- 1Microbiology and Genetics Department, University of Salamanca, Salamanca, Spain
- 2Spanish-Portuguese Institute for Agricultural Research (CIALE), Salamanca, Spain
- 3Institute of Microbiology of the Czech Academy of Sciences, Vestec, Czechia
- 4Institute of Natural Resources and Agrobiology of Salamanca, IRNASA-CSIC, Salamanca, Spain
- 5Associated R&D Unit, USAL-CSIC (IRNASA), Salamanca, Spain
Antimicrobial resistance is a worldwide problem that threatens the effectiveness of treatments for microbial infection. Consequently, it is essential to study unexplored niches that can serve for the isolation of new microbial strains able to produce antimicrobial compounds to develop new drugs. Bark beetles live in phloem of host trees and establish symbioses with microorganisms that provide them with nutrients. In addition, some of their associated bacteria play a role in the beetle protection by producing substances that inhibit antagonists. In this study the capacity of several bacterial strains, isolated from the bark beetles Ips acuminatus, Pityophthorus pityographus Cryphalus piceae, and Pityogenes bidentatus, to produce antimicrobial compounds was analyzed. Several isolates exhibited the capacity to inhibit Gram-positive and Gram-negative bacteria, as well as fungi. The genome sequence analysis of three Pseudomonas isolates predicted the presence of several gene clusters implicated in the production of already described antimicrobials and moreover, the low similarity of some of these clusters with those previously described, suggests that they encode new undescribed substances, which may be useful for developing new antimicrobial agents. Moreover, these bacteria appear to have genetic machinery for producing antitumoral and antiviral substances. Finally, the strain IA19T showed to represent a new species of the genus Pseudomonas. The 16S rRNA gene sequence analysis showed that its most closely related species include Pseudomonas lutea, Pseudomonas graminis, Pseudomonas abietaniphila and Pseudomonas alkylphenolica, with 98.6, 98.5 98.4, and 98.4% identity, respectively. MLSA of the housekeeping genes gyrB, rpoB, and rpoD confirmed that strain IA19T clearly separates from its closest related species. Average nucleotide identity between strains IA19T and P. abietaniphila ATCC 700689T, P. graminis DSM 11363T, P. alkylphenolica KL28T and P. lutea DSM 17257T were 85.3, 80.2, 79.0, and 72.1%, respectively. Growth occurs at 4-37°C and pH 6.5-8. Optimal growth occurs at 28°C, pH 7–8 and up to 2.5% NaCl. Respiratory ubiquinones are Q9 (97%) and Q8 (3%). C16:0 and in summed feature 3 are the main fatty acids. Based on genotypic, phenotypic and chemotaxonomic characteristics, the description of Pseudomonas bohemica sp. nov. has been proposed. The type strain is IA19T (=CECT 9403T = LMG 30182T).
Introduction
During thousands of years, natural molecules obtained from plants, animals or microorganisms have been the main source of drugs to treat human illnesses. However, the field of chemistry has greatly influenced the availability of drugs by using combinatorial chemistry to produce new molecules (Cragg and Newman, 2009; Mishra and Tiwari, 2011; Dias et al., 2012; Harvey et al., 2015). Currently, natural sources are receiving more attention, as the production of new drugs chemically is becoming more restricted, and it is becoming more difficult to solve the problems that arise in association with Public Health (Ravelo and Braun, 2009; Harvey et al., 2015).
Antimicrobial resistance is a global problem that threatens the effectiveness of the treatment of microbial infections. During the last few decades, the number of multidrug resistant microbes is exponentially increasing, and the WHO warns that if this tendency persists, the number of human deaths derived from microbial infections will be higher by 2050 than those resulting from cancer. This means that antimicrobial resistance is one of the major menaces to public health at the present time (Levy and Marshall, 2004; Hoffman et al., 2015; Premanandh et al., 2016).
The complex characteristics of natural molecules could make them more suitable than chemically synthesized compounds for fighting disease, since they are more similar to the endogenous metabolites of an organism. Also, the complexity of natural molecules sometimes impedes their synthesize using chemical methods (Balamurugan et al., 2005; Ravelo and Braun, 2009; Drewry and Macarron, 2010; Harvey et al., 2015). Therefore, the study of unexplored ecological niches that can serve as a novel source for the isolation of new microbial strains able to produce antimicrobial compounds, which could serve as a basis for the development of new drugs effective in the treatment of microbial infections, is of utmost importance (Kennedy et al., 2007; Cragg and Newman, 2009; Piel, 2011; Harvey et al., 2015).
Bark beetles (Curculionidae, Scolytinae) belong to a group of insects that live in and feed on the phloem, the inner layer of the bark, in their host trees (Six, 2012). As many other bark beetles, they establish a symbiotic relationship with microorganisms that provide nutrients to the insect (García-Fraile, 2018). In addition, it has also been reported that some of the bacteria associated with these beetles play a role in the protection of the beetle holobiont –the insect and its microbial symbionts– by producing substances that inhibit the development of pathogens and other antagonists (García-Fraile, 2018).
Pseudomonas is a genus of bacteria found in a wide range of habitats (for a revision see Peix et al., 2018) and also includes bacteria that are commonly associated with bark beetles (Adams et al., 2009; Boone et al., 2013; Hu et al., 2014; Menéndez et al., 2015; Xu et al., 2015, 2016). Many strains belonging to this genus are used as biocontrol agents for inhibiting some plant pathogens (for a revision see Olorunleke et al., 2015), which further supports their capability to produce useful antimicrobials. Moreover, many drugs coming from Pseudomonas strains with interesting clinical applications have been described. Thus, the search for Pseudomonas strains associated with bark beetles is interesting from the point of view of discovering new antimicrobials and other compounds that may be of potential use to the pharmaceutical industry.
As regards, the aim of this work was to study the potential of bacterial bark beetles associates to inhibit Gram positive and negative bacteria and fungi. In addition, a genome sequence analysis was carried out to test the capacity of some of the most promising strains belonging to the genus Pseudomonas to produce antimicrobial compounds, which could lead to the development of new antibiotics. Finally, based on phenotypic, genotypic and chemotaxonomic tests, we describe one of the new isolates, strain IA19T, as a new species of the genus Pseudomonas.
Materials and Methods
Strains Used in This Study
Bacterial strains used in this study were isolated from adult bark beetles of several species -Ips acuminatus, Cryphalus piceae, Pityophthorus pityographus, and Pityogenes bidentatus (all Coleoptera, Scolytinae)-. The isolation of the bacterial associates of C. piceae and P. pityographus has been previously described in Fabryová et al. (2018). The newly obtained bacteria isolated in this study were obtained from I. acuminatus and P. bidentatus beetles, extracted from branches from Pinus sylvestris exhibiting the typical boring holes of bark beetles which were collected in May 2016 in Stará Boleslav, Czech Republic (coordinates: 50°12′59.5″N, 14°41′58.4″E). The branches were taken to the laboratory and then, under aseptic conditions, the bark was removed, and the bark beetles were sorted into 5 groups of 3 individuals each. The beetles were crushed using sterile toothpicks in 500 μl of sterile water. Serial dilutions were made using the suspensions obtained, and 100 μl of each dilution were plated onto Nutrient Agar (NA) and Tryptose Soy Agar (TSA).
The plates were incubated at 28°C for 2 weeks, and the emerging bacterial colonies with different morphologies were regularly passed to new plates in order to obtain pure cultures. The isolated strains were stored in a sterile 20% glycerol solution at −80°C and sub-cultured regularly in the corresponding isolation medium.
Bacterial Identification and Genotypic Analysis
To identify the 21 bacterial isolates obtained in this study, total DNA was extracted and the 16S rRNA gene was amplified and sequenced as previously reported (Rivas et al., 2007). Almost complete (~1,400 bp) 16S rRNA sequences were compared with those available in GenBank using the BLASTn program (Altschul et al., 1990) and EzTaxon tool (Kim et al., 2012). The remaining 40 strains used in the antimicrobial screening were identified as detailed by Fabryová et al. (2018).
The amplification and sequencing of the housekeeping genes gyrB, rpoB, and rpoD was performed as described by Menéndez et al. (2015).
The phylogenetic analysis of the 16S rRNA gene sequence and the concatenated sequences of the gyrB, rpoB, and rpoD housekeeping genes of strain IA19T and all the sequences of the closely related species of the genus Pseudomonas was done using the MEGA7 software (Kumar et al., 2016), based on the Clustal_W alignment (Thompson et al., 1994; Larkin et al., 2007). The distances were calculated using the Kimura's two-parameter model (Kimura, 1980) and the phylogenetic trees were generated using maximum-likelihood (ML; Rogers et al., 1998) and neighbor-joining (NJ; Saitou and Nei, 1987) analyses.
The average nucleotide identity (ANI) values between the genome sequence of strain IA19T and the genome sequences of the type strains of the closest related species were estimated by using ANI Calculator in the EZBioCloud (http://www.ezbiocloud.net).
The mol % G+C content of DNA was determined from the complete genome sequence.
Chemotaxonomic Analysis
Biomass for the analysis of fatty acid methyl esters (FAME) and respiratory quinones of strain IA19T and its closest related type was harvested after the strains were cultivated for 2 days on TSA medium at 28°C. For FAME analyses, the cells were collected from the plates and placed into sterile plastic tubes and freeze dried. The extraction of fatty acids was carried out as described by Sasser (1990) and analyzed using the Microbial Identification System (MIDI) Sherlock 6.1 together with the library RTSBA6. Quinones extraction and identification were performed at the Identification of Microorganisms Service at the DSMZ, were they are extracted using methanol:hexane (Tindall, 1990a,b), followed by phase separation into hexane, separated into their different classes by thin layer chromatography on silica gel (Macherey-Nagel Art. No. 805 023), using hexane:tert-butylmethylether (9:1 v/v) as solvent. UV absorbing bands corresponding to the different quinone classes are removed from the plate and analyzed by HPLC.
Bacterial Characterization
Gram-staining of strain IA19T was carried out following the protocol described by Doetsch (1981), and motility was checked by phase-contrast microscopy after growing the cells at 22°C for 48 h in NA. The type of flagellation was determined by electron microscopy as previously described by García-Fraile et al. (2015).
