- 1Microbiology Research Unit, Division of Oral Biosciences, Dublin Dental University Hospital, University of Dublin, Trinity College Dublin, Dublin, Ireland
- 2National MRSA Reference Laboratory, St. James’s Hospital, Dublin, Ireland
- 3Division of Restorative Dentistry and Periodontology, Dublin Dental University Hospital, University of Dublin, Trinity College Dublin, Dublin, Ireland
Staphylococcus aureus and Staphylococcus epidermidis are frequent commensals of the nares and skin and are considered transient oral residents. Reports on their prevalence in the oral cavity, periodontal pockets and subgingivally around infected oral implants are conflicting, largely due to methodological limitations. The prevalence of these species in the oral cavities, periodontal pockets and subgingival sites of orally healthy individuals with/without implants and in patients with periodontal disease or infected implants (peri-implantitis) was investigated using selective chromogenic agar and matrix-assisted laser desorption/ionization time-of-flight mass spectrometry. Staphylococcus epidermidis was predominant in all participant groups investigated. Its prevalence was significantly higher (P = 0.0189) in periodontal pockets (30%) than subgingival sites of healthy individuals (7.8%), and in subgingival peri-implantitis sites (51.7%) versus subgingival sites around non-infected implants (16.1%) (P = 0.0057). In contrast, S. aureus was recovered from subgingival sites of 0-12.9% of the participant groups, but not from periodontal pockets. The arginine catabolic mobile element (ACME), thought to enhance colonization and survival of S. aureus, was detected in 100/179 S. epidermidis and 0/83 S. aureus isolates screened using multiplex PCR and DNA microarray profiling. Five distinct ACME types, including the recently described types IV and V (I; 14, II; 60, III; 10, IV; 15, V; 1) were identified. ACME-positive S. epidermidis were significantly (P = 0.0369) more prevalent in subgingival peri-implantitis sites (37.9%) than subgingival sites around non-infected implants (12.9%) and also in periodontal pockets (25%) compared to subgingival sites of healthy individuals (4.7%) (P = 0.0167). To investigate the genetic diversity of ACME, 35 isolates, representative of patient groups, sample sites and ACME types underwent whole genome sequencing from which multilocus sequence types (STs) were identified. Sequencing data permitted ACME types II and IV to be subdivided into subtypes IIa-c and IVa-b, respectively, based on distinct flanking direct repeat sequences. Distinct ACME types were commonly associated with specific STs, rather than health/disease states or recovery sites, suggesting that ACME types/subtypes originated amongst specific S. epidermidis lineages. Ninety of the ACME-positive isolates encoded the ACME-arc operon, which likely contributes to oral S. epidermidis survival in the nutrient poor, semi-anaerobic, acidic and inflammatory conditions present in periodontal disease and peri-implantitis.
Introduction
Staphylococcus aureus and Staphylococcus epidermidis are common commensals of human skin and the nares and are highly proficient at forming biofilms. Both species are significant causes of nosocomial infections associated with indwelling medical devices (Song et al., 2013). It is now widely acknowledged that many of the antimicrobial resistance genes identified in clinical isolates of S. aureus were acquired from coagulase negative staphylococci (CoNS) such as S. epidermidis by transfer of mobile genetic elements (MGEs) (Otto, 2013). One very significant example of this is the horizontal acquisition by S. aureus of staphylococcal cassette chromosome-mec (SCCmec) elements harboring the methicillin resistance gene mec from S. epidermidis. To date, 13 different types of SCCmec (I-XIII) have been characterized in methicillin-resistant S. aureus (MRSA)1 differentiated according to the various combinations of mec and cassette chromosome recombinase (ccr) gene complexes present (International Working Group on the Classification of Staphylococcal Cassette Chromosome Elements, 2009; Wu et al., 2015; Baig et al., 2018). Many more SCCmec variants have been described in species in which methicillin-resistance (MR) is more common, such as S. epidermidis (MRSE) and other CoNS (Shore and Coleman, 2013).
The staphylococcal arginine catabolic mobile element (ACME) plays a role in colonization of human skin and evasion of host immune responses (Diep et al., 2008; Planet et al., 2013). It is considered an SCC-like element as, like SCCmec, it is flanked by homologous inverted and direct repeat sequences (DRs) that integrate into the same attB attachment site in the chromosomal orfX locus as SCCmec (Ito et al., 2001; Diep et al., 2006). Specific clonal lineages of S. aureus are known to harbor ACME, most notably the highly successful USA300 clone, which harbors a composite island (CI) composed of SCCmec type IVa and ACME type I (Diep et al., 2006). The prevalence of ACME has also been reported to be high in sequence type (ST) 239 MRSA isolates (43.7%) from screening swabs of hospitalized patients in Singapore (Hon et al., 2014) and in ST239-like (as determined by pulsed-field gel electrophoresis) bloodstream MRSA isolates (39%) recovered in Australia (Espedido et al., 2012).
Like SCCmec, ACME is more prevalent and exhibits greater diversity in S. epidermidis. Many studies have identified ACME in multiple STs of the predominant S. epidermidis clonal lineages based on multilocus sequence typing (MLST), suggesting that ACME originated in this species (Miragaia et al., 2009; Barbier et al., 2011; Onishi et al., 2013). To date, ACME has been detected in the range of 45.8–67.9% in S. epidermidis isolates recovered from disparate geographical locations, as well as in carriage and disease isolates (Diep et al., 2006; Miragaia et al., 2009; Barbier et al., 2011; Onishi et al., 2013).
The ACME genetic island ranges between 30 and 55 kb in size and is associated with three main gene clusters, the arc operon composed of the arcR/A/D/B/C genes, the opp3 operon composed of the opp3A/B/C/D/E genes, and the recently revealed kdp operon, composed of the kdpE/D/A/B/C genes (Diep et al., 2006; O’Connor et al., 2018). These gene clusters encode an arginine deaminase pathway, an oligopeptide permease ABC transporter and a potassium ABC transporter, respectively. These three operons which can be present in ACME are in addition to the native chromosomal arc, opp1 and opp2, and kdp operons in S. aureus (Diep et al., 2008; Xue et al., 2011; Price-Whelan et al., 2013).
Five distinct ACME types have been described to date, according to the presence of the arc and opp3 operons (type I), the arc operon only (type II), the opp3 operon only (type III), the arc and kdp operons (type IV), and all three arc, opp and kdp operons (type V) (Gill et al., 2005; Diep et al., 2006; Shore et al., 2011; McManus et al., 2017; O’Connor et al., 2018). Furthermore, two distinct ACME IV subtypes, IVa and IVb have been described based on distinct combinations of flanking DRs (O’Connor et al., 2018). To date, all five types and several variants thereof have been described in S. epidermidis (Miragaia et al., 2009; Barbier et al., 2011; Onishi et al., 2013; Soroush et al., 2016; McManus et al., 2017; O’Connor et al., 2018). In contrast, types I and II and variants thereof have been detected in S. aureus, commonly collocated with other genetic elements such as SCCmec or SCC-associated genes in CIs and separated from these adjacent elements by DRs (Diep et al., 2006; Shore et al., 2011; Kawaguchiya et al., 2013; Rolo et al., 2012).
Although staphylococci are considered transient members of the oral microflora, these species are prevalent in the oral cavities of the elderly and in people with dental infections such as periodontal disease (Murdoch et al., 2004; Friedlander, 2010). Periodontal disease is an inflammatory condition that can progress from gingivitis in response to dental plaque and affects the gingiva as well as the supporting periodontal structures (Hajishengallis, 2015). As periodontal disease progresses, enlargement of the gingival crevice occurs and leads to eventual detachment of the gingival tissue from the tooth resulting in periodontal pocket formation. Periodontal pockets provide a semi-anaerobic nutrient-poor environment that is ideal for plaque accumulation by resident oral microflora and is prone to decreases in pH resulting from physiological processes such as tissue repair (Percival et al., 2014).
The titanium-based oral implant can act as an ideal substrate for staphylococcal-based biofilm formation (Thurnheer and Belibasakis, 2016), as can the oxygen-depleted environment of periodontal and peri-implantitis pockets. Dental implants are indwelling medical devices made of titanium-based alloys that are placed in the bone of the mandible or maxilla to anchor a prosthetic crown, denture or bridge (Adell, 1981; Branemark et al., 1983). They consist of a shaft that is placed directly in the jaw bone and stabilized by subsequent osseointegration, and an abutment onto which a prosthesis is fitted. Similar to gingivitis, peri-implant mucositis is an inflammatory condition that affects the gingivae surrounding a dental implant, which can progress to peri-implantitis in which supporting bone surrounding an implant is gradually lost, potentially resulting in implant failure (Renvert and Polyzois, 2018).
Both of these oral diseases have a similar etiology in that they are both associated with dental plaque in which a shift from normal resident microflora to more periodontopathogenic species appears to occur (Nibali et al., 2015).
This study investigated the prevalence of S. epidermidis and S. aureus in the oral cavities, subgingival sites and periodontal pockets of patients with implants and natural teeth in states of both health and disease. Isolates recovered were investigated for ACME to determine if ACME could be a molecular marker for periodontal disease and/or peri-implantitis. Previous studies investigated the prevalence of ACME in both S. aureus and S. epidermidis in a range of carriage and infection sites (Miragaia et al., 2009; Barbier et al., 2011; Du et al., 2013; Onishi et al., 2013), however, to our knowledge, no studies have investigated the prevalence of ACME among oral staphylococcal isolates from periodontal pockets or peri-implantitis sites. A selection of the ACME-positive isolates identified in the present study were further investigated by whole-genome sequencing (WGS) in order to elucidate the genetic organization of the ACMEs in detail. Such investigations could yield important information regarding the potential genetic reservoir of ACME that exist among S. epidermidis for potential future spread to MRSA.
Materials and Methods
Study Group
Ethical approval for this study was granted by the St. James’s Hospital and Federated Dublin Voluntary Hospitals Joint Research Ethics Committee (JREC) and the Faculty of Health Sciences Ethics Committee of Trinity College Dublin, Ireland. Prior to enrollment in the study, all participants were provided with comprehensive patient information documentation and all participants included provided written consent. All documentation (including consent forms) provided to patients was pre-approved by the Research Ethics Committees.
