- 1State Key Laboratory of Food Science and Technology, Jiangnan University, Wuxi, China
- 2School of Food Science and Technology, Jiangnan University, Wuxi, China
- 3International Joint Research Laboratory for Probiotics, Jiangnan University, Wuxi, China
- 4National Engineering Research Center for Functional Food, Jiangnan University, Wuxi, China
- 5Beijing Innovation Center of Food Nutrition and Human Health, Beijing Technology and Business University (BTBU), Beijing, China
Lead (Pb) intoxication is a serious food safety issue, and the development of relevant dietary strategies is an area of ongoing research. In this study, two different dietary supplements were designed and evaluated for their effects against Pb toxicity in mice. Dietary supplement A contained grape seed extract, tea polyphenols and Lactobacillus plantarum CCFM8661, and dietary supplement B contained vitamin C, calcium carbonate, zinc acetate, and L. plantarum CCFM8661. The results showed that both dietary supplements could effectively decrease Pb levels, protect aminolevulinic acid dehydratase, superoxide dismutase and catalase activities and recover glutathione, zinc protoporphyrin and malondialdehyde levels in tissues and blood of mice. A step-through passive avoidance task confirmed that the dietary supplements could recover the learning and memory capacities of Pb-exposed mice. The protective effects of both dietary supplements to alleviate oxidative stress and cognitive impairments were superior to the chelator treatment. Administration of the dietary supplements during Pb exposure offered more significant protection than administration after Pb exposure. Animal safety evaluation also indicated that these dietary supplements barely induced side effects in the mice. This study provides evidence that dietary supplements containing probiotics, micronutrients, and plant extracts can be considered a new dietary strategy against Pb toxicity.
Introduction
Lead (Pb) is a pollutant widely distributed in the environment and food chain. Once accumulated in the human body, Pb and its compounds induce oxidative stress and disrupt the metabolism of essential metals (Gurer and Ercal, 2000; Dobrakowski et al., 2017), which in turn leads to acute or chronic toxic effects on the nervous (Winder et al., 1982; Cecil et al., 2008), hematic (Redig et al., 1991; Ho and Ho, 1997), hepatic (Farmand et al., 2005; Xia et al., 2010), urinary (Loghman-Adham, 1997; Fels et al., 1998), and cardiovascular systems (Freeman, 1965). Pb intoxication is related with clinical symptoms including mental hypomnesis, anemia, tubular atrophy, and even death. Industrial development has caused the number of Pb poisoning cases to increase in recent years. A Pb water pollution incident occurring in Flint, MI, United States in 2015 led to a sharp increase in the blood Pb levels of local children (Hannaattisha et al., 2016). Another population-based study showed that the blood Pb levels of children living in Guiyu, one of the most seriously heavy metal-polluted regions of China, have reached 7.06 mg/dL (Guo et al., 2014).
The most common therapeutic strategy against Pb poisoning is to promote the metal’s excretion by using chelating agents such as ethylenediaminetetraacetic acid (EDTA) and dimercaptosuccinic acid (DMSA). However, these chelators have a range of side effects (Paternain et al., 1990; Jorgensen, 1993; Porru and Alessio, 1996; Chen et al., 1999) and are not suitable for high-dose and long-term treatment. Thus, increasing attention is being paid to seeking safe dietary supplements, such as micronutrients, plant extracts and probiotics, for the intervention and alleviation of Pb intoxication.
Supplementation with micronutrients including essential trace elements and vitamins has been reported to provide protection against Pb toxicity (Cerklewski and Forbes, 1976; Shaban and Said, 2011; Basha et al., 2012). Metals such as calcium (Ca) and zinc (Zn) have similar chemical properties to Pb and can compete for the binding sites of metal absorptive and enzymatic proteins in the gut, blood, and tissues of humans (Basha et al., 2012). Zn supplementation also induces the biosynthesis of metallothioneins, proteins involved in the detoxification of heavy metals (Baltaci et al., 2018). Recent studies showed that the intake of Ca and Zn can reduce the level of Pb metal in the blood and bone of pregnant women (Gulson et al., 2004) and children (Cantoral et al., 2015). Vitamin C is a non-enzymatic antioxidant and a potential chelator of Pb (Goyer and Cherian, 1979). Daily supplementation of vitamin C has been reported to reduce blood Pb levels and alleviate lipid peroxidation in erythrocytes of smokers and Pb-exposed workers (Dawson et al., 1999; Rendón-Ramírez et al., 2014).
An increasing number of studies have shown that plant extracts with antioxidative stress properties can effectively alleviate Pb toxicity (Hsu and Guo, 2002; Jiao et al., 2011). Grape seed extract, which is rich in procyanidins, could recover lesions in the cardiovascular system induced by Pb exposure and inhibit alterations in the levels of adrenaline, 5-hydroxytryptamine and glutamic pyruvic transaminase (ALT) in the brain and liver of rats (Waggas, 2012). Another common plant extract, tea polyphenols, was reported to prevent Pb-induced dysfunctions in the brain, blood and liver of humans and animals (Hamed et al., 2010; Mehana et al., 2012). Catechins, one of the main constituents of tea polyphenols, were able to ameliorate cytotoxicity in renal and nerve cells under Pb exposure (Chen et al., 2002, 2003).
Apart from the above-mentioned dietary supplements, recent reports revealed that probiotics were capable of binding Pb in vitro and diminishing oxidative stress in vivo, indicating their potential against Pb toxicity (Halttunen et al., 2007). In our previous studies, Lactobacillus plantarum CCFM8661 was screened out for its good tolerance and ability to bind to Pb (Tian et al., 2012; Yin et al., 2016). Further animal experiments confirmed that this strain decreased Pb levels in blood and tissues of mice, and offered significant protection during the recovery from Pb-induced oxidative stress and histopathological changes in mice (Tian et al., 2012).
Based on these studies, it is believed that the supplements of specific dietary components can be effective antagonists to Pb intoxication. Further studies are needed to develop relevant therapeutic dietary strategies. We noticed that previous reports mainly focused on a single component and that the synergistic effect of micronutrients, plant extracts and probiotics against Pb toxicity was rarely investigated. Therefore, in the present study, two kinds of multiple dietary supplements were designed and their safety and protective effects against Pb toxicity were evaluated in mice.
Materials and Methods
Chemicals and Reagents
Dimercaptosuccinic acid was purchased from New Asia Pharmaceutical Company (Shanghai, China). Grape seed extract was purchased from Jianfeng Natural Product Research and Development Company (Tianjin, China). Tea polyphenols were purchased from Lvkang Natural Products Company (Nanchang, China). Microcrystalline cellulose, low-substituted hydroxypropyl cellulose and silicon dioxide were purchased from Tianzheng Pharmaceutical Excipients Company (Xian, China). Kits used to measure the levels of malondialdehyde (MDA), glutathione (GSH), albumin (ALB), blood urea nitrogen (BUN), creatinine (CRE), total cholesterol (TCHO), triglyceride (TG), and total protein (TP) and the activities of superoxide dismutase (SOD), catalase (CAT), ALT, and glutamic oxalacetic transaminase (AST) were purchased from the Jiancheng Bioengineering Institute (Nanjing, China). Kits used to measure the levels of zinc protoporphyrin (ZPP) and the activities of δ-aminolevulinic acid dehydratase (ALAD) were purchased from the Chuanxiang Bioengineering Company (Wuhan, China). Pb acetate and all other analytical laboratory chemicals and reagents were purchased from Sinopharm Chemical Reagent Company (Shanghai, China).
