- 1Department of Entomology, Nanjing Agricultural University, Nanjing, China
- 2School of BioSciences, Bio21 Institute, The University of Melbourne, Parkville, VIC, Australia
Wolbachia in host germ lines are essential for their vertical transmission to the next generation. It is unclear how the regulation of host oocyte development influences Wolbachia location and the mechanistic basis of transmission. Here, we investigated whether vitellogenin influences Wolbachia transmission in Laodelphax striatellus. Wolbachia increased in density and spread from the anterior tropharium to developing oocytes as ovaries developed. Microscopic observations indicated that Wolbachia invaded ovarioles from the tropharium of its anterior side rather than the pedicel side. Wolbachia utilized the host Vg transovarial transportation system to enter the ovaries and were transmitted from the tropharium into the developing oocytes through nutritive cords. These observations were supported by knocking down the Vg transcript, in which low Wolbachia titers were detected in ovaries and fewer Wolbachia were transmitted into oocytes. Our findings establish a link between the Vg-related mode of transovarial transmission and efficient maternal transmission of Wolbachia.
Introduction
Wolbachia bacteria that occur intracellularly in reproductive tissues of a wide range of arthropods (O’Neill et al., 1992; Rousset et al., 1992; Werren et al., 1995) are vertically transmitted from parents to offspring in a transovarial manner. The transmission process controls the ability of Wolbachia to persist and disperse (Schmidt et al., 2017), particularly when coupled with effects on their hosts that enhance Wolbachia transmission, including induction of cytoplasmic incompatibility between individuals (Breeuwer and Werren, 1990; Rousset and Raymond, 1991; Turelli and Hoffmann, 1995), male-killing(Hurst et al., 1999), parthenogenesis induction (Stouthamer et al., 1993), feminization (Rousset et al., 1992), and positive host fitness effects (Weeks et al., 2007). To be vertically transmitted, Wolbachia need to invade host reproductive tissues to ensure their presence in the germ line.
Wolbachia symbionts are localized in diverse cells and tissues (Dobson et al., 1999), exhibiting strong tropism (Toomey et al., 2013). Although Wolbachia have been detected in host somatic tissues, they are primarily found in the host germ line (Dobson et al., 1999; Clark et al., 2002; Ijichi et al., 2002). In male arthropods, Wolbachia are present within developing spermatocytes (Bressac and Rousset, 1993; Clark et al., 2002; Ijichi et al., 2002), but they are not transmitted through sperm. In female arthropods, Wolbachia are transmitted to eggs, where they persist through embryogenesis and eventually become incorporated in the precursors of germ line stem cells (Kose and Karr, 1995; Ferree et al., 2005). Wolbachia accumulate in the germ line stem cells in long-term maternally infected Drosophila mauritiana (Fast et al., 2011). In D. melanogaster infected with Wolbachia through microinjection, Wolbachia enter the germ line through somatic stem cells (Frydman et al., 2006). Both host- and bacterial- derived factors appear to influence Wolbachia’s tropism during oogenesis (Toomey et al., 2013; Hughes et al., 2014).
Besides Wolbachia, other microbes including viruses, microsporidia, and fungi can be maternally transmitted (Douglas, 1989). Spiroplasma in D. melanogaster achieve vertical transmission by cooption of the yolk uptake machinery (Herren et al., 2013). In planthoppers, these microbes have evolved strategies to enter the reproductive tissues of the host involving vitellogenin (Vg). Vg, a precursor of egg yolk protein, is associated with egg maturation, embryonic growth and oviposition capacity (Dong et al., 2007; Kawakami et al., 2009; Guo et al., 2012). After its synthesis by fat body, Vg is secreted into hemolymph, absorbed into ovaries through wide channels formed between epithelium cells, then transferred toward the oocyte surface and into the oocyte via receptor-mediated endocytosis (Davey, 1993; Zimowska et al., 1994). There is a transovarial transportation system for absorbing Vg in the oviparous insects. Yeast-like symbionts (YLS) in brown planthoppers are transovarially transmitted by being wrapped in vitellogenin outside the ovary (Cheng and Hou, 2005). In the small brown planthopper Laodelphax striatellus (Hemiptera: Delphacidae), rice stripe virus (RSV) can be transmitted to oocytes only when the Vg receptor is present (Huo et al., 2014). Maternal transmission of these microbes in planthoppers is therefore potentially related to Vg, and this protein may also be associated with the efficient maternal transmission of Wolbachia.
The small brown planthopper, L. striatellus is an important pest in rice fields and is 100% infected with Wolbachia strain wStri in China (Zhang et al., 2013). Wolbachia transovarial transmission frequencies were determined to be 100% from mother to offspring (Noda et al., 2001; Zhang et al., 2013), which indicates that Wolbachia are capable of efficient transmission via L. striatellus ovaries. L. striatellus ovarioles are of the telotrophic meroistic type, which consist of a terminal filament, tropharium, and vitellarium (Figure 1). In anterior tropharium, a cluster of nurse cells are radially arranged around and connected to the central trophic core; previtellogenesis are arranged on the base of the tropharium (Szklarzewicz et al., 2007). The vitellarium house 3-4 linearly arranged developing oocytes which absorb nutrients from nurse cells through nutritive cords that connect nurse cells and oocytes (Szklarzewicz et al., 2009; Szklarzewicz et al., 2013). Based on oogenesis development, ovaries can be divided into four stages: previtellogenic, early vitellogenic, vitellogenic, and postvitellogenic stages (Dong et al., 2011). Wolbachia titers increased in females after L. striatellus adult emergence as ovaries developed (Noda et al., 2001). However, the mechanism by which Wolbachia spreads into host ovaries is unknown, and host proteins that are involved in Wolbachia overcoming barriers to transovarial transmission have not been directly characterized.
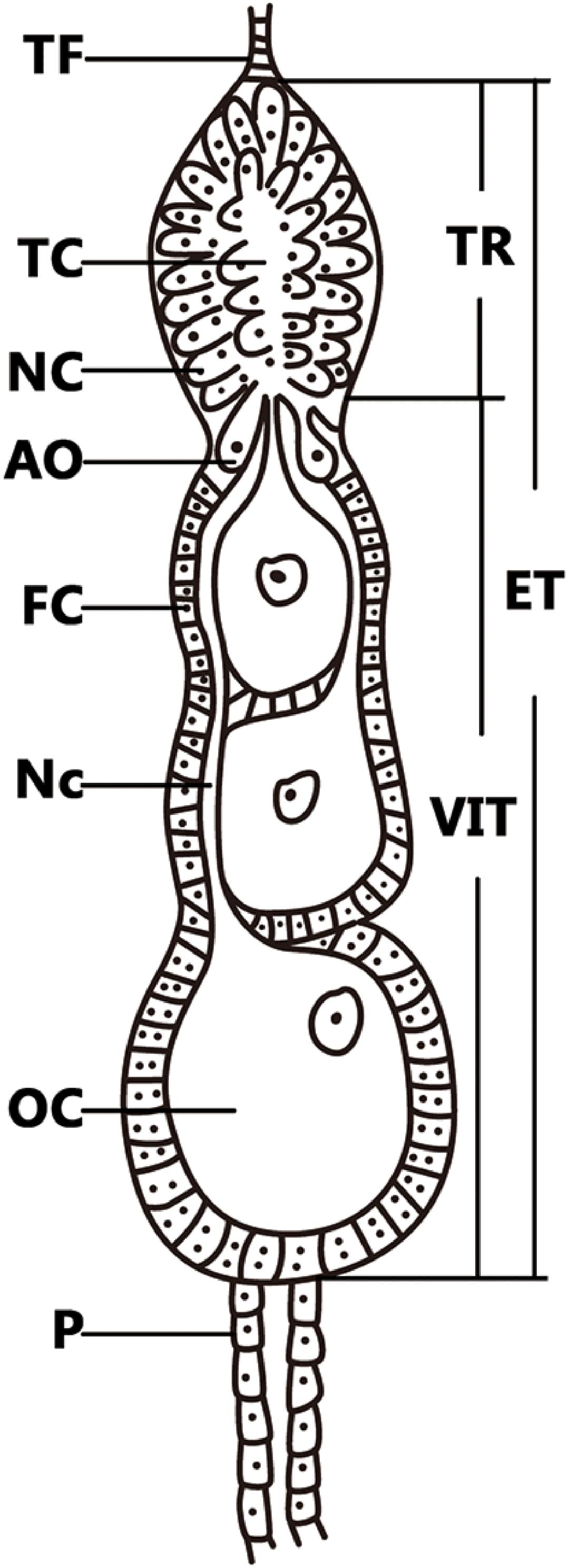
FIGURE 1. Telotrophic meroistic ovaries of L. striatellus. The L. striatellus ovariole is composed of a terminal filament (TF) at the tip, followed by the egg tube (ET). The tropharium (TR) is at the tip of the egg tube, which is composed of nurse cells (NC) and a trophic core (TC); just below the tropharium is the vitellarium (VIT), in which the oocyte (OC) is completed and there is a pedicel (P) at the bottom. The posterior region of the tropharium contains 6–10 arrested oocytes (AO). The vitellarium houses linearly arranged developing oocytes each of which is connected to the trophic core by a broad nutritive cord (Nc). Each oocyte is surrounded by a single layer of follicular cells (FC) that become binucleate at the beginning of vitellogenesis.
Here we describe how Wolbachia is transmitted to L. striatellus oocytes and where the bacteria are located in the ovarioles. Our results provide a model for Wolbachia transovarial transmission into host telotrophic meroistic-type ovaries, and they indicate that the host Vg transovarial transportation system plays a role in this process.
Materials and Methods
Insects
The Wolbachia-infected L. striatellus population was originally collected from rice fields in Nanjing, Jiangsu, China, and maintained in an incubator at 26 ± 1°C under a 16: 8 h light: dark photoperiod and 60% relative humidity on rice variety TN1 rice plants. Before experiments, the genotype of Wolbachia strain wStri was determined by cloning and sequencing five multilocus sequence typing genes and wsp, as previously described (Zhang et al., 2013). To ensure a similar nuclear background, all Wolbachia-infected insects were from offspring of one infected pair.
