- 1Laboratorio de Referencia de Escherichia coli (LREC), Departamento de Microbioloxía e Parasitoloxía, Facultade de Veterinaria, Universidade de Santiago de Compostela (USC), Lugo, Spain
- 2Unidad de Microbiología, Hospital Universitario Lucus Augusti (HULA), Lugo, Spain
This is a wide epidemiological study of 499 E. coli isolates recovered from 179 outbreaks of enteric colibacillosis from pig production farms in Spain during a period of 10 years. Most samples were of diarrheagenic cases occurred during the post-wean period (PWD) which showed to be significantly associated with ETEC (67%) followed by aEPEC (21.7%). On the contrary, aEPEC was more prevalent (60.3%) among diarrheas of suckling piglets, followed by ETEC (38.8%). STEC/ETEC or STEC were recovered in 11.3 and 0.9% of PWD and neonatal diarrhea, respectively. Detection of the F4 colonization factor was not significantly different between isolates recovered from neonatal pigs and those recovered post wean (40.5 versus 27.7%) while F18 was only present among PWD isolates (51.5% of ETEC, STEC, and STEC/ETEC isolates). We also found a high prevalence of resistance to colistin related to the presence of the mcr-1 gene (25.6% of the diarreagenic isolates). The characterization of 65 representative mcr-1 isolates showed that all were phenotypically resistant to colistin (>2 μg/ml), and most (61 of 65) multidrug-resistant (MDR). Six ETEC and one STEC mcr-1 isolates were also carriers of ESBL genes. In addition, other seven mcr-1 isolates harbored mcr-4 (three ETEC) and mcr-5 (two ETEC and two aEPEC) genes. In the phylogenetic analysis of the 65 mcr-1 diarrheagenic isolates we found that more than 50% (38 out of 65) belonged to A-ST10 Cplx and from those, 29 isolates showed the clonotype CH11-24. In this study, we also recovered 18 ST131 isolates including seven mcr-1 carriers. To the best of our knowledge, this would be the first report of ST131 mcr-1 isolation in pigs. Worryingly, the swine mcr-1 ST131 carriers also showed MDR, including to trimethoprim-sulfamethoxazole, tobramycin, gentamicin and ciprofloxacin. In the PFGE-macrorestriction comparison of clinical swine and human ST131, we found high similarities (≥85%) between two pig and two human ST131 isolates of virotype D5. Acquisition of mcr-1 by this specific clone means an increased risk due to its special feature of congregating virulence and resistance traits, together with its spread capability. Here we show a potential zoonotic swine source of ST131.
Introduction
Neonatal and post-weaning diarrheal (PWD) disease affecting pigs during the first weeks after birth result in significant economic losses for the pig industry due to mortality, decreased weight gain, costs derived of treatment and handling, and vaccinations (Fairbrother et al., 2005; Luppi, 2017).
Among the etiologic agents, there are certain Escherichia coli pathotypes commonly implicated in enteric diseases in piglets, namely, Shiga toxin-producing E. coli (STEC) and enterotoxigenic E. coli (ETEC). STEC isolates carrying the shiga toxin type 2e (Stx2e) are the causative agents of edema disease in weaning piglets, while ETEC isolates encoding the heat stable (STa, STb) and/or heat labile (LT) enterotoxins cause secretory diarrhea in newborn and weaned piglets (Nagy et al., 1999; Fairbrother et al., 2005; Fairbrother and Gyles, 2012). Furthermore, some isolates harbor both the Stx2e and enterotoxin genes, being able to cause symptoms of edema disease and diarrhea in the same animal (STEC/ETEC) (Barth et al., 2011). A common feature of STEC and/or ETEC swine pathotypes is the expression of specific fimbrial adhesins which allow bacterial colonization of the mucosal surface. The most commonly reported are of types F4 (previously known as K88) and F18 (F107, 2134P, and 8813), both with different antigenic variants: three for F4 (ab, ac, and ad), with F4ac being the most prevalent, and two main variants for F18 (F18ab associated with edema disease and F18ac with PWD) (Westerman et al., 1988; Rippinger et al., 1995; Francis, 2002). Other associated fimbriae of lower prevalence include F5 (K99), F6 (987P), and F41, whose number of active receptors present on the intestinal epithelial cells decreases with age (Vu Khac et al., 2006; Luppi et al., 2016). Enteropathogenic E. coli (EPEC) is another pathotype found in pigs with enteric colibacillosis (Brand et al., 2017; Malik et al., 2017) which was first associated with diarrhea in infants (Shuman and Stock, 1956). EPEC isolates possess an outer membrane protein adhesin or intimin (Eae), responsible for intimate attachment of the bacteria to the host intestinal epithelium, which together with a complex secretion system leads to the development of the “attaching and effacing” (AE) lesion (Frankel and Phillips, 2008).
Colistin (polymyxin E) is one of the few cationic antimicrobial peptides commercialized in both human and veterinary medicine. In humans, colistin is used as a last resort against infections of multidrug-resistant (MDR) Gram-negative bacteria (Poirel et al., 2017). However, it has been extensively used since the 1960s in food animals, and particularly in swine with different purposes: therapeutically, prophylactically, and even for growth promotion (Rhouma et al., 2016). Since the description of the plasmid-mediated colistin resistance mcr-1 gene in late 2015 (Liu et al., 2016), several mcr genes have been described (Xavier et al., 2016; Borowiak et al., 2017; Carattoli et al., 2017; Liu et al., 2017; García et al., 2018; Hammerl et al., 2018; Rebelo et al., 2018). Livestock, and particularly pig farming, has been singled out as the principal reservoir for colistin resistance spread (Rhouma et al., 2016). Besides, co-occurrence on the same plasmid of mcr and extended-spectrum beta-lactamase (ESBL) or other beta-lactamase genes (such as carbapenemase) are being increasingly identified in isolates from different origins (Bai et al., 2016; Haenni et al., 2016). In addition, one of the major health concerns associated with E. coli is the role of certain clonal groups in the emergence and dissemination of antimicrobial resistance, namely those belonging to sequence type (ST) 10, ST69, ST131, ST405, ST410, or ST648 (Hansen et al., 2014; Falgenhauer et al., 2016; Johnson et al., 2017). Of particular importance is the ST131 due to its prevalence in community- and hospital-acquired urinary tract infections (UTIs) (Nicolas-Chanoine et al., 2014; Kallonen et al., 2017), and whose potential contribution to the global dissemination of antimicrobial resistances has been also highlighted in food-producing animals (Mora et al., 2010).
In the present study, we characterized a collection of E. coli isolates obtained in Spain during the period 2006–2016 from pigs suffering enteric colibacillosis with three main aims: (i) to define clonal groups of clinical importance in swine enteric colibacillosis; (ii) to analyze the rates of antibiotic resistance in pig farming in Spain, including mobile resistance to colistin (mcr gene); and (iii) to gain knowledge about the presence and zoonotic potential of ST131 isolates of livestock origin.
Materials and Methods
E. coli Collection
A total of 464 rectal samples from pigs suffering diarrhea were tested for routine diagnosis of enteric colibacillosis at the Reference Laboratory of Escherichia coli (LREC), in Lugo, Spain. The samples were collected in farms from different Spanish regions between 2006 and 2016, mainly of diarrheas after weaning (73%) and the remaining (27%) from suckling piglets.
Swabs were plated on lactose MacConkey agar (LMAC) and sorbitol MacConkey agar (Oxoid) supplemented with cefixime (0.05 mg/l) and potassium tellurite (2.5 mg/l) (CTSMAC), and incubated at 37°C for 18–24 h. Afterward, the confluent growth of all plates were tested to detect the presence of ETEC, STEC, and EPEC by PCR based on specific genes encoding toxins (LT, STa, STb, Stx1, Stx2, Stx2e, and HlyA), fimbriae (F4, F5, F6, F18, and F41), intimin (Eae), and bundle-forming pilus (BFP) (Blanco et al., 2006; Vu Khac et al., 2006; Vu Khac et al., 2007; Supplementary Table S1). Confluents were also screened for rfbO25 using specific primers described by Clermont et al. (2008) to presumptively detect the ST131 clonal group. For each PCR-positive culture, five E. coli-like colonies from LMAC and/or CTSMAC plates were plated on tryptone soy agar (Oxoid) and individually analyzed by PCR. Those colonies showing different genetic characteristics for the selected targets were stored at room temperature in nutrient broth (DifcoTM) with 0.75% nutrient agar (DifcoTM) for further characterization. In total, 499 E. coli isolates were examined in this study representing 179 diarrheagenic outbreaks.
O and H Typing
Determination of O:H antigens was carried out following the method described by Guinée et al. (1981) with O1 to O185 and H1 to H56 antisera, respectively. Isolates that did not react with any O antisera were classified as non-typeable (ONT), and non-motile isolates (HNM) were further analyzed by PCR to determine their flagellar genes (Mora et al., 2018; Supplementary Table S2).
Detection of mcr-1 and Other mcr Genes
The 499 E. coli isolates were investigated for the presence of mcr-1 gene by PCR as detailed elsewhere (Liu et al., 2016). From the positive mcr-1 isolates, a representative group displaying different serotypes/pathotypes was further characterized as described below, including the screening of mcr-2, 3, 4, and 5 using primers and conditions of previous studies (Xavier et al., 2016; Borowiak et al., 2017; Carattoli et al., 2017; Yin et al., 2017; Supplementary Table S3).
Antimicrobial Susceptibility and Genotypic Characterization of β-Lactamases
Antimicrobial susceptibility was determined by minimal inhibitory concentrations (MICs) using the MicroScan WalkAway®-automated system (Siemens Healthcare Diagnostics, CA, United States) according to the manufacturer’s instructions. The antibiotics tested included ticarcillin, aztreonam, ceftazidime, cefepime, ampicillin-sulbactam, piperacillin-tazobactam, imipenem, meropenem, amikacin, gentamicin, tobramycin, levofloxacin, ciprofloxacin, trimethoprim-sulfamethoxazole, fosfomycin, colistin, minocycline, and tigecycline. Additionally, resistance to ampicillin, cefotaxime, chloramphenicol, and nalidixic acid was determined by disk (Becton Dickinson, Sparks, MD, United States) diffusion assays. All results were interpreted according to the CLSI (CLSI, 2017). Genetic identification of the ESBLs was performed by PCR using the TEM, SHV, CTX-M-1, and CTX-M-9 group-specific primers followed by amplicon sequencing (Mora et al., 2013; Supplementary Table S3).
