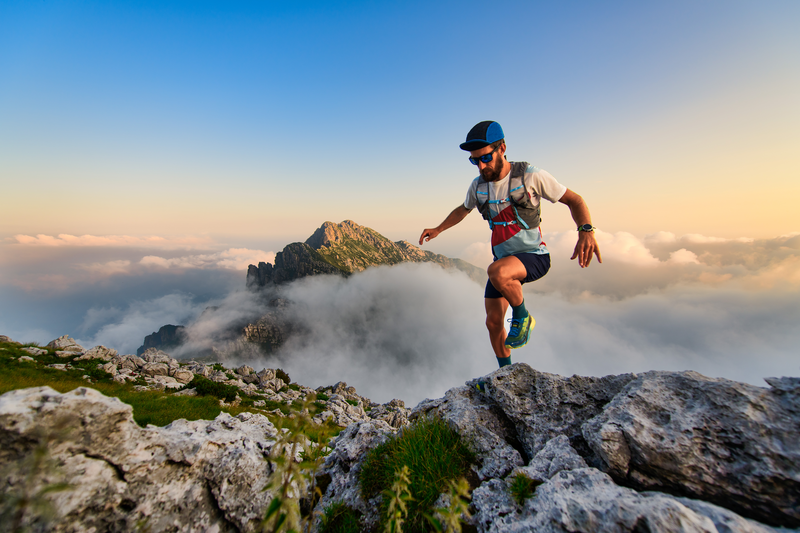
94% of researchers rate our articles as excellent or good
Learn more about the work of our research integrity team to safeguard the quality of each article we publish.
Find out more
ORIGINAL RESEARCH article
Front. Microbiol. , 03 July 2018
Sec. Infectious Agents and Disease
Volume 9 - 2018 | https://doi.org/10.3389/fmicb.2018.01472
Bacterial lipoproteins are a set of membrane proteins with various functions; many of which are virulence factors of pathogenic bacteria. In the present study, we investigated the role of an outer membrane lipoprotein Lip40 in the pathogenesis of Actinobacillus pleuropneumoniae. A mutant strain (Δlip40) lacking Lip40 and a complemented strain (CΔlip40) were constructed. Δlip40 exhibited reduced adherence to the St. Jude porcine lung cells. The ability of the Δlip40 mutant to colonize the mouse lung tissues was significantly impaired compared to that of the wild type and complementation strains. Furthermore, an infection assay revealed that pigs infected with Δlip40 showed fewer clinical signs and lung lesions, indicating that Lip40 contributed to the development of porcine pleuropneumonia. Collectively, our data suggest that Lip40 is involved in the virulence of A. pleuropneumoniae.
Actinobacillus pleuropneumoniae is a Gram-negative bacterium that is the causative agent of porcine pleuropneumonia, which is often characterized by hemorrhagic, necrotic pneumonia and fibrinous pleuropneumonia and associated with large economic losses worldwide (Fenwick and Henry, 1994). A total of 16 serovars of A. pleuropneumoniae have been classified based on the antigenicity of capsular polysaccharide (CPS) and/or lipopolysaccharide (LPS) (Sárközi et al., 2015). All these serovars of A. pleuropneumoniae are able to cause the disease. Although the pathogenesis of A. pleuropneumoniae infection is not fully understood, colonization, immune evasion and damage to the host are known as the three basic stages (Bossé et al., 2002). Several factors are involved in these processes, such as exotoxins, CPS, LPS, adhesins, proteases, outer membrane proteins and transcriptional regulators (Chiers et al., 2010).
Bacterial lipoproteins are a set of multifunctional membrane proteins that are characterized by lipid modification at the conserved N-terminal cysteine residue (Okuda and Tokuda, 2011). Bacterial lipoproteins play various roles in cellular processes, such as nutrient transport, cell division, cellular structure maintenance, and signal transduction (Okuda and Tokuda, 2011). Lipoproteins are important in many aspects of virulence of pathogenic bacteria, including adhesion, invasion, colonization, immune evasion, and immunomodulation (Kovacs-Simon et al., 2011). Although many lipoproteins are predicted in A. pleuropneumoniae (Chung et al., 2007; Hu et al., 2015), the functions of these putative lipoproteins in the physiological and pathogenic activities have rarely been investigated.
A putative lipoprotein, Lip40, was identified in our previous study (Hu et al., 2015). It is located on the outer membrane and is stress responsive and immunoprotective. We assumed that Lip40 might be linked to the pathogenicity of A. pleuropneumoniae. In this study, we constructed a Lip40 knockout mutant (Δlip40) and the corresponding complementation strain (CΔlip40) based on A. pleuropneumoniae SLW01 (serovar 1). Here, we examined the cell adherence, colonization, and virulence of these A. pleuropneumoniae strains.