Ovarian Development
Laodelphax striatellus ovarian development was divided into previtellogenic, early vitellogenic, vitellogenic, and postvitellogenic different stages, as described in Nilaparvata lugens (Dong et al., 2011), which is related to L. striatellus. Nearly no Vg is transported into the previtellogenic ovaries of adults within 12 h after eclosion. Most ovarioles were in early vitellogenesis within 1–2 days after eclosion when Vg began to be transported into oocytes from hemolymph; they were transparent and there was little yolk protein deposition in the oocytes. Vitellogenic ovarioles contain one white and opaque oocyte, with nearly half of the oocytes filled with yolk protein and oocytes gradually increasing in size. Most ovarioles in the postvitellogenic ovaries contained one full-grown egg, which was completely filled with yolk granules and surrounded by the vitelline membrane.
Reverse-Transcriptase Quantitative PCR (RT-qPCR)
Nearly no egg was laid within 72 h after L. striatellus female eclosion. Vg expression levels in 5th instar nymphs and three stages of 1-, 2-, and 3-day-old adult female L. striatellus were measured by RT-qPCR. Total RNA was extracted using TRIZOL reagent (Takara) and converted to cDNAs using PrimeScriptTM RT reagent Kit with gDNA Eraser (Takara), according to the manufacturer’s instructions. Relative Vg mRNA levels were measured with the SYBR Primex Ex TapTM Kit (Takara Biotechnology) following the manufacturer’s instructions. RT-qPCR was performed in 20 μl reactions containing 10 μl SYBR Primex Ex Tap, 2 μl of diluted cDNA template, 0.4 μl of each primer, 0.4 μl ROX Reference Dye II, and 6.8 μl ddH2O, on a 7800 real-time system (Applied Biosystems) using the following procedures: denaturation at 95°C for 30 s, followed by 40 cycles of amplification (95°C for 5 s and 60°C for 34 s). The primers are listed in Supplementary Table 1. The dissociation curve was analyzed for both primer pairs, and all experimental samples had a single sharp peak at the amplicon’s melting temperature. The relative expression levels of the Vg gene were calculated according to the 2-ΔΔCT method (Livak and Schmittgen, 2001), and the actin gene of L. striatellus was used as a housekeeping gene. All the RT-qPCR experiments were repeated five times. All the data were presented as relative mRNA expression (mean ± SD).
qPCR
Wolbachia densities in 5th instar nymphs and three stages of 1-, 2-, and 3-day-old adult female L. striatellus ovaries were determined by qPCR.DNA extraction, qPCR protocols, and standard curves have been previously described (Lu et al., 2012). The primers (Supplementary Table 1) specifically designed for the surface protein gene wsp of wStri were used in qPCR to measure Wolbachia genome copy number. The Wolbachia wsp copy number was normalized to the host actin gene.
RNA Interference (RNAi)
Laodelphax striatellus Vg-specific nucleotide conserved sequence was cloned into the pGEM-T Easy vector (Promega). Aequorea victoria green fluorescent protein (GFP) was used as a control. The specific primers used to generate DNA templates are shown in Supplementary Table 1. dsRNA was synthesized, as previously described (Huang et al., 2015).
Second instar nymphs of infected L. striatellus were used in the RNAi microinjection. Each nymph was anesthetized using carbon dioxide. Approximately 250 ng of dsVg or dsGFP was microinjected into the thorax of each nymph using the FemtoJet Microinjection System (Eppendorf). Microinjected nymphs were reared under laboratory conditions. Relative silencing efficiency of the whole body was calculated by RT-qPCR. Wolbachia titers (ovaries and whole body) were measured by qPCR, as described above.
Immunofluorescence Staining
Ovaries in different development stages were stained as previously described (Frydman and Spradling, 2001; Ferree et al., 2005). Ovaries from Wolbachia-infected adult female L. striatellus were dissected in phosphate buffered saline (PBS) and separated into individual ovarioles. Ovarioles were fixed in 4% formaldehyde solution plus 0.1% Triton X-100 (Sigma) for 15 min and washed twice in PBS for 5 min each; this was followed by two 20-min washes in PBS containing 0.2% Triton X-100 and 1 U/ml of fluorescein isothiocyanate (FITC)-conjugated phalloidin (Sigma), and another 20-min wash in PBS containing 0.2% Triton X-100 without phalloidin. The ovaries were then rinsed in PBS alone, after which they were ready for antibody staining.
Wolbachia were stained with mouse antibody Hsp-60 (1:200) (Sigma). Primary antibodies were incubated overnight at 4°C. Following six 15-min washes in PBS with 0.1% Triton-X-100, ovaries were incubated at room temperature for 1 h with tetramethylrhodamine isothiocyanate (TRITC)-coupled anti-mouse antibodies (1:200) (Sigma). After incubation, the ovarioles were washed in PBS and mounted on a glass slide with the 4′, 6-diamidino-2-phenylindole (DAPI)-containing mounting Vectashield (Vector Laboratories).
Images were generated using a Zeiss LSM-800 confocal laser scanning microscope. Care was taken to normalize the exposure times across all experiments. Taking in consideration that each Wolbachia cell was about 1.0 μm in diameter, Wolbachia titers in ovaries were scanned with Z-series stacks at 1.0-μm intervals.
Results
Wolbachia Increase Is Correlated With Vg
Wolbachia titers were detected in 5th instar nymphs and three stages of 1-, 2-, and 3-day-old adult female L. striatellus ovaries. The timing of Vg gene expression in Wolbachia-infected adult female L. striatellus was detected by RT-qPCR. Wolbachia titers in ovaries and Vg gene expression levels increased with female age at a background level in the 5th instar nymph (Figure 2A). Weakly increased Wolbachia titers were observed in the 1-day-old adult female when most ovarioles were in early vitellogenic stage. Wolbachia titers in ovaries and Vg expression sharply increased in the 2-day-old adult female and continuously increased in 3-day-old adult female. The higher Vg expression levels with age of female adults coincided with higher Wolbachia titers in the L. striatellus ovaries (Figure 2B), as expected based on previous results (Noda et al., 2001; Huo et al., 2014). These results suggest that higher Wolbachia titers in ovaries may be mediated by Vg transmission in L. striatellus.
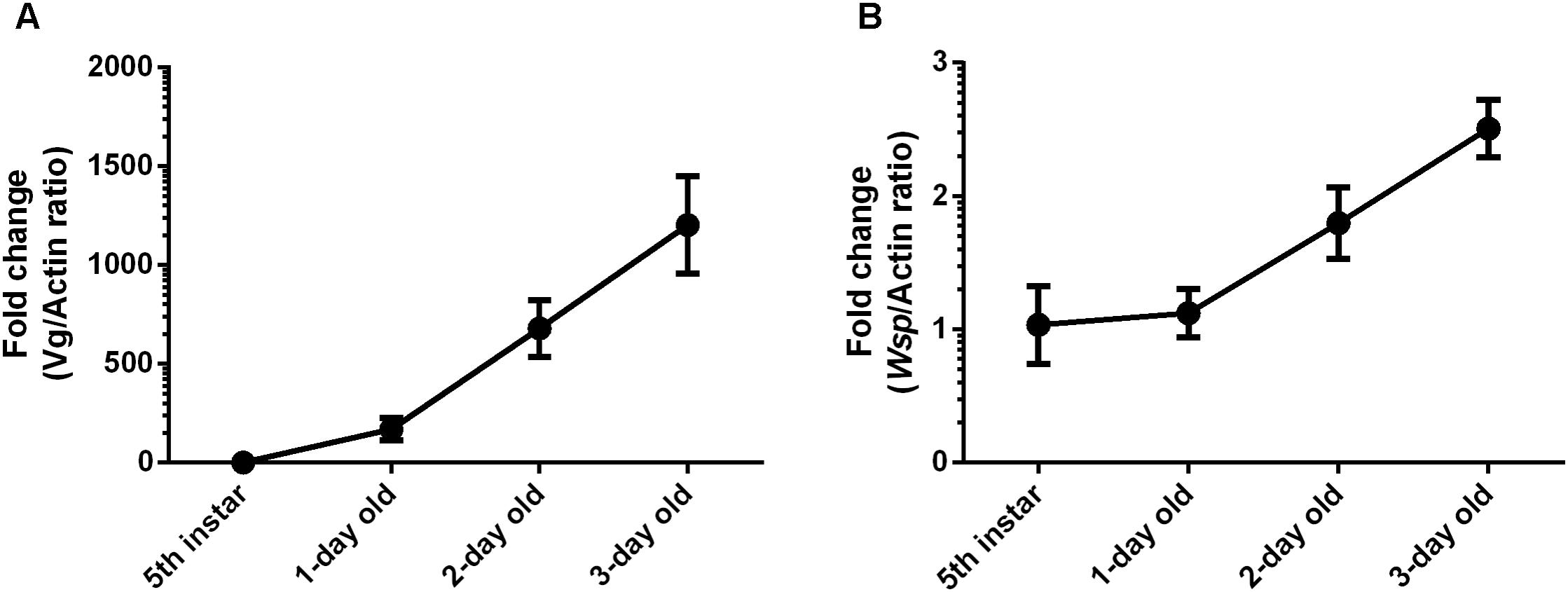
FIGURE 2. Wolbachia titers in L. striatellus ovaries at different developmental stages. (A) Vg gene transcript levels in L. striatellus. (B) Wolbachia wsp genome copies in L. striatellus ovaries. Vg mRNA expression levels in L. striatellus and wsp copy number in L. striatellus ovaries were measured in 5th instar nymphs and adults females at different emergence times (1, 2, and 3 days old). Results are expressed as fold change relative to 5th instar nymphs. Bars show the average fold change per experiment ± SD.
Wolbachia Are Transported as Ovaries Develop
To identify a possible functional role of Vg in the transovarial transmission of Wolbachia, we observed the presence of Wolbachia in ovaries and ovarioles at different developmental stages. We did not observe any Wolbachia signals in the ovarioles at the previtellogenic stage when Vg was not absorbed into ovaries. Wolbachia was found only in the terminal filaments and pedicels (Supplementary Figure 1). Ovaries in previtellogenic stage were difficult to keep intact during staining, and there was no whole ovaries image got.
