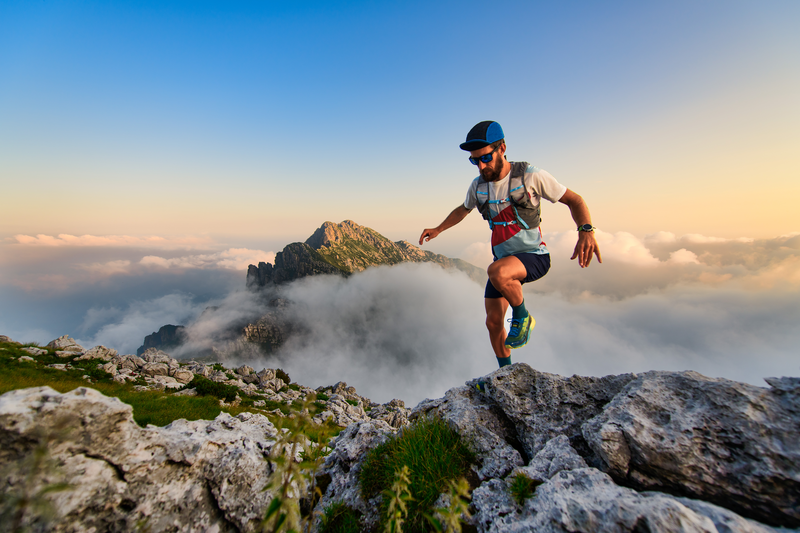
94% of researchers rate our articles as excellent or good
Learn more about the work of our research integrity team to safeguard the quality of each article we publish.
Find out more
MINI REVIEW article
Front. Microbiol. , 13 April 2018
Sec. Fungi and Their Interactions
Volume 9 - 2018 | https://doi.org/10.3389/fmicb.2018.00707
This article is part of the Research Topic Soil Fungal Biodiversity for Plant and Soil Health View all 12 articles
Soil health, and the closely related terms of soil quality and fertility, is considered as one of the most important characteristics of soil ecosystems. The integrated approach to soil health assumes that soil is a living system and soil health results from the interaction between different processes and properties, with a strong effect on the activity of soil microbiota. All soils can be described using physical, chemical, and biological properties, but adaptation to environmental changes, driven by the processes of natural selection, are unique to the latter one. This mini review focuses on fungal biodiversity and its role in the health of managed soils as well as on the current methods used in soil mycobiome identification and utilization next generation sequencing (NGS) approaches. The authors separately focus on agriculture and horticulture as well as grassland and forest ecosystems. Moreover, this mini review describes the effect of land-use on the biodiversity and succession of fungi. In conclusion, the authors recommend a shift from cataloging fungal species in different soil ecosystems toward a more global analysis based on functions and interactions between organisms.
Fungi are very successful inhabitants of soil, due to their high plasticity and their capacity to adopt various forms in response to adverse or unfavorable conditions (Sun et al., 2005). Due to their ability to produce a wide variety of extracellular enzymes, they are able to break down all kinds of organic matter, decomposing soil components and thereby regulating the balance of carbon and nutrients (Žifčáková et al., 2016). Fungi convert dead organic matter into biomass, carbon dioxide, and organic acids (Figure 1). Many species of fungi possess the ability to act as an effective biosorbent of toxic metals such as cadmium, copper, mercury, lead, and zinc, by accumulating them in their fruiting bodies. Though these elements may inhibit their growth and affect their reproduction (Baldrian, 2003). The diversity and activity of fungi is regulated by various biotic (plants and other organisms) and abiotic (soil pH, moisture, salinity, structure, and temperature) factors (López-Bucio et al., 2015; Rouphael et al., 2015). Fungi can be found in almost every environment and can live in wide range of pH and temperature (Frąc et al., 2015).
Soil fungi can be classified into three functional groups including: (1) biological controllers, (2) ecosystem regulators, and (3) species participating in organic matter decomposition and compound transformations (Swift, 2005; Gardi and Jeffery, 2009). Ecosystem regulators are responsible for soil structure formation and modification of habitats for other organisms by regulating the dynamics of physiological processes in the soil environment. Biological controllers can regulate diseases, pests, and the growth of other organisms (Bagyaraj and Ashwin, 2017). For example, the mycorrhizal fungi improve plant growth by increasing the uptake of nutrients and protect them against pathogens (Bagyaraj and Ashwin, 2017).