All participants in the study met the following criteria: they were over 18 years of age, had a minimum of 10 natural teeth and were capable of providing informed consent. Participants were excluded from the study if they had any of the following factors: diabetes or asthma, pregnancy or lactation, blood-borne illnesses, steroid treatment within the year or antibiotics within 2 months prior to sampling. Patients with periodontal disease had a minimum of one periodontal site with a probing depth of greater than 6 mm and bleeding on probing (BOP). Patients with peri-implantitis were partially dentate and had one or more oral implants in place for a minimum of 5 years, at least one of which showed clinical signs of disease (Sanz and Chapple, 2012; Holtfreter et al., 2015). The study group consisted of 31 orally healthy patients with dental implants, 20 patients with periodontal disease, 21 patients with peri-implantitis and 64 orally healthy participants.
Sample Collection and Processing
All clinical sampling was carried out by qualified Dentists at the Dublin Dental University Hospital (DDUH). Sub-gingival sites and periodontal pockets were sampled by inserting a PerioPaperTM gingival fluid collection strip (Oroflow, Plainview, NY, United States) into the sub-gingival crevice or periodontal pocket for 30 s. Following sampling the collection strips were placed in sterile 2 ml screw-capped tubes (Sarstedt AG & Co., Numbrecht, Germany) containing 1 ml of nutrient broth (NB) (Oxoid Ltd., Hampshire, United Kingdom). In addition, oral rinse samples were collected by providing participants with sterile 100 ml plastic cups (Sarstedt AG & Co.) containing 25 ml sterile phosphate buffered saline (PBS) and instructing the participant to rinse their mouths with the PBS for 30 s before returning the rinse fluid to the same container. The anterior nares of participants were sampled using nitrogen-gassed VI-packed sterile transport swabs (Sarstedt AG & Co.). Following sampling, all samples were transported immediately to the microbiology laboratory and processed within 4 h. Vials containing PerioPaperTM strips suspended in NB were vortexed at maximum speed for 1 min and 100 μl aliquots of the resulting cell suspension were plated onto mannitol salt agar (MSA) and SaSelectTM chromogenic agar (Bio-Rad Laboratories, Hertfordshire, United Kingdom) agar. Oral rinse samples were processed by transferring a 1 ml aliquot to a sterile 1.5 ml Eppendorf Safe-lockTM microfuge tube (Eppendorf, Hamburg, Germany) and centrifuged at 20,000 ×g for 1 min, after which the supernatant was discarded and the resultant pellet was resuspended in 200 μl NB. To isolate staphylococcal colonies, 100 μl aliquots of this cell suspension were plated on MSA and SaSelectTM. Nasal swabs were used to lawn the entire surface of MSA and SaSelectTM plates. Inoculated MSA and SaSelectTM plates were incubated at 37°C for 48 h in a static incubator (Gallenkamp, Leicester, United Kingdom).
Culture, Identification and Storage of Isolates
Bacterial isolates were cultured on Columbia blood agar (Fannin Ltd., Dublin, Republic of Ireland) at 37°C for 48 h prior to identification by Vitek MS Matrix-Assisted Laser Desorption Ionization-Time of Flight Mass Spectrometry system (MALDI-TOF MS) (Vitek, bioMérieux Marcy l’Etoile, France) according to the manufacturer’s instructions. Multiple isolates were identified and stored from each sample for future analysis. All isolates were stored on MicrobankTM storage beads (Pro-Lab Diagnostics, Cheshire, United Kingdom) at -80°C.
DNA Isolation and Detection of ACME by Multiplex PCR and DNA Microarray Profiling
Where possible, a minimum of two isolates were selected as representatives of each individual participant, distinct sample sites and each staphylococcal species recovered and screened by multiplex PCR to detect the presence of ACME.
Genomic DNA was extracted from isolates by enzymatic lysis using the buffers and solutions provided with the S. aureus Genotyping Kit 2 DNA microarray kit (Alere Technologies GmbH, Jena, Germany) and the DNeasy Blood and Tissue kit (Qiagen, Crawley, West Sussex, United Kingdom) according to the manufacturers’ instructions.
The presence of ACME was detected in isolates by multiplex PCR targeting the arcA, opp3B and kdpA genes harbored by ACME using the previously described arcA- and opp3B-directed primers (Diep et al., 2006; McManus et al., 2017) and incorporating primers targeting the kdpA gene (kdpF: 5′-CGGTTTAACTGGTGCGTT-3′ and kdpR: 5′-GCAATACATACAGCGTAGCC-3′) (O’Connor et al., 2018). PCR assays were carried out in 50 μl reaction volumes containing a 200 μM concentration of each deoxynucleoside triphosphate, 1.25 U of GoTaq polymerase (Promega, Madison, WI, United States), 10 μl (1×) of GoTaq FlexiBuffer (Promega), 2.5 μM MgCl2, 100 pmol of each primer, and 1 ng of the DNA template. Cycling conditions consisted of 94°C for 2 min, followed by 35 cycles of 94°C for 30 s, 60°C for 30 s, 72°C for 45 s and followed by a final elongation step of 72°C for 10 min. Amplification products (arcA product: 724 bp, opp3B product 530 bp, kdpA product: 241 bp) were separated by electrophoresis in 2% (w/v) agarose (Sigma-Aldrich Ltd., Wicklow, Republic of Ireland) containing 1X GelRed® (Biotium Inc., Fremont, CA, United States) and visualized using an Alpha Innotech UV transilluminator (Protein Simple, San Jose, CA, United States).
The presence of mec and ACME-arc genes amongst S. aureus and S. epidermidis isolates investigated was also detected by DNA microarray profiling using the S. aureus Genotyping Kit 2.0 (Alere Technologies GmbH, Jena, Germany) according to the manufacturer’s instructions and as described previously (Monecke et al., 2008).
Molecular Characterization of ACME Elements by WGS
A total of 35 S. epidermidis isolates selected as representatives of each patient group, sample site and ACME type present were subjected to WGS (Table 1). Libraries were prepared using Nextera XT library preparation reagents (Illumina, Eindhoven, Netherlands) and sequenced using an Illumina MiSeq desktop sequencer. For each isolate, reads were aligned with reference S. epidermidis and S. aureus genomes containing ACME and/or SCC elements downloaded from Genbank using a Burrows-Wheeler aligner (BWA) (Li and Durbin, 2009) to select the most appropriate reference ACME type to use as a scaffold. De novo assemblies were carried out on the reads for each ACME-harboring isolate using SPAdes version 3.62. For each isolate, the reference genome that exhibited the highest degree of alignment with the relevant reads was used in a further alignment with the annotated contigs from the de novo assembly of the relevant isolate. Contigs identified as containing ACME- or SCCmec-associated DNA sequences were aligned, annotated and visualized using BioNumerics version 7.6 (Applied Maths, Sint-Martens-Latem, Belgium) and the Artemis sequence viewer (Berriman and Rutherford, 2003).
In order to confirm the genetic organization and orientation of contigs, primers were designed using BioNumerics version 7.6 that targeted a minimum distance of 200 nucleotides from the contig boundaries. The target specificity of primers was confirmed using BLAST software3. All primers were supplied by Sigma–Aldrich Ltd. Contig gaps were closed based on PCR-based amplification and Sanger-based sequencing of these regions using the primers listed in Supplementary Table S1. Sanger-based sequencing was carried out commercially by Source BioScience (Waterford, Republic of Ireland).
Multiple alignments of the complete nucleotide sequences of the arc, opp3 and kdp operons (including intragenic regions) from each isolate investigated were carried out using the Clustal Omega tool (Sievers et al., 2011). The nucleotide sequence from the first to last base of each operon, including any intragenic regions was compared among all isolates investigated in the present study.
Determination of STs Among Isolates Subjected to WGS
The STs of ACME-harboring S. epidermidis isolates subjected to WGS were determined from the WGS data by examination of the nucleotide sequences of the loci used for the consensus S. epidermidis MLST scheme (Thomas et al., 2007). Briefly, the relevant nucleotide sequences were gleaned from the WGS data and inputted into the S. epidermidis MLST database online4 in order to define allelic profiles and STs.
Statistical Analyses
In order to determine if the differences in the prevalence of staphylococcal species and isolates harboring ACME were significant between different sample sites or patient groups, two-tailed Fisher’s exact tests were utilized. These analyses were carried out using GraphPad QuickCalcs5. A P value of <0.05 was deemed statistically significant. Statistical power analyses were calculated using the DSS research statistical power calculator tool6 with a confidence interval of 5%.
Nucleotide Accession Numbers
The Genbank database accession numbers for the nucleotide sequences of the S. epidermidis ACMEs previously characterized (McManus et al., 2017; O’Connor et al., 2018) and in the present study are listed in Table 1.
Results
Prevalence of S. epidermidis and S. aureus in the Oral Cavity
Staphylococcus epidermidis was recovered from the oral rinse samples of 18/20 (90%) patients with periodontal disease, 18/38 (47.4%) patients with peri-implantitis, 25/31 (80.6%) orally healthy patients with implants and 44/64 (68.8%) orally healthy participants (Table 2). Staphylococcus epidermidis was significantly more prevalent in the oral rinse samples of orally healthy patients with implants than in those with peri-implantitis (P = 0.0061, Power = 90%), however the difference in the prevalence of S. epidermidis in the oral rinse samples of patients with periodontal disease in comparison to orally healthy participants was not quite statistically significant (P = 0.081).