Tryptose Soy Broth (TSB) medium supplemented with 0–10% (w/v) NaCl was used to assay salt tolerance in IA19T. The same medium, with an adjusted final pH in the range of 4–10, was used for studying the growth capability of the strain at different pHs; in both cases the cultures were incubated at 28°C for up to 1 week. Also, cells were cultured on TSA plates at 4, 8, 22, 28, 37, and 42°C to determine the temperature range for growth. In all cases the presence of growth was checked during 1 week.
For the catalase test, bacterial cells growing in an TSA agar plate were collected and drops of 30% H2O2 were added over them to detect the formation of bubbles after 5 min, indicating a positive result. The oxidase test was performed following the protocol described by Kovacs (1956).
Finally, the phenotypic characterization of strain IA19T and the type strains of the closest related species was completed using the API20NE and API50CH (bioMerieux) systems.
Screening for Antimicrobial Production
The antimicrobial activity screen using the 61 isolates analyzed in this study was carried out on 6 indicator strains: A Gram-negative bacterium, Klebsiella oxytoca, a Gram-positive bacterium, Arthrobacter phenanthrenivorans two yeast-like fungi, Candida humilis and Pichia fermentans and two filamentous fungi, Aspergillus sp. and Fusarium sp.
To analyze the capability of each of the isolates to inhibit the different bacteria and yeast-like indicator strains, cross streak method was used. Each of our isolates was seeded by a single streak in the center of a NA plate. After 5 days of incubation at 28°C, the plates were seeded with the indicator microorganisms by single streaks perpendicular to the central one. After additional 48h incubation, the growth of each indicator strain was analyzed. To study the inhibition of filamentous fungi, our isolates were seeded by a single streak in the center of a NA plate. After 5 days of pre-incubation at 28°C, fungi were inoculated 2 cm far from the central strike by 0.5 cm mycelia discs. After further incubation under suitable conditions for the fungal strains tested (25°C), the diameters of fungal growth in control and sample plates were measured, and the antifungal effect was evaluated.
Draft Genome Sequencing and Annotation
The genomic DNA for genome sequencing was obtained from bacterial cells of strains IA19T, A2-NA12, and A2-NA13 grown on NA plates and collected after 24 h at 28°C, using the ZR Fungal/Bacterial DNA MiniPrep (Zymo Research).
The draft genome sequences of the selected isolates were obtained by shotgun sequencing on an Illumina MiSeq platform via a paired-end run (2 × 251 bp). The sequence data were assembled using Velvet 1.2.10 (Zerbino and Birney, 2008) and a draft genome was obtained. Gene calling and annotation was performed using RAST 2.0 (Rapid Annotation using Subsystem Technology) (Aziz et al., 2008). The SEED-viewer framework (Overbeek et al., 2014) was used for a first mining of genes related to antimicrobial production genes. Moreover, a more specific and detailed analysis of the presence of gene clusters related to antimicrobial substances productions and other secondary metabolites was performed using antiSMASH 3.0 (Weber et al., 2015).
Results
Bacterial Isolation and Identification
The list of bacterial isolates analyzed in this work, as well as their identification based on their partial (≈1,400 bp) 16S rRNA sequence, is presented in Table 1. The list includes the isolates from I. acuminatus and P. bidentatus, obtained in this study, and the ones from the bark beetles C. piceae and P. pityographus obtained in a study that tested their capacity to degrade plant cells (Fabryová et al., 2018).
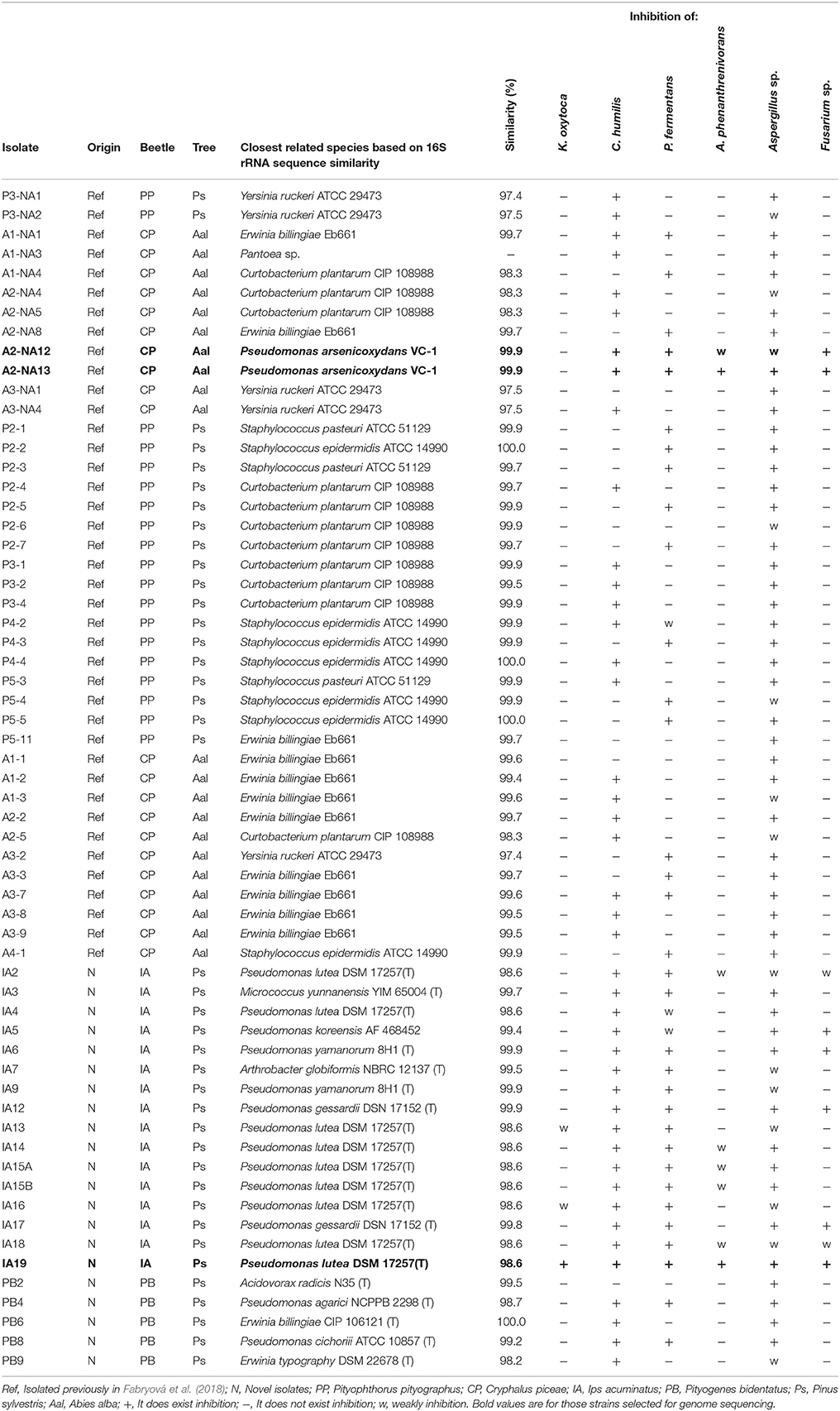
Table 1. List of the bacterial strains analyzed in this study and their capability to inhibit reference microbial strains.
Phylogenetic Analyses and Average Nucleotide Identity (ANI) Comparison
Analysis of the 16S rRNA gene sequence of strain IA19T suggested that this isolate was a member of the genus Pseudomonas but could also belong to a new species within this genus. The most closely related species were P. lutea DSM 17257T, (98.6% identity), P. graminis DSM 11363T, (98.5% identity), P. abietaniphila ATCC 700689T, (98.4% identity) and P. alkylphenolica KL28T, (98.4% identity).
ML and NJ trees including all related species within the genus Pseudomonas showed a similar 16S rRNA sequence phylogenetic clustering, in which strain IA19T clustered with P. alkylphenolica KL28T in a broader cluster that also contained P. lutea OK2T and P. graminis DSM 11363T (Figure 1 and Supplementary Figure 1). Nevertheless, the phylogenetic distances between strain IA19T and its closest related species were broader than those distances among several other different species belonging to this genus, suggesting its classification into a different species. However, the limitations of the analysis based on 16S rRNA gene sequences to discriminate the genus Pseudomonas sufficiently at the inter-species level have been described (Ramírez-Bahena et al., 2014). Therefore, the Mutli-Locus Sequence Analysis (MLSA) based on the three housekeeping genes gyrB, rpoB, and rpoD was used for species classification in Pseudomonas (Ait Tayeb et al., 2005; Mulet et al., 2009, 2010, 2012; Ramos et al., 2013; Toro et al., 2013; Ramírez-Bahena et al., 2014; Menéndez et al., 2015). Both the NJ and ML analysis of the concatenated housekeeping gyrB, rpoB and rpoD genes sequences (Figure 2 and Supplementary Figure 2) showed that the strain IA19T clustered with P. abitaniphila DSM 17554T, but the phylogenetic distance between both strains clearly indicated that they belonged to different species. The sequence similarities between strains IA19T and P. abietaniphila DSM 17554T housekeeping genes rpoD, rpoB, and gyrB were 89.3, 92.4, and 87.5%, respectively. These values were below those commonly found among different but closely related species of the genus Pseudomonas (Ramírez-Bahena et al., 2014).
