- 1Institute of Agricultural Sciences in the Tropics (Hans-Ruthenberg-Institute), University of Hohenheim, Stuttgart, Germany
- 2International Center for Tropical Agriculture (CIAT), Cali, Colombia
The tropical forage grass Brachiaria humidicola (Bh) suppresses the activity of soil nitrifiers through biological nitrification inhibition (BNI). As a result, nitrate () formation and leaching are reduced which is also expected to tighten the soil nitrogen (N) cycle. However, the beneficial relationship between reduced losses and enhanced N uptake due to BNI has not been experimentally demonstrated yet. Nitrification discriminates against the 15N isotope and leads to 15N depleted , but 15N enriched in soils. Leaching of 15N depleted enriches the residual N pool in the soil with 15N. We hypothesized that altered nitrification and leaching due to diverging BNI magnitudes in contrasting Bh genotypes influence soil 15N natural abundance (δ15N), which in turn is reflected in distinct δ15N in Bh shoot biomass. Consequently, high BNI was expected to be reflected in low plant δ15N of Bh. It was our objective to investigate under controlled conditions the link between shoot value of δ15N in several Bh genotypes and leached amounts and shoot N uptake. Additionally, plant 15N and N% was monitored among a wide range of Bh genotypes with contrasting BNI potentials in field plots for 3 years. We measured leaf δ15N of young leaves (regrown after cutback) of Bh and combined it with nitrification rates (NRs) of incubated soil to test whether there is a direct relationship between plant δ15N and BNI. Increased leached was positively correlated with higher δ15N in Bh, whereas the correlation between shoot N uptake and shoot δ15N was inverse. Field cultivation of a wide range of Bh genotypes over 3 years decreased NRs in incubated soil, while shoot δ15N declined and shoot N% increased over time. Leaf δ15N of Bh genotypes correlated positively with NRs of incubated soil. It was concluded that decreasing plant δ15N of Bh genotypes over time reflects the long-term effect of BNI as linked to lower formation and reduced leaching. Accordingly, a low δ15N in Bh shoot tissue verified its potential as indicator of high BNI activity of Bh genotypes.
Introduction
Biological nitrification inhibition (BNI) by the tropical forage grass Brachiaria humidicola (Sylvester-Bradley et al., 1988; Subbarao et al., 2007) is an ecologically evolved trait to compete with nitrifying soil organisms for available ammonium (). Certain root derived exudates (Coskun et al., 2017a), e.g., brachialactone, have been verified to block the activity of ammonia monooxygenase (AMO) and hydroxylamine oxidoreductase (HAO) pathways in nitrifiers such as Nitrosomonas europaea (Subbarao et al., 2009). By preventing the microbial transformation of less soil mobile to more soil mobile nitrate (), BNI is expected to reduce leaching of nitrogen (N) from ecosystems (Subbarao et al., 2009, 2013; Coskun et al., 2017b). Recent research has been undertaken to investigate the BNI effect by Bh on N dynamics, for example on the reduction of nitrous oxide (N2O) emissions from soils (Byrnes et al., 2017), or on the influence of residual BNI effect on N uptake of subsequent crops (Karwat et al., 2017). However, indicators for reduced leaching losses by effective BNI do not exist yet. The reduction of leaching from agroecosystems due to BNI is one of the claimed central features in terms of BNI implementation (Subbarao et al., 2013; Coskun et al., 2017b).
Nitrification and N leaching are two main soil processes that lead to 15N:14N isotope fractionation (Mariotti et al., 1981). This results in a 15N enriched and a 15N depleted pool in soils (Delwiche and Steyn, 1970; Herman and Rundel, 1989; Jones and Dalal, 2017). Under high rainfall conditions, nitrification-derived 15N-depleted is exposed to high leaching, whereby plants then would feed on remaining 15N enriched . In contrast, plants with effective BNI are expected to suppress microbial formation. Consequently, less 15N depleted would be lost from the system. Under both scenarios, Bh feeds mainly on , toward which it has a higher tolerance than other Brachiaria species (Castilla and Jackson, 1991; Rao et al., 1996). The 15N signature of soil mineral N can be reflected in a corresponding shoot 15N signature (Takebayashi et al., 2010). Previous field studies demonstrated that foliar δ15N increased in conjunction with increasing nitrification and N leaching by precipitation (Pardo et al., 2002; Stamatiadis et al., 2006; Huber et al., 2013; Yé et al., 2015). From these studies, the relationship between plant δ15N and soil nitrification and N losses was apparent, which indicates a possible strong relationship between BNI and the δ15N in plant tissue.
What remains unclear is if shoot δ15N of Bh genotypes is linked to plant induced BNI, and if this relates to reduced nitrification, thus reduced leaching. We therefore hypothesized that (i) effective BNI is linked to reduced soil nitrification rates (NRs), enhanced N nutrition, reduced leaching; and that (ii) this link is expressed in respective low δ15N in plant shoot tissue due to nutrition of Bh on a less naturally enriched N pool of 15N. In contrast, it was expected that, respectively, higher NRs and higher leaching is expressed in higher δ15N of shoot biomass due to the uptake of 15N enriched soil N.
Materials and Methods
Experiment 1: Relationship Between δ15N of Bh, N Uptake, and Leaching Under Controlled Conditions
A greenhouse study at the University of Hohenheim (UHOH), Stuttgart, Germany, was implemented as an α-design, i.e., a design with incomplete blocks, that are grouped into complete replicates. The trial contained four complete replicates with two blocks per replicate. The aim was to monitor the effect of N loss and N uptake after N fertilization on the δ15N in shoot biomass of different Bh genotypes. Experimental soil columns were manufactured from PVC-drainpipes (Ø 11 cm × 100 cm) in order to enable deep rooting of Bh and monitoring of dislocation within the soil profile. A fossil tertiary clay loam (collected in Lich, Germany, 50°31′2.0”N, 8°50′55.9”E) with pH 5.7, 36% clay content, 0.25% total C, and 0.025% total N was used. The original soil was amended with sand (25 vol%) in order to improve drainage, and the resulting substrate was filled into the experimental pipes.
Five apomictic Bh hybrids (Bh08 selection: Bh08-1149, Bh08-700, Bh08-675, Bh08-696, Bh08-1253) and two germplasm accessions of Bh (CIAT 16888, CIAT 679 cv. Tully) were used as test genotypes (Rao et al., 2014). Bh stolons were first propagated from a Bh stock collection at UHOH and transferred to a turf-based culture substrate for 3 weeks for root establishment. Then, the young Bh plants were transplanted in August 2014 to the experimental pipes were exposed to supplementary artificial light (photosynthetically active radiation averaging 800 μmol m−2 s−1) for 12 h photoperiod. Day and night temperature regimes were adjusted to 25 and 20°C, respectively. Plants were raised with a basal fertilizer of N-P-K-S (analog to 30-60-150-35 kg ha−1). After the establishment phase of 6 weeks, plants were cut to 2–3 cm height to facilitate regrowth. The experimental phase commenced with application of 150 kg N- ha−1 as (NH4)2SO4 (δ15N = −0.1) to stimulate the growth and activity of soil nitrifiers. Plants were watered every second day (with 100 ml H2O), whereas the amount of water applied was doubled at 8, 19, and 28 days after N fertilization (DANF) in order to increase leaching of derived from nitrification.