The bacterial strains, plasmids and primers used in this study are listed in Table 1. A. pleuropneumoniae strains were cultured on trypic soy agar (TSA; Dickinson and Company, Franklin Lakes, NJ, United States) or in tryptic soy broth (TSB), supplemented with 10 μg/ml nicotinamide adenine dinucleotide (NAD+; Sigma, St. Louis, MO, United States) and 5% fetal calf serum (Gibco BRL, Grand Island, NY, United States). During the construction of A. pleuropneumoniae lip40 mutants, 5 μg/ml chloramphenicol was added for the selection of single crossover mutants, 5% sucrose (m/v) was supplemented for double crossover mutant selection, and 2 μg/ml chloramphenicol was used for complementation strain selection. Escherichia coli β2155 transformed with transconjugation plasmid was grown on Luria–Bertani (LB) agar (Oxoid, Basingstoke, Hants, United Kingdom) or in LB broth with 50 μg/ml diaminopimelic acid (Sigma) and ampicillin (100 μg/ml).
The lip40 gene was deleted in the genome by polymerase chain reaction (PCR). After digestion with the appropriate restriction enzymes, the two homologous arms were ligated into the transconjugation vector pEMOC2 (Baltes et al., 2003), resulting into a lip40 knockout vector, pEΔlip40, which was then transformed into the E. coli β2155 (Oswald et al., 1999) to generate the donor cells for transconjugation. The donor cells were co-cultured with the A. pleuropneumoniae SLW01 for 4 h so as to introduce the plasmid into the recipient cells. After chloramphenicol-mediated positive selection and sucrose-mediated counter-selection, chloramphenicol-sensitive and sucrose-resistant colonies were chosen. The absence of lip40 in the genome of these colonies was confirmed by PCR and sequencing, and named as Δlip40. For construction of the complementation strain, intact lip40 gene was cloned and inserted into the E. coli–A. pleuropneumoniae shuttle vector pJFF224-XN (Frey, 1992), and the resulting complementation plasmid pJFF-lip40 was transformed into lip40 deletion mutant by electroporation. Chloramphenicol-resistant transformants were selected and recognized as CΔlip40. The presence of lip40 gene and shuttle vector was verified by PCR. The transcription of lip40 gene in the SLW01, Δlip40 and CΔlip40 was determined using reverse transcription (RT)-PCR.
The adherence assay was performed using a bacteria–cell model with a monkey origin cell line [formerly known as the St. Jude Porcine Lung Epithelial Line (SJPL)] as described previously (Li et al., 2011). Briefly, A. pleuropneumoniae fresh culture at mid-log phase (OD600 ∼0.8) was harvested and washed three times with Dulbecco’s modified Eagle’s medium (DMEM; Gibco BRL). Bacteria were incubated with monolayers of SJPL cells in 6-well plates with a multiplicity of infection of ∼100:1 for 2 h. Planktonic and loosely attached bacteria were removed by five washes of DMEM. To measure the number of adherent bacteria per well, cells were resuspended in distilled water and cell-associated bacteria were liberated by repeated pipetting. Bacteria were serially diluted and plated onto TSA agar. After incubation for 24 h at 37°C, colonies were counted.
To investigate the role of Lip40 in in vivo colonization, Twenty-four 6-week-old female BALB/c mice were purchased from the Center for Disease Control of Hubei Province (Hubei CDC, Wuhan, China), and divided into four groups of six mice each. Groups I, II and III were inoculated intraperitoneally with 106 colony-forming units (CFUs) in 200 μl TSB of A. pleuropneumoniae SLW01, Δlip40, or CΔlip40; group IV received TSB in the same manner and served as a negative control. Mice were monitored for 24 h and dying mice were euthanized. Surviving mice were euthanized at 24 h after infection. Lung tissue (0.1 g) was removed under sterile conditions from each mouse and homogenated. Bacteria in the lung homogenates were quantified by serial dilution and plating onto TSA plates. After incubation for 24 h at 37°C, bacterial colonies morphologically similar to those of A. pleuropneumoniae were confirmed using PCR with ApxIVA-specific primers (Schaller et al., 2001). All experiments involving live animals were approved by the Animal Care and Use Committee at Central China Normal University. We declare that all animals were treated humanely and in compliance with all applicable institutional animal care guidelines in China.
