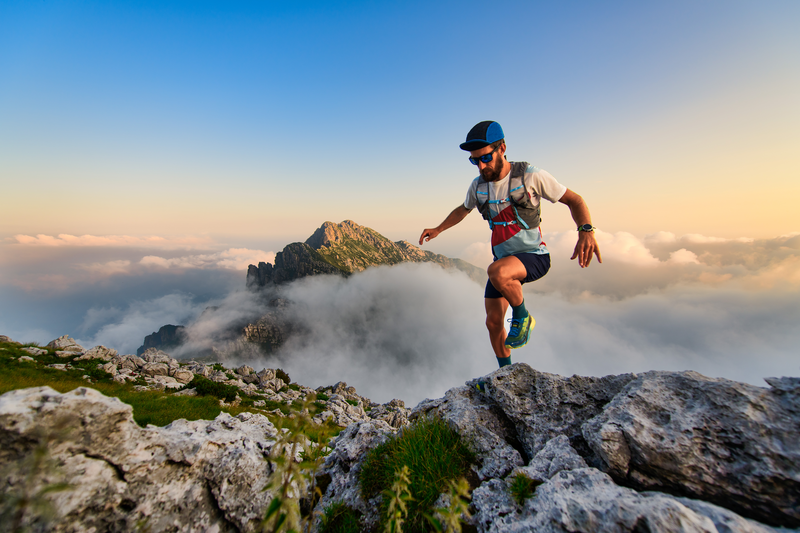
94% of researchers rate our articles as excellent or good
Learn more about the work of our research integrity team to safeguard the quality of each article we publish.
Find out more
ORIGINAL RESEARCH article
Front. Microbiol. , 30 October 2018
Sec. Antimicrobials, Resistance and Chemotherapy
Volume 9 - 2018 | https://doi.org/10.3389/fmicb.2018.02579
This article is part of the Research Topic Horizontal Gene Transfer Mediated Bacterial Antibiotic Resistance View all 24 articles
The ISCR1 (Insertion sequence Common Region) element is the most widespread member of the ISCR family, and is frequently present within γ-proteobacteria that occur in clinical settings. ISCR1 is always associated with the 3′Conserved Segment (3′CS) of class 1 integrons. ISCR1 contains outward-oriented promoters POUT, that may contribute to the expression of downstream genes. In ISCR1, there are two POUT promoters named PCR1-1 and PCR1-2. We performed an in silico analysis of all publically available ISCR1 sequences and identified numerous downstream genes that mainly encode antibiotic resistance genes and that are oriented in the same direction as the POUT promoters. Here, we showed that both PCR1-1 and PCR1-2 significantly increase the expression of the downstream genes blaCTX-M-9 and dfrA19. Our data highlight the role of ISCR1 in the expression of antibiotic resistance genes, which may explain why ISCR1 is so frequent in clinical settings.