Fungal populations are strongly influenced by the diversity and composition of the plant community and in return affect plant growth through mutualism, pathogenicity and their effect on nutrient availability and cycling (Wardle, 2002; Wagg et al., 2014; Hannula et al., 2017). Moreover, fungi participate in nitrogen fixation, hormone production, biological control against root pathogens and protection against drought (Jayne and Quigley, 2014; Baum et al., 2015; El-Komy et al., 2015). They also play an important role in stabilization of soil organic matter and decomposition of residues (Treseder and Lennon, 2015).
The advent of next generation sequencing (NGS) has facilitated a sea-change in the analysis of soil and plant-associated fungal communities. Standardized pipelines for preparing rhizosphere soil samples for Illumina sequencing are widely available (Lindahl et al., 2013; Schöler et al., 2017) and in a relatively short time following sampling, files with millions of sequences can be generated. Important points to consider when preparing samples for NGS are: sufficient biological replication (Prosser, 2010), sufficient sequencing depth (Smith and Peay, 2014; Weiss et al., 2015), adequate coverage of target organisms (i.e., primer selection and DNA extraction), and the avoidance of contamination and bias (Salter et al., 2014; Schöler et al., 2017). There are multiple pipelines for the analysis of fungal NGS data available (Bálint et al., 2014; Gweon et al., 2015), so it is not data-analysis that is problematic, but the interpretation of results.
Alpha-diversity, representing either the number of species or diversity indices that account for evenness, was proposed as an indicator for robust, healthy soil (Ferris and Tuomisto, 2015). However, it is open to question whether absolute diversity or functional diversity should be emphasized (Wagg et al., 2014; Ferris and Tuomisto, 2015). Usually the second step in analysis is to look at beta-diversity to see the effects of treatment/manipulation on the fungal community. Recently, it has been shown that fungal biodiversity in soils is strongly affected by plant community (Yang et al., 2017), soil moisture (Bagyaraj and Ashwin, 2017), and the intensity of agricultural practices (Thomson et al., 2015). Studies identified key fungal species affected by soil treatments, but it is unknown if results obtained from studies conducted on one particular soil and ecosystem can be used to infer trends and identify key fungal groups at a global or continental scale (Tedersoo et al., 2014; Delgado-Baquerizo et al., 2017).
Unlike the analysis of bacteria and archaea, where 16S rRNA is used as a barcode, fungi are usually identified based on the sequence of the Internal Transcribed Spacer (ITS) region allowing identification up to species level (Schoch et al., 2012; Porras-Alfaro et al., 2014). Although in some cases, such as for soil-dwelling Fusaria, sequencing of additional genes, such as β-tubulin gene (β-Tub), and aminoadipate reductase gene (LYS2) was proposed to obtain the correct taxonomic identification at the species level (Watanabe et al., 2011), whereas others have suggested the use of Translation Elongation Factor (Geiser et al., 2004). Many researchers have in-house databases for functional classification of fungi but recently online resources for functional annotation of fungi have been made publicly available (Nguyen et al., 2016).
Species level identification is, however only the first step from ‘What is there?’ toward the question ‘What role does it play?’ (Figure 2). Identification neither implies the microorganisms are alive and active (Blagodatskaya and Kuzyakov, 2013) nor does it describe their function (Prosser, 2015). To unravel the function of the community, either (shotgun) metagenomics (Uroz et al., 2013; Hannula and van Veen, 2016; Castañeda and Barbosa, 2017), metatranscriptomics (Damon et al., 2012; Turner et al., 2013; Hesse et al., 2015) or time-intensive culture based methods combined with functional tests must be used (Behnke-Borowczyk et al., 2012; Gałązka et al., 2017; Wolińska et al., 2017). A fast, but coarse alternative to molecular methods is MicroResp (Creamer et al., 2016) or community level physiological profiles (CLPP) approach (Frąc et al., 2017), which gives an indication on substrate use of the total microbial community. This method, however, does not identify the species responsible for the process. Increasingly, especially in studies where plant community is included alongside NGS approaches, microorganisms are isolated from the soils and plant roots for further functional testing.
