- 1Department of Chemotherapy and Mycoses, National Institute of Infectious Diseases, Tokyo, Japan
- 2Department of Infection Control and Laboratory Diagnostics, Tohoku University School of Medicine, Miyagi, Japan
- 3Department of Infectious Diseases, Toranomon Hospital, Tokyo, Japan
- 4Department of Bacteriology, The Jikei University School of Medicine, Tokyo, Japan
- 5Jikei Center for Biofilm Science and Technology, The Jikei University School of Medicine, Tokyo, Japan
- 6Okinaka Memorial Institute for Medical Research, Tokyo, Japan
- 7Department of Surgical Pathology, Toho University School of Medicine, Tokyo, Japan
Objectives: Candida species are a major cause of hospital infections, including ocular candidiasis, but few studies have examined the propensities of specific species to invade the eye or the unique immunological responses induced. This study examined the frequency and characteristics of species-specific Candida eye infections by epidemiology and experiments using a mouse ocular candidiasis model.
Methods: We reviewed medical records of candidemia patients from January 2012 to March 2017. We also evaluated ocular fungal burden, inflammatory cytokine and chemokine profiles, and inflammatory cell profiles in mice infected with Candida albicans, Candida glabrata, or Candida parapsilosis.
Results: During the study period, 20 ocular candidiasis cases were diagnosed among 99 candidemia patients examined by ophthalmologists. Although C. parapsilosis was the most frequent candidemia pathogen, only C. albicans infection was significantly associated with ocular candidiasis by multivariate analysis. In mice, ocular fungal burden and inflammatory mediators were significantly higher during C. albicans infection, and histopathological analysis revealed invading C. albicans surrounded by inflammatory cells. Ocular neutrophil and inflammatory monocyte numbers were significantly greater during C. albicans infection.
Conclusion: Candida albicans is strongly associated with ocular candidiasis due to greater capacity for invasion, induction of inflammatory mediators, and recruitment of neutrophils and inflammatory monocytes.
Introduction
Candida species are among the most common causative pathogens of bloodstream infections in hospitals, and ocular candidiasis is a potentially severe complication that can lead to visual field defects or blindness if appropriate therapy is delayed (Verfaillie et al., 1991; Wisplinghoff et al., 2004). Previous reports have shown that all Candida species can cause ocular complications and that ocular candidiasis occurs in approximately 10–25% of Candida infections (Donahue et al., 1994; Oude Lashof et al., 2011; Durand, 2017). Moreover, the guidelines of the Infectious Diseases Society of America (IDSA) recommend that the fundus of all candidemia patients be examined by ophthalmologists within 1 week of diagnosis (Pappas et al., 2016). Several clinical studies found that Candida albicans was the species most frequently causing ocular complications, whereas others reported that ocular inflammation was most often caused by Candida parapsilosis (Donahue et al., 1994; Oude Lashof et al., 2011; Ranjith et al., 2017). Therefore, the species with the greatest propensity for causing ocular candidiasis is still uncertain. According to the IDSA, neutrophils are thought to be the primary effectors of ocular inflammation during candidiasis. However, the detailed immunological responses to specific Candida species and the factors inducing ocular inflammation remain obscure (Pappas et al., 2016).
To address these issues, we retrospectively reviewed the epidemiology of in-hospital ocular candidiasis and compared fungal burdens, chemotactic factor profiles, and inflammatory cell profiles in eyes after infection by specific Candida species in a mouse model.
Materials and Methods
Epidemiology
Medical Record Review
All cases of candidemia occurring between January 2012 and March 2017 at Toranomon Hospital (Tokyo, Japan, 890 beds) were identified. Candidemia was defined by the isolation of any Candida species from one or more sets of blood cultures from patients with signs of infection. Breakthrough candidemia was defined as candidemia occurring in a patient who had received systemic antifungal therapy for over 7 days before the positive blood culture (Antoniadou et al., 2003; Abe et al., 2014).
Ocular examinations were performed by ophthalmologists. All patients with Candida bloodstream infection examined by ophthalmologists were included in this study. Candidemia cases were excluded from consideration if (1) ophthalmologists could not examine the fundus because of the poor general condition or (2) patients died before the blood cultures were confirmed positive. The medical records of each patient were also reviewed to identify the primary disease, the presence of central venous catheter insertion, neutrophil counts, usage of immunosuppressive agents, presence of pre-treatment antifungal agents, serum 1,3-beta-d-glucan (BDG) values, and 30 day all-cause mortality. Serum BDG was measured using the Wako turbidimetric assay (Wako Pure Chemical Industries, Tokyo, Japan) with positivity cutoff of 11 pg/mL (Moro et al., 2003; Kawazu et al., 2004). In the epidemiological investigation, an opt-out approach was used for obtaining consent. This investigation was approved by the Human Ethics Review Committee of Toranomon Hospital (Approved Number: 1546).
Isolation and Identification of Candida Species
Blood culture samples were processed using Bactec 9240 and Bacterc FX systems (Becton, Dickinson and Company, Sparks, MD, United States). All Candida species were isolated on Sabouraud dextrose agar (Nippon Becton Dickinson Company, Ltd., Japan) at 35°C and identified at the species level by a Vitek or Vitek 2 system (bioMérieux, Marcy l’Etoile, France) for all germ tube-negative Candida yeast in the Toranomon Hospital. The D1/D2 regions and/or internal transcribed spacer regions of the rRNA gene from Candida isolates were sequenced to provide further support for species identity by the National Institute of Infectious Diseases (White et al., 1990; O’Donnell, 1993). This research was approved by the Human Ethics Review Committee of the National Institute of Infectious Diseases (Approved Number: 841).
Animal Studies
Mice
Female C57BL/6J mice, 7–9 weeks old, were purchased from Japan SLC, Inc. (Shizuoka, Japan) and maintained under specific pathogen-free conditions at the National Institute of Infectious Diseases in Japan. All experiments were reviewed and approved by the Animal Care and Use Committee of the National Institute of Infectious Diseases. Protocols were designed to minimize animal suffering and limit the numbers used for experiments (Approved Number: 116111).
Yeast Strains and Infection Models
Reference strains of C. albicans (SC5314), Candida glabrata (CBS138), and C. parapsilosis (ATCC22019), and clinically isolated strains of C. albicans (SF-30), C. glabrata (SF-31), and C. parapsilosis (TOR-1) were used for mouse candidiasis model studies. Clinical strains were isolated from blood cultures of candidemia patients. C. albicans and C. parapsilosis were grown at 30°C and C. glabrata at 37°C for 2 days on yeast extract peptone dextrose (YPD) agar. C. albicans and C. parapsilosis were then grown at 30°C and C. glabrata at 37°C in YPD broth for 18–24 h. After incubation, yeasts were collected, washed, and resuspended in sterile PBS at approximately 2.5–3.5 × 106 colony-forming units (CFU)/mL. Mice were injected with 200 μL (approximately 5–7 × 105 CFU/mouse) via the lateral tail vein to cause Candida dissemination.
