- Department of Biology, Washington University in St. Louis, St. Louis, MO, United States
Interactions between species and their environment play a key role in the evolution of diverse communities, and numerous studies have emphasized that interactions among microbes and among trophic levels play an important role in maintaining microbial diversity and ecosystem functioning. In this study, we investigate how two of these types of interactions, public goods cooperation through the production of iron scavenging siderophores and predation by the social amoeba Dictyostelium discoideum, mediate competition between two strains of Pseudomonas fluorescens that were co-isolated from D. discoideum. We find that although we are able to generally predict the competitive outcomes between strains based on the presence and absence of either D. discoideum or iron, predator-by-environment interactions result in unexpected competitive outcomes. This suggests that while both cooperation and predation can mediate the competitive abilities and potentially the coexistence of these strains, predicting how combinations of different environments affect even the relatively simple microbiome of D. discoideum remains challenging.
Introduction
Interactions within and between microbial species play an important role in mediating competition and shaping their evolutionary histories. These types of interactions range from producing antimicrobial compounds that kill potential competitors to cooperatively scavenging poorly accessible nutrients such as iron from the environment and have been implicated in a number of important evolutionary processes such as generating and maintaining diverse microbial communities (Kerr et al., 2002; Kirkup and Riley, 2004; Cordero et al., 2012a,b; Biernaskie et al., 2013; Coyte et al., 2015; Inglis et al., 2016; Leinweber et al., 2017).
One key mechanism that has been shown to influence microbial interactions and competition between species is predation (Meyer and Kassen, 2007; Jousset et al., 2009; Ishii and Shimada, 2012; Friman et al., 2013). Predation is able to increase microbial diversity through adaptive radiations caused by frequency dependent selection where predators evolve to be best at hunting and eating the most common type (Meyer and Kassen, 2007). Conversely, predation can also be important in preventing the spread of new genetic mutants such as microbial cheaters, if the cheaters that no longer produce anti-predator toxins are preferentially eaten (Jousset et al., 2009; Friman et al., 2013). This suggests that predation can have a variety of effects on both interacting microbes and complex microbial communities. Interactions between trophic levels may therefore play a key role in determining the species composition of diverse populations of interacting microbes.
Complex trophic interactions have previously been illustrated in the social amoeba Dictyostelium discoideum, which eats a variety of soil bacteria and forms symbiotic relationships with a number of different bacterial species (Brock et al., 2011; DiSalvo et al., 2015). D. discoideum is found on the forest floor, where it inhabits the soil and leaf litter. D. discoideum’s primary food source is bacteria which it predates on during its vegetative growth stage as single celled amoebae. During this feeding stage D. discoideum comes into contact with a number of different soil associated bacteria and has been shown to form symbiotic relationships with a number of different Burkholderia species and Pseudomonas fluorescens (Brock et al., 2013; Stallforth et al., 2013; DiSalvo et al., 2015). In these symbioses, the amoebas disperse the bacteria to new locations, while the bacteria provide food or other services to the amoebas (Brock et al., 2011, 2013, 2016a,b; Stallforth et al., 2013; DiSalvo et al., 2015). These symbioses are thought to be mostly driven by the presence of certain Burkholderia (DiSalvo et al., 2015), but these Burkholderia allow D. discoideum to also carry other bacterial species such as P. fluorescens that can act as important sources of food and produce beneficial compounds for D. discoideum (Stallforth et al., 2013). For example, P. fluorescens strains PfA-QS161 (Pf2) and PfB-QS161 (Pf3) have both been cultured from the same clonal isolate of D. discoideum (Stallforth et al., 2013; Table 1). Pf3 acts as a food source and Pf2, though inedible, produces small molecules beneficial to the amoebae (Stallforth et al., 2013). Interestingly, previous work suggests Pf3 differs in its edibility and chemical profile due to a single mutation in the GacS/GacA two-component regulatory system and phylogenetic analysis shows that it is likely to be derived from Pf2 (Stallforth et al., 2013; Table 1). However, these two strains differ by at least 135 other SNPs (Stallforth et al., 2013), suggesting that this association has continued for some time, even though both strains can also grow independently.
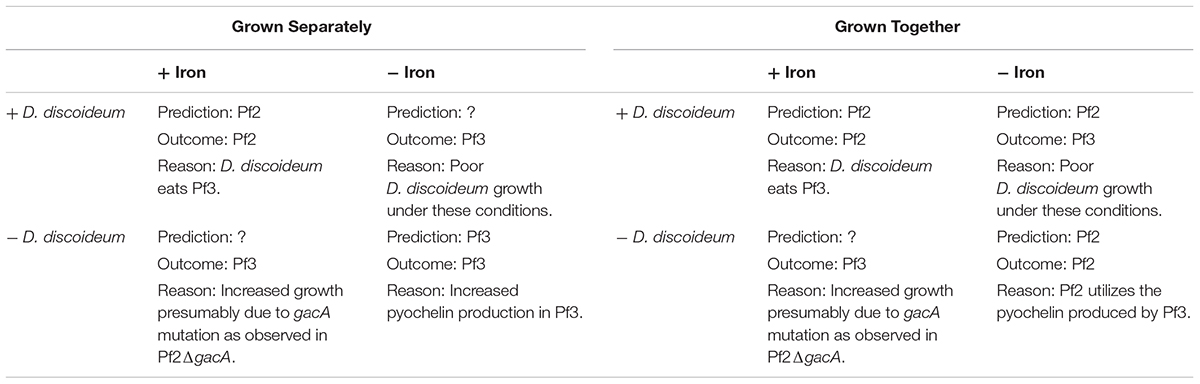
TABLE 1. An outline of the experimental conditions (i.e., with our without iron, with or without D. discoideum, and with or without direct interactions between strains), the predicted strain that should win the competition, the actual outcome, and a brief explanation of the results.