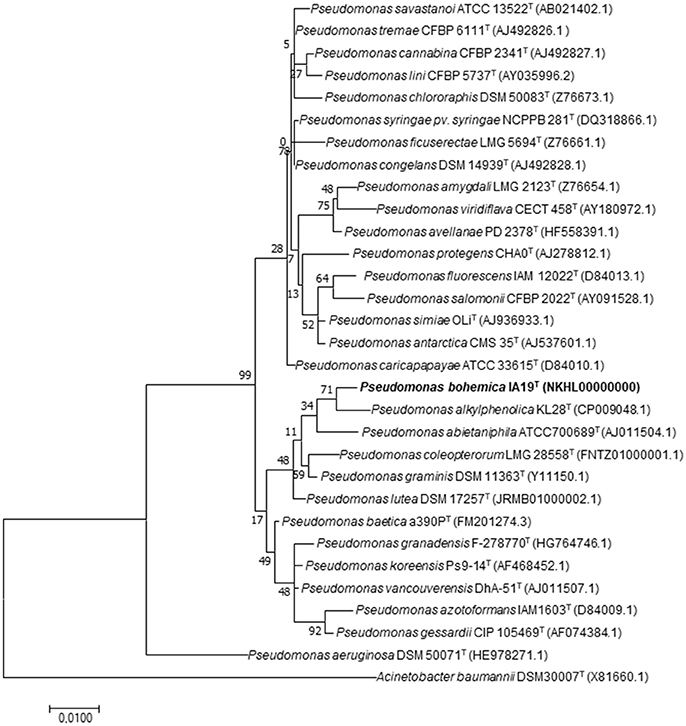
Figure 1. Maximum-likelihood phylogenetic tree based on nearly complete (1,400 bp) 16S rRNA gene sequences of all Pseudomonas species closely related to P. bohemica IA19T and the species Acinetobacter baumannii DSM30007 T, which was included as an outgroup. Bootstrap values (expressed as percentages of 1,000 replications) are shown at the branching points. Scale bar = 1 nucleotide (nt) substitutions per 100 nt. Accession numbers of the sequences are indicated in parentheses.
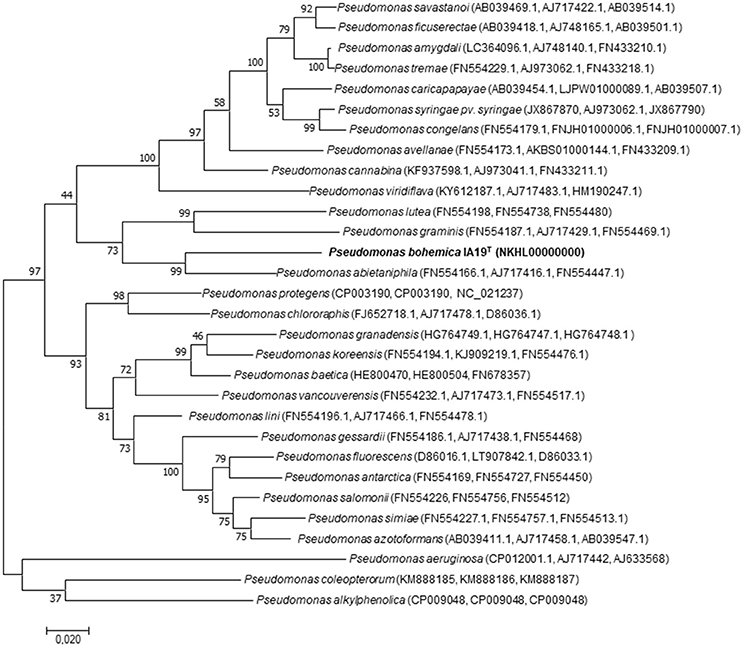
Figure 2. Maximum-likelihood phylogenetic tree based on concatenated partial gyrB, rpoB, rpoD gene sequences of strain P. bohemica IA19T and closely related species of the genus Pseudomonas. Bootstrap values (expressed as percentages of 1,000 replications) are shown at the branching points. Scale bar = Bar, 2 nucleotide (nt) substitutions per 100 nt. Accession numbers of the sequences are indicated in parentheses.
Finally, authenticity of the novel species was confirmed by ANI comparison between strain IA19T and the genome sequence of the type strains of its closest related strains P. abietaniphila ATCC 700689T (GenBank access number: FNCO00000000.1, draft genome), P. graminis DSM 11363T (GenBank access number: NZ_FOHW01000000.1, draft genome), P. alkylphenolica KL28T (GenBank access number: CP009048.1, complete genome) and P. lutea DSM 17257T (GenBank access number: FOEV01000000.1, draft genome) showed values of were 85.31, 80.21, 79.0, and 72.15%, respectively (Table 2). Considering that a threshold value of 95–96% ANI has been established for defining a bacterial species (Richter and Rosselló-Móra, 2009), our results indicated that strain IA19T represented a distinct species of the genus Pseudomonas.
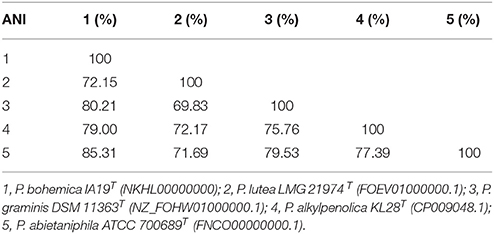
Table 2. Average nucleotide identity (ANI) comparison between strain IA19T and the genome sequence of its closest relatives.
Colony and Cellular Morphology
The strain IA19T formed round, bright, clear beige and convex colonies with entire border on TSA medium, which were visible after 24 h of incubation at 28°C; these colonies grew to a size of 1–3 mm after 72 h of growth. Also, growth was observed in the temperature range of 4–37°C, where the optimum temperature was at 28°C, and at a pH range of 6.5 and 8, with 7 being the optimum. Cells were Gram-negative, rod-shaped and motile by means of a polar flagellum (Figure 3).
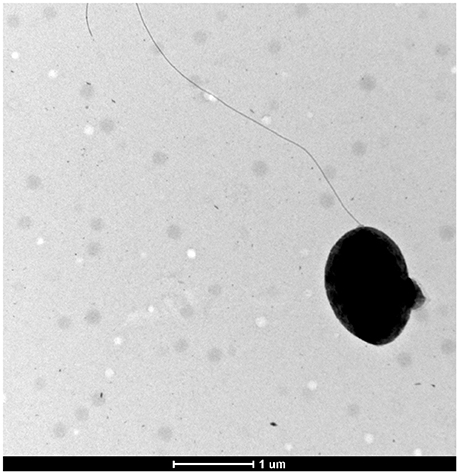
Figure 3. Electron micrograph showing the morphology and flagellation type of Pseudomonas bohemica IA19T.
Phenotypic and Chemotaxonomic Characterization
The analysis of isopropenoid quinones showed that the isolate IA19T contained ubiquinone-9 (Q9) (97%) as the main respiratory quinone, which is one of the most common quinone systems in those strains belonging to the genus Pseudomonas (Oyaizu and Komagata, 1983), and a small amount of ubiquinone-8 (Q8) (3%).
As for its closest related species, the major fatty acids of strain IA19T were 16:0 iso (30.5%) and in summed feature 3 (21.1%), being the whole FAME composition detailed in Table 3.
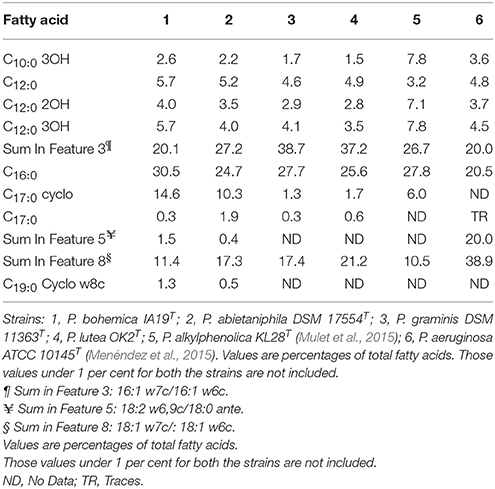
Table 3. Cellular fatty acid composition (%) of Pseudomonas bohemica IA19T and its closest related species.
The main phenotypic features observed for strain IA19T are detailed in the species description, and the main differences found between this strain and its closest related species, as well as the type species of the genus, Pseudomonas aeruginosa, are listed in Table 4.
In Vitro Detection of the Production of Antimicrobial Substances
From the total of 61 isolates, all showed antimicrobial activity against at least one of the indicator strains (Table 1). With the only exception of two strains, the analyzed isolates from this study inhibited the yeast's growth, 4.9% isolates inhibited the growth of K. oxytoca and 13.1% isolates inhibited the growth of A. phenanthrenivorans. Moreover, the strain from Aspergillus sp. was inhibited by all the strains of this study (Table 1).
Several of the isolates obtained in this study appeared to be potential candidates to produce antimicrobial substances, being several strains identified as Pseudomonas some of the best antimicrobial producers, according to our screening. This genus is known to produce antimicrobials and other interesting bioactive compounds (Laine et al., 1996; Stintzi et al., 1996; Raaijmakers et al., 1997; Marinho et al., 2009; Bauer et al., 2015; Nishanth Kumar et al., 2016; Ganne et al., 2017). Based on that, strains Pseudomonas IA19T, Pseudomonas A2-NA12, Pseudomonas A2-NA13 were selected for further analysis of their genetic potential to produce pharmaceutically interesting molecules by sequencing and mining their genomes.
Genomic Properties
The size of the genome of the isolate IA19T was estimated to be 6.487 Mb with 5,961 predicted coding sequences, A2-NA12 was estimated to be 5.933 Mb with 5,148 predicted coding sequences and A2- NA13 was estimated to be 5.938 Mb with 5,172 predicted coding sequences. The GC content was predicted to be 59.5 for IA19T and 59.7 for A2-NA12 and A2-NA13. The features of all three genomes are summarized in Table 5.
Draft genome sequences of strains Pseudomonas bohemica IA19T, A2-NA12 and A2-NA13 were deposited in GenBank under the accession numbers NKHL00000000, PEGA00000000 and PEGB00000000, respectively.
Genome Mining of Biosynthetic Gene Clusters With Potential Interest in the Pharmaceutical Industry
Using the SEED viewer, based on the automatic annotation of the bacterial genomes performed with RAST, it was found that each of the genomes of the three bacteria IA19T, A2-NA12, and A2-NA13 presented a cluster of genes implicated in the production of the peptide antibiotic colicin V. Also, all strains showed genes implicated in the synthesis, reception and transport of siderophores, molecules implicated not only in the acquisition of iron, but also in microbial inhibition (Becerra et al., 2003). Strains A2-NA12 and A2-NA13 appeared to possess clusters of genes implicated in the synthesis of the siderophores pyoverdine and enterobactin. A gene from a cluster related to achromobactin siderophore was annotated in the draft genome sequence of strain IA19T.