To construct a more complete picture of a soil fungi community their interactions with other organisms must be taken into consideration. Strong linkage was proved between functional soil biodiversity and the function of the soil ecosystem (Wagg et al., 2014; Delgado-Baquerizo et al., 2017; Morrien et al., 2017). Fungi interact with other soil organisms and thus changes in the fungal community have the potential to affect the function of the whole soil ecosystem (Yang et al., 2017). Analysis of the interactions in the soil can be achieved through indirect estimation of species interactions using co-occurrence networks (Creamer et al., 2016; Morrien et al., 2017) or directly by using isotope tracers (Hannula et al., 2017) and/or gut content analysis (Kurakov et al., 2016).
The term ‘soil health’ is widely used in reference to sustainable agriculture (Kibblewhite et al., 2008; Cardoso et al., 2013), especially in the context of soil as a dynamic, living organism functioning holistically rather than as an inert substrate (Doran and Jones, 1996). Therefore, in this article we prefer to use the terminology of soil health, rather than soil quality, which is defined as the capacity of the soil to maintain environmental quality, sustain biological productivity, and promote animal, human, and plant health (Doran and Parkin, 1994). In recent years the potential application of cultivating soil fungal biodiversity to improve soil quality and increase productivity of agricultural ecosystems has been highlighted as a new and very promising development in plant productivity (Bagyaraj and Ashwin, 2017), which may come be called ‘the 2nd Green Revolution.’ The implementation of such solutions may offer an alternative to the current overuse of fertilizers toward more sophisticated manipulations of plant productivity. Fungi participate in decomposition of organic matter and deliver nutrients for plant growth. Their role is very important in plant protection against pathogenic microorganisms as biological agents, which influences soil health (Frąc et al., 2015). The assessment of fungal biodiversity as quality indicators cannot be limited only to the determination of biodiversity indexes, but also should include a structure analysis of fungal population in order to determine the functions they play in affecting soil quality and plant health. The use of different kinds of organic manure has a strong influence on soil health, through indirect effects (i.e., via changes in physicochemical characteristics) and a direct effect on soil fungal communities. Soil management is fundamental to all agricultural systems, and the reduction of soil degradation is a priority to sustain future production. This effect can be only achieved by taking soil fungal biodiversity into account. All cultural practices, such as the use of cover and rotational crops, composts and tillage systems, besides their known effects on soil-borne pathogens (Abawi and Widmer, 2000), are likely to affect also the other groups of soil fungi, especially beneficial fungal populations. It has long been known that the suppressiveness of soils can be enhanced by adding biopolymers such as chitin and its derivatives (i.e., chitosan). This suppressiveness is related to a change in the activity and structure of soil microorganisms (Cretoiu et al., 2013). Therefore, we should utilize our knowledge on the interactions between different fungal groups and their ecology in the management of agricultural systems. It is worth mentioning that chitin addition to the soil increases bacteria and fungi that can degrade pathogenic fungal cell walls and can thus, increase the soil suppressiveness against plant pathogens. This might be a good alternative to fungicides that kill all fungi, including beneficial ones. Different tillage treatments can also impact soil fungi by soil disturbances that affect the functioning of fungal communities. Reduced tillage decreases the breakdown of hyphae causing fungal populations to remain more stable, retaining more nutrients and providing more suppressive effects against pathogenic microorganisms (Goss and deVarennes, 2002). Understanding and selecting the appropriate cultural practices, increasing fungal biodiversity, can prevent or decrease damage of root diseases and play a crucial role in the maintenance of soil quality and health. It should be taken into account that fungal diversity determines plant biodiversity, ecosystem variability, and productivity (van der Heijden et al., 1998; Wagg et al., 2014).
Arbuscular mycorrhizal fungi (AMF) are the most important class of beneficial microorganisms in agri- and horticultural soils (Smith and Read, 2008, Table 1). Significant increases in the yield of crop plants following inoculation with AMF have been observed in numerous experiments (Thilagar and Bagyaraj, 2015; Bagyaraj and Ashwin, 2017). The key effects of AMF symbiosis include: improvement of rooting and plant establishment, stimulation of nutrient cycling, improvement of soil structure, enhancement of plant tolerance to stresses, increased uptake of low mobility ions, and enhancement of plant community diversity (Azcón-Aguilar and Barea, 1997). The diseases of crop plants can be controlled by some antagonistic fungi such as Glomus sp. or Trichoderma sp. suppressing fungal pathogens (Dawidziuk et al., 2016). Species of Trichoderma (T. asperellum, T. atroviride, T. harzianum, T. virens, and T. viride) are frequently used in biocontrol and are known as biostimulants for horticultural crops (López-Bucio et al., 2015). Other positive effects of fungi on soil quality and plant health include inoculation by microbial consortia of AMF together with plant growth promoting rhizobacteria (PGPR) and others such as N-fixing and P-solubilizing microorganisms (Bagyaraj and Ashwin, 2017). A synergistic, favorable impact of AMFs and PGPRs on horticultural plant growth and soil microbial diversity and activity has been reported (Azcón-Aguilar and Barea, 1997; De Coninck et al., 2015).