Evaluation of Organ Fungal Burden and Cytokine Production
In this experiment, mice were divided into three groups as follows: C. albicans, C. glabrata, or C. parapsilosis infected group. The infected mice were euthanatized 3 days after injection, followed by aseptic collection of kidneys and eyeballs. The kidneys and eyeballs were homogenized in sterile PBS, and the homogenates of the kidneys were then serially diluted. Kidney homogenate was plated on YPD agar and eyeball homogenate on YPD agar with antibiotics (penicillin/streptomycin), both at 100 μL per plate. The fungal burden was determined by counting CFUs after 1 day incubation at 30°C. The remaining homogenates were centrifuged and stored at -30°C for cytokine analysis. Cytokines were measured by commercial enzyme-linked immunosorbent assay (ELISA) kits (interleukin [IL]-6, IL-12 p40, and tumor necrosis factor [TNF]-α kits from BD Biosciences, Franklin Lakes, NJ, United States; IL-1β, C-X-C motif ligand 1 [CXCL1]/KC, CXCL2/MIP-2, and C-C motif ligand 2 [CCL2]/MCP-1 kits from R&D Systems, Minneapolis, MN, Unites States).
Ocular Cell Isolation and Flow Cytometry
In this experiment, mice were divided into four groups as follows: uninfected control, C. albicans, C. glabrata, or C. parapsilosis infected group. The eyeballs were carefully enucleated and then washed with sterile PBS to eliminate contaminating peripheral blood. After washing, samples were incubated in RPMI medium with 2% fetal bovine serum and digestive enzymes (1 mg/mL hyaluronidase, Sigma-Aldrich, St. Louis, MO, United States; 2 mg/mL collagenase-D; and 10 μg/mL DNase I, Roche, Basel Switzerland) under rotation at 37°C for 1 h. After enzyme digestion, the eyeballs were homogenized and filtered to remove undigested particles. The ocular immune cells recovered in the filtrate were blocked with Fc-receptor blocking antibody and stained with fluorochrome-conjugated antibodies (Listed in the Supplementary Table S1). Immune cells were enumerated using the FACSCanto II® flow cytometer (BD Biosciences). Obtained data were analyzed using FlowJo, version 9.5 (Tree Star, Inc., Ashland, OR, United States).
Histopathological Analysis of Infected Mouse Eyes
For histopathological analysis, eyeballs were removed 3 days after yeast injection and fixed in 10% formalin, dehydrated with ethanol, and embedded in paraffin following standard procedures. Five micrometer tissue sections were mounted onto glass slides (Dako, Japan) and stained with hematoxylin and eosin, periodic acid Schiff, or Gomori methenamine silver. After staining, histological examination was performed under light microscopy.
Statistical Analysis
Categorical variables were compared between two groups using Fisher exact test. Continuous variables were compared among groups with equal variance by analysis of variance (ANOVA) followed by post hoc Tukey–Kramer HSD tests for pair-wise comparisons. If the standard deviations differed among groups, means were compared by Kruskal–Wallis test and post hoc Dunn’s test for pair-wise comparisons. Correlations between ocular fungal burden and cytokine/chemokine levels were evaluated by Pearson’s test. A P-value <0.05 (two tailed) was considered significant for all tests. All variables at P < 0.20 by univariate analysis were included in multivariate stepwise logistic regression analysis in epidemiological investigations. Statistical analyses in epidemiological studies were performed with EZR (Saitama Medical Center, Jichi Medical University), which is a graphical user interface for R (The R Foundation for Statistical Computing). More precisely, it is a modified version of R commander designed to add statistical functions frequently used in biostatistics (Kanda, 2013). Statistical analyses in animal studies were performed using GraphPad Prism, version 7 (GraphPad Software, La Jolla, CA, United States).
Results
Greater Incidence of Ocular Complications During C. albicans Infection Than Non-albicans Candida Infection
We retrospectively investigated the epidemiological data on Candida infection at Toranomon Hospital and identified 123 cases of candidemia during the study period, of which 99 were included in this study. Among these 99 patients, C. parapsilosis was the most common infective species, followed by C. albicans and C. glabrata. C albicans and C. parapsilosis co-infection was detected in one patient. Serum BDG was measured during the treatment period in 97 patients (98%).
Twenty of 99 candidemia patients were diagnosed with ocular candidiasis. The general clinicodemographic characteristics and Candida species distribution of the patients with ocular candidiasis and non-ocular candidiasis are summarized in Tables 1, 2, respectively. The rate of C. albicans bloodstream infection was significantly higher in the ocular candidiasis group than the non-ocular candidiasis group (76% vs. 13%). In contrast, the rates of hematological malignancies, antifungal agent prophylaxis, and neutropenia were significantly higher in the non-ocular candidiasis group. Serum BDG values did not differ between the entire candidiasis cohort and the subgroup with C. albicans bloodstream infection (Supplementary Figures S1A,B). In addition, the proportion of patients seropositive for BDG values did not differ between ocular and non-ocular candidiasis groups (75% vs. 58%). Multivariate analysis showed that only C. albicans bloodstream infection was significantly associated with ocular candidiasis [Odds ratio 20.7, 95% CI (4.84–88.4), P < 0.0001], suggesting that ocular candidiasis is most likely to occur during C. albicans bloodstream infection regardless of underlying diseases or comorbidities.