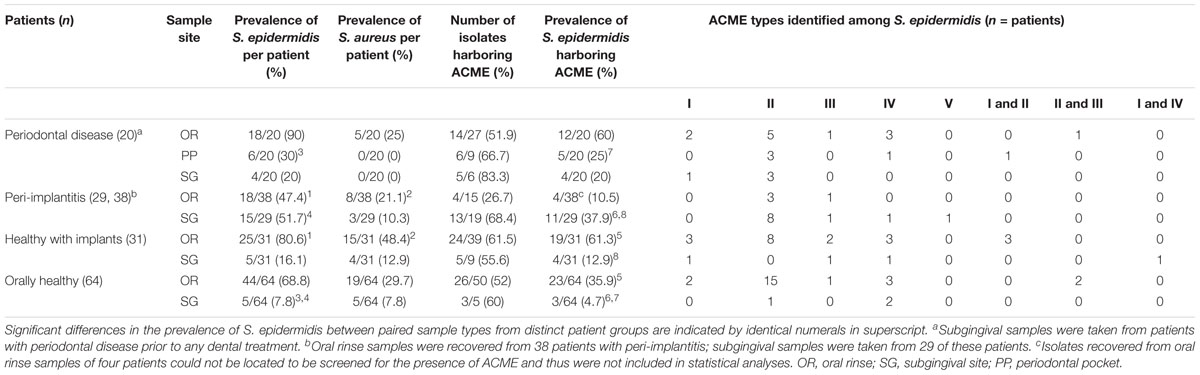
TABLE 2. Prevalence of ACME types harbored by S. epidermidis from distinct patient groups and anatomical sites.
The prevalence of S. aureus was considerably lower than S. epidermidis among all four groups of participants examined, detected in 5/20 (25%) patients with periodontal disease, 8/38 (21.1%) patients with peri-implantitis, 15/31 (48.4%) orally healthy patients with implants and 19/64 (29.7%) of orally healthy participants (Table 2). The prevalence of S. aureus was highest in the oral cavities of healthy patients with oral implants and was significantly more prevalent in the oral rinse samples of this patient group when compared to the corresponding sample sets from patients with peri-implantitis (P = 0.0219, Power = 77.8%).
Prevalence of S. epidermidis and S. aureus in Subgingival Sites, Peri-implant Sites and Periodontal Pockets
Staphylococcus epidermidis was recovered from the periodontal pockets of 6/20 (30%) patients with periodontal disease and the peri-implant sites of 15/29 (51.7%) patients with peri-implantitis. In contrast, S. epidermidis was only recovered from the subgingival sites of 5/31 (16.1%) orally healthy patients with implants and 5/64 (7.8%) of orally healthy participants (Table 2). Staphylococcus epidermidis was significantly more prevalent in the periodontal pockets of patients with periodontal disease than the subgingival sites of orally healthy participants (P = 0.0189, Power = 77.1%). Similarly, the prevalence of S. epidermidis was significantly higher in the subgingival sites of patients with peri-implantitis than similar sites in orally healthy patients with implants (P = 0.0057, Power = 91.4%).
Staphylococcus aureus was equally or less prevalent than S. epidermidis in the subgingival sites of all participant groups investigated, recovered from none of the periodontal pockets of patients with periodontal disease, 3/29 (10.3%) peri-implant pockets of patients with peri-implantitis, 4/31 (12.9%) of orally healthy patients with implants and 5/64 (7.8%) of orally healthy participants (Table 2). The prevalence of subgingival S. aureus was not significantly different in any of the four participant groups investigated.
Prevalence of ACME Among S. epidermidis and S. aureus Isolates Recovered
The Arginine catabolic mobile element was detected in 100/179 (55.9%) of the S. epidermidis isolates recovered from all four participant groups (Table 2). The mecA gene was detected in 12/179 (6.7%) isolates; two from patients with periodontal disease, three from patients with peri-implantitis, three from orally healthy patients with implants and four from orally healthy participants. In total, 5/12 of the MRSE isolates identified also harbored ACMEs, predominantly type II.
Among the samples from which S. epidermidis was recovered, ACME was detected in isolates recovered from the oral rinse samples of 12/20 (60%) patients with periodontal disease, 4/38 (10.5%) patients with peri-implantitis, 19/31 (61.3%) orally healthy patients with implants, and 23/64 (35.9%) orally healthy participants. The prevalence of S. epidermidis isolates harboring ACME was significantly higher in the oral rinse samples of orally healthy patients with implants than in those of healthy participants (P = 0.0275, Power = 76.1%).
Although the prevalence of S. epidermidis was lower in subgingival sites than in the oral rinse samples of all four participant groups, the proportion of ACME-harboring S. epidermidis isolates was higher (Table 2). The presence of ACME was detected in S. epidermidis isolates from the periodontal pockets and subgingival sites of 5/20 (25%) and 4/20 (20%) patients with periodontal disease, respectively. Similarly, ACME was detected in 11/29 (37.9%), 4/31 (12.9%) and 3/64 (4.7%) S. epidermidis isolates from the subgingival sites of patients with peri-implantitis, healthy patients with dental implants and orally healthy participants, respectively.
Isolates harboring ACME were significantly more prevalent in subgingival samples of patients with peri-implantitis than in subgingival samples of orally healthy participants (P = 0.0001, Power = 98.4%). Similarly, isolates with ACME were also significantly more prevalent in periodontal pockets of patients with periodontal disease than subgingival sites of orally healthy participants (P = 0.0167, Power = 78.5%). Interestingly, the prevalence of ACME-harboring isolates was also significantly higher in the subgingival sites of patients with peri-implantitis than subgingival sites of orally healthy patients with implants (P = 0.0369, Power = 72.9%).
In contrast, ACME was not detected in any of the 83 S. aureus isolates recovered from oral rinse samples (n = 56) and subgingival samples (n = 27) investigated.
All five previously described ACME types were detected in S. epidermidis isolates in the present investigation by multiplex PCR. In all participant groups and anatomical sites sampled, ACME type II was the predominant ACME type, identified in S. epidermidis isolates recovered from 53 of the participants investigated. The recently described ACME types IV and V were detected in S. epidermidis isolates recovered from 15 and one participants sampled, respectively (Table 2).
Pairs of separate S. epidermidis isolates recovered from the oral rinse and subgingival samples of four orally healthy patients with implants were found to harbor distinct ACME types (i.e., types I and II or types I and IV). Similarly, a pair of S. epidermidis isolates recovered the same periodontal pocket of a patient with periodontal disease harbored ACME types I and II, respectively (Table 2). Furthermore, pairs of separate S. epidermidis isolates harboring ACMEs II and III were detected in the same oral rinse sample of one patient with periodontal disease and in two orally healthy participants.
A total of 35 S. epidermidis isolates selected as representatives of each ACME type, patient group, sample site and ACME type were subjected to WGS in order to elucidate the genetic organization of the ACMEs harbored in detail (Table 1).
Genetic Diversity of ACME Type I
Two isolates recovered from the oral rinse samples of a patient with periodontal disease (P14OR1) and an orally healthy patient with implants (I12OR1) were found to harbor ACME type I by multiplex PCR and were subjected to WGS. The STs of these isolates were identified as ST17 and ST7, respectively (Table 1). These STs are double locus variants of each other, differing at the gtr and pyrR loci by a total of four bp.
In both isolates, the ACME type I element was collocated with additional modules in CIs that were 54.3 and 39.9 kb in size, respectively (Figure 1). Both of the ACME type I elements characterized harbored the arc and opp3 operons, the speG gene and were demarcated by DRs_B and _C (Table 3). In both isolates, ACME type I was located directly downstream of a module composed of the copA gene and the ars operon, separated by DR_B. In isolate I12OR1, this module was demarcated by an additional DR_B at the 5′ end in orfX, whereas in isolate P14OR1 this module was demarcated by DR_O at the 5′ end and an additional module containing three ORFs was located upstream of the copA/ars operon module (Figure 1).
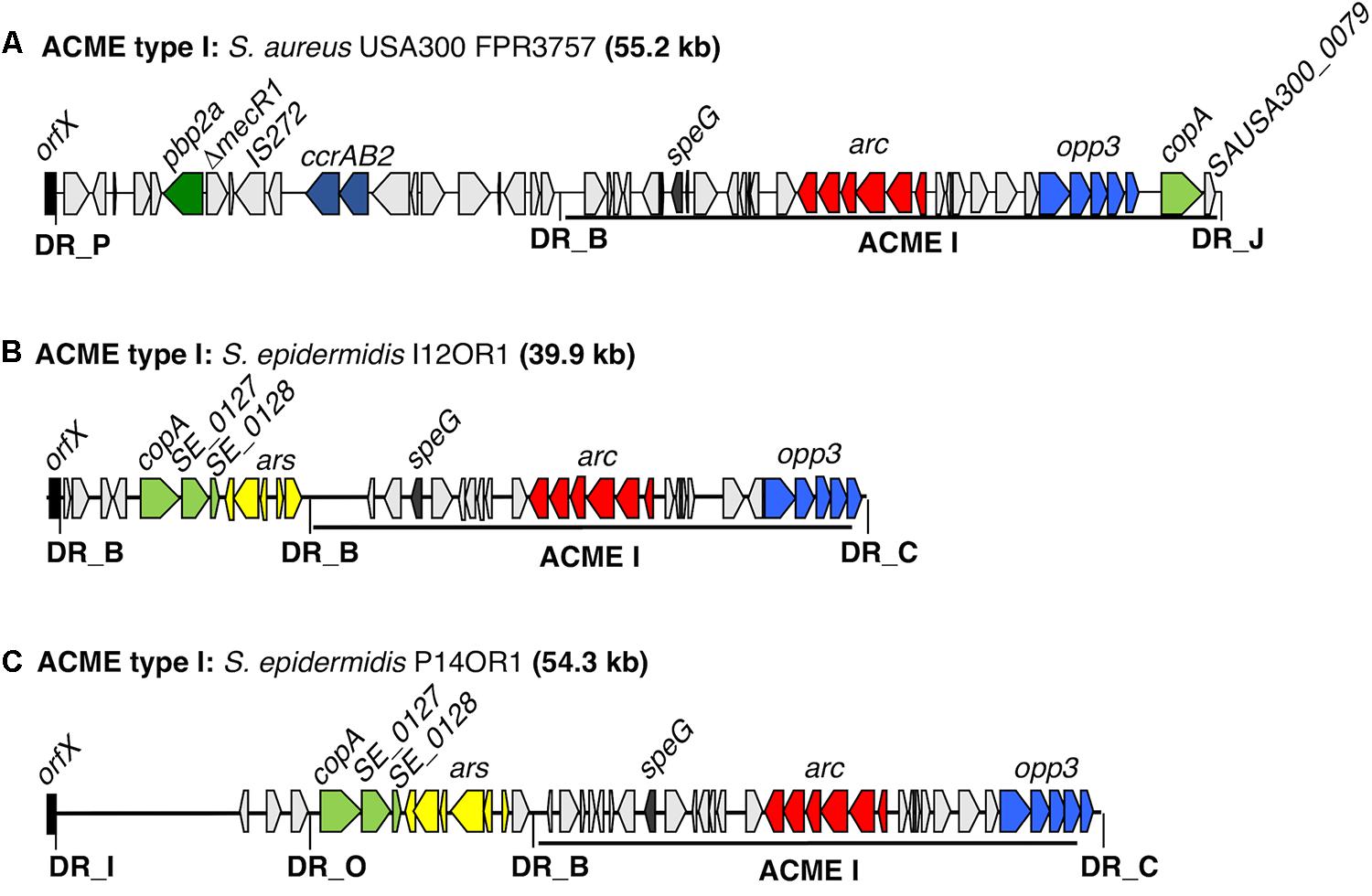
FIGURE 1. Schematic representation of ACME type I elements harbored by two distinct S. epidermidis isolates investigated by WGS. The ACME type I previously described in the MRSA reference USA300 strain FPR3757 (Genbank accession number CP000255.1) is included for comparison (A). The size of each ACME is indicated after each strain name. (B,C) Each gene or group of genes of interest is differentiated by a different color, i.e., light blue; opp3-operon, red; arc-operon, pale green; cop-operon, yellow; ars operon, dark blue; ccrAB complexes, dark green; pbp2a, dark gray; speG, light gray; genes encoding hypothetical proteins, sugar transporters, transposases and other ORFs, previously identified in ACMEs. The direction of transcription for each ORF is indicated by arrows. The DRs are indicated in bold font and correspond to DR sequences listed in Table 3.
