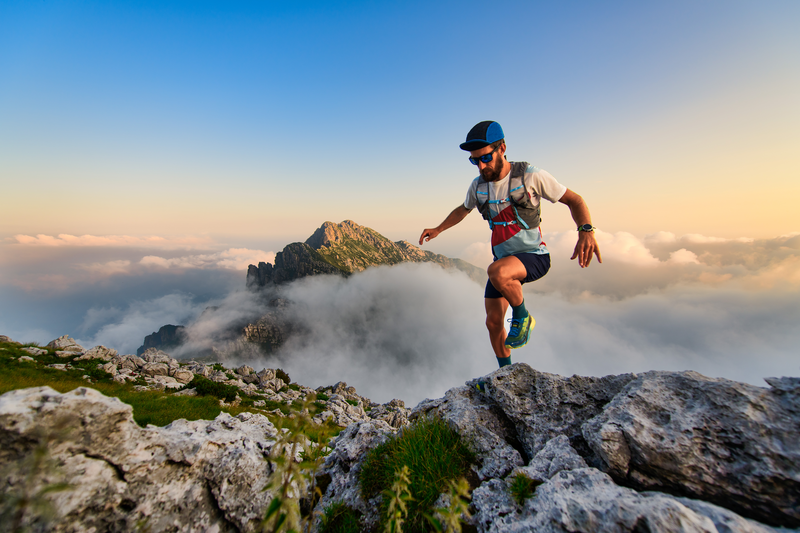
94% of researchers rate our articles as excellent or good
Learn more about the work of our research integrity team to safeguard the quality of each article we publish.
Find out more
ORIGINAL RESEARCH article
Front. Microbiol. , 16 November 2018
Sec. Microbial Physiology and Metabolism
Volume 9 - 2018 | https://doi.org/10.3389/fmicb.2018.02791
The xenobiotic response element (XRE) transcription factors belong to a regulator family frequently found in Streptomyces that are often followed by small proteins with a DUF397 domain. In fact, the pair XRE-DUF397 has been proposed to comprise toxin–antitoxin (TA) type II systems. In this work, we demonstrate that one of these putative TA-systems, encoded by the genes SCO4441 and SCO4442 of Streptomyces coelicolor, and denominated Scr1/Scr2 (which stands for S. coelicolor regulator), does not behave as a toxin–antitoxin system under the conditions used as was originally expected. Instead the pair Scr1/Scr2 acts as a strong positive regulator of endogenous antibiotic production in S. coelicolor. The analysis of the 19 Streptomyces strains tested determined that overexpression of the pair Scr1/Scr2 drastically induces the production of antibiotics not only in S. coelicolor, but also in Streptomyces lividans, Streptomyces peucetius, Streptomyces steffisburgensis and Streptomyces sp. CA-240608. Our work also shows that Scr1 needs Scr2 to exert positive regulation on antibiotic production.
Streptomyces are Gram-positive bacteria with a complex life cycle that includes the formation of mycelia and spores. In order to compete with other inhabitants of their ecosystems (mainly the soil), these bacteria have developed the capacity to produce a high number of extracellular hydrolytic enzymes and, also, secondary metabolites with antibiotic and antifungal activities among others (Chater, 2016). The production of these metabolites is tightly regulated through a large number of signal transduction proteins, including transcriptional regulators, which confer Streptomyces with the ability to rapidly respond to environmental changes by using available nutrients and producing secondary metabolites. It has been determined that 804 out of the 8300 genes in the genome of Streptomyces coelicolor are associated with this function. Of these, 499 have been classified as transcriptional regulators, 155 as one-component systems, 64 as sigma factors and 9 as DNA-binding proteins1 (Ortet et al., 2012). The xenobiotic response element (XRE) family of transcription factors (TF) is comprised of 70 TFs in S. coelicolor. This XRE family is the second most frequently occurring regulator family in bacteria, which control several diverse metabolic functions (Novichkov et al., 2013)2. Although these TF are abundant in Streptomyces genomes they have been poorly characterized. The most studied member of this group is the master regulator BldD from S. coelicolor. BldD is a small (18 kDa) protein that is a transcriptional regulator essential for morphological development and antibiotic production (den Hengst et al., 2010). WhiJ (SCO4543) is another member that has been studied in this organism, which has been associated with the repression of differentiation (Aínsa et al., 2010). WhiJ has a wide number of uncharacterized paralogous genes that are normally clustered with two additional genes. One of which, in the case of WhiJ, is SCO4542, a small protein belonging to the DNA-binding family that contains a domain of unknown function. This domain has been denominated DUF397 and is thought to interact with WhiJ, preventing it from binding to the operator sequence present in developmental genes (Aínsa et al., 2010). Actually, the DUF397-XRE gene pair encodes proteins that are most abundant in Actinobacteria, which have been assigned the function of class II toxin–antitoxin systems (TAS: TA-systems) among other functions (Makarova et al., 2009). In S. coelicolor, the XRE protein has been predicted to act as an antitoxin, with the associated small DNA-binding protein containing the DUF397 domain acting as a toxin; although its molecular mode of action has not yet been described (Sevin and Barloy-Hubler, 2007; Makarova et al., 2009; Doroghazi and Buckley, 2014).
Toxin–Antitoxin loci systems (TASs) are small genetic elements composed of a stable toxin and its cognate unstable antitoxin. The toxin, when released, prevents or alters cellular processes including translation, DNA replication, and ATP and cell wall synthesis and this activity can lead to cell death or the formation of drug-tolerant persister cells (Schuster and Bertram, 2013). Based on the molecular nature of the antitoxin modules, the TASs are currently grouped into six classes depending on their antitoxin nature: type II, IV, V, and VI are proteins and those in classes I and III are small regulatory RNAs (Lobato-Márquez et al., 2016). Type II class proteins are the most abundant and best described and include both the toxin and antitoxin, small proteins that form a stable complex. The antitoxin blocks the activity of the toxin by hiding the region responsible for toxicity (Goeders and Van Melderen, 2014; Hayes and Kedzierska, 2014).