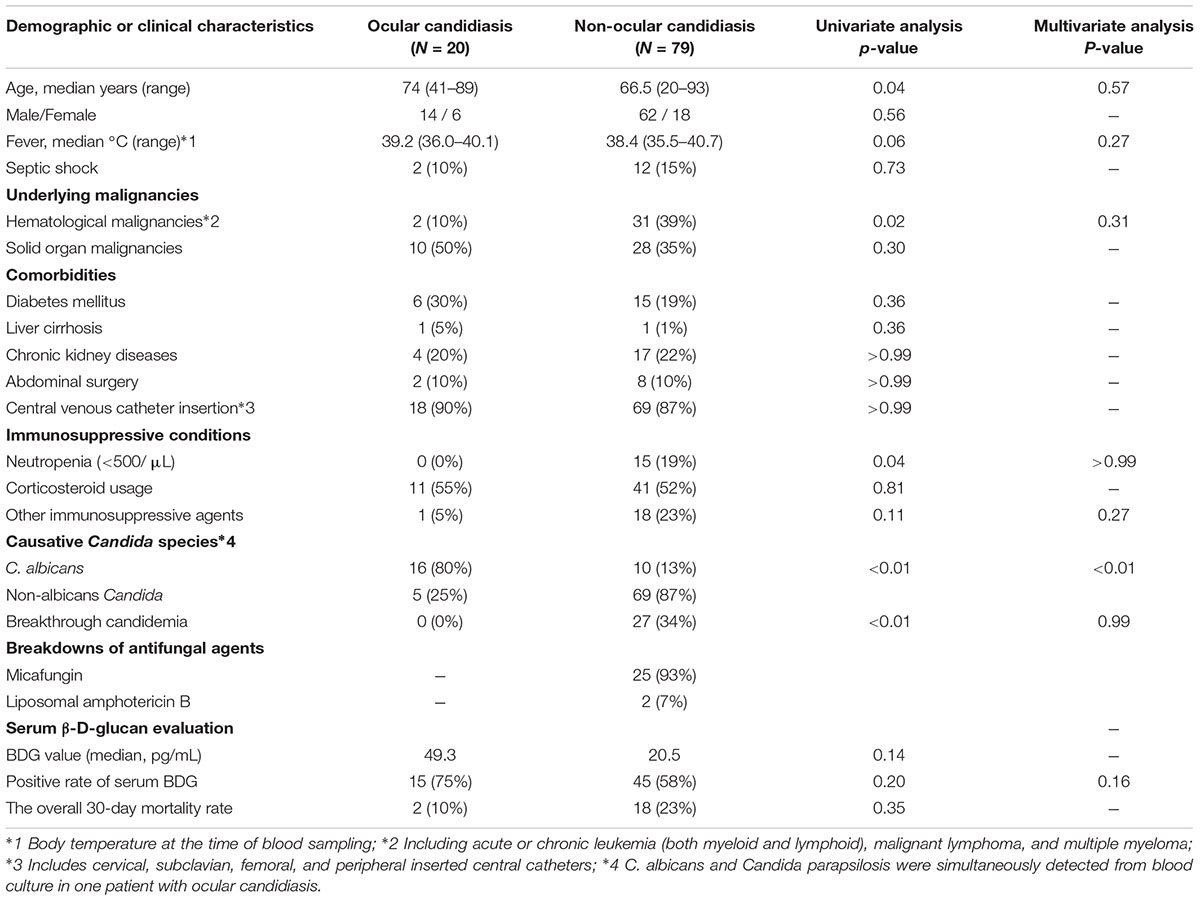
TABLE 1. Epidemiological and medical characteristics of the patients in ocular candidiasis and non-ocular candidiasis groups.
Greater Eye Invasion and Colonization by Candida albicans Than Non-albicans Candida
On the basis of the above results, we performed in vivo experiments using our mouse ocular candidiasis model to compare the propensity for eye infection and severity of ocular inflammation among Candida species following bloodstream infection. Based on our epidemiological data and previous reports, C. albicans, C. glabrata, and C. parapsilosis are the most common Candida species causing in-hospital infection; accordingly, we decided to compare these species using both reference strains and clinical isolated strains. We injected reference strains of C. albicans, C. glabrata, and C. parapsilosis into separate mouse groups as described and then examined the fungal burdens in eyes and kidneys 3 days after injection. The fungal burden in eyes was significantly higher during C. albicans infection compared with non-albicans Candida infection (Figure 1A). In the kidneys as well, C. albicans burden was over 100-fold greater than non-albicans Candida burden (Figure 1B). Similar results were found using clinically isolated strains (Figures 1C,D).
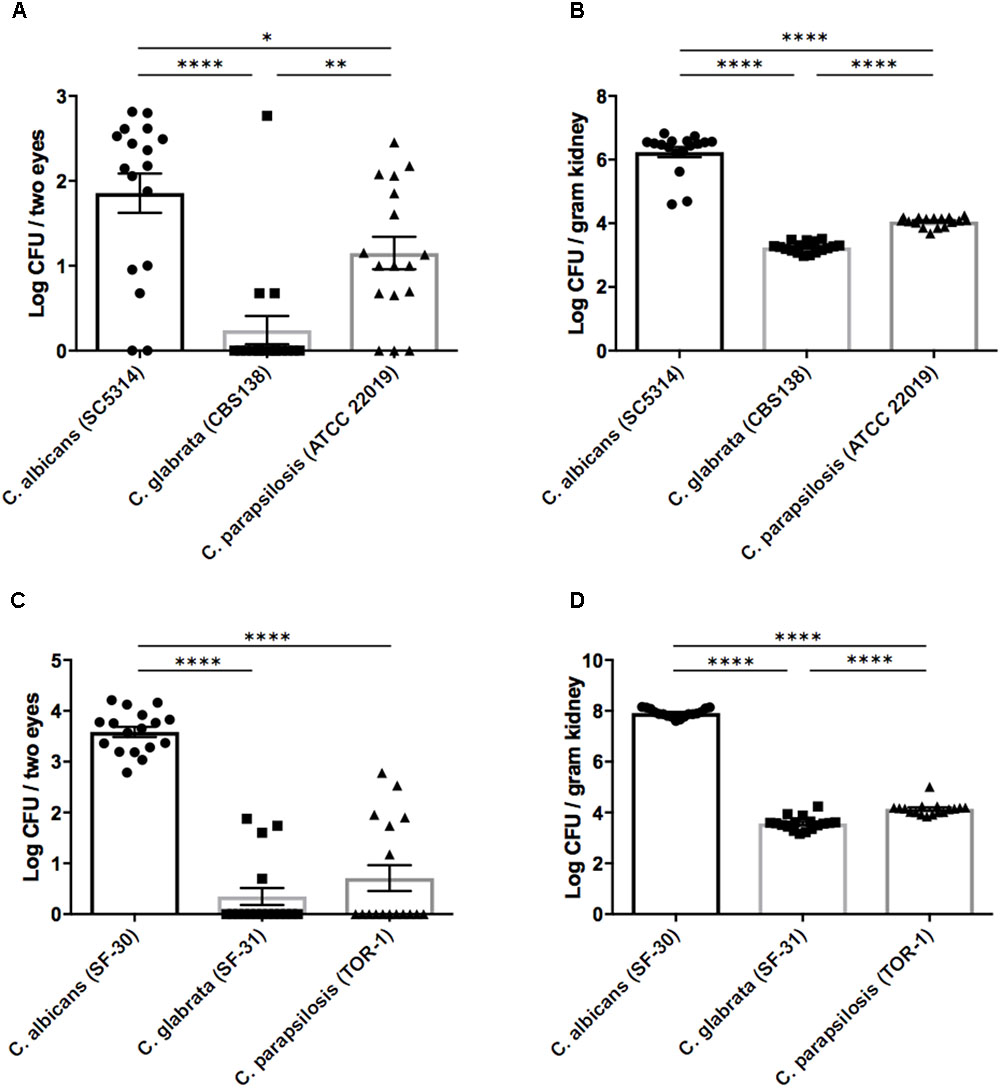
FIGURE 1. Organ fungal burden was significantly higher in mice during Candida albicans infection than non-albicans infection. (A–D) The fungal burden in the eyes and kidneys after injection of Candida species reference strains (A,B) or clinically isolated strains (C,D). Kidney and ocular fungal burdens are shown as the Log CFU/g kidney and Log CFU/2 eyes, respectively. All results are expressed as mean ± standard error of the mean from three independent experiments with a total of 17 tissue samples per group. ∗P < 0.05, ∗∗P < 0.01, and ∗∗∗∗P < 0.0001.