Antimicrobial resistance is often mediated by the dissemination of antibiotic resistance genes (ARG) that are carried by mobile genetic elements (MGEs) including plasmids, insertion sequences (IS), transposons (Tn) and integron gene cassettes (Partridge, 2011) which are harbored by bacteria across all phyla and environments (Aminov, 2011). In addition, some MGEs may carry promoters that ensure or increase expression of downstream ARG. Several IS including IS1999, ISEcp1, ISKpn23 (reviewed in Vandecraen et al., 2017) display a complete outwardly oriented functional promoter usually referred as POUT that enhances expression of downstream ARGs. Other IS like IS1 or IS257 only contain the -35 element that generates a hybrid functional promoter when associated with a downstream putative -10 element (Goussard et al., 1991; Simpson et al., 2000). Most often, these IS-borne promoters allow sufficient expression of ARGs to confer the antibiotic resistance phenotype. IS from the ISCR family are related to the IS91 family and display a rcr gene encoding a putative RCR transposase belonging to the ubiquitous HUH endonuclease superfamily (Chandler et al., 2013). HUH transposases of the IS91 family catalyze the transposition of their cognate IS by the rolling-circle replication of the element from one boundary, named oriIS, to the other referred to as the terIS (Tavakoli et al., 2000; del Pilar Garcillán-Barcia et al., 2001; Yassine et al., 2015). However, so far, there is no experimental evidence for transposition of any of the ISCR elements. Four out of the fifteen members of the ISCR family are commonly found in γ-proteobacteria, namely ISCR1, ISCR2, ISCR3 and ISCR5, and ISCR1 predominates in strains isolated in clinical settings (Toleman et al., 2006). ISCR1 was first identified as a conserved region disrupting the 3′ conserved segment (3′CS) of class 1 integrons (Figure 1A; Stokes et al., 1993). The region downstream of ISCR1 (oriIS side) is variable and often associated with ARG (Arduino et al., 2002; Toleman et al., 2006; Rodríguez-Martínez et al., 2007; Wachino et al., 2011). Previous studies identified the presence of two putative promoters located on the oriIS side of the ISCR1, namely PCR1-1 and PCR1-2, suggesting that ISCR1 could impact the expression of downstream genes (Figure 1B; Mammeri et al., 2005; Rodriguez-Martinez et al., 2006). To assess the potential function of these promoters in the expression of downstream genes, we first performed an extensive in silico analysis of all ISCR1 sequences publically available (GenBank®) to determine the diversity of putative downstream ARGs. Here, we show experimentally by means of a reporter gene assay that ISCR1 directly contributes to the expression of different ARGs via these two POUT promoters.
FIGURE 1. Structure of ISCR1. (A) Schematic representation of the ISCR1 element in its genetic context. ISCR1 is inserted 24 bp downstream of the sul1 gene (sulfonamide resistance gene) found in the class 1 integrons 3′ Conserved Segment (3′CS). ISCR1 is flanked by an oriIS-(232 bp) and a terIS-containing region (379 bp). It includes a rcr1 transposase gene, the Prcr1 promoter for rcr1 and two putative outward PCR1-1 and PCR1-2 promoters. A variable region is located downstream ISCR1, separated by an intergenic region. The oriIS-containing region is boxed. (B) Nucleotide sequence of the 232 bp oriIS-containing region of ISCR1. The oriIS sequence that delimitates ISCR1 is highlighted. The –35 and –10 elements of the putative PCR1-1 and PCR1-2 promoters are written in bold. Their corresponding transcriptional start sites as previously mapped are indicated in italics.
Bacterial strains and plasmids are listed in (Supplementary Table S1). Bacterial strains were grown in Lysogeny Broth (LB) broth at 37°C. Media were supplemented with kanamycin (25 μg/mL) when required.
We used the reporter plasmid pSU38ΔtotlacZ (Jové et al., 2010) and three derived plasmids in which ISCR1 and/or regions adjacent to ISCR1 were inserted in transcriptional fusion with the reporter gene lacZ. Fragments of ISCR1 and/or regions adjacent to ISCR1 were amplified from two Salmonella enterica subsp. enterica strains carrying ISCR1 followed by either blaCTX-M-9, or dfrA19 genes (Espéli et al., 2001) as they belong to the most prevalent antibiotic resistance gene families found in the variable region downstream ISCR1. Primers (Sigma-Aldrich®) used for cloning are listed in Supplementary Table S2. For each construction, amplifications were performed using the Phusion® Polymerase (Thermo Fisher Scientific). PCR products were loaded and visualized by means of a 0.8% agarose gel, extracted and purified with the Wizard® SV Gel and PCR Clean-Up System (Promega, Madison, WI, United States). PCR products were cloned into the EcoRI and BamHI unique restriction sites of pSU38ΔtotlacZ. Transformants were selected on LB medium supplemented with kanamycin. Recombinant plasmids were verified by PCR with primers targeting the insert and by sequencing.
β-galactosidase assays were performed in the E. coli MG1656 strain (Supplementary Table S1; Espéli et al., 2001) as previously described (Miller, 1993) for nine independent assays for each construct.
Minimum inhibitory concentrations were performed by the microdilution method in Mueller-Hinton broth in three independent experiments as recommended by the French Antibiogram committee guidelines1.