The use of the TA finder 2.0 http://202.120.12.133/TAfinder/index.php (a TA-systems database web tool) predicted the presence of 42 TAS pairs in the S. coelicolor genome3, of which 15 are classified as XRE/DUF397 (Shao et al., 2011; Xie et al., 2018). In the present work, the putative TAS functionality of one of these XRE/DUF397 protein pairs from S. coelicolor, encoded by SCO4441/SCO4442 and paralogous to whiJ and its downstream gene (SCO4543/SCO4542, respectively), was studied. Consequently it was found that the overexpression of the putative toxin SCO4442 was neither deleterious in the S. coelicolor wild-type strain or in the deletion mutant obtained in this work. These same results were obtained when Streptomyces lividans wild-type strain was used as the host. Therefore, this gene pair does not function as a toxin–antitoxin system, at least under the conditions assayed, as was originally predicted using bioinformatics.
Additionally, we found that the proteins encoded by SCO4441/4442 act as a positive regulator of endogenous antibiotic production in S. coelicolor and were named Scr1 and Scr2, respectively. The overexpression of Scr1, in combination with Scr2, drastically induces the production of antibiotics not only in S. coelicolor, but also in S. lividans, Streptomyces peucetius, Streptomyces steffisburgensis, and Streptomyces sp. CA-240608, as determined from the 19 strains tested. Analysis of the chromatographic peaks of the molecules induced in each case was performed, and an increment in some endogenous compounds and the appearance of new induced metabolites were detected. In conclusion, this protein pair seems to function as a positive regulator in the complex regulatory network of antibiotic production. These results open new doors to the application of Scr1/Scr2 in biotechnology, with the possibility of discovering new and natural products.
Streptomyces strains used in this study are: S. albus J1074, S. argillaceus ATCC 12596, S. coelicolor M145, S. glaucescens Tü49, S. griseus ATCC13273, S. lividans 1326, S. parvulus JI2283, S. peucetius ATCC 27952, S. rochei CECT 3329, S. steffisburgensis NRRL3193, S. vinaceus JI2838, and 8 Streptomyces sp. strains isolated from different soil samples (Supplementary Table S1). These strains were grown on R2YE, MS, PGA, and NA solid media for transformation, sporulation, conjugation, and phenotypic assays, respectively (Coco et al., 1991; Kieser et al., 2000). YES xylose (Sevillano et al., 2016) or NMMP (Kieser et al., 2000) containing 1% of xylose were used in the overexpression assays. Routine plasmid construction and plasmid isolation was done in Escherichia coli DH5α, and E. coli ET12567, a non-methylating strain, was used to obtain the plasmids to be transformed into S. coelicolor. E. coli strain BW25113 (pIJ790) (containing the λ Red system) (Datsenko and Wanner, 2000) and ET12567 (pUZ8002) (harboring the tra genes in the non-transmissible RP4-derivative plasmid pUZ8002) (MacNeil et al., 1992) were used for PCR-targeted mutagenesis of S. coelicolor M145 and conjugation plasmid transfer to the different Streptomyces species. Staphylococcus aureus MB5393, E. coli ATCC25922 and Candida albicans ATCC64124 were used in the antibiogram analysis. Antibiotics were used when needed for plasmid selection (E. coli: 100 μg mL-1 for ampicillin; 50 μg mL-1 for apramycin; 50 μg mL-1 for kanamycin; 34 μg mL-1 for chloramphenicol, and 25 μg mL-1 for nalidixic acid. S. coelicolor: 20 μg mL-1 for neomycin, 10 μg mL-1 for apramycin and 10 μg mL-1 for thiostrepton).
DNA transformation and manipulation of E. coli and S. coelicolor were carried out using the methods by Green and Sambrook (2012) and Kieser et al. (2000), respectively. The plasmids used in this work are listed in Table 1.
The coding regions of SCO4441, SCO4442 or both genes were replaced by an apramycin resistance cassette (aac(3)IV gene) by using REDIRECT PCR-targeting technology (Gust et al., 2003). The primers LS-090 and LS-091 (Supplementary Table S2) were used to generate the mutation cassette from the plasmid pIJ773 (Gust et al., 2003), which was used as the template. The mutated genes were obtained using cosmid SCD6 (Redenbach et al., 1996) and transferred by conjugation from ET12567 (pUZ8002) to S. coelicolor M145. The desired mutants were selected by apramycin resistance and sensitivity to kanamycin. PCR assays confirmed the deletion of the SCO4441, SCO4442 or both genes in S. coelicolor M145.
Qualitative actinorhodin (ACT) production of the S. coelicolor strains was observed on different solid media. Approximately 105 spores were deposited in 5 μL drops onto plates that were incubated at 30°C. ACT production was detected after three to 10 days of growth as a blue halo around the colonies.
Colorimetric quantification of prodiginines (RED) and ACT production was determined by the spectrophotometric method described in Yepes et al. (2011). All experiments were performed in triplicate.
Liquid cultures (10 mL) of S. coelicolor or S. lividans in YES+Xylose, or NMMP+Xylose, containing 20 μg mL-1 for neomycin or 10 μg mL-1 for apramycin, depending on the plasmid used, were incubated at 28°C for 8 days at 200 rpm. Then, 1 mL of the culture was extracted using 0.7 volume of 1% formic acid acidified ethyl acetate and the organic layer was dried in vacuo. The dry extracts were finally resuspended in methanol (100 μL). LC-HRMS-analyses were performed as previously described using a Bruker maXis QTOF mass spectrometer coupled to an Agilent 1200 LC (Martin et al., 2014; Perez-Victoria et al., 2016). Differential peaks where selected by direct comparison of the DAD signal base peak chromatograms.
The multicopy overexpression vectors pNX4441, pNX4442, and pNX4441-42 were derivatives of the pN703GEM3 plasmid (Fernández-Ábalos et al., 2003) and carry the neomycin resistance gene as selective marker. They were obtained by cloning the corresponding coding sequences SCO4441 (scr1), SCO4442 (scr2) or both, previously amplified by PCR using the corresponding oligonucleotides (see Supplementary Table S1), into the NdeI and XhoI sites of the intermediate plasmid pXHis1 (Adham et al., 2001b), yielding pX4441His, pX4442His, and pX4441/42His, respectively. In a second step, the corresponding BgIII/HindIII fragments of these three plasmids were cloned into the same sites of pN702GEM3, obtaining pNX4441, pNX4442, and pNX4441-42. In these constructs, the strong xylanase promoter xysAp (Rodríguez et al., 2005) controls the ORFs of scr1, scr2 and scr1 (scr2 was under the control of its own promoter in pNX4441-42), respectively. In these plasmids the corresponding Scr1, Scr2, and Scr2 were tagged, respectively, with a six His tag at the carboxy terminus.