Ocular complications were compared among pathogens by histopathological analysis. Both the reference strain and clinically isolated strain of C. albicans invaded the mouse retina in the form of hyphae and also invaded the vitreous body in one sample (Figures 2A,B and Supplementary Figures S2A,B). In addition, accumulation of inflammatory cells around C. albicans was detected. Conversely, there were no signs of fungal invasion into the eye during infection by C. glabrata or C. parapsilosis (Figures 2C,D and Supplementary Figures S2C,D). These results suggest that C. albicans can more easily invade the retina and cause profound inflammation compared with non-albicans Candida, indicating that these species-specific propensities for ocular candidiasis are due to differences in invasive capacity.
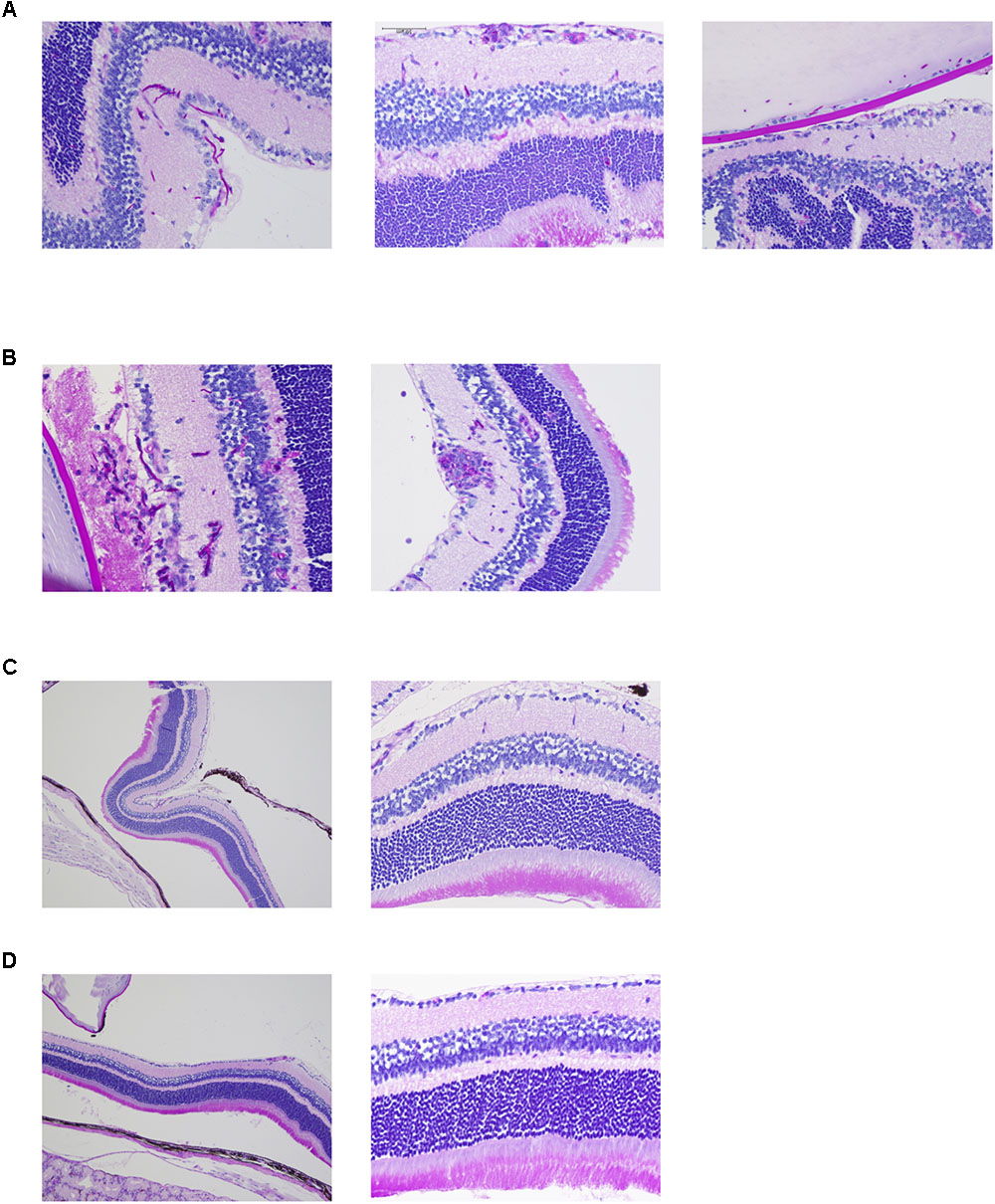
FIGURE 2. Candida albicans demonstrates greater invasive capacity into mouse retina than non-albicans Candida. (A–D) Histopathological analysis of mouse eyes infected by C. albicans reference strain (A), C. albicans clinically isolated strain (B), Candida glabrata clinically isolated strain (C, left panel showing lower magnification), and Candida parapsilosis clinically isolated strain (D, left panel showing lower magnification). These sections were stained with Periodic acid Schiff.
Elevation of Pro-inflammatory Mediators and Chemotactic Factors in Mouse Eyes During C. albicans Infection but Not Non-albicans Candida Infection
To identify factors inducing ocular inflammation following invasion, we measured several cytokines and chemokines in mouse eye homogenates by ELISA after Candida injection. Ocular concentrations of IL-6, CXCL1/KC, CXCL2/MIP-2, and CCL2/MCP-1 were significantly elevated during C. albicans infection compared with non-albicans Candida infection by the reference strains (Figure 3A). Surprisingly, IL-6 was not detected in most eyes following C. parapsilosis injection despite detection of this species in some eye samples. In contrast, the levels of IL-1β, IL-12p40, and TNF-α in ocular homogenate did not differ significantly among the three reference strain infection groups (Supplementary Figure S3A). In the preliminary experiment, these cytokines and chemokines were not elevated in uninfected mouse eyes (data not shown). Ocular concentrations of IL-6, CXCL1/KC, CXCL2/MIP-2, and CCL2/MCP-1 were also higher following injection of the C. albicans clinical strain compared with the other clinical strains (Figure 3B). In addition, ocular IL-1β was higher in mice injected with the clinical C. albicans strain than in mice injected with clinically isolated non-albicans Candida. Conversely, ocular TNF-α and IL-12p40 were significantly lower in mice with C. albicans infection compared with those with C. parapsilosis infection (Supplementary Figure S3B).