Wolbachia was first detected in the ovariole tropharium of adults at early vitellogenic ovaries when Vg began to be absorbed into ovaries (Figure 3). Wolbachia accumulated in the ovariole tropharium (Figure 3B, arrowhead) and was distributed among all cells (Figure 3B, arrowhead), including the centrally located trophic core and nurse cells (Szklarzewicz et al., 2007). These results suggest that Wolbachia may be transported into the tropharium at an early developmental stage, establishing an early infection.
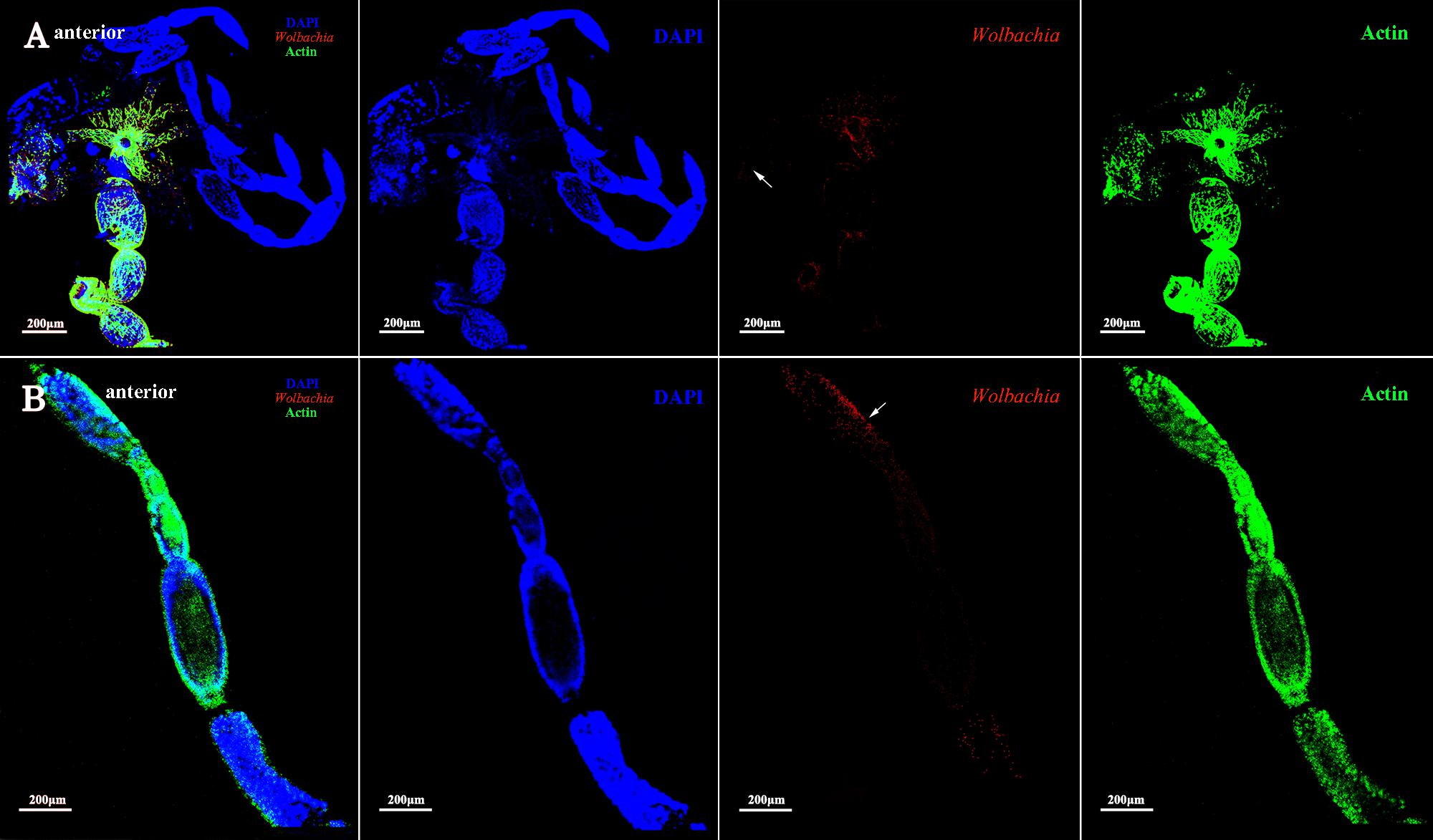
FIGURE 3. Wolbachia entering the early vitellogenic ovaries. Morphology of the structure and organization of ovaries (A) and ovarioles (B) of early vitellogenic adults stained with FITC-Phalloidin (green) and DAPI (blue). Wolbachia stained with an antibody to Wolbachia heat shock protein 60 (Hsp-60). Arrowheads point to Wolbachia aggregated within ovaries and ovarioles. Red: Wolbachia; green: actin; blue: L. striatellus DNA.
The development of vitellogenic ovaries are divided into vitellogenic I and vitellogenic II stages according to ovarioles development. Vitellogenic I ovaries contain one developing oocyte. At this development stage, high quantities of Vg are incorporated into developing oocytes (Huo et al., 2014). Wolbachia signals were observed in the posterior tropharium, which is occupied by prefollicular cells and several arrested oocytes, and in the ooecium of anterior vitellarium, which had arrested and developing oocytes surrounded by follicular cells (Figure 4, arrowheads). These results show that Wolbachia migrated from the tropharium to the vitellarium in vitellogenic I ovarioles.
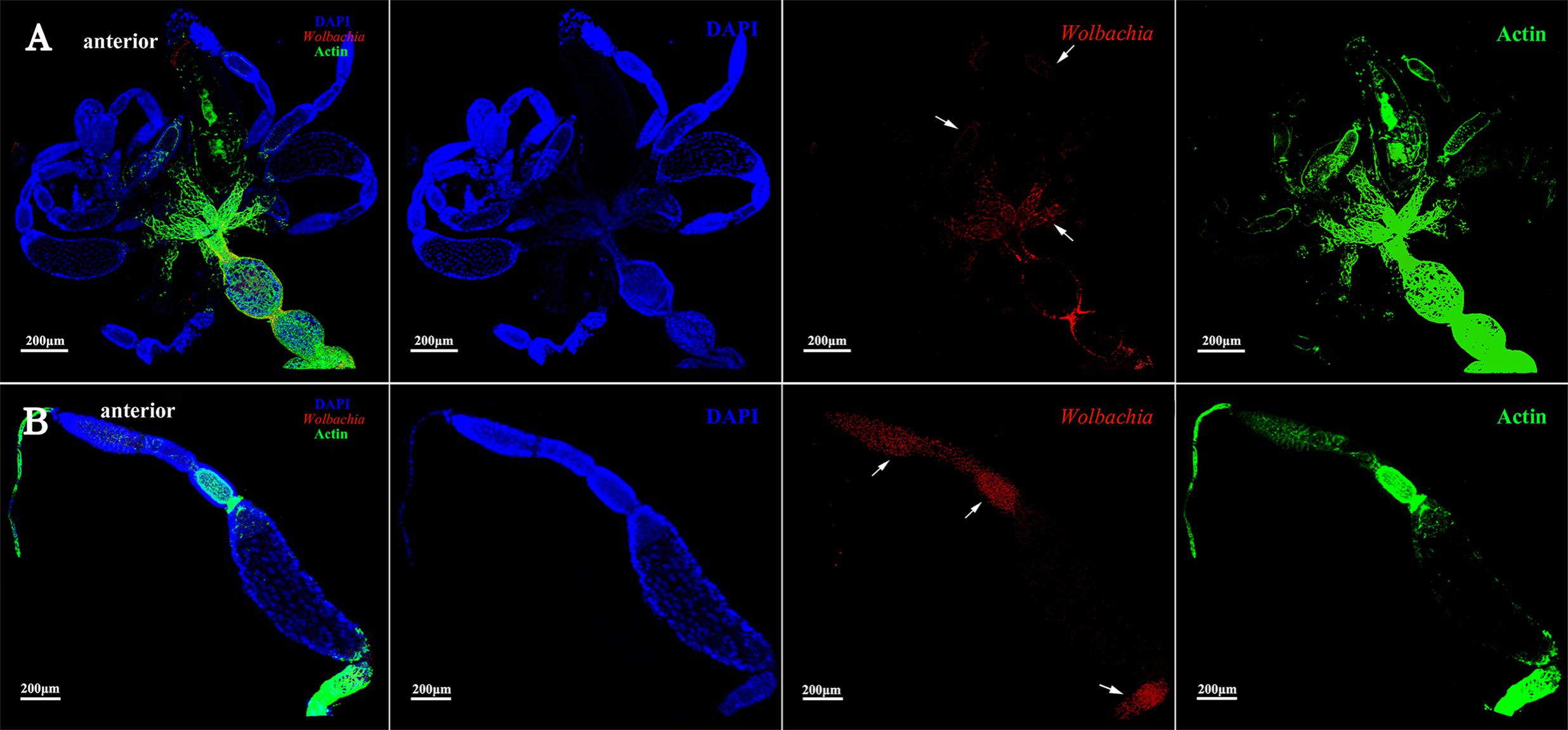
FIGURE 4. Wolbachia localization in vitellogenic I ovaries. Wolbachia in ovaries (A) and ovarioles (B) of vitellogenic I adults were observed by antibody Hsp-60 (red). White arrowheads point that Wolbachia within the posterior tropharium, anterior vitellarium, and pedicel. Red: Wolbachia; green: actin; blue: L. striatellus DNA.
The vitellarium of vitellogenic II ovarioles house one developing oocyte which is nearly matured (Figure 5A). Wolbachia signals were concentrated at the anterior pole region in the developing oocytes (Figure 5B, arrowheads). Wolbachia were also observed in the ooecium of arrested oocytes. Obviously, Wolbachia signals concentrated around the arrested oocytes (Figure 5B, arrowheads), where is an area of nutritive cords connecting nurse cells. These observations suggest that Wolbachia has successfully invaded the developing oocytes from tropharium.