Bacterial Strains and Culture
Lactobacillus plantarum CCFM8661 were obtained from the Culture Collections of Food Microbiology, Jiangnan University (Wuxi, China). The bacteria were cultured in de Man, Rogosa and Sharpe broth (Hopebio Company, Qingdao, China) at 37°C for 18 h. The bacterial cells were freeze-dried as previously reported (Zhai et al., 2014) with the protectant replaced by trehalose.
Design of the Dietary Supplements
The components of the two different dietary supplements are listed in Table 1. Microcrystalline cellulose, low-substituted hydroxypropyl cellulose and silicon dioxide served as filler, disintegrant and lubricant, respectively (Liu et al., 2009; Mahmoud et al., 2009). The doses of micronutrients, plant extracts, and probiotics were selected based on previous studies to provide protective effects against Pb toxicity (Liao et al., 2008; Mehana et al., 2012; Tian et al., 2012; Waggas, 2012). Dietary supplements A (DSA) and B (DSB) were designed to evaluate the protection of a plant extracts-probiotics mixture and a micronutrients-probiotics mixture, respectively. Both supplements were pressed into tablets of 240–260 mg each.
Based on our previous study, no significant alterations in the levels of procyanidins, catechins, and vitamin C could be observed after tableting, whereas the number of living bacterial cells of L. plantarum CCFM8661 decreased to approximately 8 × 109 cfu/g tablet (Liu et al., 2016).
Animals and Design of the Pb Intoxication Experiment
Six-week-old male C57black/6 mice (weighing 25–30 g) obtained from the Shanghai Laboratory Animal Center (Shanghai, China) were used in the experiments. Mice were maintained in a room kept on a 12-h light/dark cycle at a constant temperature and humidity and had free access to standard commercial mouse food and distilled water. All animal experiments described herein were approved by the Ethics Committee of Jiangnan University, China (JN No. 20150811-1211-51) and performed in accordance with the guidelines set by the European Community (directive 2010/63/EU).
As shown in Table 2, 120 mice were randomly divided into three groups. The intervention and therapy groups were designed to evaluate the protective effects of the dietary supplements against Pb toxicity during and after chronic exposure, respectively. The DMSA groups were set as a drug control. The intervention and therapy groups were divided into five subgroups, and an oral dose of Pb at 1 g/L of drinking water was used to model chronic exposure (Tangpong et al., 2010).
At the end of the experiment, mice underwent a step-through passive avoidance task and were then sacrificed under light ether anesthesia. A portion of blood was collected in anticoagulant tubes for routine hematological examination. The remaining blood was centrifuged to obtain serum and stored at −80°C. The liver, whole kidneys, and brains were weighed and stored in metal-free Eppendorf tubes at −80°C for the biochemical assays and estimation of Pb levels.
Step-Through Passive Avoidance Task
The step-through task was performed on the last 2 days of the gavage according to previous reports (Lu et al., 2011; Ferlemi et al., 2014). The step-through latency (time taken to completely enter the darkened chamber) was recorded to evaluate the learning and memory of mice.
Determination of Pb in Tissues and Blood
The liver, whole kidneys, brain, and blood samples were digested in concentrated HNO3 with a Microwave Digestion System (MARS; CEM, United Kingdom). The contents of Pb in tissues and blood were determined by using flame or graphite furnace atomic absorption spectrophotometry (Spectr AAS or AA; Varian, United States).
Determination of Biochemical Indicators in Tissues and Serum
The levels of MDA and GSH and the activities of SOD and CAT in tissues were measured with kits purchased from Jiancheng Bioengineering Institute (Nanjing, China). The levels of ZPP and the activities of ALAD in serum were measured with kits obtained from Chuanxiang Bioengineering Company (Wuhan, China).
Determination of Red Blood Cell and Hemoglobin Levels in the Blood
The levels of red blood cells (RBCs) and hemoglobin in blood were measured using an automated hematology analyzer (XE-2100, Sysmex, Japan).
Animals and Design of the Safety Evaluation Experiment
The safety of the two dietary supplements was evaluated by an acute toxicity test and a 30-day feeding trial in mice (Silva et al., 2011). The animal experiments were approved by the Ethics Committee of Jiangnan University, China (JN No.20150519-0704-34 and JN No 20150707-0820-47).
For the acute toxicity test, 60 C57black/6 mice (half male and half female, 3 weeks of age on arrival) were obtained from the Shanghai Laboratory Animal Center (Shanghai, China). After acclimation, mice were randomly divided into three groups: control group (receiving a single oral dose of PBS at 0.4 mL/20 g body weight via gavage), DSA group (receiving a single oral dose of dietary supplement A at 4 g/20 g body weight via gavage) and DSB group (receiving a single oral dose of dietary supplement B at 4 g/20 g body weight via gavage). Male and female mice were housed in separate cages. After the oral administration, mice were kept for 2 weeks and their body weight, food consumption, and behavior were recorded. At the end of the experiment, mice were sacrificed, and the histopathological alterations of liver, kidneys, and spleen were observed.
For the 30-day feeding trial, 140 C57black/6 mice (half male and half female, 3 weeks of age on arrival) were obtained from the Shanghai Laboratory Animal Center (Shanghai, China). After acclimation, mice were randomly divided into seven groups: control group (receiving 0.2 mL PBS via gavage each day) and low-dose DSA group, mid-dose DSA group, high-dose DSA group, low-dose DSB group, mid-dose DSB group, and high-dose DSB group. Mice in the latter six groups received dietary supplement A or B at 10, 15, and 20 g/kg body weight each day, respectively. The functional components of the dietary supplements including probiotics, micronutrients, and plant extracts were given via gavage in 0.2 mL PBS, and the filler, disintegrant, and lubricant were mixed into the feed of the mice. Male and female mice were housed in separate cages. Their body weight, food consumption, and behavior were recorded during the experiment. After sacrifice, the routine hematological indicators were measured using an automated hematology analyzer (XE-2100, Sysmex, Japan). The biochemical parameters in blood including ALB, ALT, AST, BUN, CRE, TCHO, TG, and TP were determined using commercial kits. The histopathological alterations of liver, kidneys, and spleen were also observed.
Statistical Analysis
All data are presented as the mean ± standard deviation and were analyzed by one-way analysis of variance (ANOVA) and Duncan post hoc-test. The data were considered to show statistical significance at a P-value of 0.05 or less.
Results
Pb Levels in Tissues and Blood of Mice
As shown in Table 3, Pb levels in the liver, kidneys, brain, and blood of Pb-treated mice were significantly higher than those of the control group (P < 0.05). Oral administration of both dietary supplements decreased the levels of Pb in tissues and blood (P < 0.05), although the protection was not as obvious as that with the DMSA treatments. Dietary supplements induced more marked reduction in Pb levels in the liver and blood of mice in the intervention groups than in the therapy groups.
ALAD Activities in Serum of Mice
Lead exposure induced a significant decrease in ALAD activities in the serum of mice (Figure 1). Oral administration of both DSA and DSB recovered the ALAD activities (P < 0.05). No marked difference in the activities of this enzyme could be observed after the intake of dietary supplements between the intervention and therapy groups.