To evaluate the influence of Lip40 on the pathogenicity of A. pleuropneumoniae, a pig infection assay was performed as described previously (Liu et al., 2009). Eighteen 7-week-old pigs were purchased from an A. pleuropneumoniae-free herd and randomly divided into four groups, with five pigs in groups I, II and III, and three pigs in group IV. A. pleuropneumoniae fresh cultures at OD600 ∼0.8 were harvest (deduced viable count ∼109 CFU/ml). The cultures were placed on ice and diluted in ice-cold TSB to the desired live cell counts (appropriate 2.5 × 107 CFU for each pig). The viable bacterial counts of these cultures were determined simultaneously by the plate-counting method. Pigs were anesthetized by intravenous injection of ketamine (4 mg/kg) and xylazine (2 mg/kg) before infection. Pigs in groups I, II, and III were injected intratracheally with A. pleuropneumoniae SLW01, Δlip40, or CΔlip40 suspended in 5 mL TSB, separately. Pigs in group IV received 5 ml TSB and served as a negative control. Clinical signs of pigs were monitored for 7 days post-infection (dpi). Dying pigs with severe dyspnea and low temperature (<38°C) were humanely euthanized. At 0, 6, 12, 24, 36, 48, 60, and 72 hour post-infection (hpi), rectal temperature was measured. Pigs with a temperature >41°C were considered to have high fever. Other clinical signs, such as appetite decrease, breathing difficulty and lethargy were monitored and evaluated as described previously (Fuller et al., 2000). Blood samples were collected every morning before infection and after infection, blood glucose (BGlu) was detected using a kit from Nanjing Jiancheng Biotech, Co., Ltd. (Nanjing, China). At 7 dpi, the surviving pigs were euthanized for postmortem examinations. Lung tissues were excluded carefully and fibrinous adhesion to pleura was recorded. The lung lesion scores were determined as described previously (Hannan et al., 1982). For histological analysis, lung samples were fixed in 10% formalin buffer (pH 7.2). Thin sections (5 μm) were stained using hemotoxylin and eosin and examined by light microscopy.
The data obtained from the present study were expressed as mean ± SD. Student’s t-test was used to compare the differences between two groups for adherence and colonization abilities, clinical signs, BGlu levels and pathological lesions. P < 0.05 was considered significant, and P < 0.01 were considered highly significant.
After sucrose counter-selection, these chloramphenicol-sensitive and sucrose-resistant transconjugants were selected and named as Δlip40. During PCR verification, SLW01 showed a long fragment (2917 bp), whereas Δlip40 exhibited a short truncated fragment (2020 bp) using primers Lip40-F1 and Lip40-R2 (Supplementary Figure S1A), and the mutant was negative in PCR using primers pEM-F and pEM-R (Supplementary Figure S1A). CΔlip40 was constructed based on Δlip40. The E. coli–A. pleuropneumoniae shuttle vector pJFF-lip40 was confirmed to be stable in CΔlip40, when they were passaged in the medium without antibiotic pressure (Supplementary Figure S1B). Besides, both SLW01 and CΔlip40 were positive in the RT-PCR analysis, whereas no amplicon was obtained from the lip40 gene deleted mutant Δlip40 (Supplementary Figure S1C). Growth curves of the A. pleuropneumoniae in the TSB medium showed no obvious differences between the WT and mutant strains (Supplementary Figure S1D), suggesting that mutation in lip40 and transformation with pJFF-lip40 did not affect the growth of A. pleuropneumoniae.
To understand better the roles of Lip40 in A. pleuropneumoniae infection, we analyzed the adherence of the A. pleuropneumoniae mutant to SJPL cells. Viable bacteria before and after interaction with SJPL cells were grown on the TSA plates and the CFUs were counted. The adherence of Δlip40 was significantly lower than that of SLW01 (P < 0.01, Figure 1A). The decrease in adherence was recovered by trans-complementation, the difference in cell adherence between SLW01 and CΔlip40 was not significant (P > 0.05, Figure 1A). The results indicated that absence of Lip40 could lead to reduced adherence of A. pleuropneumoniae to SJPL cells.
FIGURE 1. Roles of Lip40 in the Actinobacillus pleuropneumoniae Adherence (A) and Colonization (B). The adherence assays were performed as described previously (Li et al., 2011). Bacteria adhered to the surface of cells were cultured on TSA plates and CFUs were counted. The adherence assay was repeated at least three times. The percentage CFU was normalized to the WT group and designated as 100%. For the in vivo colonization assay, lung tissues were taken under sterile conditions and homogenized. Homogenates were diluted in TSB and plated onto TSA agar. Bacteria loads were calculated according to the colonies on the plates and dilution ratio (CFU/g). Double asterisks represent highly statistically significant differences (P < 0.01).