Statistical analyses were performed using the Mann-Whitney test with two paired groups.
The amino acid sequence of the RCR1 transposase encoded by ISCR1 (accession number CAJ84008) was blasted with BLASTp (NCBI). The matching sequences were filtered to retain RCR1 peptide sequences with an amino acid identity level (equal or) higher than 98%. Corresponding nucleotide sequences in which the oriIS region was partial or truncated were discarded. The remaining nucleotide sequences were sorted out in 93 groups according to the nature of the first gene adjacent to ISCR1: non-annotated nucleotide sequences with identified open reading frame (ORF) longer than 150 bp were included into the analysis. To define a novel gene group, we used a cut-off of 95% amino acid identity of the encoded protein, except for antimicrobial resistance genes (ARGs) for which a single amino-acid variation was used as threshold for inclusion into a group. Finally, one nucleotide sequence representing each gene group was submitted to blastN to identify previously non-annotated nucleotide sequences (only 100% identical sequences were kept). This data extraction was performed on 2017-01-19.
Total RNA was extracted with the NucleoSpin® RNA Extraction Kit (Macherey-Nagel Inc.). Contaminating DNA was removed from RNA samples by using the Turbo DNA-free Kit (Ambion). cDNAs were synthesized from 1 μg of DNase-treated total RNA by using PrimeScriptTM RT Reagent kit (TaKaRa Clontech). cDNA was quantified by PerfeCTa® SYBR® Green FastMix® Kit (Quanta BioSciencesTM) with adequate oligonucleotides (Supplementary Table S2). Three independent experiments were performed, each in triplicate. Relative expressions of the blaCTX-M-9 (Primers 16 and 17) and dfrA19 (Primers 18 and 19) genes were estimated by normalizing transcript copy number to those of the housekeeping gene rpoB (Primer 20 and 21). The impact of ISCR1 oriIS has been calculated as ratio between the relative expression of each gene in presence and in absence of the ISCR1 oriIS.
In this study, 1127 distinct sequences containing the ISCR1 element extracted from GenBank® were analyzed in silico. The majority of these sequences was recovered from γ-proteobacteria (99.9%) while others were found to be present in uncultured bacteria (n = 4) (Supplementary Table S3). All ISCR1 sequences in this study were associated with the 3′ CS region of class 1 integrons (left-hand side, Figure 1). In contrast, the downstream region of ISCR1 was identified to be very variable (right-hand side, Figure 1). Interestingly, a large percentage of the analyzed ISCR1 elements (n = 946, 84%) carried an adjacent gene oriented in the same direction as the rcr1 encoding transposase gene (top strand). This suggests that these genes might be expressed from the ISCR1 POUT promoters (Figure 1). The functions of these top strand genes adjacent to ISCR1 fell into three categories (Supplementary Table S4). The most represented genes (n = 429) encoded truncated insertion sequence transposases, most often ISEc28 (n = 418), more rarely ISEc29, ISAba125 or ISEcp1 (Supplementary Table S4). The second most important group (n = 379) was identified as known or putative ARGs encoding resistance to five families of antibiotics: trimethoprim (n = 125), β-lactams (including extended-spectrum β-lactamase, ESBL, genes) (n = 121), quinolones (n = 113), chloramphenicol (n = 12), and aminoglycosides (n = 8). For each antibiotic family, different genes or alleles were identified, that may lead to different resistance phenotypes (Supplementary Table S4). The last group (n = 138) includes genes involved in other cellular process or genes of unknown function (Supplementary Table S4).