Rhizons (Soil Moisture Sampler, Ø 2.3 × 50 mm, porosity 0.1 μm, Eijkelkamp Agrisearch Equipment, Giesbeek, Netherlands) were installed horizontally into the pipes at 7.5 and 50 cm depth. This procedure enabled sampling of soil solution by suction pressure using a common medical syringe. Soil solution sampling (0, 3, 6, 7, 10, 14, and 20 DANF from topsoil and at 0, 18, and 27 DANF from 50 cm depth) was conducted at 3 h after irrigation with 100 ml of tap water to warrant sufficient soil moisture and time for establishing equilibrium for level in the topsoil. A sample of 10 ml of soil solution was collected and frozen immediately. Concentration of in soil solution samples was analyzed by complete reduction to through hydrazine (in alkaline solution, with copper as catalyst) and subsequent reaction with sulfanilamide and Griess reagent (N-(1-Naphthyl)ethylenediamine) to form a pink compound measured photometrically at 550 nm (method DIN38405, ISO/DIS 13395; photometer: AutoAnalyzer 3, QuAAtro AQ2, SEAL Analytical Ltd., Southampton, United Kingdom).
Resin bags (ion-exchange resin in a fine nylon mesh) were installed at the bottom of the experimental pipe to trap leached . This allowed the quantification of cumulative -losses during the experiment. For this purpose, Resinex MX-11 (Jacobi Carbons GmbH, Frankfurt, Germany), a mixed anion/cation exchange resin with a maximum anion sorption capacity of 0.4 eq l−1 was used. Each bag contained 200 ml resin amended with 200 ml of washed sand in order to slow down percolate passage through the coarse resin matrix. The anion traps were removed at the end of the experiment (i.e., at 42 DANF). The resin was thoroughly homogenized and an aliquot of 40 ml resin of each bag was further processed. Extraction of was performed twice with 200 ml of 2 M KCL and extracts were subsequently analyzed photometrically for content (see analysis of percolate samples). A pre-test on the extractability of Resinex MX-11 verified that a twofold extraction was sufficient to achieve extraction rates of 98% of trapped .
At 42 DANF, soil solution sampling showed levels below the detection limit using a fast test (<5 mg L−1). This indicated that the main effect of N fertilization should be reflected in terms of the δ15N and N uptake amount (mg N g plant dry matter−1). The aboveground shoot biomass (cut 3 cm above soil surface) of all seven Bh genotypes was sampled subsequently and oven-dried (3 days 60°C). Dry matter was determined and ground aliquots of plant material were filled into tin capsules (HEKAtech GmbH, Wegberg, Germany).
15N and N% were measured for all plant samples from Experiments 1–3 at UHOH by using a Euro Elemental analyzer coupled to a Finnigan Delta continuous-flow isotope ratio mass spectrometer IRMS (Thermo Scientific, Bremen, Germany). The 15N natural abundance of the sample relative to the standard (atmospheric N2) was expressed as: δ15N‰ = [(Rsample/Rstandard) − 1] × 1000 (‰), where R represents the isotope ratio (15N/14N) and Rstandard is 15N/14N for atmospheric N2 that is 0.0036765 (δ15N‰ = 0) (Robinson, 2001).
Experiment 2: Differences in δ15N Leaf and Shoot of a Wide Range of Bh Genotypes Grown Under Field Conditions
A field trial was established in August 2013 with a range of Bh genotypes with contrasting BNI activity at Corpoica-La Libertad Research Center in the Piedmont region of Colombia (4°03′46” N, 73°27′47” W). The experimental field site was located at an altitude of 338 m above sea level with an annual mean temperature of 21.4°C and an average annual rainfall of 3,685 mm. The soil is classified as an Oxisol with a pH of 4.9, clay content of 42%, total N of 0.11%, and C/N ratio of 12.4. The trial included four out of the five Bh apomictic hybrids used in Experiment 1 and four Bh germplasm accessions. Each main plot had a size of 4 × 4 (16 m2) and received basal fertilization (kg ha−1) in September 2013 of 69 N (urea, δ15N = 0.05), 25 P, 50 K, 50 Ca, 15 Mg, 10 S, 0.5 B, and 2.6 Zn. The second N (100 kg N ha−1 as urea) fertilization (26 October 2015) was conducted after leaf sampling for Experiment 3 to stimulate both, BNI and soil nitrification.
For forage productivity and forage quality evaluation, grasses were cut every 6 weeks from October 2013 to November 2015. Aboveground shoot biomass samples of each plot were oven dried at 60°C. A sub-sample was ground and sent to UHOH for IRMS analysis (see above in Experiment 1).
Experiment 3: δ15N of Young Regrown Bh Leaves Linked to BNI Indicated by Soil Incubation
Two years after initiation of the field trial described for Experiment 2, a substantial BNI effect was expected based on preliminary tests (soil incubation, data not shown). The grass was cut on 5 October 2015 (end of the rainy season) and all plots received maintenance fertilization (kg ha−1) of 40 P, 75 K, 110 Ca, 65 Mg, 19 S, 0.9 B, and 5.3 Zn. No N fertilizer was applied. It was intended that the plants take up soil mineral N and consequently reflect the δ15N of the soil mineral N. To avoid border effects during sampling, one sub-plot (1 m2) per main plot was randomly defined and marked. At 11 days after grass cutting (16 October), recently fully expended (second youngest) leaves from the regrown plants within the sub-plots were collected from the plots of three CIAT accessions as well as from Bh08 hybrids. Oven-dried and ground shoot samples were sent to UHOH for 15N and N% measurement (see above in Experiment 1).
Before cutting the grass in the field trial (Experiment 3) and adding the fertilizer in October 2015, topsoil samples (0–10 cm) were collected with an auger from eight randomly chosen points within each sub-plot. About 500 g of soil per sub-plot was thoroughly mixed, air-dried for 48 h, sieved (2 mm mesh size), and small stones as well as visible root material were removed. Representative sub-samples of 5 g of soil from each plot were filled in small glass tubes followed by application of 1.5 ml ammonium sulfate ((NH4)2SO4) solution as substrate for soil nitrifiers. Tubes were sealed with parafilm that contained two holes for aeration and placed in a dark incubation chamber with constant 25°C and 60% air humidity. Soil was extracted before starting the incubation (basal) and after 5 and 25 days (main active phase of nitrification) with 50 ml of 1 M KCl. concentrations were determined as described above (Experiment 1). NRs were expressed as mg N- kg dry soil−1 day−1.
Statistical Analysis
Statistical analysis was performed using the SAS® version 9.4 (SAS Institute Inc., Cary, NC, United States) with a mixed model approach. The assumptions of homogeneity of variance and normal distribution of residual errors were tested through the plots of studentized residuals vs. predicted value and quantile-quantile-plots, respectively, for all data sets. For the analysis of data from Experiment 2, the following model was set up: Genotype + Year + Rep + Genotype × Year. Block was set as random factor. Model-based least square means were used for data visualization with SigmaPlot for Windows version 12.0 (Copyright Systat Software, Inc.). The same software was also used for correlation analysis conducted for Experiments 1 and 3.
Results
Experiment 1 (Greenhouse at UHOH, Germany)
Plant δ15N at harvest (42 DANF) was found to be negatively correlated with plant N uptake amounts (p < 0.001) (Figure 1A). Furthermore, the relationship between plant δ15N and the amount of cumulative leached (p = 0.018; R2 = 0.16) was moderately positive (Figure 1B). In the case of low N uptake, plant δ15N was high, whereas plants with high N uptake were found to have lower plant δ15N (Figure 1). Increased leaching of -N lead to an increase of the δ15N of remaining N accumulating in the grass shoot biomass (Table 1). Furthermore, plant δ15N of all samples was relatively enriched compared to the δ15N of the applied fertilizer (δ15N = −0.1). A linear regression analysis showed an inverse relationship (p < 0.001; R2 = 0.37) between plant N uptake and leached N (Supplementary Material).