GacS/GacA mutants are fairly common in the environment (Jousset et al., 2009; Driscoll et al., 2011) and have a disrupted environmental sensing system, which can lead gene expression changes for up to 10% of the genome (Hassan et al., 2010). The loss of GacS/GacA function often involves regulatory changes to extra-cellular products and loss of secondary metabolism (van den Broek et al., 2005). This is accompanied by an increase in growth compared the wild-type strain, which may allow better colonization of new environmental niches (van den Broek et al., 2005). In Pf3 this loss of function in GacS/GacA system results in the increased expression of pyochelin, an iron-chelating siderophore that acts as a cooperative public good (Dumas et al., 2013; Stallforth et al., 2013). From an evolutionary perspective this is interesting, because selection appears to have favored a macro-mutation that affects a great many traits. However, from an ecological standpoint some of these changes may provide the niche differences that may facilitate coexistence between these strains.
We therefore sought to test whether environmental conditions pertinent to the difference in GacS/GacA expression, such as presence or absence of iron and predation by D. discoideum, can explain the competitive abilities of these two strains. Taking these environmental factors singly, we can make the following predictions (see also Table 1):
1a. Presence of D. discoideum should favor Pf2, because Pf3 is edible (Stallforth et al., 2013).
1b. Absence of D. discoideum should favor Pf3, assuming predator defense is costly (Steiner, 2007; Jousset et al., 2010).
2a. Iron-rich medium should favor Pf2, assuming the production of siderophores by Pf3 is costly (Ross-Gillespie et al., 2007; Kummerli and Brown, 2010).
2b. Iron-poor medium should favor siderophore-producing Pf3 when the two clones are grown separately, but when they are grown mixed together, Pf2 should do better by gaining the benefit of Pf3’s siderophores without paying the cost (Griffin et al., 2004).
We tested these predictions in a fully factorial design varying (1) presence or absence of D. discoideum (2) iron-rich or iron-poor media and (3) whether the P. fluorescens strains are grown separately or mixed together. Figure 1 shows the design. Where both factors predict that one the two clones should do better (e.g., D. discoideum + and Iron + both favor Pf2) then we predict that clone will outcompete the other (shown in Figure 1 as red for Pf2 or blue for Pf3). When one factor favors one clone and the other factor favors the other clone (such as D. discoideum - and Iron +), we make no prediction (shown as purple) but the outcomes might show interesting interactions. A final prediction concerns the genetic basis of these competitive abilities. If the GacS/GacA regulatory system is responsible then a mutant of Pf2 with a defective in GacS/GacA would act similarly to Pf3 in all environments.
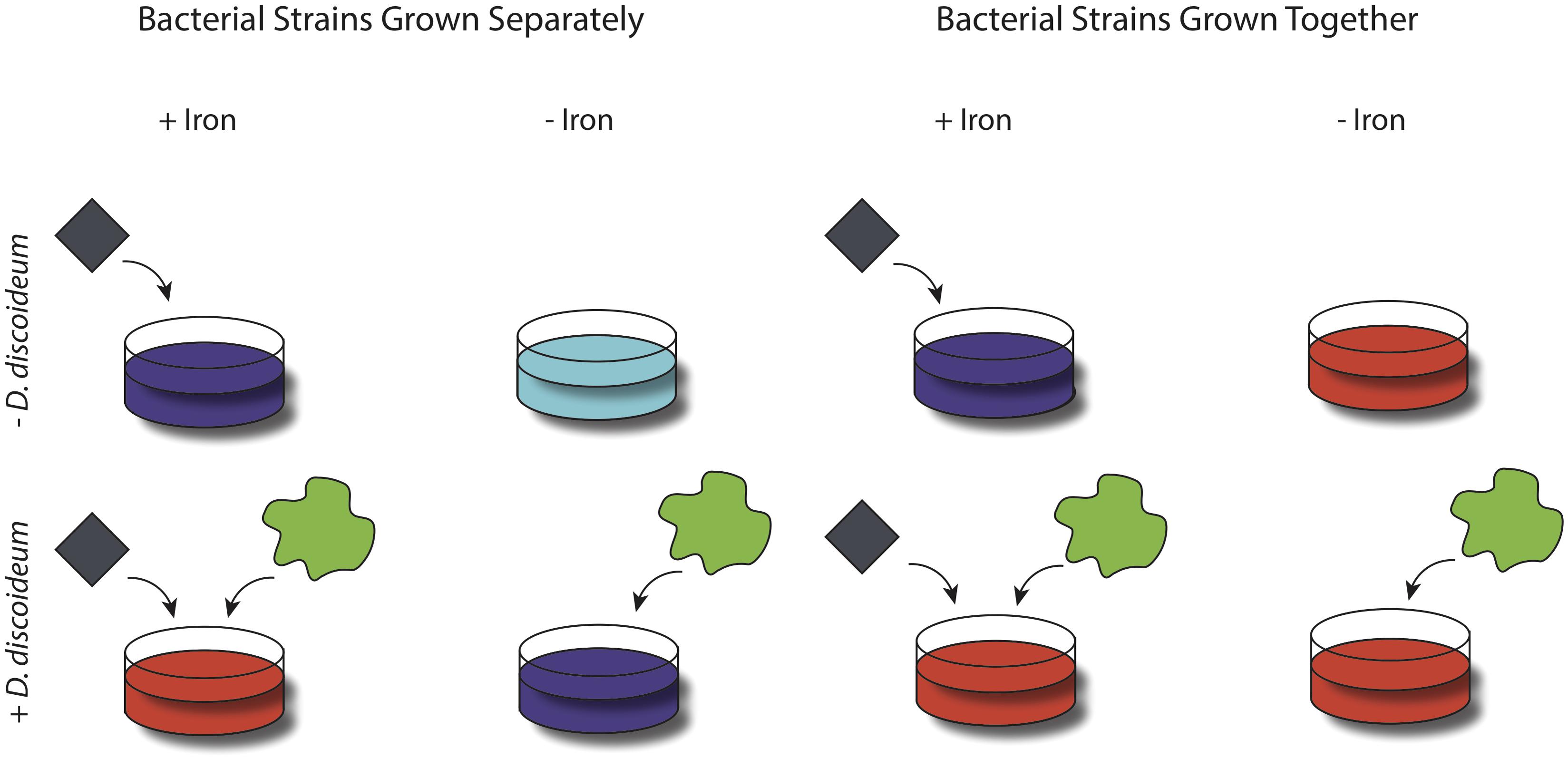
FIGURE 1. A cartoon schematic of the experimental design. Bacterial strains were grown either separately or together in iron-rich and iron limited media (as represented by the gray diamond), in both the presence and absence of D. discoideum (as represented by the green amoeba). Colors of the petri dish refer to which strain we predict to win the competition between strains based on their competitive abilities as outlined in Table 1. If the petri dishes are colored red we predict Pf2 to win and if colored blue we predict Pf3 to win. When there is no clear prediction petri dishes are colored purple.