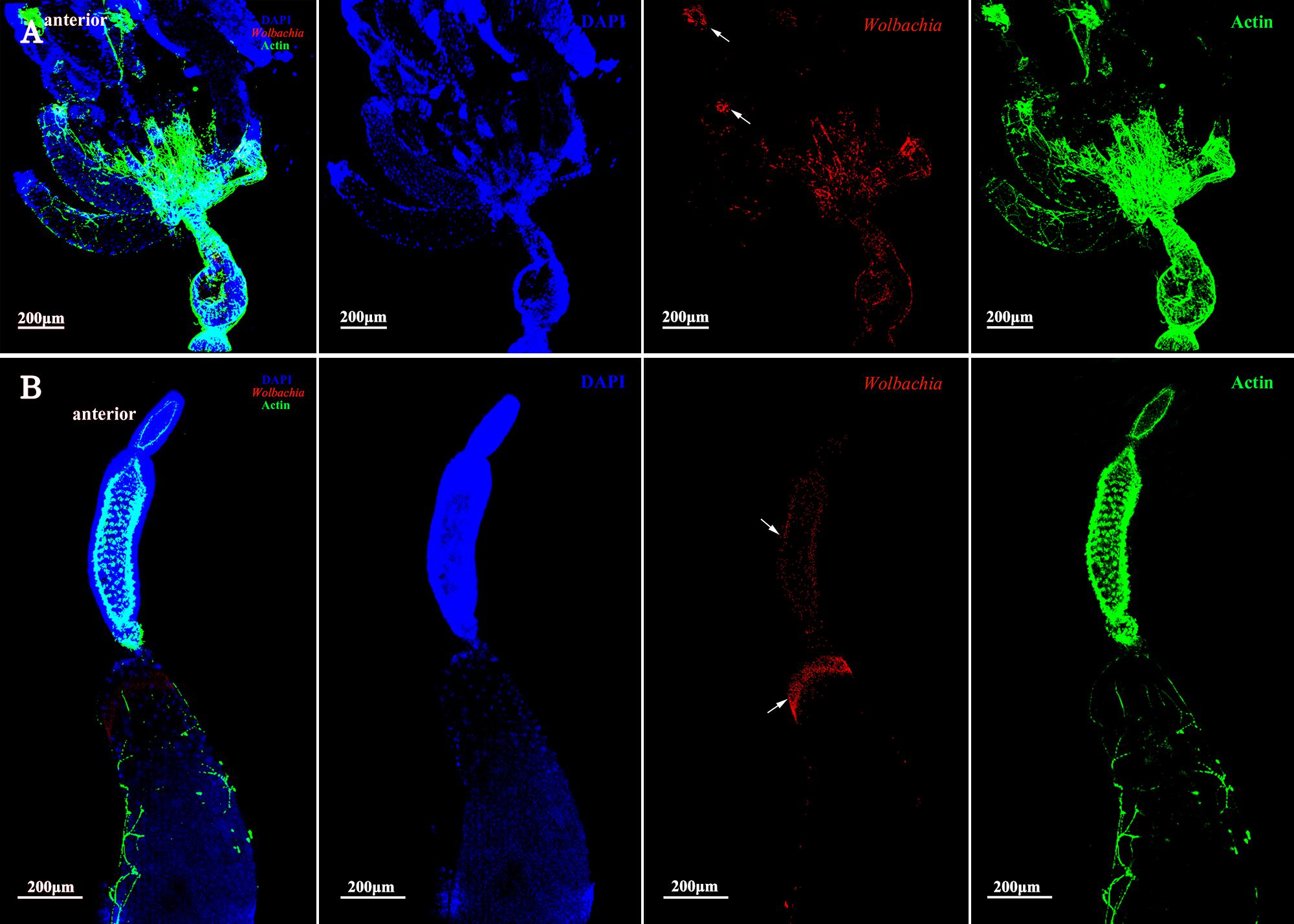
FIGURE 5. Wolbachia distribution in vitellogenic II ovaries. Ovaries (A) and ovarioles (B) of vitellogenic II adults were observed. White arrowheads indicate Wolbachia concentrated around the arrested oocytes and the anterior region of developing oocyte. Red: Wolbachia; green: actin; blue: L. striatellus DNA.
Ovarioles at postvitellogenic stage contain one matured oocyte and Vg transmission is nearly finished. At this developmental stage, most Wolbachia were observed in the anterior vitellarium (Figure 6B, arrowheads), in which the next oocytes were developing. Wolbachia in the matured oocytes kept the anterior region (Figure 6B, arrowheads). Wolbachia signals were also observed in the pedicel and oviduct (Figure 6A, arrowheads). These results suggest that Wolbachia transmission into oocytes have finished as oocytes matured.
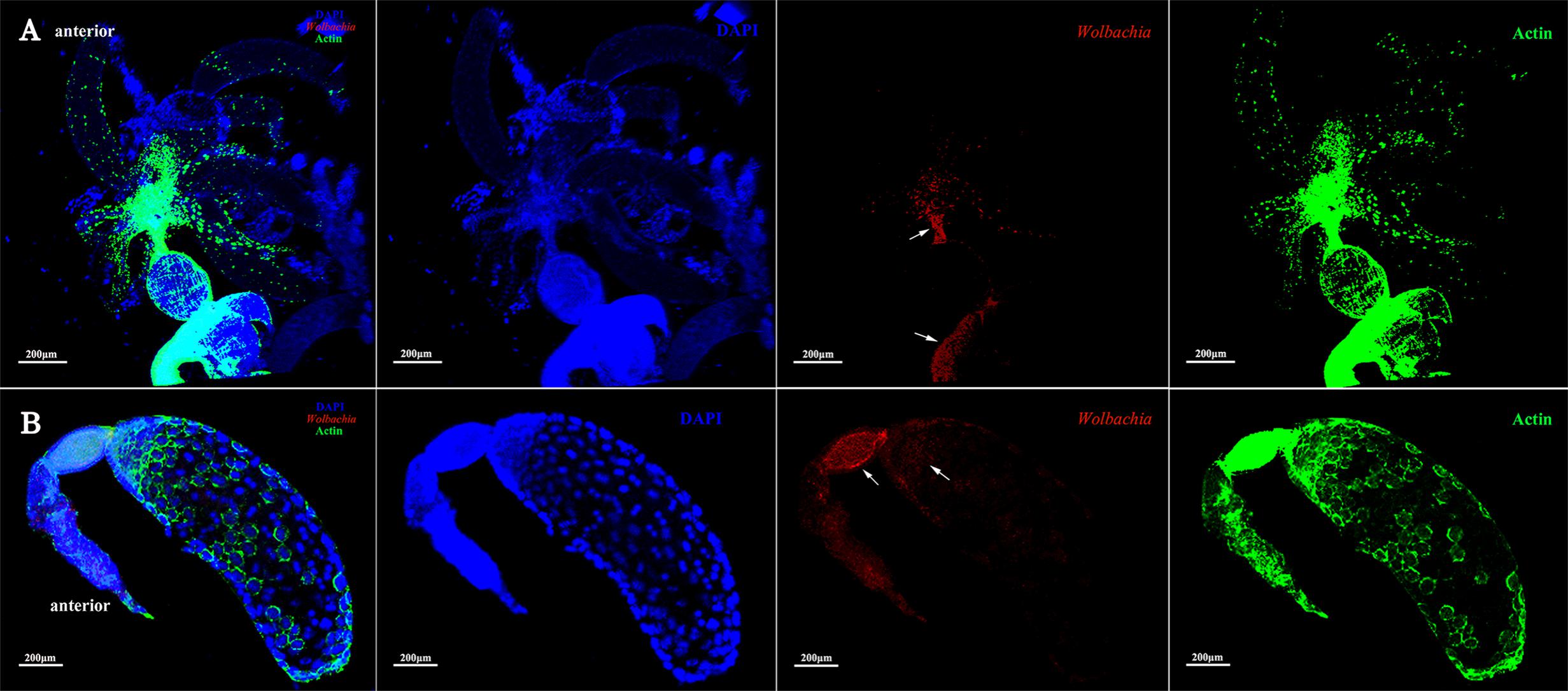
FIGURE 6. Wolbachia spread in postvitellogenic ovaries. Postvitellogenic ovaries (A) and ovarioles (B) infected with Wolbachia (red). Arrowheads point to Wolbachia localized in adult pedicels and oviducts. Wolbachia signals were also observed in the anterior vitellarium. Red: Wolbachia; green: actin; blue: L. striatellus DNA.
Wolbachia Transferred Into Oocytes From the Tropharium
Immunohistochemistry using a Wolbachia Hsp-60 antibody revealed characteristic localization patterns of Wolbachia in the ovarioles, which were suggestive of the route and mechanism of its vertical transmission. At the tip of the ovarioles, the Wolbachia signals were found inside the terminal filament and nurse cells in the anterior pole of the tropharium (Figures 7A,B, arrowheads). We also show that Wolbachia are present in the extracellular space between nurse cells (Figures 7A,B, arrowheads). Wolbachia became dispersed throughout the ooecium of arrested oocytes (Figures 7A,C, arrowheads). However, a few arrested oocytes exhibited a residual accumulation of Wolbachia at the posterior region (Figures 7A,D, arrowheads), which may facilitate stable vertical transmission of Wolbachia. Wolbachia were also found in nutritive cords connected to nurse cells and developing oocytes (Figure 7D, arrowheads and Supplementary Figure 2), which indicated that Wolbachia in the nurse cells may spread into the developing oocytes binding with Vg protein through nutritive cords. The nutritive cords disappeared as the oocytes matured (Figures 7A,E, arrowheads). Many Wolbachia accumulated in the next developing oocytes rather than in matured oocytes (Figures 7A,E,F, arrowheads), which indicates that Wolbachia transmission was prevented during the completion of oocyte development. In matured oocytes, Wolbachia localized within the anterior region and the surrounding follicular cells (Figures 7A,F,G, arrowheads). Many Wolbachia were observed in the pedicels (Figures 7A,H, arrowheads), while smaller numbers were located in the posterior region of the matured oocytes (Figures 7A,G, arrowheads). These observations indicated that Wolbachia invaded oocytes from tropharium nurse cells on its anterior side rather than the pedicel side, pointing to a barrier between the pedicel and vitellarium preventing Wolbachia entry into the oocyte directly from the pedicel.