To obtain conjugative plasmids, a BamHI fragment of 1380 bp containing the oriT and the apramycin resistance, from pIJ773 (Gust et al., 2003), was cloned in the BlgII site of the plasmids pNX4441/42 and pN702Gem3, respectively, obtaining the plasmids pNX4441/42c and pN702Gem3c. Later a 3900-bp band was obtained from the plasmid pNX4441/42c and a 1600-bp band was obtained from the plasmid pN702Gem3c using the enzymes HindIII and NheI. These fragments were individually cloned into the low copy plasmid pHJL401 (Larson and Hershberger, 1986) digested with HindIII and XbaI to obtain the final plasmids pHAX4441/42c and its control pHJL401c, respectively. These plasmids carry resistance genes to thiostrepton and apramycin.
The SCO4441 gene (scr1) encodes a 295 aa protein with a 7.18 isoelectric point, has a molecular weight of 33.59 kDa and has been predicted to be a putative antitoxin. This protein contains two regions, the HTH-XRE domain (aas 23 to 80) and the HipB domain (aas 24 to 62) (NCBI database4). The gene SCO4442 (scr2) encodes a 63 aa protein with a 6.49 isoelectric point, has a molecular weight of 6.48 kDa and has been predicted to be a putative toxin. This protein contains a DUF397 region (aas 11 to 62).
As Scr1 and Scr2 are proposed to form part of a putative toxin–antitoxin system (TAS) (Shao et al., 2011; Xie et al., 2018), here we carried out a study to analyze the effect of its putative toxicity using the over-expression of Scr1, and/or Scr2 in S. coelicolor M145 and in S. lividans 1326. Three multicopy plasmids, pNX4441, pNX4442, and pNX4441/42 (see section “Materials and Methods”) were generated, where the expression of these genes was controlled by the xylanase strong promoter xysAp (Rodríguez et al., 2005). The plasmids and the control, the empty plasmid pN703Gem3, were introduced into both Streptomyces species to assess the possibility of Scr2 acting as a toxin.
The S. coelicolor cells overexpressing the putative toxin in plasmid pNX4442 were viable, generating colonies which were able to differentiate like those obtained with both the control plasmid and the putative antitoxin (pNX4441). A slight delay in differentiation was observed when both genes of the putative TAS were overexpressed (pNX4441/42) (Figure 1A). The same results were obtained when the plasmids were transformed into S. lividans where no toxic effect was observed when the putative toxin encoded in the plasmid pNX4442 was expressed (data not shown).
FIGURE 1. Overexpression of the putative SCO4441/SCO4442 TAS (Scr1/Scr2). (A) S. coelicolor M145 (wt); (B) S. coelicolor Δscr1/scr2. In each panel: colonies obtained after transformation with the different multicopy plasmids overexpressing the putative antitoxin Scr1 (pNX4441), the putative toxin Scr2 (pNX4442), both proteins Scr1/Scr2 (pNX4441/42) and the empty plasmid (pN702Gem3) in R2YE medium (Top); Detail of the morphology of the colonies obtained after 10 days (Bottom).
These results indicated that the protein encoded by scr2 was not acting as a conventional toxin, inducing lethality when overexpressed in S. coelicolor and S. lividans, at least under the conditions used (Figure 1A), as was the case of YefM/YoeBsl, isolated from S. lividans, which was previously characterized in our laboratory (Sevillano et al., 2012).
To exclude the possibility that a single gene copy of scr1, present in the S. coelicolor genome (wild-type), could be sufficient to counteract the toxicity of Scr2 overexpression, a deletion mutant strain lacking both putative TAS genes, ΔSCO4441/42 (Δscr1/scr2), was generated (see section “Materials and Methods”) and used as a host for plasmids pNX4441, pNX4442, and pNX4441/42. The results obtained when overexpressing these plasmids in the new mutant strain were similar to those described for the wild-type (Figure 1B). Hence, the function of these two genes did not correspond to a TAS under these particular conditions. Interestingly, the mutant Δscr1/scr2 did not show any phenotype divergent from that of the wild-type in the conditions used (see section “Discussion”).
Furthermore, a clear phenotype of colored antibiotic induction was observed when the strains of S. coelicolor wild-type and Δscr1/scr2, carrying the overexpression plasmid pNX4441/42, were grown on several solid media such as NMMP+Xyl. A high overproduction of the blue-red antibiotic ACT was observed when scr1 was overexpressed either alone or with scr2 in the wild-type strain. Nevertheless, when the S. coelicolor Δscr1/scr2 strain was used as a host, this high overproduction of ACT (blue color) was only observed with the overexpression of both genes at the same time (pNX4441/42) (Figure 2). These results indicated that Scr1 was a positive regulator of ACT production and required the presence of Scr2 to function.
FIGURE 2. Study of the induction of ACT by overexpression of scr1, scr2, and scr1/scr2 in S. coelicolor M145 and S. coelicolor Δscr1/scr2 strains on NMMP+xyl. Two clones are shown for the empty control, plasmid pN702Gem3, and five clones for those transformed with pNX4441 (Scr1), pNX4442(Scr2), or pNX4441/42 (Scr1/Scr2).
To further delve into the functionality of these genes, two new mutants lacking only one of the two genes, S. coelicolor Δscr1 and S. coelicolor Δscr2, were generated (see section “Materials and Methods”). These two new single mutant strains, the double Δscr1/scr2 mutant and the wild-type were transformed with four plasmids, pNX4441, pNX4442, pNX4441/42, and pN702Gem3, and the production in liquid cultures was analyzed. The increase of the production of colored antibiotics was observed in all of the liquid media assayed [R2YE, NMMP+Xyl (data not shown) and YES+Xyl] when pNX4441/42 was used. Since the highest production was obtained in YES+Xyl, this medium was used to carry out the rest of the experiments.