Besides beneficial fungi, agri- and horticultural ecosystems contain also plant pathogens. The major groups of soil-borne root pathogenic fungi and oomycetes constitute of genera Fusarium (Michielse and Rep, 2009), Verticillium (Klosterman et al., 2009), Rhizoctonia (Gonzalez et al., 2011), Pythium, Phytophthora (van West et al., 2003) and many others, of global and local importance. The soil fungal diversity and methods of increasing it, particularly the populations of beneficial fungi within ecosystems should be used in practice for more sustainable plant production, decrease of chemical applications and protection of the soil environment.
Soil microorganisms, including fungi are an important component of grassland ecosystems due to their biochemical activity and engagement in nutrient cycling (Dengler et al., 2014). Grasslands provide many forms of ecosystem services including: supporting, provisioning, regulatory, and cultural services. Importantly, the role of biodiversity has been established as fundamental in ensuring the performance of ecosystem functioning. Grazing activities influence soil fungal community structure by changing edaphic conditions and the vegetation biodiversity in plant communities (Yang et al., 2017). It has been proven that moderate grazing sustains plants diversity while heavy grazing results in species loss (Joubert et al., 2017). Furthermore, plant-fungal interactions can inhibit biodiversity in grasslands due to the production of different root exudates such as enzymes, organic compounds, and polysaccharides (Huhe et al., 2017).
Plant pathogenic fungi also have a large impact on plant diversity in grasslands by limiting the abundance of their hosts, affecting biomass production. The study by Allan et al. (2010) suggests that fungal pathogens could affect nutrient cycling in grasslands reducing the abundance of dominant grasses and enhancing the growth of legumes. Soil fungal communities in grasslands can also be influenced by human activities and the components of long-term fertilization and other treatments (Cassman et al., 2016). Unlike in agricultural soils, where ascomycetes dominate, in grasslands, basidiomycetes are major decomposers of dead organic matter (Deacon et al., 2006).
Knowledge of the soil chemical and physical properties has always been of interest to foresters to evaluate the capacity of sites and to increase forest productivity (Schoenholtz et al., 2000). Forest soils (including humus, litter, and coarse woody debris) are an important reservoir of microorganisms and soil biota that in turn influence carbon storage, soil structure, fertility, productivity, and plant/tree growth.
Ectomycorrhizal associations are created by a specific group of plant families that includes the Pinaceae, Fabaceae, Betulaceae, and Fagaceae (Phosri et al., 2012). The results of research obtained by Högberg and Högberg (2002), indicate a significant contribution by ectomycorrhizal mycelium to forest soil microbial biomass and by ectomycorrhizal roots to the production of extractable dissolved organic carbon, which is a carbon source for other microbes.
During the processes of thinning, the transfer of nutrients from aboveground biomass to forest soil takes place (Tian et al., 2010). A higher concentration of nutrients comes from the green litter of thinned trees than litter returned to the forest floor after senescence (Girisha et al., 2003) or from the woody residue left on the ground after harvesting (Cookson et al., 2008). Consequently, the quality and quantity of organic substrates presented to the soil fungal community by thinned and non-thinned forests may vary to a great extent. The community of soil microorganisms depends highly on organic matter as it provides a suitable environment and energy sources for them that are critical to maintain the nutritional quality and water-retaining capacity of forest soils (Jiménez-Morillo et al., 2016). Soil organic matter is of key relevance in maintaining soil resistance and stability, although it is uncertain how deterioration of soil properties and changes in fungal communities affect the functional stability of soils. Degradation of soil properties followed by deforestation may lead to decreases in soil fungal diversity and functional stability (Chaer et al., 2009).