Materials and Methods
Strains and Culture Conditions
In order to investigate how predation affects bacterial social interactions we used a naturally isolated D. discoideum QS161 which we isolated from forest soil at Mountain Lake Biological Station and two strains of the bacterium P. fluorescens Pf2 (previously referred to as PfA) and Pf3 (previously referred to as PfB) isolated from QS161, as described in Stallforth et al. (2013) (Table 2). The chemical profiles and edibility of Pf2 and Pf3 can presumably be attributed a single stop-codon in the GacS/GacA regulatory system of Pf3, which causes the loss of a highly conserved DNA binding domain of the gacA gene. We therefore also made use of a previously constructed Pf2 strain with a deletion of the gacA gene (Pf2ΔgacA) (Stallforth et al., 2013) to test whether competitive differences between Pf2 and Pf3 could be explained by this mutation alone. In order to distinguish between these strains during competition assays, we tagged both Pf3 and Pf2 ΔgacA with a chromosomal insertion of YFP (attTn7::e-yfp) which allowed us to distinguish these strains when competed against an untagged Pf2, and used these strains for all subsequent experiments (Choi and Schweizer, 2006). We cured QS161 cured of associated bacterial symbionts by culturing with tetracycline (DiSalvo et al., 2015).
We revived D. discoideum QS161 from frozen glycerol stocks by re-suspending spores in 100ul KK2 [2.25 g KH2PO4 (Sigma-Aldrich) and 0.67 g K2HPO4 (Fisher Scientific) per liter] and plating on SM/5 agar plates [2 g glucose (Fisher Scientific), 2 g Bacto Peptone (Oxoid), 2 g yeast extract (Oxoid), 0.2 g MgCl2 (Fisher Scientific), 1.9 g KHPO4 (Sigma-Aldrich), 1 g K2HPO5 (Fisher Scientific), and 15 g agar (Fisher Scientific) per liter] with 100 ul of Klebsiella pneumoniae, diluted to an OD600nm of 1.5, and incubating at room temperature. All three strains of P. fluorescens (Pf2, Pf3, and Pf2 ΔgacA) were grown from frozen glycerol stocks in LB broth (Fisher Scientific) shaking at 220 rpm at room temperature.
Measuring Iron-Chelating Ability
Previous work has identified that P. fluorescens strains Pf2 and Pf3 differ in their production of the iron-chelating siderophore, pyochelin. We therefore, first tested whether the production of pyochelin in Pf3 results in an increased ability to chelate iron from its environment (Supplementary Figure S1). We measured each strain’s relative ability to chelate iron by growing six replicate populations (Pf2, Pf3, and Pf2 ΔgacA) in iron limited CAA media [5 g casamino acids, 1.18 g K2HPO4 ∗ 3H2O (Sigma-Aldrich), 0.25 g MgSO4 ∗ 7H2O (Sigma-Aldrich) per liter supplemented with 20 mM NaHCO3 (Sigma-Aldrich) and 100 μg/ml human apo-transferrin (Sigma-Aldrich)] shaking at room temperature for 24 h. We then performed a modified chrome azurol S (CAS) assay in order to measure the overall iron-chelating ability of each strain (Schwyn and Neilands, 1987; Inglis et al., 2016). We added 100 μl of CAS solution to 100 μl of bacterial culture. We incubated this mixture at room temperature, in darkness for 30 min, and we measured the color change associated with the removal of iron (through the activity of siderophores) from the CAS solution using absorbance at 630 nm (Infinite 200 PRO, Tecan, Switzerland). We also measured bacterial density using OD at 600 nm in the samples before the CAS solution had been added, and we calculated iron-chelating activity as [1 - (Ai/Aref)]/(Densityi), where A = absorbance at 630 nm of the assay mixture (Supplementary Figure S1).
Competition Experiments
We performed competition experiments between our three bacterial strains (Pf2, Pf3, and Pf2 ΔgacA) (Figure 1). These strains were grown both separately and mixed together in direct competition, in both presence and absence of D. discoideum, and in two different environments: relatively iron rich and iron poor. In order to perform these competitions we harvested D. discoideum amoebae after 40 h of growth and re-suspended them in KK2 with a density of 107 amoebas per milliliter. We grew overnight cultures of Pf2, Pf3, and Pf2ΔgacA and diluted each to an OD600nm of 1. When strains were grown in the presence of D. discoideum, we plated 100 ul of the amoeba suspension with 100 ul of either a single strain or 50 ul of both competing strains (making a total of 100 ul). When strains were grown in the absence of D. discoideum, we substituted the addition of amoebae with 100 ul KK2. We plated these various combinations of P. fluorescens and D. discoideum on CAA agar supplemented with either 100 uM FeCl3 (for relatively iron rich environments) or with 20 mM NaHCO3 (sodium bicarbonate) and 100 μg/ml human apo-transferrin (for relatively iron poor environments), with six replicates for each unique combination. These combinations include the presence and of D. discoideum, in iron rich and iron limited media, for all three strains growing separately and pairwise competitions between Pf2 vs. Pf3 and Pf2 vs. Pf2ΔgacA. In order to correct for effects that iron rich and iron poor media might have on D. discoideum growth, we grew amoebae in both conditions supplementing both with our standard laboratory food bacterium K. pneumoniae, finding no difference in growth between iron rich and iron poor media (Supplementary Figure S2).
We incubated the plates at room temperature for 96 h. We subsequently harvested both bacteria and amoebae by flooding individual plates with 5 ml of KK2 and then re-suspended each mixture in 15 ml falcon tube. We then performed a low gravity spin (3 min at 310 rcf) to separate the bacteria from the amoebae. We counted both amoebae and bacteria using flow cytometry (BD Accuri C6). We stained bacterial samples with SYTO-62 (Thermo Fisher) in order to obtain a total count of the bacterial population and the numbers of Pf3 and Pf2 ΔgacA could easily be differentiated because they express YFP. Additionally we added cell-counting beads (Thermo Fisher) to each sample to standardize counts between samples.