Once again, a high level of ACT was induced by Scr1 (pNX4441) in the wild-type strain. This induction was also observed in the S. coelicolor Δscr1 strain, but not in the two strains lacking the SCO4442 gene (Δscr2 and Δscr1/scr2) (Figure 3A). Overproduction of the colored antibiotics was also observed in strains Δscr2 and Δscr1/scr2, but only when the plasmid pNX4441/42 was used (Figures 3A,B). Antibiotic production in these cultures was quantified, and the yield of ACT was between 450 and 550 μM after expressing Scr1 alone or together with Scr2 in the wild-type M145 strain. The production of prodiginines (RED) was also induced under the same conditions reaching concentrations of 35–45 μM in this strain (Figure 3C). The quantification of these antibiotics in the Δscr2 and Δscr1/scr2 transformant strains reinforced the hypothesis that Scr1 acts as a positive regulator of antibiotic production and requires the presence of the protein encoded by scr2. Therefore, these two genes must act in conjunction (Figures 3B,C).
FIGURE 3. Actinorhodin (ACT) and RED induction by Scr1 and/or Scr2 in liquid YES+Xyl. (A) Colored antibiotic production induced by Scr1 (pNX4441), Scr2 (pNX4442), and Scr1/Scr2 (pNX4441/42) in different strains of S. coelicolor (M145, Δscr1, Δscr2, and Δscr1/scr2); (B) colorimetric quantification of ACT production of the cultures shown in panel A; (C) colorimetric quantification of RED production of the cultures shown in panel A. The cultures were grown in YES+Xyl for 8 days. Error bars correspond to three independent experiments.
Additionally, the analysis of the S. coelicolor M145 cultures overexpressing Scr1 and Scr2 by LC-HRMS was performed for identifying new putative compounds, originating from silent pathways that may be promoted by Scr1/Scr2. Several compounds were produced de novo by the action of Scr1/2 and were putatively identified as SEK 4, SEK 4b, ε-actinorhodin and γ-actinorhodin, and one putative new compound with the molecular formula C32H24O14 and an UV absorbance similar to actinorhodin (Figure 4). SEK 4 and SEK 4b have been previously described as shunt polyketide intermediates, non-enzymatically cyclized products from the biosynthesis of actinorhodin (Fu et al., 1994; Jetter et al., 2013).
FIGURE 4. Metabolites induced by Scr1/2 in S. coelicolor. (A) Metabolite production induced by the overexpression of Scr1/Scr2 (pNX4441/42) in S. coelicolor M145 in YES+Xyl and detected by UV-Vis absorbance (200–900 nm) base peak chromatography. (B) Amplified region of the four most different peaks produced and their predicted compounds (1) SEK 4/SEK 4B; (2) ε-actinorhodin; (3) C32H24O14; (4) γ-actinorhodin.
The ability of Scr1, Scr2, and Scr1/Scr2 from S. coelicolor to induce antibiotics was also tested in S. lividans 1326 in the same way as above. The plasmids pN702Gem3, pNX4441, pNX4442, and pNX4441/42 were introduced in this strain, cultured in liquid YES+Xyl and compared. As in S. coelicolor, production of ACT (59 μM) and RED (430 μM) was detected when this strain was transformed with the plasmids pNX4441 and pNX4441/42, but not observed when transformed with pNX4442. Interestingly the production of RED was almost ten times higher than the production reached for this antibiotic in S. coelicolor (Figures 5A,B). LC-HRMS analysis of the cultures of S. lividans 1326 overexpressing scr1 and scr2 detected the production of SEK 4, SEK 4b, fogacin, a putative new compound with the molecular formula C32H28O14 and a prodigiosin of the molecular formula C25H33N3O with six isomeric prodigiosins found in the Dictionary of Natural Products (DNP) (Figure 6). Fogacin has been previously described as a cyclic octaketide obtained from a Streptomyces sp. (strain Tü 6319) isolated from a contaminated soil in Romania (Radzom et al., 2006).
FIGURE 5. Antibiotic induction by Scr1, Scr2 and Scr1/Scr2 in S. lividans 1326 in liquid YES+Xyl. (A) Colored antibiotic production induced by Scr1 (pNX4441), Scr2 (pNX4442), and Scr1/Scr2 (pNX4441/42). (B) Colorimetric quantification of ACT and RED production of the cultures shown in panel A. Control: empty plasmid pN702Gem3. The cultures were grown in YES+Xyl for 8 days. Error bars correspond to three independent experiments.
FIGURE 6. Metabolites induced by Scr1/2 in S. lividans. (A) Metabolite production induced by overexpression of Scr1/Scr2 (pNX4441/42) in S. lividans in YES+Xyl and detected by UV-Vis absorbance (200–900 nm) base peak chromatography. (B) Amplified region of the four most different peaks produced and their predicted compounds (1) SEK 4/SEK 4B; (2) Fogacin; (3) C32H28O14; (4) Prodigiosin 25b.
A potential strategy for improving or inducing cryptic metabolites of different species of Streptomyces producers is the cloning of a positive regulator of antibiotic production. Based on the results obtained from the overexpression of Scr1/2 in S. coelicolor and S. lividans, these genes appeared to be good candidates for obtaining new natural products using this type of strategy. Therefore, we generated a plasmid that could be used to transfer these regulators into other Streptomyces species. To do so, a conjugative plasmid derived from pHJL401 was generated (pHJL401c) and used to introduce scr1 and scr2 in the final plasmid pHAX4441/42c. These two new plasmids were transferred from E. coli to nine Streptomyces species known to produce different antibiotics: S. albus, S. argillaceus, S. glaucescens, S. griseus, S. parvulus, S. peucetius, S. rochei, S. steffisburgensis and S. vinaceus. The conjugants obtained were grown in liquid YES+Xyl containing 10 μg mL-1 of apramycin for 8 days and the metabolites produced and extracted with acidified ethyl acetate were analyzed using LC-HRMS to look for the increased production of known or new compounds. This strategy was successful in S. peucetius and S. steffisburgensis. In S. peucetius, two putative new compounds were produced (peaks B and C) and an additional one (peak A) was greatly induced by Scr1/Scr2 (Figure 7). The analysis of the differentially detected peaks did not correspond with those of known compounds. This may have occurred in two of the cases (peaks A and B) due to the lack of ionization in both positive and negative ESI. In the case of peak C, the only component found in the DNP with the predicted molecular formula C18H14N2O4 (Supplementary Figure S1) corresponded to Flazine methyl ester, which had an UV spectrum that did not coincide with the spectrum determined experimentally (Buckingham, 2017). In S. steffisburgensis, an induced compound, N-[1-Hydroxy-2-(1H-indol-3-yl)-2-oxoethyl]acetamide, was highly produced and putatively identified (Figure 8). No changes were observed in the peaks obtained in the other studied strains (Supplementary Figures S2–S5).
