- 1Unité de Glycobiologie Structurale et Fonctionnelle, UMR CNRS 8576, Université des Sciences et Technologies de Lille, Université de Lille, Lille, France
- 2Université de Lille, Lille, France
Osmoregulated periplasmic glucans (OPGs) are general constituents of alpha-, beta-, and gamma-Proteobacteria. This polymer of glucose is required for full virulence of many pathogens including Dickeya dadantii (D. dadantii). The phytopathogenic enterobacterium D. dadantii causes soft-rot disease in a wide range of plants. An OPG-defective mutant is impaired in environment sensing. We previously demonstrated that (i) fluctuation of OPG concentration controlled the activation level of the RcsCDB system, and (ii) RcsCDB along with EnvZ/OmpR controlled the mechanism of OPG succinylation. These previous data lead us to explore whether OPGs are required for other two-component systems. In this study, we demonstrate that inactivation of the EnvZ/OmpR system in an OPG-defective mutant restores full synthesis of pectinase but only partial virulence. Unlike for the RcsCDB system, the EnvZ-OmpR system is not controlled by OPG concentration but requires OPGs for proper activation.
Introduction
Osmoregulated periplasmic glucans (OPGs), β-D-glucans oligosaccharides, are major envelope components found in the periplasm of almost all proteobacteria. Their concentration increases as the osmolarity of the medium decreases (Kennedy, 1996; Bohin and Lacroix, 2006; Bontemps-Gallo et al., 2017). In enterobacteria, the gene products of the opgGH operon synthesize the OPG glucose backbone, which is composed of 5–12 glucose units joined by β,1-2 linkages and branched by β,1-6 linkages. The opgG and opgH mutant strains are completely devoid of OPGs (Bontemps-Gallo et al., 2017). These glucans are well described as virulence factors of animal and plant pathogens including Dickeya dadantii (D. dadantii) (Bontemps-Gallo and Lacroix, 2015).
D. dadantii, the agent of soft rot disease, is directly responsible for 5 to 25% of potato crop loss in Europe and Israel (Toth et al., 2011). This phytopathogen is listed as an A2 quarantine organism by the European and Mediterranean Plant Protection Organization(EPPO, 1982, 1988, 1990). Maceration is the result of the synthesis and secretion of plant cell wall-degrading enzymes (PCWDEs), in particular, pectinases (Collmer and Keen, 1986). However, additional factors, such as motility, are required for full virulence (Charkowski et al., 2012; Reverchon and Nasser, 2013; Leonard et al., 2017). During infection, D. dadantii must overcome several stressors including osmotic stress. Previous studies suggest that bacteria encounter hypoosmotic stress at the early stage of infection and hyperosmotic stress later due to plant maceration (Reverchon and Nasser, 2013; Jiang et al., 2016; Reverchon et al., 2016).
In our model, OPG concentration dramatically increases during the first hour of infection (Bontemps-Gallo et al., 2013). Mutants devoid of OPGs show a pleiotropic phenotype including a loss of motility, decreased synthesis and secretion of PCWDEs, increased synthesis of exopolysaccharide, induction of a general stress response, and complete loss of virulence on potato tubers or chicory leaves (Page et al., 2001; Bouchart et al., 2007). These phenotypes suggest that strains lacking OPGs are impaired in the sensing of their environment. Previously, our laboratory demonstrated a strong relationship between OPGs and the RcsCDB two-component system.
Two-component systems are key regulators of gene expression plasticity in response to environmental changes. Under stimuli, often unknown, a transmembrane sensor histidine kinase (HK) autophosphorylates on a histidine residue. This phosphate group is subsequently transferred to an aspartate residue on a cognate cytoplasmic response regulator (RR), which in turn regulates the expression of a set of target genes (Hoch, 2000; Groisman, 2016).
Inactivation of the RcsCDB system in an OPG-defective mutant restores several of the D. dadantii wild-type phenotypes (motility, mucoidy, and virulence) (Bouchart et al., 2010), indicating that OPGs are involved in the perception of environmental changes. We have also shown that RcsCDB and OPG are tightly connected: (i) fluctuation of OPG concentration controls the activation level of the RcsCDB system (Bontemps-Gallo et al., 2013), and (ii) RcsCDB, along with the two-component system EnvZ/OmpR, controls the mechanism of OPG succinylation (Bontemps-Gallo et al., 2016). These facts lead us to question whether the link between OPGs and the RcsCDB system is a unique feature.
Thirty years ago, Fiedler and Rotering (1988) isolated revertants in OPG-defective mutants of E. coli. The mutation was localized to the ompB locus now known as the envZ-ompR operon. EnvZ-OmpR, the paradigm of two-component systems, regulates the balance between OmpF (large pore diameter) and OmpC (small pore diameter) to control the diffusion rate of nutrients (Cowan et al., 1992; Forst and Roberts, 1994; Egger et al., 1997; Castillo-Keller et al., 2006; Barbieri et al., 2013). This system is also known to control motility in several bacteria (Barker et al., 2004; Clemmer and Rather, 2007; Raczkowska et al., 2011; Lee and Park, 2013; Li et al., 2014; Tipton and Rather, 2016; Pruss, 2017) and is required for full virulence in Yersinia pestis (Gao et al., 2011; Reboul et al., 2014). In D. dadantii, the EnvZ/OmpR system regulates ompF expression (no ompC homolog is present) as well as kdgN, which is required for transport of oligosaccharides arising from pectin degradation during plant infection (Condemine and Ghazi, 2007). Recently, in a global in vitro transcriptomic analysis of various stressors encountered during the infectious process, Jiang et al. (2016) showed that the EnvZ-OmpR system was up-regulated during osmotic stress.
In this study, we demonstrate that EnvZ-OmpR system is not involved in virulence. Instead, inactivation of envZ or ompR in an OPG-defective mutant restores full synthesis of pectinase and partial virulence. We also show that EnvZ-OmpR is involved in regulation of motility. Finally, we demonstrate that ompF and kdgN are osmoregulated by EnvZ-OmpR and are required for proper regulation of OPGs.
Materials and Methods
Bacterial Strains, Media, and Growth Conditions
Bacterial strains are described in Table 1. Bacteria were grown at 30°C in lysogeny broth (LB) (Bertani, 2004), or in minimal medium M63 glycerol [15 mM (NH4)2SO4, 1.8 μM FeSO4, 1 mM MgSO4, and 100 mM K2PHO4] supplemented with 0.2% glycerol as a carbon source (Miller, 1992). Solid media were obtained by adding agar at 15 g.L-1. Motility tests were performed on LB plates containing agar at 4 g.L-1.
Osmolarity (mOsM) was measured with a vapor pressure osmometer (Advanced Instruments, United States). M63 osmolarity was 330 mOsM. Osmolarity was decreased by diluting twofold M63 with H2O to 170 mOsM. Addition of 0.1 and 0.2 M NaCl increased the osmolarity to 500 and 700 mOsM, respectively. Glycerol was added after dilution with water or addition of NaCl.
The solid media used to test the pectinase [M63 supplemented with 0.4% polygalacturonate (PGA) and 0.2% glycerol], cellulases [M63 supplemented with 0.2% carboxymethylcellulose (CMC), 0.2% glycerol, and 7 mM MgSO4], and proteases (LB complemented with 1% of Fat milk) activities have been described previously (Page et al., 2001).
Antibiotics were used at following concentrations: spectinomycin, 2.5 μg.mL-1; chloramphenicol, 12.5 μg.mL-1; and gentamycin, 2 μg.mL-1.
Transduction, Conjugation, and Transformation
Construction of strains was performed by transferring genes from one strain of D. dadantii to another by generalized transduction with phage ΦEC2, as described previously (Resibois et al., 1984). Plasmids were introduced in D. dadantii by conjugation or electroporation.
Expression Analysis
Bacteria were grown until the exponential phase at various osmolarities. RNAs were extracted using Nucleospin RNA Plus Kit (Macherey Nagel) following the manufacturer’s instructions. RNAs were treated with DNase I (BioLabs). RNA qualities were checked by gel and nanodrop.
cDNAs were retrotranscribed using the Superscript IV First-Strand Synthesis (Invitrogen) according to the manufacturer’s instructions.
qPCR was performed using SYBR method as described previously by Hommais et al. (2011). Primers used are listed in Table 2. Further, ipxC, an UDP-N-acetylglucosamine deacetylase, was used as a reference gene (Hommais et al., 2011).
Phenotypic Evaluation
A total of 107 bacteria in 5 μL were spotted onto pectinase (PGA), cellulase (CMC), protease or motility plates. After 48 h incubation, the PGA plates were flooded with a 10% copper acetate solution, which forms a blue complex with the PGA. Diameters of the clear haloes around the colony were measured as an indication of pectinase production. After 48 h incubation, CMC plates were flooded with a 1 mg/ml red Congo solution and washed several times with 1 M NaCl, allowing formation of a red complex with the CMC. Diameters of the clear haloes around the colony were measured as an indication of cellulase production. After 48 h of incubation, the abilities of the strain to degrade milk protein were observed. Swim diameters were measured after 48 h of incubation.
Pathogenicity Test
Potato tubers and chicory leaves were inoculated as previously described (Page et al., 2001). Bacteria from an overnight culture in LB medium were recovered by centrifugation and diluted in water. For potato tubers, sterile pipette tips containing a bacterial suspension of 107 cells in 5 μL were inserted into the tuber (Amandine variety). After 72 h of incubation in a dew chamber, the tubers were sliced vertically through the inoculation point, and the weight of the maceration was measured. For chicory leaves, the leaves were wounded prior to inoculation of 107 bacteria and incubated in a dew chamber at 30°C until 48 h.