To investigate the impact of POUT promoters on the expression of downstream genes, we focused on the two following ARGs: blaCTX-M-9 (conferring an Extended-Spectrum Beta-Lactamase resistance phenotype) (accession number: AM234698) and dfrA19 (resistance to trimethoprim) (accession number: AF174129), which were the ARG sequences most frequently found in our in silico analysis. Their coding sequences are located 94 and 532 bp away from the oriIS, respectively (Figure 2); this distance is thereafter referred as to the intergenic region or IGR. We cloned each IGR in front of the promoter-less lacZ gene in absence or in presence of the ISCR1 oriIS region (that contains the POUT promoters) and compared the resulting β-galactosidase activities. When the lacZ coding sequence was preceded by each IGR alone, the level of β-galactosidase activity ranged from 84 MU (Miller Units) to 155 MU for dfrA19, and blaCTX-M-9, respectively (Figure 3A, pULP1 and Figure 3B, pULP3). These results indicate the presence of a functional promoter in each IGR. Accordingly, a conserved σ70 promoter sequence was identified in the IGR of blaCTX-M-9 (TTGCTT-N15-TAATGA) and dfrA19 (TTGAAG-N15-TGN-AATCAT), which could account for the observed β-galactosidase expression (Figure 2). However, when both the oriIS and the IGR were present, the β-galactosidase activity was enhanced by 13- and 15-fold for dfrA19 and blaCTX-M-9, respectively (Figure 3A, pULP2 and Figure 3B, pULP4). These results indicate that the ISCR1 oriIS region significantly increases the expression level of downstream genes and confirm that ISCR1 harbors a functional POUT promoter. We thus showed that both ISCR1 oriIS and IGR are involved in gene expression of dfrA19 and blaCTX-M-9. However, as we observed in our in silico analysis (Supplementary Table S4), the sequence and length of the IGR vary from 0 to 1211 bp. Further analysis with other ISCR1 elements with different downstream genes are needed to elucidate to which extent the sequence of the IGR contribute to downstream gene expression.
FIGURE 2. Structure of the genetic context of blaCTX-M-9 and dfrA19 genes downstream of ISCR1. (A) Schematic representation of the genetic context of blaCTX-M-9 as found in our in silico analysis, located 94 bp away from ISCR1 (accession number: AF174129). PCTX-M-9 indicates a putative promoter for blaCTX-M-9 located in the intergenic region, 28 bp away from ISCR1. (B) Schematic representation of the genetic context of dfrA19 as found in our in silico analysis, located 532 bp away from ISCR1 (accession number: AM234698). dfrA19 indicates a putative promoter for dfrA19 located in the intergenic region, 328 bp away from ISCR1.
FIGURE 3. Activities and characterization of the ISCR1 POUT promoters. β-galactosidase activities were measured from lacZ-transcriptional fusions with the intergenic region (IGR) cloned either in absence (oriIS–) or in presence (oriIS+) of the 232 bp long ISCR1 oriIS region. The genes tested were blaCTX-M-9 (A) and dfrA19 (B). Derivatives in which one or both PCR1 promoters were mutated were also tested for blaCTX-M-9 (A). Constructions are described in (Supplementary Table S1). The results are the average of at least three independent experiments. ∗p < 0.001.
To determine whether the positive effect of the ISCR1 oriIS on the expression of the downstream gene relies on the two POUT promoters, PCR1-1 (TAAACG-N17-TAAGAT) and PCR1-2 (TTCGGA-N18-TTTATA), we constructed derivatives of pULP2 (oriIS-IGRCTX-M-9-lacZ) in which the putative -10 element, of either PCR1-1 or PCR1-2 or both promoters was mutated. Mutation of PCR1-1 (pULP5) or PCR1-2 (pULP6) reduced by 85 and 40%, respectively, the overall β-galactosidase activity compared to the pULP2 wild-type construction (Figure 2A). Concomitant mutations of both PCR1-1 and PCR1-2 dropped the expression to the basal level detected in absence of oriIS (Figure 3A, pULP7 versus pULP1). These results indicated that both PCR1-1 and PCR1-2 are functional and, together, are responsible for the contribution of ISCR1 to the expression of downstream top strand genes. Consistently, several earlier reports mapped transcriptional START sites in the oriIS that are compatible with the PCR1-1 and PCR1-2 (Mammeri et al., 2005; Rodriguez-Martinez et al., 2006). PCR1-1 also appears to be stronger than PCR1-2 in agreement with its higher conservation degree of its -10 hexamer, with respect to the σ70 consensus sequence (four bases out of six versus three for PCR1-2). These tandem promoters display a synergistic effect but the exact underlying mechanisms remain to be elucidated.