FIGURE 7. Metabolites induced by Scr1/2 in S. peucetius. Metabolite production induced by overexpression of Scr1/Scr2 (pHAX4441/42c) in YES+Xyl for 8 days and detected by UV-Vis absorbance (200–900 nm) base peak chromatography. Peaks A, B, C: unknown compounds.
FIGURE 8. Metabolites induced by Scr1/2 in S. steffisburgensis. (A) Metabolite production induced by overexpression of Scr1/Scr2 (pHAX4441/42c) in S. steffisburgensis in YES+Xyl for 8 days and detected by UV-Vis absorbance (200–900 nm) base peak chromatography. (B) Amplified region of the peak produced and their predicted compound: N-[1-Hydroxy-2-(1H-indol-3-yl)-2-oxoethyl]acetamide.
Moreover, eight additional strains of Streptomyces sp., with no clear antibiotic activity against the microorganisms tested (S. aureus, E. coli, and C. albicans) when grown in seven different types of media, were also used in the experiment. Conjugants were only obtained for two of the strains: Streptomyces sp. CA-240608 and Streptomyces sp. CA-258987. LC-HRMS-analysis of liquid culture extracts of Streptomyces sp. CA-240608 detected the overproduction of one component with the molecular formula of C25H16N4O6 (Supplementary Figure S1) that could correspond to any of the isomers Izumiphenazine A, Izumiphenazine B, Phenazinoline D or Phenazinoline E (Figure 9). These were the only compounds described in the DNP with this predicted molecular formula. Interestingly, the antibiogram activity of the clones of this strain overexpressing Scr1/Scr2 exhibited slight antibiotic activity against E. coli. By contrast, the control strain did not show any antibiotic activity (Figure 9). No changes in the production pattern of the strain Streptomyces sp. CA-258987 were detected (Supplementary Figure S5).
FIGURE 9. Metabolites induced by Scr1/2 in Streptomyces sp. CA-240608. (A) Metabolite production induced by overexpression of Scr1/Scr2 (pHAX4441/42c) in Streptomyces sp. CA-240608 in YES+Xyl and detected by UV-Vis absorbance (200–900 nm) base peak chromatography. (B) Amplified region of the peak produced and their predicted compound C25H16N4O6: Izumiphenazine A/B, Phenazinoline D/E. (C) Antibiogram against E. coli using 100 μL of the supernatant of Streptomyces sp. CA-240608 cultures carrying the indicated plasmid.
Therefore, these results validate the use of Scr1/Scr2 as a biotechnological tool for increasing or promoting different metabolite pathways in some Streptomyces species. The overexpression of Scr1/Scr2 strongly activates antibiotic production in S. coelicolor and S. lividans, and may be useful as a means for searching for new natural products in some Streptomyces species.
In this work, the positive role of the Scr1/Scr2 proteins in the regulation of antibiotic production has been established. This role has not been described previously and their function as a putative toxin–antitoxin system has only been inferred by bioinformatic comparison (Shao et al., 2011; Xie et al., 2018). One orthologous gene of scr1, with 99% identity, was previously cloned from S. lividans and denominated cpb1. The protein encoded for this gene was identified as a DNA binding protein that specifically binds to the promoter of the chitinase encoding gene chiA. Deletion of the gene cpb1 partially relieves the glucose repression of chitinase production and chitinases are produced up to wild-type levels when grown in a medium containing colloidal chitin without glucose (Fujii et al., 2005). A qualitative experiment has been conducted, comparing the chitinase halo of the S. coelicolor wild-type strain and the Δscr1 in the presence of 0.15% colloidal chitin with or without glucose. No clear differences were observed for both strains (Supplementary Figure S6A).
Based on sequence homology, Aínsa et al. (2010) classified the gene scr1 (SCO4441) as one of the 26 whiJ (SCO4543) paralogs on the S. coelicolor chromosome. These whiJ genes vary considerably with respect to their conservation in other organisms, being widespread in streptomycetes but generally absent from any other bacterial genomes. The scr1 gene is present in all Streptomycineae and also in other complex actinomycetes such as Kitasatospora, Microtetraspora, Streptosporangium (Chandra and Chater, 2014). In S. coelicolor, most of these whiJ paralogs (22 out of 26 members) are neighbors of genes that encode either a very small binding protein with a DUF397 domain, like in the case of Scr2 (SCO4442), or by an antisigma factor or by both (Chandra and Chater, 2014). A model in which WhiJ binds to some operator sequences repressing developmental genes has been described. Interestingly, this repression might be released by its interaction with the small WhiJ-associated protein, SCO4542 (scr2 paralog), whose activity is prevented by an unknown signal (Aínsa et al., 2010). These authors demonstrated that the total deletion of whiJ (scr1 paralog) does not lead to a clear effect. However, the deletion of SCO4542 causes colonies to appear bald (bld) in MSA medium and results in the whi phenotype in R2YE. Both of these phenotypes were suppressed by the simultaneous deletion of whiJ. The authors also proposed that the deletion of SCO4542 would result in the constitutive binding of WhiJ to its target(s), repressing development and leading to a bld colony phenotype. In other words, SCO4542 prevents WhiJ from binding to target DNA and represses development (Aínsa et al., 2010).