Phylogroups, Clonotypes, and Sequence Types (STs)
The phylogenetic relatedness of the isolates was analyzed on the basis of their phylogroups, clonotypes, and STs. The assignment to the main E. coli phylogenetic groups (A, B1, B2, C, D, E, and F) was performed using the quadruplex phylogroup assignment method described by Clermont et al. (2013) based on the presence/absence of the four genetic targets arpA, chuA, yjaA, and TspE4.C2 (Supplementary Table S4). MLST was performed following the Achtman seven-locus scheme. Briefly, internal fragments of seven housekeeping genes (adk, fumC, gyrB, icd, mdh, purA, and recA) were amplified and sequenced using published criteria and primers (Wirth et al., 2006; Supplementary Table S4). The allelic profile for each isolate was determined through the Enterobase website.1 The clonotyping was based on the internal 469-nucleotide (nt) and 489-nt sequence of the fumC and fimH genes, respectively (Weissman et al., 2012; Supplementary Table S4). Allele assignments for fimH were determined using the fimtyper database available at the Center for Genomic Epidemiology website.2 The combination of fumC (allele obtained from MLST) and fimH allele designations was used as the CH “type.” Finally, a neighbor-joining tree was constructed by MEGA6 (Tamura et al., 2013) with the concatenated sequences of the seven housekeeping genes to confirm the consistency of the phylogroup assignations.
Characterization of ST131 Isolates
Isolates confirmed as ST131 by MLST were additionally investigated for extraintestinal virulence markers. Based on the results, the isolates were considered to conform the extraintestinal pathogenic E. coli (ExPEC) status if positive for two or more of five markers, including papAH and/or papC, sfa/focDE, afa/draBC, kpsM II, and iutA (Johnson et al., 2003), and the uropathogenic (UPEC) status if positive for three or more of four markers, including chuA, fyuA, vat, and yfcV (Spurbeck et al., 2012; Supplementary Table S1). The virotype of the ST131 isolates was established according to the scheme described by Dahbi et al. (2014) based on the presence or absence of certain extraintestinal virulence genes (afa/draBC, afa operon FM955459, iroN, sat, ibeA, papG II, papG III, cnf1, hlyA, cdtB, neuC-K, kpsM II-K2, and kpsM II-K5).
Pulsed Field Gel Electrophoresis (PFGE)
The similarity within the E. coli clonal group ST131 was established comparing the XbaI-PFGE profiles of the isolates which were obtained following the PulseNet protocol,3 and imported into BioNumerics (Applied Maths, St-Martens-Latern Belgium) to perform a dendrogram with the UPGMA algorithm based on the Dice similarity coefficient and applying 1% of tolerance in the band position.
Statistical Analysis
Differences were compared by a two-tailed Fisher’s exact test. P values <0.05 were considered statistically significant.
Results
Diarrheagenic Pathotypes and Serogroups
The 499 E. coli isolates analyzed in this work had been obtained from 464 fecal samples of 179 diarrheagenic outbreaks occurred in different geographic areas of Spain. By PCR, 481 of those 499 isolates shown diarrheagenic pathotypes, while 18 isolates recovered by means of the rfbO25 screening and negative for the enteric virulence genes were later investigated concerning the ST131 clonal group.
Specifically, the major pathotypes found among the 481 diarrheagenic isolates were ETEC (277 isolates; 57.6%) positive for genes encoding enterotoxins (eltA, and/or estA, and/or estB) and aEPEC (156 isolates; 32.4%) positive for eae but negative for bfpA (therefore classified as atypical EPEC, aEPEC). The remaining isolates were assigned as STEC/ETEC (33 isolates; 6.9%) positive for both Stx2e and enterotoxin-encoding genes (stx2e and estB and/or estA) and STEC (15 isolates; 3.1%) positive for stx2e. According to the age of the affected animals, aEPEC was the most prevalent pathotype in suckling piglets (60.3%) followed by ETEC (38.8%). By contrast, ETEC isolates were the most prevalent in PWD (67.0%) followed by aEPEC (21.7%) (P < 0.001 for both comparisons). Furthermore, STEC/ETEC were recovered in 9.0% of PWD versus 0.9% of neonatal diarrheas (P < 0.005); and STEC only in piglets after weaning (2.3%). The colonization factors identified among ETEC isolated from neonatal diarrhea were F4, F5 + F41, and F6 (40.5, 16.7, and 11.9%, respectively); while for PWD no isolates were positive for F5, F6, or F41; however F4 was detected at a level not significantly different to neonatal diarrhea isolates (27.7% of PWD ETEC isolates; P > 0.05). Finally, F18 was only detected in PWD (51.5%) including 45.5% of ETEC, 82.1% of STEC/ETEC, and 80.0% of STEC isolates.
Among the 481 diarrheagenic isolates, a total of 50 different serogroups were distinguished by serotyping. Also, 114 (23.7%) isolates that did not react with any of the O1 to O185 antisera were classified as non-typeable (ONT). In spite of this variability, we found a significant association between pathotypes and certain serogroups. Thus, O108, O138, O141, O149, and O157 accounted for 75.1% of ETEC; O26, O49, O80, and O111 for 39.1% of aEPEC; O138 and O141 for 57.6% of STEC/ETEC; and O139 accounted for 46.7% of STEC (Table 1).
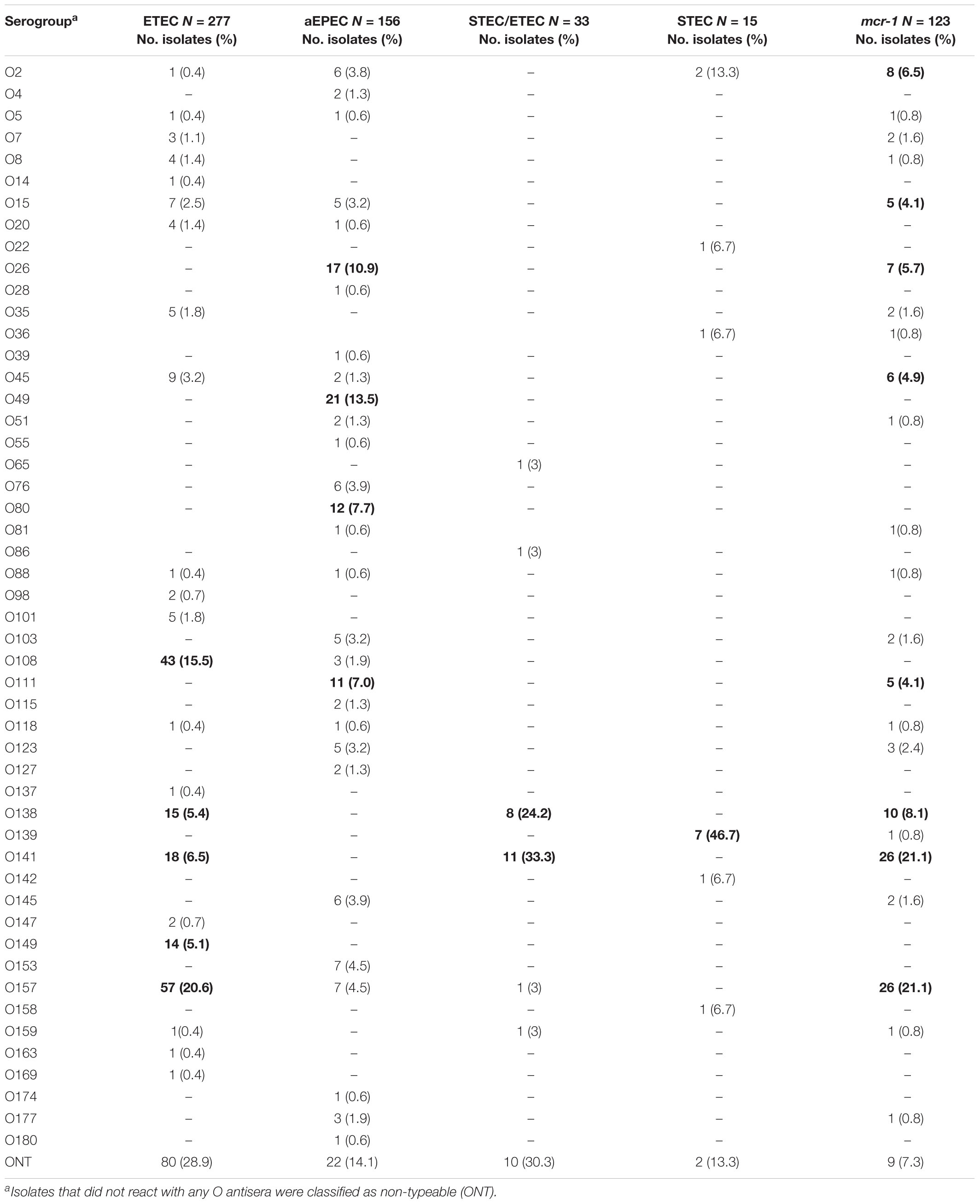
TABLE 1. Association between serogroups, pathotypes, and presence of mcr-1 gene among the 481 E. coli isolates involved in swine colibacillosis (Spain, 2006–2016).
Prevalence of mcr-1 Among Diarrheagenic Isolates
A total of 123 (25.6%) out of 481 diarreagenic isolates harbored the mcr-1 gene, without significant differences in relation to their pathotyes (71 ETEC, 36 aEPEC, 10 STEC/ETEC, and 6 STEC isolates) (P > 0.05 for all comparisons); nevertheless, there was a significant association with the age of the affected animals (33.5% of PWD isolates versus 8.7% of neonatal diarrhea) (P < 0.001). The 123 isolates showed 23 serogroups, although 75.6% belonged to only 8 (O2, O15, O26, O45, O111, O138, O141, and O157) (Table 1).
Serotypes, Antimicrobial Resistances, ESBL Types, and mcr Genes
From the 123 mcr-1 E. coli involved in swine colibacillosis, a representative group of 65 isolates was selected for further characterization. The selection included approximately 50% of each pathotype (33 out of 71 ETEC, 24 out of 36 aEPEC, 5 out of 10 STEC/ETEC, and 3 out of 6 STEC), as well as the serogroups representing 75.6% of the mcr-1 isolates (O2, O15, O26, O45, O111, O138, O141, and O157) together with other less prevalent (O7, O8, O35, O51, O103, O118, O123, O139, O145, and O177).
By serotyping, the 33 ETEC mcr-1 isolates showed 11 different O:H combinations being O157:HNM, O141:H4, and O138:H14 the most prevalent (12, seven, and three isolates, respectively); the five STEC/ETEC isolates were O141:H4; and the three STEC showed three different serotypes (O2:HNM, O139:H1, and O141:HNM) (Table 2). Finally, the 24 aEPEC mcr-1 isolates belonged to 12 serotypes, with O26:H11, O2:H40, and O123:H11 as the most prevalent (five, four, and three isolates, respectively) (Table 3).
The antimicrobial susceptibility testing of the 65 mcr-1 isolates confirmed that all were colistin resistant (MIC of ≥ 4 mg/L). High rates of resistance were also found against ampicillin (75.4%), ticarcillin (73.8%), trimethoprim-sulfamethoxazole (72.3%), ampicillin-sulbactam (64.6%), nalidixic acid (60.0%), and chloramphenicol (58.5%) (Table 4). It is important to note that three isolates were fosfomycin-resistant and, in addition, other eight isolates showed MIC values of 64 mg/L, which is considered as resistant according to EUCAST criteria (MIC breakpoint of 32 mg/L) (EUCAST, 2017). Furthermore, most of the mcr-1 isolates (61 of 65) met the definition of MDR (Magiorakos et al., 2012) being resistant to at least one agent of ≥3 different antimicrobial categories (Tables 2, 3).