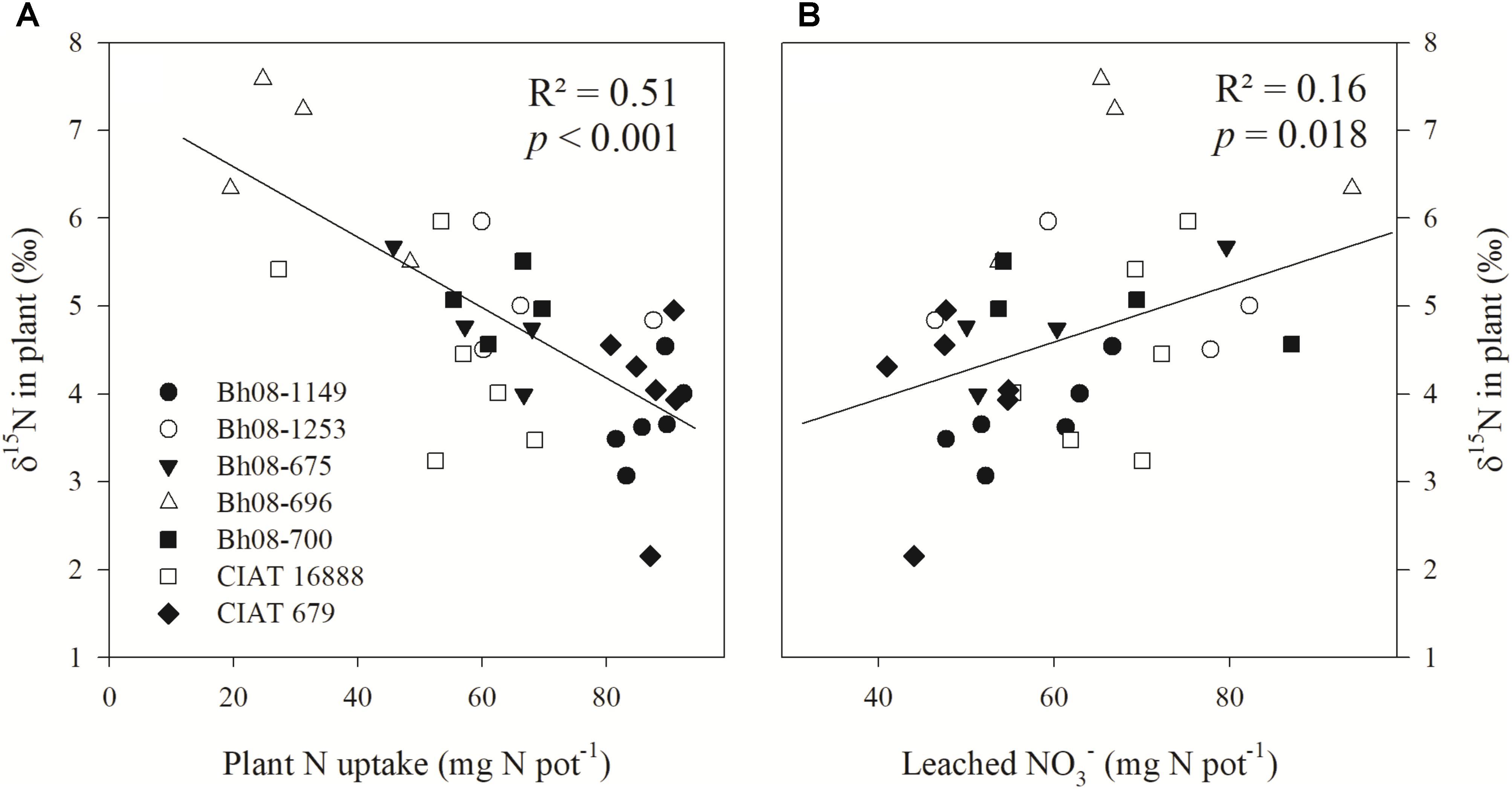
FIGURE 1. Linear regression of plant δ15N (‰) of Brachiaria humidicola (Bh) and plant N uptake (mg N pot−1) (A) and cumulative leached (mg N pot−1) (B). The greenhouse study (Experiment 1) included seven Bh genotypes and was established in August 2014. Plants were sampled at 42 days after fertilization (6 weeks after transplanting to experimental pots) with ()2SO4 (δ15N = –0.1‰). Plant N uptake and leached are cumulative amounts determined at the date of harvest.

TABLE 1. Plant δ15N (‰), plant N uptake (mg N pot−1), and leached N (mg N pot−1) at 42 days after N [()2SO4; δ15N = −0.1‰] fertilization of two Brachiaria humidicola (Bh) CIAT accessions and five Bh08 hybrids.
Experiment 2: Yearly δ15N Monitoring of Bh Genotypes in the Field (Field Trial La Libertad, Colombia)
The overall year effect showed an obvious trend of decreasing plant δ15N over the experimental seasons (p < 0.0001) (Figure 2). One month after experiment establishment and N fertilization (October 2013), most genotypes tended to a plant δ15N of around 7‰, except for the Bh08-1149 hybrid with a δ15N of almost 8‰. One year later (October 2014), δ15N of all genotypes had dropped below 5‰, however, a genotypic effect on δ15N abundance was absent (p = 0.13). At the last sampling (November 2015), δ15N of CIAT 26149 and CIAT 26146 had higher δ15N than the other three CIAT accessions and the two Bh08 hybrids (p = 0.02).
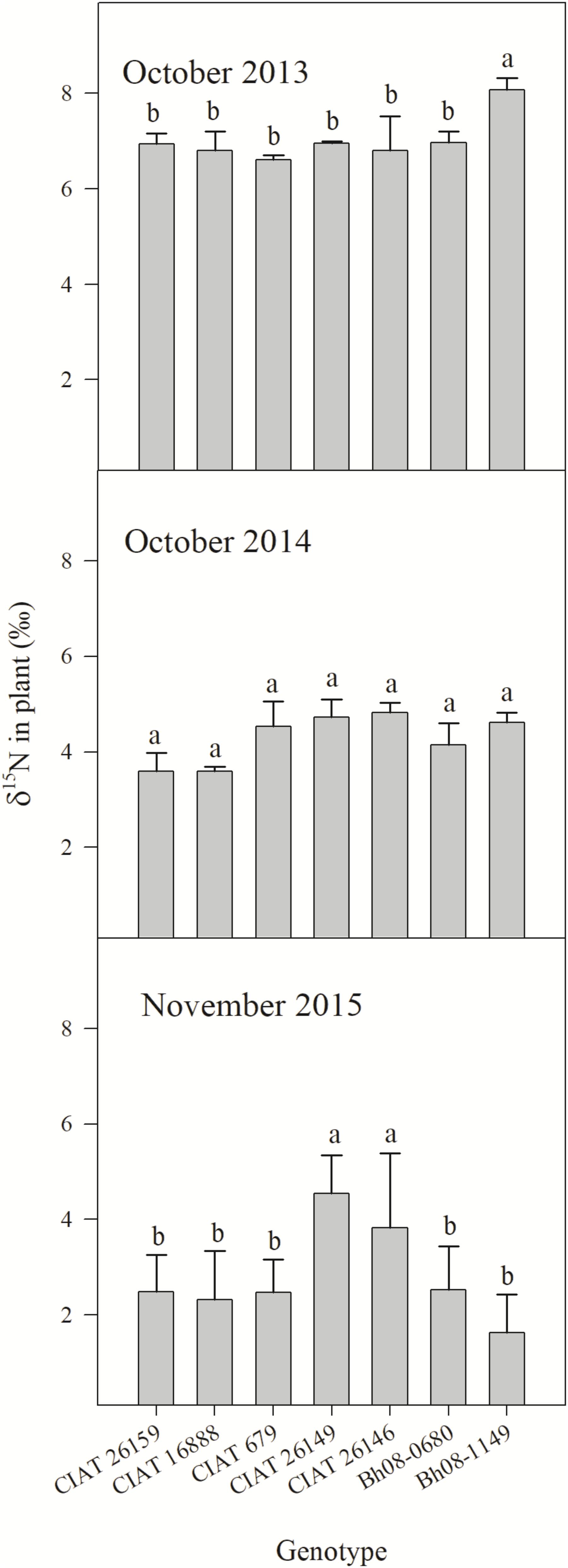
FIGURE 2. Plant δ15N (‰) of B. humidicola (Bh) of five contrasting (in terms of BNI) CIAT accessions and two hybrids (Bh08-population) with unknown BNI capacity sampled after the rainy season in the Colombian Llanos (Experiment 2). The field trail was established in August 2013. N fertilizer was applied as urea (δ15N = 0.05‰) in September 2013 (69 kg N ha−1) and in October 2015 (100 kg N ha−1). Error bars represent the standard error of the mean based on collected plants of three replicated completely randomized field plots. Means that share a common letter within the same year do not differ significantly at the a = 5% level.