Transmission Electron Microscopy
Samples were analyzed by the Bio Imaging Center of University of Lille (France). Wild-type and opgG strains were grown until the mid-log phase. Cells were spun for 5 min at 7,000 × g at 4°C. Bacteria were fixed with 3.125% glutaraldehyde, washed in 0.1 M phosphate buffer pH 7.4, and postfixed with 1% OsO4. The samples were dehydrated with graded acetone series, embedded in EMBED resin, and air dried at 60°C. Thin and ultrathin sections were prepared using an ultramicrotome (Reichert OM U3 or LKB Ultrotome III 8800) and stained with uranyl acetate. Microscopy was performed with a Hitachi H600 microscope at 75 keV electron energy. The periplasm length was measured using ImageJ software.
Statistical Analysis
For statistical analyses, Graph-prism 6 software was used to analyze the data using one-way ANOVA.
Results
Characterization of envZ and ompR Deletion in Wild-Type and opgG Background
To determine whether the EnvZ-OmpR system interacts with OPG, we inactivated envZ or ompR in wild-type and opgG mutant backgrounds (Figure 1A). We then looked at envZ and ompR expression at various osmolarities (Figure 1B). As expected, the expression of both genes was low in the wild-type strain and not affected by osmolarity. In an opgG mutant, the expression level was similar to that observed in the wild-type strain. No expression of envZ or ompR was observed in their respective mutant strain. Interestingly, in the ompR background, a low but measurable expression of envZ was observed. Based on the locus organization, we would expect the ompR mutation to be polar. Expression of envZ in an ompR deletion background suggests the presence of a secondary promoter.
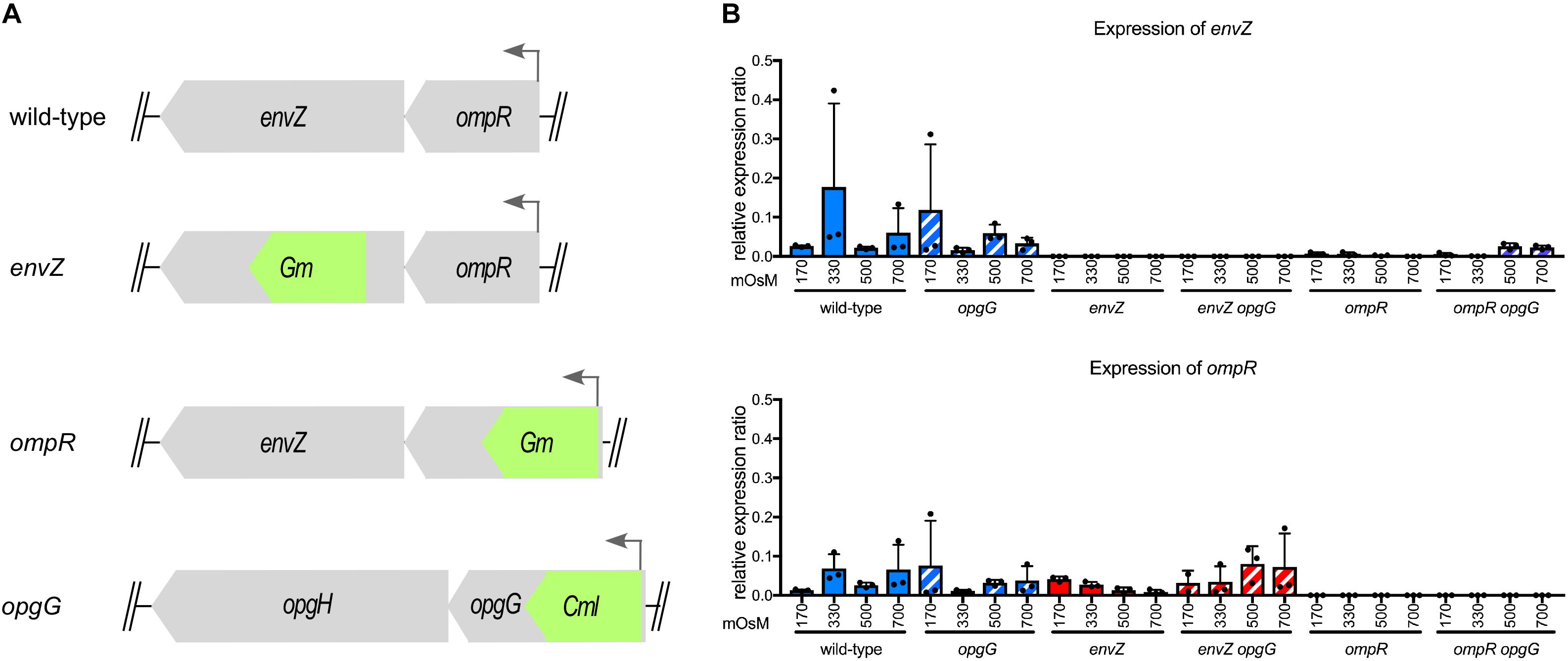
FIGURE 1. Characterization of the envZ and ompR deletion in wild-type and opgG background. (A) Schematic of the envZ-ompR locus in the wild-type strain and genetic organization of the mutant strains. (B) Expression of envZ and ompR was analyzed by qPCR. Bacteria were grown at 170, 330, 500, and 700 mOsM. Relative gene expression was calculated using ipxC as a reference (Hommais et al., 2011). Data represent mean ± standard deviation of three independent experiments.
Inactivation of envZ or ompR Restores the Synthesis of Pectinase in an OPG-Defective Strain
Strains devoid of OPGs are impaired in their ability to synthesize virulence factors, leading to a total loss of virulence. We first assayed plant cell-degrading enzyme activity (Figure 2 and Supplementary Figure 1), which is required for full virulence. Pectinase production and secretion were evaluated on a minimal medium containing polygalacturonate, a substrate for pectinase, and after 48 h of incubation, haloes of degradation were measured (Figure 2A). As expected, the opgG mutant showed a 40% decrease in pectinase production compared to the wild-type. While inactivation of envZ or ompR did not decrease the synthesis of pectinases, envZ opgG and ompR opgG double mutants showed full restoration of pectinase production to levels similar to the wild-type.
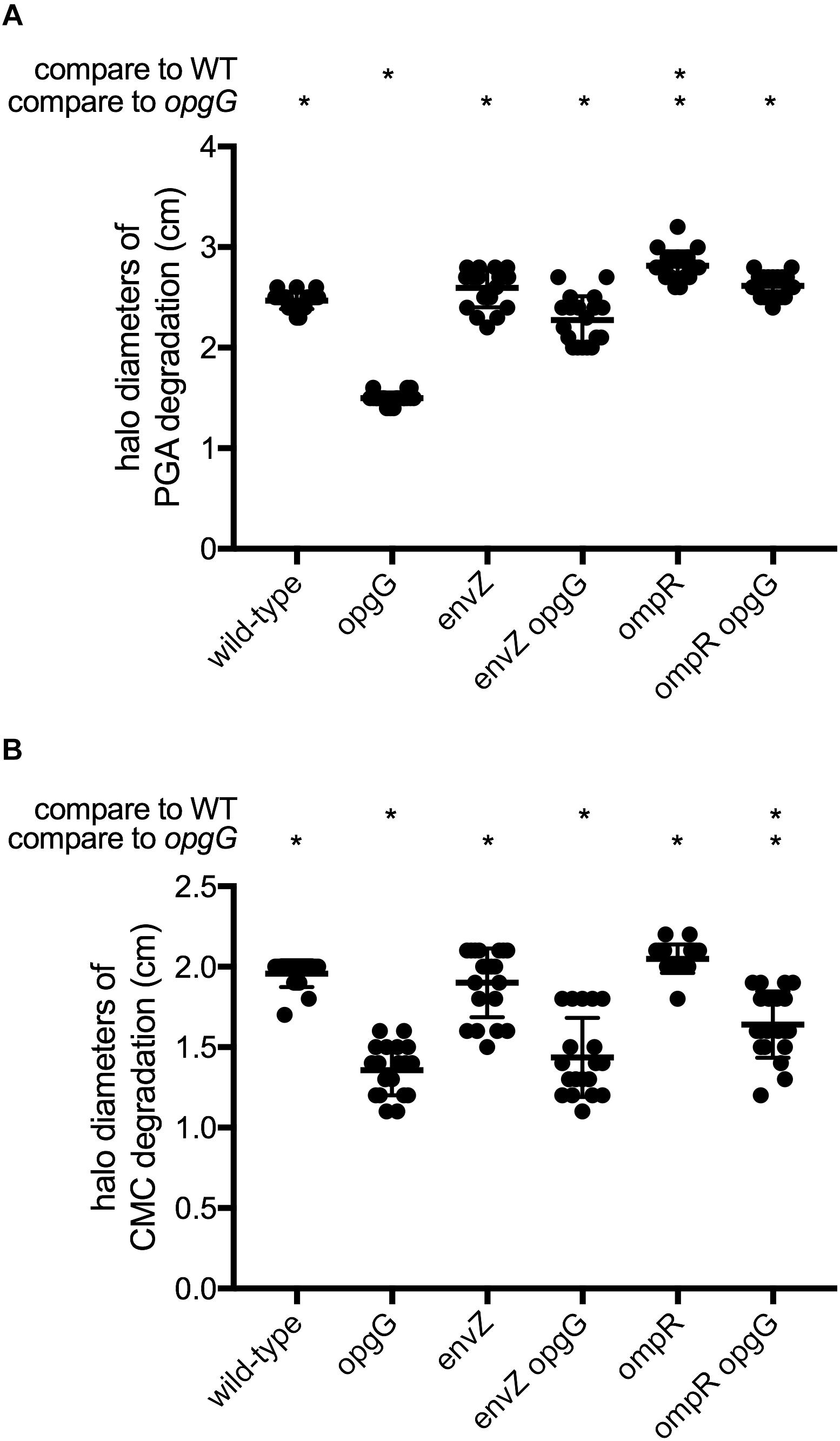
FIGURE 2. Pectinase (A) and cellulase (B) activities. Exoenzyme activities were estimated on plates by the measurement of halo diameters, expressed in cm of substrate degradation. Data represent mean ± standard deviation of 20 independent experiments. An asterisk indicates a significant difference with p < 0.0001.