Soil health conditions have a tremendous impact on environmental sustainability including sustainability in agriculture, horticulture, and forestry. Moreover, soil health is directly connected with the production of healthy food which impacts public and animal health. More research is required to find the best way to maintain fungal biodiversity in soil, taking into consideration fungal functions and ecosystem services, including disease control, contamination detection, and bioremediation. Having the right tools, and being able to both identify species and characterize their role in the environment is important. The ability to compare functional structures between ecosystems and predict responses to environmental changes and interventions would be a useful advance.
MF, SH, MB, and MJ: wrote, drafted, read, corrected, improved, revised, and accepted the last version of manuscript.
This paper was co-financed by The National Centre for Research and Development in frame of the project BIOSTRATEG, contract number BIOSTRATEG3/347464/5/NCBR/2017.
The authors declare that the research was conducted in the absence of any commercial or financial relationships that could be construed as a potential conflict of interest.
The authors greatly thank Dr. William Truman, Institute of Plant Genetics, Polish Academy of Sciences for the critical correction of the manuscript.
Abawi, G. S., and Widmer, T. L. (2000). Impact of soil health management practices on soilborne pathogens, nematodes and root diseases of vegetable crops. Appl. Soil Ecol. 15, 37–47. doi: 10.1016/S0929-1393(00)00070-6
Allan, E., Van Ruijven, J., and Crawley, M. J. (2010). Foliar fungal pathogens and grassland biodiversity. Ecology 91, 2572–2582. doi: 10.1890/09-0859.1
Arnolds, E. (2001). “The future of fungi in Europe: threats, conservation and management,” in Fungal Conservation - Issues and Solutions, eds D. Moore, M. M. Nauta, S. E. Evans, and M. Rotheroe (Cambridge: Cambridge University Press), 64–80. doi: 10.1017/CBO9780511565168.005
Azcón-Aguilar, C., and Barea, J. M. (1997). Applying mycorrhiza biotechnology to horticulture: significance and potentials. Sci. Hortic. 68, 1–24. doi: 10.1016/S0304-4238(96)00954-5
Bagyaraj, D. J., and Ashwin, R. (2017). Soil biodiversity: role in sustainable horticulture. Biodivers. Hortic. Crops 5, 1–18. doi: 10.1016/j.jenvman.2017.08.001
Baldrian, P. (2003). Interactions of heavy metals with white-rot fungi. Enzyme Microb. Technol. 32, 78–91. doi: 10.1016/S0141-0229(02)00245-4
Bálint, M., Schmidt, P. A., Sharma, R., Thines, M., and Schmitt, I. (2014). An Illumina metabarcoding pipeline for fungi. Ecol. Evol. 4, 2642–2653. doi: 10.1002/ece3.1107
Bastida, F., Torres, I. F., Hernandez, T., and García, C. (2017). The impacts of organic amendments: do they confer stability against drought on the soil microbial community? Soil Biol. Biochem. 113, 173–183. doi: 10.1016/j.soilbio.2017.06.012
Baum, C., El-Tohamy, W., and Gruda, N. (2015). Increasing the productivity and product quality of vegetable crops using arbuscular mycorrhizal fungi: a review. Sci. Hortic. 187, 131–141. doi: 10.1016/j.scienta.2015.03.002
Behnke-Borowczyk, J., Kwaśna, H., and Bełka, M. (2012). Molecular methods used in studies of diversity of the soil microorganisms. Sylwan 156, 294–304.