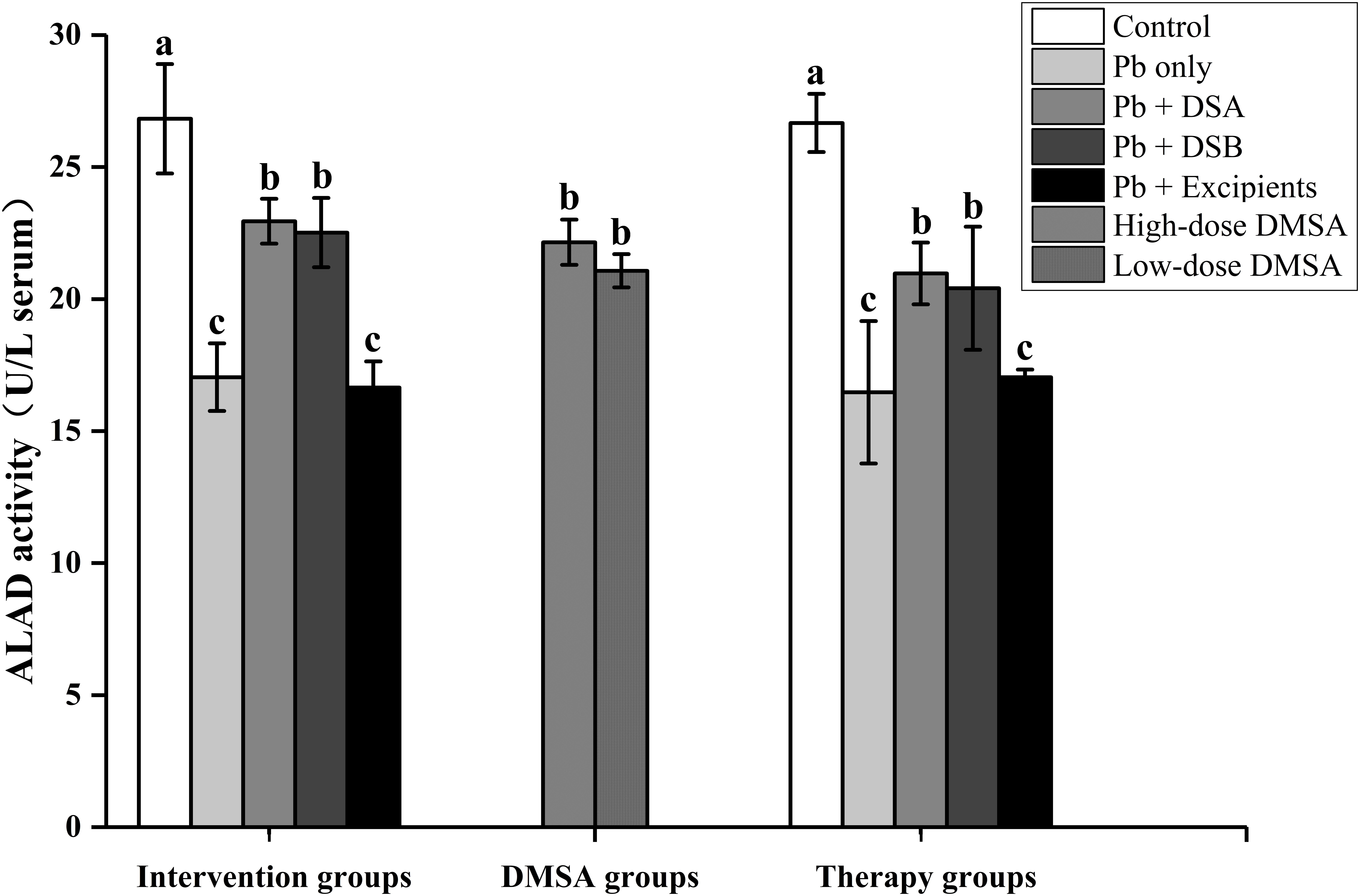
FIGURE 1. Effects of dietary supplements on ALAD activities in the serum of mice. Values are for 10 mice per group. Letters a to c indicate statistically significant differences (P < 0.05) within each group comparison. Abbreviations of group names are as in Table 2.
ZPP Levels in Serum of Mice
Supplementation of both DSA and DSB inhibited the Pb-induced increase of ZPP levels in the serum of mice (Figure 2, P < 0.05). However, the protection was not as obvious as that with the DMSA treatments.
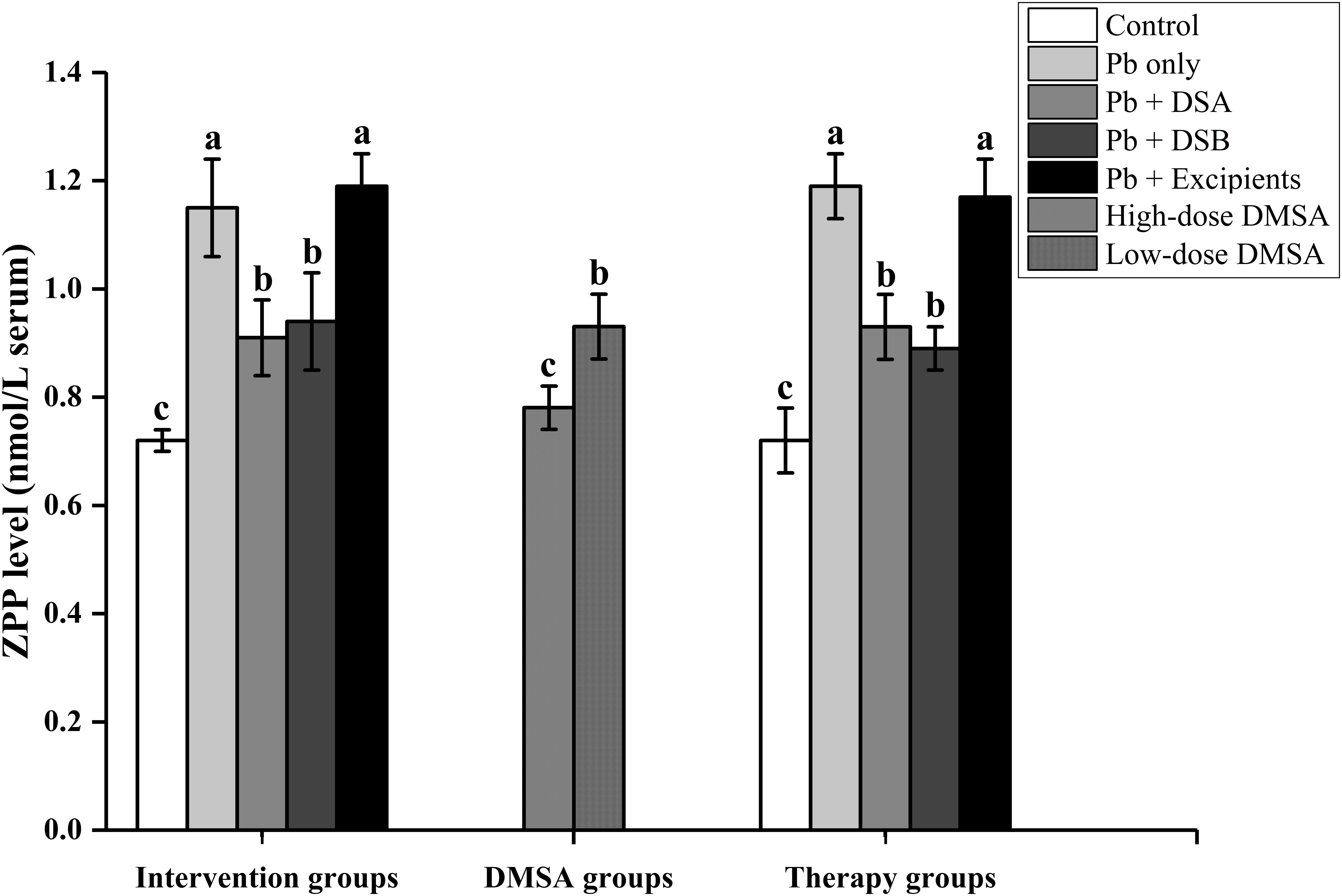
FIGURE 2. Effects of dietary supplements on ZPP levels in the serum of mice. Values are for 10 mice per group. Letters a to c indicate statistically significant differences (P < 0.05) within each group comparison. Abbreviations of group names are as in Table 2.