The cell adhesion assay revealed that mutation of Lip40 significantly decreased the cell adherence of A. pleuropneumoniae. To understand better the effects of Lip40 deletion on the virulence-associated phenotypes, an in vivo colonization assay was carried out using a mouse infection model. BALB/c mice infected with WT, Δlip40 and CΔlip40 were monitored intensively for 24 h. Lung tissues of all these mice were removed under sterile conditions and weighed immediately after the animals were euthanized. Lung homogenates were diluted and cultured on TSA plates. The negative control group showed no visible colonies on the plates. The average lung bacterial load of the WT group (3.5 × 107 CFU/g) was ∼4.6-fold more than that of the Δlip40 group (7.6 × 106 CFU/g) (Figure 1B, P < 0.01). The average bacterial load of the CΔlip40 group was 3.9 × 107 CFU/g, which was significantly higher than that of the Δlip40 group (P < 0.01). Results suggest that Lip40 is involved in in vivo colonization.
The results of the cell adherence and bacterial load assays suggest that Lip40 is related to A. pleuropneumoniae infection. Therefore, the role of Lip40 in bacterial virulence was determined using a pig infection model. Pigs inoculated with SLW01 and CΔlip40 strains developed typical clinical symptoms of porcine pleuropneumonia, including high temperature, reduced appetite, accelerated breathing, cough and lethargy, whereas pigs infected with Δlip40 mutant showed milder signs. Clinical sign scores of pigs were monitored and summarized in Table 2. Rectal temperature of pigs at eight time points after infection was monitored. Pigs in the negative control group had normal temperature. The infected pigs showed elevated temperature at 6 hpi; temperature in the Δlip40-infected group gradually recovered from 12 hpi and returned to normal at 24 hpi; in the SLW01- and CΔlip40-infected groups, fever remained and recovered after 48 hpi. Pigs in groups I and III exhibited reduced willingness to take food, and more food was left in their hoppers; the appetite indexes were significantly higher than those of the animals infected with Δlip40 (P < 0.05) and in the negative control group (P < 0.01) (Table 2). Pigs infected with SLW01 and CΔlip40 moved slowly or laid down; the lethargy indexes of pigs in these groups were significantly higher than those of the Δlip40 and control groups (P < 0.01) (Table 2). Pigs in groups I and III exhibited significantly higher dyspnea indexes than those of the Δlip40 and control groups (P < 0.01) (Table 2). One pig in the SLW01 infected group developed severe symptoms at 3 dpi and was euthanized; other pigs were alive during the observation period. These results indicated that mutation in lip40 dramatically reduced the ability of A. pleuropneumoniae to cause clinical signs in pigs.
Blood glucose was determined every morning before and after infection. Δlip40-infected animals showed slight but not significant alteration of BGlu at 24 hpi, relative to that in the negative control group (Figure 2). BGlu levels in pigs in the SLW01- and CΔlip40-infected groups dropped significantly at 24 hpi, compared to those in the Δlip40-infected group (P < 0.01). The BGlu level of a seriously diseased pig in the SLW01-infected group was only half the normal level at 72 hpi, and this pig was then euthanized for ethical reasons. These results revealed that the mutation in lip40 alleviates changes in BGlu caused by A. pleuropneumoniae infection.
FIGURE 2. Detection of Blood Glucose (BGlu) in Pigs Inoculated with A. pleuropneumoniae. BGlu were evaluated using detection kits produced by Nanjing Jiancheng Biotech, Co., Ltd. (Nanjing, China). aSignificant difference between the SLW01-infected group (P < 0.05); bP < 0.01 compared with the CΔlip40-infected group; cP < 0.01 compared with the SLW01-infected group.
The lung tissues in the SLW01 and CΔlip40 groups were red with several dark areas, and showed a rough pleural surface. In contrast, the lungs of Δlip40-infected pigs were pink with no obvious changes. SLW01 and CΔlip40 induced significantly higher lung lesion scores than Δlip40 did (P < 0.01) (Table 2). The lung sections from the SLW01- and CΔlip40-infected groups were different from those from Δlip40-infected pigs. A mass of inflammatory cells, erythrocytes and exudations were observed in the alveoli of pigs in the SLW01 and CΔlip40 groups (Figure 3), suggesting that these pigs had hemorrhagic pneumonia. Pigs infected with Δlip40 exhibited normal lung sections except for the presence of slightly thickened pleura and alveolar walls (Figure 3). These results indicated that Lip40 contributed significantly to the virulence of A. pleuropneumoniae.
FIGURE 3. Histopathological examinations. Pigs were inoculated with A. pleuropneumoniae WT, Δlip40, CΔlip40 or TSB (from A–D). Scale bar at the lower right corner of each picture represents 10 μm. (A) Numerous inflammatory cells, erythrocytes and exudations were observed in the alveoli of pigs infected with WT. (B) Lung sections of Δlip40-infected pigs exhibited slightly thickened pleura and alveolar walls. (C) Presence of erythrocytes in alveoli indicated hemorrhagic pneumonia in the CΔlip40-inoculated pigs. (D) Normal lung tissue of the TSB control group.