To investigate an expected relationship between δ15N and N uptake by Bh, a regression analysis was conducted between the measured plant δ15N and the N concentration (N%) in the respective sampled Bh grass genotypes (Figure 3). A negative correlation (p < 0.001) was observed between plant δ15N and plant N%, indicating that the higher the N status of the plant is the lower the respective δ15N becomes.
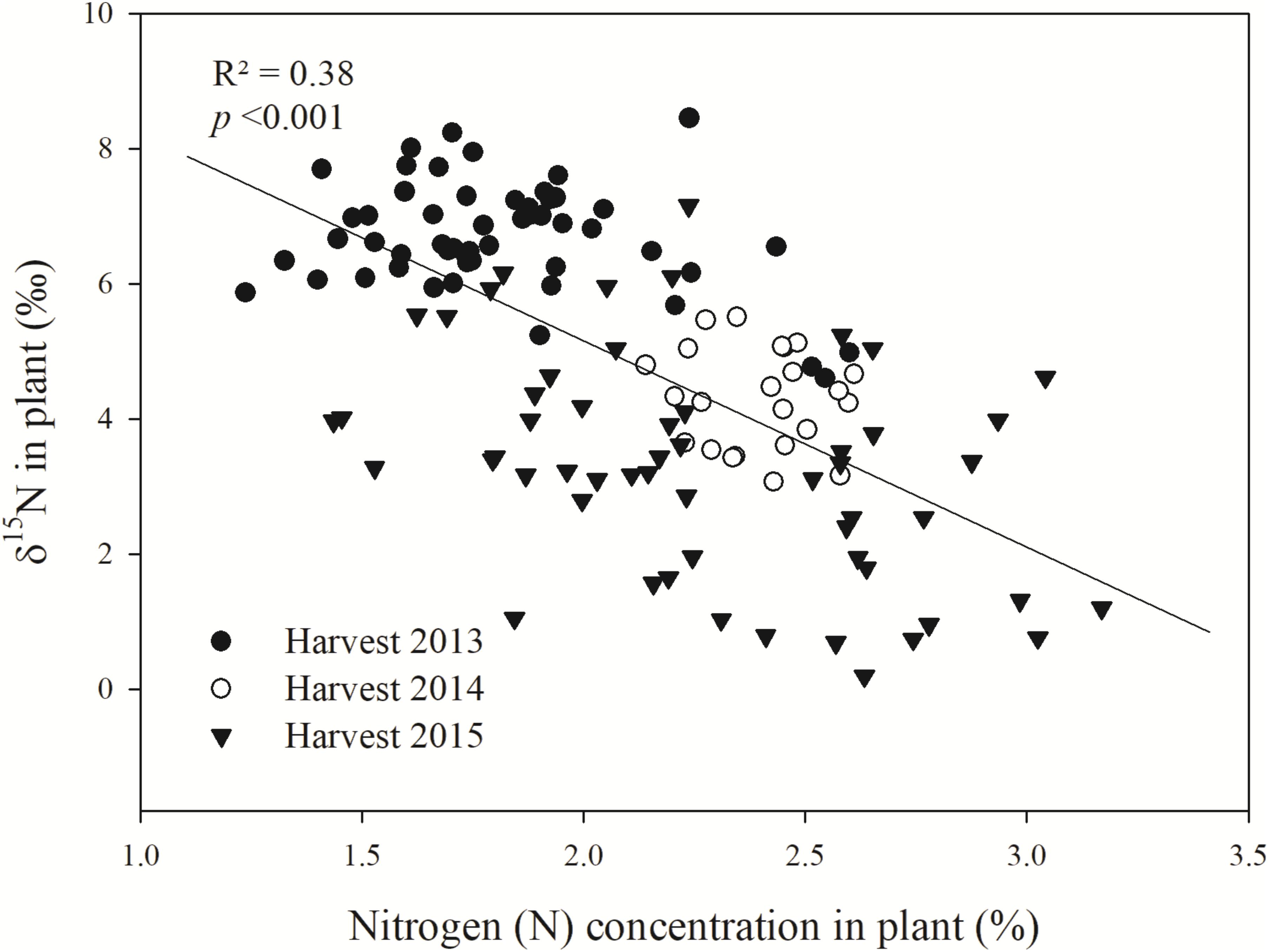
FIGURE 3. Linear regression of plant δ15N (‰) and plant N concentration (%) of contrasting B. humidicola (Bh) genotypes (in terms of BNI) sampled after the rainy season in the Colombian Llanos (Experiment 2). The field trail was established in August 2013. N fertilizer was applied as urea (δ15N = 0.05‰) in September 2013 (69 kg N ha−1) and in October 2015 (100 kg N ha−1).
Experiment 3: Genotypic Leaf δ15N and Relation to Soil Nitrification (Field Trial La Libertad, Colombia)
Means of δ15N values of regrown leaves at 11 days after regrowth (October 2015) of the selected Bh genotypes were positively correlated with the observed NRs of the respective Bh genotypes (p = 0.007) (Figure 4). Leaf δ15N means were found to be different among genotypes (p = 0.001), whereas the genotype effect was not significant for NRs (p = 0.74) (Table 2). In more detail, CIAT 26146 had highest δ15N leaf signal and the corresponding incubated soil showed highest NR. CIAT 16888 and CIAT 679, with reported high BNI, had, compared to CIAT 26146, significantly lower 15N leaf signals and were among the genotypes tested those with lower NRs.