Another protein containing a DUF397 conserved domain, which is critical for the correct progression of the developmental program and antibiotic production, is BldB (SCO5723) (Eccleston et al., 2006; Akpe San Román et al., 2010). It has been suggested that a partner, included in the Hpb (for helix-turn-helix partner of BldB) family, modulates its activity (Eccleston et al., 2006; Doroghazi and Buckley, 2014). Hpb is predicted to have an XRE-class helix-turn-helix domain, and diversification of the bldb/hpb family gene pairs suggests that a mutation in one gene encourages a compensatory change in its partner at the same locus. Based on different aspects of the relationship between this gene pair, some authors have proposed that the proteins containing the XRE domain function as antitoxins whereas DUF397 family proteins are novel toxins (Makarova et al., 2009; Doroghazi and Buckley, 2014).
As stated previously, S. coelicolor has 15 XRE-DUF397 pairs that have been predicted to act as toxin–antitoxin systems (Shao et al., 2011; Xie et al., 2018). Until now, this group of genes has not been studied experimentally. In this work, we chose to begin by studying the gene pair comprised of scr1/scr2 in an attempt to describe the mode of action of a new group of TAS. However, under the conditions used in this work, this system did not behave as a typical TAS on which overexpression of the putative toxin (Scr2 in this case), in the absence of adequate levels of the putative antitoxin, (Scr1 in our study) produces a lethal effect. In our experiments, the number of colonies obtained in the transformation with the plasmids containing the antitoxin, the toxin or both genes was similar suggesting the no toxic effect of the toxin.
The mutation of these genes did not have a drastic phenotype when grown on several of the media assayed (Supplementary Figure S6B). Only the Δscr1 strain showed an accelerated differentiation when grown in NA solid media. No other clear differences were observed for the different strains in R2YE, R5A, or in MSA, as observed by Aínsa et al. (2010) with WhiJ/SCO4542 pair.
According to our results it is possible that Scr1 and Scr2 interact, although evidence of this interaction could not be obtained using purified proteins due to the insolubility of Scr2. Nevertheless, an indirect relationship was observed in S. coelicolor using strains Δscr2 and Δscr1/scr2 overexpressing Scr1. Induction of colored antibiotics was only observed when overexpression of Scr1 and Scr2 was carried out in these strains, but not with Scr1 on its own.
It is worth noting that several additional products of ACT and RED were induced in S. coelicolor by the overexpression of the regulator Scr1 (Figure 4). For example, production of the compounds Sek4 and Sek4b were detected in this strain. These two products were also detected in S. lividans. In this species, fogacin was also overproduced due to the overexpression of Scr1 and Scr2. This compound differs from all known intermediates of actinorhodin described and is a product of stereospecific cyclization and miscellaneous tailoring steps (Radzom et al., 2006). To our knowledge, the production of these three compounds has not been previously reported in S. lividans. In addition, the induction of other compounds was observed under the culture conditions used. This was the case for S. steffisburgensis in which the production of the putative N-[1-Hydroxy-2-(1H-indol-3-yl)-2-oxoethyl] acetamide was detected. This compound was previously described in Streptomyces ramulosus Tü 34 (Chen et al., 1983). Here, the overexpression of Scr1/Scr2 in a strain that did not present any clear antibiotic activity against Gram positive, Gram negative bacteria or yeasts, under different culture conditions, was also analyzed. The overexpression of Scr1/Scr2 in this strain permitted us to detect a weak antibiotic activity against E. coli that was absent in the control strain. Under these conditions, this strain, Streptomyces sp. CA-240608, produces izumiphenazines A/B or Phenazinoline D/E (Figure 9). The production of izumiphenazines A/B was previously reported from Streptomyces sp. IFM 11204, a strain isolated from a soil sample collected from Izumi forest in Chiba city, Japan. These compounds exert moderate activity over TRAIL-resistant AGS cells, a cell line typically used for evaluating cancer cell apoptosis (Abdelfattah et al., 2010). The compound Phenazinoline was previously reported from S. diastaticus YIM DT26, isolated from a soil sample collected in Yunnan, China (Ding et al., 2011), and from the strain of S. fradiae A196 that was isolated from hailstone in Gijón, Spain (Sarmiento-Vizcaino et al., 2018). These results suggest that this strategy may be useful for some Streptomyces strains for increasing the level of production of some compounds. However, the regulators analyzed do not present a general activating action in all of the strains studied, as was initially expected.
RS and LS conducted most of the experiments. JM performed the LC-HRMS-analysis. LS, JM, IG, OG, MD, and RS analyzed the results. MD, LS, and RS conceived the experiments and wrote the manuscript. All authors have read and approved the final manuscript.
The laboratory of RS and MD was funded by the grants PCIN-2014-067 and BIO2015-66958-R from the Spanish Ministry of Economy, Industry and Competitiveness (MINECO) and CLU-2017-03 from the Junta de Castilla y León (JCyL).
The authors declare that the research was conducted in the absence of any commercial or financial relationships that could be construed as a potential conflict of interest.
We acknowledge support of the publication fee by the CSIC Open Access Publication Support Initiative through its Unit of Information Resources for Research (URICI). We also would like to thank Ana M. Martínez-Carrasco for her excellent technical support and Emma Keck for her help in editing the English.
The Supplementary Material for this article can be found online at: https://www.frontiersin.org/articles/10.3389/fmicb.2018.02791/full#supplementary-material
Abdelfattah, M. S., Kazufumi, T., and Ishibashi, M. (2010). Izumiphenazines A-C: isolation and structure elucidation of phenazine derivatives from Streptomyces sp. IFM 11204. J. Nat. Prod. 73, 1999–2002. doi: 10.1021/np100400t
Adham, S. A., Campelo, A. B., Ramos, A., and Gil, J. A. (2001a). Construction of a xylanase-producing strain of Brevibacterium lactofermentum by stable integration of an engineered xysA gene from Streptomyces halstedii JM8. Appl. Environ. Microbiol. 67, 5425–5430.
Adham, S. A., Honrubia, P., Díaz, M., Fernández-Ábalos, J. M., Santamaría, R. I., and Gil, J. A. (2001b). Expression of the genes coding for the xylanase Xys1 and the cellulase cel1 from the straw-decomposing Streptomyces halstedii JM8 cloned into the amino-acid producer Brevibacterium lactofermentum ATCC13869. Arch. Microbiol. 177, 91–97.
