, E. K., Gradon, J. D., Kreiswirth, B., Lutwick, L. I., Schaffer, B. C., Schiano, T. D., et al. (1996). Comparative killing kinetics of methicillin-resistant Staphylococcus aureus by bacitracin or mupirocin. Infect. Control. Hosp. Epidemiol. 17, 178–180.
Clinical and Laboratory Standards Institute. (2015). Methods for Dilution Antimicrobial Susceptibility Tests for Bacteria that Grow Aerobically; Approved Standard, 10th Edn. Wayne, PA: CLSI.
Friães, A., Resina, C., Manuel, V., Lito, L., Ramirez, M., and Melo-Cristino, J. (2015). Epidemiological survey of the first case of vancomycin-resistant Staphylococcus aureus infection in europe. Epidemiol. Infect. 143, 745–748. doi: 10.1017/S0950268814001423
Gette, M. T., Marks, J. G., and Maloney, M. E. (1992). Frequency of postoperative allergic contact dermatitis to topical antibiotics. Arch. Dermatol. 128, 365–367.
Gordon, R. J., and Lowy, F. D. (2008). Pathogenesis of methicillin-resistant Staphylococcus aureus infection. Clin. Infect. Dis. 46, S350–S359. doi: 10.1086/533591
Haaber, J., Friberg, C., McCreary, M., Lin, R., Cohen, S. N., and Ingmer, H. (2015). Reversible antibiotic tolerance induced in Staphylococcus aureus by concurrent drug exposure. MBio 6:e2268–14. doi: 10.1128/mBio.02268-14
Hendley, J. O., and Ashe, K. M. (1991). Effect of topical antimicrobial treatment on aerobic bacteria in the stratum corneum of human skin. Antimicrob. Agents Chemother. 35, 627–631.
Hiramatsu, K. L., Cui, L., Kuroda, M., and Ito, T. (2001). The emergence and evolution of methicillin-resistant Staphylococcus aureus. Trends Microbiol. 9, 486–493.
Johnson, B. A., Anker, H., and Meleney, F. L. (1945). Bacitracin: a new antibiotic produced by a member of the B. Subtilis group. Science. 102, 376–377.
Karaiskos, I., Souli, M., Galani, I., and Giamarellou, H. (2017). Colistin: still a lifesaver for the 21st century? Expert. Opin. Drug Metab. Toxicol. 13, 59–71.
Koike, M., Iida, K., and Matsuo, T. (1969). Electron microscopic studies on mode of action of polymyxin. J. Bacteriol. 97, 448–452.
Li, J., Rayner, C. R., Nation, R. L., Owen, R. J., Spelman, D., Tan, K. E., et al. (2006). Heteroresistance to colistin in multidrug-resistant Acinetobacter baumannii. Antimicrob. Agents Chemother. 50, 2946–2950.
Li, L., Zhou, L., Wang, L., Xue, H., and Zhao, X. (2015). Characterization of methicillin-resistant and-susceptible staphylococcal isolates from bovine milk in northwestern china. PLoS One 10:e0116699. doi: 10.1371/journal.pone.0116699
Limbago, B. M., Kallen, A. J., Zhu, W., Eggers, P., McDougal, L. K., and Albrecht, V. S. (2014). Report of the 13th vancomycin-resistant Staphylococcus aureus isolate from the united states. J. Clin. Microbiol. 52, 998–1002. doi: 10.1128/JCM.02187-13
Mataraci, E., and Dosler, S. (2012). In vitro activities of antibiotics and antimicrobial cationic peptides alone and in combination against methicillin-resistant Staphylococcus aureus biofilms. Antimicrob. Agents Chemother. 56, 6366–6371. doi: 10.1128/AAC.01180-12
Meehl, M., Herbert, S., Götz, F., and Cheung, A. (2007). Interaction of the GraRS two-component system with the VraFG ABC transporter to support vancomycin-intermediate resistance in Staphylococcus aureus. Antimicrob. Agents Chemother. 51, 2679–2689.
Miksusanti, M., Jenie, B. S. L., Priosoeryanto, B. P., Syarief, R., and Rekso, G. T. (2008). Mode of action Temu kunci (Kaempferia pandurata) essential oil on E. coli K1. 1 cell determined by leakage of material cell and salt tolerance assays. HAYATI J. Biosci. 15, 56–60. doi: 10.4308/hjb.15.2.56
Ming, L. J., and Epperson, J. D. (2002). Metal binding and structure–activity relationship of the metalloantibiotic peptide bacitracin. J. Inorg. Biochem. 91, 46–58. doi: 10.1016/S0162-0134(02)00464-6
Mun, S. H., Joung, D. K., Kim, Y. S., Kang, O. H., Kim, S. B., Seo, Y. S., et al. (2013). Synergistic antibacterial effect of curcumin against methicillin-resistant Staphylococcus aureus. Phytomedicine 20, 714–718. doi: 10.1016/j.phymed.2013.02.006
Naghmouchi, K., Baah, J., Hober, D., Jouy, E., Rubrecht, C., Sané, F., et al. (2013). Synergistic effect between colistin and bacteriocins in controlling gram-negative pathogens and their potential to reduce antibiotic toxicity in mammalian epithelial cells. Antimicrob. Agents Chemother. 57, 2719–2725. doi: 10.1128/AAC.02328-12
Pankuch, G. A., Lin, G., Seifert, H., and Appelbaum, P. C. (2008). Activity of meropenem with and without ciprofloxacin and colistin against Pseudomonas aeruginosa and Acinetobacter baumannii. Antimicrob. Agents Chemother. 52, 333–336.