Genetic Diversity of ACME Type II
Seventeen isolates harboring ACME type II were identified by multiplex PCR and were further investigated by WGS (Figure 2). All 17 isolates harbored ACMEs with the arc operon only and lacked both the opp3 and kdp operons. Based on the differing combinations of DRs identified at the 5′ and 3′ ends, the ACME type II elements characterized could be divided into three distinct subtypes (IIa-c) of which ACME type IIb predominated (12/17, 70.6%). ACME type IIa was demarcated by the DRs _H and _C (Table 3) and was identified in 3/17 isolates (Figures 2B–D), all of which belonged to ST59. ACME type IIb (Figures 2E–I) was demarcated by the DRs _D and _C and was identified in 12/17 isolates belonging to ST89 (n = 1), ST14 (n = 2), ST73 (n = 8) and ST701, a single locus variant of ST73 (n = 1). The remaining two isolates harbored ACME type IIc demarcated by the DRs _A and _E and belonged to ST672 (Figures 2J,K). Interestingly in the case of the latter two isolates, the copA and ars operon were internalized within ACME type IIc in both isolates (Figures 2J,K) and both lacked the internal DR_G commonly identified downstream of the arc operon in ACME type II. The ACME type IIc-harboring CIs in these isolates were almost identical, differing only by the presence of an ORF upstream of the sdrH gene in isolate P14PPP2 (Figure 2K).
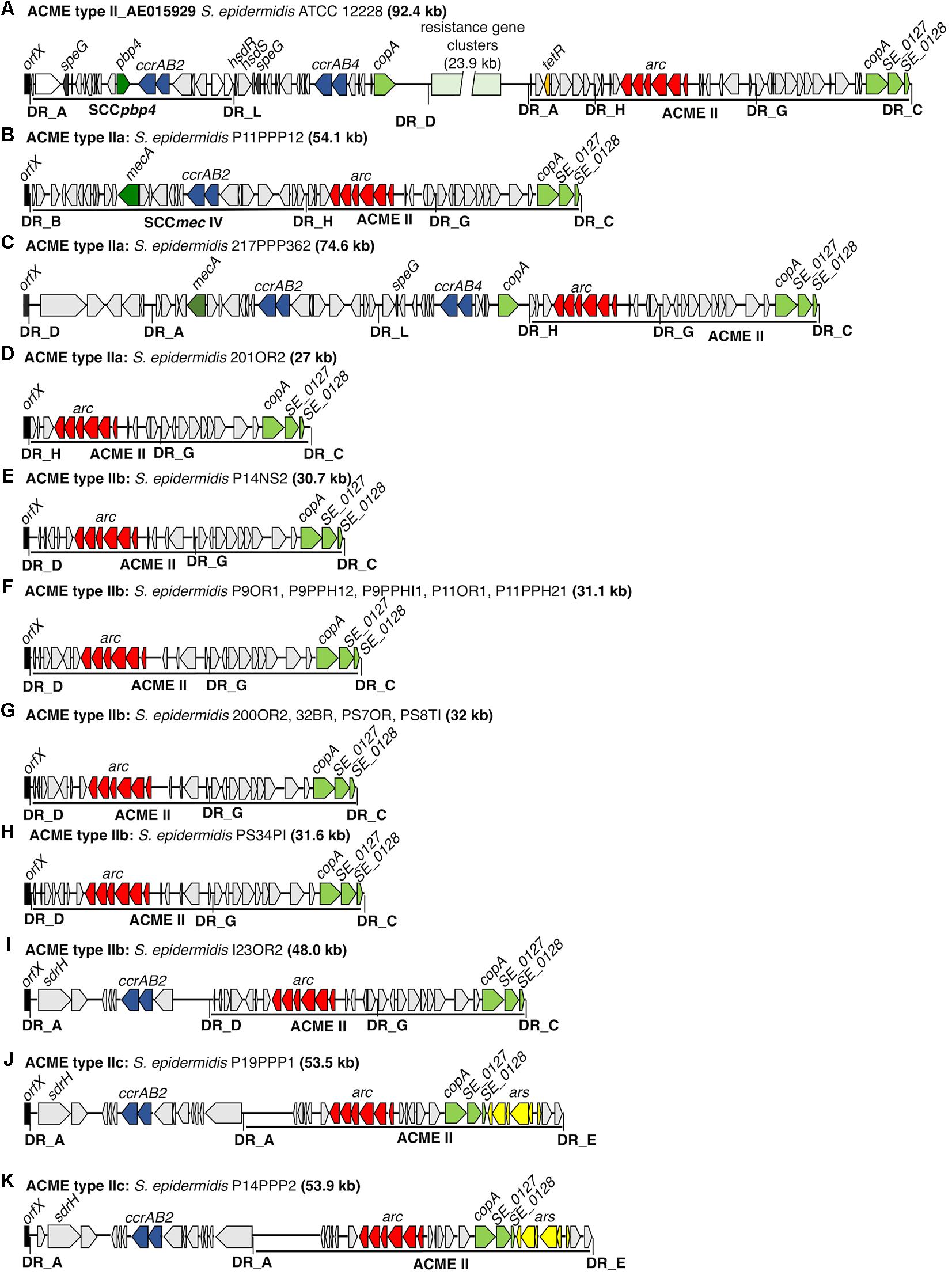
FIGURE 2. Schematic representation of ACME II subtypes a-c and ACME type II-harboring CIs investigated in the present study. The previously described ACME type II CI harbored by the S. epidermidis reference strain ATCC12228 (Genbank accession number AE015929) is included for reference (A). The size of each ACME is shown after the strain name. Each gene or group of genes of interest is differentiated by a different color, i.e., red; arc-operon, pale green; mustard; tetR, cop-operon, yellow; ars operon, dark blue; ccrAB genes, dark green; mecA, dark gray; speG, light gray; genes encoding hypothetical proteins, sugar transporters, transposases and other ORFs, previously identified in ACMEs. The direction of transcription for each ORF is indicated by the arrow. The DRs are indicated in bold font and correspond to each DR sequence listed in Table 3.
Five of the 17 ACME type II structures characterized by WGS existed as modules of larger CIs and were collocated with modules which harbored genes associated with SCC elements such as ccr and mec (Figures 2B,C,I–K). In each of these five CIs, the ACME type II structure was divided from these SCC-associated modules by DRs _A, _H, or _D (Table 3).
Interestingly, in one isolate (217PPP362) two separate and distinct SCC-associated modules were detected in tandem upstream of ACME type IIa (Figure 2C). The module immediately upstream of ACME type IIa was demarcated by the DRs _L and _H and harbored the speG and ccrAB4 genes and was collocated immediately downstream of an additional SCC-associated module which harbored the mecA and ccrAB2 genes. The ACME type IIa and IIb structures in the remaining 12/17 isolates investigated had integrated directly into orfX in the absence of any adjacent modules and were not components of larger CIs (Figure 2).
The presence of sdr genes, members of the serine/aspartate repeat family encoding fibrinogen-binding proteins was detected in modules adjacent to ACME type II in 3/17 isolates investigated and was collocated with ACME subtype IIb and IIc identified in the present study.
The speG gene encoding spermidine acetyltransferase was detected in only one isolate harboring ACME type II, (217PPP362) located in a SCC-associated module upstream of ACME type IIa (Figure 2C). The copA gene was located near the 3′ end of all 17 ACME type II structures investigated. In all ACME type IIa and IIb structures investigated, this gene was separated from the arc operon by DR_G and additional open reading frames commonly identified within ACMEs (Figure 2).
Genetic Diversity of ACME Type III
Four isolates harbored ACME type III, all of which were identified as ST329 (Table 1). Three of the ST329 isolates (P16OR1, 204OR1 and I11OR1) have been described previously (McManus et al., 2017). The CI harbored by the fourth isolate (I1PPP121) consisted of two distinct modules separated by DR_G (Figure 3). The module at the 5′ end harbored two pairs of ccr genes, of which ccrA4 and ccrB2 were prematurely truncated. This module also harbored the copA and ars genes, located upstream of ACME type III as previously observed in the other three isolates. The ACME type III harbored by I1PPP121 exhibited a minimum of 99.81% nucleotide identity to the ACME type IIIs harbored by isolates P16OR1, 204OR1, and I11OR1.