The prediction of gene clusters involved in secondary metabolite biosynthesis by antiSMASH version 3.0.5 (Weber et al., 2015) suggested that the genome of the three bacterial isolates contained several potential biosynthetic gene clusters encoding the production of antimicrobial substances, as well as other compounds with potential interest in the pharmaceutical industry, which are listed in Table 6.
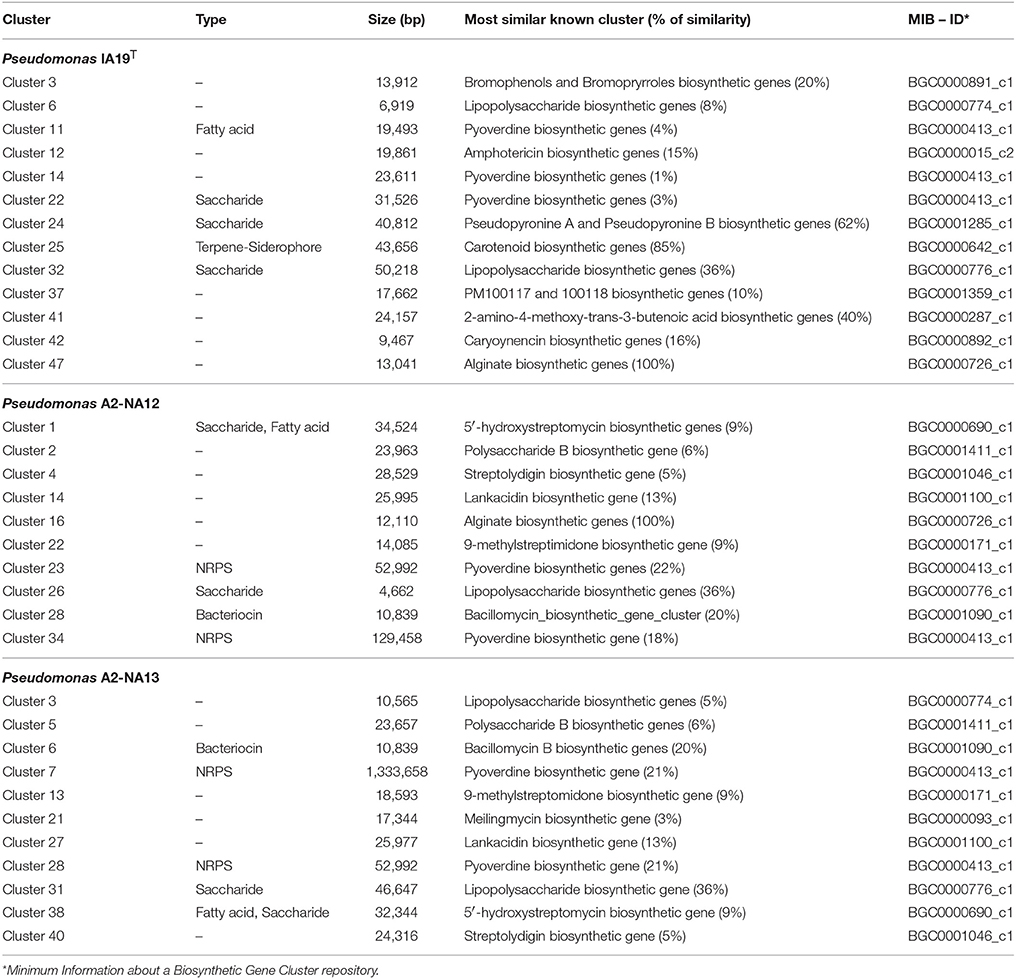
Table 6. Cluster of genes predicted to encode the synthesis of bioactive compounds in the genome sequences of the strains of this study based on the analysis of genome sequences with AntiSMASH 3.0 program.
Specifically, the draft genome of isolate IA19T included 49 gene clusters related to the synthesis of secondary metabolites. Out of these, 13 were predicted to be related to already described metabolic pathways involved in antimicrobials production: 2 polyketide synthases (PKSs), 2 non-ribosomal peptide synthetases (NRPS), a terpene-siderophore hybrid, 2 bromophenols (caryoynecins), 5 saccharides and a fatty acid.
In the case of strain A2-NA12, its draft genome contained 37 clusters encoding secondary metabolites, of which 10 are related to described clusters of genes implicated in the synthesis of enzymes related to the production of antimicrobial compounds: 2 NRPS, a PKS, a bacteriocin, 3 saccharides, a NRPS-PKS hybrid, a NRP-PKS-saccharide hybrid and a fatty acid-saccharide hybrid.
Finally, the draft genome of strain A2-NA13 included 40 clusters encoding the synthesis of secondary metabolites, where 10 of them were associated to known metabolic pathways for antimicrobials synthesis. These included 2 PKS, a lipopolysaccharide, a bacteriocin, 2 NRPS, a NRPS-PKS hybrid, a saccharide, a fatty acid-saccharide hybrid and a NRPS-PKS-saccharide hybrid.
Discussion
Several recent studies have suggested the implication of some bacteria associated with bark beetles in the protection of the bark beetle holobiont. This occurs through the inhibition of antagonists of the beetle itself or the beetle's symbionts, or the detoxification of the bark beetle environment (García-Fraile, 2018). Indeed, all bacterial isolates from this study were able to reduce or inhibit Aspergillus, a fungus which has been shown to greatly reduced the number of larvae in the mountain pine beetle (Therrien et al., 2015) and the spruce bark beetle (Cardoza et al., 2006).
In this study, it has been shown that many bacterial isolates from bark beetles, such as I. acuminatus, C. piceae, P. pityographus, and P. bidentatus, have the potential to produce antimicrobial compounds. Therefore, the genetic potential of some of these isolates to produce antibiotics, as well as other bioactive compounds was further analyzed. Bacteria from the genus Pseudomonas are frequently isolated from bark beetles of different species and life cycle stages (Adams et al., 2009, 2013; Morales-Jiménez et al., 2012; Menéndez et al., 2015; Fabryová et al., 2018; García-Fraile, 2018). Members of the genus Pseudomonas have been broadly studied for their capability to produce several different secondary metabolites with potential biotechnological applications. In several articles, different strains of Pseudomonas are described as biological control agents of plant diseases (Haas and Keel, 2003) and bioactive substances described from Pseudomonas strains are diverse, and include quinolines, pyrroles, pseudopeptide pyrrolidinediones, pseudopyronines, siderophores, phthalates, phenazine, phloroglucinol, benzaldehyde, phenanthren, moiramides, andrimid, zafrin, caryoynencin, and bushrin (Pierson et al., 1994; Sarniguet et al., 1995; Schnider et al., 1995; Yamaguchi et al., 1995; Raaijmakers et al., 1997; Isnansetyo and Kamei, 2009; Marinho et al., 2009; Bauer et al., 2015; Ganne et al., 2017). Therefore, these bacteria may be implicated in the protection of the bark beetle by inhibiting microbial antagonists. In this sense, it was observed that bacteria belonging to this genus were among the best microbial inhibitors in our study. Thus, the genomes of those isolates identified as Pseudomonas were selected and sequenced, and the exploration of their metabolic capacity to produce bioactive compounds revealed the presence of numerous clusters that are potentially implicated in the synthesis of important bioactive metabolites (Table 6).
A cluster related to the synthesis of 2-amino-4-methoxy-3-butenoic acid, which is a compound also found in P. aeruginosa that has been described as being antitumoral (Tisdale, 1980) and to have antiparasitic activity against Acantamoeba castellani and antimicrobial activity against Erwinia amylovora, Bacillus spp. and Escherichia coli (Lee et al., 2010, 2012, 2013), was predicted in strain IA19T.
A cluster of genes for the synthesis of PM100117 and PM100118, two bioactive polyhydroxyl macrolide lactones, which have been isolated from the culture broth of the marine-derived Streptomyces caniferus (Pérez et al., 2016), was discovered in the genome sequence of strain IA19T. It has been shown that these molecules have antitumoral activity as well as slight antifungal activity against Candida albicans (Pérez et al., 2016).
A cluster related to the synthesis of caryoynencin, an antibiotic found in liquid cultures of the plant pathogen Pseudomonas caryophylli (Yamaguchi et al., 1995) and in a Burkholderia sp. isolate from a beetle (Flórez et al., 2017), showing potent antimicrobial activities against Gram-positive and Gram-negative bacteria, as well as antifungal activity, has been predicted in the genome sequence of strain IA19T. The spectrum of activity of the described caryoynencin includes: methicillin-resistant Staphylococcus aureus, Bacillus subtilis, Enterococcus faecalis, Escherichia coli, “Salmonella enteritidis,” Klebsiella pneumoniae, Serratia marcescens, Proteus vulgaris, Shigella flexneri, Enterobacter cloacae, P. aeruginosa, Candida albicans, “Cryptococcus neoformans,” Mucor mucedo, Aspergillus fumigatus, Microsporum gypseum, Trichophyton mentagrophytes, Trichophyton interdigitale, and Trichophyton rubrum (Yamaguchi et al., 1995).
In the genome sequences of the three isolates, a cluster related to the synthesis of a lipopolysaccharide compound, also found in Escherichia coli and described as a potent inhibitor of HIV-1 replication in T lymphocytes and macrophages (Verani et al., 2002), was predicted.
A cluster for the synthesis of a carotenoid was predicted in the genome sequence of strain IA19T. Carotenoids have been reported to have many health benefits, such as prevention of cancer, improvement of visual function and enhancement of immune responses (Sedkova et al., 2005).
A cluster for the synthesis of an alginate, which has been described to improve the efficacy of some antibiotics (Onsoyen et al., 2010), has been predicted in the genome sequence of strains IA19T and A2-NA12.