Silhavy, T. J., Kahne, D., and Walker, S. (2010). The bacterial cell envelope. Cold Spring Harb. Perspect. Biol. 2:a000414. doi: 10.1101/cshperspect.a000414
Song, J. Y., Lee, J., Heo, J. Y., Noh, J. Y., Kim, W. J., Cheong, H. J., et al. (2008). Colistin and rifampicin combination in the treatment of ventilatorassociated pneumonia caused by carbapenem-resistant Acinetobacter baumannii. Int. J. Antimicrob. Agents. 32, 281–284. doi: 10.1016/j.ijantimicag.2008.04.013
Soto, N. E., Vaghjimal, A., Stahl-Avicolli, A., Protic, J. R., Lutwick, L. I., and Chapnick, E. K. (1999). Bacitracin versus mupirocin for Staphylococcus aureus nasal colonization. Infect. Control Hosp. Epidemiol. 20, 351–353.
Souli, M., Rekatsina, P. D., Chryssouli, Z., Galani, I., Giamarellou, H., and Kanellakopoulou, K. (2009). Does the activity of the combination of imipenem and colistin in vitro exceed the problem of resistance in metallo-beta-lactamase-producing Klebsiella pneumoniae isolates? Antimicrob. Agents Chemother. 53, 2133–2135. doi: 10.1128/AAC.01271-08
Spann, C. T., Tutrone, W. D., Weinberg, J. M., Scheinfeld, N., and Ross, B. (2009). Topical antibacterial agents for wound care: a primer. Dermatol. Surg. 29, 620–626.
Stone, K. J., and Strominger, J. L. (1971). Mechanism of action of bacitracin: complexation with metal ion and C55-isoprenyl pyrophosphate. Proc. Natl. Acad. Sci. 68, 3223–3227.
Stone, K. J., and Strominger, J. L. (1973). Complex formation between bacitracin peptides and isoprenyl pyrophosphates the specificity of lipid-peptide interactions. J. Biol. Chem. 248, 3940–3945.
Storm, D. R., Rosenthal, K. S., and Swanson, P. E. (1977). Polymyxin and related peptide antibiotics. Annu. Rev. Biochem. 46, 723–763.
Ventola, C. L. (2015). The antibiotic resistance crisis Part 1: causes and threats. P T 40, 277–283.
Warren, H. S., Kania, S. A., and Siber, G. R. (1985). Binding and neutralization of bacterial lipopolysaccharide by colistin nonapeptide. Antimicrob. Agents Chemother. 28:107.
Werner, A. H., and Russell, A. D. (1999). Mupirocin, fusidic acid and bacitracin: activity, action and clinical uses of three topical antibiotics. Vet. Dermatol. 10, 225–240. doi: 10.1046/j.1365-3164.1999.00185.x
WHO. (2014). WHO’s first global report on antibiotic resistance reveals serious, worldwide threat to public health. Available at: http://www.who.int/mediacentre/news/releases/2014/amr-report/en/
Wu, Z., Li, F., Liu, D., Xue, H., and Zhao, X. (2015). ). Novel type XII staphylococcal cassette chromosome mec harboring a new cassette chromosome recombinase, CcrC2. Antimicrob. Agents Chemother. 59, 7597–7601. doi: 10.1128/AAC.01692-15
You, Y. O., Choi, N. Y., Kang, S. Y., and Kim, K. J. (2013). Antibacterial activity of rhus javanica against methicillin-resistant staphylococcus aureus. Evid. Based Complement. Altern. Med. 2:549207. doi: 10.1155/2013/549207
Keywords: bacitracin, colistin, Staphylococcus aureus, antibiotic, susceptibility
Citation: Si W, Wang L, Usongo V and Zhao X (2018) Colistin Induces S. aureus Susceptibility to Bacitracin. Front. Microbiol. 9:2805. doi: 10.3389/fmicb.2018.02805
Received: 14 June 2018; Accepted: 31 October 2018;
Published: 20 November 2018.
Edited by:
Santi M. Mandal, Indian Institute of Technology Kharagpur, IndiaReviewed by:
Neelam Singh, Babasaheb Bhimrao Ambedkar University, IndiaPiyush Baindara, University of Arkansas for Medical Sciences, United States
Copyright © 2018 Si, Wang, Usongo and Zhao. This is an open-access article distributed under the terms of the Creative Commons Attribution License (CC BY). The use, distribution or reproduction in other forums is permitted, provided the original author(s) and the copyright owner(s) are credited and that the original publication in this journal is cited, in accordance with accepted academic practice. No use, distribution or reproduction is permitted which does not comply with these terms.
*Correspondence: Xin Zhao, eGluLnpoYW9AbWNnaWxsLmNh