Extended-spectrum beta-lactamase genes were detected in six ETEC and one STEC of the 65 mcr-1 isolates which were typed by sequencing as CTX-M-14 and SHV-12 (six and one isolates, respectively). The PCR screening of other mcr variants among the 65 mcr-1 positive isolates determined that three ETEC isolates carried both mcr-1/mcr-4 genes and other four isolates (two ETEC and two aEPEC) were simultaneous carriers of mcr-1/mcr-5 (Tables 2, 3).
Phylogroups, STs, Clonotypes, and Virulence-Gene Typing
The 65 mcr-1 diarrheagenic isolates belonged to phylogroups A (46 isolates), B1 (11 isolates), and E (eight isolates), with 18 different STs including a new one (Figure 1 and Supplementary Table S5). However, more than 50% of these isolates (23 ETEC, eight aEPEC, five STEC/ETEC, and two STEC) were A-ST10 Cplx. The new ST showed by an aEPEC isolate was a single locus variant (SLV) of ST301 and 2 SLV in relation to ST165, so they would be included in the same ST165 Cplx.
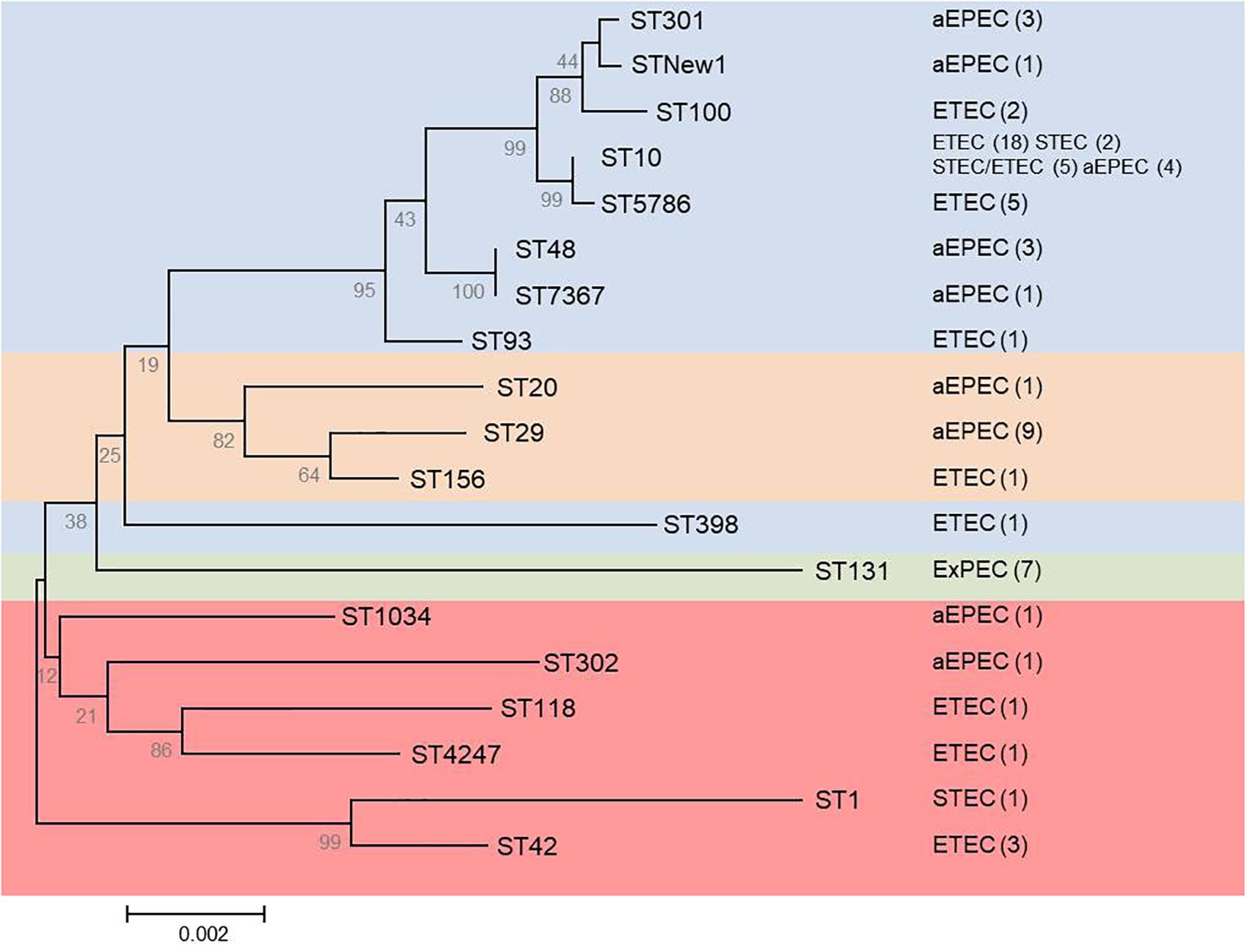
FIGURE 1. Phylogenetic tree based on concatenated sequences of the seven housekeeping genes from the MLST Achtman scheme by the neighbor-joining method using MEGA6. The analysis includes the 19 STs determined for the mcr-1 (65 diarrheagenic and seven ST131-B2) positive isolates. Numbers on the tree indicate bootstrap values calculated for 1,000 replicates. Colors indicate: blue (phylogroup A), orange (phylogroup B1), green (phylogroup B2), and red (phylogroup E). Pathotype and number of isolates (in parentheses) are shown on the right.
Clonotyping identified 19 fumC-fimH allele combinations (CH), being CH11-24 the most prevalent (29 isolates, 44.6%) which was here associated to STs 10 and 5,796 of the ST10 Cplx, and to different pathotypes (19 ETEC, five STEC/ETEC, four aEPEC, and one STEC). Besides, three of the 65 mcr-1 isolates were fimH negative (Supplementary Table S5).
The F18 and K88 colonizing factors detected in 18 and 12 of the 65 mcr-1 isolates, respectively, were typed by PCR sequencing. Specifically, the variant K88ac was identified by PCR in the 12 positive isolates, while F18ac and F18ab were determined by sequencing in 17 and one isolate, respectively (Table 2, Supplementary Table S6, and Supplementary Figure S1). The intimin type of the 24 eae-positive isolates was also established by sequencing: eae-β1 in 11 isolates, eae-ξ in four isolates, eae-𝜀1 in four isolates, eae-γ1 in one isolate, eae-γ2 in three isolates, and eae-𝜃2 in one isolate (Table 3). All 65 mcr-1 diarrheagenic isolates were additionally analyzed for the HlyA encoding gene which was detected in 23 ETEC, five STEC/ETEC, two STEC, and three aEPEC (Tables 2, 3).
Characterization of ST131 Isolates and Detection of mcr-1 Gene
Eighteen isolates recovered by means of the rfbO25 screening were confirmed by serotyping, phylogroup and MLST as O25b:H4-B2-ST131. According to their virulence profile, the 18 ST131 isolates belonged to the virotype D, being the majority D5 (13 isolates), and the remaining showed virotypes D2 (two isolates) and D-non typeable (D-nt, three isolates). Importantly, all exhibited the ExPEC and UPEC status. Clonotyping showed eight different clonotypes: CH40-22 (nine isolates), CH40-161 (two), CH40-326 (one), CH40-330 (one), CH40-332 (one), CH40-336 (one), CH40-338 (one), and CH40-374 (two); being five of them (fimH161, fimH326, fimH330, fimH332, and fimH338) single locus variants of fimH22 and two of them (fimH336 and fimH374) two loci variants of fimH22 (Table 5, Supplementary Tables S7, S8, and Supplementary Figures S2, S3). The genetic relatedness and diversity of the ST131 isolates were additionally analyzed by XbaI macrorestriction followed by PFGE and compared with human clinical ST131 of our collection (Figure 2). Globally, the pig macrorestriction profiles showed high heterogeneity in the dendrogram where grouped in relation to their virotype with three small cluster identified among D5 clinical isolates (≥85% similarity). Importantly, we detected two human ST131 D5 clinical isolates of high identity (≥85% similarity) with two pig clusters, respectively.
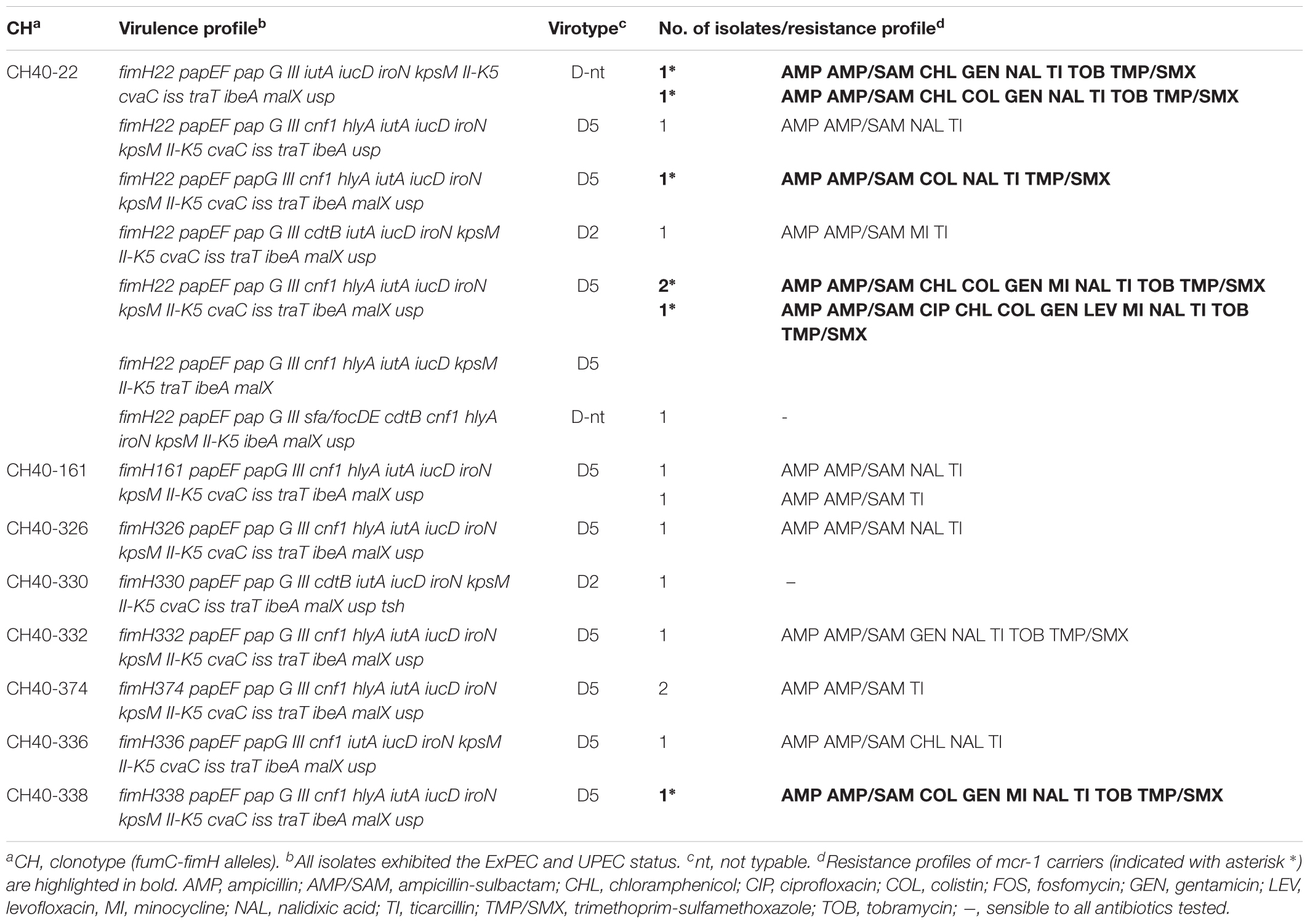
TABLE 5. Phenotypic and genotypic characterization of the 18 O25b:H4-B2-ST131 isolates recovered from the 464 fecal samples of pigs with diarrhea.