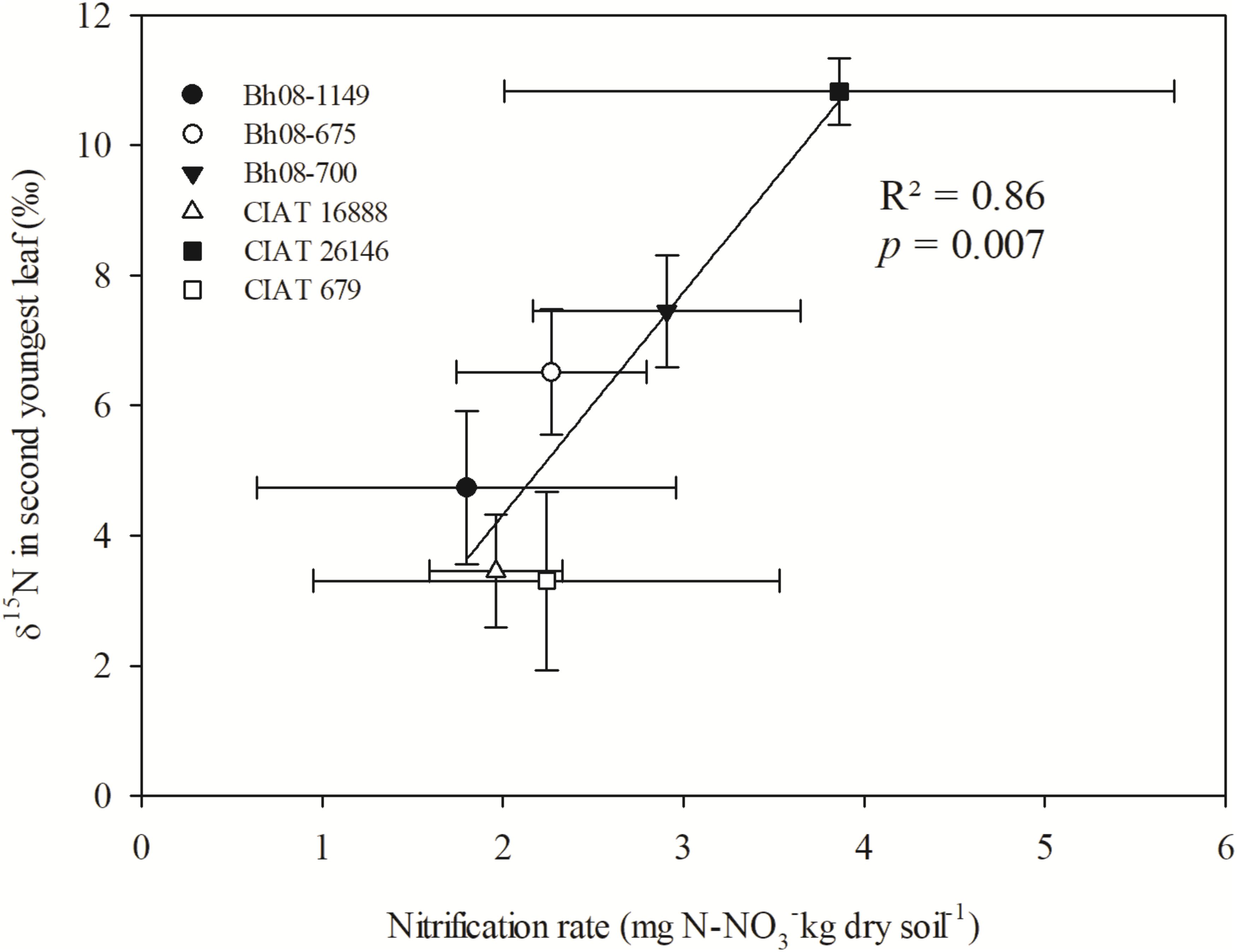
FIGURE 4. Linear regression of leaf δ15N (‰) of B. humidicola (Bh) and NRs (mg N- kg dry soil−1) determined from incubated soil sampled from the respective field plots (Experiment 3). The field trial included Bh CIAT accessions with potential of high BNI (CIAT 16888), mid-high BNI (CIAT 679), and low BNI (CIAT 26146). Additionally, three hybrids (Bh08-population) with unknown BNI capacity were sampled. The field trail was established in August 2013 in the Colombian Llanos. N fertilizer was applied as urea (δ15N = 0.05‰) in September 2013 (69 kg N ha−1). Plants were cut back at 5 October 2015 and topsoil (0–10 cm) samples were collected for incubation in the laboratory. Last fully developed leaves of Bh were sampled at 11 days after regrowth. Error bars represent the standard error of the mean based on collected leaf and soil samples of three replicated completely randomized field plots.
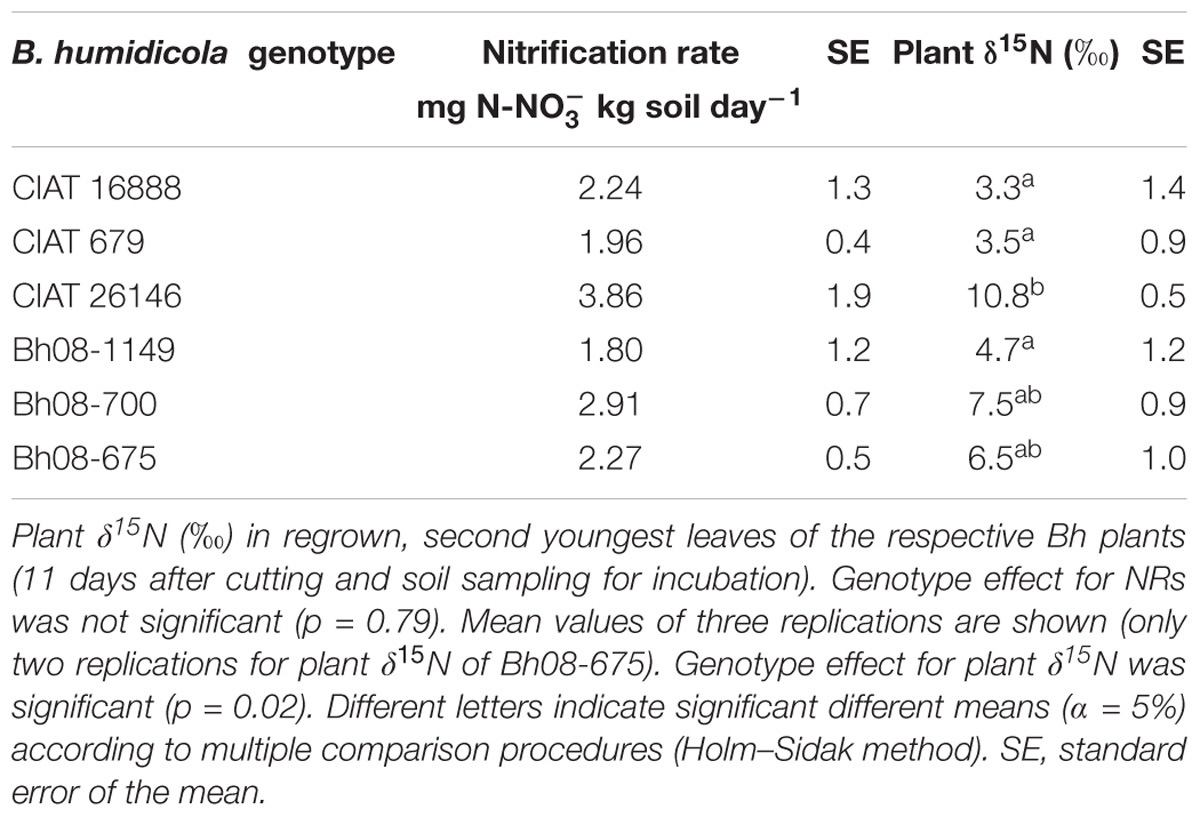
TABLE 2. Nitrification rates (NRs) determined from incubated topsoil (0–20 cm, Oxisol, Colombian Llanos) sampled from field plots (October 2015) of three B. humidicola (Bh) CIAT accessions and three Bh hybrids (Experiment 3).