Aínsa, J. A., Bird, N., Ryding, N. J., Findlay, K. C., and Chater, K. F. (2010). The complex whiJ locus mediates environmentally sensitive repression of development of Streptomyces coelicolor A3(2). Antonie Van Leeuwenhoek 98, 225–236. doi: 10.1007/s10482-010-9443-3
Akpe San Román, S., Facey, P. D., Fernández-Martínez, L. T., Rodríguez, C., Vallín, C., Del Sol, R., et al. (2010). A heterodimer of EsxA and EsxB is involved in sporulation and is secreted by a Type VII secretion system in Streptomyces coelicolor. Microbiology 156(Pt 6), 1719–1729. doi: 10.1099/mic.0.037069-0
Buckingham, J. (2017). Dictionary of Natural Products on DVD v26:1, 2017 Edn. Boca Raton, FL: Chapman & Hall/CRC.
Chandra, G., and Chater, K. F. (2014). Developmental biology of Streptomyces from the perspective of 100 actinobacterial genome sequences. FEMS Microbiol. Rev. 38, 345–379. doi: 10.1111/1574-6976.12047
Chater, K. F. (2016). Recent advances in understanding Streptomyces. F1000Res 5:2795. doi: 10.12688/f1000research.9534.1
Chen, Y., Zeeck, A., Chen, Z., and Zahner, H. (1983). Metabolic products of microorganisms. 222. beta-oxotryptamine derivatives isolated from Streptomyces ramulosus. J Antibiot. 36, 913–915. doi: 10.7164/antibiotics.36.913
Coco, E. A., Narva, K. E., and Feitelson, J. S. (1991). New classes of Streptomyces coelicolor A3(2) mutants blocked in undecylprodigiosin (Red) biosynthesis. Mol. Gen. Genet. 227, 28–32. doi: 10.1007/BF00260702
Datsenko, K. A., and Wanner, B. L. (2000). One-step inactivation of chromosomal genes in Escherichia coli K-12 using PCR products. Proc. Natl. Acad. Sci. U.S.A. 97, 6640–6645. doi: 10.1073/pnas.120163297
den Hengst, C. D., Tran, N. T., Bibb, M. J., Chandra, G., Leskiw, B. K., and Buttner, M. J. (2010). Genes essential for morphological development and antibiotic production in Streptomyces coelicolor are targets of BldD during vegetative growth. Mol. Microbiol. 78, 361–379. doi: 10.1111/j.1365-2958.2010.07338.x
Ding, Z. G., Li, M. G., Ren, J., Zhao, J. Y., Huang, R., Wang, Q. Z., et al. (2011). Phenazinolins A-E: novel diphenazines from a tin mine tailings-derived Streptomyces species. Org. Biomol. Chem. 9, 2771–2776. doi: 10.1039/c1ob05044c
Doroghazi, J. R., and Buckley, D. H. (2014). Intraspecies comparison of Streptomyces pratensis genomes reveals high levels of recombination and gene conservation between strains of disparate geographic origin. BMC Genomics 15:970. doi: 10.1186/1471-2164-15-970
Eccleston, M., Willems, A., Beveridge, A., and Nodwell, J. R. (2006). Critical residues and novel effects of overexpression of the Streptomyces coelicolor developmental protein BldB: evidence for a critical interacting partner. J. Bacteriol. 188, 8189–8195. doi: 10.1128/JB.01119-06
Fernández-Ábalos, J. M., Reviejo, V., Díaz, M., Rodríguez, S., Leal, F., and Santamaría, R. I. (2003). Posttranslational processing of the xylanase Xys1L from Streptomyces halstedii JM8 is carried out by secreted serine proteases. Microbiology 149, 1623–1632. doi: 10.1099/mic.0.26113-0
Fu, H., Ebert-Hhosla, S., Hopwood, D. A., and Khosla, C. (1994). Engineered biosynthesis of novel polyketides: dissection of the catalytic specificiy of the act ketoreductase. J. Am. Chem. Soc. 116, 4166–4170. doi: 10.1021/ja00089a003
Fujii, T., Miyashita, K., Ohtomo, R., and Saito, A. (2005). DNA-binding protein involved in the regulation of chitinase production in Streptomyces lividans. Biosci. Biotechnol. Biochem. 69, 790–799. doi: 10.1271/bbb.69.790
Goeders, N., and Van Melderen, L. (2014). Toxin-antitoxin systems as multilevel interaction systems. Toxins 6, 304–324. doi: 10.3390/toxins6010304
Green, M. R., and Sambrook, J. (2012). Molecular Cloning: A Laboratory Manual, 4th Edn. New York, NY: Cold Spring Harbor Laboratory Press.
Gust, B., Challis, G. L., Fowler, K., Kieser, T., and Chater, K. F. (2003). PCR-targeted Streptomyces gene replacement identifies a protein domain needed for biosynthesis of the sesquiterpene soil odor geosmin. Proc. Natl. Acad. Sci. U.S.A. 100, 1541–1546. doi: 10.1073/pnas.0337542100
Hayes, F., and Kedzierska, B. (2014). Regulating toxin-antitoxin expression: controlled detonation of intracellular molecular timebombs. Toxins 6, 337–358. doi: 10.3390/toxins6010337
Jetter, P., Steinert, C., Knauer, M., Zhang, G., Bruhn, T., Wiese, J., et al. (2013). New bhimamycins from Streptomyces sp. AK 671. J. Antibiot. 66, 719–726. doi: 10.1038/ja.2013.82
Kieser, T., Hopwood, D. A., Bibb, J. M., Chater, K. F., and Buttner, M. J. (2000). Practical Streptomyces Genetics. Norwich: John Innes Foundation.