Blagodatskaya, E., and Kuzyakov, Y. (2013). Active microorganisms in soil: critical review of estimation criteria and approaches. Soil Biol. Biochem. 67, 192–211. doi: 10.1016/j.soilbio.2013.08.024
Cai, X. B., Peng, Y. L., Yang, M. N., Zhang, T., and Zhang, Q. (2014). Grassland degradation decrease the diversity of arbuscular mycorrhizal fungi species in Tibet Plateau. Not. Bot. Horti Agrobot. 42, 333–339. doi: 10.15835/nbha4229458
Cardoso, E. J. B. N., Vasconcellos, R. L. F., Bini, D., Miyauchi, M. Y. H., dos Santos, C. A., Alves, P. R. L., et al. (2013). Soil health: looking for suitable indicators. What should be considered to assess the effects of use and management on soil health? Sci. Agric. 70, 274–289. doi: 10.1590/S0103-90162013000400009
Cassman, N. A., Leite, M. F. A., Pan, Y., de Hollander, M., van Veen, J. A., and Kuramae, E. E. (2016). Plant and soil fungal but not soil bacterial communities are linked in long-term fertilized grassland. Sci. Rep. 6:23680. doi: 10.1038/srep23680
Castañeda, L. E., and Barbosa, O. (2017). Metagenomic analysis exploring taxonomic and functional diversity of soil microbial communities in Chilean vineyards and surrounding native forests. PeerJ 5:e3098. doi: 10.7717/peerj.3098
Chaer, G., Fernandes, M., Myrold, D., and Bottomley, P. (2009). Comparative resistance and resilience of soil microbial communities and enzyme activities in adjacent native forest and agricultural soils. Microb. Ecol. 58, 414–424. doi: 10.1007/s00248-009-9508-x
Cookson, W. R., O’Donnell, A. J., Grant, C. D., Grierson, P. F., and Murphy, D. V. (2008). Impact of ecosystem management on microbial community level physiological profiles of postmining forest rehabilitation. Microb. Ecol. 55, 321–332. doi: 10.1007/s00248-007-9278-2
Creamer, R. E., Hannula, S. E., and van Leeuwen, J. P. (2016). Ecological network analysis reveals the inter-connection between soil biodiversity and ecosystem function as affected by land use across Europe. Appl. Soil Ecol. 97, 112–124. doi: 10.1016/j.apsoil.2015.08.006
Cretoiu, M. S., Korthals, G. W., Visser, J. H. M., and van Elsas, J. D. (2013). Chitin amendment increases soil suppressiveness toward plant pathogens and modulates the actinobacterial and oxalobacteraceal communities in an experimental agricultural field. Appl. Environ. Microbiol. 79, 5291–5301. doi: 10.1128/AEM.01361-13
Damon, C., Lehembre, F., Oger-Desfeux, C., Luis, P., Ranger, J., Fraissinet-Tachet, L., et al. (2012). Metatranscriptomics reveals the diversity of genes expressed by eukaryotes in forest soils. PLoS One 7:e28967. doi: 10.1371/journal.pone.0028967
Dawidziuk, A., Popiel, D., Kaczmarek, J., Strakowska, J., and Jedryczka, M. (2016). Morphological and molecular properties of Trichoderma species help to control stem canker of oilseed rape. BioControl 61, 755–768.
De Coninck, B., Timmermans, P., Vos, C., Cammue, B. P. A., and Kazan, K. (2015). What lies beneath: belowground defense strategies in plants. Trends Plant Sci. 20, 91–101. doi: 10.1016/j.tplants.2014.09.007
Deacon, L. J., Pryce-Miller, E. J., Frankland, J. C., Bainbridge, B. W., Moore, P. D., and Robinson, C. H. (2006). Diversity and function of decomposer fungi from a grassland soil. Soil Biol. Biochem. 38, 7–20. doi: 10.1016/j.soilbio.2005.04.013
Delgado-Baquerizo, M., Powell, J. R., Hamonts, K., Reith, F., Mele, P., Brown, M. V., et al. (2017). Circular linkages between soil biodiversity, fertility and plant productivity are limited to topsoil at the continental scale. New Phytol. 215, 1186–1196. doi: 10.1111/nph.14634
Dengler, J., Janisová, M., Török, P., and Wellstein, C. (2014). Biodiversity of Palaearctic grasslands: a synthesis. Agric. Ecosyst. Environ. 182, 1–14. doi: 10.1016/j.agee.2013.12.015
Ding, J., Jiang, X., Guan, D., Zhao, B., Ma, M., Zhou, B., et al. (2017). Influence of inorganic fertilizer and organic manure application on fungal communities in a long-term field experiment of Chinese Mollisols. Appl. Soil Ecol. 111, 114–122. doi: 10.1016/j.apsoil.2016.12.003
Doran, J. W., and Jones, A. J. (1996). “Soil quality and health: indicators of sustainability,” in Methods for Assessing Soil Quality. SSSA Special Publication Number 49, eds J. W. Doran and A. J. Jones (Madison, WI: Soil Science Society of America), XI–XIV.