Rapid attachment to the respiratory tract and efficient adaptation to the challenging in vivo environment are required for the development of bacterial disease. Many lipoproteins have been found to contribute to these processes, and are considered to be involved in the pathogenicity of bacteria of public health importance (Becker and Sander, 2016), as well as pathogens of veterinary importance (Hatfaludi et al., 2010). A. pleuropneumoniae is one of the most important swine pathogens causing severe damage to the respiratory system. Involvement of lipoproteins in the virulence of A. pleuropneumoniae has been reported previously. The transferrin-binding protein TbpB is important for bacterial virulence and enables A. pleuropneumoniae to utilize porcine transferrin (Baltes et al., 2002). Besides, a recent study indicated that another virulence-associated lipoprotein VacJ of A. pleuropneumoniae was implicated in outer membrane integrity maintenance, biofilm formation, and complement resistance (Xie et al., 2016). These findings revealed the significance of lipoproteins in A. pleuropneumoniae infection. However, sixty lipoproteins have been predicted from A. pleuropneumoniae genome (Hu et al., 2015), only few lipoproteins have been investigated. Therefore, more studies are still needed to gain better understanding of the roles of A. pleuropneumoniae lipoproteins.
Lip40 of A. pleuropneumoniae was annotated as a hypothetical protein during genome sequencing analysis (Xu et al., 2008). It was presumed to be required for the virulence of A. pleuropneumoniae (Hu et al., 2015). In this study, we confirmed that Lip40 mediated adhesion of the bacteria to SJPL cells. Although the SJPL cell line is of monkey origin (Silversides et al., 2010), it is useful for investigating A. pleuropneumoniae–cell interactions (Auger et al., 2009; Li et al., 2011). Cell adherence is recognized as one of the most important factors that determines the entry of pathogenic bacteria into the host, and is associated with the virulence and pathogenicity (Stones and Krachler, 2015). Several factors, like ApfA and Adh, have been shown to mediate adherence of A. pleuropneumoniae to host cells, vaccination with these adhesins provided effective protection against lethal A. pleuropneumoniae challenge in murine models (Zhou et al., 2013; Wang et al., 2015). Besides, mutation in the coding sequences of these adhesins reduced A. pleuropneumoniae virulence to animals (Zhou et al., 2013; Wang et al., 2015). Our previous study demonstrated that Lip40 is an immunoprotective agent (Hu et al., 2015), together with the present result, indicating the importance of this adhesin in A. pleuropneumoniae infection. Moreover, Lip40 was not only involved in adhesion to host cells, but also found to be required for in vivo colonization in this study. This inferred that the failure of in vivo proliferation may be partially due to the reduced capacity of bacteria–cell adherence. Colonization is critical for the virulence of pathogenic bacteria (Siegel and Weiser, 2015). Previous studies have revealed that mutation in A. pleuropneumoniae potential virulence factors TolC2, PdxS/PdxT, and SapA results in reduced in vivo colonization, as well as attenuated virulence (Li et al., 2017; Xie et al., 2017a,b). Therefore, it is reasonable to speculate that Lip40 plays an important role in the virulence of A. pleuropneumoniae, by mediating bacterial adherence and colonization.
Actinobacillus pleuropneumoniae is considered to be an important pathogen that has pigs as its natural host, despite several studies indicating that mice can be used for A. pleuropneumoniae immunization and infection assays (Zhou et al., 2013). Therefore, a pig infection model was used to confirm whether Lip40 contributes to the virulence of A. pleuropneumoniae. The pig infection model has been commonly used to investigate relevant genes involved in the pathogenesis of A. pleuropneumoniae infection and evaluate the efficacy of vaccines against A. pleuropneumoniae (Hannan et al., 1982; Fuller et al., 2000; Liu et al., 2009). A set of clinical sign indexes, postmortem examination as well as histological analysis, make the assessment of porcine pleuropneumonia efficient and reliable. In this study, we found that pigs in the Δlip40-infected group developed less clinical symptoms, much milder necrotic lung lesions and histopathological lesions, relative to those of the SLW01-infected group. Trans-complementation of the mutant restored these virulence-associated phenotypes. Additionally, we found that the BGlu levels of the WT and CΔlip40 groups were lower than those of the Δlip40 group, at 24 and 48 hpi. The decreased appetite may have been partly responsible for the drop in BGlu levels. Besides, hypoglycemia was considered as an indicator of bacterial infection, and associated with high mortality during pneumococcal infections (Jan et al., 2009). Here, we observed that the BGlu level of one pig in the SLW01-infected group dropped to half the normal level at 72 hpi; this pig was in poor condition and died soon after the biopsy, this pig showed extensive pleural adhesion and necrotic lung lesions in the subsequent postmortem examination, indicating that it had severe pleuropneumonia. Taken together, these results confirmed that Lip40 was required for the infection of A. pleuropneumoniae.