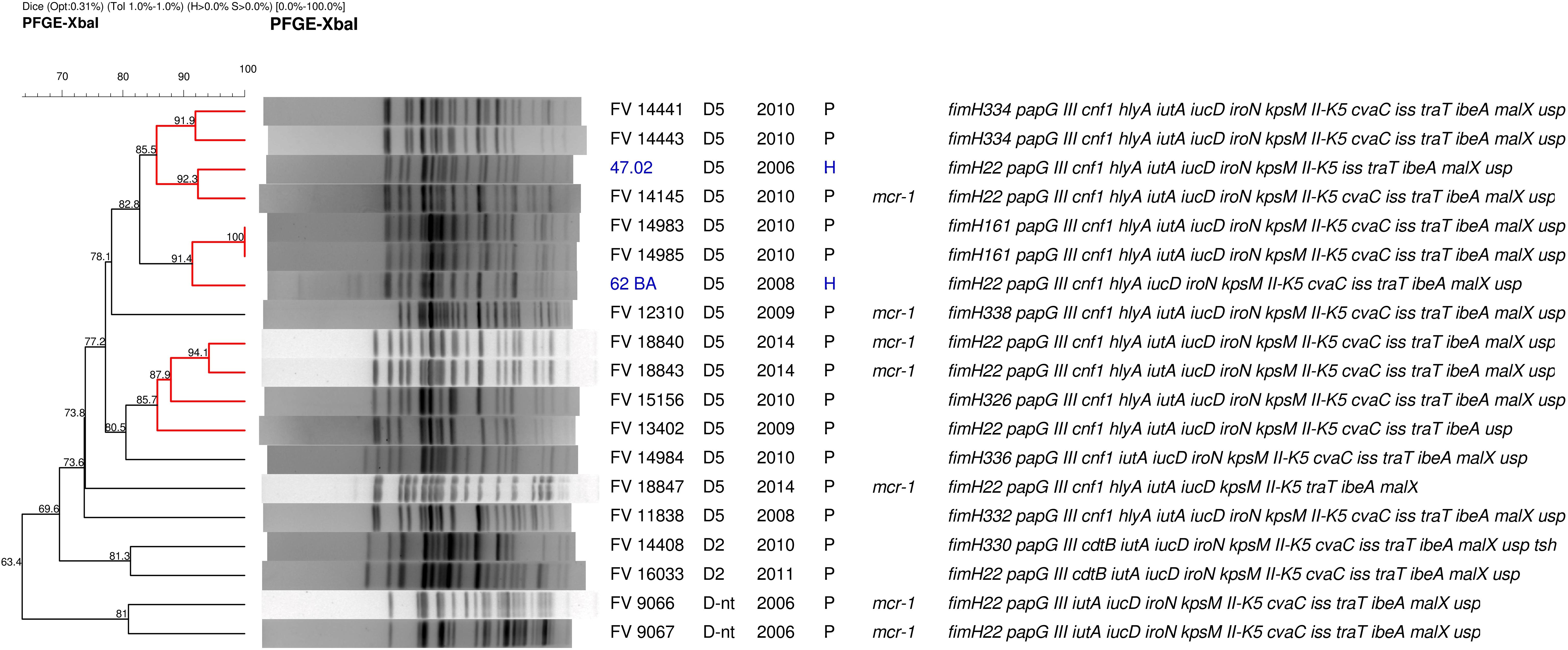
FIGURE 2. PFGE macrorrestriction profile of 17 pig E. coli isolates belonging to the clonal group O25:H4-B2-ST131 (one ST131 isolate resulted autodigested) compared with two human clinical isolates of the LREC collection (showed in blue): association between isolation code, virotype, year, and origin of isolation (P, pig; H, human), mcr-1 presence, and virulence profile is indicated on the right. Highlighted in red clusters of similarity >85%.
Seven CH40-22 isolates out of the 18 ST131 were mcr-1 carriers. Their antimicrobial susceptibility determined by MIC confirmed that all but one were colistin resistant. Furthermore, the seven showed MDR (Table 5).
Discussion
Pig diarrhea caused by E. coli is a worldwide economically important disease for the swine industry. While ETEC neonatal diarrhea can be effectively controlled by vaccination of pregnant sows, passive protection is quickly lost after weaning (Melkebeek et al., 2013; Matías et al., 2017). Currently, few vaccines are commercially available for PWD, and few provide protection against different E. coli pathotypes (Nadeau et al., 2017; Won and John Hwa, 2017). In the present study, we performed a wide epidemiological study on a collection of E. coli isolates recovered from 464 faecal samples of enteric colibacillosis in pig production farms of Spain over a 10-year period to know the E. coli traits (pathotypes) presently implicated in swine diarrheas, and how the use of antibiotics (including colistin) could have affected the selection and emergence of resistant strains. Most samples (73%) were of diarrheagenic cases occurred during the post-wean period which showed to be significantly associated with ETEC (67%) followed by aEPEC (21.7%). By contrast, aEPEC was more prevalent (60.3%) among diarrheas of suckling piglets, followed by ETEC (38.8%). STEC/ETEC or STEC were recovered in 11.3 and 0.9% of PW and neonatal diarrhea, respectively. It is noteworthy that the F4 colonization factor was identified among neonatal ETEC without significant differences compared to PWD ETEC (40.5 versus 27.7%) while, as expected, F18 was only present among PWD isolates.
Complete data on the prevalence, serotypes, and pathotypes are not frequently available, which makes comparisons difficult. However, previous analysis had already suggested differences between countries (Frydendahl, 2002; Blanco et al., 2006; Vu Khac et al., 2006). A recent study across Europe regarding ETEC and STEC PWD pathotypes describes a higher prevalence of F4 compared to F18 isolates in Belgium and The Netherlands, France, and Italy (Luppi et al., 2016). Although pairwise comparisons for each country gave no significant differences, the epidemiologic situation of PWD is clearly different from that found in our study for Spain (23.9% F4 isolates versus 51.5% F18 of ETEC, STEC, and STEC/ETEC isolates; P < 0.001). The reported association of F18 E. coli isolates with PWD in other countries varied widely, from 1.7% in Australia (Smith et al., 2010), 35% in Slovakia (Vu Khac et al., 2006), 39.3% in Denmark (Frydendahl, 2002), 53% in United States (Post et al., 2000), to 62% in Poland (Osek et al., 1999). High F18 prevalence (62.9% of 648 isolates) was as well reported in a study performed in Japan where, in addition, most isolates carried stx2e gene (60.1% of 648 isolates) (Kusumoto et al., 2016) which describes a very different pathogenic profile in relation to other geographic areas. Thus, we found 10% of stx2e-positive isolates among the Spanish collection of 481 E. coli, similar to the numbers reported in the European study of Luppi et al. (2016). Like the Japanese study, most of our stx2e isolates (73.9%) were positive for F18.
The majority of ETEC associated with diarrhea in pigs appear to belong to a limited number of serogroups, being O8, O138, O139, O141, O147, O149, and O157 the most commonly reported worldwide. Similarly, STEC causing disease primarily belong to O138, O139, and O141 (Nagy and Fekete, 1999; Frydendahl, 2002; Fairbrother et al., 2005). The majority of ETEC and STEC isolates analyzed in this work belonged to the most common serogroups O8, O138, O139, O141, O147, O149, and O157. In addition, O15, O35, O45, O101, and O108 serogroups, less frequently reported, were also present within the ETEC isolates ranging between 15.5% for O108 and 1.8% for O35; likewise, STEC single isolates presented the infrequent O2, O22, O36, O142, and O158 serogroups. Apart from the 50 serogroups determined within 337 isolates, it is of note that other 144 (23.7%) isolates of different pathotypes did not react with any of the O1 to O185 antisera and remained as non-typeable (ONT).
We found in our study a high prevalence of aEPEC (156 out of 481; 32.4%) as presumptive agents of the clinical condition, and significantly associated with neonatal samples. For atypical EPEC, both animals and humans can be reservoirs and are known as pathogenic for children and young animals (Trabulsi et al., 2002; Watson et al., 2017). aEPEC have also been implicated in PWD in pigs but their pathogenicity still remains unclear (Malik et al., 2017). According to these and other authors, there exist differences of serotypes and intimin types among porcine eae-positive isolates in relation to the pathogenic potential, such as sero/intimin type O123:H11/eae-β1 or O45/eae-β1 (both determined in our collection) with AE activity on ileal villi and frequently occurring in diarrheagenic pigs (Zhu et al., 1994; Malik et al., 2017). In previous studies on swine diarrhea in Slovakia, we had found a much lower aEPEC involvement (3.2 and 0.9% of neonatal and PWD isolates, respectively) (Vu Khac et al., 2006, Vu Khac et al., 2007).
Since the discovery of the mcr-1 gene in late 2015, its detection has been reported globally, but its detection rate has been variable depending on the geographic region, source, and method of identification. Overuse of colistin in food animals is believed to have trigged the emergence and spread of mcr-1, consistently with the higher figures found in poultry and pig industry (Sun et al., 2018). Here, the characterization of 65 representative mcr-1 isolates, including 33 ETEC, 24 aEPEC, five STEC/ETEC, and three STEC showed that all were phenotypically resistant to colistin, and most (61 of 65) met the MDR definition of Magiorakos et al. (2012). MDR in pig industry has been previously reported in different studies, and associated with the widely use of aminoglycosides and beta-lactams in veterinary medicine (Rhouma et al., 2016). The 37 mcr-1 positive isolates recovered from PWD in Italy also showed genetically diverse pathotypes and all but one were resistant to ≥3 different antimicrobial families (Curcio et al., 2017). Li et al. (2017) found a higher prevalence of mcr-1 positie isolates among pathogenic E. coli from diseased pigs than from healthy pigs (45.1 versus 15.7%, P = 0.000); besides, resistance profiles of mcr-1 positive E. coli were more extensive than those of mcr-1 negative isolates. In our study, it is also of concern the finding of 11 out of the 65 mcr-1 isolates with MIC >32 mg/L for fosfomycin. Fosfomycin resistance is uncommon and primarily associated with specific chromosomal mutations, however plasmid-mediated resistance in livestock has been detected in Asian countries (Chan et al., 2014), and quite recently in France (Lupo et al., 2018). This antibiotic is considered an “old” broad-spectrum antibiotic such as colistin, reconsidered as a good candidate for treating infections caused by MDR microorganisms (Dijkmans et al., 2017).