Cellulase production and secretion were evaluated on a minimal medium containing carboxymethylcellulose, the substrate for cellulase, and haloes of degradation were measured after 48 h of incubation (Figure 2B). As previously shown, opgG inactivation decreased the production of cellulase by 30% (Page et al., 2001). The envZ or ompR null mutants exhibited similar cellulase levels to the wild-type. The envZ opgG and ompR opgG double mutants displayed a reduction in cellulase production similar to the opgG strain.
We also assayed for the production of protease on plates containing 1% milk fat (Table 3). The ability of each strain to degrade milk protein was evaluated after 48 h. No restoration of protease activity was observed in any of the double-mutant strains.
Taken together, our data show that EnvZ-OmpR is not involved in the regulation of PCWDEs. However, disruption of either envZ or ompR is enough to restore full pectinase production in an OPG-defective strain, but not cellulase or protease synthesis.
The EnvZ-OmpR System Is Involved in Motility Regulation
Motility is known to be an important virulence factor (Reverchon and Nasser, 2013). Furthermore, by screening motility in OPG-defective mutants of E. coli, Fiedler and Rotering isolated revertants in the envZ-ompR operon (Fiedler and Rotering, 1988). To determine whether the disruption of envZ-ompR could restore the loss of motility in the opgG mutant, we assayed for motility by measuring swim diameters on 0.4% agar plates (Figure 3A and Supplementary Figure 1). As described previously, the opgG mutant showed a reduction in motility (one third of wild-type levels). Inactivation of envZ or ompR resulted in a 40% reduction in motility compared to the wild-type strain. However, the same mutation in the opgG background did not restore motility.
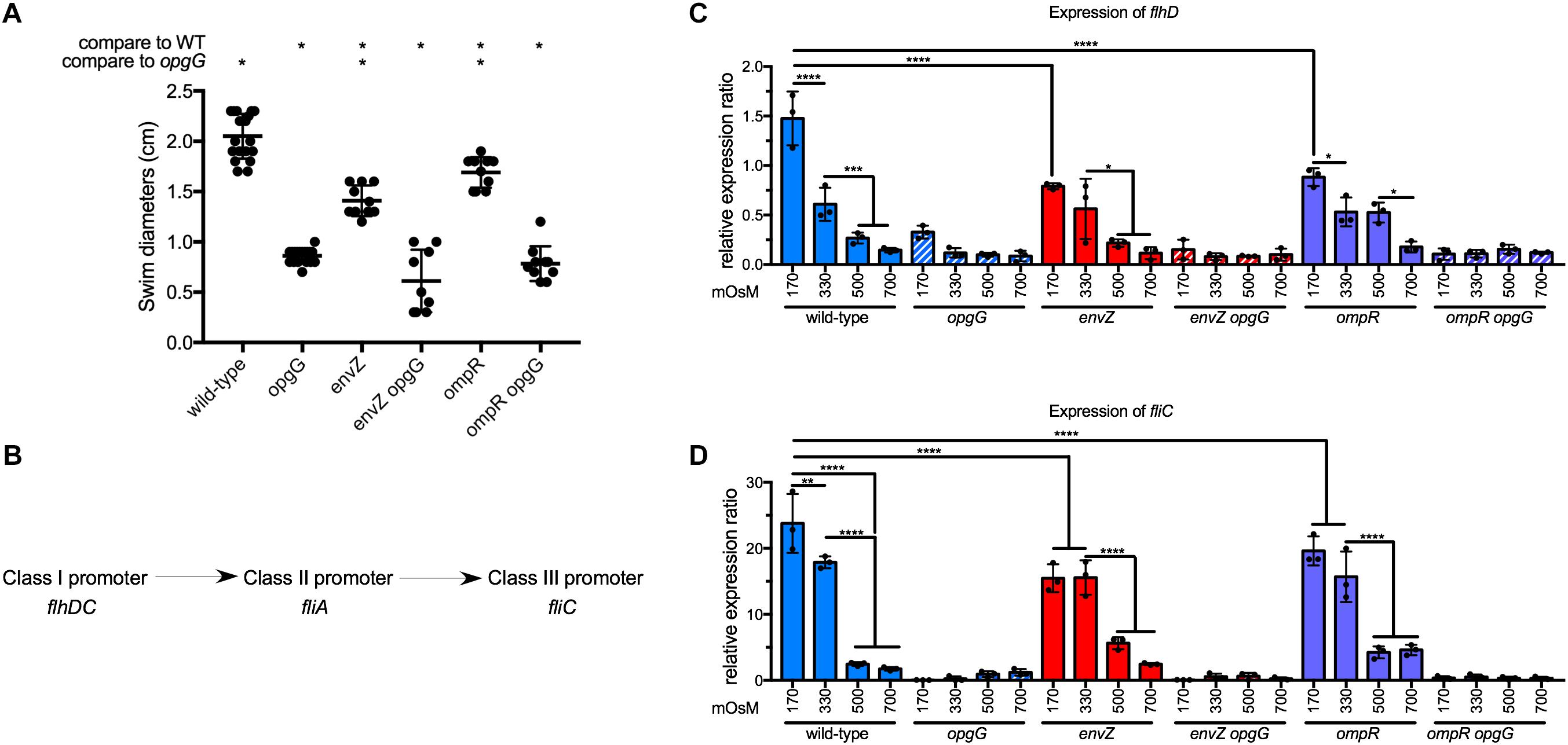
FIGURE 3. Effect of EnvZ-OmpR on motility in wild-type and opgG background. (A) Motility of wild-type, opgG, envZ, envZ opgG, ompR, and ompR opgG strains. Motility was measured in M63 semisolid plates. Swim diameters were measured after 48 h of incubation at 30°C. (B) Schematic of the regulatory cascade of motility. FlhDC, a master regulator and a class I promoter, modulates gene expression with a class II promoter (e.g., fliA). In return, the products of those genes regulate the genes with a class III promoter (e.g., fliC). (C,D) Expression of (C) flhD and (D) fliC in wild-type, opgG, envZ, envZ opgG, ompR, and ompR opgG strains. Bacteria were grown at 170, 330, 500, and 700 mOsM. The expression of (C) flhD, (D) fliC was analyzed by qPCR. Relative gene expression was calculated using ipxC as a reference (Hommais et al., 2011). Data represent mean ± standard deviation of 10 independent experiments. An asterisk indicates a significant difference with ∗∗∗∗p < 0.0001, ∗∗∗p < 0.001, ∗∗p < 0.01, and ∗p < 0.05.
The regulatory cascade for motility is separated into three classes of promoters (Figure 3B). Under motility-inducing conditions, flhDC, the master regulator, is up-regulated to modulate the expression of genes under the control of a class II promoter. Finally, class II genes regulate genes with class III promoters (e.g., fliC, the flagellin). We then tested the effect of the EnvZ-OmpR system on the regulation of motility. In wild-type background, the expression of the master regulator flhD, and consequently fliC, decreased 10-fold from low (170 mOsM) to high (700 mOsM) osmolarity (Figures 3C,D). This data agrees with our previous observation of a twofold decrease in wild-type motility in the same osmolarity range (Bontemps-Gallo et al., 2013). Inactivation of envZ or ompR lead to a decrease in flhD expression but, save for 170 mOsM, this decrease was not statistically significant (Figure 3C). The fliC expression decreased 1.5-fold at 170 and 330 mOsM in the envZ and ompR mutants, respectively, compared to the wild-type (Figures 3C,D). Disruption of opgG resulted in low expression of both flhD and fliC regardless of the genetic background and osmolarity (Figures 3C,D). Our results show that EnvZ-OmpR is involved in the regulation of motility but not as a main regulator of this cascade. Inactivation of this system cannot rescue motility in the opgG background.
Inactivation of EnvZ-OmpR Systems Partially Restores Virulence in an OPG-Defective Strain
Previously, we demonstrated that restoration of pectinase production in an OPG-defective strain is enough to restore virulence in potato tubers but not in chicory leaves (Bontemps-Gallo et al., 2014). We observed that inactivation of the EnvZ-OmpR system in an opgG mutant lead to restoration of full pectinase synthesis (Figure 2A). We therefore determined whether inactivation of this system could restore virulence in both potato tubers (Figure 4 and Supplementary Figure 1) and chicory leaves (Figure 5). Following the inoculation of bacteria in both vegetables and incubation at 30°C, we analyzed the virulence levels. Inactivation of envZ or ompR in a wild-type background had no effect on the virulence levels regardless of the infection model used (Figures 4, 5). Interestingly, when the system was inactivated in an OPG-defective strain, macerations were observed in the tubers (Figure 4). However, the severity of disease was not as strong as for the wild-type strain (only a third of the average maceration weight of the wild-type). No restoration of virulence was observed for envZ opgG or ompR opgG double mutants in chicory leaves (Figure 5). Our data demonstrate that EnvZ-OmpR is not involved in virulence in D. dadantii. Furthermore, restoration of pectinase synthesis in the double mutants allows for maceration but only in potato tubers.