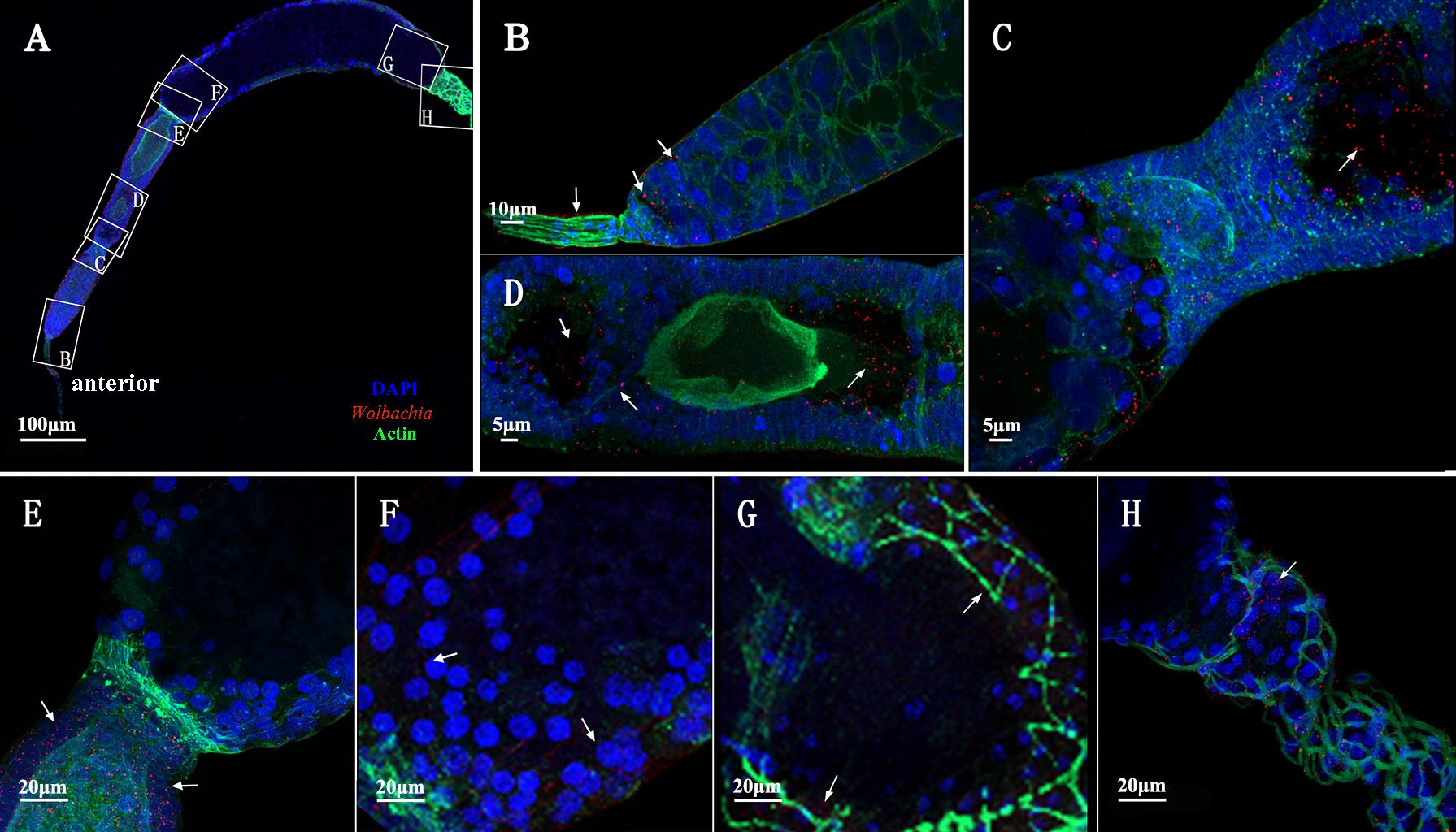
FIGURE 7. Wolbachia transferred into oocytes. (A) Representative ovariole include developing and matured oocytes. Higher magnification of the terminal filament and stem cells in the tropharium (B), the connection between the tropharium and vitellarium (C), developing oocyte (D), the connection between developing and matured oocyte (E), anterior region of the matured oocyte (F), posterior region of the matured oocyte (G), and pedicel (H). White arrowheads indicate Wolbachia. Red: Wolbachia; green: actin; blue: L. striatellus DNA.
Reduced Vg Expression Affected Wolbachia Invasion
To confirm that Vg has a role in transovarial transmission of Wolbachia, we knocked down Vg expression using RNAi by microinjecting dsRNA for Vg (dsVg) into the L. striatellus body with dsGFP as a control. Compared with dsGFP-microinjected insects, the Vg mRNA level in dsVg-microinjected 2-day-old emerged female insects decreased by approximately 87% throughout the whole body (Figures 8A). We used Wolbachia wsp qPCR primers to measure the total number of wsp copies, which revealed that the Wolbachia titers significantly decreased by 80% in the dsVg-microinjected insect ovaries compared with that of the control (Figure 8B). However, the Wolbachia titers in the whole body of dsVg-microinjected insects did not differ greatly among the treatments (Figure 8C), which indicates that knockdown of Vg expression by RNAi specifically affected Wolbachia invasion in the L. striatellus ovaries. Moreover, the dsVg-treated females failed to produce offspring (Supplementary Figure 3), consisting with previous finding (Huo et al., 2014).
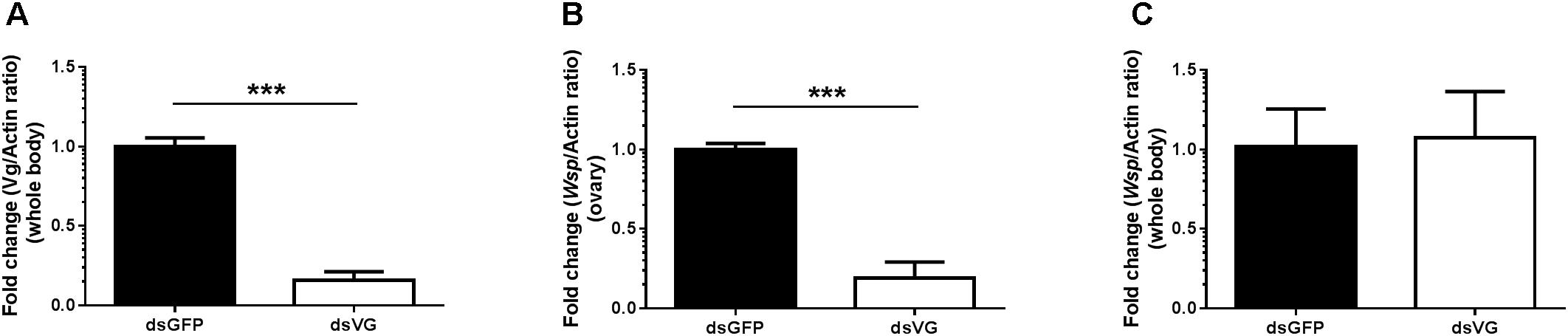
FIGURE 8. Wolbachia titers in dsVg-microinjected L. striatellus. Second instar nymphs were microinjected with Vg dsRNA or dsGFP as a control. After dsRNA injection, 2-day-old emerged female insects were selected to detect Vg expression in the whole body (A). Wolbachia density significantly decreased in the dsVg-injected ovaries of 2-day-old adult insects (B) and did not differ in the whole body (C). Bars show the average fold change per experiment ± SD. (∗∗∗P < 0.001).
We did not observe differences in degree of development among ovaries dissected from the treated insects. However, as Vg expression was reduced after dsVg injection, the ooecium of oocyte was smaller, and the oocyte in each ovariole was less distinct than that in the dsGFP-treated insects (Figures 9A,D, arrowheads and Supplementary Figure 4). Wolbachia distribution in the 2-day-old treated adult insect ovarioles was examined. Using immunofluorescence, we observed that Wolbachia were irregularly dispersed throughout the whole ovariole, including in the terminal filament (Figures 9A,B, arrowheads), tropharium (Figures 9A,C, arrowheads), and vitellarium (Figures 9A,D, arrowheads). The Wolbachia densities were lower in dsVg-treated than in the dsGFP-treated ovarioles (Supplementary Figure 5). All of these results suggest that Vg expression reduction by RNAi prevented Wolbachia transmission into L. striatellus ovaries.