Six ETEC and one STEC mcr-1 isolates were also carriers of ESBL genes. In addition, other seven mcr-1 isolates showed to harbor mcr-4 (three ETEC) and mcr-5 (two ETEC and two aEPEC) genes. We have not investigated if the double carriage of our isolates was in the same or different plasmids. Previous studies have reported the co-existence on the same plasmid of other resistance genes, such as blaCTX-M, blaCMY, blaTEM, fosA, qnrS, floR, and oqxAB, in various combinations, alongside mcr-1 (Li R. et al., 2017). At the same time, new mcr genes have been successively discovered in plasmids of different origins, as well as co-transfer of variants in promiscuous plasmids (Sun et al., 2018). Since colistin is commonly thought as the last line antibiotic for the treatment of infections caused by MDR and extensively drug-resistant Gram-negative pathogens, such as carbapenem-resistant Enterobacteriaceae, it must be beared in mind that its use could lead to co-selection of colistin with other antibiotic-resistant isolates.
Of the 41 ETEC, STEC and STEC/ETEC mcr-1 isolates (Table 2), 18 were carriers of F18. Three F18 subtypes have been reported so far (F18ab, F18ac, and F18New) differentiated by five amino acids at specific positions (31, 57, 59, 83, and 122) with strong association between subtypes and pathotypes (Bosworth et al., 1998; DebRoy et al., 2009; Barth et al., 2011; Byun et al., 2013). Here, the F18 positive isolates subtyped as F18ac corresponded with the ETEC and STEC/ETEC pathotypes (15 and two isolates, respectively) while F18ab was detected in a single STEC isolate. Furthermore, the predicted amino acid sequences of the fedA genes identified in this study together with 13 sequences from Barth et al. (2011) were compared and their relatedness analyzed, demonstrating its polymorphic variability (Bosworth et al., 1998).Thus, 10 different amino acid sequences were found among the F18ac subtype of our collection, one of them (detected in four isolates) showed 100% similarity with the GQ325624 sequence identified by Barth et al. (2011) in Germany (Supplementary Figure S1). Interestingly, the deduced amino acid sequence of one isolate (FV18854) presented a novel glycine residue in position 59; however, it was classified as F18ac since the other four amino acids positions are equal to those described for this subtype (Supplementary Table S6) and clustered together with F18ac group in the phylogenetic tree (Supplementary Figure S1).
We found high diversity in the phylogenetic analysis of the 65 mcr-1 diarrheagenic isolates; however, more than 50% (38 out of 65) belonged to A-ST10 Cplx and from those, 29 isolates of different pathotypes showed CH11-24. The ST10 Cplx of E. coli, widely disseminated among animal and human intestinal samples both as a commensal or as a pathogen, is commonly encountered as antimicrobial susceptible but also linked with MDR and ESBL, and recognized as an emerging food-borne ExPEC lineage (Manges and Johnson, 2012). A recent study on genotyping of 68 mcr-1-like-positive E. coli from food animals at slaughter in Europe between 2002 and 2014, found a high genetic diversity among the isolates with 38 different STs, but being ST10 Cplx and, specifically the ST10, the most prevalent (El Garch et al., 2017, 2018). This finding is also consistent with the observation of Matamoros et al. (2017) who stated that within the overall diversity in the E. coli population, two lineages (ST10 and ST155) might function as reservoirs of the mcr-1 gene, the largest of which was linked to ST10 (Matamoros et al., 2017). In our particular case, it is also important to consider that ST10 Cplx is one of the main E. coli clonal complexes associated with porcine ETEC. In fact, Shepard et al. (2012) found that the majority of the porcine ETEC isolates belonged to three clonal complexes: 10, 23, and 165, and pointed out the association showed by ST10 Cplx and ST23 Cplx with certain resistance-associated elements, such as AmpC-type beta-lactamases, NDM-type carbapenemases, and other ESBLs. Other STs established in the present study such as ST1, ST29, ST42, ST100, or ST4247 have been previously associated to enteric pathogenic E. coli in pigs (Abraham et al., 2014; Kusumoto et al., 2016).
Interestingly, nine of the 24 aEPEC mcr-1 characterized in this work belonged to B1-ST29 and carried eae-β1 gene. They showed six serogroups, including O26, with two H-antigen combinations (H9 or H11). Additionally, two aEPEC belonged to the clonal groups O103:H2-B1-ST20 (eae-β1) and O145:H28-E-ST1034 of the ST32 Cplx (eae-γ1), respectively. Enterohemorrhagic E. coli (EHEC) is the causative agent of bloody diarrhea, the hemolytic-uremic syndrome (HUS), and thrombotic thrombocytopenic purpura (TTP). Besides the intimin (Eae) which confers the ability to cause AE lesions, EHEC harbors bacteriophage-encoded stx genes. Currently, the vast majority of EHEC infections are caused by isolates belonging to five O serogroups, namely O157, O26, O103, O111, and O145 (Mellmann et al., 2008). Eichhorn et al. (2015) analyzed by MLST the phylogenetic relationships in a collection of 250 isolates belonging to serogroups O26, O103, O111, and O145 defined as EHEC, obtained from different sources of isolation. As a result, the majority of the O26 and O111 EHEC isolates clustered into the ST29 Cplx. O103 isolates clustered mainly in ST20 Cplx, and most isolates of O145 were found within ST32 Cplx. In addition to EHEC, the ST29 Cplx cluster also included aEPEC isolates. According to these authors, the finding that aEPEC and EHEC isolates of non-O157 serogroups share the same phylogeny suggests an ongoing microevolutionary scenario in which the phage-encoded stx is transferred between aEPEC and EHEC. The concept of interconversion between STEC and aEPEC had been previously suggested by Bielaszewska et al. (2007) for O26 isolates by the loss, as well as by the gain, of the stx-encoding prophage through the lysogenic conversion. Applying the concept of bidirectional conversion, it could be hypothesized that the EPEC strains could function as pre-STEC strains that integrate the stx prophage into their genomes. However, this hypothesis together with the potential human pathogenicity of EPEC isolates from sources such as swine requires extensive research.
A highly important finding of the present study was the recovery of 18 ST131 isolates among the 464 fecal samples of diarrheanic pigs. As far as we know, there are only two reports of pig origin for this clonal group: the first obtained from pork meat in Denmark in 2003 (Trobos et al., 2009), and the second was a CTX-M-1 ST131 recovered from a gastrointestinal tract infection in a pig among 1,378 isolates analyzed in the frame of an ESBL monitoring program performed in Germany during the years 2006–2007 (Schink et al., 2013). None of the 18 ST131 detected in this work were carriers of ESBL genes but instead, most were MDR, harbored 12–15 virulence-gene traits and, surprisingly, seven isolates were mcr-1 carriers. Very few mcr-1 isolates have been reported belonging to the pandemic clone ST131, responsible for the high incidence of ExPEC infections as well as the worldwide dissemination of multidrug resistance (Nicolas-Chanoine et al., 2008, 2014). So far, ST131 carrying mcr-1 was first described in an isolate from chicken meat (Hasman et al., 2015), then in poultry (Ewers et al., 2016) and in a few human clinical isolates (Kuo et al., 2016; Sonnevend et al., 2016; Ortiz de la Tabla et al., 2017). To the best of our knowledge, this would be the first report of ST131 mcr-1 isolation in pigs. The antimicrobial susceptibility of the seven mcr-1 ST131 swine isolates of this work determined by MIC, confirmed that all but one isolate were colistin resistant. The presence of the mcr-1 gene in susceptible isolates has been described (Fernandes et al., 2016; El Garch et al., 2017). Here, we investigated the mcr-1 gene from the susceptible isolate with primers listed in Supplementary Table S3, and failed when trying to obtain the entire gene sequence, suggesting the gene is truncated or has been modified by agents such as insertion sequences like those previously reported (Terveer et al., 2017; Zhou et al., 2018); however, further work is necessary to determine the cause of loss of function. Besides, the seven mcr-1 ST131 swine isolates showed resistance against 6–12 antibiotics, including a ciprofloxacin-resistance determined in 1 isolate (Table 5). In a nationwide study performed in Spain during 2005–2012 in five hospitals of different regions, the ST131 accounted for 490 (16%) of the 2,995 isolates obtained from clinical human samples (Dahbi et al., 2014). The majority (78%) of the ST131 isolates belonged to the recently emerged fluoroquinolone-resistant ST131-H30R subclone and 61.6% to the H30-Rx additionally carrier of CTX-M15, while ST131-H22 subclone was the second most prevalent of the human collection. Dahbi et al. (2014) found in their work different patterns of associated antimicrobial resistances in relation to the fimH allele (fimH30 or fimH22). Ciprofloxacin, trimethoprim-sulfamethoxazole, gentamicin, and tobramycin resistances were significantly associated with fimH30 isolates in comparison with fimH22 isolates. In fact, they only detected one and eight isolates resistant to ciprofloxacin and trimethoprim-sulfamethoxazole, respectively, among 20 ST131-H22. Dahbi et al. (2014) also found association of the fimH alleles with the virulence profile, being all 20 fimH22 isolates of virotype D, subtypes D1, D2, D4, and D5. In the present work, we found high genetic variability within the eight fimH alleles of 18 ST131 isolates; however, fimH22 was the most prevalent (nine isolates) and the other seven alleles were fimH22 variants whose nucleotide and aminoacidic differences were compared with those reported by Dahbi et al. (2014) (Supplementary Tables S7, S8 and Supplementary Figures S2, S3). All our swine isolates conformed the D virotype and showed D5 (13 isolates) and D2 (two isolates) also described within the human collection. Worryingly, the seven swine ST131 carriers of mcr-1 also showed a higher number of resistances, including to trimethoprim-sulfamethoxazole (seven isolates), tobramycin (six isolates), gentamicin (six isolates), and ciprofloxacin (one isolate), more associated to the fimH30 subclone according to Dahbi et al. (2014). It is also remarkable that the 18 ST131 swine isolates exhibited virulence traits that satisfied the ExPEC and UPEC status according to Johnson et al. (2003) and Spurbeck et al. (2012) definitions. In the PFGE macrorestriction, comparison of our swine isolates with those of clinical human origin, two pig isolates clustered with two human ST131 D5 showing high similarities (≥85%).