To assess whether the increased level of gene expression due to the ISCR1 oriIS region has a phenotypic impact, we measured the level of resistance conferred by dfrA19 and blaCTX-M-9 genes in absence or in presence of the oriIS region in Escherichia coli. For this purpose, each gene was cloned in pSU38ΔtotlacZ (Supplementary Table S1) with its own IGR preceded or not by the oriIS region. Subsequently, we determined the MIC of the respective clones in presence of the corresponding antibiotic (cefotaxime or trimethoprim) (see below). The Escherichia coli MG1655lac-strain harboring the empty plasmid (pSU38ΔtotlacZ) was susceptible to both cefotaxime (MIC < 0.5 μg/mL) and trimethoprim (MIC < 4 μg/mL). When MG1655lac- is transformed with a pSU38ΔtotlacZ derivative that harbors the blaCTX-M-9 coding sequence alone (pULP13) or preceded by its own IGR (pULP12), the MIC for cefotaxime was also <0.5 μg/mL. The MIC for cefotaxime significantly increased in pULP11 which contains both IGR and oriIS region (MIC > 512 μg/mL). These findings demonstrated that the ISCR1 oriIS region is required for blaCTX-M-9 to confer a cefotaxime resistant phenotype, most likely mediated by the activity of PCR1-1 and PCR1-2. We performed quantification of transcripts and showed that the blaCTX-M-9 transcript number increased by 99-fold (98.82 ± 8.29) in presence of IGR. These results correlate with MIC findings.
In contrast, when preceded by its IGR, the level of trimethoprim resistance conferred by dfrA19 was similar in absence or in presence of the oriIS region (pULP08 MIC = > 2048 μg/mL, pULP09 MIC = > 2048 μg/mL), while the dfrA19 coding sequence alone did not confer any resistance (pULP10, MIC < 4 μg/mL). Susceptibility to higher concentrations of trimethoprim could not be determined since they excess its solubility in DMSO. The dfrA19 resistance gene confers a higher level of resistance compared to other dfrA alleles, such as dfrA10 for example (MIC: 500 μg/mL) (Parsons et al., 1991). We observed a similar resistance phenotype (trimethoprim MIC = > 2048 μg/mL) in absence or in presence of the oriIS region. These results were surprising according to β-galactosidase results obtained with or without the IGR sequence (Figure 3). Quantification of transcripts of dfrA19 confirmed that the IGR plays a role in the expression of the gene. Indeed, we obtained a 20-fold change of transcript number (20.03 ± 4.1, dfrA19) in presence of IGR. Such dissociation between the resistance phenotype and the level of gene expression has been previously described (Barraud and Ploy, 2015). Furthermore, this might also be explained by the nature of the enzyme encoded by dfr genes. Indeed, DFR enzymes are insensitive toward trimethoprim, so neither a high concentration of antibiotic nor the quantity of DFR enzymes will affect the level of resistance. Little is known about the dfrA19 gene and noticeably, it seems to only occur associated with ISCR1 element.
Our data highlight the functionality of the two POUT promoters carried by the ISCR1 element. The fact that those two functional POUT promoters contribute to the expression of various downstream genes, including ARG may explain why ISCR1 is so frequent in clinical settings. Indeed, ISCR1 gives an advantage to the bacteria for antimicrobial resistance expression and one can hypothesize that antibiotic selective pressure has promoted the selection of ISCR1-carrying bacteria.
M-CP and TJ conceived the study. TJ coordinated the study. CL, TJ, and CP performed the experiments. All authors analyzed the data and wrote the manuscript.
CL gratefully acknowledges the Ministère de l’Enseignement Supérieur, de la Recherche et de l’Innovation (MESRI), and the Fonds Européen de Développement Régional (FEDER) for her doctoral training grant. The funders had no role in study design, data collection and interpretation, or the decision to submit the work for publication.