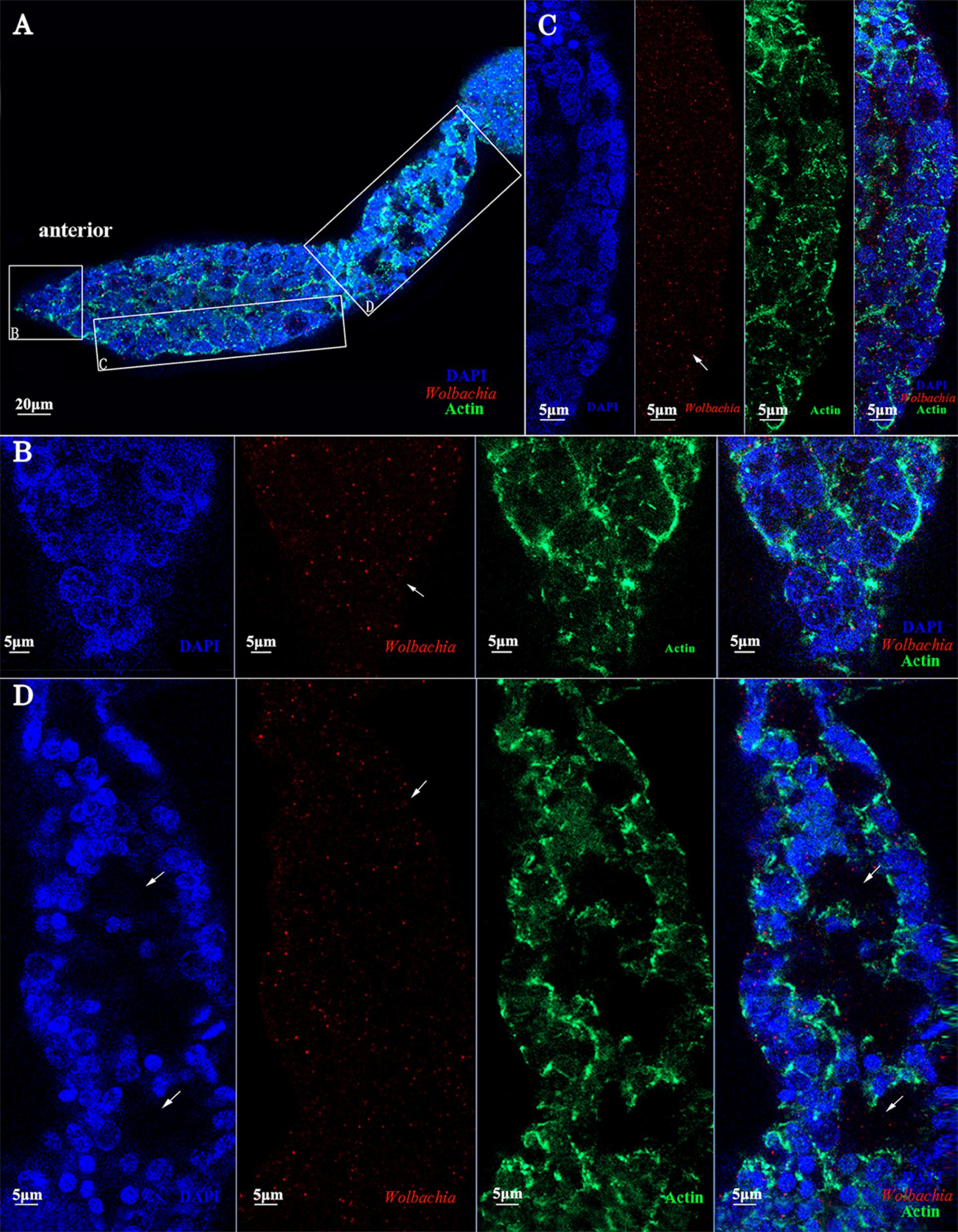
FIGURE 9. dsVg microinjection reduced the Wolbachia titer in ovaries. Low Wolbachia densities were detected in ovarioles of dsVg-treated 2-day-old emerged female insects (A), including in the terminal filament (B), tropharium (C), and vitellarium (D). Wolbachia dispersed throughout the whole ovariole irregularly. Red: Wolbachia; green: actin; blue: L. striatellus DNA.
Discussion
Wolbachia infect an estimated 40–69% of arthropod species (Hilgenboecker et al., 2008). For Wolbachia to become established in a new host species, it must be horizontally acquired and vertically transmitted at a high rate (Turelli and Hoffmann, 1995). Regardless of how Wolbachia reach a new host, after the initial infection, they must reach the germ line for successful transmission to the next generation (Werren et al., 2008). Hence, their presence in the oocyte is essential for their vertical propagation to the next generation. In Drosophila, Wolbachia entry into oocyte has been intensively investigated (Ferree et al., 2005; Newton et al., 2015). During early oogenesis, Wolbachia are distributed among all of the germ cells in the germarium of ovarioles, allowing them to establish infection very early in development (Ferree et al., 2005). Within the germarium, the major route for Wolbachia to enter the germ cells is through the somatic stem cell niche. While little is known about these mechanisms and no mutation blocking Wolbachia vertical transmission has been previously described.
In the present study, we have investigated transmission in L. striatellus, which efficiently transmits Wolbachia given that all individuals tested so far were infected with Wolbachia wStri (Noda, 1984; Zhang et al., 2013). In this study, a high correlation was found between Vg and Wolbachia. Wolbachia may bind with Vg in the hemolymph and then be absorbed into ovaries via Vg receptor-mediated endocytosis. Before Vg were absorbed into ovarioles, Wolbachia was only observed in the terminal filaments and pedicels of previtellogenic ovaries (Supplementary Figure 1). As ovaries development, Wolbachia were dispersed throughout all cells in the tropharium of early vitellogenic ovaries (Figure 3) when Vg were absorbed into the ovaries from hemolymph via endocytosis. Then Wolbachia spread into all ooecium at vitellogenic and postvitellogenic stages (Figures 4–6). Moreover, the density of Wolbachia in the ovarioles increased with development (Supplementary Figure 6). It was obvious that Wolbachia location in ovarioles was correlated with the transportation of Vg, which suggested that Vg is important for Wolbachia to overcome the transovarial transmission barrier.
In insect, there is a transovarial transportation system for absorbing nutrients Vg (Huo et al., 2018). Wolbachia in L. striatellus may use Vg existing transport systems for efficiently transovarial transmission. Wolbachia and Vg bind together in the hemolymph, and were transported into the tropharium via wide channels formed between nurse cells, endocytosed into the central trophic core establishing an early infection at an early developmental stage. The distribution of Wolbachia in the ovarioles of dsVg-injected L. striatellus supports our results. Compared with the dsGFP-microinjected insects, dsVg injection obviously affected Wolbachia invasion into the ovaries (Figure 9). Wolbachia were irregularly dispersed throughout the whole ovariole (Figure 9), and the Wolbachia titer in the ovarioles was greatly decreased (Supplementary Figure 5). These results further suggested that Vg was specifically involved in Wolbachia invasion of the insect ovaries. However, the detailed functions of Vg in Wolbachia transmission and how Wolbachia binds to Vg remain unclear.
We first observed Wolbachia signals in the ovarioles of adults at early vitellogenic ovaries when Vg began to be transferred into ovaries (Figure 3). At this stage, Wolbachia was distributed among all cells in the anterior area of tropharium, including the centrally located trophic core and nurse cells (Figure 3B, arrowhead). In fruit fly, the anterior area of ovarioles is called germarium, where contain stem cells and further differentiate into oocytes and nurse cells (Ferree et al., 2005). Wolbachia reach oocytes through the stem cells in the germarium (Toomey et al., 2013). While oocytes are mainly differentiated from stem cells in L. striatellus nymph stage, when Vg is not highly expressed (Huo et al., 2014). Therefore, despite Wolbachia invading the tropharium at early vitellogenic stage L. striatellus, it does not seem possible that Wolbachia spreads to the oocytes from the stem cells as Wolbachia does in D. melanogaster.
Wolbachia exhibited movement within nurse cells of D. melanogaster (Ferree et al., 2005). In this study, Wolbachia was also observed in nurse cells of L. striatellus ovarioles. Nurse cells in telotrophic meroistic ovarioles usually provide nutrients to the developing oocytes via nutritive cords. Vg was found in the nurse cells of L. striatellus by electron and immunoelectron microscopy (Huo et al., 2014). Wolbachia and Vg may be transported into the nurse cells together via endocytosis. In the nurse cells, Vg was processed into yolk granules (Rouillé et al., 1995) and Wolbachia was released into the cytoplasm. Finally, Wolbachia in the nurse cells spread into the developing oocytes as Vg flows through the nutritive cords. This scenario could explain why Wolbachia accumulation in nutritive cords (Figure 7D and Supplementary Figure 2).
Endosymbiotic organisms in L. striatellus appear to use different pathways for transovarial transmission. YLSs pass through the ovariole pedicel and enter the oocyte through a deep depression at the posterior pole (Noda, 1974, 1977). RSV does not directly invade the oocyte from the terminal filament or the pedicel, but instead invades nurse cells at the tip of the tropharium and then spreads into oocytes as the ovaries development (Huo et al., 2014). Bacteriocytes (Nysius ericae, Nithecus jacobaeaein, Marchalina hellenica, and Scaphoideus titanus) in insects with telotrophic ovarioles enter the tropharium and then infect oocytes through the nutritive cords (Sacchi et al., 2008; Swiatoniowska et al., 2013; Szklarzewicz et al., 2013). Tightly packed endosymbiotic microorganisms were observed in the apex tropharium of Cixius nervosus (Szklarzewicz et al., 2007). Actin in the polytrophic ovarioles may affect the location of Wolbachia in the Drosophila oocytes (Ferree et al., 2005; Newton et al., 2015). In D. melanogaster, Wolbachia enter into ovaries from the anterior tip of the germarium, which includes a terminal filament and cap cells (Frydman et al., 2006). The Wolbachia pattern of strong tropism in host ovaries was controlled by evolutionarily conserved Wolbachia-encoded factors (Toomey et al., 2013). In L. striatellus, Wolbachia were first found in the anterior tropharium of ovarioles (Figure 3). Wolbachia invaded each ovariole from tropharium on its anterior side rather than the pedicel side (Figure 7), pointing to a barrier between the pedicel and vitellarium preventing Wolbachia entry into the oocyte directly from the pedicel.
Based on the above observations, we propose a model for Wolbachia transovarial transmission into L. striatellus oocytes (Figure 10). Wolbachia bind to Vg outside the ovarioles and then endocytosed into nurse cells and central located trophic core in the tropharium via wide channels formed between nurse cells during the early phase of vitellogenesis (Figures 10A,B). As central located trophic core divide into oocytes, some Wolbachia enter the arrested oocyte and establish an early infection. Wolbachia in the nurse cells spread into the developing oocytes through the nutritive cords with or without binding with Vg (Figures 10A,C). However, Wolbachia transmission is prevented when the oocyte is completely developed and the nutritive cords disappear. This model suggests that Wolbachia invade the L. striatellus ovariole from anterior tropharium cells rather than the pedicel side (Figures 10A,D), and use the existing Vg transovarial transport system to enter into oocytes for efficient maternal transmission.