The roles of Lip40 protein in the pathogenesis of A. pleuropneumoniae have been investigated in the present study. Our results suggest that Lip40 protein is involved in bacterial cell adhesion. We also illustrated that Lip40 contributes to in vivo colonization. The A. pleuropneumoniae Lip40 knockout strain exhibited reduced virulence in the pig infection model. Further investigations of the potential of Lip40 protein as part of a subunit vaccine would be valuable for the prevention of A. pleuropneumoniae infection, and studies focusing on the Lip40–host cell interaction and structural features of Lip40 protein may provide new insight into the pathogenesis of A. pleuropneumoniae.
CQ and QG designed the research and provided experiment conditions. JL and CQ wrote the paper. JL, YC, LG, and LZ executed the experiments. DY, JZ, and JM contributed to the animal experiments. SG, JY, and HZ helped with the data analysis. All authors reviewed and approved the manuscript.
This research was supported by grants from the National Natural Science Foundation of China (31101820, 31272645, and 31672284) and the project of Hubei Key Laboratory of Genetic Regulation and Integrative Biology (GRIB201707).
The authors declare that the research was conducted in the absence of any commercial or financial relationships that could be construed as a potential conflict of interest.
We would like to thank Dr. Chen Tan (at College of Veterinary Medicine, Huazhong Agricultural University, Wuhan, China), and Dr. Shun Lu and Mr. Caiyao Zhan (at Wuhan Institute of Animal Husbandry and Veterinary Science, Wuhan Academy of Agricultural Sciences, Wuhan, China) for their kind assistance in the pig infection experiment.
The Supplementary Material for this article can be found online at: https://www.frontiersin.org/articles/10.3389/fmicb.2018.01472/full#supplementary-material
FIGURE S1 | Verification of Actinobacillus pleuropneumoniae mutants and growth curves. (A) PCR verification of the A. pleuropneumoniae gene-deleted mutant primer pairs Lip40-F1/R2 and pEM-F/R. Lane M, DNA ladder DL15000 (Takara, Dalian, China); lane 1, WT; lane 2, single cross-over transconjugant; lanes 3 to 6, Δlip40. (B) PCR analysis of the complementation strain CΔlip40 using primers pJF-F and pJF-R. Lane M, DNA ladder DL2000; lanes 1 to 10, CΔlip40 of different passages; lane 11, positive control (plasmid pJFF-lip40); lane 12, negative control. (C) RT-PCR confirmation. RNA samples were extracted from A. pleuropneumoniae WT, Δlip40 and CΔlip40 cultures and reverse-transcribed into cDNA, separately. The cDNA was used as template in the subsequently PCR analysis with primers Lip40-F4 and Lip40-R4. Lane M, DNA ladder DL2000; lane 1, WT; lane 2, Δlip40; lane 3, CΔlip40; lane 4, negative control. (D) Growth curves of the A. pleuropneumoniae WT, Δlip40 and CΔlip40. The growth curves were obtained from the average of at least three repeats.
Auger, E., Deslandes, V., Ramjeet, M., Contreras, I., Nash, J. H., Harel, J., et al. (2009). Host-pathogen interactions of Actinobacillus pleuropneumoniae with porcine lung and tracheal epithelial cells. Infect. Immun. 77, 1426–1441. doi: 10.1128/IAI.00297-08
Baltes, N., Hennig-Pauka, I., and Gerlach, G. F. (2002). Both transferrin binding proteins are virulence factors in Actinobacillus pleuropneumoniae serotype 7 infection. FEMS Microbiol. Lett. 209, 283–287. doi: 10.1111/j.1574-6968.2002.tb11145.x
Baltes, N., Tonpitak, W., Hennig-Pauka, I., Gruber, A. D., and Gerlach, G. F. (2003). Actinobacillus pleuropneumoniae serotype 7 siderophore receptor FhuA is not required for virulence. FEMS Microbiol. Lett. 220, 41–48. doi: 10.1016/S0378-1097(03)00064-8
Becker, K., and Sander, P. (2016). Mycobacterium tuberculosis lipoproteins in virulence and immunity - fighting with a double-edged sword. FEBS Lett. 590, 3800–3819. doi: 10.1002/1873-3468.12273