Activities of SOD and CAT and the Levels of MDA and GSH in Tissues of Mice
As shown in Tables 4–6, the activities of SOD and CAT were inhibited in the livers, kidneys, and brains of Pb-treated mice (P < 0.05), except for undetected CAT activities in the brains. Pb exposure also induced alterations in the levels of GSH and MDA in these tissues of mice. Oral administration of both DSA and DSB recovered the changes in these biochemical indicators (P < 0.05), and the protection was more significant than that offered by the DMSA treatment.
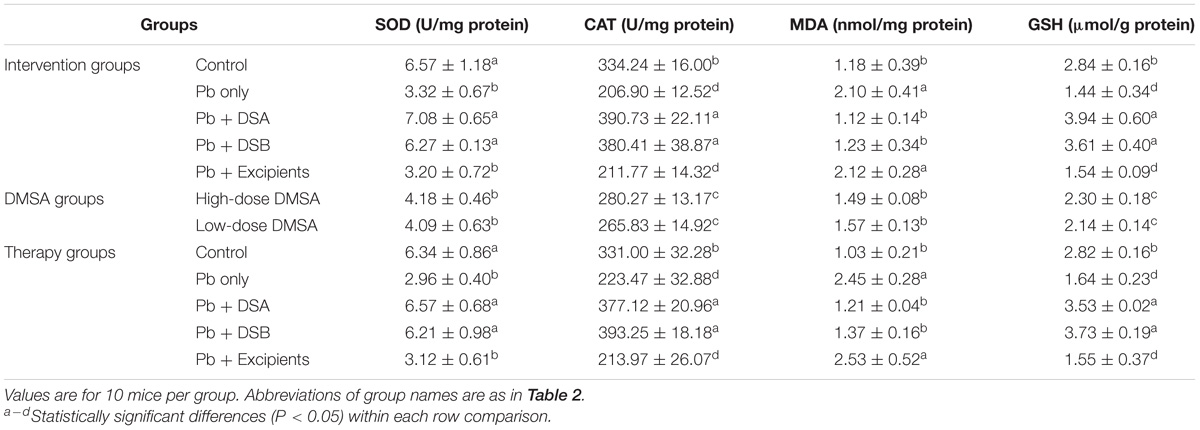
TABLE 4. Effects of dietary supplements on the activities of SOD and CAT and the levels of MDA and GSH in the livers of mice.
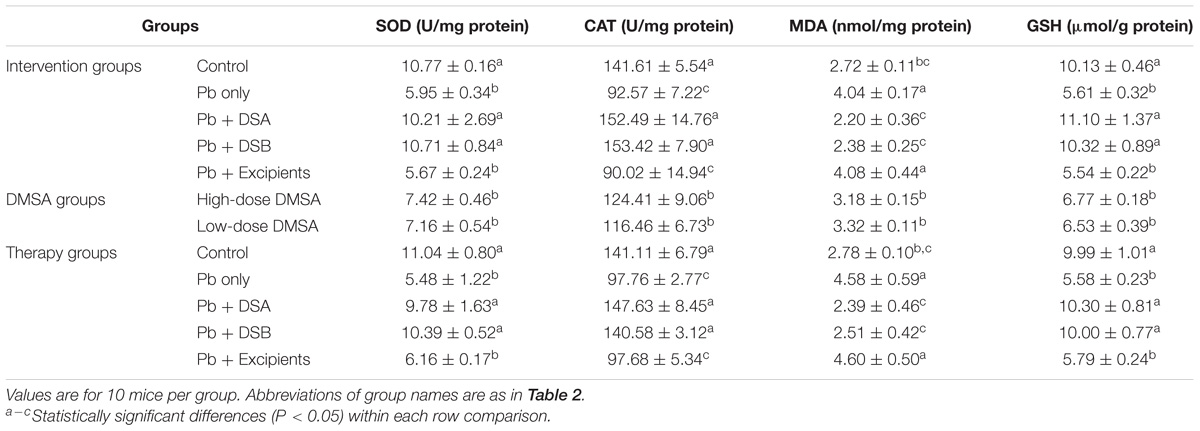
TABLE 5. Effects of dietary supplements on the activities of SOD and CAT and the levels of MDA and GSH in the kidneys of mice.
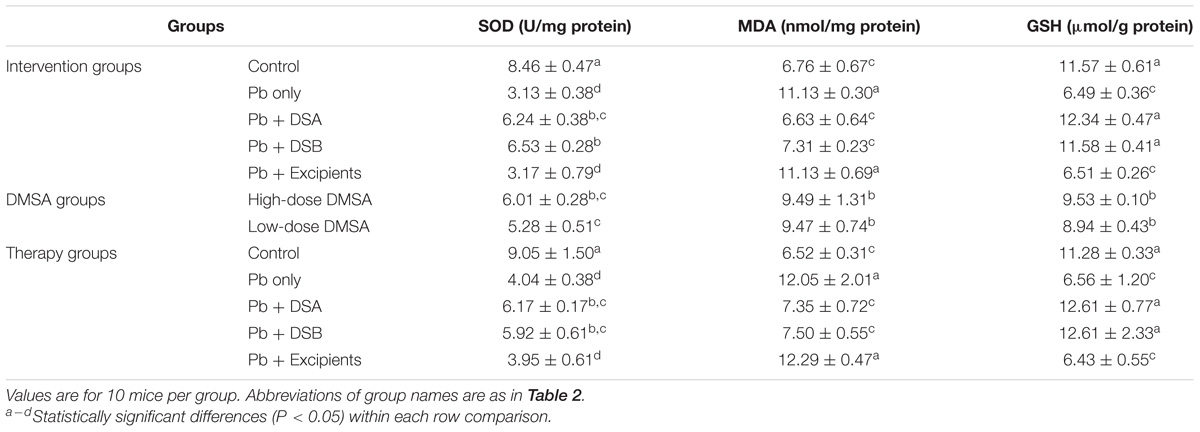
TABLE 6. Effects of dietary supplements on SOD activity and the levels of MDA and GSH in the brains of mice.
Levels of RBCs and Hemoglobin in the Blood of Mice
Lead exposure decreased the levels of RBCs and hemoglobin in the serum of mice (Table 7). Both DSA and DSB supplementation recovered the alterations in these hematological indicators (P < 0.05), and the protection was as obvious as that with DMSA treatment.