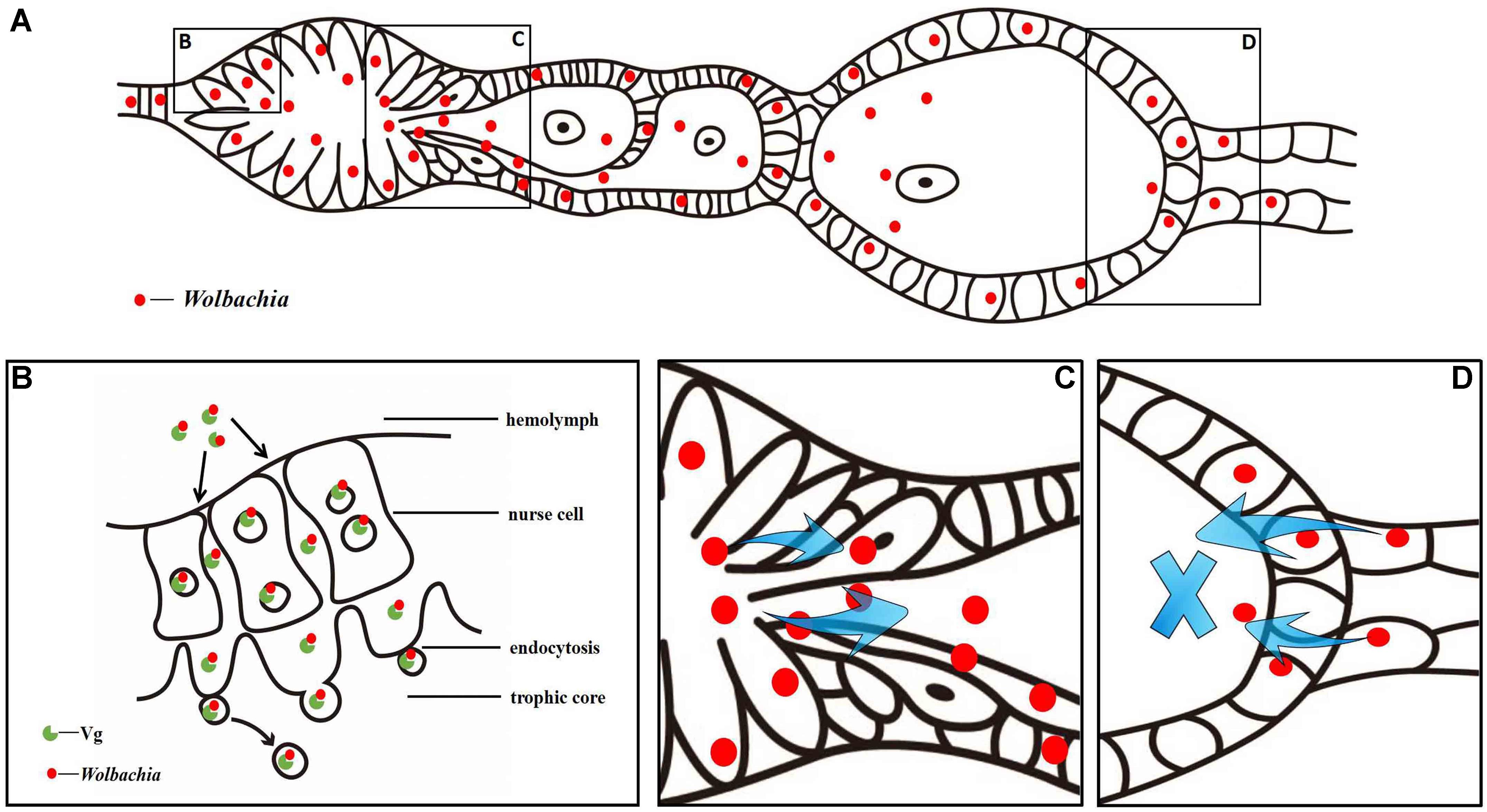
FIGURE 10. Wolbachia transovarial transmission model. (A) Diagram of Wolbachia concentrated in an L. striatellus ovariole. Wolbachia invade the ovariole from tropharium cells of its anterior side (B) via wide channels formed between nurse cells and migrate from the tropharium to the oocytes through the nutritive cord (C). Wolbachia invaded the ovariole from its anterior side rather than the pedicel side (D).
Due to lack of anti-Vg monoclonal and polyclonal antibody in L. striatellus, RT-qPCR, qPCR, RNAi and immunofluorescence staining were used to point to interactions between the Wolbachia infection and the presence of host Vg. These interactions may play a role in Wolbachia motility and replication in the host, and thus may be responsible for the efficient maternal transmission of Wolbachia. Further studies to characterize Wolbachia proteins that bind to or alter host Vg are needed to understand the molecular basis of the interaction between Wolbachia and egg development.
Author Contributions
YG, AH and X-YH wrote the manuscript. X-QX, P-WM, and H-JH performed the experiment. J-TG and J-FJ performed the images processing.
Funding
This work was supported by a grant-in-aid for Scientific Research (31672035, 31371944, and 31172131) from the National Natural Science Foundation of China. AH is supported by a Fellowship from the National Health and Medical Research Council.
Conflict of Interest Statement
The authors declare that the research was conducted in the absence of any commercial or financial relationships that could be construed as a potential conflict of interest.
Acknowledgments
We thank Zhiyong Xi of Michigan State University, United States for his thoughtful suggestions on the research. We thank Zhao-Can Shen and Peng-Yu Jin of the Department of Entomology, Nanjing Agricultural University, China for help with rearing planthoppers in the laboratory.
Supplementary Material
The Supplementary Material for this article can be found online at: https://www.frontiersin.org/articles/10.3389/fmicb.2018.02016/full#supplementary-material
References
Breeuwer, J. A., and Werren, J. H. (1990). Microorganisms associated with chromosome destruction and reproductive isolation between two insect species. Nature 346, 558–560. doi: 10.1038/346558a0
Bressac, C., and Rousset, F. (1993). The reproductive incompatibility system in Drosophila simulans: DAPI-staining analysis of the Wolbachia symbionts in sperm cysts. J. Invertebr. Pathol. 61, 226–230. doi: 10.1006/jipa.1993.1044
Cheng, D. J., and Hou, R. F. (2005). Determination and distribution of a female-specific protein in the brown planthopper, Nilaparvata lugens Stål (Homoptera: Delphacidae). Tissue Cell 37, 37–45. doi: 10.1016/j.tice.2004.09.003
Clark, M. E., Veneti, Z., Bourtzis, K., and Karr, T. L. (2002). The distribution and proliferation of the intracellular bacteria Wolbachia during spermatogenesis in Drosophila. Mech. Dev. 111, 3–15. doi: 10.1016/S0925-4773(01)00594-599
Davey, K. G. (1993). Hormonal integration of egg production in Rhodnius prolixus. Am. Zool. 33, 397–402. doi: 10.1093/icb/33.3.397
Dobson, S. L., Bourtzis, K., Braig, H. R., Jones, B. F., Zhou, W. G., Rousset, F., et al. (1999). Wolbachia infections are distributed throughout insect somatic and germ line tissues. Insect Biochem. Mol. Biol. 29, 153–160. doi: 10.1016/S0965-1748(98)00119-112
Dong, S. Z., Ma, Y., Hou, Y., Yu, X., and Ye, G. Y. (2011). Development of an ELISA for evaluating the reproductive status of female brown planthopper, Nilaparvata lugens, by measuring vitellogenin and vitellin levels. Entomol. Exp. Appl. 139, 103–110. doi: 10.1111/j.1570-7458.2011.01109.x
Dong, S. Z., Ye, G. Y., Zhu, J. Y., Chen, Z. X., Hu, C., and Liu, S. (2007). Vitellin of Pteromalus puparum (Hymenoptera: Pteromalidae), a pupal endoparasitoid of Pieris rapae (Lepidoptera: Pieridae): biochemical characterization, temporal patterns of production and degradation. J. Insect Physiol. 53, 468–477. doi: 10.1016/j.jinsphys.2007.01.008
Douglas, A. (1989). Mycetocyte symbiosis in insects. Biol. Rev. 64, 409–434. doi: 10.1111/j.1469-185X.1989.tb00682.x
Fast, E. M., Toomey, M. E., Panaram, K., Desjardins, D., Kolaczyk, E. D., and Frydman, H. M. (2011). Wolbachia enhance Drosophila stem cell proliferation and target the germline stem cell niche. Science 334, 990–992. doi: 10.1126/science.1209609
Ferree, P. M., Frydman, H. M., Li, J. M., Cao, J., Wieschaus, E., and Sullivan, W. (2005). Wolbachia utilizes host microtubules and Dynein for anterior localization in the Drosophila oocyte. PLoS Pathog. 1:e14. doi: 10.1371/journal.ppat.0010014
Frydman, H. M., Li, J. M., Robson, D. N., and Wieschaus, E. (2006). Somatic stem cell niche tropism in Wolbachia. Nature 441, 509–512. doi: 10.1038/nature04756
Frydman, H. M., and Spradling, A. C. (2001). The receptor-like tyrosine phosphatase Lar is required for epithelial planar polarity and for axis determination with Drosophila ovarian follicles. Development 128, 3209–3220.