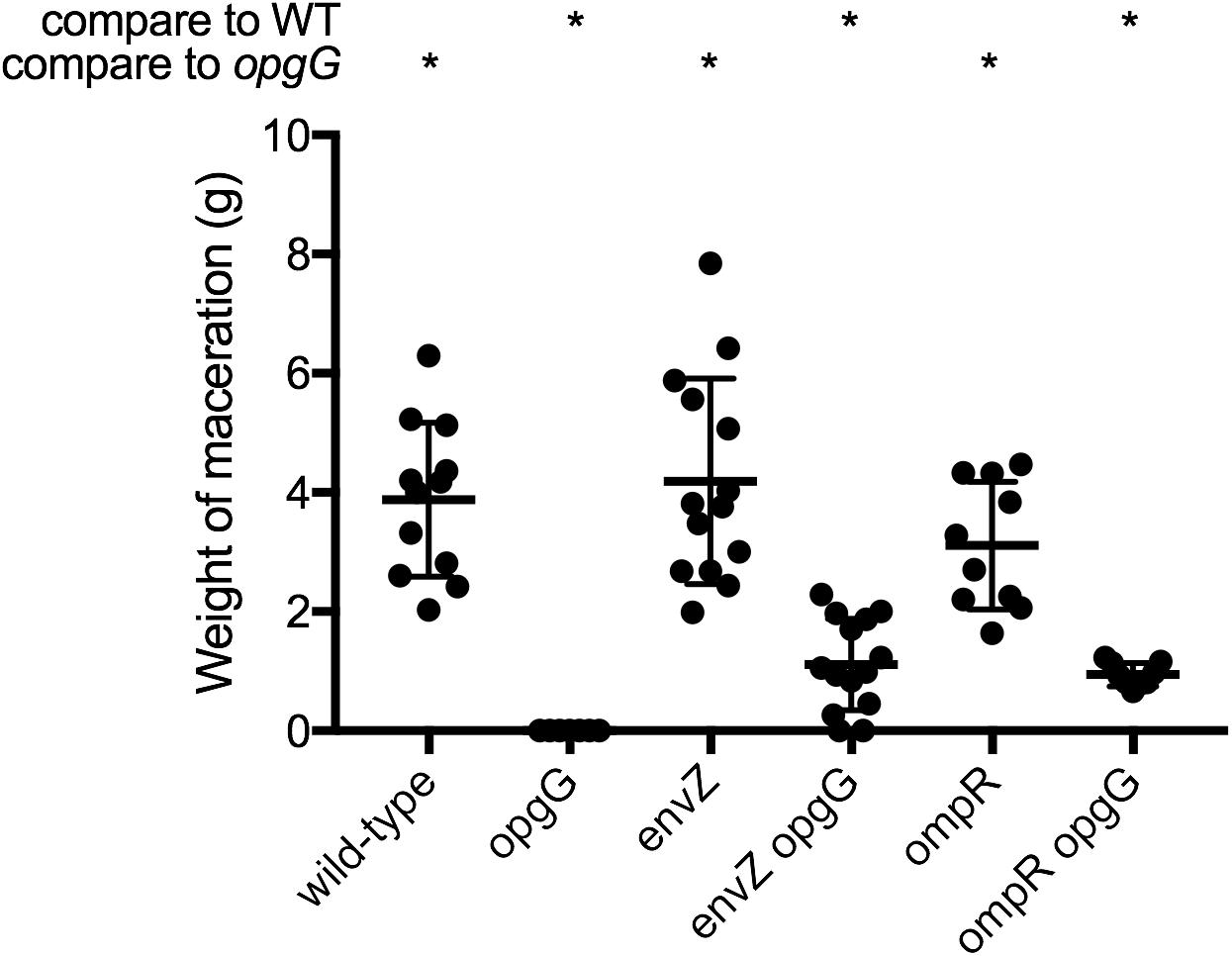
FIGURE 4. Weight of maceration on potato tubers for wild-type, opgG, envZ, envZ opgG, ompR, and ompR opgG strains. Bacteria were inoculated into holes on potato tubers. Maceration (g) was weighed after 72 h of incubation at 30°C. Data represent mean ± standard deviation of at least 10 independent experiments. An asterisk indicates a significant difference with p < 0.0001.
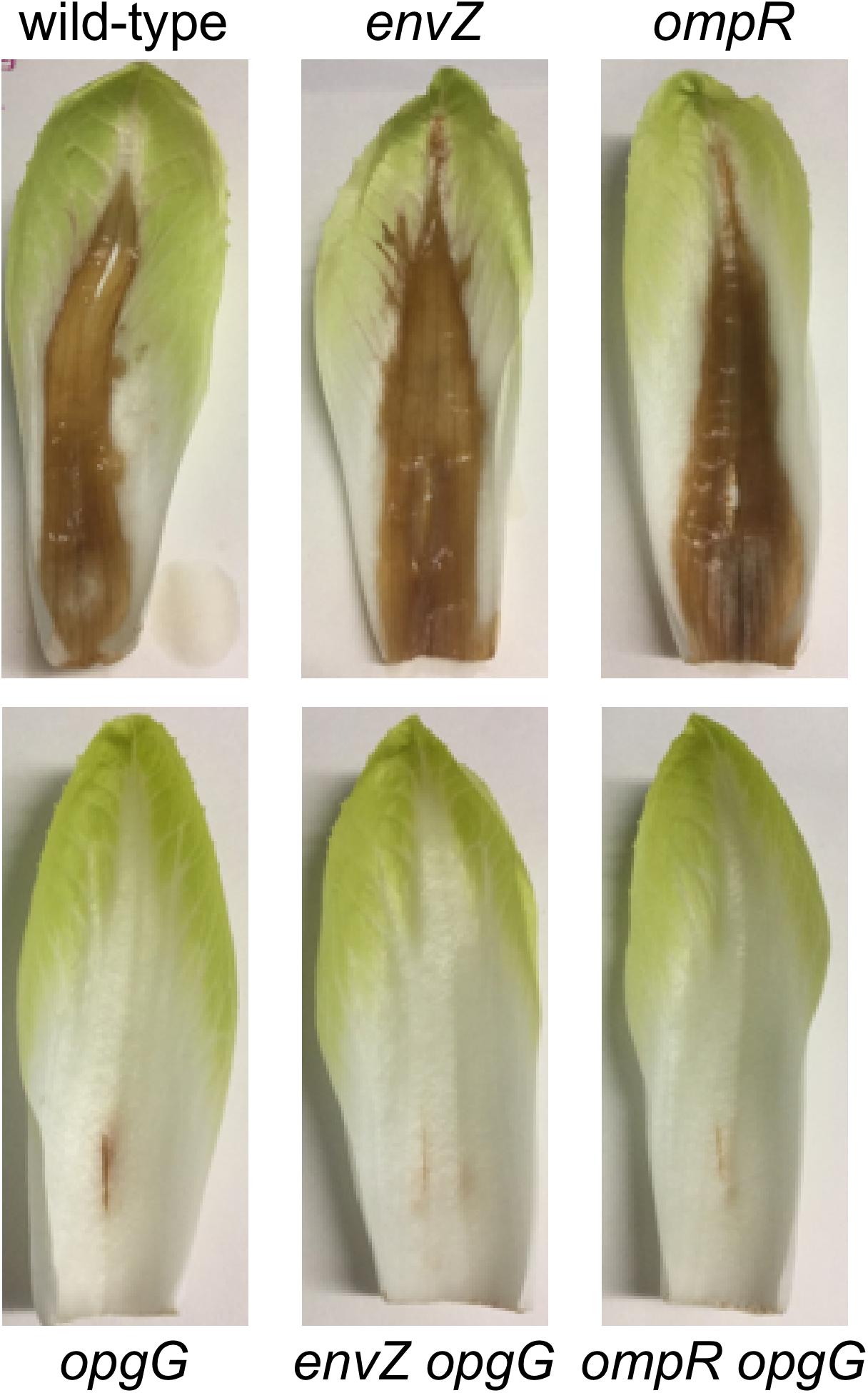
FIGURE 5. Pathogenicity of wild-type, opgG, envZ, envZ opgG, ompR, and ompR opgG strains on chicory leaves. Bacteria were inoculated into scarified chicory leaves. Disease symptoms were observed after 48 h of incubation at 30°C. The results presented are of one of the three independent experiments performed.
ompF and kdgN Are Osmoregulated Through EnvZ-OmpR and Require OPG for Regulation
In D. dadantii, EnvZ-OmpR regulates at least two genes involved in transport – ompF and kdgN (Condemine and Ghazi, 2007). KdgN transports oligosaccharides arising from pectin-mediated degradation during plant infection. OmpF is a porin with a pore diameter of 1.12 nm that allows a non-specific import of hydrophilic metabolites of less than 600 Da. We analyzed the expression of these two genes at 170, 330, 500, and 700 mOsM in a wild-type background (Figures 6A,B). Expression increased 16-fold for ompF and 22-fold for kdgN between 170 and 330 mOsM. Subsequently, the expression decreased twofold for both genes between 330 and 500 mOsM, and twofold for ompF when osmolarity increased to 700 mOsM. In envZ or ompR single mutants, regulation was completely lost showing that ompF and kdgN are part of the regulon (Figures 6A,B). Both genes followed a classic bell curve observed for gene regulation by EnvZ-OmpR in E. coli (Lan and Igo, 1998). Interestingly, in the opgG mutant, regulation was completely lost (Figures 6A,B). At 170 mOsM, the expression level of ompF or kdgN in the OPG-defective strain was at a similar level to the wild-type, regardless of medium osmolarity. These data indicate that the EnvZ-OmpR system regulates the expression of ompF and kdgN in an OPG-dependent manner.