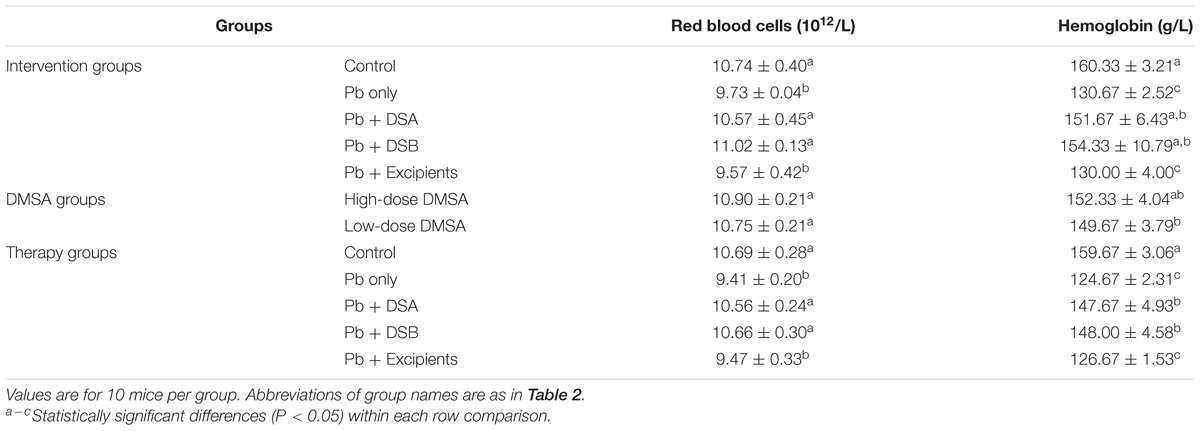
TABLE 7. Effects of dietary supplements on red blood cell and hemoglobin levels in the blood of mice.
Learning and Memory Ability of Mice
Compared with control groups, Pb exposure induced marked deficits in the learning and memory abilities of mice (Table 8). Both dietary supplements significantly reduced the step-through latency time, and their effects were superior to that of the DMSA treatment (P < 0.05). We also noticed that both DSA and DSB administration showed better protection in the intervention groups than that in the therapy groups.
Safety Evaluation of the Dietary Supplements
Both the acute toxicity test and 30-day feeding trial showed that the supplementation of DSA and DSB caused no significant alterations in the body weight, food consumption, and behavior of mice (data not shown). No changes in the levels of RBCs, hemoglobin, leukocytes, ALB, ALT, AST, BUN, CRE, TCH, TG, and TP in the blood could be observed after the intake of the dietary supplements (Supplementary Tables S1, S2). The supplements did not induce changes in organ coefficients (Supplementary Table S3) or pathological lesions in livers, kidneys, and spleens (data not shown).
Discussion
Lead is a toxic heavy metal that can induce a range of adverse health effects in humans. The body burden of Pb in the general population has been estimated to be 1,000 times higher than that of prehistoric humans (Patterson et al., 1991). In the last 10 years, the world consumption of Pb has continued to increase, and developing countries are especially facing serious Pb pollution problems (Zhai et al., 2015). Once accumulated in the human body, Pb is hard to excrete, and its biological half-time can be several decades (Sugita, 1978). This is confirmed by our results, as the Pb levels in mice of the Pb-only group did not decrease even after exposure had been stopped for 9 weeks (Table 3). Also noted was the lack of effectiveness of excipients including microcrystalline cellulose, low-substituted hydroxypropyl cellulose, and silicon dioxide against Pb toxicity, indicating the necessity to develop potential dietary strategies.
In the present study, nutrients including essential elements, vitamins, plant extracts, and probiotics were selected and incorporated into the design of two different dietary supplements. Both DSA and DSB could significantly reduce Pb levels in the tissues and blood of mice (Table 3). The protection offered by the former supplement may be due to the Pb-chelating capacities of catechins and L. plantarum CCFM8661 (Chen et al., 2002; Yin et al., 2016). Grape seed extracts, tea polyphenols, and probiotics can also protect the intestinal barrier, which may in turn inhibit Pb absorption in the gut (Watson et al., 2004; Goodrich et al., 2012; Zhai et al., 2016). Based on previous studies, essential metals, and vitamin C supplementation could prevent heavy metal absorption and decrease Pb levels in humans and animals (Goyer and Cherian, 1979; Zhai et al., 2015). This may explain the protective effects of DSB supplementation against the increase of Pb in the tissues and blood of mice.
Lead accumulation can trigger abnormal oxidative stress and in turn induces histopathological alterations in the tissues, which is a main mechanism of Pb toxicity (Stohs and Bagchi, 1995). Downstream protective effects of Pb removal from the body by the two dietary supplements included recovery of the alterations in oxidative stress-related parameters in the livers, kidneys, and brains of mice (Tables 4–6). Moreover, nutrients including vitamin C, grape seed extract, tea polyphenols, and probiotics have been reported to be natural anti-oxidants (Noroozi et al., 1998; Bagchi et al., 2000; Zhai et al., 2014), which may offer direct protection against Pb-induced oxidative stress. Zn functions as a cofactor of Cu/Zn SOD, and the supplementation of this essential metal may also play a role in the alleviation of oxidative stress (Prasanthi et al., 2010).
Hematological and nervous systems are key targets in the progression of Pb toxicity. ALAD is a vital enzyme in the hematological system, which is sensitive to Pb exposure (Wang and Fowler, 2008). The Pb-induced inhibition of ALAD damages heme synthesis and increases the level of ZPP, which further affects the levels of RBCs and hemoglobin in the blood (Alexander et al., 1998). In the present study, oral administration of DSA and DSB significantly recovered both of these hematological indicators (Figures 1, 2 and Table 7), indicating protection of both dietary supplements against Pb-induced dysfunctions in the hematological system. Ca and Zn have been reported to alleviate Pb-induced neurotoxicity by competing for the binding sites of enzymatic proteins in the nervous system (Prasanthi et al., 2006). Vitamin C, procyanidins and tea polyphenols can inhibit the production of reactive oxygen species induced in the nervous system by Pb, which further reverses the effects of hippocampal injury and reduces neuronal cell apoptosis in animals (Liu et al., 2014; Ebrahimzadehbideskan et al., 2016). Moreover, recent clinical studies confirmed that probiotics may play a role in alleviating nerve dysfunctions via the “brain-gut axis” (Pinto-Sanchez et al., 2017). These analyses can explain the marked improvement of the learning and memory abilities of the Pb-exposed mice after the DSA and DSB treatments.
We noticed that both DSA and DSB offered superior protection against Pb toxicity over that of any single component of the dietary supplements. Compared with a single L. plantarum CCFM8661 treatment (Tian et al., 2012), DSA and DSB were more efficient in recovering antioxidant enzyme activities and inhibiting lipid peroxidation in the tissues of mice. This may be due to the antioxidative stress role of vitamin C and plant extracts in the dietary supplements. Compared with the supplementation of micronutrients, catechins, or procyanidins alone (Patra et al., 2001; Zhang et al., 2004; Liao et al., 2008; Yin et al., 2008), the dietary supplements in the present study provided more significant protective effects to reduce the Pb burden in the mice. This may be explained by a synergistic effect of multiple nutrients from DSA and DSB to increase Pb excretion and prohibit Pb absorption. Also of importance is that besides the above-mentioned protection against Pb toxicity, these dietary supplements may provide other beneficial effects for humans such as the supplementation of essential metals, scavenging of free radicals, and modulation of the gut microbiota.
The intervention and therapy groups in the present study were designed to understand the optimal therapeutic strategy for the dietary supplements. Taking all effects into consideration, DSA and DSB treatment in the intervention groups offered superior protective effects on decreasing Pb levels in the tissues (livers and brains) and recovering the learning and memory abilities of mice than those in the therapy groups (Tables 3, 8). As the dietary supplements in the intervention groups were administered during the exposure to Pb, they may inhibit the initial absorption of Pb in the gut, thus preventing the accumulation of this metal in the tissues and alleviating downstream adverse effects including pathological injuries and cognitive disorders. If administered after exposure (therapy groups), the dietary supplements seemed to be less effective as Pb had already accumulated in the body and triggered a range of physiological dysfunctions.