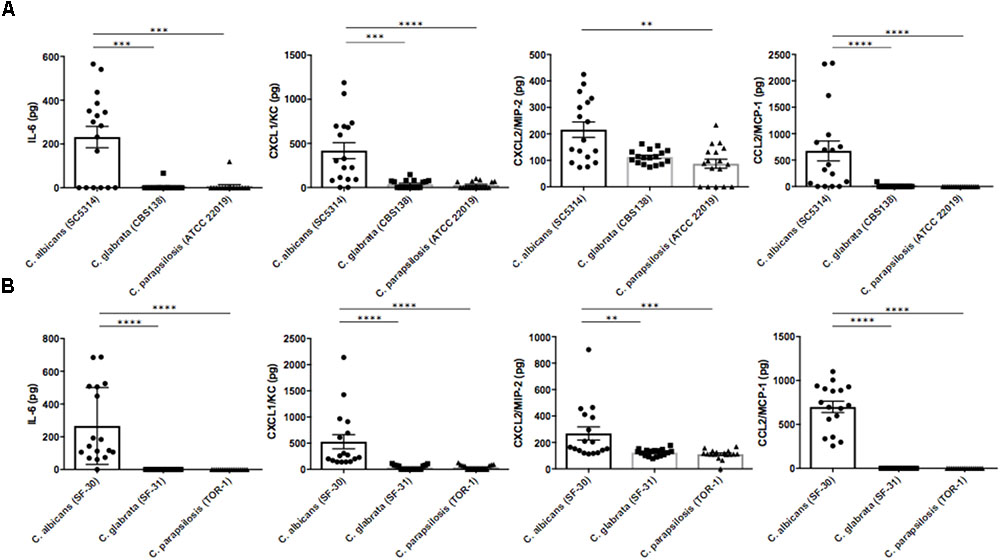
FIGURE 3. Interleukin-6 and neutrophil- or monocyte-attractive chemokines were highly induced in mouse eyes during C. albicans systemic infection. (A,B) Intraocular concentrations of IL-6, CXCL1/KC, CXCL2/MIP-2, and CCL2/MCP-1 3 days after injection of the C. albicans reference strain (A) or clinically isolated strain (B). Ocular concentrations are expressed in pg/mouse. All results are expressed as mean ± standard error of three independent experiments with a total of 17 tissue samples per group (corresponding to Figure 2A). ns, not significant, ∗∗P < 0.01, and ∗∗∗∗P < 0.0001.
Correlation analysis revealed strong positive correlations between ocular fungal burden and IL-6, CXCL1/KC, CXCL2/MIP-2, and CCL2/MCP-1 after injection of both the reference strain and clinically isolated strain of C. albicans (Figures 4A,B). On the other hand, there were no correlations between ocular C. parapsilosis fungal burden and the levels of these chemokines/cytokines (Supplementary Figures S4A,B). During infection by the C. glabrata reference strain, ocular IL-6 and MCP-1 were positively correlated with ocular fungal burden; however, the concentrations of these mediators were low and not detected in most infected mice, indicating only mild inflammation induced by C. glabrata (Supplementary Figures 4C,D). Consistent with the propensity of C. albicans for high ocular fungal burden, invading C. albicans induced severe inflammation by strongly stimulating the release of inflammatory cytokines and neutrophil or monocyte chemotactic factors. Conversely, non-albicans Candida did not exhibit the same invasive capacity or induce the release of inflammatory mediators, especially C. parapsilosis. These differences in the ocular inflammatory cytokine and chemokine profiles between C. albicans and non-albicans Candida likely explain the difference in ocular candidiasis frequency and severity following blood infection.
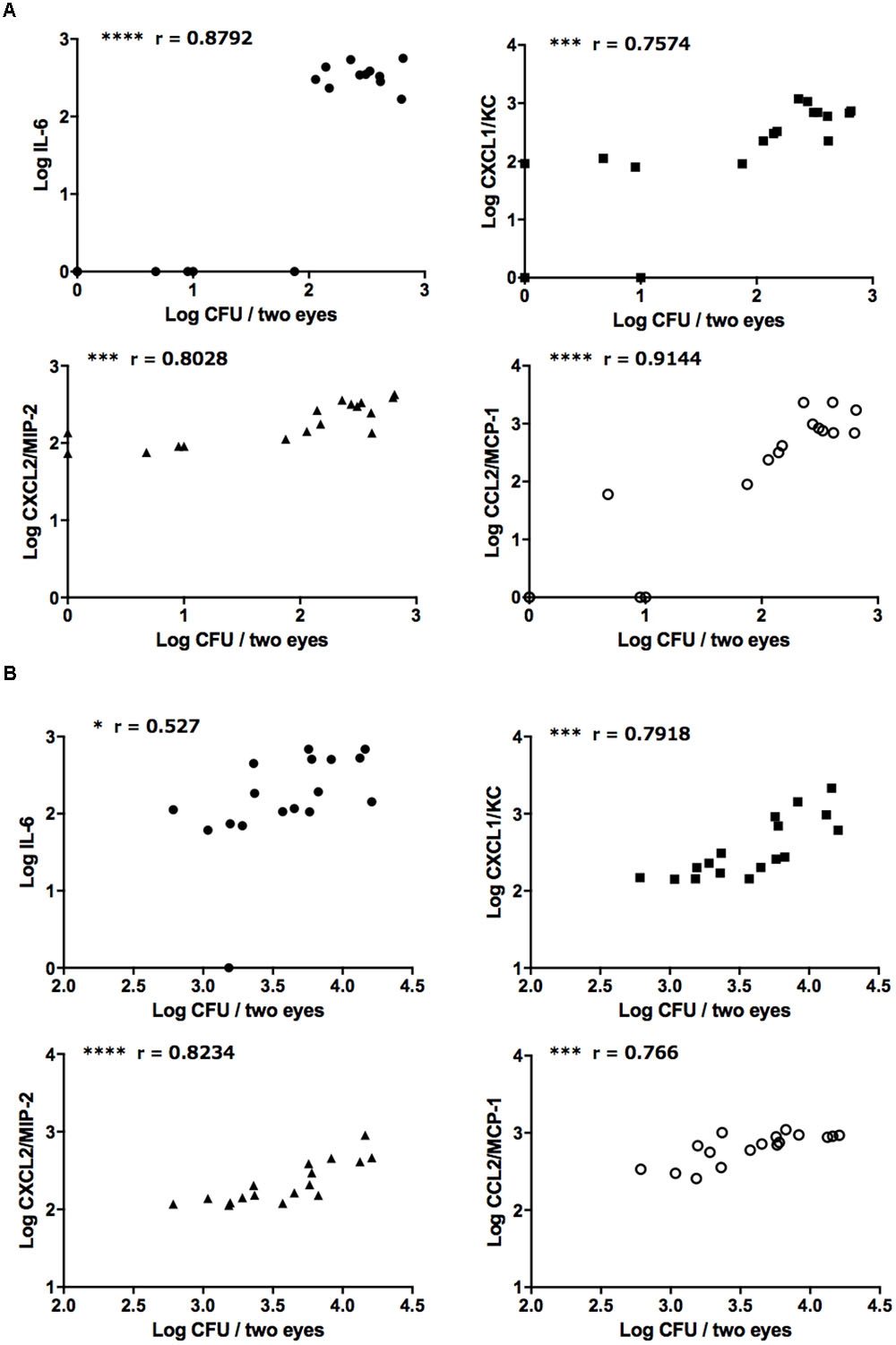
FIGURE 4. Strongly positive relationships between ocular C. albicans burden and ocular concentrations of IL-6 and neutrophil/monocyte-attractive chemokines. (A,B) Relationships between ocular C. albicans burden and IL-6, CXCL1/KC, CXCL2/MIP-2, and CCL2/MCP-1 following injection of the reference strains (A) or clinically isolated strains (B). Ocular fungal burden is shown as Log CFU/2 eyes and ocular cytokine or chemokine levels as Log cytokine or chemokine (pg/mouse). The results of three independent experiments were pooled for analysis, each with 17 tissue samples. “r” is the Pearson’s correlation coefficient. ∗P < 0.05, ∗∗∗P < 0.001, and ∗∗∗∗ P < 0.0001.