Flow Cytometry and Statistical Analyses
Flow cytometry data were analyzed using Bioconductor (Gentleman et al., 2004) as previously described (Smith et al., 2016). We used a linear model to analyze our bacterial density data. We used the presence and absence of iron, the presence and absence of D. discoideum, strain identity, and whether strains were grown/competed together or separately as factors (fixed effects) to explain bacterial density (bacterial density ∼ iron∗strain∗D. discoideum∗competition). T-tests were used to compare between strains in the different conditions and listed in Table 3. All statistics were performed in R version 3.2.3 (R Core Team, 2015).
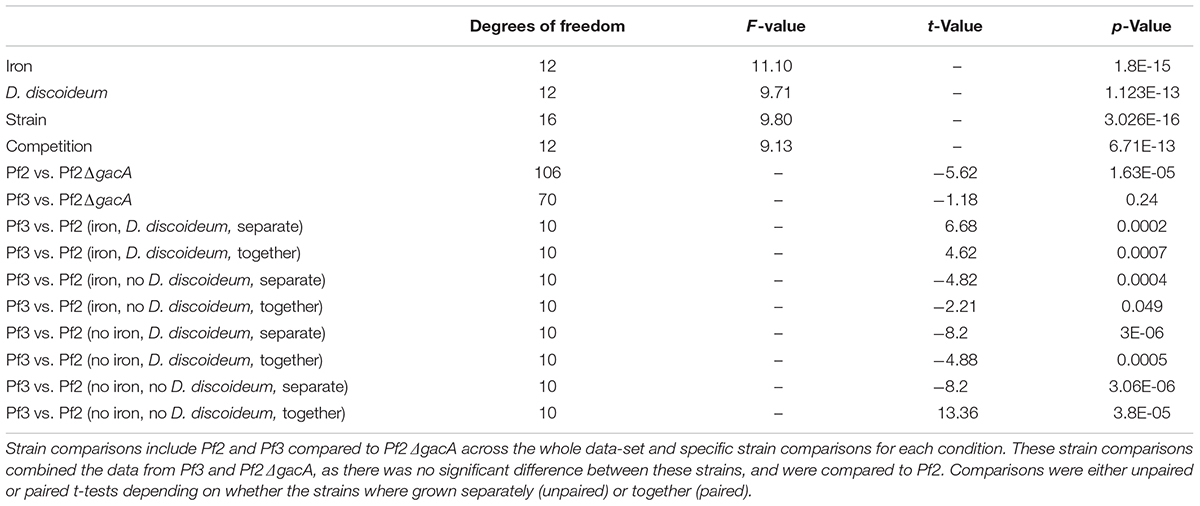
TABLE 3. Summary statistics for the main model effects (iron, D. discoideum, Strain, and Competition) and relevant strain comparisons.
Results
Our results reveal a context-dependent pattern of competitive fitness between Pf2 and Pf3 that depend on two environmental conditions: the presence of iron and D. discoideum that support four out of our five initial predictions (summarized in Table 1). As predicted, we find that the Pf2ΔgacA mutant and Pf3 behave similarly across all our experimental treatments and reach similar bacterial densities (t = -1.18, p > 0.24) (Figure 2 and Table 3). This suggests that although Pf2 and Pf3 differ by over 100 SNPs, their growth and competitive abilities under these experimental conditions can in large part be explained by a non-functional GacS/GacA regulatory system (Stallforth et al., 2013). This is perhaps somewhat unsurprising as the GacS/GacA regulatory system is responsible for regulating up to 10% of the P. fluorescens genome (Hassan et al., 2010), but represents an interesting example of a single mutation conferring large competitive fitness effects that vary considerably over different environments. However, it is also important to note that changes to the GacS/GacA system may affect more than just pyochelin production and edibility, so some of the competitive fitness differences we observe may be due to other functions of GacS/GacA.
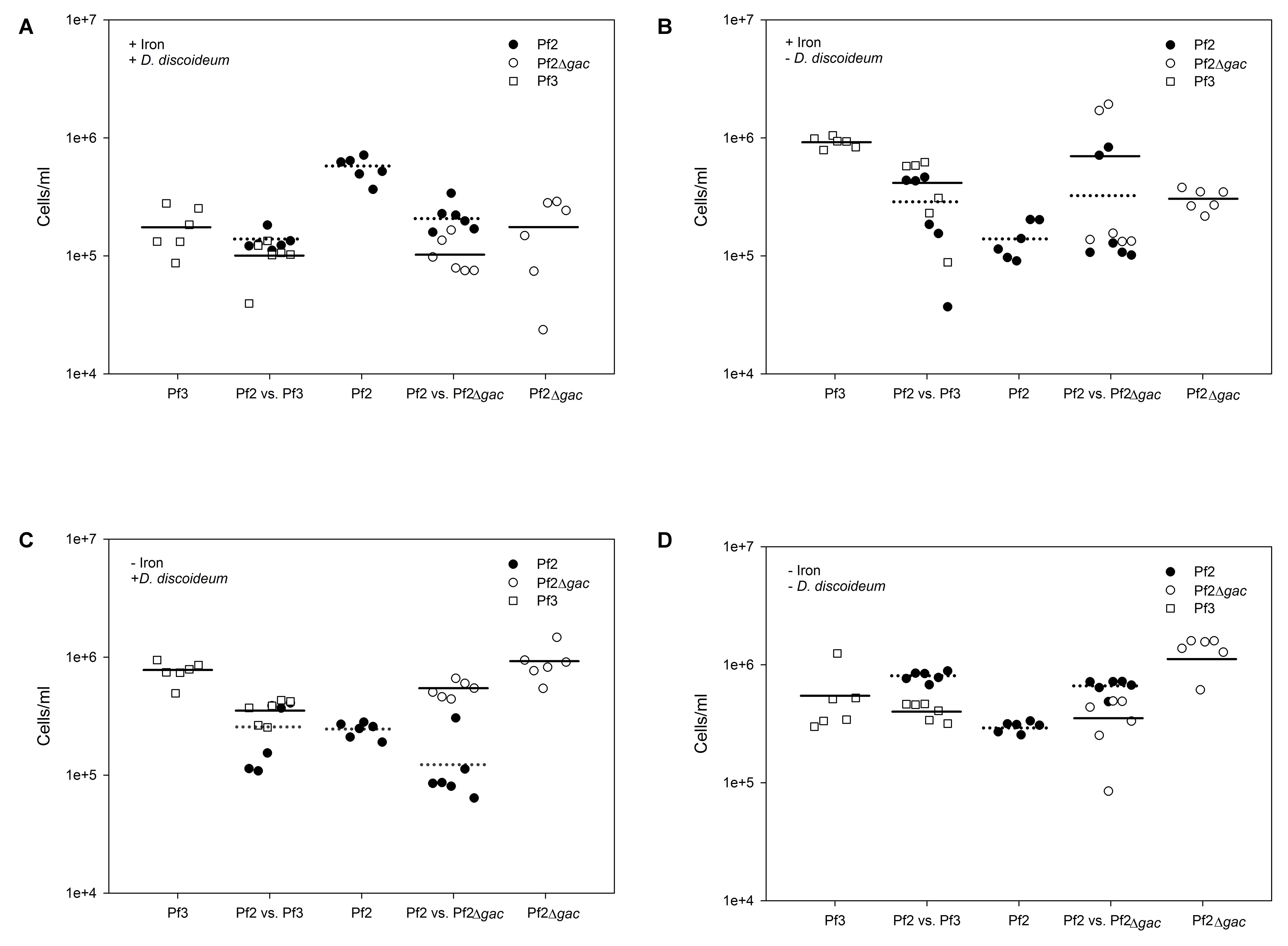