Dimercaptosuccinic acid is a chelator commonly used for the treatment of Pb toxicity (Chisolm, 2000). Compared with the DMSA treatment, the dietary supplements did not exhibit the same protection in reducing the Pb burden (Table 3) and recovery of the ZPP level (Figure 2), but they did show more obvious alleviation of oxidative stress (Tables 4–6) and cognitive dysfunctions (Table 8). This may indicate that the dietary supplements designed in the present study may offer a route of protection against Pb toxicity besides metal chelation. The anti-oxidant and neuroregulation properties of the nutritional components may be involved in this mode of protection. Safety evaluations are essential for the development of novel dietary supplements (Kruger and Mann, 2003). The nutrients selected in the present study have been widely used in food and dietary supplements. The acute toxicity test and 30-day feeding trial also confirmed that the consumption of DSA and DSB at different doses did not induce physiological disorders in mice, indicating that these dietary supplements appeared to be relatively safe. Further clinical safety assessments are needed to evaluate their application in humans.
Conclusion
This study showed that the probiotics, micronutrients, and plant extracts contained in the tested dietary supplements appear to have protective effects against Pb toxicity in mice. The supplements could decrease tissue Pb accumulation, alleviate tissue oxidative stress and recover dysfunctions in the hematological and nervous systems of mice. The animal safety assessment confirmed that these dietary supplements barely induced any side effects in the studied mice.
Author Contributions
QZ and LY carried out the experiments and drafted the manuscript. JZ and HZ participated in the analysis of the data. FT conceived of the study and managed the project design. WC helped to revise the manuscript. All authors read and approved the final manuscript.
Funding
This work was supported by the Natural Science Foundation of Jiangsu Province (BK20160175), the National Natural Science Foundation of China (Nos. 31601452 and 31470161), the BBSRC Newton Fund Joint Centre Award, the National first-class discipline program of Food Science and Technology (JUFSTR20180102) and Collaborative innovation center of food safety and quality control in Jiangsu Province.
Conflict of Interest Statement
The authors declare that the research was conducted in the absence of any commercial or financial relationships that could be construed as a potential conflict of interest.
Supplementary Material
The Supplementary Material for this article can be found online at: https://www.frontiersin.org/articles/10.3389/fmicb.2018.02134/full#supplementary-material
References
Alexander, B. H., Checkoway, H., Costa-Mallen, P., Faustman, E. M., Woods, J. S., Kelsey, K. T., et al. (1998). Interaction of blood lead and delta-aminolevulinic acid dehydratase genotype on markers of heme synthesis and sperm production in lead smelter workers. Environ. Health Perspect. 106, 213–216. doi: 10.1289/ehp.98106213
Bagchi, D., Bagchi, M., Stohs, S. J., Das, D. K., Ray, S. D., Kuszynski, C. A., et al. (2000). Free radicals and grape seed proanthocyanidin extract: importance in human health and disease prevention. Toxicology 148, 187–197. doi: 10.1016/s0300-483x(00)00210-9
Baltaci, A. K., Yuce, K., and Mogulkoc, R. (2018). Zinc Metabolism and Metallothioneins. Biol. Trace Elem. Res. 183, 22–31. doi: 10.1007/s12011-017-1119-7
Basha, D. C., Rani, M. U., Devi, C. B., Kumar, M. R., and Reddy, G. R. (2012). Perinatal lead exposure alters postnatal cholinergic and aminergic system in rat brain: reversal effect of calcium co-administration. Int. J. Dev. Neurosci. 30, 343–350. doi: 10.1016/j.ijdevneu.2012.01.004
Cantoral, A., Téllez-Rojo, M. M., Levy, T. S., Hernández-Ávila, M., Schnaas, L., Hu, H., et al. (2015). Differential association of lead on length by zinc status in two-year old Mexican children. Environ. Health Glob. 14:95. doi: 10.1186/s12940-015-0086-8
Cecil, K. M., Brubaker, C. J., Adler, C. M., Dietrich, K. N., Altaye, M., Egelhoff, J. C., et al. (2008). Decreased brain volume in adults with childhood lead exposure. PLoS Med. 5:e112. doi: 10.1371/journal.pmed.0050112
Cerklewski, F. L., and Forbes, R. M. (1976). Influence of dietary zinc on lead toxicity in the rat. J. Nutr. 106, 689–696. doi: 10.1093/jn/106.5.689
Chen, L., Yang, X., Jiao, H., and Zhao, B. (2002). Tea catechins protect against lead-induced cytotoxicity, lipid peroxidation, and membrane fluidity in HepG2 cells. Toxicol. Sci. 69, 149–156. doi: 10.1093/toxsci/69.1.149
Chen, L., Yang, X., Jiao, H., and Zhao, B. (2003). Tea catechins protect against lead-induced ROS formation, mitochondrial dysfunction, and calcium dysregulation in PC12 cells. Chem. Res. Toxicol. 16, 1155–1161. doi: 10.1021/tx0340605
Chen, S., Golemboski, K. A., Sanders, F. S., and Dietert, R. R. (1999). Persistent effect of in utero meso-2, 3-dimercaptosuccinic acid (DMSA) on immune function and lead-induced immunotoxicity. Toxicology 132, 67–79. doi: 10.1016/s0300-483x(98)00139-5
Chisolm, J. J. Jr. (2000). Safety and efficacy of meso-2,3-dimercaptosuccinic acid (DMSA) in children with elevated blood lead concentrations. J. Toxicol. Clin. Toxicol. 38, 365–375. doi: 10.1081/clt-100100945
Dawson, E. B., Evans, D. R., Harris, W. A., Teter, M. C., and Mcganity, W. J. (1999). The effect of ascorbic acid supplementation on the blood lead levels of smokers. J. Am. Coll. Nutr. 18, 166–170. doi: 10.1080/07315724.1999.10718845
Dobrakowski, M., Boroń, M., Birkner, E., Kasperczyk, A., Chwalińska, E., Lisowska, G., et al. (2017). The effect of a short-term exposure to lead on the levels of essential metal ions, selected proteins related to them, and oxidative stress parameters in humans. Oxid. Med. Cell. Longev. 2017:8763793. doi: 10.1155/2017/8763793
Ebrahimzadehbideskan, A. R., Hami, J., Alipour, F., Haghir, H., Fazel, A. R., and Sadeghi, A. (2016). Protective effects of ascorbic acid and garlic extract against lead-induced apoptosis in developing rat hippocampus. Metab. Brain Dis. 31, 1123–1132. doi: 10.1007/s11011-016-9837-7
Farmand, F., Ehdaie, A., Roberts, C. K., and Sindhu, R. K. (2005). Lead-induced dysregulation of superoxide dismutases, catalase, glutathione peroxidase, and guanylate cyclase. Environ. Res. 98, 33–39. doi: 10.1016/j.envres.2004.05.016