Conclusion
In conclusion, the comprehensive characterization of this wide collection of E. coli isolates recovered from enteric swine colibacillosis in Spain provides valuable epidemiological information about the current pathotypes involved in this economically important pathology, as well as alerts about the worrisome presence of MDR clones such as ST131. Acquisition of mcr-1 by this specific clone means an increased risk due to its special feature of congregating virulence and resistance traits, together with its spread capability. Here, we give evidences of the potential human pathogenicity of ST131 D5 isolates of swine origin due to their genetic identity.
Author Contributions
AM and JB conceived and designed the experiments. IG-M, VG, DD-J, SF-S, and MA performed the experiments. AM, JB, VG, IG-M, JB, and MB analyzed and interpreted the data. AM, VG, JB, and IG-M drafted the manuscript. AM, JB, VG, IG-M, JB, MB, MA, DD-J, and SF-S provided critical input and approved the final manuscript.
Funding
This study was supported by projects AGL2016-79343-R from the Agencia Estatal de Investigación (AEI, Spain) and FEDER; PI16/01477 from Plan Estatal de I + D + I 2013–2016, Instituto de Salud Carlos III (ISCIII), Subdirección General de Evaluación y Fomento de la Investigación, and FEDER; CN2012/303 and ED431C 2017/57 from the Consellería de Cultura, Educación e Ordenación Universitaria (Xunta de Galicia), and FEDER.
Conflict of Interest Statement
The authors declare that the research was conducted in the absence of any commercial or financial relationships that could be construed as a potential conflict of interest.
Acknowledgments
IG-M acknowledges the Conselleria de Cultura, Educación e Ordenación Universitaria, Xunta de Galicia for his grant (Ref. ED481A-2015/149) “Axudas de apoio á etapa predoutoral do Plan galego de investigación, innovación e crecemento 2011-2015 (Plan I2C)”. SF-S acknowledges the FPU program for her grant (FPU15/02644) from the Secretaría General de Universidades, Spanish Ministerio de Educación, Cultura y Deporte, Gobierno de España.
Supplementary Material
The Supplementary Material for this article can be found online at: https://www.frontiersin.org/articles/10.3389/fmicb.2018.02659/full#supplementary-material
Footnotes
- ^ http://enterobase.warwick.ac.uk/species/ecoli/allele_st_search
- ^ https://bitbucket.org/genomicepidemiology/fimtyper_db/downloads/
- ^ http://www.pulsenetinternational.org/
References
Abraham, S., Trott, D. J., Jordan, D., Gordon, D. M., Groves, M. D., Fairbrother, J. M., et al. (2014). Phylogenetic and molecular insights into the evolution of multidrug-resistant porcine enterotoxigenic Escherichia coli in Australia. Int. J. Antimicrob. Agents 44, 105–111. doi: 10.1016/j.ijantimicag.2014.04.011
Bai, L., Hurley, D., Li, J., Meng, Q., Wang, J., Fanning, S., et al. (2016). Characterisation of multidrug-resistant Shiga toxin-producing Escherichia coli cultured from pigs in China: co-occurrence of extended-spectrum beta-lactamase- and mcr-1-encoding genes on plasmids. Int. J. Antimicrob. Agents 48, 445–448. doi: 10.1016/j.ijantimicag.2016.06.021
Barth, S., Schwanitz, A., and Bauerfeind, R. (2011). Polymerase chain reaction-based method for the typing of F18 fimbriae and distribution of F18 fimbrial subtypes among porcine Shiga toxin-encoding Escherichia coli in Germany. J. Vet. Diagn. Invest. 23, 454–464. doi: 10.1177/1040638711403417
Bielaszewska, M., Prager, R., Kock, R., Mellmann, A., Zhang, W., Tschape, H., et al. (2007). Shiga toxin gene loss and transfer in vitro and in vivo during enterohemorrhagic Escherichia coli O26 infection in humans. Appl. Environ. Microbiol. 73, 3144–3150. doi: 10.1128/AEM.02937-06
Blanco, M., Lazo, L., Blanco, J. E., Dahbi, G., Mora, A., López, C., et al. (2006). Serotypes, virulence genes, and PFGE patterns of enteropathogenic Escherichia coli isolated from Cuban pigs with diarrhea. Int. Microbiol. 9, 53–60.
Borowiak, M., Fischer, J., Hammerl, J. A., Hendriksen, R. S., Szabo, I., and Malorny, B. (2017). Identification of a novel transposon-associated phosphoethanolamine transferase gene, mcr-5, conferring colistin resistance in d-tartrate fermenting Salmonella enterica subsp. enterica serovar Paratyphi B. J. Antimicrob. Chemother. 72, 3317–3324. doi: 10.1093/jac/dkx327
Bosworth, B. T., Dean-Nystrom, E. A., Casey, T. A., and Neibergs, H. L. (1998). Differentiation of F18ab + from F18ac + Escherichia coli by single-strand conformational polymorphism analysis of the major fimbrial subunit gene (fedA). Clin. Diagn. Lab. Immunol. 5, 299–302.
Brand, P., Gobeli, S., and Perreten, V. (2017). Pathotyping and antibiotic resistance of porcine enterovirulent Escherichia coli strains from Switzerland (2014-2015). Schweiz. Arch. Tierheilkd. 159, 373–380. doi: 10.17236/sat00120
Byun, J. W., Jung, B. Y., Kim, H. Y., Fairbrother, J. M., Lee, M. H., and Lee, W. K. (2013). Real-time PCR for differentiation of F18 variants among enterotoxigenic and Shiga toxin-producing Escherichia coli from piglets with diarrhoea and oedema disease. Vet. J. 198, 538–540. doi: 10.1016/j.tvjl.2013.07.021
Carattoli, A., Villa, L., Feudi, C., Curcio, L., Orsini, S., Luppi, A., et al. (2017). Novel plasmid-mediated colistin resistance mcr-4 gene in Salmonella and Escherichia coli, Italy 2013, Spain and Belgium, 2015 to 2016. Euro. Surveill. 22:30589. doi: 10.2807/1560-7917.ES.2017.22.31.30589
Chan, J., Lo, W. U., Chow, K. H., Lai, E. L., Law, P. Y., and Ho, P. L. (2014). Clonal diversity of Escherichia coli isolates carrying plasmid-mediated fosfomycin resistance gene fosA3 from livestock and other animals. Antimicrob. Agents Chemother. 58, 5638–5639. doi: 10.1128/AAC.02700-14
Clermont, O., Christenson, J. K., Denamur, E., and Gordon, D. M. (2013). The Clermont Escherichia coli phylo-typing method revisited: improvement of specificity and detection of new phylo-groups. Environ. Microbiol. Rep. 5, 58–65. doi: 10.1111/1758-2229.12019
Clermont, O., Lavollay, M., Vimont, S., Deschamps, C., Forestier, C., Branger, C., et al. (2008). The CTX-M-15-producing Escherichia coli diffusing clone belongs to a highly virulent B2 phylogenetic subgroup. J. Antimicrob. Chemother. 61, 1024–1028. doi: 10.1093/jac/dkn084
CLSI (2017). Performance Standards for Antimicrobial Susceptibility Testing, 27th Edn. CLSI supplement M100S. Wayne, PA: Clinical and Laboratory Standards Institute.
Curcio, L., Luppi, A., Bonilauri, P., Gherpelli, Y., Pezzotti, G., Pesciaroli, M., et al. (2017). Detection of the colistin resistance gene mcr-1 in pathogenic Escherichia coli from pigs affected by post-weaning diarrhoea in Italy. J. Glob. Antimicrob. Resist. 10, 80–83. doi: 10.1016/j.jgar.2017.03.014
Dahbi, G., Mora, A., Mamani, R., López, C., Alonso, M. P., Marzoa, J., et al. (2014). Molecular epidemiology and virulence of Escherichia coli O16:H5-ST131: comparison with H30 and H30-Rx subclones of O25b:H4-ST131. Int. J. Med. Microbiol. 304, 1247–1257. doi: 10.1016/j.ijmm.2014.10.002
DebRoy, C., Roberts, E., Scheuchenzuber, W., Kariyawasam, S., and Jayarao, B. M. (2009). Comparison of genotypes of Escherichia coli strains carrying F18ab and F18ac fimbriae from pigs. J. Vet. Diagn. Invest. 21, 359–364. doi: 10.1177/104063870902100310
Dijkmans, A. C., Zacarias, N. V. O., Burggraaf, J., Mouton, J. W., Wilms, E. B., van Nieuwkoop, C., et al. (2017). Fosfomycin: pharmacological, clinical and future perspectives. Antibiotics 6:E24. doi: 10.3390/antibiotics6040024
Eichhorn, I., Heidemanns, K., Semmler, T., Kinnemann, B., Mellmann, A., Harmsen, D., et al. (2015). Highly virulent non-O157 Enterohemorrhagic Escherichia coli (EHEC) serotypes reflect similar phylogenetic lineages, providing new insights into the evolution of EHEC. Appl. Environ. Microbiol. 81, 7041–7047. doi: 10.1128/AEM.01921-15
El Garch, F., de Jong, A., Bertrand, X., Hocquet, D., and Sauget, M. (2018). mcr-1-like detection in commensal Escherichia coli and Salmonella spp. from food-producing animals at slaughter in Europe. Vet. Microbiol. 213, 42–46. doi: 10.1016/j.vetmic.2017.11.014
El Garch, F., Sauget, M., Hocquet, D., LeChaudee, D., Woehrle, F., and Bertrand, X. (2017). mcr-1 is borne by highly diverse Escherichia coli isolates since 2004 in food-producing animals in Europe. Clin. Microbiol. Infect. 23, 51–54. doi: 10.1016/j.cmi.2016.08.033
EUCAST (2017). The European Committee on Antimicrobial Susceptibility Testing. Breakpoint Tables for Interpretation of MICs and Zone Diameters. Version 7.0, 2017. Available at: http://www.eucast.org
Ewers, C., Gottig, S., Bulte, M., Fiedler, S., Tietgen, M., Leidner, et al. (2016). Genome sequence of avian Escherichia coli strain IHIT25637, an extraintestinal pathogenic E. coli strain of ST131 encoding colistin resistance determinant MCR-1. Genome Announc. 4:e00863-16. doi: 10.1128/genomeA.00863-16
Fairbrother, J., and Gyles, C. (2012). “Colibacillosis,” in Diseases of Swine, 10th Edn, eds J. Zimmerman, L. Karriker, A. Ramírez, K. Schwartz, and G. Stevenson (Hoboken, NJ: Wiley-Blackwell), 723–749.
Fairbrother, J. M., Nadeau, E., and Gyles, C. L. (2005). Escherichia coli in postweaning diarrhea in pigs: an update on bacterial types, pathogenesis, and prevention strategies. Anim. Health Res. Rev. 6, 17–39.