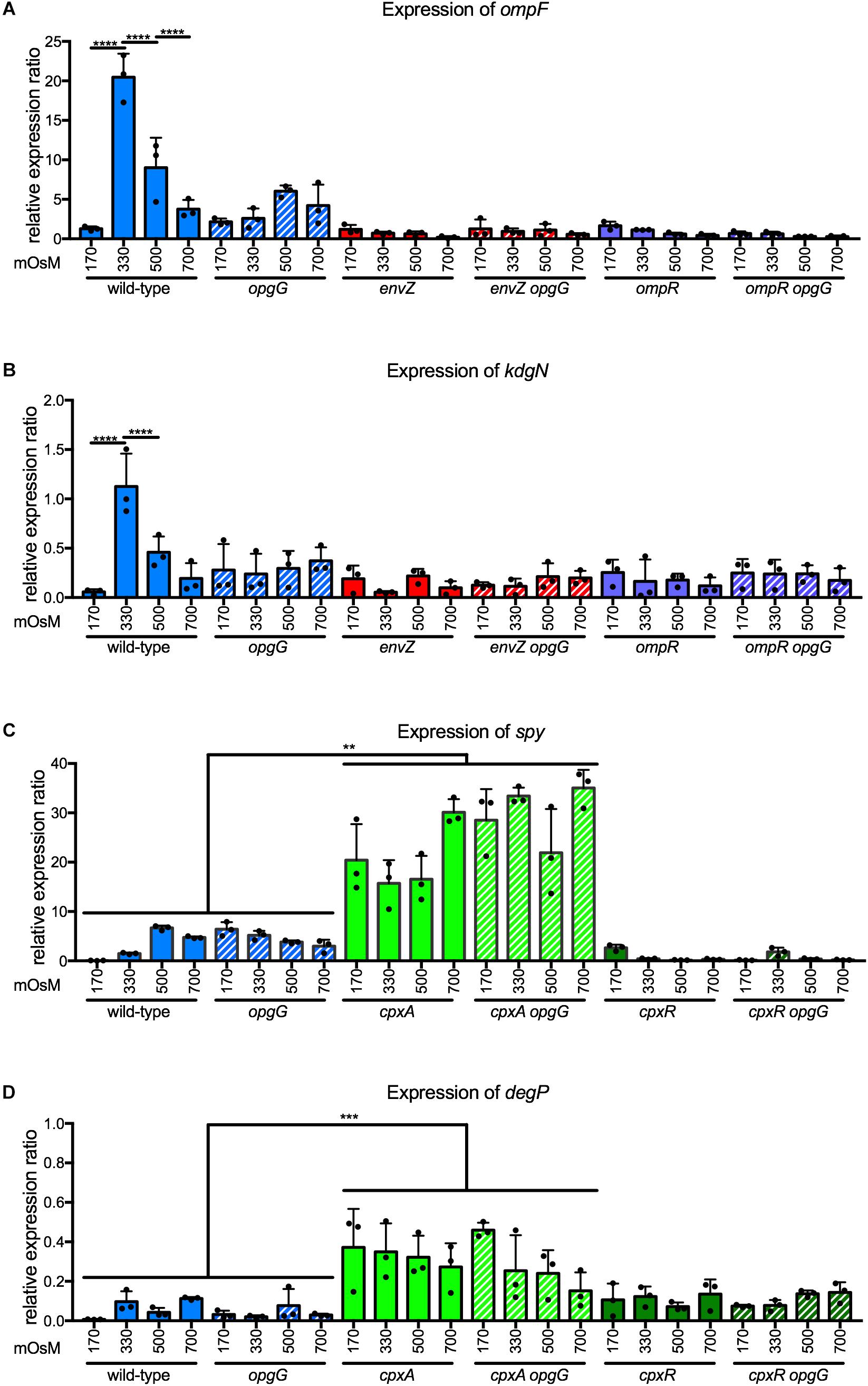
FIGURE 6. Expression of (A) ompF and (B) kdgN in wild-type, opgG, envZ, envZ opgG, ompR, and ompR opgG strains and of (C) spy and (D) degP in wild-type, opgG, cpxA, cpxA opgG, cpxR, and cpxR opgG strains at various osmolarities. Bacteria were grown at 170, 330, 500, and 700 mOsM. The expression of (A) ompF, (B) kdgN (C) spy and (D) degP were analyzed by qPCR. Relative gene expression was calculated using ipxC as a reference (Hommais et al., 2011). Data represent mean ± standard deviation of three independent experiments. An asterisk indicates a significant difference with ∗∗∗∗p < 0.0001, ∗∗∗p < 0.001, and ∗∗p < 0.01.
OPGs Are Not Required for the Activation of the CpxAR Two-Component System
To show whether two-component system dysfunction is a general feature of bacteria lacking OPGs, we investigated the potential relationship between another two-component system and OPGs. Among the 32 two-component systems in D. dadantii, three systems are involved in sensing stress – RcsCDB, EnvZ-OmpR, and CpxAR. CpxAR is involved in the perception of envelope stress (Bontemps-Gallo et al., 2015). Inactivation of this system in an opgG background does not restore any phenotype (Bontemps Gallo, 2013). CpxAR regulates spy, encoding for a periplasmic chaperon, and degP, a periplasmic protease (Bontemps-Gallo et al., 2015). As previously observed, the expression of spy (Figure 6C) and degP (Figure 6D) were up-regulated in a cpxA background. In a cpxR mutant, spy was down-regulated and degP had an expression similar to wild-type (Figures 6C,D; Bontemps-Gallo et al., 2015). Disruption of opgG does not affect the regulation of spy or degP by the CpxAR system (Figures 6C,D). Taken together, our data shows that OPGs have a specific relationship with certain two-component systems.
Periplasmic Size Is Maintained in an OPG-Defective Mutant
Periplasmic size is subject to fluctuations during osmotic stress (Bohin and Lacroix, 2006) and loss of OPGs, and major periplasmic components representing up to 5% of the dry weight of a cell, could affect this size. Recently, Asmar et al. (2017) demonstrated that the activation of two-component systems also relies on the distance between the two membranes. To determine whether a change in periplasm width may be one of the consequences of a lack of EnvZ-OmpR system activation in the opgG mutant, we grew bacteria until mid-log phase in low and high osmolarities and analyzed the cell ultrastructure using transmission electron microscopy (Figure 7). At low osmolarity (Figures 7A,B), the cells exhibited an altered cytoplasmic content with small dense granules being observed. Since poly-phosphate granules, often accumulated by D. dadantii, typically appear white by TEM (Ogawa et al., 2000; Ayraud et al., 2005; Stumpf and Foster, 2005), we suspect that the black granules are filled with ferrous poly-phosphates (Lechaire et al., 2002). This cytoplasmic modification had no effect on the growth of D. dadantii. At high osmolarity (Figure 7), the cell displayed a classic rod-shaped form. Despite the strong structural difference observed for bacteria grown in low and high osmolarities, no significant difference was observed in the bacterial structure between the wild-type and the opgG mutant strains at any osmolarity. In addition, no relevant difference in periplasmic size was observed between the wild-type and the opgG mutant. Both strains displayed an equivalent periplasmic space: 23.99 nm ± 3.26 for wild-type and 22.92 nm ± 3.04 for the OPG-defective strain at low osmolarity and 22.23 nm ± 3.21 for wild-type and 24.28 nm ± 3.41 for the OPG-defective strain at high osmolarity (Figure 7E). This suggests that OPGs are not involved in the control of periplasmic size. These periplasmic space measurements are similar to those observed by Asmar et al. (2017) for the closely related E. coli Enterobacterium in LB medium (around 350 mOsM). Taken together, the gene expression experiments and the microscopy observations strongly suggest that EnvZ-OmpR requires OPGs in the periplasm to be able to sense the osmolarity, but this sensing is not based on periplasmic size.
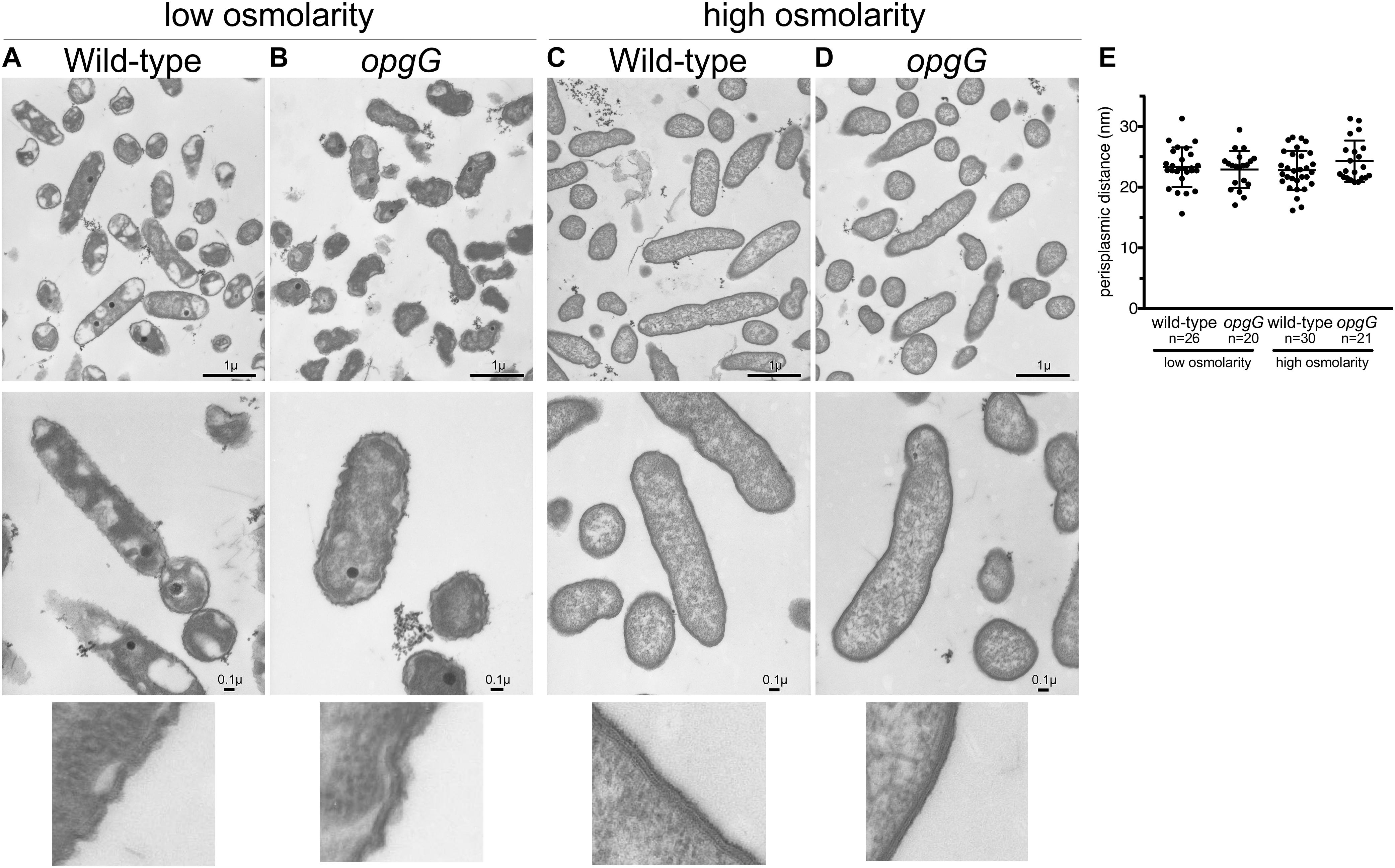
FIGURE 7. Transmission electron microscopy images of wild-type (A,C) and opgG mutant (B,D) at low osmolarity (A,B) and high osmolarity (C,D). Images show similar architecture for both strains when grown in the same medium but differences when osmolarity is varied. (E) Periplasm size (nm) from TEM images.