Guo, J. Y., Dong, S. Z., Yang, X. L., Cheng, L., Wan, F. H., Liu, S. S., et al. (2012). Enhanced vitellogenesis in a whitefly via feeding on a begomovirus-infected plant. PLoS One 7:e43567. doi: 10.1371/journal.pone.0043567
Herren, J. K., Paredes, J. C., Schüpfer, F., and Lemaitre, B. (2013). Vertical transmission of a Drosophila endosymbiont via cooption of the yolk transport and internalization machinery. mBio 4:e00532-12. doi: 10.1128/mBio.00532-12
Hilgenboecker, K., Hammerstein, P., Schlattmann, P., Telschow, A., and Werren, J. H. (2008). How many species are infected with Wolbachia?-a statistical analysis of current data. FEMS Microbiol. Lett. 281, 215–220. doi: 10.1111/j.1574-6968.2008.01110.x
Huang, H. J., Bao, Y. Y., Lao, S. H., Huang, X. H., Ye, Y. Z., Wu, J. X., et al. (2015). Rice ragged stunt virus-induced apoptosis affects virus transmission from its insect vector, the brown planthopper to the rice plant. Sci. Rep. 5:11413. doi: 10.1038/Srep11413
Hughes, G. L., Dodson, B. L., Johnson, R. M., Murdock, C. C., Tsujimoto, H., Suzuki, Y., et al. (2014). Native microbiome impedes vertical transmission of Wolbachia in Anopheles mosquitoes. Proc. Natl. Acad. Sci. U.S.A. 111, 12498–12503. doi: 10.1073/pnas.1408888111
Huo, Y., Liu, W. W., Zhang, F. J., Chen, X. Y., Li, L., Liu, Q. F., et al. (2014). Transovarial transmission of a plant virus is mediated by vitellogenin of its insect vector. PLoS Pathog. 10:e1003949. doi: 10.1371/journal.ppat.1003949
Huo, Y., Yu, Y. L., Chen, L. Y., Li, Q., Zhang, M. T., Song, Z. Y., et al. (2018). Insect tissue-specific vitellogenin facilitates transmission of plant virus. PLoS Pathog. 14:e1006909. doi: 10.1371/journal.ppat.1006909
Hurst, G. D., Jiggins, F. M., von der Schulenburg, J. H. G., Bertrand, D., West, S. A., et al. (1999). Male-killing Wolbachia in two species of insect. Proc. Biol. Sci. 266, 735–740. doi: 10.1098/rspb.1999.0698
Ijichi, N., Kondo, N., Matsumoto, R., Shimada, M., Ishikawa, H., and Fukatsu, T. (2002). Internal spatiotemporal population dynamics of infection with three Wolbachia strains in the adzuki bean beetle, Callosobruchus chinensis (Coleoptera: Bruchidae). Appl. Environ. Microb. 68, 4074–4080. doi: 10.1128/AEM.68.8.4074-4080.2002
Kawakami, Y., Goto, S. G., Ito, K., and Numata, H. (2009). Suppression of ovarian development and vitellogenin gene expression in the adult diapause of the two-spotted spider mite Tetranychus urticae. J. Insect Physiol. 55, 70–77. doi: 10.1016/j.jinsphys.2008.10.007
Kose, H., and Karr, T. L. (1995). Organization of Wolbachia pipientis in the Drosophila fertilized egg and embryo revealed by an anti-Wolbachia monoclonal antibody. Mech. Dev. 51, 275–288. doi: 10.1016/0925-4773(95)00372-X
Livak, K. J., and Schmittgen, T. D. (2001). Analysis of relative gene expression data using real-time quantitative PCR and the 2-ΔΔCT method. Methods 25, 402–408. doi: 10.1006/meth.2001.1262
Lu, P., Bian, G., Pan, X., and Xi, Z. Y. (2012). Wolbachia induces density-dependent inhibition to dengue virus in mosquito cells. PLoS Negl. Trop. Dis. 6:e1754. doi: 10.1371/journal.pntd.0001754
Newton, I. L., Savytskyy, O., and Sheehan, K. B. (2015). Wolbachia utilize host actin for efficient maternal transmission in Drosophila melanogaster. PLoS Pathog. 11:e1004798. doi: 10.1371/journal.ppat.1004798
Noda, H. (1974). Preliminary histological observation and population dynamics of intracellular yeast-like symbiotes in the smaller brown planthopper, Laodelphax striatellus (Homoptera: Delphacidae). Appl. Entomol. Zool. 9, 275–277. doi: 10.1303/aez.9.275
Noda, H. (1977). Histological and histochemical observation of intracellular yeastlike symbiotes in the fat body of the smaller brown planthopper, Laodelphax striatellus (Homoptera: Delphacidae). Appl. Entomol. Zool. 12, 134–141. doi: 10.1303/aez.12.134
Noda, H. (1984). Cytoplasmic incompatibility in allopatric field populations of the small brown planthopper, Laodelphax striatellus, in Japan. Entomol. Exp. Appl. 35, 263–267. doi: 10.1111/j.1570-7458.1984.tb03391.x
Noda, H., Koizumi, Y., Zhang, Q., and Deng, K. (2001). Infection density of Wolbachia and incompatibility level in two planthopper species, Laodelphax striatellus and Sogatella furcifera. Insect Biochem. Mol. Biol. 31, 727–737. doi: 10.1016/S0965-1748(00)00180-186
O’Neill, S. L., Giordano, R., Colbert, A., Karr, T. L., and Robertson, H. M. (1992). 16S rRNA phylogenetic analysis of the bacterial endosymbionts associated with cytoplasmic incompatibility in insects. Proc. Natl. Acad. Sci. U.S.A. 89, 2699–2702. doi: 10.1073/pnas.89.7.2699
Rouillé, Y., Duguay, S. J., Lund, K., Furuta, M., Gong, Q., Lipkind, G., et al. (1995). Proteolytic processing mechanisms in the biosynthesis of neuroendocrine peptides: the subtilisin-like proprotein convertases. Front. Neuroendocrinol. 16, 322–361. doi: 10.1006/frne.1995.1012
Rousset, F., Bouchon, D., Pintureau, B., Juchault, P., and Solignac, M. (1992). Wolbachia endosymbionts responsible for various alterations of sexuality in arthropods. Proc. Biol. Sci. 250, 91–98. doi: 10.1098/rspb.1992.0135
Rousset, F., and Raymond, M. (1991). Cytoplasmic incompatibility in insects: why sterilize females? Trends Ecol. Evol. 6, 54–57. doi: 10.1016/0169-5347(91)90123-F
Sacchi, L., Genchi, M., Clementi, E., Bigliardi, E., Avanzati, A., Pajoro, M., et al. (2008). Multiple symbiosis in the leafhopper Scaphoideus titanus (Hemiptera: Cicadellidae): details of transovarial transmission of Cardinium sp. and yeast-like endosymbionts. Tissue Cell 40, 231–242. doi: 10.1016/j.tice.2007.12.005
Schmidt, T. L., Barton, N. H., Rašić, G., Turley, A. P., Montgomery, B. L., Iturbe-Ormaetxe, I., et al. (2017). Local introduction and heterogeneous spatial spread of dengue-suppressing Wolbachia through an urban population of Aedes aegypti. PLoS Biol. 15:e2001894. doi: 10.1371/journal.pbio.2001894
Stouthamer, R., Breeuwer, J., Luck, R., and Werren, J. (1993). Molecular identification of microorganisms associated with parthenogenesis. Nature 361, 66–68. doi: 10.1038/361066a0
Swiatoniowska, M., Ogorzalek, A., Golas, A., Michalik, A., and Szklarzewicz, T. (2013). Ultrastructure, distribution, and transovarial transmission of symbiotic microorganisms in Nysius ericae and Nithecus jacobaeae (Heteroptera: Lygaeidae: Orsillinae). Protoplasma 250, 325–332. doi: 10.1007/s00709-012-0416-4
Szklarzewicz, T., Jankowska, W., Łukasiewicz, K., and Szymańska, B. (2007). Structure of the ovaries and oogenesis in Cixius nervosus (Cixiidae), Javesella pellucida and Conomelus anceps (Delphacidae) (Insecta, Hemiptera, Fulgoromorpha). Arthropod Struct. Dev. 36, 199–207. doi: 10.1016/j.asd.2006.09.001
Szklarzewicz, T., Jankowska, W., Wieczorek, K., and Wegierek, P. (2009). Structure of the ovaries of the primitive aphids Phylloxera coccinea and Phylloxera glabra (Hemiptera, Aphidinea: Phylloxeridae). Acta Zool. 90, 123–131. doi: 10.1111/j.1463-6395.2008.00335.x
Szklarzewicz, T., Kalandyk-Kolodziejczyk, M., Kot, M., and Michalik, A. (2013). Ovary structure and transovarial transmission of endosymbiotic microorganisms in Marchalina hellenica (Insecta, Hemiptera, Coccomorpha: Marchalinidae). Acta Zool. 94, 184–192. doi: 10.1111/j.1463-6395.2011.00538.x
Toomey, M. E., Panaram, K., Fast, E. M., Beatty, C., and Frydman, H. M. (2013). Evolutionarily conserved Wolbachia-encoded factors control pattern of stem-cell niche tropism in Drosophila ovaries and favor infection. Proc. Natl. Acad. Sci. U.S.A. 110, 10788–10793. doi: 10.1073/pnas.1301524110
Turelli, M., and Hoffmann, A. A. (1995). Cytoplasmic incompatibility in Drosophila simulans: dynamics and parameter estimates from natural populations. Genetics 140, 1319–1338.
Weeks, A. R., Turelli, M., Harcombe, W. R., Reynolds, K. T., and Hoffmann, A. A. (2007). From parasite to mutualist: rapid evolution of Wolbachia in natural populations of Drosophila. PLoS Biol. 5:e114. doi: 10.1371/journal.pbio.0050114
Werren, J. H., Baldo, L., and Clark, M. E. (2008). Wolbachia: master manipulators of invertebrate biology. Nat. Rev. Microbiol. 6, 741–751. doi: 10.1038/nrmicro1969
Werren, J. H., Zhang, W., and Guo, L. R. (1995). Evolution and phylogeny of Wolbachia: reproductive parasites of arthropods. Proc. Biol. Sci. 261, 55–63. doi: 10.1098/rspb.1995.0117
Zhang, K. J., Han, X., and Hong, X. Y. (2013). Various infection status and molecular evidence for horizontal transmission and recombination of Wolbachia and Cardinium among rice planthoppers and related species. Insect Sci. 20, 329–344. doi: 10.1111/j.1744-7917.2012.01537.x
Keywords: Wolbachia, vertical transmission, vitellogenin, tropharium, nutritive cord, oocyte
Citation: Guo Y, Hoffmann AA, Xu X-Q, Mo P-W, Huang H-J, Gong J-T, Ju J-F and Hong X-Y (2018) Vertical Transmission of Wolbachia Is Associated With Host Vitellogenin in Laodelphax striatellus. Front. Microbiol. 9:2016. doi: 10.3389/fmicb.2018.02016
Received: 07 May 2018; Accepted: 09 August 2018;
Published: 28 August 2018.
Edited by:
Suhelen Egan, University of New South Wales, AustraliaReviewed by:
Grant Leslie Hughes, Liverpool School of Tropical Medicine, United KingdomJonathan Badger, National Cancer Institute (NCI), United States
Copyright © 2018 Guo, Hoffmann, Xu, Mo, Huang, Gong, Ju and Hong. This is an open-access article distributed under the terms of the Creative Commons Attribution License (CC BY). The use, distribution or reproduction in other forums is permitted, provided the original author(s) and the copyright owner(s) are credited and that the original publication in this journal is cited, in accordance with accepted academic practice. No use, distribution or reproduction is permitted which does not comply with these terms.
*Correspondence: Xiao-Yue Hong, eHlob25nQG5qYXUuZWR1LmNu