Falgenhauer, L., Imirzalioglu, C., Ghosh, H., Gwozdzinski, K., Schmiedel, J., Gentil, K., et al. (2016). Circulation of clonal populations of fluoroquinolone-resistant CTX-M-15-producing Escherichia coli ST410 in humans and animals in Germany. Int. J. Antimicrob. Agents 47, 457–465. doi: 10.1016/j.ijantimicag.2016.03.019
Fernandes, M. R., Moura, Q., Sartori, L., Silva, K. C., Cunha, M. P., Esposito, F., et al. (2016). Silent dissemination of colistin-resistant Escherichia coli in South America could contribute to the global spread of the mcr-1 gene. Euro. Surveill. 21:30214. doi: 10.2807/1560-7917.ES.2016.21.17.30214
Francis, D. (2002). Enterotoxigenic Escherichia coli infection in pigs and its diagnosis. J. Swine. Health Prod. 10, 171–175.
Frankel, G., and Phillips, A. D. (2008). Attaching effacing Escherichia coli and paradigms of Tir-triggered actin polymerization: getting off the pedestal. Cell Microbiol. 10, 549–556. doi: 10.1111/j.1462-5822.2007.01103.x
Frydendahl, K. (2002). Prevalence of serogroups and virulence genes in Escherichia coli associated with postweaning diarrhoea and edema disease in pigs and a comparison of diagnostic approaches. Vet. Microbiol. 85, 169–182.
García, V., García-Meniño, I., Mora, A., Flament-Simon, S. C., Díaz-Jiménez, D., Blanco, J. E., et al. (2018). Co-occurrence of mcr-1, mcr-4 and mcr-5 genes in multidrug-resistant ST10 Enterotoxigenic and Shiga toxin-producing Escherichia coli in Spain (2006-2017). Int. J. Antimicrob. Agents 52, 104–108. doi: 10.1016/j.ijantimicag.2018.03.022
Guinée, P. A. M., Jansen, W. H., and Wasdtröm, T. R. S. (1981). Laboratory Diagnosis in Neonatal Calf and Pigs Diarrhoea: Current Topics in Veterinary and Animal Science. Netherlands: Springer.
Haenni, M., Poirel, L., Kieffer, N., Châtre, P., Saras, E., Métayer, V., et al. (2016). Co-occurrence of extended spectrum beta lactamase and MCR-1 encoding genes on plasmids. Lancet Infect. Dis. 16, 281–282. doi: 10.1016/S1473-3099(16)00007-4
Hammerl, J. A., Borowiak, M., Schmoger, S., Shamoun, D., Grobbel, M., Malorny, B., et al. (2018). mcr-5 and a novel mcr-5.2 variant in Escherichia coli isolates from food and food-producing animals, Germany, 2010 to 2017. J. Antimicrob. Chemother. 73, 1433–1435. doi: 10.1093/jac/dky020
Hansen, F., Olsen, S. S., Heltberg, O., Justesen, U. S., Fuglsang-Damgaard, D., Knudsen, J. D., et al. (2014). Characterization of third-generation cephalosporin-resistant Escherichia coli from bloodstream infections in Denmark. Microb. Drug. Resist. 20, 316–324. doi: 10.1089/mdr.2013.0157
Hasman, H., Hammerum, A. M., Hansen, F., Hendriksen, R. S., Olesen, B., Agersø, Y., et al. (2015). Detection of mcr-1 encoding plasmid-mediated colistin-resistant Escherichia coli isolates from human bloodstream infection and imported chicken meat, Denmark 2015. Euro. Surveill. 20:30085. doi: 10.2807/1560-7917.ES.2015.20.49.30085
Johnson, J. R., Johnston, B. D., and Gordon, D. M. (2017). Rapid and specific detection of the Escherichia coli Sequence Type 648 Complex within phylogroup F. J. Clin. Microbiol. 55, 1116–1121. doi: 10.1128/JCM.01949-16
Johnson, J. R., Murray, A. C., Gajewski, A., Sullivan, M., Snippes, P., Kuskowski, M. A., et al. (2003). Isolation and molecular characterization of nalidixic acid-resistant extraintestinal pathogenic Escherichia coli from retail chicken products. Antimicrob. Agents Chemother. 47, 2161–2168.
Kallonen, T., Brodrick, H. J., Harris, S. R., Corander, J., Brown, N. M., Martin, V., et al. (2017). Systematic longitudinal survey of invasive Escherichia coli in England demonstrates a stable population structure only transiently disturbed by the emergence of ST131. Genome Res. 27, 1437–1449. doi: 10.1101/gr.216606.116
Kuo, S. C., Huang, W. C., Wang, H. Y., Shiau, Y. R., Cheng, M. F., and Lauderdale, T. L. (2016). Colistin resistance gene mcr-1 in Escherichia coli isolates from humans and retail meats. Taiwan. J. Antimicrob. Chemother. 71, 2327–2329. doi: 10.1093/jac/dkw122
Kusumoto, M., Hikoda, Y., Fujii, Y., Murata, M., Miyoshi, H., Ogura, Y., et al. (2016). Emergence of a multidrug-resistant Shiga toxin-producing Enterotoxigenic Escherichia coli lineage in diseased swine in Japan. J. Clin. Microbiol. 54, 1074–1081. doi: 10.1128/JCM.03141-15
Li, R., Xie, M., Zhang, J., Yang, Z., Liu, L., Liu, X., et al. (2017). Genetic characterization of mcr-1-bearing plasmids to depict molecular mechanisms underlying dissemination of the colistin resistance determinant. J. Antimicrob. Chemother. 72, 393–401. doi: 10.1093/jac/dkw411
Li, X. S., Liu, B. G., Dong, P., Li, F. L., Yuan, L., and Hu, G. Z. (2017). The prevalence of mcr-1 and resistance characteristics of Escherichia coli isolates from diseased and healthy pigs. Diagn. Microbiol. Infect. Dis. 91, 63–65. doi: 10.1016/j.diagmicrobio.2017.12.014
Liu, L., Feng, Y., Zhang, X., McNally, A., and Zong, Z. (2017). New variant of mcr-3 in an extensively drug-resistant Escherichia coli clinical isolate carrying mcr-1 and blaNDM-5. Antimicrob. Agents Chemother. 61:e01757-17. doi: 10.1128/AAC.01757-17
Liu, Y. Y., Wang, Y., Walsh, T. R., Yi, L. X., Zhang, R., Spencer, J., et al. (2016). Emergence of plasmid-mediated colistin resistance mechanism MCR-1 in animals and human beings in China: a microbiological and molecular biological study. Lancet Infect. Dis. 16, 161–168. doi: 10.1016/S1473-3099(15)00424-7
Lupo, A., Saras, E., Madec, J. Y., and Haenni, M. (2018). Emergence of blaCTX-M-55 associated with fosA, rmtB and mcr gene variants in Escherichia coli from various animal species in France. J. Antimicrob. Chemother. 73, 867–872. doi: 10.1093/jac/dkx489
Luppi, A. (2017). Swine enteric colibacillosis: diagnosis, therapy and antimicrobial resistance. Porcine Health Manag. 3:16. doi: 10.1186/s40813-017-0063-4
Luppi, A., Gibellini, M., Gin, T., Vangroenweghe, F., Vandenbroucke, V., Bauerfeind, R., et al. (2016). Prevalence of virulence factors in enterotoxigenic Escherichia coli isolated from pigs with post-weaning diarrhoea in Europe. Porcine Health Manag. 2:20. doi: 10.1186/s40813-016-0039-9
Magiorakos, A. P., Srinivasan, A., Carey, R. B., Carmeli, Y., Falagas, M. E., Giske, C. G., et al. (2012). Multidrug-resistant, extensively drug-resistant and pandrug-resistant bacteria: an international expert proposal for interim standard definitions for acquired resistance. Clin. Microbiol. Infect. 18, 268–281. doi: 10.1111/j.1469-0691.2011.03570.x
Malik, A., Nagy, B., Kugler, R., and Szmolka, A. (2017). Pathogenic potential and virulence genotypes of intestinal and faecal isolates of porcine post-weaning enteropathogenic Escherichia coli. Res. Vet. Sci. 115, 102–108. doi: 10.1016/j.rvsc.2017.02.002
Manges, A. R., and Johnson, J. R. (2012). Food-borne origins of Escherichia coli causing extraintestinal infections. Clin. Infect. Dis. 55, 712–719. doi: 10.1093/cid/cis502
Matamoros, S., van Hattem, J. M., Arcilla, M. S., Willemse, N., Melles, D. C., Penders, J., et al. (2017). Global phylogenetic analysis of Escherichia coli and plasmids carrying the mcr-1 gene indicates bacterial diversity but plasmid restriction. Sci. Rep. 7:15364. doi: 10.1038/s41598-017-15539-7
Matías, J., Berzosa, M., Pastor, Y., Irache, J. M., and Gamazo, C. (2017). Maternal vaccination. immunization of sows during pregnancy against ETEC Infections. Vaccines 5:E48. doi: 10.3390/vaccines5040048
Melkebeek, V., Goddeeris, B. M., and Cox, E. (2013). ETEC vaccination in pigs. Vet. Immunol. Immunopathol. 152, 37–42. doi: 10.1016/j.vetimm.2012.09.024
Mellmann, A., Bielaszewska, M., Köck, R., Friedrich, A. W., Fruth, A., Middendorf, B., et al. (2008). Analysis of collection of hemolytic uremic syndrome-associated enterohemorrhagic Escherichia coli. Emerg. Infect. Dis. 14, 1287–1290. doi: 10.3201/eid1408.071082
Mora, A., García-Pena, F. J., Alonso, M. P., Pedraza-Díaz, S., Ortega-Mora, L. M., García-Parraga, D., et al. (2018). Impact of human-associated Escherichia coli clonal groups in Antarctic pinnipeds: presence of ST73, ST95, ST141 and ST131. Sci. Rep. 8:4678. doi: 10.1038/s41598-018-22943-0
Mora, A., Herrera, A., Mamani, R., López, C., Alonso, M. P., Blanco, J. E., et al. (2010). Recent emergence of clonal group O25b:K1:H4-B2-ST131 ibeA strains among Escherichia coli poultry isolates, including CTX-M-9-producing strains, and comparison with clinical human isolates. Appl. Environ. Microbiol. 76, 6991–6997. doi: 10.1128/AEM.01112-10
Mora, A., Viso, S., López, C., Alonso, M. P., García-Garrote, F., Dabhi, G., et al. (2013). Poultry as reservoir for extraintestinal pathogenic Escherichia coli O45:K1:H7-B2-ST95 in humans. Vet. Microbiol. 167, 506–512. doi: 10.1016/j.vetmic.2013.08.007
Nadeau, E., Fairbrother, J. M., Zentek, J., Belanger, L., Tremblay, D., Tremblay, C. L., et al. (2017). Efficacy of a single oral dose of a live bivalent E. coli vaccine against post-weaning diarrhea due to F4 and F18-positive enterotoxigenic E. coli. Vet. J. 226, 32–39. doi: 10.1016/j.tvjl.2017.07.004
Nagy, B., and Fekete, P. Z. (1999). Enterotoxigenic Escherichia coli (ETEC) in farm animals. Vet. Res. 30, 259–284.
Nagy, B., Wilson, R. A., and Whittam, T. S. (1999). Genetic diversity among Escherichia coli isolates carrying f18 genes from pigs with porcine postweaning diarrhea and edema disease. J. Clin. Microbiol. 37, 1642–1645.