Increasing Concentrations of OPGs Do Not Affect the Level of EnvZ-OmpR System Activation
Previously, we demonstrated that the level of RcsCDB activation is controlled by the concentration of OPGs (Bontemps-Gallo et al., 2013). Therefore, we examined whether the concentration of OPGs could also modulate the level of EnvZ-OmpR activation (Figure 8). For this, we used a system in which the opgGH operon is under the control of the PBAD promoter from E. coli. Control of L-arabinose concentration enables tight regulation of the opgGH operon (Guzman et al., 1995). We grew the PBAD-opgGH, envZ PBAD-opgGH, ompR PBAD-opgGH, as well as the wild-type and opgG strains, in a M63 medium at various L-arabinose concentrations ranging from 0 to 1 g/L. We first confirmed that the expression of the opgG and opgH genes increased in line with the increasing concentration of L-arabinose (Figures 8A,B). As shown previously, without L-arabinose, no OPG is detected. OPG concentration increased in accordance with the L-arabinose concentration, as described previously (Bontemps-Gallo et al., 2013). We then analyzed the expression of ompF and kdgN in the same strains under the same conditions (Figures 8C,D). Without L-arabinose, the expression of ompF and kdgN in the PBAD-opgGH strain was similar to that measured for the opgG mutant (Figures 6, 8C,D). In the presence of L-arabinose, regardless of the concentration, the expression of both genes was similar to the expression in the wild-type strain (Figures 6, 8C,D). Inactivation of either envZ or ompR in the PBAD-opgGH strain lead to a low expression level regardless of the presence of L-arabinose. Our data show that OPGs are required for the transmission of the sensing signal but they do not control the level of EnvZ-OmpR activation.
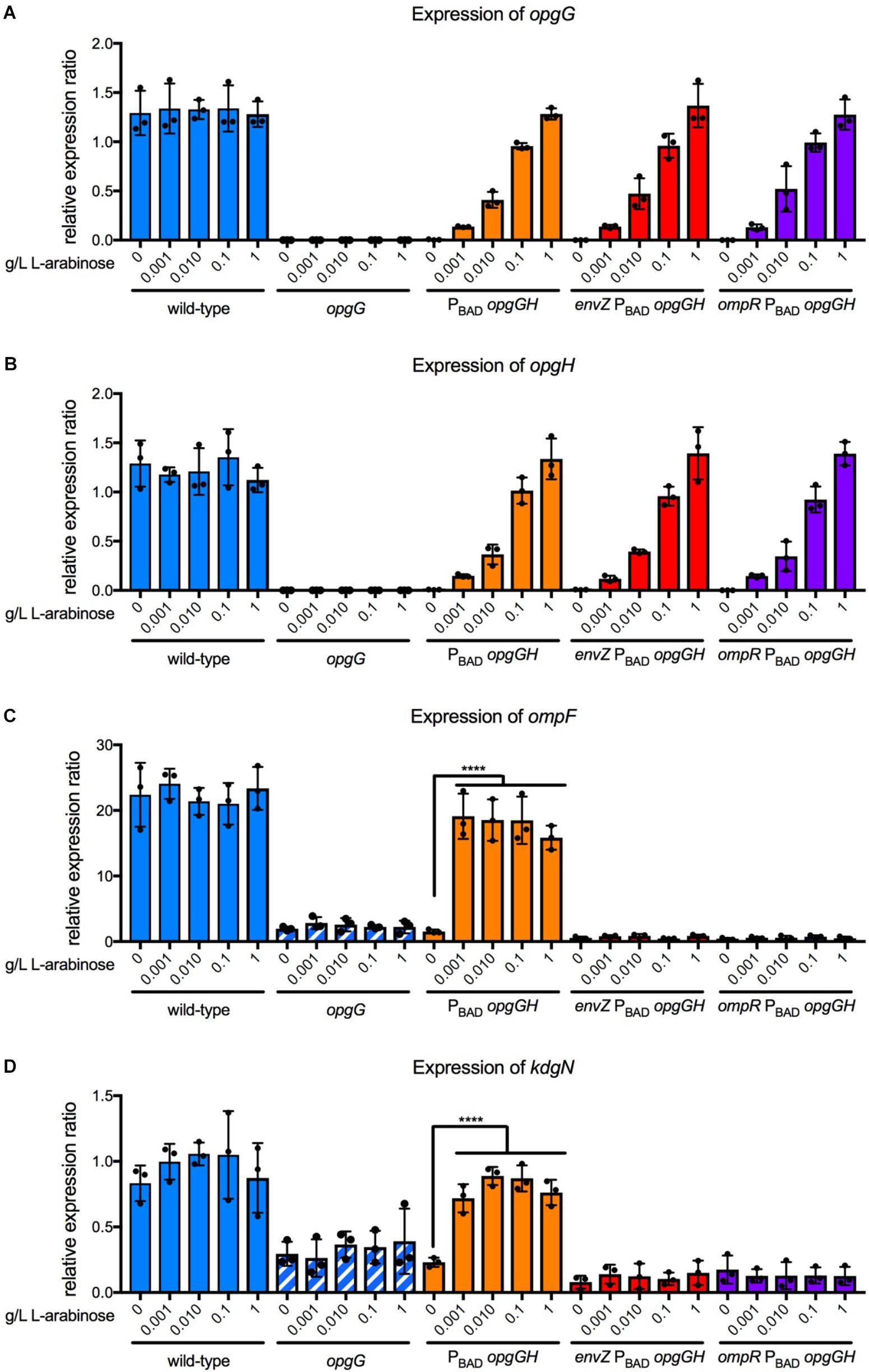
FIGURE 8. Effect of OPG concentration on expression of opgG, opgH, ompF, and kdgN. Bacteria were grown in M63 medium (330 mOsM) with increasing L-arabinose concentration ranging from 0 to 1 g/L. The expression of (A) opgG, (B) opgH, (C) ompF, and (D) kdgN was analyzed by qPCR. Relative gene expression was calculated using ipxC as a reference (Hommais et al., 2011). Data represent mean ± standard deviation of three independent experiments. An asterisk indicates a significant difference with ∗∗∗∗p < 0.0001, ∗∗∗p < 0.001, ∗∗p < 0.01, and ∗p < 0.05.
Discussion
Since their first characterization in 1973 by E.P. Kennedy’s group at Harvard Medical School, the OPGs have been described as playing an important role in osmoprotection (Kennedy, 1982; Lacroix, 1989; Breedveld and Miller, 1994; Cayley et al., 2000; Bontemps-Gallo et al., 2017), in envelope structure (Delcour et al., 1992; Banta et al., 1998; Bontemps-Gallo et al., 2017), in virulence (Bhagwat et al., 2009) as well as in cell signaling (Fiedler and Rotering, 1988; Ebel et al., 1997; Bouchart et al., 2010). Among the different models used to study the biological function of this carbohydrate, D. dadantii is the most developed model for understanding their role in virulence and cell signaling.
The mutant devoid of OPG is described as having a complex pleiotropic phenotype: increased mucoid appearance (Breedveld and Miller, 1994; Ebel et al., 1997; Page et al., 2001), a decrease in motility (Fiedler and Rotering, 1988; Page et al., 2001; Bhagwat et al., 2009), and a loss of virulence (Bontemps-Gallo and Lacroix, 2015). The mucoid appearance of bacterial colonies is the consequence of activation of the RcsCDB two-component system (Bouchart et al., 2010; Bontemps-Gallo et al., 2013). This activation leads to up-regulation of the eps operon (Ebel et al., 1997; Bouchart et al., 2010), the genes of which are responsible for the synthesis of exopolysaccharides. The dramatic decrease in motility is also demonstrated to be a consequence of inactivation of the RcsCDB two-component system (Bouchart et al., 2010; Bontemps-Gallo et al., 2013; Bontemps-Gallo and Lacroix, 2015). Here, we showed that if EnvZ-OmpR is involved in co-regulation of motility, inactivation of the system cannot restore motility in a strain lacking OPGs (Figure 3).
Loss of virulence, certainly the most investigated phenotype, is more complex to explain. Several mutations have now been described that partially [in genes encoding RcsCDB (Bouchart et al., 2010), KdgR, PecT (Bontemps-Gallo et al., 2014)] or fully restore virulence [in the gene encoding PecS (Bontemps-Gallo et al., 2014)] in D. dadantii. Restoration of virulence in potato tubers, the reserve organs, depends only on the ability to restore full production of pectinase (Bontemps-Gallo et al., 2014). Restoration of virulence in non-reserve organs requires restoration of more factors, as bacteria will encounter several plant defense mechanisms (e.g., the oxidative burst) (Reverchon and Nasser, 2013; Bontemps-Gallo et al., 2014). In this study, we showed that inactivation of the EnvZ-OmpR system partially restores virulence in potato tubers (Figure 4) but not in chicory leaves (Figure 5). The result matched with the restoration of the pectinase production (Figure 1).