In the genome sequence of strain IA19T, a cluster for the synthesis of pseudopyronines A and B was predicted. Both substances have antibacterial activity based on selective membrane disruption and inhibition of fatty-acid synthase against Mycobacterium tuberculosis, Bacillus subtilis, Pseudomonas savastanoi, methicillin-resistant Staphylococcus aureus, Moraxella catarrhalis, and vancomycin-resistant Enterococci. This strain also displayed moderate inhibition of other Firmicutes (Listeria welshimeri) and Actinobacteria (Micrococcus luteus, Arthrobacter crystallopoietes, and Corynebacterium xerosis), as well as anti-leishmanial and algaecide activities (Bauer et al., 2015). Moreover, pseudopyronine B has also shown antitumoral activity (Nishanth Kumar et al., 2016). These compounds have been identified in different species of the genus Pseudomonas and in the genus Alteromonas (Bauer et al., 2015).
In the genome sequences of the three bacterial isolates, clusters related to the synthesis of pyoverdine, a siderophore and an iron chelating agent produced also be P. aeruginosa (Ganne et al., 2017), which can act as antibacterial (Becerra et al., 2003), have been predicted. This molecule has also been described as producer of oxidative stress in leukocytes (Becerra et al., 2003). Moreover, when pyoverdina is conjugated with antibiotics it facilitates them to overcome the bacterial membrane (Kinzel et al., 1998; Kinzel and Budzikiewicz, 1999), which could be used against antibiotic resistance.
A cluster of genes predicted to be encoding the synthesis of the bromopyrrole pentabromopseudilin was found within the genome sequence of strain IA19T. Pentabromopseudilin was isolated for the first time from Alteromonas luteoviolacea (Laatsch et al., 1995). It is the most active member in a group of more than 20 pyrrole antibiotics that effectively interferes with the macromolecular syntheses in Gram-positive and Gram-negative bacteria, and also has antifungal activity, as well as the activity against the biosynthesis of cholesterol. In addition, it shows pronounced in vitro activity against experimental leukemia and melanoma cell lines (Laatsch et al., 1995).
In the genome sequences of isolates A2-NA12 and A2-NA13, a cluster related to the synthesis of 5′-hydroxystreptomycin was predicted. This compound is an aminoglycoside antibiotic that can be biosynthesized by different Streptomyces species (Beyer et al., 1998).
The genome sequences of isolates IA19T show a cluster of genes for the biosynthesis of amphotericin, a polyene macrolide produced by Streptomyces nodosus, which is a potent antifungal compound and also has activity against some viruses, protozoans and prions (Caffrey et al., 2001).
A cluster of genes related to that encoding the biosynthesis of streptolydigin, a tetramic acid also produced by Streptomyces lydicus, has been predicted in the genome sequences of strains A2-NA12 and A2-NA13. This compound is a potent antibiotic which inhibits the bacterial RNA polymerase. It can be also used in acute limphoblastic leukemia or even in acute and chronic myelocytic leukemia (Olano et al., 2009).
The genomes of strains A2-NA12 and A2-NA13 both contain a group of genes that have been related to clusters of genes from Streptomyces, which have been described to encode the synthesis of lankacidin, a macrocyclic antibiotic and antitumor against leukemia (Arakawa et al., 2005).
Also, in the genome sequences of strains A2-NA12 and A2-NA13, there are cluster of genes related to other genes implicated in the biosynthesis of 9-methylstreptimidone. This substance is an antifungal (Allen et al., 1976) and antiviral agent which inhibits the growth of polyvirus, vesicular stomatitis virus (VSV) and Newcastle disease virus (NDV) (Saito et al., 1974) and also inhibit NF-κB (nuclear factor-κB) (Ishikawa et al., 2009).
Clusters related to the synthesis of bacillomycin have been predicted in strains A2-NA12 and A2-NA13. This antibiotic, described in Bacillus subtilis, possesses antifungal activity against practically all the important dermatophytes and systemic infectious fungi (Landy et al., 1948).
A cluster related to the synthesis of meilingmycin, which possesses potent, broad-spectrum anthelmintic, insecticidal and acaricidal activities and is produced by Streptomyces nanchangensis (Zhuang et al., 2006), has been found in the genome sequence of the strain A2-NA13.
As shown above, the selected isolates encoded a large number of clusters involved in the synthesis of secondary metabolites. The strains encoded in their genomes 37 (A2-NA12), 40 (A2-NA13), and 49 (IA19T) of these clusters. These values are higher than the average of clusters found in the genomes of other Pseudomonas strains isolated from different origins. For example, recently published genomes of Pseudomonas strains isolated from soil or rhizosphere encode 9 (Hennessy et al., 2015), 13 (Vida et al., 2017) or 16 (Adam et al., 2015) of these clusters; in the same way, Pseudomonas strains isolated from plant tissues present 4 (Wemheuer et al., 2017) or 6 (Maggini et al., 2017) of these clusters. Most of these new genomes have revealed new potentially bioactive compounds in this genus, but importantly, many of the substances predicted in the genome sequences of the strains of this study have never been identified in other Pseudomonas strains. Moreover, the low similarity found between some of the described predicted clusters and those of the microorganisms in the AntiSMASH database seem to indicate that several of those substances are potential new chemical compounds, which increases the interest of these bacteria as potential producers of new medical drugs. These results are in accordance with previous findings in the genomes of bacterial symbionts in other insects (Arnam et al., 2018), demonstrating the large variety of cryptic metabolites encoded by these strains, of which only a small quantity have been characterized so far. Therefore, the genomic information and the clusters of genes predicted to be involved in secondary metabolite biosynthesis of the bacterial isolates of this study will require further analysis regarding the structure and function of the bioactive compounds encoded in their genomes.
Our results show that several Pseudomonas strains associated with bark beetles possess the genetic potential to produce several antimicrobial substances, as well as other chemical compounds with pharmaceutical interest. Although the bacterial strains identified as Pseudomonas were selected and their genome sequences studied, many other bark beetle isolates screened in this study for the capacity to produce antimicrobials seem to have great potential as producers of bioactive compounds. The genome sequencing and mining of other isolates, as well as the production, purification and identification of the predicted bioactive compounds in silico are necessary and could have a potential positive impact on the availability of new compounds for the development of drugs.
Regarding the taxonomic study of the strain IA19T, the analysis of its 16S rRNA sequence supports its classification within the genus Pseudomonas. Nevertheless, the phylogenetic analysis of this gene sequence, as well as the sequences of the housekeeping genes, and those genes of related species within the genus, suggests that IA19T is a new species of the genus Pseudomonas. Furthermore, ANI values with the type strains of its closest related species confirm that this strain belongs to a new species. On the other hand, our isolate differs from its closest related species in several phenotypic characters. Upon considering all phenotypic, chemotaxonomic and phylogenetic data of this study, the strain IA19T appears to represent a novel species within the genus Pseudomonas, for which the name Pseudomonas bohemica sp. nov. is proposed.
Description of Pseudomonas bohemica sp. nov.
Pseudomonas bohemica (bo.he'mi.ca. M.L.adj. related to Bohemia, the region in the Czech Republic were the type strain was isolated). Temperature for growth ranges between 4 and 37, growth pH ranges between 6.5 and 8. Optimal growth occurs at 25°C and pH 7–8. Able to grow with up to 2.5% NaCl in TSB. The respiratory ubiquinones are Q9 (97%) and Q8 (3%). C16:0 (30.5%) and in summed feature 3 (20.1%) are the main fatty acids. Oxidase- and catalase-positive. In the API20NE system, aesculin hydrolysis, assimilation of D-glucose, L-arabinose, D-mannose, D-mannitol, potassium gluconate and trisodium citrate are positive whereas reduction of nitrates, glucose fermentation, production of gelatinase, urease, indole arginine dihydrolase and ß-galactosidase and assimilation of adonitol, methyl-xyloside, N-acetyl glucosamine, D-maltose, caprate, adipate, malate and phenylacetate are negative. In API 50CH, it produces acid from D-glucose, glycerol, ribose, L-xylose, L-fructose, D-mannose, L-arabinose, amigdalin, arbutine and salicin.
The type strain, IA19T (=CECT 9403T = LMG 30182T), was isolated from a bark beetle from the species Ips acuminatus in the Czech Republic. The DNA G+C content of the type strain is 59.5 mol%.
Author Contributions
ZS-S, AJ-G, AD-M, JI performed the experiments. MK made the sampling. RL-M, TV, ZS-S, AJ-G, PG-F performed the bioinformatic analysis of the data. PG-F and RR designed the research project. PG-F, ZS-S, RL-M, RR, EV analyzed the data. PG-F, ZS-S, AJ-G, EV interpreted the results. ZS-S and PG-F contributed to the writing of the manuscript.
Conflict of Interest Statement
The authors declare that the research was conducted in the absence of any commercial or financial relationships that could be construed as a potential conflict of interest.
Acknowledgments
This work was supported by the Czech Science Foundation (GACR) under the project number 16-15293Y. Authors thank A. Fabryová and R. Martínez for their collaboration in the strains isolation and Emma J. Keck for English language edition.
Supplementary Material
The Supplementary Material for this article can be found online at: https://www.frontiersin.org/articles/10.3389/fmicb.2018.00913/full#supplementary-material
Supplementary Figure 1. Neighbor-joining phylogenetic tree based on nearly complete (1,400 bp) 16S rRNA gene sequences of all Pseudomonas species closely related to P. bohemica IA19T and the species Acinetobacter baumannii DSM30007 T, which was included as an outgroup. Bootstrap values (expressed as percentages of 1,000 replications) are shown at the branching points. Scale bar = 2 nucleotides (nt) substitutions per 100 nt. Accession numbers of the sequences are indicated in parentheses.
Supplementary Figure 2. Neighbor-joining phylogenetic tree based on concatenated partial gyrB, rpoB, rpoD and gene sequences of strain P. bohemica IA19T and closely related species of the genus Pseudomonas. Bootstrap values (expressed as percentages of 1,000 replications) are shown at the branching points. Scale bar = 2 nucleotides (nt) substitutions per 100 nt. Accession numbers of the sequences are indicated in parentheses.