Bossé, J. T., Janson, H., Sheehan, B. J., Beddek, A. J., Rycroft, A. N., Kroll, J. S., et al. (2002). Actinobacillus pleuropneumoniae: pathobiology and pathogenesis of infection. Microbes Infect. 4, 225–235. doi: 10.1016/S1286-4579(01)01534-9
Chiers, K., De Waele, T., Pasmans, F., Ducatelle, R., and Haesebrouck, F. (2010). Virulence factors of Actinobacillus pleuropneumoniae involved in colonization, persistence and induction of lesions in its porcine host. Vet. Res. 41:65. doi: 10.1051/vetres/2010037
Chung, J. W., Ng-Thow-Hing, C., Budman, L. I., Gibbs, B. F., Nash, J. H., Jacques, M., et al. (2007). Outer membrane proteome of Actinobacillus pleuropneumoniae: LC-MS/MS analyses validate in silico predictions. Proteomics 7, 1854–1865. doi: 10.1002/pmic.200600979
Frey, J. (1992). Construction of a broad host range shuttle vector for gene cloning and expression in Actinobacillus pleuropneumoniae and other Pasteurellaceae. Res. Microbiol. 143, 263–269. doi: 10.1016/0923-2508(92)90018-J
Fuller, T. E., Thacker, B. J., Duran, C. O., and Mulks, M. H. (2000). A genetically-defined riboflavin auxotroph of Actinobacillus pleuropneumoniae as a live attenuated vaccine. Vaccine 18, 2867–2877. doi: 10.1016/S0264-410X(00)00076-1
Hannan, P. C., Bhogal, B. S., and Fish, J. P. (1982). Tylosin tartrate and tiamutilin effects on experimental piglet pneumonia induced with pneumonic pig lung homogenate containing mycoplasmas, bacteria and viruses. Res. Vet. Sci. 33, 76–88.
Hatfaludi, T., Al-Hasani, K., Boyce, J. D., and Adler, B. (2010). Outer membrane proteins of Pasteurella multocida. Vet. Microbiol. 144, 1–17. doi: 10.1016/j.vetmic.2010.01.027
Hu, X., Yan, H., Liu, K., Hu, J., Qi, C., Yang, J., et al. (2015). Identification and characterization of a novel stress-responsive outer membrane protein Lip40 from Actinobacillus pleuropneumoniae. BMC Biotechnol. 15:106. doi: 10.1186/s12896-015-0199-8
Jan, I. S., Tsai, T. H., Chen, J. M., Jerng, J. S., Hsu, H. F., Hung, P. L., et al. (2009). Hypoglycemia associated with bacteremic pneumococcal infections. Int. J. Infect. Dis. 13, 570–576. doi: 10.1016/j.ijid.2008.08.026
Kovacs-Simon, A., Titball, R. W., and Michell, S. L. (2011). Lipoproteins of bacterial pathogens. Infect. Immun. 79, 548–561. doi: 10.1128/IAI.00682-10
Li, L., Xu, Z., Zhou, Y., Li, T., Sun, L., Chen, H., et al. (2011). Analysis on Actinobacillus pleuropneumoniae LuxS regulated genes reveals pleiotropic roles of LuxS/AI-2 on biofilm formation, adhesion ability and iron metabolism. Microb. Pathog. 50, 293–302. doi: 10.1016/j.micpath.2011.02.002
Li, Y., Cao, S., Zhang, L., Yuan, J., Yang, Y., Zhu, Z., et al. (2017). TolC2 is required for the resistance, colonization and virulence of Actinobacillus pleuropneumoniae. J. Med. Microbiol. 66, 1170–1176. doi: 10.1099/jmm.0.000544
Lin, L., Bei, W., Sha, Y., Liu, J., Guo, Y., Liu, W., et al. (2007). Construction and immunogencity of a DeltaapxIC/DeltaapxIIC double mutant of Actinobacillus pleuropneumoniae serovar 1. FEMS Microbiol. Lett. 274, 55–62. doi: 10.1111/j.1574-6968.2007.00813.x
Liu, J., Chen, X., Tan, C., Guo, Y., Chen, Y., Fu, S., et al. (2009). In vivo induced RTX toxin ApxIVA is essential for the full virulence of Actinobacillus pleuropneumoniae. Vet. Microbiol. 137, 282–289. doi: 10.1016/j.vetmic.2009.01.011
Okuda, S., and Tokuda, H. (2011). Lipoprotein sorting in bacteria. Annu. Rev. Microbiol. 65, 239–259. doi: 10.1146/annurev-micro-090110-102859
Oswald, W., Tonpitak, W., Ohrt, G., and Gerlach, G. (1999). A single-step transconjugation system for the introduction of unmarked deletions into Actinobacillus pleuropneumoniae serotype 7 using a sucrose sensitivity marker. FEMS Microbiol. Lett. 179, 153–160. doi: 10.1111/j.1574-6968.1999.tb08721.x
Sárközi, R., Makrai, L., and Fodor, L. (2015). Identification of a proposed new serovar of Actinobacillus pleuropneumoniae: serovar 16. Acta Vet. Hung. 63, 444–450. doi: 10.1556/004.2015.041