Fels, L. M., Wünsch, M., Baranowski, J., Norska-Borówka, I., Price, R. G., Taylor, S. A., et al. (1998). Adverse effects of chronic low level lead exposure on kidney function–a risk group study in children. Nephrol. Dial. Transplant. 13, 2248–2256. doi: 10.1093/ndt/13.9.2248
Ferlemi, A. V., Avgoustatos, D., Kokkosis, A. G., Protonotarios, V., Constantinou, C., and Margarity, M. (2014). Lead-induced effects on learning/memory and fear/anxiety are correlated with disturbances in specific cholinesterase isoform activity and redox imbalance in adult brain. Physiol. Behav. 131, 115–122. doi: 10.1016/j.physbeh.2014.04.033
Freeman, R. (1965). Reversible myocarditis due to chronic lead poisoning in childhood. Arch. Dis. Child. 40, 389–393. doi: 10.1136/adc.40.212.389
Goodrich, K. M., Fundaro, G., Griffin, L. E., Grant, A., Hulver, M. W., Ponder, M. A., et al. (2012). Chronic administration of dietary grape seed extract increases colonic expression of gut tight junction protein occludin and reduces fecal calprotectin: a secondary analysis of healthy Wistar Furth rats. Nutr. Res. 32, 787–794. doi: 10.1016/j.nutres.2012.09.004
Goyer, R. A., and Cherian, M. G. (1979). Ascorbic acid and EDTA treatment of lead toxicity in rats. Life Sci. 24, 433–438. doi: 10.1016/0024-3205(79)90215-7
Gulson, B. L., Mizon, K. J., Palmer, J. M., Korsch, M. J., Taylor, A. J., and Mahaffey, K. R. (2004). Blood lead changes during pregnancy and postpartum with calcium supplementation. Environ. Health Perspect. 112, 1499–1507. doi: 10.1289/ehp.6548
Guo, P., Xu, X., Huang, B., Sun, D., Zhang, J., Chen, X., et al. (2014). Blood lead levels and associated factors among children in guiyu of china: a population-based study. PLoS One 9:e105470. doi: 10.1371/journal.pone.0105470
Gurer, H., and Ercal, N. (2000). Can antioxidants be beneficial in the treatment of lead poisoning? Free Radic. Biol. Med. 29, 927–945. doi: 10.1016/s0891-5849(00)00413-5
Halttunen, T., Salminen, S., and Tahvonen, R. (2007). Rapid removal of lead and cadmium from water by specific lactic acid bacteria. Int. J. Food Microbiol. 114, 30–35. doi: 10.1016/j.ijfoodmicro.2006.10.040
Hamed, E. A., Meki, A. R., and El-Mottaleb, N. A. A. (2010). Protective effect of green tea on lead-induced oxidative damage in rat’s blood and brain tissue homogenates. J. Physiol. Biochem. 66, 143–151. doi: 10.1007/s13105-010-0019-5
Hannaattisha, M., Lachance, J., Sadler, R. C., and Champney, S. A. (2016). Elevated blood lead levels in children associated with the flint drinking water crisis: a spatial analysis of risk and public health response. Am. J. Public Health 106, 283–290. doi: 10.2105/AJPH.2015.303003
Ho, J. W., and Ho, A. W. (1997). Environmental lead exposure induces changes in the heme biosynthetic pathway. Environ. Toxicol. 12, 245–248. doi: 10.1002/(SICI)1098-2256(1997)12:3<245::AID-TOX7>3.0.CO;2-8
Hsu, P. C., and Guo, Y. L. (2002). Antioxidant nutrients and lead toxicity. Toxicology 180, 33–44. doi: 10.1016/s0300-483x(02)00380-3
Jiao, J., Lü, G., Liu, X., Zhu, H., and Zhang, Y. (2011). Reduction of blood lead levels in lead-exposed mice by dietary supplements and natural antioxidants. J. Sci. Food Agric. 91, 485–491. doi: 10.1002/jsfa.4210
Jorgensen, F. (1993). Succimer: the first approved oral lead chelator. Am. Fam. Physician 48, 1496–1502.
Kruger, C. L., and Mann, S. W. (2003). Safety evaluation of functional ingredients. Food Chem. Toxicol. 41, 793–805. doi: 10.1016/s0278-6915(03)00018-8
Liao, Y., Yu, F., Jin, Y., Lu, C., Li, G., Zhi, X., et al. (2008). Selection of micronutrients used along with DMSA in the treatment of moderately lead intoxicated mice. Arch. Toxicol. 82, 37–43. doi: 10.1007/s00204-007-0233-2
Liu, C., Liu, X., Tong, J., Chen, D., and Bi, K. (2009). Design and evaluation of San-huang dispersible tablet–an efficient delivery system for traditional Chinese medicine. Pharm. Dev. Technol. 14, 506–515. doi: 10.1080/10837450902814164
Liu, C. M., Ma, J. Q., Liu, S. S., Zheng, G. H., Feng, Z. J., and Sun, J. M. (2014). Proanthocyanidins improves lead-induced cognitive impairments by blocking endoplasmic reticulum stress and nuclear factor-κB-mediated inflammatory pathways in rats. Food Chem. Toxicol. 72, 295–302. doi: 10.1016/j.fct.2014.07.033
Liu, Y., Zhai, Q., Zhang, H., Jin, J., and Chen, W. (2016). Studies on the preparation technology and storage stability of active microbial tablets of Lactobacillus plantarum with lead toxicity-alleviating activities. J. Chin. Inst. Food Sci. Technol. 6, 71–78.
Loghman-Adham, M. (1997). Renal effects of environmental and occupational lead exposure. Environ. Health Perspect. 105, 928–938. doi: 10.1289/ehp.97105928
Lu, J., Wu, D. M., Zheng, Z. H., Zheng, Y. L., Hu, B., and Zhang, Z. F. (2011). Troxerutin protects against high cholesterol-induced cognitive deficits in mice. Brain 134, 783–797. doi: 10.1093/brain/awq376
Mahmoud, E. A., Bendas, E. R., and Mohamed, M. I. (2009). Preparation and evaluation of self-nanoemulsifying tablets of carvedilol. AAPS PharmSciTech 10, 183–192. doi: 10.1208/s12249-009-9192-7
Mehana, E. E., Meki, A. R., and Fazilic, K. M. (2012). Ameliorated effects of green tea extract on lead induced liver toxicity in rats. Exp. Toxicol. Pathol. 64, 291–295. doi: 10.1016/j.etp.2010.09.001
Noroozi, M., Angerson, W. J., and Lean, M. E. (1998). Effects of flavonoids and vitamin C on oxidative DNA damage to human lymphocytes. Am. J. Clin. Nutr. 67, 1210–1218. doi: 10.1093/ajcn/67.6.1210
Paternain, J., Ortega, A., Domingo, J., Llobet, J., and Corbella, J. (1990). Oral meso-2, 3-dimercaptosuccinic acid in pregnant sprague-dawley rats: teratogenicity and alterations in mineral metabolism. II. Effect on mineral metabolism. J. Toxicol. Environ. Health A 30, 191–197. doi: 10.1080/15287399009531422
Patra, R. C., Swarup, D., and Dwivedi, S. K. (2001). Antioxidant effects of alpha tocopherol, ascorbic acid and L-methionine on lead induced oxidative stress to the liver, kidney and brain in rats. Toxicology 162, 81–88. doi: 10.1016/s0300-483x(01)00345-6
Patterson, C., Ericson, J., Maneakrichten, M., and Shirahata, H. (1991). Natural skeletal levels of lead in Homo sapiens sapiens uncontaminated by technological lead. Sci. Total Environ. 107, 205–236. doi: 10.1016/0048-9697(91)90260-l