The authors declare that the research was conducted in the absence of any commercial or financial relationships that could be construed as a potential conflict of interest.
We thank Amy Gassama for providing us with the S. enterica subsp. enterica serovar Keurmassar strain and Manon Mellier for her assistance. We also thank Elena Buelow for critical reading of the manuscript.
The Supplementary Material for this article can be found online at: https://www.frontiersin.org/articles/10.3389/fmicb.2018.02579/full#supplementary-material
Aminov, R. I. (2011). Horizontal gene exchange in environmental microbiota. Front. Microbiol. 2:158. doi: 10.3389/fmicb.2011.00158
Arduino, S. M., Roy, P. H., Jacoby, G. A., Orman, B. E., Pineiro, S. A., and Centron, D. (2002). blaCTX-M-2 is located in an unusual class 1 integron (In35) which includes Orf513. Antimicrob. Agents Chemother. 46, 2303–2306. doi: 10.1128/AAC.46.7.2303-2306.2002
Barraud, O., and Ploy, M.-C. (2015). Diversity of class 1 integron gene cassette rearrangements selected under antibiotic pressure. J. Bacteriol. 197, 2171–2178. doi: 10.1128/JB.02455-14
Chandler, M., de la Cruz, F., Dyda, F., Hickman, A. B., Moncalian, G., and Ton-Hoang, B. (2013). Breaking and joining single-stranded DNA: the HUH endonuclease superfamily. Nat. Rev. Microbiol. 11, 525–538. doi: 10.1038/nrmicro3067
del Pilar Garcillán-Barcia, M., Bernales, I., Mendiola, M. V., and de la Cruz, F. (2001). Single-stranded DNA intermediates in IS91 rolling-circle transposition. Mol. Microbiol. 39, 494–501. doi: 10.1046/j.1365-2958.2001.02261.x
Espéli, O., Moulin, L., and Boccard, F. (2001). Transcription attenuation associated with bacterial repetitive extragenic BIME elements. J. Mol. Biol. 314, 375–386. doi: 10.1006/jmbi.2001.5150
Garnier, F., Raked, N., Gassama, A., Denis, F., and Ploy, M.-C. (2006). Genetic environment of quinolone resistance gene qnrB2 in a complex sul1-type integron in the newly described Salmonella enterica serovar Keurmassar. Antimicrob. Agents Chemother. 50, 3200–3202. doi: 10.1128/AAC.00293-06
Goussard, S., Sougakoff, W., Mabilat, C., Bauernfeind, A., and Courvalin, P. (1991). An IS1-like element is responsible for high-level synthesis of extended-spectrum β-lactamase TEM-6 in enterobacteriaceae. Microbiology 137, 2681–2687.
Jové, T., Da, Re, S., Denis, F., Mazel, D., and Ploy, M.-C. (2010). Inverse correlation between promoter strength and excision activity in class 1 integrons. PLoS Genet. 6:e1000793. doi: 10.1371/journal.pgen.1000793
Mammeri, H., Loo, M. V. D., Poirel, L., Martinez-Martinez, L., and Nordmann, P. (2005). Emergence of plasmid-mediated quinolone resistance in Escherichia coli in Europe. Antimicrob. Agents Chemother. 49, 71–76. doi: 10.1128/AAC.49.1.71-76.2005
Miller, J. H. (1993). A Short Course In Bacterial Genetics: A Laboratory Manual And Handbook For Escherichia coli And Related Bacteria. Cold Spring Harbor, NY: Cold Spring Harbor Laboratory Press.