Greater Inflammatory Cell Invasion Into Mouse Eyes During C. albicans Infection Than Non-albicans Candida Infection
On the basis of the above results, we examined the ocular inflammatory cell profile associated with each Candida species by flow cytometry. We infected mice with reference strains of each Candida species and quantified inflammatory cells in the eye after 3 days (Figure 5A). The number of CD45+ cells was higher in mice infected by C. albicans compared with non-albicans Candida infection; in fact, there were no differences in CD45+ cell numbers between the non-albicans Candida group and uninfected controls (Figure 5B). Among CD45+ cells, the numbers of ocular neutrophils and inflammatory monocytes were significantly elevated in mice infected by C. albicans compared with the non-albicans Candida group and uninfected controls (Figures 5C,D). In addition, the number of resident monocytes was also higher in mouse eyes during C. albicans infection (Supplementary Figure S5A). Conversely, the number of ocular macrophages was slightly lower in mice infected by C. albicans compared with non-albicans Candida and control groups (Supplementary Figures S5B,C). There was also a difference in neutrophil number between C. parapsilosis and C. glabrata groups, although this was quite small. Collectively, these results suggest that the greater invasive capacity of C. albicans induces a stronger inflammatory cytokine and chemokine response, leading to greater accumulation of neutrophils and inflammatory monocytes in the eye and more severe retinal inflammation (e.g., as manifested by white spots or focal infiltrates).
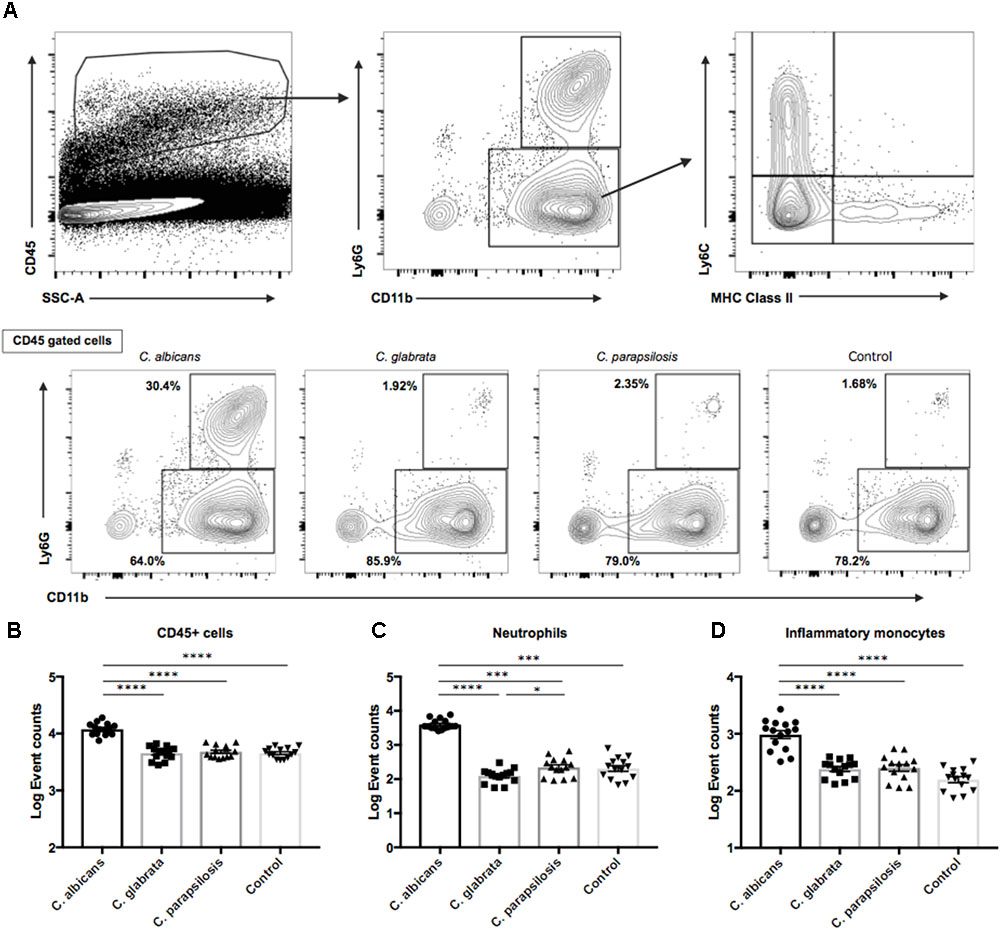
FIGURE 5. Neutrophil and inflammatory monocyte accumulations in mouse eyes were significantly greater during C. albicans infection than non-albicans Candida infection. (A) For flow cytometry analysis, gates were set for CD45 + cells. (B–D) Event counts of (B) ocular hematopoietic cells (CD45 +), (C) neutrophils (CD11b + Ly6G +), and (D) inflammatory monocytes (CD11b + Ly6G-Ly6C + MHC classII-). Cell numbers are shown as Log Event count. All results are expressed as mean ± standard error of the mean from three independent experiments including 15 C. albicans-infected samples and 14 samples for each non-albicans group. ∗P < 0.05, ∗∗P < 0.01, ∗∗∗P < 0.001, and ∗∗∗∗P < 0.0001.
Discussion
Although ocular candidiasis is a serious complication of candidemia, there are few previous reports describing the differences in ocular complications among Candida species. In our epidemiological investigation, C. albicans tended to cause ocular complications more frequently than non-albicans Candida, in accord with previous reports. Moreover, in our mouse ocular candidiasis model, the fungal burden, inflammatory cytokine and chemokine concentrations, and the numbers of ocular neutrophils and inflammatory monocytes were significantly higher following C. albicans injection compared with non-albicans Candida injections. These results indicate the stronger propensity for ocular candidiasis following C. albicans infection results from greater ocular invasive capacity and concomitantly greater secretion of inflammatory mediators and recruitment of neutrophils and inflammatory monocytes.