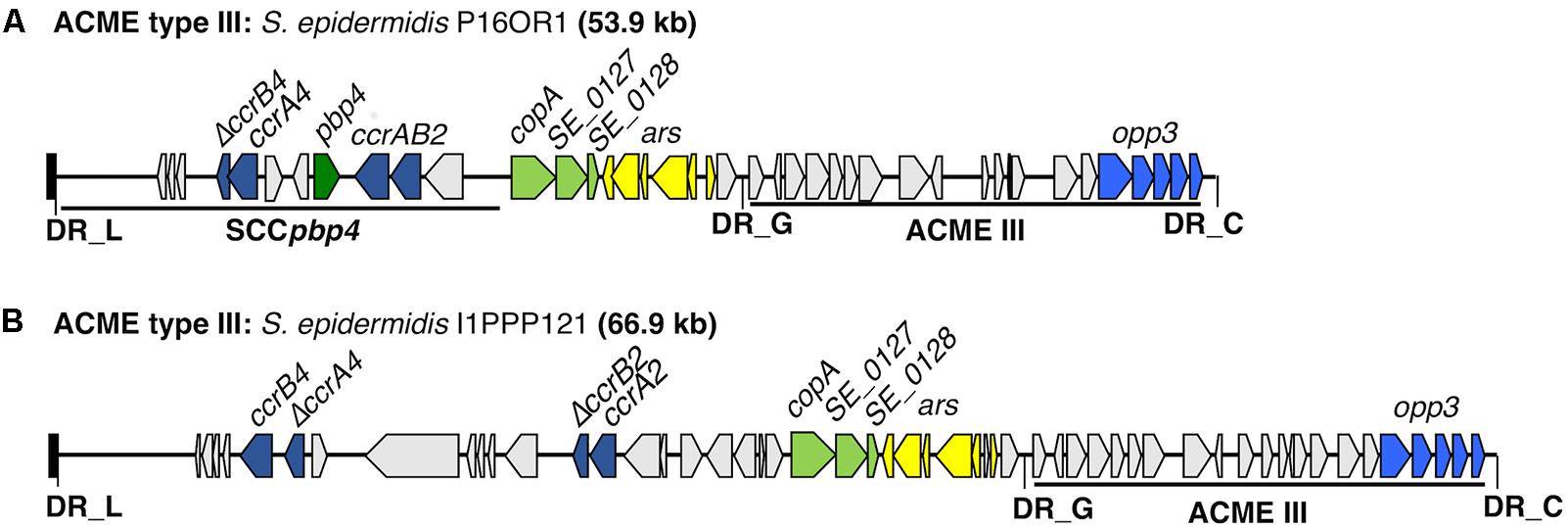
FIGURE 3. Schematic representation of ACME type III structure from oral S. epidermidis isolate I1PPP121 characterized in the present study. The previously described ACME type III structure from an S. epidermidis isolate (P16OR1) recovered from an oral rinse sample from a patient with periodontal disease (McManus et al., 2017) is included for comparison (A). The size of the ACME characterized is shown after the isolate name I1PPP121 (B). Each gene or group of genes of interest is differentiated by a different color, i.e., pale green; cop-operon, dark blue; ccrAB gene complexes (of which the genes ccrA4 and ccrB2 were prematurely truncated), light blue; opp3 operon, dark green; pbp4, light gray; genes encoding hypothetical proteins, sugar transporters, transposases and other ORFs, previously identified in ACMEs. The direction of transcription for each ORF is indicated by the arrow. The DRs are indicated in bold font and correspond to each DR sequence listed in Table 3.
Genetic Diversity of ACME Types IV and V
Eleven isolates harboring ACME type IV were identified and belonged to ST210 (n = 1), ST153 (n = 6), ST130 (n = 1), ST17 (n = 1), ST297 (n = 1), and ST432 (n = 1) (Table 1). Nine of these isolates were described recently in a study that first defined ACME types IV and V in S. epidermidis (O’Connor et al., 2018). The two additional ST153 isolates (PS7P2 and PS23P1) were characterized in the present study and harbored ACME types IVa and IVb, respectively. The ACME type IVa harbored by isolate PS7P2 was identical to those previously described in isolates 120PPC, I9OR1 and I14OR1, and the ACME type IVb harbored by isolate PS23P1 was identical to that previously described in isolate P8OR3 (O’Connor et al., 2018). ACME type V was identified in only one isolate, recovered from the subgingival site of a patient with peri-implantitis and has been previously described (O’Connor et al., 2018).
Genetic Diversity Among the arc, opp3 and kdp Operons Harbored by ACME Types I–V
The arc Operon
The percentage nucleotide identity between all ACME-arc operons identified in the present study, in ACME types I, II, IV and V ranged from 99.06 to 100%. The ACME-arc operons harbored by isolates 200OR2, 32BR, PS7OR and PS8TI (all ACME type IIb) exhibited 100% nucleotide identity to each other, as did that harbored by isolates 120PPC, I9OR1, I14OR1 (all ACME type IVa). Similarly, the ACME-arc operon harbored by P9OR1, P9PPH12, P9PPHI1, P11OR1 and P11PPH21 (all ACME type IIb) exhibited 100% nucleotide identity with each other (Supplementary Table 2).
The opp3 Operon
The percentage nucleotide identity between all opp3-operons identified in the present study, in ACME types I, III and V ranged from 97.22 to 100% (Supplementary Table S3). The opp3 operon harbored by isolates P16OR1 and P11OR1 (both ACME type III) exhibited 100% nucleotide identity to each other. The opp3 operons harbored by ACME type I exhibited 99.91% to each other and 99.96% nucleotide homology to the opp3 operon harbored by the reference ACME type I (Genbank accession number CP000255.1). The opp3 operon harbored by the recently described ACME type V (O’Connor et al., 2018) exhibited 99.22-99.24% and 97.48–97.52% nucleotide identity to those harbored by ACME types III and I, respectively (Supplementary Table S3).
The kdp Operon
The kdp operon was highly conserved, exhibiting a minimum of 99.86% nucleotide identity amongst isolates harboring ACME types IV and V. The kdp operons harbored by isolates 120PPC, PS30PH, 33BR, 218PP361, PS7P2 and I9OR1 (all ACME type IVa) exhibited 100% nucleotide identity to each other, and to that harbored by PS23P1 (ACME type IVb). The kdp operon harbored by the remaining ACME type IVb isolates, PS36PD and P8OR3 exhibited 99.94% nucleotide identity to each other. The kdp operon harbored by ACME type V exhibited a minimum of 99.86% nucleotide identity to the kdp operon harbored by ACME types IVa and IVb (Supplementary Table S4).
Comparison of ACMEs Among Multiple S. epidermidis Isolates From the Same Patients
Three isolates recovered from the periodontal pockets and oral rinse sample of a patient with periodontal disease (P9) were all identified as ST73 (Table 1) and harbored genetically identical ACME type IIb structures (Supplementary Figure S1). In contrast, three distinct S. epidermidis isolates recovered from the oral rinse, nasal swab and periodontal pocket of a patient (P14) with periodontal disease were identified as STs 17, 14 and 672, and harbored ACME types I, IIb and IIc, respectively (Supplementary Figure S1). Similarly, an isolate identified as ST59 and harboring ACME type IIa was recovered from the periodontal pocket of a patient with periodontal disease (P11) and was genetically distinct to two other isolates identified as ST73 and harboring ACME type IIb which were recovered from the oral rinse and another periodontal pocket of the same patient.
Discussion
The Prevalence of Staphylococci in the Oral Cavity, Subgingival Sites and Periodontal Pockets
Previous investigations of the prevalence of staphylococcal species in the oral cavity are conflicting and/or ambiguous for several probable reasons. Firstly, studies did not definitively distinguish between distinct CoNS species and S. aureus (Leonhardt et al., 1999). Secondly, different studies used semi-discriminatory agar media such as Baird Parker or MSA for primary recovery, which may have resulted in failure to select and further distinguish between morphologically similar colonies of distinct CoNS species (Loberto et al., 2004; Cuesta et al., 2010; dos Santos et al., 2014). Thirdly, several previous studies relied on checkerboard DNA-DNA hybridization techniques for definitive identification of oral staphylococcal species from patients with dental implants, an approach that does not distinguish between viable and dead bacteria (Fürst et al., 2007; Renvert et al., 2008; Salvi et al., 2008). Subsequently, real-time PCR with species-specific primers demonstrated that the previously used DNA:DNA hybridization probes showed cross reactivity between S. aureus and S. epidermidis DNA (Cashin, 2013).
Patient sampling and recovery of viable isolates was undertaken using a uniform, systematic methodology for all sample sites and patient groups and identification of both S. epidermidis and S. aureus isolates was determined using robust procedures. The utilization of the chromogenic SaSelectTM medium for primary isolation of oral staphylococci enabled direct visualization and presumptive identification of both S. epidermidis and S. aureus isolates based on the growth of these species as white/pale pink and pink colonies, respectively. This approach ensured that the differences in the prevalence of S. epidermidis and S. aureus observed are a true reflection of the patient groups and sample sites investigated.
Staphylococcus epidermidis
Staphylococcus epidermidis was detected in the oral cavities of 47.4 – 90% of the four participant groups investigated (Table 2) which was higher than previous studies that reported its recovery from the oral cavities of 27.3% patients with periodontal disease (Loberto et al., 2004) and 41% of orally healthy participants (Ohara-Nemoto et al., 2008).
Previous reports of the prevalence of subgingival S. epidermidis vary greatly, ranging between 15.9 and 64.3% in periodontal pockets (Loberto et al., 2004; Murdoch et al., 2004; dos Santos et al., 2014) and between 42.9 and 60.7% in the subgingival sites of healthy participants (Murdoch et al., 2004; Ohara-Nemoto et al., 2008), most likely due to different methods used. Data on the subgingival prevalence of S. epidermidis in patients with oral implants and/or peri-implantitis are largely lacking or did not undertake definitive identification of this species (Leonhardt et al., 1999).