References
Adam, Z., Chen, Q., Xu, R., Diange, A. E., Bromfield, E. S. P., and Tambong, J. T. (2015). Draft genome sequence of Pseudomonas simiae strain 2-36, an in vitro antagonist of Rhizoctonia solani and Gaeumannomyces graminis. Genome Announc. 3, e01534–e01514. doi: 10.1128/genomeA.01534-14
Adams, A. S., Aylward, F. O., Adams, S. M., Erbilgin, N., Aukema, B. H., Currie, C. R., et al. (2013). Mountain pine beetles colonizing historical and naïve host trees are associated with a bacterial community highly enriched in genes contributing to terpene metabolism. Appl. Environ. Microbiol. 79, 3468–3475. doi: 10.1128/AEM.00068-13
Adams, A. S., Currie, C. R., Cardoza, Y., Klepzig, K. D., and Raffa, K. F. (2009). Effects of symbiotic bacteria and tree chemistry on the growth and reproduction of bark beetle fungal symbionts. Can. J. For. Res. 39, 1133–1147. doi: 10.1139/X09-034
Ait Tayeb, L., Ageron, E., Grimont, F., and Grimont, P. A. D. (2005). Molecular phylogeny of the genus Pseudomonas based on rpoB sequences and application for the identification of isolates. Res. Microbiol. 156, 763–773. doi: 10.1016/j.resmic.2005.02.009
Allen, M. S., Becker, A. M., and Rickards, R. W. (1976). The glutarimide antibiotic 9-methylstreptimidone: structure, biogenesis and biological activity. Aust. J. Chem. 29, 673–679. doi: 10.1071/ch9760673
Altschul, S. F., Gish, W., Miller, W., Myers, E. W., and Lipman, D. J. (1990). Basic local alignment search tool. J. Mol. Biol. 215, 403–410. doi: 10.1016/S0022-2836(05)80360-2
Arakawa, K., Sugino, F., Kodama, K., Ishii, T., and Kinashi, H. (2005). Cyclization mechanism for the synthesis of macrocyclic antibiotic lankacidin in Streptomyces rochei. Chem. Biol. 12, 249–256. doi: 10.1016/j.chembiol.2005.01.009
Arnam, E. B. V., Currie, R. C., and Clardy, J. (2018). Defense contracts: molecular protection in insect-microbe symbioses. Chem. Soc. Rev. 47, 1638–1651. doi: 10.1039/C7CS00340D
Aziz, R. K., Bartels, D., Best, A. A., DeJongh, M., Disz, T., Edwards, R. A., et al. (2008). The RAST server: rapid annotations using subsystems technology. BMC Genomics 9:75. doi: 10.1186/1471-2164-9-75
Balamurugan, R., Dekker, F. J., and Waldmann, H. (2005). Design of compound libraries based on natural product scaffolds and protein structure similarity clustering (PSSC). Mol. Biosyst. 1, 36–45. doi: 10.1039/B503623B
Bauer, J. S., Ghequire, M. G. K., Nett, M., Josten, M., Sahl, H.-G., De Mot, R., et al. (2015). Biosynthetic origin of the antibiotic Pseudopyronines a and b in Pseudomonas putida BW11M1. ChemBioChem 16, 2491–2497. doi: 10.1002/cbic.201500413
Becerra, M. C., Eraso, A. J., and Albesa, I. (2003). Comparison of oxidative stress induced by ciprofloxacin and pyoverdin in bacteria and in leukocytes to evaluate toxicity. Luminescence 18, 334–340. doi: 10.1002/bio.742
Beyer, S., Mayer, G., and Piepersberg, W. (1998). The StrQ protein encoded in the gene cluster for 5′-hydroxystreptomycin of Streptomyces glaucescens GLA.0 is a α- D-glucose-1-phosphate cytidylyltransferase (CDP- D-glucose synthase). Eur. J. Biochem. 258, 1059–1067. doi: 10.1046/j.1432-1327.1998.2581059.x
Boone, C. K., Keefover-Ring, K., Mapes, A. C., Adams, A. S., Bohlmann, J., and Raffa, K. F. (2013). Bacteria associated with a tree-killing insect reduce concentrations of plant defense compounds. J. Chem. Ecol. 39, 1003–1006. doi: 10.1007/s10886-013-0313-0
Caffrey, P., Lynch, S., Flood, E., Finnan, S., and Oliynyk, M. (2001). Amphotericin biosynthesis in Streptomyces nodosus: deductions from analysis of polyketide synthase and late genes. Chem. Biol. 8, 713–723. doi: 10.1016/S1074-5521(01)00046-1
Cardoza, Y. J., Klepzig, K. D., and Raffa, K. F. (2006). Bacteria in oral secretions of an endophytic insect inhibit antagonistic fungi. Ecol. Entomol. 31, 636–645. doi: 10.1111/j.1365-2311.2006.00829.x
Clark, L. L., Dajcs, J. J., McLean, C. H., Bartell, J. G., and Stroman, D. W. (2006). Pseudomonas otitidis sp. nov., isolated from patients with otic infections. Int. J. Syst. Evol. Microbiol. 56, 709–714. doi: 10.1099/ijs.0.63753-0
Cragg, G. M., and Newman, D. J. (2009). Biodiversity: a continuing source of novel drug leads. Pure Appl. Chem. 77, 7–24. doi: 10.1351/pac200577010007
Dias, D. A., Urban, S., and Roessner, U. (2012). A historical overview of natural products in drug discovery. Metabolites 2, 303–336. doi: 10.3390/metabo2020303
Doetsch, R. N. (1981). “Determinative methods of light microscopy,” in Manual of Methods for General Bacteriology, eds P. Gerdhardt, R. G. E. Murray, R. N. Costilow, E. W. Nester, W. A. Wood, N. R. Krieg, and G. B. Phillips (Washington, DC: American Society for Microbiology), 21–33.
Drewry, D. H., and Macarron, R. (2010). Enhancements of screening collections to address areas of unmet medical need: an industry perspective. Curr. Opin. Chem. Biol. 14, 289–298. doi: 10.1016/j.cbpa.2010.03.024
Fabryová, A., Kostovčík, M., Díez-Méndez, A., Jiménez-Gómez, A., Celador-Lera, L., Saati-Santamaría, Z., et al. (2018). On the bright side of a forest pest-the metabolic potential of bark beetles' bacterial associates. Sci. Total Environ. 619–620, 9–17. doi: 10.1016/j.scitotenv.2017.11.074
Flórez, L. V., Scherlach, K., Gaube, P., Ross, C., Sitte, E., Hermes, C., et al. (2017). Antibiotic-producing symbionts dynamically transition between plant pathogenicity and insect-defensive mutualism. Nat. Commun. 8:15172. doi: 10.1038/ncomms15172
Frasson, D., Opoku, M., Picozzi, T., Torossi, T., Balada, S., Smits, T. H. M., et al. (2017). Pseudomonas wadenswilerensis sp. nov. and Pseudomonas reidholzensis sp. nov., two novel species within the Pseudomonas putida group isolated from forest soil. Int. J. Syst. Evol. Microbiol. 67, 2853–2861. doi: 10.1099/ijsem.0.002035
Ganne, G., Brillet, K., Basta, B., Roche, B., Hoegy, F., Gasser, V., et al. (2017). Iron release from the siderophore pyoverdine in Pseudomonas aeruginosa involves three new actors: FpvC, FpvG, and FpvH. ACS Chem. Biol. 12, 1056–1065. doi: 10.1021/acschembio.6b01077
García-Fraile, P. (2018). Roles of bacteria in the bark beetle holobiont – how do they shape this forest pest? Ann. Appl. Biol. 172, 111–125. doi: 10.1111/aab.12406
García-Fraile, P., Chudíčková, M., Benada, O., Pikula, J., and Kolarík, M. (2015). Serratia myotis sp. nov. and Serratia vespertilionis sp. nov., isolated from bats hibernating in caves. Int. J. Syst. Evol. Microbiol. 65, 90–94. doi: 10.1099/ijs.0.066407-0
Haas, D., and Keel, C. (2003). Regulation of antibiotic production in root-colonizing pseudomonas spp. and relevance for biological control of plant disease. Annu. Rev. Phytopathol. 41, 117–153. doi: 10.1146/annurev.phyto.41.052002.095656
Harvey, A. L., Edrada-Ebel, R., and Quinn, R. J. (2015). The re-emergence of natural products for drug discovery in the genomics era. Nat. Rev. Drug Discov. 14, 111–129. doi: 10.1038/nrd4510
Hennessy, R. C., Glaring, M. A., Michelsen, C. F., Olsson, S., and Stougaard, P. (2015). Draft genome sequence of pseudomonas sp. strain in5 isolated from a greenlandic disease suppressive soil with potent antimicrobial activity. Genome Announc. 3:e01251–15. doi: 10.1128/genomeA.01251-15
Hoffman, S. J., Outterson, K., Røttingen, J.-A., Cars, O., Clift, C., Rizvi, Z., et al. (2015). An international legal framework to address antimicrobial resistance. Bull. World Health Organ. 93, 66–66. doi: 10.2471/BLT.15.152710
Hu, X., Yu, J., Wang, C., and Chen, H. (2014). Cellulolytic bacteria associated with the gut of dendroctonus armandi larvae (coleoptera: curculionidae: scolytinae). Forests 5, 455–465. doi: 10.3390/f5030455
Ishikawa, Y., Tachibana, M., Matsui, C., Obata, R., Umezawa, K., and Nishiyama, S. (2009). Synthesis and biological evaluation on novel analogs of 9-methylstreptimidone, an inhibitor of NF-KB. Bioorg. Med. Chem. Lett. 19, 1726–1728. doi: 10.1016/j.bmcl.2009.01.107
Isnansetyo, A., and Kamei, Y. (2009). Bioactive substances produced by marine isolates of Pseudomonas. J. Ind. Microbiol. Biotechnol. 36, 1239–1248. doi: 10.1007/s10295-009-0611-2
Kennedy, J., Marchesi, J. R., and Dobson, A. D. W. (2007). Metagenomic approaches to exploit the biotechnological potential of the microbial consortia of marine sponges. Appl. Microbiol. Biotechnol. 75, 11–20. doi: 10.1007/s00253-007-0875-2