In the epidemiological study, C. albicans was the most frequent pathogen in ocular candidiasis, in accord with previous studies (Donahue et al., 1994; Shah et al., 2008; Oude Lashof et al., 2011; Lingappan et al., 2012; Nagao et al., 2012; Durand, 2013). In these previous reports, however, C. albicans was also the most frequently isolated species from blood cultures (Oude Lashof et al., 2011; Nagao et al., 2012). In contrast, C. parapsilosis was the most frequent pathogen in our investigation; nevertheless, the rate of ocular candidiasis caused by C. albicans was significantly higher than that caused by non-albicans Candida, suggesting that C. albicans has a stronger propensity for eye invasion, colonization, and induction of ocular candidiasis. In addition, serum BDG values were not significantly different between patients with ocular candidiasis and those without ocular candidiasis in our study, whereas previous reports concluded that serum BDG could be the marker of ocular candidiasis (Takebayashi et al., 2006; Nagao et al., 2012). One possible explanation for this discrepancy is the unique distribution of Candida species among sites. In our study, C. parapsilosis was the most frequent pathogen for candidemia, and non-albicans Candida accounted for approximately three quarters of all candidemia cases. The other possibility is the presence of antifungal prophylaxis. A previous report found that serum BDG was significantly lower in patients with breakthrough candidemia (Abe et al., 2014). Given our result that BDG seropositivity is not significantly higher in patients with ocular candidiasis, clinicians should not exclude the possibility of ocular candidiasis even if serum BDG is low.
In mouse experiments, C. albicans but not the other species test strongly induced inflammatory cytokine or neutrophil chemotactic chemokine release. Neutrophil accumulation was also found in the rabbit ocular candidiasis model, although the factors inducing neutrophil migration were not identified (Omuta et al., 2007). Our mouse experiments revealed that IL-6, KC/CXCL1, MIP-2/CXCL2, and MCP-1/CCL2 were strongly induced by C. albicans retinal invasion. On the other hand, other inflammatory cytokines, such as IL-12p40, IL-1β, or TNF-α, were not elevated; therefore, the aforementioned mediators appear to have central roles in C. albicans-induced ocular inflammation. Although there are no previous reports describing ocular candidiasis in mice, it was reported that KC/CXCL1, but not IL-6, impacts intraocular inflammation in mice during Bacillus cereus infection (Parkunan et al., 2016). In that report, neutrophil infiltration and inflammation as confirmed by histology were significantly less severe in CXCL1-/- mice compared with WT mice, indicating the importance of CXCL1 during B. cereus intraocular infection. On the other hand, another study reported a substantial role for IL-6 in the retinal inflammatory response in a mouse ocular toxoplasmosis reactivation model (Rochet et al., 2015). In our study, IL-6 and other chemokines also increased during C. albicans infection aside from neutrophil-activating KC/CXCL1. These chemokines and IL-6 may recruit other immune cells as flow cytometry revealed inflammatory monocyte infiltration into mouse eyes. Given our results and previous reports, IL-6, CXCL1, and other neutrophil or monocyte chemotactic factors likely all contribute to inflammation in ocular candidiasis.
There are several limitations to this study. First, the epidemiological study was retrospective and the number of cases involved was not large. However, most cases of candidemia were reviewed by experts in infectious diseases, so there were relatively few missing data on ocular candidiasis or serum BDG except for patients in terminal phases. Second, the variety of Candida species and the number of strains was limited to three in mouse experiments. Although many Candida species cause bloodstream infections, these are the top three according to previous studies and accounted for over 80% of candidemia cases in our epidemiological study (Oude Lashof et al., 2011; Nagao et al., 2012). Therefore, our findings are likely applicable to other institutions. Finally, our mouse ocular candidiasis model may reflect only the early phase of inflammation as we injected sublethal fungal burden to cause ocular candidiasis. Although the accumulations of neutrophils and inflammatory monocytes were apparent in the early phase and likely made major contributions to ocular inflammation, further investigation using other ocular candidiasis models are necessary to evaluate the late phase.
In summary, this is the first report describing differences in fungal burden, histopathological manifestations, chemotactic factor profiles, and invading inflammatory cell profiles among ocular candidiasis cases induced by C. albicans and non-albicans Candida in mice. Notably, results were in accord with epidemiological investigations in that C. albicans was both the most prevalent causative pathogen in humans and exhibited the greatest ocular invasive capacity in mice. Ocular candidiasis appeared more likely in cases of systemic infection by C. albicans compared with other Candida species, and the inflammation is more severe because of the greater cytokine/chemokine release and concomitant recruitment of neutrophils and monocytes compared with non-albicans Candida infection.
Author Contributions
MA and YK designed the experiments. MA performed the experiments, acquired, and analyzed the data. KU, ST, SN, MiK, and YM contributed to the mouse experiments. SO, MuK, HA, and AY contributed to the epidemiological study. SS, MS, and KS contributed to the histopathological study. MA and YK drafted the manuscript. All the authors reviewed the manuscript.
Funding
This study was supported in part by grants from the Ministry of Education, Culture, Sports, Science, and Technology of Japan (KAKENHI 18K19529); The Japan Agency for Medical Research and Development, AMED (Grant Nos. JP18fk0108045 and JP18fk0108008); The Ministry of Health Labour and Welfare of Japan; The Yakult Bio-Science Foundation; and the Life Science Foundation of Japan.
Conflict of Interest Statement
The authors declare that the research was conducted in the absence of any commercial or financial relationships that could be construed as a potential conflict of interest.
Acknowledgments
We thank Nobuko Nakayama for technical help, and we also thank medical technologists of Toranomon Hospital: Hiroko Inagawa, Chikako Okada, Reiko Yabusaki, Mayumi Yamanaka, Masaru Baba, Emiko Miyajima, Noriko Watahiki, Hiromi Baba, Chiemi Yoshino, Tomohiro Tsubaki, Yoshiko Kakinohana, Ayumi Takamura, and Yusuke Endo for their invaluable technical assistance.