Finally, the second major finding of this study is the requirement for OPGs for the activation of the EnvZ-OmpR system. In E. coli, the EnvZ-OmpR system senses osmolarity in an unknown manner and modulates the expression of genes necessary for adaptation to the new conditions (Forst and Roberts, 1994; Castillo-Keller et al., 2006). This system is characterized both as a repressor (high osmolarity) and as an activator (low osmolarity) of ompF in E. coli (Lan and Igo, 1998). Surprisingly, in D. dadantii, the EnvZ-OmpR system only acts as an activator (Figure 6). This activation required OPGs in the periplasm (Figure 6). In contrast to RcsCDB (Bontemps-Gallo et al., 2013), periplasmic OPG concentration does not affect the level of activation of the EnvZ-OmpR system (Figure 8). The relationship between EnvZ-OmpR and OPGs is most likely indirect yet specific, since the CpxAR system was not affected by OPGs (Figure 6).
Several questions remain and require further investigations. Do other two-component systems need OPGs to be functional in D. dadantii? Preliminary data from our laboratory suggests that, among the 32 two-component systems, only RcsCDB and EnvZ-OmpR activation is affected by OPG presence/concentration. Does the specific relationship between the RcsCDB or EnvZ-OmpR system and OPGs also exist in phylogenetically closely related bacterial species? In non-pathogenic E. coli, inactivation of RcsCDB or EnvZ-OmpR restores motility in an opgG mutant (Fiedler and Rotering, 1988; Girgis et al., 2007). In Salmonella enterica serovar Typhimurium, inactivation of RcsCDB restores motility but not virulence in mice (Kannan et al., 2009). However, the relationship between OPGs and two-component systems has not been investigated in other bacteria. Finally, the more intriguing feature is the mechanism(s) by which OPGs modulate two-component system activation.
Author Contributions
SB-G and J-ML conceived and designed the study, and wrote the manuscript. MC and SB-G performed all experiments with the assistance of EM, PG, and BD. MC, SB-G, EM, and J-ML analyzed the data.
Funding
This study was supported by the Centre National de la Recherche Scientifique (CNRS), the Université de Lille Sciences et Technologies, and the Ministère de l’Enseignement Supérieur et de la Recherche. MC and SB-G were funded by a doctoral fellowship of University of Lille. The funding bodies were not involved in the study design, data collection and analysis, decision to publish, or preparation of the manuscript.
Conflict of Interest Statement
The authors declare that the research was conducted in the absence of any commercial or financial relationships that could be construed as a potential conflict of interest.
Acknowledgments
We thank Loic Brunet for the electronic microscopy. We are grateful to Dr. Ciaran Finn for the manuscript improvements.
Supplementary Material
The Supplementary Material for this article can be found online at: https://www.frontiersin.org/articles/10.3389/fmicb.2018.02459/full#supplementary-material
References
Asmar, A. T., Ferreira, J. L., Cohen, E. J., Cho, S. H., Beeby, M., Hughes, K. T., et al. (2017). Communication across the bacterial cell envelope depends on the size of the periplasm. PLoS Biol. 15:e2004303. doi: 10.1371/journal.pbio.2004303
Ayraud, S., Janvier, B., Labigne, A., Ecobichon, C., Burucoa, C., and Fauchere, J. L. (2005). Polyphosphate kinase: a new colonization factor of Helicobacter pylori. FEMS Microbiol. Lett. 243, 45–50. doi: 10.1016/j.femsle.2004.11.040
Banta, L. M., Bohne, J., Lovejoy, S. D., and Dostal, K. (1998). Stability of the Agrobacterium tumefaciens VirB10 protein is modulated by growth temperature and periplasmic osmoadaption. J. Bacteriol. 180, 6597–6606.
Barbieri, C. M., Wu, T., and Stock, A. M. (2013). Comprehensive analysis of OmpR phosphorylation, dimerization, and DNA binding supports a canonical model for activation. J. Mol. Biol. 425, 1612–1626. doi: 10.1016/j.jmb.2013.02.003
Barker, C. S., Pruss, B. M., and Matsumura, P. (2004). Increased motility of Escherichia coli by insertion sequence element integration into the regulatory region of the flhD operon. J. Bacteriol. 186, 7529–7537. doi: 10.1128/JB.186.22.7529-7537.2004
Bertani, G. (2004). Lysogeny at mid-twentieth century: P1, P2, and other experimental systems. J. Bacteriol. 186, 595–600. doi: 10.1128/jb.186.3.595-600.2004
Bhagwat, A. A., Jun, W., Liu, L., Kannan, P., Dharne, M., Pheh, B., et al. (2009). Osmoregulated periplasmic glucans of Salmonella enterica serovar Typhimurium are required for optimal virulence in mice. Microbiology 155(Pt 1), 229–237. doi: 10.1099/mic.0.023747-0
Bohin, J. P., and Lacroix, J. M. (2006). “Osmoregulation in the periplasm,” in The Periplasm, ed. Dr. M. Ehrmann (Washington, DC: American Society for Microbiology), 325–341.
Bontemps Gallo, S. (2013). Dickeya dadantii: Towards the Understanding of the Biological Role of the Osmoregulated Periplasmic Glucans (in French). Ph.D. thesis, Université de Lille, Roubaix.
Bontemps-Gallo, S., Bohin, J. P., and Lacroix, J. M. (2017). Osmoregulated periplasmic glucans. EcoSal Plus 7. doi: 10.1128/ecosalplus.ESP-0001-2017
Bontemps-Gallo, S., and Lacroix, J. M. (2015). New insights into the biological role of the osmoregulated periplasmic glucans in pathogenic and symbiotic bacteria. Environ. Microbiol. Rep. 7, 690–697. doi: 10.1111/1758-2229.12325
Bontemps-Gallo, S., Madec, E., Dondeyne, J., Delrue, B., Robbe-Masselot, C., Vidal, O., et al. (2013). Concentration of osmoregulated periplasmic glucans (OPGs) modulates the activation level of the RcsCD RcsB phosphorelay in the phytopathogen bacteria Dickeya dadantii. Environ. Microbiol. 15, 881–894. doi: 10.1111/1462-2920.12054
Bontemps-Gallo, S., Madec, E., and Lacroix, J. M. (2014). Inactivation of pecS restores the virulence of mutants devoid of osmoregulated periplasmic glucans in the phytopathogenic bacterium Dickeya dadantii. Microbiology 160(Pt 4), 766–777. doi: 10.1099/mic.0.074484-0
Bontemps-Gallo, S., Madec, E., and Lacroix, J. M. (2015). The two-component system CpxAR is essential for virulence in the phytopathogen bacteria Dickeya dadantii EC3937. Environ. Microbiol. 17, 4415–4428. doi: 10.1111/1462-2920.12874
Bontemps-Gallo, S., Madec, E., Robbe-Masselot, C., Souche, E., Dondeyne, J., and Lacroix, J. M. (2016). The opgC gene is required for OPGs succinylation and is osmoregulated through RcsCDB and EnvZ/OmpR in the phytopathogen Dickeya dadantii. Sci. Rep. 6:19619. doi: 10.1038/srep19619
Bouchart, F., Boussemart, G., Prouvost, A. F., Cogez, V., Madec, E., Vidal, O., et al. (2010). The virulence of a Dickeya dadantii 3937 mutant devoid of osmoregulated periplasmic glucans is restored by inactivation of the RcsCD-RcsB phosphorelay. J. Bacteriol. 192, 3484–3490. doi: 10.1128/JB.00143-10
Bouchart, F., Delangle, A., Lemoine, J., Bohin, J. P., and Lacroix, J. M. (2007). Proteomic analysis of a non-virulent mutant of the phytopathogenic bacterium Erwinia chrysanthemi deficient in osmoregulated periplasmic glucans: change in protein expression is not restricted to the envelope, but affects general metabolism. Microbiology 153(Pt 3), 760–767. doi: 10.1099/mic.0.2006/000372-0
Breedveld, M. W., and Miller, K. J. (1994). Cyclic beta-glucans of members of the family Rhizobiaceae. Microbiol. Rev. 58, 145–161.
Castillo-Keller, M., Vuong, P., and Misra, R. (2006). Novel mechanism of Escherichia coli porin regulation. J. Bacteriol. 188, 576–586. doi: 10.1128/JB.188.2.576-586.2006
Cayley, D. S., Guttman, H. J., and Record, M. T. Jr. (2000). Biophysical characterization of changes in amounts and activity of Escherichia coli cell and compartment water and turgor pressure in response to osmotic stress. Biophys. J. 78, 1748–1764. doi: 10.1016/S0006-3495(00)76726-9
Charkowski, A., Blanco, C., Condemine, G., Expert, D., Franza, T., Hayes, C., et al. (2012). The role of secretion systems and small molecules in soft-rot Enterobacteriaceae pathogenicity. Annu. Rev. Phytopathol. 50, 425–449. doi: 10.1146/annurev-phyto-081211-173013
Clemmer, K. M., and Rather, P. N. (2007). Regulation of flhDC expression in Proteus mirabilis. Res. Microbiol. 158, 295–302. doi: 10.1016/j.resmic.2006.11.010
Collmer, A., and Keen, N. T. (1986). The role of pectic enzymes in plant pathogenesis. Annu. Rev. Phytopathol. 24, 383–409. doi: 10.1146/annurev.py.24.090186.002123
Condemine, G., and Ghazi, A. (2007). Differential regulation of two oligogalacturonate outer membrane channels, KdgN and KdgM, of Dickeya dadantii (Erwinia chrysanthemi). J. Bacteriol. 189, 5955–5962. doi: 10.1128/JB.00218-07
Cowan, S. W., Schirmer, T., Rummel, G., Steiert, M., Ghosh, R., Pauptit, R. A., et al. (1992). Crystal structures explain functional properties of two E. coli porins. Nature 358, 727–733. doi: 10.1038/358727a0
Delcour, A. H., Adler, J., Kung, C., and Martinac, B. (1992). Membrane-derived oligosaccharides (MDO’s) promote closing of an E. coli porin channel. FEBS Lett. 304, 216–220. doi: 10.1016/0014-5793(92)80622-N
Ebel, W., Vaughn, G. J., Peters, H. K. III, and Trempy, J. E. (1997). Inactivation of mdoH leads to increased expression of colanic acid capsular polysaccharide in Escherichia coli. J. Bacteriol. 179, 6858–6861. doi: 10.1128/jb.179.21.6858-6861.1997
Egger, L. A., Park, H., and Inouye, M. (1997). Signal transduction via the histidyl-aspartyl phosphorelay. Genes Cells 2, 167–184. doi: 10.1046/j.1365-2443.1997.d01-311.x
EPPO (1982). Data Sheets on Quarantine Organisms, Erwinia chrysanthemi Burkholder. Paris: Bull OEPP.