Schaller, A., Djordjevic, S. P., Eamens, G. J., Forbes, W. A., Kuhn, R., Kuhnert, P., et al. (2001). Identification and detection of Actinobacillus pleuropneumoniae by PCR based on the gene apxIVA. Vet. Microbiol. 79, 47–62. doi: 10.1016/S0378-1135(00)00345-X
Siegel, S. J., and Weiser, J. N. (2015). Mechanisms of bacterial colonization of the respiratory tract. Annu. Rev. Microbiol. 69, 425–444. doi: 10.1146/annurev-micro-091014-104209
Silversides, D. W., Music, N., Jacques, M., Gagnon, C. A., and Webby, R. (2010). Investigation of the species origin of the St. Jude Porcine Lung epithelial cell line (SJPL) made available to researchers. J. Virol. 84, 5454–5455. doi: 10.1128/JVI.00042-10
Stones, D. H., and Krachler, A. M. (2015). Fatal attraction: how bacterial adhesins affect host signaling and what we can learn from them. Int. J. Mol. Sci. 16, 2626–2640. doi: 10.3390/ijms16022626
Wang, L., Qin, W., Yang, S., Zhai, R., Zhou, L., Sun, C., et al. (2015). The Adh adhesion domain is required for trimeric autotransporter Apa1-mediated Actinobacillus pleuropneumoniae adhesion, autoaggregation, biofilm formation and pathogenicity. Vet. Microbiol. 177, 175–183. doi: 10.1016/j.vetmic.2015.02.026
Xie, F., Li, G., Wang, Y., Zhang, Y., Zhou, L., Wang, C., et al. (2017a). Pyridoxal phosphate synthases PdxS/PdxT are required for Actinobacillus pleuropneumoniae viability, stress tolerance and virulence. PLoS One 12:e0176374. doi: 10.1371/journal.pone.0176374
Xie, F., Li, G., Zhang, W., Zhang, Y., Zhou, L., Liu, S., et al. (2016). Outer membrane lipoprotein VacJ is required for the membrane integrity, serum resistance and biofilm formation of Actinobacillus pleuropneumoniae. Vet. Microbiol. 183, 1–8. doi: 10.1016/j.vetmic.2015.11.021
Xie, F., Wang, Y., Li, G., Liu, S., Cui, N., Langford, P. R., et al. (2017b). The SapA protein is involved in resistance to antimicrobial peptide PR-39 and virulence of Actinobacillus pleuropneumoniae. Front. Microbiol. 8:811. doi: 10.3389/fmicb.2017.00811
Xu, Z., Zhou, Y., Li, L., Zhou, R., Xiao, S., Wan, Y., et al. (2008). Genome biology of Actinobacillus pleuropneumoniae JL03, an isolate of serotype 3 prevalent in China. PLoS One 3:e1450. doi: 10.1371/journal.pone.0001450
Keywords: Actinobacillus pleuropneumoniae, Lip40, adherence, colonization, virulence
Citation: Liu J, Cao Y, Gao L, Zhang L, Gong S, Yang J, Zhao H, Yang D, Zhao J, Meng J, Gao Q and Qi C (2018) Outer Membrane Lipoprotein Lip40 Modulates Adherence, Colonization, and Virulence of Actinobacillus pleuropneumoniae. Front. Microbiol. 9:1472. doi: 10.3389/fmicb.2018.01472
Received: 10 May 2018; Accepted: 13 June 2018;
Published: 03 July 2018.
Edited by:
Yuji Morita, Meiji Pharmaceutical University, JapanReviewed by:
Martha H. Mulks, Michigan State University, United StatesCopyright © 2018 Liu, Cao, Gao, Zhang, Gong, Yang, Zhao, Yang, Zhao, Meng, Gao and Qi. This is an open-access article distributed under the terms of the Creative Commons Attribution License (CC BY). The use, distribution or reproduction in other forums is permitted, provided the original author(s) and the copyright owner(s) are credited and that the original publication in this journal is cited, in accordance with accepted academic practice. No use, distribution or reproduction is permitted which does not comply with these terms.
*Correspondence: Qishuang Gao, ODUwODgxNjY1QHFxLmNvbQ== Chao Qi, cWljaGFvQG1haWwuY2NudS5lZHUuY24=
Disclaimer: All claims expressed in this article are solely those of the authors and do not necessarily represent those of their affiliated organizations, or those of the publisher, the editors and the reviewers. Any product that may be evaluated in this article or claim that may be made by its manufacturer is not guaranteed or endorsed by the publisher.
Research integrity at Frontiers
Learn more about the work of our research integrity team to safeguard the quality of each article we publish.