Pinto-Sanchez, M. I., Hall, G. B., Ghajar, K., Nardelli, A., Bolino, C., Lau, J. T., et al. (2017). Probiotic bifidobacterium longum NCC3001 reduces depression scores and alters brain activity: a pilot study in patients with irritable bowel syndrome. Gastroenterology 153, 448–459. doi: 10.1053/j.gastro.2017.05.003
Porru, S., and Alessio, L. (1996). The use of chelating agents in occupational lead poisoning. Occup. Med. 46, 41–48. doi: 10.1093/occmed/46.1.41
Prasanthi, R. P. J., Devi, C. B., Basha, D. C., Reddy, N. S., and Reddy, G. R. (2010). Calcium and zinc supplementation protects lead (Pb)-induced perturbations in antioxidant enzymes and lipid peroxidation in developing mouse brain. Int. J. Dev. Neurosci. 28, 161–167. doi: 10.1016/j.ijdevneu.2009.12.002
Prasanthi, R. P. J., Reddy, G. H., and Reddy, G. R. (2006). Calcium or zinc supplementation reduces lead toxicity: assessment of behavioral dysfunction in young and adult mice. Nutr. Res. 26, 537–545. doi: 10.1016/j.nutres.2006.09.004
Redig, P. T., Lawler, E. M., Schwartz, S., Dunnette, J. L., Stephenson, B., and Duke, G. E. (1991). Effects of chronic exposure to sublethal concentrations of lead acetate on heme synthesis and immune function in red-tailed hawks. Arch. Environ. Contam. Toxicol. 21, 72–77. doi: 10.1007/bf01055559
Rendón-Ramírez, A. L., Maldonado-Vega, M., Quintanar-Escorza, M. A., Hernández, G., Arévalo-Rivas, B. I., Zentella-Dehesa, A., et al. (2014). Effect of vitamin E and C supplementation on oxidative damage and total antioxidant capacity in lead-exposed workers. Environ. Toxicol. Pharmacol. 37, 45–54. doi: 10.1016/j.etap.2013.10.016
Shaban, E. M., and Said, E. Y. (2011). Influence of vitamin C supplementation on lead-induced histopathological alterations in male rats. Exp. Toxicol. Pathol. 63, 221–227. doi: 10.1016/j.etp.2009.12.003
Silva, M. G., Aragão, T. P., Vasconcelos, C. F., Ferreira, P. A., Andrade, B. A., Costa, I. M., et al. (2011). Acute and subacute toxicity of Cassia occidentalis L. stem and leaf in Wistar rats. J. Ethnopharmacol. 136, 341–346. doi: 10.1016/j.jep.2011.04.070
Stohs, S. J., and Bagchi, D. (1995). Oxidative mechanisms in the toxicity of metal ions. Free Radic. Biol. Med. 18, 321–336. doi: 10.1016/0891-5849(94)00159-h
Sugita, M. (1978). The biological half-time of heavy metals. Int. Arch. Occup. Environ. Health 41, 25–40. doi: 10.1007/bf00377797
Tangpong, J., Satarug, S., Satarug, S., and Gobe, G. (2010). Alleviation of lead poisoning in the brain with aqueous leaf extract of the Thunbergia laurifolia (Linn.). Toxicol. Lett. 198, 83–88. doi: 10.1016/j.toxlet.2010.04.031
Tian, F., Zhai, Q., Zhao, J., Liu, X., Wang, G., Zhang, H., et al. (2012). Lactobacillus plantarum CCFM8661 alleviates lead toxicity in mice. Biol. Trace Elem. Res. 150, 264–271. doi: 10.1007/s12011-012-9462-1
Waggas, A. M. (2012). Grape seed extract (Vitisvinifera) alleviate neurotoxicity and hepatotoxicity induced by lead acetate in male albino rats. J. Behav. Brain Sci. 2, 176–184. doi: 10.4236/jbbs.2012.22021
Wang, G., and Fowler, B. A. (2008). Roles of biomarkers in evaluating interactions among mixtures of lead, cadmium and arsenic. Toxicol. Appl. Pharmacol. 233, 92–99. doi: 10.1016/j.taap.2008.01.017
Watson, J. L., Ansari, S., Cameron, H., Wang, A., Akhtar, M., and Mckay, D. M. (2004). Green tea polyphenol (-)-epigallocatechin gallate blocks epithelial barrier dysfunction provoked by IFN-γ but not by IL-4. Am. J. Physiol. Gastrointest. Liver Physiol. 287, G954–G961. doi: 10.1152/ajpgi.00302.2003
Winder, C., Carmichael, N. G., and Lewis, P. D. (1982). Effects of chronic low level lead exposure on brain development and function. Trends Neurosci. 5, 207–209. doi: 10.1016/0166-2236(82)90116-3
Xia, D., Yu, X., Liao, S., Shao, Q., Mou, H., and Ma, W. (2010). Protective effect of Smilax glabra extract against lead-induced oxidative stress in rats. J. Ethnopharmacol. 130, 414–420. doi: 10.1016/j.jep.2010.05.025
Yin, R., Zhai, Q., Yu, L., Xiao, Y., Wang, G., Yu, R., et al. (2016). The binding characters study of lead removal by Lactobacillus plantarum CCFM8661. Eur. Food Res. Technol. 242, 1621–1629. doi: 10.1007/s00217-016-2661-9
Yin, S. T., Tang, M. L., Su, L., Chen, L., Hu, P., Wang, H. L., et al. (2008). Effects of Epigallocatechin-3-gallate on lead-induced oxidative damage. Toxicology 249, 45–54. doi: 10.1177/0960327110392087
Zhai, Q., Narbad, A., and Chen, W. (2015). Dietary strategies for the treatment of cadmium and lead toxicity. Nutrients 7, 552–571. doi: 10.3390/nu7010552
Zhai, Q., Tian, F., Zhao, J., Zhang, H., Narbad, A., and Chen, W. (2016). Oral administration of probiotics inhibits absorption of the heavy metal cadmium by protecting the intestinal barrier. Appl. Environ. Microbiol. 82, 4429–4440. doi: 10.1128/AEM.00695-16
Zhai, Q., Wang, G., Zhao, J., Liu, X., Narbad, A., Chen, Y. Q., et al. (2014). Protective effects of Lactobacillus plantarum CCFM8610 against chronic cadmium toxicity in mice indicate routes of protection besides intestinal sequestration. Appl. Environ. Microbiol. 80, 4063–4071. doi: 10.1128/AEM.00762-14
Keywords: lead toxicity, dietary supplements, Lactobacillus plantarum, micronutrients, plant extracts
Citation: Zhai Q, Yang L, Zhao J, Zhang H, Tian F and Chen W (2018) Protective Effects of Dietary Supplements Containing Probiotics, Micronutrients, and Plant Extracts Against Lead Toxicity in Mice. Front. Microbiol. 9:2134. doi: 10.3389/fmicb.2018.02134
Received: 27 May 2018; Accepted: 21 August 2018;
Published: 11 September 2018.
Edited by:
Zhihong Sun, Inner Mongolia Agricultural University, ChinaReviewed by:
Jun Tian, Jiangsu Normal University, ChinaGi-Seong Moon, Korea National University of Transportation, South Korea
Copyright © 2018 Zhai, Yang, Zhao, Zhang, Tian and Chen. This is an open-access article distributed under the terms of the Creative Commons Attribution License (CC BY). The use, distribution or reproduction in other forums is permitted, provided the original author(s) and the copyright owner(s) are credited and that the original publication in this journal is cited, in accordance with accepted academic practice. No use, distribution or reproduction is permitted which does not comply with these terms.
*Correspondence: Fengwei Tian, fwtian@jiangnan.edu.cn
†These authors have contributed equally to this work