Doran, J. W., and Parkin, T. B. (1994). “Defining and assessing soil quality,” in Defining Soil Quality for a Sustainable Environment. SSSA Special Publication Number 35, eds J. W. Doran, D. C. Coleman, D. F. Bezdicek, and B. A. Stewart (Madison, WI: Soil Science Society of America), 3–21. doi: 10.2136/sssaspecpub35.c1
El-Komy, M. H., Saleh, A. A., Eranthodi, A., and Molan, Y. Y. (2015). Characterization of novel Trichoderma asperellum isolates to select effective biocontrol agents against tomato Fusarium wilt. Plant Pathol. J. 31, 50–60. doi: 10.5423/PPJ.OA.09.2014.0087
Ferris, H., and Tuomisto, H. (2015). Unearthing the role of biological diversity in soil health. Soil Biol. Biochem. 85, 101–109. doi: 10.1016/j.soilbio.2015.02.037
Frąc, M., Jezierska-Tys, S., and Takashi, Y. (2015). Occurrence, detection, and molecular and metabolic characterization of heat-resistant fungi in soils and plants and their risk to human health. Adv. Agron. 132, 161–204.
Frąc, M., Weber, J., Gryta, A., Dêbicka, M., Kocowicz, A., Jamroz, E., et al. (2017). Microbial functional diversity in podzol ectohumus horizons affected by alkaline fly ash in the vicinity of electric power plant. Geomicrobiol. J. 34, 579–586.
Gałązka, A., Gawryjołek, K., Grządziel, J., Frąc, M., and Księżak, J. (2017). Microbial community diversity and the interaction of soil under maize growth in different cultivation techniques. Plant Soil Environ. 63, 264–270. doi: 10.17221/171/2017-PSE
Geiser, D. M., del Mar Jiménez-Gasco, M., Kang, S., Makalowska, I., Veeraraghavan, N., Ward, T. J., et al. (2004). FUSARIUM-ID v. 1.0: a DNA sequence database for identifying Fusarium. Eur. J. Plant Pathol. 110, 473–479.
Girisha, G. K., Condron, L. M., Clinton, P. W., and Davis, M. R. (2003). Decomposition and nutrient dynamics of green and freshly fallen radiata pine (Pinus radiata) needles. For. Ecol. Manag. 179, 169–181. doi: 10.1016/S0378-1127(02)00518-2
Gonzalez, M., Pujol, M., Metraux, J. P., Gonzalez-Garcia, V., Bolton, M. D., and Borrás-Hidalgo, O. (2011). Tobacco leaf spot and root rot caused by Rhizoctonia solani Kuhn. Mol. Plant Pathol. 12, 209–216. doi: 10.1111/j.1364-3703.2010.00664.x
Goss, M. J., and deVarennes, A. (2002). Soil disturbance reduces the efficacy of mycorrhizal associations for early soybean growth and N2 fixation. Soil Biol. Biochem. 34, 1167–1173. doi: 10.1016/S0038-0717(02)00053-6
Gweon, H. S., Oliver, A., Taylor, J., Booth, T., Gibbs, M., Read, D. S., et al. (2015). PIPITS: an automated pipeline for analyses of fungal internal transcribed spacer sequences from the Illumina sequencing platform. Methods Ecol. Evol. 6, 973–980. doi: 10.1111/2041-210X.12399
Hannula, S. E., Morrien, E., and de Hollander, M. (2017). Shifts in rhizosphere fungal community during secondary succession following abandonment from agriculture. ISME J. 11, 2294–2304. doi: 10.1038/ismej.2017.90
Hannula, S. E., and van Veen, J. A. (2016). Primer sets developed for functional genes reveal shifts in functionality of fungal community in soils. Front. Microbiol. 7:1897. doi: 10.3389/fmicb.2016.01897
Hesse, C. N., Mueller, R. C., Vuyisich, M., Gallegos-Graves, L. V., Gleasner, C. D., Zak, D. R., et al. (2015). Forest floor community metatranscriptomes identify fungal and bacterial responses to N deposition in two maple forests. Front. Microbiol. 6:337. doi: 10.3389/fmicb.2015.00337
Högberg, M. N., and Högberg, P. (2002). Extramatrical ectomycorrhizal mycelium contributes one-third of microbial biomass and produces, together with associated roots, half the dissolved organic carbon in a forest soil. New Phytol. 154, 791–795. doi: 10.1046/j.1469-8137.2002.00417.x