Staphylococcus aureus
In the present study, S. aureus was considerably less prevalent than S. epidermidis in the oral cavities of participant groups and was rarely detected in subgingival sites or periodontal pockets (Table 2). The dramatic contrast in the oral prevalence of S. epidermidis and S. aureus was not surprising, as a negative correlation between the prevalence of these species has been reported previously (Brescó et al., 2017). Previous reports of the prevalence of subgingival S. aureus vary greatly, ranging from 13.4 to 68.2% (Cuesta et al., 2010; Zhuang et al., 2014) in periodontal pockets, 40–70.4% of peri-implant pockets and 25–44% of healthy subgingival sites around dental implants (Fürst et al., 2007; Renvert et al., 2008; Salvi et al., 2008). The contrasting results between the present and previous studies most likely reflects differences in methodology used as discussed above.
The Prevalence of ACME in S. epidermidis and S. aureus
The prevalence of ACME has previously been investigated amongst S. epidermidis populations and has been reported to range from 40 to 65.4% in MRSE, and from 64.4 to 83% in methicillin susceptible S. epidermidis (Miragaia et al., 2009; Barbier et al., 2011; Du et al., 2013; Onishi et al., 2013). In the present investigation, only 12/179 (6.7%) of the S. epidermidis isolates recovered were MRSE. This finding correlates with previous studies that reported a higher prevalence of ACME in methicillin-susceptible S. epidermidis. Five of the 12 MRSE isolates identified here harbored ACMEs, predominantly ACME type II.
This is the first investigation into the prevalence of ACME amongst S. epidermidis and S. aureus isolates recovered from both above and below the gumline in patients with and without oral disease. The prevalence of ACME was greater than 50% amongst the populations of S. epidermidis recovered. In contrast, ACME was not detected in any of the S. aureus isolates recovered.
This study revealed for the first time that the prevalence of ACME-harboring S. epidermidis was significantly higher in subgingival sites of patients with peri-implantitis and periodontal disease in comparison to healthy individuals (P = 0.0001 and P = 0.0167, respectively). Furthermore, S. epidermidis harboring ACME were significantly more prevalent in the subgingival sites of patients with peri-implantitis than in orally healthy patients with implants. Together, these results suggest a strong association of S. epidermidis isolates harboring ACME with diseased, semi-anaerobic subgingival tissue sites. No correlation between specific ACME types or subtypes and specific disease state or oral site was identified.
One of the potential limitations of the present investigation was the number of patients sampled. The study group was limited to patients attending DDUH who did not have any underlying conditions and had not received antibiotics in the 2 months prior to sampling. It is likely that larger investigations would further support the findings of this study as well as likely identify additional ACME types. Furthermore, it would be interesting to determine if ACME is more abundant in S. epidermidis isolates recovered from other diseased anatomical sites or wounds.
The Genetic Diversity of ACME
Previous investigations of the prevalence and structural diversity of ACME relied on primers targeting the ACME-arc and ACME-opp3 genes to detect ACME types I-III only (Miragaia et al., 2009; Onishi et al., 2013). This study is the first to include primers targeting the recently described ACME-kdp operon in a multiplex ACME-typing PCR and therefore the results of this study accurately reflect the true prevalence of ACME types currently described in S. epidermidis, at least of oral origin. The application of WGS to the characterization of ACME has revealed the additional ACME types harbored by S. epidermidis in addition to the detailed structural diversity of these ACMEs. It is highly likely that S. epidermidis isolates harboring the opp3 and kdp operons and the kdp operon only will also be identified in the future using WGS.
Common DRs were often observed amongst multiple ACME types and subtypes (Table 3). Many distinct DRs have been identified at the 5′ end of ACME, which is clearly demarcated by the integration of this element into orfX. The demarcation of the 3′ end is less obvious. Previously described ACMEs terminated at the 3′ end by DR_J in ACME type I of S. aureus (Genbank accession number CP000255.1) (Diep et al., 2006) and by DR_C in ACME types II-V of S. epidermidis (Gill et al., 2005; McManus et al., 2017; O’Connor et al., 2018), even though an internal DR_G is present downstream of the arc operon in both the reference ACME type II (Genbank accession number AE015929) and subsequently described in ACME types II (Figure 2) and IV (O’Connor et al., 2018). Many of the ACMEs characterized in the present and previous studies were components of CIs that were separated by multiple DRs, and greatly contributed to the diversity of these MGEs. Interestingly, the copA gene and ars operon were commonly detected in various distinct positions within or adjacent to ACME types I, II, III, and V. These genes were identified downstream of the ACME type II element in several isolates investigated in the present study (data not shown) but were internalized within the ACME type IIc structures (Figure 2). The identification of multiple DRs in common amongst distinct ACME types and the frequent organizational differences observed both within and between each ACME type supports previous studies that suggested ACMEs are assembled in a stepwise, modular fashion (Thurlow et al., 2013). Indeed, ACME diversity was also observed among separate isolates recovered from the oral cavities of the same patient (Supplementary Figure S1) in three cases.
The Association of ACME With Specific S. epidermidis Lineages
Isolates harboring ACME type I investigated here were identified as STs 7 and 17 (Table 1), both of which have been previously assigned to GC6 by Bayesian clustering analysis (Thomas et al., 2014; Tolo et al., 2016), a GC that is enriched with ACME-harboring isolates and associated with infection sources. Isolates harboring ACME type II were identified as STs 73 (and ST701, a single locus variant of ST73), 59, 89, 14, and 672, four of which have previously been assigned to GC1, a GC enriched with isolates from non-hospital sources (Thomas et al., 2014; Tolo et al., 2016). Two isolates harboring ACME type IIc from periodontal pockets of two separate patients were identified as ST672, however, this ST was not previously assigned to a GC. All isolates harboring ACME type III were recovered from separate patients and all belonged to ST329, previously associated with GC4. Isolates belonging to GC4 have been associated with a more commensal lifestyle (Thomas et al., 2014). Isolates harboring ACME type IV were identified as STs 153, 297, 130, and 17, of which four were previously assigned to GC6. The isolate harboring ACME type V, identified as ST5, also belonged to GC6.
Distinct ACME types were more commonly associated with isolates belonging to identical or closely related STs, rather than the participant group or sample sites from which each ACME-harboring isolate was recovered. Indeed, many STs identified amongst isolates recovered from the oral rinse samples of healthy participants belonged to GC6 (Table 2), a cluster enriched with isolates from infections (Tolo et al., 2016). These findings strongly suggest that the stepwise accumulation of ACMEs occurs in specific lineages of S. epidermidis, rather than in specific anatomical sites.
The Potential Role of ACME in Disease
In the present study, the predominant ACME types detected (II and IV) in S. epidermidis harbored the arc operon. Researchers have hypothesized that ACME enhances the transmissibility, colonization and persistence of the MRSA USA300 strain on human skin, contributing to the success of this lineage (Diep et al., 2008; Planet et al., 2013). The arginine deaminase pathway encoded by arc is responsible for the breakdown of polyamines which are largely L-arginine based. This results in the formation of ornithine, ATP, CO2 and ammonia, the latter of which contributes to the internal pH regulation of staphylococci in acidic conditions (Planet et al., 2013; Lindgren et al., 2014). The contribution of the constitutively expressed arc operon is likely to be highly advantageous to staphylococcal survival in the acidic environments present in dental plaque. In addition, the ATP generated is likely beneficial to organisms living in nutrient poor, semi-anaerobic environments such as present in periodontal pockets. Polyamines are associated with biological processes such as wound healing and infection clearance and it is therefore likely that they would be highly associated with oral inflammatory diseases such as periodontal disease and peri-implantitis. The speG gene, encoding a spermidine acetyltransferase, is thought to mitigate the lethal effects of polyamines on staphylococci. The exact benefit of the opp3 operon is unclear but encodes an oligopeptide permease ABC transporter (Diep et al., 2006, 2008). The speG gene and opp3 operon were detected in only eight and seven of the 35 ACMEs characterized, respectively, suggesting that these genes are relatively dispensable for S. epidermidis in oral environments (McManus et al., 2017; O’Connor et al., 2018).
Interestingly, the kdp operon was detected in 12 of the 35 ACMEs characterized by WGS, suggesting that these genes contribute to the survival of S. epidermidis in oral environments. This operon encodes a potassium transporter that is important for maintaining intracellular pH homeostasis and metabolic processes in S. aureus (Price-Whelan et al., 2013) and likely plays an important role in the adaptation and survival of S. epidermidis in dental plaque, which has a significant concentration of K+ ions (Margolis and Moreno, 1994).
Conclusion
This study revealed the significantly high prevalence of S. epidermidis in periodontal pockets and subgingival sites of patients with periodontal disease or peri-implantitis, respectively. There was also a very significant difference in the prevalence of S. epidermidis harboring ACME in these diseased subgingival sites and periodontal pockets compared to those recovered from healthy subgingival sites and oral rinse samples (Table 2). As yet, it is unclear if this organism contributes to disease progression directly. The presence of five main ACME types among oral S. epidermidis isolates was identified and extensive genetic diversity among these types was revealed using WGS, which would have been overlooked using previously described multiplex PCRs (Miragaia et al., 2009; Onishi et al., 2013). The arc and kdp operons harbored by the predominant ACME types identified (II and IV) very likely contribute to the survival of oral S. epidermidis under diseased and inflammatory conditions such as periodontal disease and peri-implantitis.
ETHICS STATEMENT
This study was carried out in accordance with the recommendations of the St. James’s Hospital and Federated Dublin Voluntary Hospitals Joint Research Ethics Committee (JREC) and the Faculty of Health Sciences Ethics Committee of Trinity College Dublin, Ireland. The protocol and all documentation (including consent forms) provided to patients was pre-approved by the Research Ethics Committees. Prior to enrollment in the study, all participants were provided with comprehensive patient information documentation and provided written consent in accordance with the Declaration of Helsinki.
Author Contributions
AO’ conceived and designed the study, performed the WGS data analysis, and drafted the manuscript. BM conceived and designed the study and helped with the study co-ordination, WGS data analysis, and wrote the manuscript. PK assisted with bioinformatic analyses. GB and TF performed definitive identification of staphylococcal isolates by MALDI_TOF. PC assisted with laboratory processing and isolation of staphylococcal isolates. MOS and IP performed clinical sampling of patients and participants included in the study. DC conceived and designed the study, purchased the required materials, assisted with data analysis, and drafted the manuscript. All authors read and approved the final manuscript.