EPPO (1988). A1 and A2 Lists of Quarantine Pests. Specific Quarantine Requirements. Paris: EPPO/EPPO Publications Series B no. 92.
Fiedler, W., and Rotering, H. (1988). Properties of Escherichia coli mutants lacking membrane-derived oligosaccharides. J. Biol. Chem. 263, 14684–14689.
Forst, S. A., and Roberts, D. L. (1994). Signal transduction by the EnvZ-OmpR phosphotransfer system in bacteria. Res. Microbiol. 145, 363–373. doi: 10.1016/0923-2508(94)90083-3
Gao, H., Zhang, Y., Han, Y., Yang, L., Liu, X., Guo, Z., et al. (2011). Phenotypic and transcriptional analysis of the osmotic regulator OmpR in Yersinia pestis. BMC Microbiol. 11:39. doi: 10.1186/1471-2180-11-39
Girgis, H. S., Liu, Y., Ryu, W. S., and Tavazoie, S. (2007). A comprehensive genetic characterization of bacterial motility. PLoS Genet. 3, 1644–1660. doi: 10.1371/journal.pgen.0030154
Groisman, E. A. (2016). Feedback control of two-component regulatory systems. Annu. Rev. Microbiol. 70, 103–124. doi: 10.1146/annurev-micro-102215-095331
Guzman, L. M., Belin, D., Carson, M. J., and Beckwith, J. (1995). Tight regulation, modulation, and high-level expression by vectors containing the arabinose PBAD promoter. J. Bacteriol. 177, 4121–4130. doi: 10.1128/jb.177.14.4121-4130.1995
Hoch, J. A. (2000). Two-component and phosphorelay signal transduction. Curr. Opin. Microbiol. 3, 165–170. doi: 10.1016/S1369-5274(00)00070-9
Hommais, F., Zghidi-Abouzid, O., Oger-Desfeux, C., Pineau-Chapelle, E., Van Gijsegem, F., Nasser, W., et al. (2011). lpxC and yafS are the most suitable internal controls to normalize real time RT-qPCR expression in the phytopathogenic bacteria Dickeya dadantii. PLoS One 6:e20269. doi: 10.1371/journal.pone.0020269
Jiang, X., Zghidi-Abouzid, O., Oger-Desfeux, C., Hommais, F., Greliche, N., Muskhelishvili, G., et al. (2016). Global transcriptional response of Dickeya dadantii to environmental stimuli relevant to the plant infection. Environ. Microbiol. 18, 3651–3672. doi: 10.1111/1462-2920.13267
Kannan, P., Dharne, M., Smith, A., Karns, J., and Bhagwatt, A. A. (2009). Motility revertants of opgGH mutants of Salmonella enterica serovar Typhimurium remain defective in mice virulence. Curr. Microbiol. 59, 641–645. doi: 10.1007/s00284-009-9486-8
Kennedy, E. P. (1982). Osmotic regulation and the biosynthesis of membrane-derived oligosaccharides in Escherichia coli. Proc. Natl. Acad. Sci. U.S.A. 79, 1092–1095. doi: 10.1073/pnas.79.4.1092
Kennedy, E. P. (1996). “Membrane derived oligosaccharides (periplasmic beta-D-glucans) of Escherichia coli,” in Escherichia coli and Salmonella. Cellular and Molecular Biology, 2nd Edn, eds F. C. Neidhardt and H. E. Umbarger (Washington, D.C: ASM Press), 1064–1074.
Lacroix, J.-M. (1989). Etude Génétique et Physiologique de la Régulation Osmotique de la Biosynthèse du MDO Chez Escherichia coli. PhD thesis, Université de Paris-Sud, Orsay.
Lan, C. Y., and Igo, M. M. (1998). Differential expression of the OmpF and OmpC porin proteins in Escherichia coli K-12 depends upon the level of active OmpR. J. Bacteriol. 180, 171–174.
Lechaire, J. P., Shillito, B., Frebourg, G., and Gaill, F. (2002). Elemental characterization of microorganism granules by EFTEM in the tube wall of a deep-sea vent invertebrate. Biol. Cell 94, 243–249. doi: 10.1016/S0248-4900(02)01199-1
Lee, C., and Park, C. (2013). Mutations upregulating the flhDC operon of Escherichia coli K-12. J. Microbiol. 51, 140–144. doi: 10.1007/s12275-013-2212-z
Leonard, S., Hommais, F., Nasser, W., and Reverchon, S. (2017). Plant-phytopathogen interactions: bacterial responses to environmental and plant stimuli. Environ. Microbiol. 19, 1689–1716. doi: 10.1111/1462-2920.13611
Li, W., Ancona, V., and Zhao, Y. (2014). Co-regulation of polysaccharide production, motility, and expression of type III secretion genes by EnvZ/OmpR and GrrS/GrrA systems in Erwinia amylovora. Mol. Genet. Genomics 289, 63–75. doi: 10.1007/s00438-013-07904
Miller, J. H. (1992). A Short Course in Bacterial Genetics: a Laboratory Manual and Handbook for Escherichia coli and Related Bacteria. New York, NY: Cold Spring Harbor Laboratory Press.
Ogawa, N., Tzeng, C. M., Fraley, C. D., and Kornberg, A. (2000). Inorganic polyphosphate in Vibrio cholerae: genetic, biochemical, and physiologic features. J. Bacteriol. 182, 6687–6693. doi: 10.1128/JB.182.23.6687-6693.2000
Page, F., Altabe, S., Hugouvieux-Cotte-Pattat, N., Lacroix, J. M., Robert-Baudouy, J., and Bohin, J. P. (2001). Osmoregulated periplasmic glucan synthesis is required for Erwinia chrysanthemi pathogenicity. J. Bacteriol. 183, 3134–3141. doi: 10.1128/JB.183.10.3134-3141.2001
Pruss, B. M. (2017). Involvement of two-component signaling on bacterial motility and biofilm development. J. Bacteriol. 199:e00259-17. doi: 10.1128/JB.00259-17
Raczkowska, A., Skorek, K., Bielecki, J., and Brzostek, K. (2011). OmpR controls Yersinia enterocolitica motility by positive regulation of flhDC expression. Antonie Van Leeuwenhoek 99, 381–394. doi: 10.1007/s10482-010-9503-8
Reboul, A., Lemaitre, N., Titecat, M., Merchez, M., Deloison, G., Ricard, I., et al. (2014). Yersinia pestis requires the 2-component regulatory system OmpR-EnvZ to resist innate immunity during the early and late stages of plague. J. Infect. Dis. 210, 1367–1375. doi: 10.1093/infdis/jiu274
Resibois, A., Colet, M., Faelen, M., Schoonejans, E., and Toussaint, A. (1984). phiEC2, a new generalized transducing phage of Erwinia chrysanthemi. Virology 137, 102–112. doi: 10.1016/0042-6822(84)90013-8
Reverchon, S., Muskhelisvili, G., and Nasser, W. (2016). Virulence program of a bacterial plant pathogen: the Dickeya model. Prog. Mol. Biol. Transl. Sci. 142, 51–92. doi: 10.1016/bs.pmbts.2016.05.005
Reverchon, S., and Nasser, W. (2013). Dickeya ecology, environment sensing and regulation of virulence programme. Environ. Microbiol. Rep. 5, 622–636. doi: 10.1111/1758-2229.12073
Stumpf, J. D., and Foster, P. L. (2005). Polyphosphate kinase regulates error-prone replication by DNA polymerase IV in Escherichia coli. Mol. Microbiol. 57, 751–761. doi: 10.1111/j.1365-2958.2005.04724.x
Tipton, K. A., and Rather, P. N. (2016). An ompR/envZ two-component system ortholog regulates phase variation, osmotic tolerance, motility, and virulence in Acinetobacter baumannii strain AB5075. J. Bacteriol. 199:e00705-16. doi: 10.1128/JB.00705-16
Keywords: EnvZ/OmpR, osmoregulated periplasmic glucans, osmotic stress, plant pathogen, D. dadantii
Citation: Caby M, Bontemps-Gallo S, Gruau P, Delrue B, Madec E and Lacroix J-M (2018) The EnvZ-OmpR Two-Component Signaling System Is Inactivated in a Mutant Devoid of Osmoregulated Periplasmic Glucans in Dickeya dadantii. Front. Microbiol. 9:2459. doi: 10.3389/fmicb.2018.02459
Received: 07 June 2018; Accepted: 25 September 2018;
Published: 30 October 2018.
Edited by:
Ivan Mijakovic, Chalmers University of Technology, SwedenReviewed by:
Fei Gan, University of California, Berkeley, United StatesSabina Zoledowska, University of Gdańsk, Poland
Copyright © 2018 Caby, Bontemps-Gallo, Gruau, Delrue, Madec and Lacroix. This is an open-access article distributed under the terms of the Creative Commons Attribution License (CC BY). The use, distribution or reproduction in other forums is permitted, provided the original author(s) and the copyright owner(s) are credited and that the original publication in this journal is cited, in accordance with accepted academic practice. No use, distribution or reproduction is permitted which does not comply with these terms.
*Correspondence: Jean-Marie Lacroix, jean-marie.lacroix@univ-lille.fr
†Present address: Sébastien Bontemps-Gallo, Center for Infection and Immunity of Lille, U1019-UMR 8204, Institut Pasteur de Lille, Inserm, CNRS, Université de Lille, Lille, France