Nicolas-Chanoine, M. H., Bertrand, X., and Madec, J. Y. (2014). Escherichia coli ST131, an intriguing clonal group. Clin. Microbiol. Rev. 27, 543–574. doi: 10.1128/CMR.00125-13
Nicolas-Chanoine, M. H., Blanco, J., Leflon-Guibout, V., Demarty, R., Alonso, M. P., Caniça, M. M., et al. (2008). Intercontinental emergence of Escherichia coli clone O25:H4-ST131 producing CTX-M-15. J. Antimicrob. Chemother. 61, 273–281. doi: 10.1093/jac/dkm464
Ortiz de la Tabla, V., Ortega, A., Buñuel, F., Pérez-Vázquez, M., Marcos, B., and Oteo, J. (2017). Detection of the high-risk clone ST131 of Escherichia coli carrying the colistin resistance gene mcr-1 and causing acute peritonitis. Int. J. Antimicrob. Agents 49, 115–116. doi: 10.1016/j.ijantimicag.2016.10.003
Osek, J., Gallien, P., Truszczyñski, M., and Protz, D. (1999). The use of polymerase chain reaction for determination of virulence factors of Escherichia coli strains isolated from pigs in Poland. Comp. Immunol. Microbiol. Infect. Dis. 22, 163–174.
Poirel, L., Jayol, A., and Nordmann, P. (2017). Polymyxins: antibacterial activity, susceptibility testing, and resistance mechanisms encoded by plasmids or chromosomes. Clin. Microbiol. Rev. 30, 557–596. doi: 10.1128/CMR.00064-16
Post, K., Bosworth, B., and Knoth, J. (2000). Frequency of virulence factors in Escherichia coli isolated from pigs with postweaning diarrhea and edema disease in North Carolina. Swine Health Prod. 8, 119–120.
Rebelo, A. R., Bortolaia, V., Kjeldgaard, J. S., Pedersen, S. K., Leekitcharoenphon, P., Hansen, I. M., et al. (2018). Multiplex PCR for detection of plasmid-mediated colistin resistance determinants, mcr-1, mcr-2, mcr-3, mcr-4 and mcr-5 for surveillance purposes. Euro. Surveill. 23:17-00672. doi: 10.2807/1560-7917.ES.2018.23.6.17-00672
Rhouma, M., Beaudry, F., Thériault, W., and Letellier, A. (2016). Colistin in pig production: chemistry, mechanism of antibacterial action, microbial resistance emergence, and One Health perspectives. Front. Microbiol. 7:1789. doi: 10.3389/fmicb.2016.01789
Rippinger, P., Bertschinger, H. U., Imberechts, H., Nagy, B., Sorg, I., Stamm, M., et al. (1995). Designations F18ab and F18ac for the related fimbrial types F107, 2134P and 8813 of Escherichia coli isolated from porcine postweaning diarrhoea and from oedema disease. Vet. Microbiol. 45, 281–295.
Schink, A. K., Kadlec, K., Kaspar, H., Mankertz, J., and Schwarz, S. (2013). Analysis of extended-spectrum-beta-lactamase-producing Escherichia coli isolates collected in the GERM-Vet monitoring programme. J. Antimicrob. Chemother. 68, 1741–1749. doi: 10.1093/jac/dkt123
Shepard, S. M., Danzeisen, J. L., Isaacson, R. E., Seemann, T., Achtman, M., and Johnson, T. J. (2012). Genome sequences and phylogenetic analysis of K88- and F18-positive porcine enterotoxigenic Escherichia coli. J. Bacteriol. 194, 395–405. doi: 10.1128/JB.06225-11
Shuman, M. E., and Stock, A. H. (1956). Epidemiologic studies on enteropathogenic Escherichia coli diarrhea. Ann. N. Y. Acad. Sci. 66, 108–111.
Smith, M. G., Jordan, D., Chapman, T. A., Chin, J. J., Barton, M. D., Do, T. N., et al. (2010). Antimicrobial resistance and virulence gene profiles in multi-drug resistant enterotoxigenic Escherichia coli isolated from pigs with post-weaning diarrhoea. Vet. Microbiol. 145, 299–307. doi: 10.1016/j.vetmic.2010.04.004
Sonnevend, A., Ghazawi, A., Alqahtani, M., Shibl, A., Jamal, W., Hashmey, et al. (2016). Plasmid-mediated colistin resistance in Escherichia coli from the Arabian Peninsula. Int. J. Infect. Dis. 50, 85–90. doi: 10.1016/j.ijid.2016.07.007
Spurbeck, R. R., Dinh, PC Jr, Walk, S. T., Stapleton, A. E., Hooton, T. M., Nolan, L. K., et al. (2012). Escherichia coli isolates that carry vat, fyuA, chuA, and yfcV efficiently colonize the urinary tract. Infect. Immun. 80, 4115–4122. doi: 10.1128/IAI.00752-12
Sun, J., Zhang, H., Liu, Y. H., and Feng, Y. (2018). Towards understanding MCR-like colistin resistance. Trends Microbiol. 26, 794–808. doi: 10.1016/j.tim.2018.02.006
Tamura, K., Stecher, G., Peterson, D., Filipski, A., and Kumar, S. (2013). MEGA6: Molecular evolutionary genetics analysis version 6.0. Mol. Biol. Evol. 30, 2725–2729. doi: 10.1093/molbev/mst197
Terveer, E. M., Nijhuis, R. H. T., Crobach, M. J. T., Knetsch, C. W., Veldkamp, K. E., Gooskens, J., et al. (2017). Prevalence of colistin resistance gene (mcr-1) containing Enterobacteriaceae in feces of patients attending a tertiary care hospital and detection of a mcr-1 containing, colistin susceptible E. coli. PLoS One 12:e0178598. doi: 10.1371/journal.pone.0178598
Trabulsi, L. R., Keller, R., and Tardelli Gomes, T. A. (2002). Typical and atypical enteropathogenic Escherichia coli. Emerg. Infect. Dis. 8, 508–513. doi: 10.3201/eid0805.010385
Trobos, M., Christensen, H., Sunde, M., Nordentoft, S., Agersø, Y., Simonsen, G. S., et al. (2009). Characterization of sulphonamide-resistant Escherichia coli using comparison of sul2 gene sequences and multilocus sequence typing. Microbiology 155, 831–836. doi: 10.1099/mic.0.024190-0
Vu Khac, H., Holoda, E., Pilipcinec, E., Blanco, M., Blanco, J. E., Mora, A., et al. (2006). Serotypes, virulence genes, and PFGE profiles of Escherichia coli isolated from pigs with postweaning diarrhoea in Slovakia. BMC Vet. Res. 2:10. doi: 10.1186/1746-6148-2-10
Vu Khac, H., Holoda, E., Pilipcinec, E., Blanco, M., Blanco, J. E., Dahbi, G., et al. (2007). Serotypes, virulence genes, intimin types and PFGE profiles of Escherichia coli isolated from piglets with diarrhoea in Slovakia. Vet. J. 174, 176–187. doi: 10.1016/j.tvjl.2006.05.019
Watson, V. E., Jacob, M. E., Flowers, J. R., Strong, S. J., DebRoy, C., and Gookin, J. L. (2017). Association of atypical enteropathogenic Escherichia coli with diarrhea and related mortality in kittens. J. Clin. Microbiol. 55, 2719–2735. doi: 10.1128/JCM.00403-17
Weissman, S. J., Johnson, J. R., Tchesnokova, V., Billig, M., Dykhuizen, D., Riddell, K., et al. (2012). High-resolution two-locus clonal typing of extraintestinal pathogenic Escherichia coli. Appl. Environ. Microbiol. 78, 1353–1360. doi: 10.1128/AEM.06663-11
Westerman, R. B., Mills, K. W., Phillips, R. M., Fortner, G. W., and Greenwood, J. M. (1988). Predominance of the ac variant in K88-positive Escherichia coli isolates from swine. J. Clin. Microbiol. 26, 149–150.
Wirth, T., Falush, D., Lan, R., Colles, F., Mensa, P., Wieler, L. H., et al. (2006). Sex and virulence in Escherichia coli: an evolutionary perspective. Mol. Microbiol. 60, 1136–1151. doi: 10.1111/j.1365-2958.2006.05172.x
Won, G., and John Hwa, L. (2017). Potent immune responses induced by a Salmonella ghost delivery system that expresses the recombinant Stx2eB, FedF, and FedA proteins of the Escherichia coli-producing F18 and Shiga toxin in a murine model and evaluation of its protective effect as a porcine vaccine candidate. Vet. Q. 37, 81–90. doi: 10.1080/01652176.2017.1308040
Xavier, B. B., Lammens, C., Ruhal, R., Kumar-Singh, S., Butaye, P., Goossens, H., et al. (2016). Identification of a novel plasmid-mediated colistin-resistance gene, mcr-2, in Escherichia coli, Belgium, June 2016. Euro. Surveill. 21:30280. doi: 10.2807/1560-7917.ES.2016.21.27.30280
Yin, W., Li, H., Shen, Y., Liu, Z., Wang, S., Shen, Z., et al. (2017). Novel plasmid-mediated colistin resistance gene mcr-3 in Escherichia coli. MBio 8:e00543-17. doi: 10.1128/mBio.00543-17
Zhou, K., Luo, Q., Wang, Q., Huang, C., Lu, H., John, R. W. A., et al. (2018). Silent transmission of an IS1294b-deactivated mcr-1 gene with inducible colistin resistance. Int. J. Antimicrob. Agents 51, 822–828. doi: 10.1016/j.ijantimicag.2018.01.004
Keywords: E. coli, ST10, ST131, mcr-1, EPEC, ETEC, STEC, post-weaning diarrhea
Citation: García-Meniño I, García V, Mora A, Díaz-Jiménez D, Flament-Simon SC, Alonso MP, Blanco JE, Blanco M and Blanco J (2018) Swine Enteric Colibacillosis in Spain: Pathogenic Potential of mcr-1 ST10 and ST131 E. coli Isolates. Front. Microbiol. 9:2659. doi: 10.3389/fmicb.2018.02659
Received: 23 August 2018; Accepted: 18 October 2018;
Published: 05 November 2018.
Edited by:
Sebastian Guenther, University of Greifswald, GermanyReviewed by:
Catherine M Logue, University of Georgia, United StatesMontserrat Llagostera, Autonomous University of Barcelona, Spain
Copyright © 2018 García-Meniño, García, Mora, Díaz-Jiménez, Flament-Simon, Alonso, Blanco, Blanco and Blanco. This is an open-access article distributed under the terms of the Creative Commons Attribution License (CC BY). The use, distribution or reproduction in other forums is permitted, provided the original author(s) and the copyright owner(s) are credited and that the original publication in this journal is cited, in accordance with accepted academic practice. No use, distribution or reproduction is permitted which does not comply with these terms.
*Correspondence: Azucena Mora, YXp1Y2VuYS5tb3JhQHVzYy5lcw==
†These authors have equally contributed to this work