Funding
This work was supported by the Microbiology Research Unit, Dublin Dental University Hospital. The funders had no role in study design, data collection and interpretation, or the decision to submit the work for publication.
Conflict of Interest Statement
The authors declare that the research was conducted in the absence of any commercial or financial relationships that could be construed as a potential conflict of interest.
Acknowledgments
We thank Peter Slickers at the InfectoGnostics Research Campus, Jena, Germany for technical assistance with de novo assemblies using SPAdes software, Maria Miragaia and Keith Jolley for the continuous curation of the S. epidermidis MLST database, and Anna Shore for her comments on the manuscript.
Supplementary Material
The Supplementary Material for this article can be found online at: https://www.frontiersin.org/articles/10.3389/fmicb.2018.01558/full#supplementary-material
Footnotes
- ^ www.SCCmec.org
- ^ http://cab.spbu.ru/software/spades/
- ^ https://blast.ncbi.nlm.nih.gov/Blast.cgi
- ^ http://sepidermidis.mlst.net/
- ^ http://www.graphpad.com/quickcalcs/index.cfm
- ^ https://www.dssresearch.com/KnowledgeCenter/toolkitcalculators/statisticalpowercalculators.aspx
References
Adell, R. (1981). A 15-year study of osseointegrated implants in the treatment of the edentulous jaw. Int. J. Oral Surg. 10, 387–416. doi: 10.1016/S0300-9785(81)80077-4
Baig, S., Johannesen, T. B., Overballe-Petersen, S., Larsen, J., Larsen, A. R., and Stegger, M. (2018). Novel SCCmec type XIII (9A) identified in an ST152 methicillin-resistant Staphylococcus aureus. Infect. Genet. Evol. 61, 74–76. doi: 10.1016/j.meegid.2018.03.013
Barbier, F., Lebeaux, D., Hernandez, D., Delannoy, A. S., Caro, V., François, P., et al. (2011). High prevalence of the arginine catabolic mobile element in carriage isolates of methicillin-resistant Staphylococcus epidermidis. J. Antimicrob. Chemother. 66, 29–36. doi: 10.1093/jac/dkq410
Berriman, M., and Rutherford, K. (2003). Viewing and annotating sequence data with Artemis. Br. Bioinform. 4, 124–132.
Branemark, P. I., Adell, R., Albrektsson, T., Lekholm, U., Lundkvist, S., and Rockler, B. (1983). Osseointegrated titanium fixtures in the treatment of edentulousness. Biomaterials 4, 25–28.
Brescó, M. S., Harris, L. G., Thompson, K., Stanic, B., Morgenstern, M., O’Mahony, L., et al. (2017). Pathogenic mechanisms and host interactions in Staphylococcus epidermidis device-related infection. Front. Microbiol. 8:1401. doi: 10.3389/fmicb.2017.01401
Cashin, P. J. (2013). Molecular Characterisation of Staphylococcus aureus and Staphylococcus epidermidis Populations from Healthy and Diseased Oral Implant and Natural Tooth Sites in a Cohort of Patients with Periimplantitis. Available at: http://hdl.handle.net/2262/77912
Cuesta, A. I., Jewtuchowicz, V., Brusca, M. I., Nastri, M. L., and Rosa, A. C. (2010). Prevalence of Staphylococcus spp and Candida spp in the oral cavity and periodontal pockets of periodontal disease patients. Acta Odontol. Latinoam. 23, 20–26.
Diep, B. A., Gill, S. R., Chang, R. F., Phan, T. H., Van Chen, J. H., Davidson, M. G., et al. (2006). Complete genome sequence of USA300, an epidemic clone of community-acquired meticillin-resistant Staphylococcus aureus. Lancet 367, 731–739. doi: 10.1016/S0140-6736(06)68231-7
Diep, B. A., Stone, G. G., Basuino, L., Graber, C. J., Miller, A., des Etages, S. A., et al. (2008). The arginine catabolic mobile element and staphylococcal chromosomal cassette mec linkage: convergence of virulence and resistance in the USA300 clone of methicillin-resistant Staphylococcus aureus. J. Infect. Dis. 197, 1523–1530. doi: 10.1086/587907
dos Santos, B. R., Demeda, C. F., da Silva, E. E., de Britto, M. H., Lima, K. C., and de Melo, M. C. (2014). Prevalence of subgingival Staphylococcus at periodontally healthy and diseased sites. Braz. Dent. J. 25, 271–276. doi: 10.1590/0103-6440201302285
Du, X., Zhu, Y., Song, Y., Li, T., Luo, T., Sun, G., et al. (2013). Molecular analysis of Staphylococcus epidermidis strains isolated from community and hospital environments in China. PLoS One 8:e62742. doi: 10.1371/journal.pone.0062742
Espedido, B. A., Steen, J. A., Barbagiannakos, T., Mercer, J., Paterson, D. L., Grimmond, S. M., et al. (2012). Carriage of an ACME II variant may have contributed to methicillin- resistant Staphylococcus aureus sequence type 239-like strain replacement in Liverpool Hospital, Sydney, Australia. Antimicrob. Agents Chemother. 56, 3380–3383. doi: 10.1128/AAC.00013-12
Friedlander, A. H. (2010). Oral cavity staphylococci are a potential source of prosthetic joint infection. Clin. Infect. Dis. 50, 1682–3; author reply 1683. doi: 10.1086/653003
Fürst, M. M., Salvi, G. E., Lang, N. P., and Persson, G. R. (2007). Bacterial colonization immediately after installation on oral titanium implants. Clin. Oral Implants Res. 18, 501–508. doi: 10.1111/j.1600-0501.2007.01381.x
Gill, S. R., Fouts, D. E., Archer, G. L., Mongodin, E. F., Deboy, R. T., Ravel, J., et al. (2005). Insights on evolution of virulence and resistance from the complete genome analysis of an early methicillin-resistant Staphylococcus aureus strain and a biofilm-producing methicillin-resistant Staphylococcus epidermidis strain. J. Bacteriol. 187, 2426–2438. doi: 10.1128/JB.187.7.2426-2438.2005
Hajishengallis, G. (2015). Periodontitis: from microbial immune subversion to systemic inflammation. Nat. Rev. Immunol. 15, 30–44. doi: 10.1038/nri3785
Holtfreter, B., Albandar, J. M., Dietrich, T., Dye, B. A., Eaton, K. A., Eke, P. I., et al. (2015). Standards for reporting chronic periodontitis prevalence and severity in epidemiologic studies: proposed standards from the Joint EU/USA Periodontal Epidemiology Working Group. J. Clin. Periodontol. 42, 407–412. doi: 10.1111/jcpe.12392
Hon, P. Y., Koh, T. H., Tan, T. Y., Krishnan, P., Leong, J. W. Y., Jureen, R., et al. (2014). Changing molecular epidemiology and high rates of mupirocin resistance among meticillin-resistant Staphylococcus aureus in Singaporean hospitals. J. Glob. Antimicrob. Resist. 2, 53–55. doi: 10.1016/j.jgar.2013.10.002
International Working Group on the Classification of Staphylococcal Cassette Chromosome Elements (2009). Classification of staphylococcal cassette chromosome mec (SCCmec): guidelines for reporting novel SCCmec elements. Antimicrob. Agents Chemother. 53, 4961–4967. doi: 10.1128/AAC.00579-09
Ito, T., Katayama, Y., Asada, K., Mori, N., Tsutsumimoto, K., Tiensasitorn, C., et al. (2001). Structural comparison of three types of staphylococcal cassette chromosome mec integrated in the chromosome in methicillin-resistant Staphylococcus aureus. Antimicrob. Agents Chemother. 45, 1323–1336. doi: 10.1128/AAC.45.5.1323-1336.2001
Kawaguchiya, M., Urushibara, N., Ghosh, S., Kuwahara, O., Morimoto, S., Ito, M., et al. (2013). Genetic diversity of emerging Panton-Valentine leukocidine/arginine catabolic mobile element (ACME)-positive ST8 SCCmec-IVa meticillin-resistant Staphylococcus aureus (MRSA) strains and ACME-positive CC5 (ST5/ST764) MRSA strains in northern Japan. J. Med. Microbiol. 62, 1852–1863. doi: 10.1099/jmm.0.062125-0
Leonhardt,Å., Renvert, S., and Dahlén, G. (1999). Microbial findings at failing implants. Clin. Oral Implants Res. 10, 339–345. doi: 10.1034/j.1600-0501.1999.100501.x
Li, H., and Durbin, R. (2009). Fast and accurate short read alignment with Burrows-Wheeler transform. Bioinformatics 25, 1754–1760. doi: 10.1093/bioinformatics/btp324
Lindgren, J. K., Thomas, V. C., Olson, M. E., Chaudhari, S. S., Nuxoll, A. S., Schaeffer, C. R., et al. (2014). Arginine deiminase in Staphylococcus epidermidis functions to augment biofilm maturation through pH homeostasis. J. Bacteriol. 196, 2277–2289. doi: 10.1128/jb.00051-14
Loberto, J. C. S., De Paiva Martins, C. A., Ferreira Dos Santos, S. S., Cortelli, J. R., and Cardoso Jorge, A. O. (2004). Staphylococcus spp. in the oral cavity and periodontal pockets of chronic periodontitis patients. Braz. J. Microbiol. 35, 64–68. doi: 10.1590/S1517-83822004000100010
Margolis, H. C., and Moreno, E. C. (1994). Composition and cariogenic potential of dental plaque fluid. Crit. Rev. Oral Biol. Med. 5, 1–25.