Larson, J. L., and Hershberger, C. L. (1986). The minimal replicon of a streptomycete plasmid produces an ultrahigh level of plasmid DNA. Plasmid 15, 199–209. doi: 10.1016/0147-619X(86)90038-7
Lobato-Márquez, D., Díaz-Orejas, R., and García-Del Portillo, F. (2016). Toxin-antitoxins and bacterial virulence. FEMS Microbiol. Rev. 40, 592–609. doi: 10.1093/femsre/fuw022
MacNeil, D. J., Gewain, K. M., Ruby, C. L., Dezeny, G., Gibbons, P. H., and MacNeil, T. (1992). Analysis of Streptomyces avermitilis genes required for avermectin biosynthesis utilizing a novel integration vector. Gene 111, 61–68. doi: 10.1016/0378-1119(92)90603-M
Makarova, K. S., Wolf, Y. I., and Koonin, E. V. (2009). Comprehensive comparative-genomic analysis of type 2 toxin-antitoxin systems and related mobile stress response systems in prokaryotes. Biol. Direct 4:19. doi: 10.1186/1745-6150-4-19
Martin, J., Crespo, G., Gonzalez-Menendez, V., Perez-Moreno, G., Sanchez-Carrasco, P., Perez-Victoria, I., et al. (2014). MDN-0104, an antiplasmodial betaine lipid from Heterospora chenopodii. J. Nat. Prod. 77, 2118–2123. doi: 10.1021/np500577v
Novichkov, P. S., Kazakov, A. E., Ravcheev, D. A., Leyn, S. A., Kovaleva, G. Y., Sutormin, R. A., et al. (2013). RegPrecise 3.0–a resource for genome-scale exploration of transcriptional regulation in bacteria. BMC Genomics 14:745. doi: 10.1186/1471-2164-14-745
Ortet, P., De Luca, G., Whitworth, D. E., and Barakat, M. (2012). P2TF: a comprehensive resource for analysis of prokaryotic transcription factors. BMC Genomics 13:628. doi: 10.1186/1471-2164-13-628
Perez-Victoria, I., Martin, J., and Reyes, F. (2016). Combined LC/UV/MS and NMR strategies for the dereplication of marine natural products. Planta Med. 82, 857–871. doi: 10.1055/s-0042-101763
Radzom, M., Zeeck, A., Antal, N., and Fiedler, H. P. (2006). Fogacin, a novel cyclic octaketide produced by Streptomyces strain Tu 6319. J. Antibiot. 59, 315–317. doi: 10.1038/ja.2006.45
Redenbach, M., Kieser, H. M., Denapaite, D., Eichner, A., Cullum, J., Kinashi, H., et al. (1996). A set of ordered cosmids and a detailed genetic and physical map for the 8 Mb Streptomyces coelicolor A3(2) chromosome. Mol. Microbiol. 21, 77–96. doi: 10.1046/j.1365-2958.1996.6191336.x
Rodríguez, S., Santamaría, R. I., Fernández-Ábalos, J. M., and Díaz, M. (2005). Identification of the sequences involved in the glucose-repressed transcription of the Streptomyces halstedii JM8 xysA promoter. Gene 351, 1–9. doi: 10.1016/j.gene.2005.03.007
Sarmiento-Vizcaino, A., Espadas, J., Martin, J., Brana, A. F., Reyes, F., Garcia, L. A., et al. (2018). Atmospheric precipitations, hailstone and rainwater, as a novel source of Streptomyces producing bioactive natural products. Front. Microbiol. 9:773. doi: 10.3389/fmicb.2018.00773
Schuster, C. F., and Bertram, R. (2013). Toxin-antitoxin systems are ubiquitous and versatile modulators of prokaryotic cell fate. FEMS Microbiol. Lett. 340, 73–85. doi: 10.1111/1574-6968.12074
Sevillano, L., Díaz, M., Yamaguchi, Y., Inouye, M., and Santamaría, R. I. (2012). Identification of the first functional toxin-antitoxin system in Streptomyces. PLoS One 7:e32977. doi: 10.1371/journal.pone.0032977
Sevillano, L., Vijgenboom, E., van Wezel, G. P., Díaz, M., and Santamaría, R. I. (2016). New approaches to achieve high level enzyme production in Streptomyces lividans. Microb. Cell Fact. 15:28. doi: 10.1186/s12934-016-0425-7
Sevin, E. W., and Barloy-Hubler, F. (2007). RASTA-bacteria: a web-based tool for identifying toxin-antitoxin loci in prokaryotes. Genome Biol. 8:R155. doi: 10.1186/gb-2007-8-8-r155
Shao, Y., Harrison, E. M., Bi, D., Tai, C., He, X., Ou, H. Y., et al. (2011). TADB: a web-based resource for Type 2 toxin-antitoxin loci in bacteria and archaea. Nucleic Acids Res. 39, D606–D611. doi: 10.1093/nar/gkq908
Xie, Y., Wei, Y., Shen, Y., Li, X., Zhou, H., Tai, C., et al. (2018). TADB 2.0: an updated database of bacterial type II toxin-antitoxin loci. Nucleic Acids Res. 46, D749–D753. doi: 10.1093/nar/gkx1033
Keywords: Streptomyces, positive regulator, antibiotic production, xenobiotic response element, toxin–antitoxin
Citation: Santamaría RI, Sevillano L, Martín J, Genilloud O, González I and Díaz M (2018) The XRE-DUF397 Protein Pair, Scr1 and Scr2, Acts as a Strong Positive Regulator of Antibiotic Production in Streptomyces. Front. Microbiol. 9:2791. doi: 10.3389/fmicb.2018.02791
Received: 14 September 2018; Accepted: 30 October 2018;
Published: 16 November 2018.
Edited by:
Marie-Joelle Virolle, Centre National de la Recherche Scientifique (CNRS), FranceReviewed by:
Sébastien Rigali, Université de Liège, BelgiumCopyright © 2018 Santamaría, Sevillano, Martín, Genilloud, González and Díaz. This is an open-access article distributed under the terms of the Creative Commons Attribution License (CC BY). The use, distribution or reproduction in other forums is permitted, provided the original author(s) and the copyright owner(s) are credited and that the original publication in this journal is cited, in accordance with accepted academic practice. No use, distribution or reproduction is permitted which does not comply with these terms.
*Correspondence: Ramón I. Santamaría, c2FudGFAdXNhbC5lcw== Margarita Díaz, bWFyZGlAdXNhbC5lcw==
Disclaimer: All claims expressed in this article are solely those of the authors and do not necessarily represent those of their affiliated organizations, or those of the publisher, the editors and the reviewers. Any product that may be evaluated in this article or claim that may be made by its manufacturer is not guaranteed or endorsed by the publisher.
Research integrity at Frontiers
Learn more about the work of our research integrity team to safeguard the quality of each article we publish.