Discussion
Lower Plant δ15N Is Linked to Reduced Losses and High Plant N Uptake
Our results of the greenhouse study confirmed the hypothesis that low plant δ15N is linked to enhanced N nutrition and reduced leaching. Hence, the altered plant isotopic signals were indirectly linked to isotopic fractionation of 15N:14N between product () and substrate () during bacterial and archaeal nitrification (Delwiche and Steyn, 1970; Shearer et al., 1974). This led to subsequent leaching of the relatively 15N depleted . Our observations (Experiment 1) indicated that with increasing amounts of leached , the δ15N of the Bh grass increased (Figure 1B). These results suggest that losses of 15N depleted resulted in relative enrichment of the plant available N. Similar observations of leaching processes leading to an 15N enrichment of the remaining soil have been made by Pardo et al. (2007), Craine et al. (2009), and Stevenson et al. (2010). Furthermore, our observation of the positive relationship between increasing 15N:14N in vegetation due to increasing nitrification and N losses has also been described for leaves of forest trees (Pardo et al., 2002; Garten et al., 2008), cotton (Stamatiadis et al., 2006), mixed systems such as grass-heath-woodlands (Huber et al., 2013), as well as for comparative studies between perennial and annual grasses (Yé et al., 2015). Furthermore, evaluations at various sites demonstrated lower δ15N of compared to δ15N of in soil due to nitrification (Takebayashi et al., 2010). The yearly field evaluation revealed that 15N signals in Bh decreased with improved N status of the grass over time, indicating increased utilization of plant N as also observed in Central European grasslands by Kleinebecker et al. (2014). We suggest that in our field trial this was caused by decreasing losses from the system due to expanded plant (e.g., root system) growth and development over the years.
Long-Term BNI Effect in the Field Expressed in Low Plant δ15N
The general trend of decreasing plant δ15N of Bh genotypes in the field study over the years indicated that N isotope fractionation and consequently the δ15N of the mineral N in the soil changed over time. Thus, our results confirmed that during the early stage after establishment of the Bh genotypes, there was no significant influence of BNI on microbial nitrification. This observation is consistent with other studies on BNI expression with young Bh plants (Miranda et al., 1994; Castoldi et al., 2013; O’Sullivan et al., 2016). Therefore, it is suggested that applied urea N (hydrolyzed to within a few days) during transplanting had a strong stimulation effect on growth and activity of soil nitrifiers, and that the microbial formed was leached rapidly in the first rainy season when the grass was still small. BNI was unlikely to be strongly expressed, since plants were less than 2 months old and a strong BNI effect, due to an accumulation of BNI substances in the soil, needs about 1 year of Bh establishment (Nuñez et al., 2018). The high δ15N of Bh plants observed in October 2013 could therefore reflect the strong 15N enrichment of the soil mineral N pool caused by substantial nitrification and leaching loss of 15N depleted during the establishment of the trial (Nadelhoffer and Fry, 1994; Song et al., 2014). Plant δ15N after the second rainy season (October 2014) were lower than the first sampling. BNI ability has been shown to be promoted with developing root biomass leading to less nitrification over time (Subbarao et al., 2009). Reduced N losses during the second rainy season explained the lower plant δ15N caused by a lower 15N enrichment of soil mineral N pool. The general tendency of decreasing 15N natural abundance of Bh genotypes over the years was also visible in the third year. This indicated further reduction of formation and loss due to increase of BNI. This was verified by other soil incubation studies (Arango et al., unpublished data) revealing low NRs during the second (2014) rainy season. In contrast, incubation of soil sampled during the third rainy season (2015) evidenced significantly lower average NRs (3.5 mg N- kg dry soil−1 day−1). However, since root systems expand over the years a general higher uptake of is expected. This could, additionally to BNI substance release, increase the competiveness of Bh for and indirectly reduce nitrifier activity.
Link Between High BNI and Low Leaf δ15N of Bh Genotypes
In our study, we linked leaf δ15N to BNI by Bh. It was evident that low nitrification in incubated soil taken from plots, where Bh was cultivated for more than 2 years, correlated with lower leaf δ15N. Exudation of brachialactone by Bh and other known nitrification inhibiting substances (Gopalakrishnan et al., 2009; Subbarao et al., 2009) are supposed to increase the relative -to- uptake, thereby reflecting primarily the δ15N signal of the plant available soil pool (Kahmen et al., 2008). Furthermore, Bh root exudates have been shown to reduce N. europaea populations in soil (Gopalakrishnan et al., 2009). Our results thus support the hypothesis that high BNI (low NRs) results in lower leaf δ15N, as observed in our field study. Robinson (2001) suggested to measure whole plant δ15N when studies intend to indicate source δ15N in plant tissues to avoid uncertainties in isotopic discrimination during partitioning in the plant. In this respect, however, it has to be considered that intra-plant 15N discrimination (e.g., root-to-shoot) is generally small when N availability is low or when is the primary mineral N form taken up by the plant (Evans, 2001).
Different Plant δ15N Among Contrasting Bh Genotypes in Terms of BNI
Biological nitrification inhibition differences among Bh accessions or hybrids have been revealed (Subbarao et al., 2007; Rao et al., 2014; Nuñez et al., 2018) and the effect of high BNI is expected to reduce formation and leaching (Subbarao et al., 2009, 2013). But experimental evidence for the latter is lacking. We observed a strong genotypic effect on leaf δ15N in our field studies. For instance, Bh genotypes with low NRs in the field (CIAT 16888, CIAT 679, CIAT 26159) showed a strong BNI effect from their root exudates on N. europaea (Subbarao et al., 2007). At the end of the field study, the same genotypes showed lower δ15N than CIAT 26149 with known low BNI potential (Subbarao et al., 2007). Furthermore, the higher leaf δ15N of CIAT 26146 compared to CIAT 16888 and CIAT 679 fit to our hypotheses, and earlier BNI evaluations (Subbarao et al., 2009; Nuñez et al., 2018). However, apart from BNI, other factors could have altered shoot δ15N of Bh genotypes. Exemplary is the acknowledged symbiosis of mycorrhizae with plants (Evans, 2001). However, under low N availability the cycling of N through the fungus to the plant is rather negligible for plant δ15N (Högberg et al., 1999).
Conclusion
We studied the interlinkages of plant δ15N, BNI, microbial nitrification, N uptake, and N leaching losses under controlled as well as under field conditions based on a selection of contrasting Bh genotypes. Our main conclusion is that high BNI activity decreases plant δ15N of Bh. Thus, the 15N natural abundance of grass tissue might be linked to BNI activity in soil, suppressing the growth and activity of bacterial and archaeal nitrifiers. As a result, this led to enhanced uptake by Bh and reduced losses. This ecological concept is enhanced if: (i) BNI is expressed in soil due to long-term presence of Bh; (ii) there is a continuous substrate () supply (mineralization, fertilization) so that the source N for plant uptake is never converted completely (into ); and (iii) a significant amount of formed by nitrification is leached of the rooting zone of the plants. Since Bh can take up both N forms (Castilla and Jackson, 1991), the cumulative 15N shoot signal would be confounded in case of and uptake without nitrate loss. Furthermore, other microbial enzymatic reactions should not mask the discrimination process by nitrifiers, such as: (i) volatilization (driven by high soil pH, heat, not incorporated N fertilizer); (ii) denitrification (anaerobic conditions, high C availability, substrate present). However, nitrate substrate left would even be higher enriched in 15N; and (iii) uptake of 15N depleted N derived by free living N fixing bacteria.