Parsons, Y., Hall, R. M., and Stokes, H. W. (1991). A new trimethoprim resistance gene, dhfrX, in the In7 integron of plasmid pDGO100. Antimicrob. Agents Chemother. 35, 2436–2439. doi: 10.1128/AAC.35.11.2436
Partridge, S. R. (2011). Analysis of antibiotic resistance regions in gram-negative bacteria. FEMS Microbiol. Rev. 35, 820–855. doi: 10.1111/j.1574-6976.2011.00277.x
Rodriguez-Martinez, J.-M., Poirel, L., Canton, R., and Nordmann, P. (2006). Common region CR1 for expression of antibiotic resistance genes. Antimicrob. Agents Chemother. 50, 2544–2546. doi: 10.1128/AAC.00609-05
Rodríguez-Martínez, J. M., Velasco, C., García, I., Cano, M. E., Martínez-Martínez, L., and Pascual, A. (2007). Characterisation of integrons containing the plasmid-mediated quinolone resistance gene qnrA1 in Klebsiella pneumoniae. Int. J. Antimicrob. Agents 29, 705–709. doi: 10.1016/j.ijantimicag.2007.02.003
Simpson, A. E., Skurray, R. A., and Firth, N. (2000). An IS257-derived hybrid promoter directs transcription of a tetA(K) tetracycline resistance gene in the Staphylococcus aureus chromosomal mecregion. J. Bacteriol. 182, 3345–3352. doi: 10.1128/JB.182.12.3345-3352.2000
Stokes, H. W., Tomaras, C., Parsons, Y., and Hall, R. M. (1993). The partial 3”-conserved segment duplications in the integrons In6 from pSa and In7 from pDGO100 have a common origin. Plasmid 30, 39–50. doi: 10.1006/plas.1993.1032
Tavakoli, N., Comanducci, A., Dodd, H. M., Lett, M.-C., Albiger, B., and Bennett, P. (2000). IS1294, a DNA element that transposes by RC transposition. Plasmid 44, 66–84. doi: 10.1006/plas.1999.1460
Toleman, M. A., Bennett, P. M., and Walsh, T. R. (2006). ISCR elements: novel gene-capturing systems of the 21st century? Microbiol. Mol. Biol. Rev. 70, 296–316. doi: 10.1128/MMBR.00048-05
Vandecraen, J., Chandler, M., Aertsen, A., and Van Houdt, R. (2017). The impact of insertion sequences on bacterial genome plasticity and adaptability. Crit. Rev. Microbiol. 43, 709–730. doi: 10.1080/1040841X.2017.1303661
Wachino, J.-I., Yoshida, H., Yamane, K., Suzuki, S., Matsui, M., Yamagishi, T., et al. (2011). SMB-1, a novel subclass B3 metallo- -lactamase, associated with ISCR1 and a class 1 integron, from a carbapenem-resistant Serratia marcescens clinical isolate. Antimicrob. Agents Chemother. 55, 5143–5149. doi: 10.1128/AAC.05045-11
Keywords: antibiotic resistance, promoters, ISCR1, expression, insertion sequence element
Citation: Lallement C, Pasternak C, Ploy M-C and Jové T (2018) The Role of ISCR1-Borne POUT Promoters in the Expression of Antibiotic Resistance Genes. Front. Microbiol. 9:2579. doi: 10.3389/fmicb.2018.02579
Received: 10 July 2018; Accepted: 09 October 2018;
Published: 30 October 2018.
Edited by:
Katy Jeannot, UMR6249 Chrono Environnement, FranceReviewed by:
Apostolos Liakopoulos, Leiden University, NetherlandsCopyright © 2018 Lallement, Pasternak, Ploy and Jové. This is an open-access article distributed under the terms of the Creative Commons Attribution License (CC BY). The use, distribution or reproduction in other forums is permitted, provided the original author(s) and the copyright owner(s) are credited and that the original publication in this journal is cited, in accordance with accepted academic practice. No use, distribution or reproduction is permitted which does not comply with these terms.
*Correspondence: Marie-Cécile Ploy, bWFyaWUtY2VjaWxlLnBsb3lAdW5pbGltLmZy Thomas Jové, dGhvbWFzLmpvdmVAdW5pbGltLmZy
Disclaimer: All claims expressed in this article are solely those of the authors and do not necessarily represent those of their affiliated organizations, or those of the publisher, the editors and the reviewers. Any product that may be evaluated in this article or claim that may be made by its manufacturer is not guaranteed or endorsed by the publisher.
Research integrity at Frontiers
Learn more about the work of our research integrity team to safeguard the quality of each article we publish.