Kim, O.-S., Cho, Y.-J., Lee, K., Yoon, S.-H., Kim, M., Na, H., et al. (2012). Introducing EzTaxon-e: a prokaryotic 16S rRNA gene sequence database with phylotypes that represent uncultured species. Int. J. Syst. Evol. Microbiol. 62, 716–721. doi: 10.1099/ijs.0.038075-0
Kimura, M. (1980). A simple method for estimating evolutionary rates of base substitutions through comparative studies of nucleotide sequences. J. Mol. Evol. 16, 111–120. doi: 10.1007/BF01731581
Kinzel, O., and Budzikiewicz, H. (1999). Synthesis and biological evaluation of a pyoverdin-β-lactam conjugate: a new type of arginine-specific cross-linking in aqueous solution. J. Pept. Res. 53, 618–625. doi: 10.1034/j.1399-3011.1999.00053.x
Kinzel, O., Tappe, R., Gerus, I., and Budzikiewicz, H. (1998). The synthesis and antibacterial activity of two pyoverdin-ampicillin conjugates, entering Pseudomonas aeruginosa via the pyoverdin-mediated iron uptake pathway. J. Antibiot 51, 499–507. doi: 10.7164/antibiotics.51.499
Kovacs, N. (1956). Identification of Pseudomonas pyocyanea by the oxidase reaction. Nature 178, 703. doi: 10.1038/178703a0
Kumar, S., Stecher, G., and Tamura, K. (2016). MEGA7: molecular evolutionary genetics analysis version 7.0 for bigger datasets. Mol. Biol. Evol. 33, 1870–1874. doi: 10.1093/molbev/msw054
Laatsch, H., Renneberg, B., Hanefeld, U., Kellner, M., Pudleiner, H., Hamprecht, G., et al. (1995). Structure-activity relationships of phenyl- and benzoylpyrroles. Chem. Pharm. Bull. 43, 537–546. doi: 10.1248/cpb.43.537
Laine, M. H., Karwoski, M. T., Raaska, L. B., and Mattila-Sandholm, T.-M. (1996). Antimicrobial activity of Pseudomonas spp. against food poisoning bacteria and moulds. Lett. Appl. Microbiol. 22, 214–218. doi: 10.1111/j.1472-765X.1996.tb01146.x
Landy, M., Warren, G. H., Rosenman, M. S. B., and Colio, L. G. (1948). Bacillomycin: an antibiotic from Bacillus subtilis active against pathogenic fungi*. Proc. Soc. Exp. Biol. Med. 67, 539–541. doi: 10.3181/00379727-67-16367
Larkin, M. A., Blackshields, G., Brown, N. P., Chenna, R., McGettigan, P. A., McWilliam, H., et al. (2007). Clustal W and Clustal X version 2.0. Bioinformatics 23, 2947–2948. doi: 10.1093/bioinformatics/btm404
Lee, X., Azevedo, M. D., Armstrong, D. J., Banowetz, G. M., and Reimmann, C. (2013). The Pseudomonas aeruginosa antimetabolite L-2-amino-4-methoxy-trans-3-butenoic acid inhibits growth of Erwinia amylovora and acts as a seed germination-arrest factor. Environ. Microbiol. Rep. 5, 83–89. doi: 10.1111/j.1758-2229.2012.00395.x
Lee, X., Fox, Á., Sufrin, J., Henry, H., Majcherczyk, P., Haas, D., et al. (2010). Identification of the biosynthetic gene cluster for the pseudomonas aeruginosa antimetabolite l-2-amino-4-methoxy-trans-3-butenoic acid. J. Bacteriol. 192, 4251–4255. doi: 10.1128/JB.00492-10
Lee, X., Reimmann, C., Greub, G., Sufrin, J., and Croxatto, A. (2012). The Pseudomonas aeruginosa toxin L-2-amino-4-methoxy-trans-3-butenoic acid inhibits growth and induces encystment in Acanthamoeba castellanii. Microbes Infect. 14, 268–272. doi: 10.1016/j.micinf.2011.10.004
Levy, S. B., and Marshall, B. (2004). Antibacterial resistance worldwide: causes, challenges and responses. Nat. Med. N. Y. 10(Suppl. 1), S122–S129. doi: 10.1038/nm1145
Maggini, V., Presta, L., Miceli, E., Fondi, M., Bosi, E., Chiellini, C., et al. (2017). Draft genome sequence of pseudomonas sp. strain ep r1 isolated from echinacea purpurea roots and effective in the growth inhibition of human opportunistic pathogens belonging to the burkholderia cepacia complex. Genome Announc. 5:e00351-17. doi: 10.1128/genomeA.00351-17
Marinho, P. R., Moreira, A. P., Pellegrino, F. L., Muricy, G., Bastos Mdo, C., Santos, K. R., et al. (2009). Marine Pseudomonas putida: a potential source of antimicrobial substances against antibiotic-resistant bacteria. Mem. Inst. Oswaldo Cruz 104, 678–682. doi: 10.1590/S0074-02762009000500002
Menéndez, E., Ramírez-Bahena, M. H., Fabryová, A., Igual, J. M., Benada, O., Mateos, P. F., et al. (2015). Pseudomonas coleopterorum sp. nov., a cellulase-producing bacterium isolated from the bark beetle Hylesinus fraxini. Int. J. Syst. Evol. Microbiol. 65, 2852–2858. doi: 10.1099/ijs.0.000344
Mishra, B. B., and Tiwari, V. K. (2011). Natural products: an evolving role in future drug discovery. Eur. J. Med. Chem. 46, 4769–4807. doi: 10.1016/j.ejmech.2011.07.057
Morales-Jiménez, J., Zúñiga, G., Ramírez-Saad, H. C., and Hernández-Rodríguez, C. (2012). Gut-associated bacteria throughout the life cycle of the bark beetle Dendroctonus rhizophagus thomas and bright (Curculionidae: scolytinae) and their cellulolytic activities. Microb. Ecol. 64, 268–278. doi: 10.1007/s00248-011-9999-0
Mulet, M., Bennasar, A., Lalucat, J., and García-Valdés, E. (2009). An rpoD-based PCR procedure for the identification of Pseudomonas species and for their detection in environmental samples. Mol. Cell. Probes 23, 140–147. doi: 10.1016/j.mcp.2009.02.001
Mulet, M., Gomila, M., Lemaitre, B., Lalucat, J., and García-Valdés, E. (2012). Taxonomic characterisation of Pseudomonas strain L48 and formal proposal of Pseudomonas entomophila sp. nov. Syst. Appl. Microbiol. 35, 145–149. doi: 10.1016/j.syapm.2011.12.003
Mulet, M., Lalucat, J., and García-Valdés, E. (2010). DNA sequence-based analysis of the Pseudomonas species. Environ. Microbiol. 12, 1513–1530. doi: 10.1111/j.1462-2920.2010.02181.x
Mulet, M., Sánchez, D., Lalucat, J., Lee, K., and García-Valdés, E. (2015). Pseudomonas alkylphenolica sp. nov. a bacterial species able to form special aerial structures when grown on p-cresol. Int. J. Syst. Evol. Microbiol. 65, 4013–4018. doi: 10.1099/ijsem.0.000529
Nishanth Kumar, S., Aravind, S. R., Jacob, J., Gopinath, G., Lankalapalli, R. S., Sreelekha, T. T., et al. (2016). Pseudopyronine B: A potent antimicrobial and anticancer molecule isolated from a pseudomonas mosselii. Front. Microbiol. 7:1307. doi: 10.3389/fmicb.2016.01307
Olano, C., Gómez, C., Pérez, M., Palomino, M., Pineda-Lucena, A., Carbajo, R. J., et al. (2009). Deciphering biosynthesis of the RNA polymerase inhibitor streptolydigin and generation of glycosylated derivatives. Chem. Biol. 16, 1031–1044. doi: 10.1016/j.chembiol.2009.09.015
Olorunleke, F. E., Kieu Phuong, N., and Höfte, M. (2015). “Recent advances in Pseudomonas biocontrol,” in Bacteria-Plant Interactions: Advanced Research and Future Trends, eds J. Murillo, B. A. Vinatzer, R. W. Jackson, and D. L. Arnold (Poole: Caister Academic Press), 167–198.
Onsoyen, E., Myrvold, R., Dessen, A., Thomas, D., and Walsh, T. R. (2010). Treatment of Acinetobacter with Alginate Oligomers and Antibiotics. Patent number: WO2010139956.
Overbeek, R., Olson, R., Pusch, G. D., Olsen, G. J., Davis, J. J., Disz, T., et al. (2014). The SEED and the rapid annotation of microbial genomes using subsystems technology (RAST). Nucleic Acids Res. 42, D206–D214. doi: 10.1093/nar/gkt1226
Oyaizu, H., and Komagata, K. (1983). Grouping of Pseudomonas species on the basis of cellular fatty acid composition and the quinone system with special reference to the existence of 3-hydroxy fatty acids. J. Gen. Appl. Microbiol. 29, 17–40. doi: 10.2323/jgam.29.17
Palleroni, N. (2005). Genus I Pseudomonas Migula 1894, 237. Bergeys Man. Syst. Bacteriol. 2, 323–378.
Peix, A., Ramírez-Bahena, M.-H., and Velázquez, E. (2018). The current status on the taxonomy of Pseudomonas revisited: an update. Infect. Genet. Evol. 57, 106–116. doi: 10.1016/j.meegid.2017.10.026
Pérez, M., Schleissner, C., Fernández, R., Rodríguez, P., Reyes, F., Zuñiga, P., et al. (2016). PM100117 and PM100118, new antitumor macrolides produced by a marine Streptomyces caniferus GUA-06-05-006A. J. Antibiot. 69, 388–394. doi: 10.1038/ja.2015.121
Piel, J. (2011). Approaches to capturing and designing biologically active small molecules produced by uncultured microbes. Annu. Rev. Microbiol. 65, 431–453. doi: 10.1146/annurev-micro-090110-102805
Pierson, L. S., Keppenne, V. D., and Wood, D. W. (1994). Phenazine antibiotic biosynthesis in Pseudomonas aureofaciens 30-84 is regulated by PhzR in response to cell density. J. Bacteriol. 176, 3966–3974. doi: 10.1128/jb.176.13.3966-3974.1994
Premanandh, J., Samara, B. S., and Mazen, A. N. (2016). Race against antimicrobial resistance requires coordinated action – an overview. Front. Microbiol. 6:1536. doi: 10.3389/fmicb.2015.01536
Raaijmakers, J. M., Weller, D. M., and Thomashow, L. S. (1997). Frequency of antibiotic-producing pseudomonas spp. in natural environments. Appl. Environ. Microbiol. 63, 881–887.