Our observations suggest that high BNI along with reduced microbial nitrification (one of the main reactions causing 15N:14N fractionation) and N leaching (enriching the remaining soil mineral N with 15N) are reflected in low δ15N leaf or shoot biomass signals under environments with high leaching potential. We also suggest that the method described here can serve as an indicator of the extent of leakiness for BNI field evaluations over the years, if combined with other BNI indicators like the abundance and activity of soil nitrifiers under the given conditions set out above.
Author Contributions
HK wrote the manuscript and had the overall task to modify it according to suggestions and corrections of the co-authors. Furthermore, HK measured all samples with the IRMS that have been used for this study. KE installed the greenhouse trial (Experiment 1) and conducted the sampling. JN assisted in sampling of plant material (Experiment 3) and delivered further plant material from other experiments for pre-tests (data not shown). IR was the leading senior scientist of the BMZ project at CIAT Colombia. FR contributed to the scientific interpretation and the concept of the study. JA was the leading young professional of the BMZ project at CIAT Colombia and enabled HK the access to the field trial of Experiments 2 and 3 for sampling. DM had the responsibility of taking samples of Experiment 2. AA assisted in the incubation study of Experiment 3. GC was the leading senior scientist of this study. All authors contributed to manuscript revision, read, and approved the submitted version.
Funding
The authors very much appreciate the financial support of the project “Climate-smart crop-livestock systems for smallholders in the tropics: Integration of new forage hybrids to intensify agriculture and to mitigate climate change through regulation of nitrification in soil” by the Federal Ministry for Economic Cooperation and Development (BMZ, Germany) under the GIZ Project Number 11.7860.7-001.00. In addition, this study was undertaken as part of the LivestockPlus project funded by CGIAR Research Program (CRP) on Climate Change, Agriculture and Food Security (CCAFS), which is a strategic partnership of CGIAR and Future Earth.
Conflict of Interest Statement
The authors declare that the research was conducted in the absence of any commercial or financial relationships that could be construed as a potential conflict of interest.
Acknowledgments
We would like to thank Marc-André Sparke for his cooperation during the sampling period in the greenhouse and in the field trials in Colombia. For the support with the IRMS we are grateful to Elke Dachtler, Carolin Röhl, and Christian Brandt. We would also like to thank the Livestock CRP and the Ministry of Forestry and Fisheries of Japan (MAFF) for their support that allowed maintaining the field trials for this study. We thank all donors that globally support the work of the CRP programs through their contributions to the CGIAR system.
Supplementary Material
The Supplementary Material for this article can be found online at: https://www.frontiersin.org/articles/10.3389/fmicb.2018.02383/full#supplementary-material
References
Byrnes, R. C., Nùñez, J., Arenas, L., Rao, I., Trujillo, C., Alvarez, C., et al. (2017). Biological nitrification inhibition by Brachiaria grasses mitigates soil nitrous oxide emissions from bovine urine patches. Soil Biol. Biochem. 107, 156–163. doi: 10.1016/j.soilbio.2016.12.029
Castilla, C. E., and Jackson, W. A. (1991). “Nitrogen uptake mechanism in two Brachiaria species: Y-315,” in Tropsoils Technical Report 1988-1989, ed. T. P. McBride (Raleigh, NC: Tropsoils Management Entity), 164–167.
Castoldi, G., Reis, J. G., Pivetta, L. A., and Rosolem, C. A. (2013). Soil nitrogen dynamics after Brachiaria desiccation. Rev. Bras de Ciênc Solo 37, 1620–1627. doi: 10.1590/S0100-06832013000600018
Coskun, D., Britto, D. T., Shi, W., and Kronzucker, H. J. (2017a). How plant root exudates shape the nitrogen cycle. Trends Plant Sci. 8, 661–673. doi: 10.1016/j.tplants.2017.05.004
Coskun, D., Britto, D. T., Shi, W., and Kronzucker, H. J. (2017b). Nitrogen transformations in modern agriculture and the role of biological nitrification inhibition. Nat. Plants 3:17074. doi: 10.1038/nplants.2017.74
Craine, J. M., Elmore, A. J., Aidar, M. P., Bustamante, M., Dawson, T. E., Hobbie, E. A., et al. (2009). Global patterns of foliar nitrogen isotopes and their relationships with climate, mycorrhizal fungi, foliar nutrient concentrations, and nitrogen availability. New Phytol. 183, 980–992. doi: 10.1111/j.1469-8137.2009.02917.x
Delwiche, C. C., and Steyn, P. L. (1970). Nitrogen isotope fractionation in soils and microbial reactions. Environ. Sci. Technol. 4, 929–935. doi: 10.1021/es60046a004
Evans, R. D. (2001). Physiological mechanisms influencing plant nitrogen isotope composition. Trends Plant Sci. 6, 121–126. doi: 10.1016/S1360-1385(01)01889-1
Garten, C. T., Paul, J. R., Hanson, J., Todd, D. E., Bonnie, J. R., Lu, B., et al. (2008). “Natural 15N- and 13C-abundance as indicators of forest nitrogen status and soil carbon dynamics,” in Stable Isotopes in Ecology and Environmental Science, eds R. Michener and K. Lajtha (Hoboken, NJ: John Wiley & Sons).
Gopalakrishnan, S., Watanabe, T., Pearse, S. J., Ito, O., Hossain, Z. A., and Subbarao, G. V. (2009). Biological nitrification inhibition by Brachiaria humidicola roots varies with soil type and inhibits nitrifying bacteria, but not other major soil microorganisms. Soil Sci. Plant Nutr. 55, 725–733. doi: 10.1111/j.1747-0765.2009.00398.x
Herman, D. J., and Rundel, P. (1989). Nitrogen isotope fractionation in burned and unburned chaparral soils. Soil Sci. Soc. Am. J. 53, 1229–1236. doi: 10.2136/sssaj1989.03615995005300040040x
Högberg, P., Högberg, M. N., Quist, M. E., Ekblad, A. L. F., and Näsholm, T. (1999). Nitrogen isotope fractionation during nitrogen uptake by ectomycorrhizal and non-mycorrhizal Pinus sylvestris. New Phytol. 142, 569–576. doi: 10.1046/j.1469-8137.1999.00404.x
Huber, E., Bell, T. L., and Adams, M. A. (2013). Combustion influences on natural abundance nitrogen isotope ratio in soil and plants following a wildfire in a sub-alpine ecosystem. Acta Oecol. 173, 1063–1074. doi: 10.1007/s00442-013-2665-0
Jones, A. R., and Dalal, R. C. (2017). Enrichment of natural 15N abundance during soil N losses under 20 years of continuous cereal cropping. Sci. Tot. Environ. 574, 282–287. doi: 10.1016/j.scitotenv.2016.08.192
Kahmen, A., Wanek, W., and Buchmann, N. (2008). Foliar δ15N values characterize soil N cycling and reflect nitrate or ammonium preference of plants along a temperate grassland gradient. Acta Oecol. 156, 861–870. doi: 10.1007/s00442-008-1028-8
Karwat, H., Moreta, D., Arango, J., Núñez, J., Rao, I., Rincón, Á., et al. (2017). Residual effect of BNI by Brachiaria humidicola pasture on nitrogen recovery and grain yield of subsequent maize. Plant Soil 420, 389–406. doi: 10.1007/s11104-017-3381-z
Kleinebecker, T., Hölzel, N., Prati, D., Schmitt, B., Fischer, M., and Klaus, V. H. (2014). Evidence from the real world: 15N natural abundances reveal enhanced nitrogen use at high plant diversity in Central European grasslands. J. Ecol. 102, 456–465. doi: 10.1111/1365-2745.12202
Mariotti, A., Germon, J., Hubert, P., Kaiser, P., Letolle, R., Tardieux, A., et al. (1981). Experimental determination of nitrogen kinetic isotope fractionation: some principles; illustration for the denitrification and nitrification processes. Plant Soil 62, 413–430. doi: 10.1007/BF02374138
Miranda, C. H. B., Cadisch, G., Urquiaga, S., Boddey, R. M., and Giller, K. E. (1994). Mineral nitrogen in an oxisol from the Brazilian cerrados in the presence of Brachiaria spp. Eur. J. Agron. 3, 333–337. doi: 10.1016/S1161-0301(14)80163-9
Nadelhoffer, K. J., and Fry, B. (1994). “Nitrogen isotope studies in forest ecosystems,” in Stable Isotopes in Ecology, eds K. Lajtha and R. Michener (Oxford: Blackwell), 22–44.