FIGURE 2. Bacterial densities across all competitions in the presence and absence of both iron and D. discoideum. Bacterial densities in the strains grown separately (i.e., single strains) were divided by 2 to give a per capita measure of cells produced. Horizontal lines represent the mean bacterial density of the strain. Dotted lines represent the mean of Pf2, whereas solid lines represent the means of Pf3 or Pf2ΔgacA. (A) In the presence of D. discoideum and iron-rich media, Pf2 outcompetes Pf3 and Pf2ΔgacA both when it is grown separately and together. (B) However, in the absence of D. discoideum and in the presence of iron, Pf3 and Pf2ΔgacA outcompete Pf2. (C) In iron-limited environments when D. discoideum is present, this pattern is surprisingly maintained. (D) However, when D. discoideum is absent in iron-limited environment, Pf2 grows poorly on its own, and it is able to outcompete Pf3 and Pf2ΔgacA when grown together. This suggests it is acting like a social cheater and exploiting the pyochelin produced by Pf3 and Pf2ΔgacA.
We also find that when the strains are grown separately, we see a clear correspondence to our initial predictions. Pf2 grows better in iron-rich media in the presence of D. discoideum compared to Pf3 (t = 6.68, p < 0.002) (Figures 2A, 3A and Table 3), probably because Pf3 is eaten by D. discoideum under these conditions and Pf2 presumably does not pay the cost of producing siderophores that are not needed (Ross-Gillespie et al., 2007; Kummerli and Brown, 2010). This contrasts to environmental conditions where iron is limiting and no D. discoideum is present as now Pf3 reaches higher densities compared to Pf2 (t = -8.2, p < 0.001) (Figure 2D and Table 3), presumably as Pf3 is able to obtain more iron from the environment through the overexpression of siderophores and because it also probably avoids Pf2’s costs of predator defense (Steiner, 2007; Jousset et al., 2010).
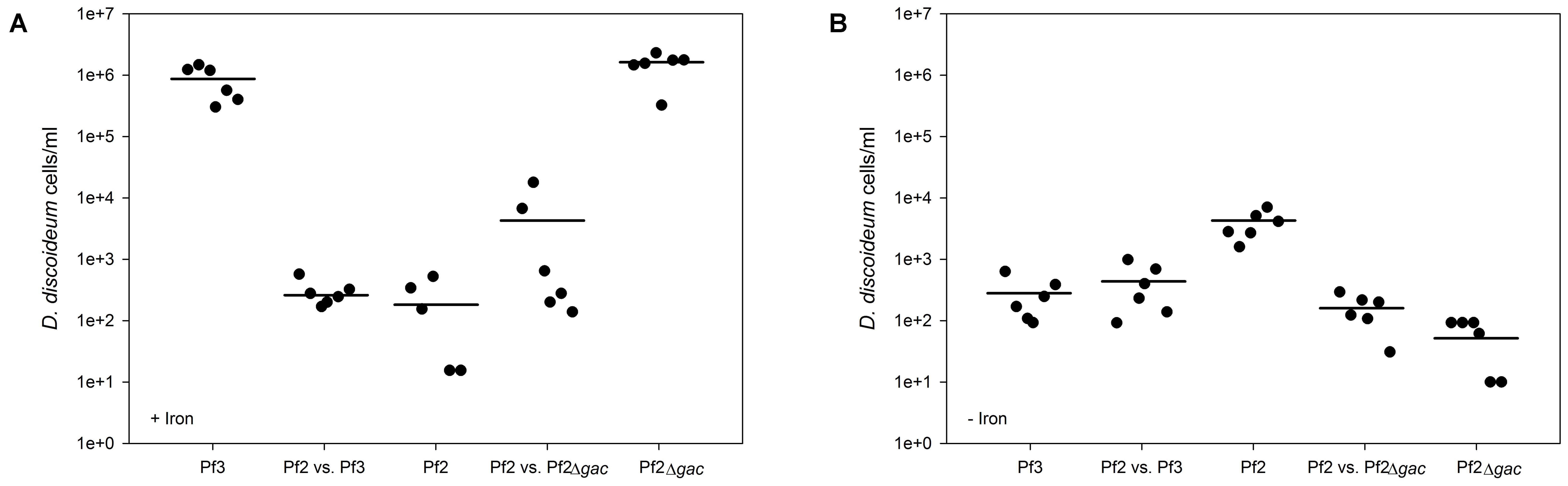
FIGURE 3. D. discoideum densities in iron-limited and iron-rich environments after 96 h. (A) Amoebae reach the highest densities in iron-rich environments, when grown in the presence of Pf3 and Pf2ΔgacA. (B) When grown in iron-limited environments D. discoideum has overall lower densities but has the highest density in the presence of Pf2.