McManus, B. A., O’Connor, A. M., Kinnevey, P. M., O’Sullivan, M., Polyzois, I., and Coleman, D. C. (2017). First detailed genetic characterization of the structural organization of type III arginine catabolic mobile elements harbored by Staphylococcus epidermidis by using whole-genome sequencing. Antimicrob. Agents Chemother. 61, e1216-17. doi: 10.1128/AAC.01216-17
Miragaia, M., de Lencastre, H., Perdreau-Remington, F., Chambers, H. F., Higashi, J., Sullam, P. M., et al. (2009). Genetic diversity of arginine catabolic mobile element in Staphylococcus epidermidis. PLoS One 4:e7722. doi: 10.1371/journal.pone.0007722
Monecke, S., Jatzwauk, L., Weber, S., Slickers, P., and Ehricht, R. (2008). DNA microarray-based genotyping of methicillin-resistant Staphylococcus aureus strains from Eastern Saxony. Clin. Microbiol. Infect. 14, 534–545. doi: 10.1111/j.1469-0691.2008.01986.x
Murdoch, F. E., Sammons, R. L., and Chapple, I. L. C. (2004). Isolation and characterization of subgingival staphylococci from periodontitis patients and controls. Oral Dis. 10, 155–162. doi: 10.1046/j.1601-0825.2003.01000.x
Nibali, L., Pometti, D., Chen, T. T., and Tu, Y. K. (2015). Minimally invasive non-surgical approach for the treatment of periodontal intrabony defects: a retrospective analysis. J. Clin. Periodontol. 42, 853–859. doi: 10.1111/jcpe.12443
O’Connor, A. M., McManus, B. A., and Coleman, D. C. (2018). First description of novel arginine catabolic mobile elements (ACMEs) types IV and V harboring a kdp operon in Staphylococcus epidermidis characterized by whole genome sequencing. Infect. Genet. Evol. 61, 60–66. doi: 10.1016/j.meegid.2018.03.012
Ohara-Nemoto, Y., Haraga, H., Kimura, S., and Nemoto, T. K. (2008). Occurrence of staphylococci in the oral cavities of healthy adults and nasal-oral trafficking of the bacteria. J. Med. Microbiol. 57, 95–99. doi: 10.1099/jmm.0.47561-0
Onishi, M., Urushibara, N., Kawaguchiya, M., Ghosh, S., Shinagawa, M., Watanabe, N., et al. (2013). Prevalence and genetic diversity of arginine catabolic mobile element (ACME) in clinical isolates of coagulase-negative staphylococci: identification of ACME type I variants in Staphylococcus epidermidis. Infect. Genet. Evol. 20, 381–388. doi: 10.1016/j.meegid.2013.09.018
Otto, M. (2013). Coagulase-negative staphylococci as reservoirs of genes facilitating MRSA infection: Staphylococcal commensal species such as Staphylococcus epidermidis are being recognized as important sources of genes promoting MRSA colonization and virulence. Bioessays 35, 4–11. doi: 10.1002/bies.201200112
Percival, S. L., McCarty, S., Hunt, J. A., and Woods, E. J. (2014). The effects of pH on wound healing, biofilms, and antimicrobial efficacy. Wound Repair Regen. 22, 174–186. doi: 10.1111/wrr.12125
Planet, P. J., LaRussa, S. J., Dana, A., Smith, H., Xu, A., Ryan, C., et al. (2013). Emergence of the epidemic methicillin-resistant Staphylococcus aureus strain USA300 coincides with horizontal transfer of the arginine catabolic mobile element and speG-mediated adaptations for survival on skin. mBio 4:e889-13. doi: 10.1128/mBio.00889-13
Price-Whelan, A., Poon, C. K., Benson, M. A., Eidem, T. T., Roux, C. M., Boyd, J. M., et al. (2013). Transcriptional profiling of Staphylococcus aureus during growth in 2 M NaCl leads to clarification of physiological roles for Kdp and Ktr K+ uptake systems. mBio 4:e407-13. doi: 10.1128/mBio.00407-13
Renvert, S., Lindahl, C., Renvert, H., and Persson, G. R. (2008). Clinical and microbiological analysis of subjects treated with Branemark or Astra Tech implants: a 7-year follow-up study. Clin. Oral Implants Res. 19, 342–347. doi: 10.1111/j.1600-0501.2007.01476.x
Renvert, S., and Polyzois, I. (2018). Treatment of pathologic peri-implant pockets. Periodontol 2000, 180–190. doi: 10.1111/prd.12149
Rolo, J., de Lencastre, H., and Miragaia, M. (2012). Strategies of adaptation of Staphylococcus epidermidis to hospital and community: amplification and diversification of SCCmec. J. Antimicrob. Chemother. 67, 1333–1341. doi: 10.1093/jac/dks068
Salvi, G. E., Fürst, M. M., Lang, N. P., and Persson, G. R. (2008). One-year bacterial colonization patterns of Staphylococcus aureus and other bacteria at implants and adjacent teeth. Clin. Oral Implants Res. 19, 242–248. doi: 10.1111/j.1600-0501.2007.01470.x
Sanz, M., and Chapple, I. L. (2012). Clinical research on peri-implant diseases: consensus report of Working Group 4. J. Clin. Periodontol. 39, 202–206. doi: 10.1111/j.1600-051X.2011.01837.x
Shore, A. C., and Coleman, D. C. (2013). Staphylococcal cassette chromosome mec: recent advances and new insights. Int. J. Med. Microbiol. 303, 350–359. doi: 10.1016/j.ijmm.2013.02.002
Shore, A. C., Rossney, A. S., Brennan, O. M., Kinnevey, P. M., Humphreys, H., Sullivan, D. J., et al. (2011). Characterization of a novel arginine catabolic mobile element (ACME) and staphylococcal chromosomal cassette mec composite island with significant homology to Staphylococcus epidermidis ACME type II in methicillin-resistant Staphylococcus aureus genotype. Antimicrob. Agents Chemother. 55, 1896–1905. doi: 10.1128/AAC.01756-10
Sievers, F., Wilm, A., Dineen, D., Gibson, T. J., Karplus, K., Li, W., et al. (2011). Fast, scalable generation of high-quality protein multiple sequence alignments using Clustal Omega. Mol. Syst. Biol. 7:539. doi: 10.1038/msb.2011.75
Song, Z., Borgwardt, L., Hoiby, N., Wu, H., Sorensen, T. S., and Borgwardt, A. (2013). Prosthesis infections after orthopedic joint replacement: the possible role of bacterial biofilms. Orthop. Rev. 5, 65–71. doi: 10.4081/or.2013.e14
Soroush, S., Jabalameli, F., Taherikalani, M., Amirmozafari, N., Imani Fooladi, A. A., Asadollahi, K., et al. (2016). Investigation of biofilm formation ability, antimicrobial resistance and the staphylococcal cassette chromosome mec patterns of methicillin resistant Staphylococcus epidermidis with different sequence types isolated from children. Microb. Pathog. 93, 126–130. doi: 10.1016/j.micpath.2016.01.018
Thomas, J. C., Vargas, M. R., Miragaia, M., Peacock, S. J., Archer, G. L., and Enright, M. C. (2007). Improved multilocus sequence typing scheme for Staphylococcus epidermidis. J. Clin. Microbiol. 45, 616–619. doi: 10.1128/jcm.01934-06
Thomas, J. C., Zhang, L., and Robinson, D. A. (2014). Differing lifestyles of Staphylococcus epidermidis as revealed through Bayesian clustering of multilocus sequence types. Infect. Genet. Evol. 22, 257–264. doi: 10.1016/j.meegid.2013.06.020
Thurlow, L. R., Joshi, G. S., Clark, J. R., Spontak, J. S., Neely, C. J., Maile, R., et al. (2013). Functional modularity of the arginine catabolic mobile element contributes to the success of USA300 methicillin-resistant Staphylococcus aureus. Cell Host Microbe 13, 100–107. doi: 10.1016/j.chom.2012.11.012
Thurnheer, T., and Belibasakis, G. N. (2016). Incorporation of staphylococci into titanium-grown biofilms: an in vitro “submucosal” biofilm model for peri-implantitis. Clin. Oral Implants Res. 27, 890–895. doi: 10.1111/clr.12715
Tolo, I., Thomas, J. C., Fischer, R. S. B., Brown, E. L., Gray, B. M., and Robinson, D. A. (2016). Do Staphylococcus epidermidis genetic clusters predict isolation sources? J. Clin. Microbiol. 54, 1711–1719. doi: 10.1128/JCM.03345-15
Wu, Z., Li, F., Liu, D., Xue, H., and Zhao, X. (2015). Novel type XII staphylococcal cassette chromosome mec harboring a new cassette chromosome recombinase, CcrC2. Antimicrob. Agents Chemother. 59, 7597–7601. doi: 10.1128/AAC.01692-15
Xue, T., You, Y., Hong, D., Sun, H., and Sun, B. (2011). The Staphylococcus aureus KdpDE two-component system couples extracellular K+ sensing and Agr signaling to infection programming. Infect. Immun. 79, 2154–2167. doi: 10.1128/IAI.01180-10
Keywords: ACME, Staphylococcus epidermidis, periodontal disease, peri-implantitis, subgingival sites, oral cavity, periodontal pockets, kdp operon
Citation: O’Connor AM, McManus BA, Kinnevey PM, Brennan GI, Fleming TE, Cashin PJ, O’Sullivan M, Polyzois I and Coleman DC (2018) Significant Enrichment and Diversity of the Staphylococcal Arginine Catabolic Mobile Element ACME in Staphylococcus epidermidis Isolates From Subgingival Peri-implantitis Sites and Periodontal Pockets. Front. Microbiol. 9:1558. doi: 10.3389/fmicb.2018.01558
Received: 18 April 2018; Accepted: 22 June 2018;
Published: 12 July 2018.
Edited by:
Peter Mullany, University College London, United KingdomReviewed by:
Christopher L. Hemme, University of Rhode Island, United StatesJose Luiz Proenca-Modena, Universidade Estadual de Campinas, Brazil
Copyright © 2018 O’Connor, McManus, Kinnevey, Brennan, Fleming, Cashin, O’Sullivan, Polyzois and Coleman. This is an open-access article distributed under the terms of the Creative Commons Attribution License (CC BY). The use, distribution or reproduction in other forums is permitted, provided the original author(s) and the copyright owner(s) are credited and that the original publication in this journal is cited, in accordance with accepted academic practice. No use, distribution or reproduction is permitted which does not comply with these terms.
*Correspondence: David C. Coleman, ZGF2aWQuY29sZW1hbkBkZW50YWwudGNkLmll
†These authors have contributed equally to this work.