Ramírez-Bahena, M.-H., Cuesta, M. J., Flores-Félix, J. D., Mulas, R., Rivas, R., Castro-Pinto, J., et al. (2014). Pseudomonas helmanticensis sp. nov., isolated from forest soil. Int. J. Syst. Evol. Microbiol. 64, 2338–2345. doi: 10.1099/ijs.0.063560-0
Ramos, E., Ramírez-Bahena, M.-H., Valverde, A., Velázquez, E., Zúñiga, D., Velezmoro, C., et al. (2013). Pseudomonas punonensis sp. nov., isolated from straw. Int. J. Syst. Evol. Microbiol. 63, 1834–1839. doi: 10.1099/ijs.0.042119-0
Ravelo, A. G., and Braun, A. E. (2009). Relevancia de los productos naturales en el descubrimiento de nuevos fármacos en el s. XXI. Rev. Real Acad. Cienc. Exactas Físicas Nat. 103, 409–420.
Richter, M., and Rosselló-Móra, R. (2009). Shifting the genomic gold standard for the prokaryotic species definition. Proc. Natl. Acad. Sci. 106, 19126–19131. doi: 10.1073/pnas.0906412106
Rivas, R., García-Fraile, P., Mateos, P. F., Martínez-Molina, E., and Velázquez, E. (2007). Characterization of xylanolytic bacteria present in the bract phyllosphere of the date palm Phoenix dactylifera. Lett. Appl. Microbiol. 44, 181–187. doi: 10.1111/j.1472-765X.2006.02050.x
Rogers, J. S., Swofford, D. L., and Cannatella, D. (1998). A fast method for approximating maximum likelihoods of phylogenetic trees from nucleotide sequences. Syst. Biol. 47, 77–89. doi: 10.1080/106351598261049
Saito, N., Kitame, F., Kikuchi, M., and Ishida, N. (1974). Studies on a new antiviral antibiotic, 9-methylstreptimidone. I. Physicochemical and biological properties. J. Antibiot. 27, 206–214. doi: 10.7164/antibiotics.27.206
Saitou, N., and Nei, M. (1987). The neighbor-joining method: a new method for reconstructing phylogenetic trees. Mol. Biol. Evol. 4, 406–425. doi: 10.1093/oxfordjournals.molbev.a040454
Sarniguet, A., Kraus, J., Henkels, M. D., Muehlchen, A. M., and Loper, J. E. (1995). The sigma factor sigma s affects antibiotic production and biological control activity of Pseudomonas fluorescens Pf-5. Proc. Natl. Acad. Sci. 92, 12255–12259. doi: 10.1073/pnas.92.26.12255
Sasser, M. (1990). Identification of Bacteria by Gas Chromatography of Cellular Fatty Acids, MIDI Technical Note 101. Newark, DE: MIDI Inc.
Schnider, U., Keel, C., Blumer, C., Troxler, J., Défago, G., and Haas, D. (1995). Amplification of the housekeeping sigma factor in Pseudomonas fluorescens CHA0 enhances antibiotic production and improves biocontrol abilities. J. Bacteriol. 177, 5387–5392. doi: 10.1128/jb.177.18.5387-5392.1995
Sedkova, N., Tao, L., Rouvière, P. E., and Cheng, Q. (2005). Diversity of carotenoid synthesis gene clusters from environmental enterobacteriaceae strains. Appl. Environ. Microbiol. 71, 8141–8146. doi: 10.1128/AEM.71.12.8141-8146.2005
Six, D. L. (2012). Ecological and evolutionary determinants of bark beetle —fungus symbioses. Insects 3, 339–366. doi: 10.3390/insects3010339
Stintzi, A., Cornelis, P., Hohnadel, D., Meyer, J.-M., Dean, C., Poole, K., et al. (1996). Novel pyoverdine biosynthesis gene(s) of Pseudomonas aeruginosa PAO. Microbiology 142, 1181–1190. doi: 10.1099/13500872-142-5-1181
Therrien, J., Mason, C. J., Cale, J. A., Adams, A., Aukema, B. H., Currie, C. R., et al. (2015). Bacteria influence mountain pine beetle brood development through interactions with symbiotic and antagonistic fungi: implications for climate-driven host range expansion. Oecologia 179, 467–485. doi: 10.1007/s00442-015-3356-9
Thompson, J. D., Higgins, D. G., and Gibson, T. J. (1994). CLUSTAL W: improving the sensitivity of progressive multiple sequence alignment through sequence weighting, position-specific gap penalties and weight matrix choice. Nucleic Acids Res. 22, 4673–4680. doi: 10.1093/nar/22.22.4673
Tindall, B. J. (1990a). A comparative study of the lipid composition of halobacterium saccharovorum from various sources. Syst. Appl. Microbiol. 13, 128–130. doi: 10.1016/S0723-2020(11)80158-X
Tindall, B. J. (1990b). Lipid composition of Halobacterium lacusprofundi. FEMS Microbiol. Lett. 66, 199–202. doi: 10.1111/j.1574-6968.1990.tb03996.x
Tisdale, M. J. (1980). The effect of the methionine antagonist l-2-amino-4-methoxy-trans-3-butenoic acid on the growth and metabolism of walker carcinosarcoma in vitro. Biochem. Pharmacol. 29, 501–508. doi: 10.1016/0006-2952(80)90369-X
Toro, M., Ramírez-Bahena, M.-H., Cuesta, M. J., Velázquez, E., and Peix, A. (2013). Pseudomonas guariconensis sp. nov., isolated from rhizospheric soil. Int. J. Syst. Evol. Microbiol. 63, 4413–4420. doi: 10.1099/ijs.0.051193-0
Verani, A., Sironi, F., Siccardi, A. G., Lusso, P., and Vercelli, D. (2002). Inhibition of CXCR4-tropic HIV-1 infection by lipopolysaccharide: evidence of different mechanisms in macrophages and T Lymphocytes. J. Immunol. 168, 6388–6395. doi: 10.4049/jimmunol.168.12.6388
Vida, C., de Vicente, A., and Cazorla, F. M. (2017). Draft genome sequence of the rhizobacterium Pseudomonas chlororaphis PCL1601, displaying biocontrol against soilborne phytopathogens. Genome Announc. 5, e00130–e00117. doi: 10.1128/genomeA.00130-17
Weber, T., Blin, K., Duddela, S., Krug, D., Kim, H. U., Bruccoleri, R., et al. (2015). AntiSMASH 3.0—a comprehensive resource for the genome mining of biosynthetic gene clusters. Nucleic Acids Res. 43, W237–W243. doi: 10.1093/nar/gkv437
Wemheuer, F., Hollensteiner, J., Poehlein, A., Granzow, S., Daniel, R., Vidal, S., et al. (2017). Draft genome sequence of pseudomonas putida strain GM4FR, an endophytic bacterium isolated from Festuca rubra L. Genome Announc. 5, e00086–e00017. doi: 10.1128/genomeA.00086-17
Xiao, Y.-P., Hui, W., Wang, Q., Roh, S. W., Shi, X.-Q., Shi, J.-H., et al. (2009). Pseudomonas caeni sp. nov., a denitrifying bacterium isolated from the sludge of an anaerobic ammonium-oxidizing bioreactor. Int. J. Syst. Evol. Microbiol. 59, 2594–2598. doi: 10.1099/ijs.0.005108-0
Xu, L., Lou, Q., Cheng, C., Lu, M., and Sun, J. (2015). Gut-associated bacteria of dendroctonus valens and their involvement in verbenone production. Microb. Ecol. 70, 1012–1023. doi: 10.1007/s00248-015-0625-4
Xu, L.-T., Lu, M., and Sun, J.-H. (2016). Invasive bark beetle-associated microbes degrade a host defensive monoterpene. Insect Sci. 23, 183–190. doi: 10.1111/1744-7917.12255
Yamaguchi, M., Park, H. J., Ishizuka, S., Omata, K., and Hirama, M. (1995). Chemistry and antimicrobial activity of caryoynencins analogs. J. Med. Chem. 38, 5015–5022. doi: 10.1021/jm00026a008
Zerbino, D. R., and Birney, E. (2008). Velvet: algorithms for de novo short read assembly using de Bruijn graphs. Genome Res. 18, 821–829. doi: 10.1101/gr.074492.107
Keywords: antimicrobials, anticarcinogenic, antiviral, genome mining, bark beetles, antibiotic resistance, NRPS-PKS, secondary metabolites
Citation: Saati-Santamaría Z, López-Mondéjar R, Jiménez-Gómez A, Díez-Méndez A, Větrovský T, Igual JM, Velázquez E, Kolarik M, Rivas R and García-Fraile P (2018) Discovery of Phloeophagus Beetles as a Source of Pseudomonas Strains That Produce Potentially New Bioactive Substances and Description of Pseudomonas bohemica sp. nov. Front. Microbiol. 9:913. doi: 10.3389/fmicb.2018.00913
Received: 15 December 2017; Accepted: 20 April 2018;
Published: 08 May 2018.
Edited by:
Jesus L. Romalde, Universidade de Santiago de Compostela, SpainReviewed by:
Learn-Han Lee, Monash University Malaysia, MalaysiaMari Carmen Macián, Universitat de València, Spain
Copyright © 2018 Saati-Santamaría, López-Mondéjar, Jiménez-Gómez, Díez-Méndez, Větrovský, Igual, Velázquez, Kolarik, Rivas and García-Fraile. This is an open-access article distributed under the terms of the Creative Commons Attribution License (CC BY). The use, distribution or reproduction in other forums is permitted, provided the original author(s) and the copyright owner are credited and that the original publication in this journal is cited, in accordance with accepted academic practice. No use, distribution or reproduction is permitted which does not comply with these terms.
*Correspondence: Paula García-Fraile, Z2FyY2lhQGJpb21lZC5jYXMuY3o=; cGF1bGFnZjgxQHVzYWwuZXM=