In the cases where each strain is expected to be favored by one of the two environmental variables, somewhat surprisingly we found that Pf3 outcompeted Pf2 in both these sets of conditions when grown separately (iron-rich with no D. discoideum (t = -4.82, p < 0.001) and iron-limited with D. discoideum (t = -8.2, p < 0.001) (Figures 2B,C and Table 3). Pf2ΔgacA’s ability to reach higher final densities in the iron-rich conditions where no D. discoideum is present shows that the GacS/GacA mutation confers a simple growth yield advantage under these conditions. In iron-limited environments with D. discoideum Pf3 should suffer from predation by the amoebae, but we again find that Pf3 reaches higher final densities which can likely be explained by a combination of the growth enhancing effects of over expressing siderophores and amoebae’s poor growth in the presence of Pf3 under these conditions (Figure 3B and Table 1), which reduces their predatory ability. This reduction in growth might be in part due to expression changes in Pf3 under these conditions, which affect amoebae growth.
When Pf2 and Pf2ΔgacA are grown together in iron-rich media without the presence of D. discoideum, conditions that can either favor both Pf2 or PfΔgacA, we see that Pf2ΔgacA outcompetes Pf2 and reaches higher final densities (t = -2.21, p < 0.049) (Figure 2B and Table 3). This is the same result as when the strains are grown separately, again illustrating that the gac mutation confers some general growth yield advantage in iron-rich conditions. This increase in final density observed in Pf3 and the Pf2 gacA mutant (Pf2ΔgacA) may help explain the relatively frequent occurrence of gac mutants in nature (Driscoll et al., 2011), which may simply be due to direct fitness benefits in some environments through drastic expression changes.
When Pf2 and Pf3 are grown together in iron-rich environments in the presence of D. discoideum Pf2 reaches final higher densities because the amoebae predate on Pf3, as seen when grown separately (t = 4.62, p < 0.001) (Figures 2A, 3A and Table 3). However, under iron-limited conditions where no D. discoideum is present, Pf2 is now able to outcompete Pf3 when grown together by using the siderophores produced by Pf3 (t = 13.36, p < 0.001), in this case displaying completely differing competitive abilities compared to when they are grown separately. Competition between Pf2 and Pf3 under these conditions is presumably dominated the social interactions of pyochelin use. Although Pf3 has an advantage when grown separately as it over-expresses the iron chelating pyochelin, which is advantageous during iron limitation (Figure 2D and Table 1), in direct competition, our results seem to indicate that Pf2 is able to utilize the excess pyochelin produced by Pf2, acting like a social cheater. This interaction is particularly interesting because previous studies have identified gac mutants that act as social cheaters (the opposite of our findings, although other studies have also shown gac mutants to be adaptive (Driscoll et al., 2011), benefitting from a variety of cooperative behaviors without paying any associated costs (Jousset et al., 2009).
Somewhat more puzzling are our results when Pf2 and Pf3 are grown together under iron limitation and in the presence of D. discoideum. Both these environmental variables should favor Pf2, as it should be able to utilize the over expressed siderophores produced by Pf3, which in turn should be eaten by D. discoideum. Instead we see the opposite results of our predictions, with Pf3 outcompeting Pf2 (t = 4.88, p < 0.001) (Table 3), suggesting that these environmental variables (i.e., the presence of D. discoideum and absence of iron) that are individually beneficial to Pf2, reduce each other’s effects in an predator-by-environment (G × E × E) interaction (Thompson, 1999; Wade, 2007). The biological rationale for this interaction is unclear. However, it is interesting to note that D. discoideum, which performs relatively poorly under iron limitation, grows better in the presence of Pf2 (Figure 3B). Previous work has identified that Pf2 produces chromene, a compound that actively increases D. discoideum growth (Stallforth et al., 2013), but it could be equally plausible that expression changes in Pf2 under iron limitation render it edible thereby increasing D. discoideum growth and reducing it’s competitive advantage compared to Pf3.
Discussion
Competition between these two different bacteria (Pf2 and Pf3/Pf2 ΔgacA) then, largely seems to rely on the environmental conditions they experience. Neither the presence of iron nor D. discoideum could alone predict the competitive success of these competing bacteria, and the interactions of these two environmental conditions created unexpected outcomes. When grown separately, Pf2 reached higher densities (than Pf3 and Pf2ΔgacA) only in one treatment condition (iron-rich media and in the presence of D. discoideum, Figure 2A). However, when Pf2 is in direct competition with Pf3, it outcompetes it in two of the four treatments (Figures 2A,D), suggesting that these strains may only be able to coexist in fluctuating environments.
It is also important to note that we have only tested the competitive abilities of Pf2 and Pf3 under relatively simple environmental conditions and more complex, natural environments may allow for stable coexistence (or at least less drastic differences in competitive fitness). However, these conditions (iron limitation and D. discoideum) are likely to be extremely important in both the ecology and evolution for both strains. Both strains were isolated together from D. discoideum, and only one of them (Pf3) is edible. Furthermore, during the characterization of these strains, one of the largest differences in the secreted chemical profiles was the drastically increased expression of pyochelin, important for iron acquisition in iron-limited environments (Stallforth et al., 2013).
We have also not considered other ecological factors such as negative frequency dependent selection and snowdrift dynamics, which may play an important role in determining the outcome of this competition (Ross-Gillespie et al., 2007; Gore et al., 2009). In the case of public goods production, it has previously been shown that siderophore cheaters perform better when rare in the population (Ross-Gillespie et al., 2007). However, negative frequency dependent selection through predation may be equally important for Pf3: It may suffer the effects of predation more when common (Allen, 1988). Similarly, density dependent effects on both D. discoideum and the P. fluorescens strains can also have important consequences for this competition. For example, siderophore cheaters perform better under high population densities (Ross-Gillespie et al., 2009). Taken together these frequency and density dependent interactions might result in interesting and potentially complex feedback loops, which allow both strains to stably coexist.