Supplementary Material
The Supplementary Material for this article can be found online at: https://www.frontiersin.org/articles/10.3389/fmicb.2018.02477/full#supplementary-material
References
Abe, M., Kimura, M., Araoka, H., Taniguchi, S., and Yoneyama, A. (2014). Serum (1,3)-beta-D-glucan is an inefficient marker of breakthrough candidemia. Med. Mycol. 52, 835–840. doi: 10.1093/mmy/myu066
Antoniadou, A., Torres, H. A., Lewis, R. E., Thornby, J., Bodey, G. P., Tarrand, J. P., et al. (2003). Candidemia in a tertiary care cancer center: in vitro susceptibility and its association with outcome of initial antifungal therapy. Medicine 82, 309–321. doi: 10.1097/01.md.0000091182.93122.8e
Donahue, S. P., Greven, C. M., Zuravleff, J. J., Eller, A. W., Nguyen, M. H., Peacock, J. E. Jr., et al. (1994). Intraocular candidiasis in patients with candidemia. Clinical implications derived from a prospective multicenter study. Ophthalmology 101, 1302–1309. doi: 10.1016/S0161-6420(94)31175-4
Durand, M. L. (2013). Endophthalmitis. Clin. Microbiol. Infect. 19, 227–234. doi: 10.1111/1469-0691.12118
Durand, M. L. (2017). Bacterial and fungal endophthalmitis. Clin. Microbiol. Rev. 30, 597–613. doi: 10.1128/CMR.00113-16
Kanda, Y. (2013). Investigation of the freely available easy-to-use software ‘EZR’ for medical statistics. Bone Marrow Transplant. 48, 452–458. doi: 10.1038/bmt.2012.244
Kawazu, M., Kanda, Y., Nannya, Y., Aoki, K., Kurokawa, M., Chiba, S., et al. (2004). Prospective comparison of the diagnostic potential of real-time PCR, double-sandwich enzyme-linked immunosorbent assay for galactomannan, and a (1– > 3)-beta-D-glucan test in weekly screening for invasive aspergillosis in patients with hematological disorders. J. Clin. Microbiol. 42, 2733–2741. doi: 10.1128/JCM.42.6.2733-2741.2004
Lingappan, A., Wykoff, C. C., Albini, T. A., Miller, D., Pathengay, A., Davis, J. L., et al. (2012). Endogenous fungal endophthalmitis: causative organisms, management strategies, and visual acuity outcomes. Am. J. Ophthalmol. 153, 162–166. doi: 10.1016/j.ajo.2011.06.020
Moro, H., Tsukada, H., Ohara, T., Susa, R., Tanabe, Y., Suzuki, E., et al. (2003). Clinical evaluation of performance of a new diagnostic method for deep mycosis by measuring β-glucan concentration in the blood. Kansenshogaku Zasshi 77, 219–226. doi: 10.11150/kansenshogakuzasshi1970.77.219
Nagao, M., Saito, T., Doi, S., Hotta, G., Yamamoto, M., Matsumura, Y., et al. (2012). Clinical characteristics and risk factors of ocular candidiasis. Diagn. Microbiol. Infect. Dis. 73, 149–152. doi: 10.1016/j.diagmicrobio.2012.03.006
O’Donnell, K. (1993). “Fusarium and its near relatives,” in The Fungal Holomorph: Mitotic, Meiotic and Pleomorphic Speciation in Fungal Systematics, eds D. R. Reynolds and J. W. Taylor (Wallingford: CAB International), 225–233.
Omuta, J., Uchida, K., Yamaguchi, H., and Shibuya, K. (2007). Histopathological study on experimental endophthalmitis induced by bloodstream infection with Candida albicans. Jpn. J. Infect. Dis. 60, 33–39.
Oude Lashof, A. M., Rothova, A., Sobel, J. D., Ruhnke, M., Pappas, P. G., and Viscoli, C. (2011). Ocular manifestations of candidemia. Clin. Infect. Dis. 53, 262–268. doi: 10.1093/cid/cir355
Pappas, P. G., Kauffman, C. A., Andes, D. R., Clancy, C. J., Marr, K. A., Ostrosky-Zeichner, L., et al. (2016). Clinical practice guideline for the management of Candidiasis: 2016 update by the infectious diseases Society of America. Clin. Infect. Dis. 62, e1–e50. doi: 10.1093/cid/civ933
Parkunan, S. M., Randall, C. B., Astley, R. A., Furtado, G. C., Lira, S. A., and Callegan, M. C. (2016). CXCL1, but not IL-6, significantly impacts intraocular inflammation during infection. J. Leukoc. Biol. 100, 1125–1134. doi: 10.1189/jlb.3A0416-173R
Ranjith, K., Sontam, B., Sharma, S., Joseph, J., Chathoth, K. N., Sama, K. C., et al. (2017). Candida species from eye infections: drug susceptibility, virulence factors, and molecular characterization. Invest. Ophthalmol. Vis. Sci. 58, 4201–4209. doi: 10.1167/iovs.17-22003
Rochet,É, Brunet, J., Sabou, M., Marcellin, L., Bourcier, T., Candolfi, E., et al. (2015). Interleukin-6-driven inflammatory response induces retinal pathology in a model of ocular toxoplasmosis reactivation. Infect. Immun. 83, 2109–2117. doi: 10.1128/IAI.02985-14
Shah, C. P., McKey, J., Spirn, M. J., and Maguire, J. (2008). Ocular candidiasis; a review. Br. J. Ophthalmol. 92, 466–468. doi: 10.1136/bjo.2007.133405
Takebayashi, H., Mizota, A., and Tanaka, M. (2006). Relation between stage of endogenous fungal endophthalmitis and prognosis. Graefes Arch. Clin. Exp. Ophthalmol. 244, 816–820. doi: 10.1007/s00417-005-0182-5
Verfaillie, C., Weisdorf, D., Haake, R., Hostetter, M., Ramsay, N. K., and McGlave, P. (1991). Candida infections in bone marrow transplant recipients. Bone Marrow Transplant. 8, 177–184.
White, T. J., Bruns, T., Lee, S., and Taylor, J. W. (1990). “Amplification and direct sequencing of fungal ribosomal RNA genes for phylogenetics,” in PCR Protocols: A Guide to Methods and Applications, eds M. A. Innis, D. H. Gelfand, J. J. Sninsky, and T. J. White (New York, NY: Academic Press, Inc.), 315–322.
Keywords: candidemia, ocular candidiasis, Candida albicans, neutrophils, monocytes, cytokines, chemokines
Citation: Abe M, Kinjo Y, Ueno K, Takatsuka S, Nakamura S, Ogura S, Kimura M, Araoka H, Sadamoto S, Shinozaki M, Shibuya K, Yoneyama A, Kaku M and Miyazaki Y (2018) Differences in Ocular Complications Between Candida albicans and Non-albicans Candida Infection Analyzed by Epidemiology and a Mouse Ocular Candidiasis Model. Front. Microbiol. 9:2477. doi: 10.3389/fmicb.2018.02477
Received: 17 July 2018; Accepted: 28 September 2018;
Published: 17 October 2018.
Edited by:
Hector Mora Montes, Universidad de Guanajuato, MexicoReviewed by:
Joseph M. Bliss, Women & Infants Hospital of Rhode Island, United StatesLuis Antonio Pérez-García, Universidad Autónoma de San Luis Potosí, Mexico
Copyright © 2018 Abe, Kinjo, Ueno, Takatsuka, Nakamura, Ogura, Kimura, Araoka, Sadamoto, Shinozaki, Shibuya, Yoneyama, Kaku and Miyazaki. This is an open-access article distributed under the terms of the Creative Commons Attribution License (CC BY). The use, distribution or reproduction in other forums is permitted, provided the original author(s) and the copyright owner(s) are credited and that the original publication in this journal is cited, in accordance with accepted academic practice. No use, distribution or reproduction is permitted which does not comply with these terms.
*Correspondence: Masahiro Abe, bWFzYS1hQG5paWQuZ28uanA= Yuki Kinjo, eWtpbmpvQG5paWQuZ28uanA=