Nuñez, J., Arevalo, A., Karwat, H., Egenolf, K., Miles, J., Ngonidzashe, C., et al. (2018). Biological nitrification inhibition activity in a soil-grown biparental population of the forage grass Brachiaria humidicola. Plant Soil 426, 401–411. doi: 10.1007/s11104-018-3626-5
O’Sullivan, C. A., Fillery, I. R., Roper, M. M., and Richards, R. A. (2016). Identification of several wheat landraces with biological nitrification inhibition capacity. Plant Soil 404, 61–74. doi: 10.1007/s11104-016-2822-4
Pardo, L. H., Hemond, H., Montoya, J., Fahey, T., and Siccama, T. (2002). Response of the natural abundance of 15N in forest soils and foliage to high nitrate loss following clear-cutting. Can. J. For. Res. 32, 1126–1136. doi: 10.1139/x02-041
Pardo, L. H., McNulty, S. G., Boggs, J. L., and Duke, S. (2007). Regional patterns in foliar 15N across a gradient of nitrogen deposition in the northeastern US. Environ. Pollut. 149, 293–302. doi: 10.1016/j.envpol.2007.05.030
Rao, I. M., Ishitani, M., Miles, J., Peters, M., Tohme, J., Arango, J., et al. (2014). Climate-smart crop-livestock systems for smallholders in the tropics: integration of new forage hybrids to intensify agriculture and to mitigate climate change through regulation of nitrification in soil. Trop. Grassl.-Forrajes Trop. 2, 130–132. doi: 10.17138/TGFT(2)130-132
Rao, I. M., Kerridge, P. C., and Macedo, M. C. M. (1996). “Nutritional requirements of Brachiaria and adaption to acid soils,” in Brachiaria: Biology, Agronomy, and Improvement, eds J. W. Miles, B. L. Maass, and C. B. do Valle (Cali, DC: CIAT Publication; no. 259), 53–71.
Robinson, D. (2001). δ15N as an integrator of the nitrogen cycle. Trend Ecol. Evol. 16, 153–162. doi: 10.1016/S0169-5347(00)02098-X
Shearer, G. B., Kohl, D. H., and Commoner, B. (1974). The precision of determinations of the natural abundance of nitrogen-15 in soils, fertilizers, and shelf chemicals. Soil Sci. 118, 08–316. doi: 10.1097/00010694-197411000-00005
Song, G., Zhao, X., Wang, S. Q., and Xing, G. X. (2014). Nitrogen isotopic fractionation related to nitrification capacity in agricultural soils. Pedosphere 24, 186–195. doi: 10.1016/S1002-0160(14)60005-9
Stamatiadis, S., Christofides, C., Tsadilas, C., Samaras, V., and Schepers, J. S. (2006). Natural abundance of foliar 15N as an early indicator of nitrogen deficiency in fertilized cotton. J. Plant Nutr. 29, 113–125. doi: 10.1080/01904160500416505
Stevenson, B. A., Parfitt, R. L., Schipper, L. A., Baisden, W. T., and Mudge, P. (2010). Relationship between soil δ15N, C/N and N losses across land uses in New Zealand. Agric. Ecosyst. Environ. 139, 736–741. doi: 10.1016/j.agee.2010.10.020
Subbarao, G. V., Nakahara, K., Hurtado, M. D. P., Ono, H., Moreta, D., Salcedo, A. F., et al. (2009). Evidence for biological nitrification inhibition in Brachiaria pastures. Proc. Natl. Acad. Sci. U.S.A. 106, 17302–17307. doi: 10.1073/pnas.0903694106
Subbarao, G. V., Rondon, M., Ito, O., Ishikawa, T., Rao, I., Nakahara, K., et al. (2007). Biological nitrification inhibition (BNI) - is it a widespread phenomenon? Plant Soil 294, 5–18. doi: 10.1007/s11104-006-9159-3
Subbarao, G. V., Sahrawat, K. L., Nakahara, K., Rao, I. M., Ishitani, M., Hash, C. T., et al. (2013). A paradigm shift towards low-nitrifying production systems: the role of biological nitrification inhibition (BNI). Anal. Bot. 112, 297–316. doi: 10.1093/aob/mcs230
Sylvester-Bradley, R., Mosquera, D., and Mendez, J. (1988). Inhibition of nitrate accumulation in tropical grassland soils: effect of nitrogen fertilization and soil disturbance. J. Soil Sci. 39, 407–416. doi: 10.1111/j.1365-2389.1988.tb01226.x
Takebayashi, Y., Koba, K., Sasaki, Y., Fang, Y., and Yoh, M. (2010). The natural abundance of 15N in plant and soil-available N indicates a shift of main plant N resources to NO3- from NH4 + along the N leaching gradient. Rapid Commun. Mass Spectrom. 24, 1001–1008. doi: 10.1002/rcm.4469
Keywords: δ15N isotopic discrimination, 15N:14N fractionation, soil microbial nitrification, N uptake, N assimilation, nitrate leaching, soil incubation
Citation: Karwat H, Egenolf K, Nuñez J, Rao I, Rasche F, Arango J, Moreta D, Arevalo A and Cadisch G (2018) Low 15N Natural Abundance in Shoot Tissue of Brachiaria humidicola Is an Indicator of Reduced N Losses Due to Biological Nitrification Inhibition (BNI). Front. Microbiol. 9:2383. doi: 10.3389/fmicb.2018.02383
Received: 17 April 2018; Accepted: 18 September 2018;
Published: 04 October 2018.
Edited by:
Per Bengtson, Lund University, SwedenCopyright © 2018 Karwat, Egenolf, Nuñez, Rao, Rasche, Arango, Moreta, Arevalo and Cadisch. This is an open-access article distributed under the terms of the Creative Commons Attribution License (CC BY). The use, distribution or reproduction in other forums is permitted, provided the original author(s) and the copyright owner(s) are credited and that the original publication in this journal is cited, in accordance with accepted academic practice. No use, distribution or reproduction is permitted which does not comply with these terms.
*Correspondence: Georg Cadisch, Z2VvcmcuY2FkaXNjaEB1bmktaG9oZW5oZWltLmRl
†Present address: Jonathan Nuñez, Landcare Research, Lincoln, New Zealand; Idupulapati Rao, Plant Polymer Research Unit, National Center for Agricultural Utilization Research, Agricultural Research Service, United States Department of Agriculture, Peoria, IL, United States