These fluctuations in bacterial fitness, which depend on D. discoideum and iron, may provide some insight into the conditions that led to the evolution Pf3 from the ancestral Pf2 strain (Stallforth et al., 2013). In particular, it is difficult to explain why a mutation that renders a Pf3 edible should persist. However, our results suggest that the fitness effects of Pf3’s edibility depend on environmental context, and mutations in gacA may provide other benefits such as an increased growth rate irrespective of environmental conditions. Moreover, iron is likely to be relatively important nutrient for both P. fluorescens strains, with its patchy distribution within soil and low bioavailability (Kraemer, 2004), favoring adaptions that increase iron acquisition such as the up-regulation of pyochelin production (Dumas et al., 2013). However, it is important to note that there may also be some benefit to edibility because D. discoideum is able to disperse bacteria to new habitats, providing bacteria with new sources of food (Brock et al., 2011; DiSalvo et al., 2015). This suggests that differences between Pf2 and Pf3 may in part be due to transition from a specialized and mutualistic association between Pf2 and D. discoideum to a free-living environmental generalist in Pf3.
More generally our results suggest that making predictions about the microbial composition and competitive ability, even in the extremely simple microbiome of D. discoideum, is challenging not just because of species interactions but also because of predator-by-environment (G × E × E) interactions (Thompson, 1999; Wade, 2007). Nevertheless, with the increasing interest in understanding how complex mammalian microbiomes respond to changing environments and medical interventions, using ecological and evolutionary theory to test how simple microbiomes respond may offer the best hope for beginning to understand these kinds of inter-species interactions.
Author Contributions
RI conceived the study, performed experiments, analyzed the data, and wrote the paper. OA performed experiments and wrote the paper. ER performed experiments and wrote the paper. DQ conceived the study, analyzed the data, and wrote the paper. JS conceived the study, analyzed the data, and wrote the paper.
Funding
This material is based upon work supported by the National Science Foundation under grant number NSF DEB 1146375.
Conflict of Interest Statement
The authors declare that the research was conducted in the absence of any commercial or financial relationships that could be construed as a potential conflict of interest.
Supplementary Material
The Supplementary Material for this article can be found online at: https://www.frontiersin.org/articles/10.3389/fmicb.2018.00781/full#supplementary-material
References
Allen, J. A. (1988). Frequency-dependent selection by predators. Philos. Trans. R. Soc. Lond. B Biol. Sci. 319, 485–503. doi: 10.1098/rstb.1988.0061
Biernaskie, J. M., Gardner, A., and West, S. A. (2013). Multicoloured greenbeards, bacteriocin diversity and the rock-paper-scissors game. J. Evol. Biol. 26, 2081–2094. doi: 10.1111/jeb.12222
Brock, D. A., Callison, W. E., Strassmann, J. E., and Queller, D. C. (2016a). Sentinel cells, symbiotic bacteria and toxin resistance in the social amoeba Dictyostelium discoideum. Proc. Biol. Sci. 283:20152727. doi: 10.1098/rspb.2015.2727
Brock, D. A., Jones, K., Queller, D. C., and Strassmann, J. E. (2016b). Which phenotypic traits of Dictyostelium discoideum farmers are conferred by their bacterial symbionts? Symbiosis 68, 39–48. doi: 10.1007/s13199-015-0352-0
Brock, D. A., Douglas, T. E., Queller, D. C., and Strassmann, J. E. (2011). Primitive agriculture in a social amoeba. Nature 469, 393–396. doi: 10.1038/nature09668
Brock, D. A., Read, S., Bozhchenko, A., Queller, D. C., and Strassmann, J. E. (2013). Social amoeba farmers carry defensive symbionts to protect and privatize their crops. Nat. Commun. 4:2385. doi: 10.1038/ncomms3385
Choi, K. H., and Schweizer, H. P. (2006). mini-Tn7 insertion in bacteria with single attTn7 sites: example Pseudomonas aeruginosa. Nat. Protoc. 1, 153–161. doi: 10.1038/nprot.2006.24
Cordero, O. X., Ventouras, L. A., DeLong, E. F., and Polz, M. F. (2012a). Public good dynamics drive evolution of iron acquisition strategies in natural bacterioplankton populations. Proc. Natl. Acad. Sci. U.S.A. 109, 20059–20064. doi: 10.1073/pnas.1213344109
Cordero, O. X., Wildschutte, H., Kirkup, B., Proehl, S., Ngo, L., Hussain, F., et al. (2012b). Ecological populations of bacteria act as socially cohesive units of antibiotic production and resistance. Science 337, 1228–1231. doi: 10.1126/science.1219385
Coyte, K. Z., Schluter, J., and Foster, K. R. (2015). The ecology of the microbiome: networks, competition, and stability. Science 350, 663–666. doi: 10.1126/science.aad2602
DiSalvo, S., Haselkorn, T. S., Bashir, U., Jimenez, D., Brock, D. A., Queller, D. C., et al. (2015). Burkholderia bacteria infectiously induce the proto-farming symbiosis of Dictyostelium amoebae and food bacteria. Proc. Natl. Acad. Sci. U.S.A. 112, E5029–E5037. doi: 10.1073/pnas.1511878112
Driscoll, W. W., Pepper, J. W., Pierson, L. S. III, and Pierson, E. A. (2011). Spontaneous Gac mutants of Pseudomonas biological control strains: cheaters or mutualists? Appl. Environ. Microbiol. 77, 7227–7235. doi: 10.1128/AEM.00679-11
Dumas, Z., Ross-Gillespie, A., and Kummerli, R. (2013). Switching between apparently redundant iron-uptake mechanisms benefits bacteria in changeable environments. Proc. Biol. Sci. 280:20131055. doi: 10.1098/rspb.2013.1055
Friman, V. P., Diggle, S. P., and Buckling, A. (2013). Protist predation can favour cooperation within bacterial species. Biol. Lett. 9:20130548. doi: 10.1098/rsbl.2013.0548
Gentleman, R. C., Carey, V. J., Bates, D. M., Bolstad, B., Dettling, M., Dudoit, S., et al. (2004). Bioconductor: open software development for computational biology and bioinformatics. Genome. Biol. 5:R80.
Gore, J., Youk, H., and van Oudenaarden, A. (2009). Snowdrift game dynamics and facultative cheating in yeast. Nature 459, 253–256. doi: 10.1038/nature07921
Griffin, A. S., West, S. A., and Buckling, A. (2004). Cooperation and competition in pathogenic bacteria. Nature 430, 1024–1027. doi: 10.1038/nature02744
Hassan, K. A., Johnson, A., Shaffer, B. T., Ren, Q., Kidarsa, T. A., Elbourne, L. D., et al. (2010). Inactivation of the GacA response regulator in Pseudomonas fluorescens Pf-5 has far-reaching transcriptomic consequences. Environ. Microbiol. 12, 899–915. doi: 10.1111/j.1462-2920.2009.02134.x
Inglis, R. F., Biernaskie, J. M., Gardner, A., and Kummerli, R. (2016). Presence of a loner strain maintains cooperation and diversity in well-mixed bacterial communities. Proc. Biol. Sci. 283:20152682. doi: 10.1098/rspb.2015.2682
Ishii, Y., and Shimada, M. (2012). Learning predator promotes coexistence of prey species in host-parasitoid systems. Proc. Natl. Acad. Sci. U.S.A. 109, 5116–5120. doi: 10.1073/pnas.1115133109
Jousset, A., Rochat, L., Pechy-Tarr, M., Keel, C., Scheu, S., and Bonkowski, M. (2009). Predators promote defence of rhizosphere bacterial populations by selective feeding on non-toxic cheaters. ISME J. 3, 666–674. doi: 10.1038/ismej.2009.26
Jousset, A., Rochat, L., Scheu, S., Bonkowski, M., and Keel, C. (2010). Predator-prey chemical warfare determines the expression of biocontrol genes by rhizosphere-associated Pseudomonas fluorescens. Appl. Environ. Microbiol. 76, 5263–5268. doi: 10.1128/AEM.02941-09
Kerr, B., Riley, M. A., Feldman, M. W., and Bohannan, B. J. M. (2002). Local dispersal promotes biodiversity in a real-life game of rock-paper-scissors. Nature 418, 171–174. doi: 10.1038/nature00823
Kirkup, B. C., and Riley, M. A. (2004). Antibiotic-mediated antagonism leads to a bacterial game of rock-paper-scissors in vivo. Nature 428, 412–414. doi: 10.1038/nature02429
Kraemer, S. M. (2004). Iron oxide dissolution and solubility in the presence of siderophores. Aquat. Sci. 66, 3–18. doi: 10.1007/s00027-003-0690-5
Kummerli, R., and Brown, S. P. (2010). Molecular and regulatory properties of a public good shape the evolution of cooperation. Proc. Natl. Acad. Sci. U.S.A. 107, 18921–18926. doi: 10.1073/pnas.1011154107
Leinweber, A., Fredrik Inglis, R., and Kummerli, R. (2017). Cheating fosters species co-existence in well-mixed bacterial communities. ISME J. 11, 1179–1188. doi: 10.1038/ismej.2016.195
Meyer, J. R., and Kassen, R. (2007). The effects of competition and predation on diversification in a model adaptive radiation. Nature 446, 432–435. doi: 10.1038/nature05599
R Core Team (2015). R: A Language and Environment for Statistical Computing. Vienna: R Foundation for Statistical Computing. Available at: https://www.r-project.org/
Ross-Gillespie, A., Gardner, A., Buckling, A., West, S. A., and Griffin, A. S. (2009). Density dependence and cooperation: theory and a test with bacteria. Evolution Int. J. Org. Evolution 63, 2315–2325. doi: 10.1111/j.1558-5646.2009.00723.x
Ross-Gillespie, A., Gardner, A., West, S. A., and Griffin, A. S. (2007). Frequency dependence and cooperation: theory and a test with bacteria. Am. Nat. 170, 331–342. doi: 10.1086/519860
Schwyn, B., and Neilands, J. B. (1987). Universal chemical assay for the detection and determination of siderophores. Anal. Biochem. 160, 47–56. doi: 10.1016/0003-2697(87)90612-9
Smith, J., Strassmann, J. E., and Queller, D. C. (2016). Fine-scale spatial ecology drives kin selection relatedness among cooperating amoebae. Evolution 70, 848–859. doi: 10.1111/evo.12895
Stallforth, P., Brock, D. A., Cantley, A. M., Tian, X., Queller, D. C., Strassmann, J. E., et al. (2013). A bacterial symbiont is converted from an inedible producer of beneficial molecules into food by a single mutation in the gacA gene. Proc. Natl. Acad. Sci. U.S.A. 110, 14528–14533. doi: 10.1073/pnas.1308199110
Steiner, U. K. (2007). Investment in defense and cost of predator-induced defense along a resource gradient. Oecologia 152, 201–210. doi: 10.1007/s00442-006-0645-3
Thompson, J. N. (1999). Specific hypotheses on the geographic mosaic of coevolution. Am. Nat. 153, S1–S14. doi: 10.1086/303208
van den Broek, D., Bloemberg, G. V., and Lugtenberg, B. (2005). The role of phenotypic variation in rhizosphere Pseudomonas bacteria. Environ. Microbiol. 7, 1686–1697. doi: 10.1111/j.1462-2920.2005.00912.x
Keywords: siderophores, Pseudomonas fluorescens, Gac regulatory system, cooperation, predation
Citation: Inglis RF, Asikhia O, Ryu E, Queller DC and Strassmann JE (2018) Predator-by-Environment Interactions Mediate Bacterial Competition in the Dictyostelium discoideum Microbiome. Front. Microbiol. 9:781. doi: 10.3389/fmicb.2018.00781
Received: 30 November 2017; Accepted: 06 April 2018;
Published: 24 April 2018.
Edited by:
Haiwei Luo, The Chinese University of Hong Kong, Hong KongReviewed by:
David Van Dyken, University of Miami, United StatesDavid Johnson, Swiss Federal Institute of Aquatic Science and Technology, Switzerland
Elizabeth A. Pierson, Texas A&M University, United States
Copyright © 2018 Inglis, Asikhia, Ryu, Queller and Strassmann. This is an openaccess article distributed under the terms of the Creative Commons Attribution License (CC BY). The use, distribution or reproduction in other forums is permitted, provided the original author(s) and the copyright owner are credited and that the original publication in this journal is cited, in accordance with accepted academic practice. No use, distribution or reproduction is permitted which does not comply with these terms.
*Correspondence: R. Fredrik Inglis, aW5nbGlzQHd1c3RsLmVkdQ==