Huhe, Y. C., Chen, X., Hou, F., Wu, Y., and Cheng, Y. (2017). Bacterial and fungal community structures in loess plateau grasslands with different grazing intensities. Front. Microbiol. 8:606. doi: 10.3389/fmicb.2017.00606
Jayne, B., and Quigley, M. (2014). Influence of arbuscular mycorrhiza on growth and reproductive response of plants under water deficit: a meta-analysis. Mycorrhiza 24, 109–119. doi: 10.1007/s00572-013-0515-x
Jiménez-Morillo, N. T., González-Pérez, J. A., Jordán, A., Zavala, L. M., de la Rosa, J. M., Jiménez-González, M. A., et al. (2016). Organic matter fractions controlling soil water repellency in sandy soils from the Doñana National Park (Southwestern Spain). Land Degrad. Dev. 27, 1413–1423. doi: 10.1002/ldr.2314
Johnson, D., Vandenkoornhuyse, P. J., Leake, J. R., Gilbert, L., Booth, R. E., Grime, J. P., et al. (2003). Plant communities affect arbuscular mycorrhizal fungal diversity and community composition in grassland microcosms. New Phytol. 161, 503–515. doi: 10.1046/j.1469-8137.2003.00938.x
Joubert, L., Pryke, J. S., and Samways, M. J. (2017). Applied vegetation science 20 (2017) 340–351 moderate grazing sustains plant diversity in Afromontane grassland. Appl. Veg. Sci. 20, 340–351. doi: 10.1111/avsc.12310
Kibblewhite, M. G., Ritz, K., and Swift, M. J. (2008). Soil health in agricultural systems. Philos. Trans. R. Soc. B Biol. Sci. 363, 685–701. doi: 10.1098/rstb.2007.2178
Klosterman, S. J., Atallah, Z. K., Vallad, G. E., and Subbarao, K. V. (2009). Diversity, pathogenicity, and management of Verticillium species. Annu. Rev. Phytopathol. 47, 39–62. doi: 10.1146/annurev-phyto-080508-081748
Kurakov, A. V., Kharin, S. A., and Byzov, B. A. (2016). Changes in the composition and physiological and biochemical properties of fungi during passage through the digestive tract of earthworms. Biol. Bull. 43, 290–299.
Leff, J. W., Jones, S. E., Prober, S. M., Barberán, A., Borer, E. T., Firn, J. L., et al. (2015). Consistent responses of soil microbial communities to elevated nutrient inputs in grasslands across the globe. Proc. Natl. Acad. Sci. U.S.A. 112, 10967–10972. doi: 10.1073/pnas.1508382112
Lindahl, B. D., Nilsson, R. H., Tedersoo, L., Abarenkov, K., Carlsen, T., Kjøller, R., et al. (2013). Fungal community analysis by high-throughput sequencing of amplified markers – a user’s guide. New Phytol. 199, 288–299. doi: 10.1111/nph.12243
Liu, J., Sui, Y., Yu, Z., Shi, Y., Chu, H., Jin, J., et al. (2015). Soil carbon content drives the biogeographical distribution of fungal communities in the black soil zone of northeast China. Soil Biol. Biochem. 83, 29–39. doi: 10.1016/j.soilbio.2015.01.009
López-Bucio, J., Pelagio-Flores, R., and Herrera-Estrell, A. (2015). Trichoderma as biostimulant: exploiting the multilevel properties of a plant beneficial fungus. Sci. Hortic. 196, 109–123. doi: 10.1016/j.scienta.2015.08.043
Lucas, R. W., Casper, B. B., Jackson, J. K., and Balser, T. C. (2007). Soil microbial communities and extracellular enzyme activity in the New Jersey Pinelands. Soil Biol. Biochem. 39, 2508–2519. doi: 10.1016/j.soilbio.2007.05.008
Małecka, I., Blecharczyk, A., Sawińska, Z., Swêdrzyńska, D., and Piechota, T. (2015). Winter wheat yield and soil properties response to long-term non-inversion tillage. J. Agric. Sci. Technol. 17, 1571–1584.
Michielse, C. B., and Rep, M. (2009). Pathogen profile update: Fusarium oxysporum. Mol. Plant Pathol. 10, 311–324. doi: 10.1111/j.1364-3703.2009.00538.x
Morrien, E., Hannula, S. E., Snoek, L. B., Helmsing, N. R., Zweers, H., de Hollander, M., et al. (2017). Soil networks become more connected and take up more carbon as nature restoration progresses. Nat. Commun. 8:14349. doi: 10.1038/ncomms14349
Nguyen, N. H., Song, Z., Bates, S. T., Branco, S., Tedersoo, L., Menke, J., et al. (2016). FUNGuild: an open annotation tool for parsing fungal community datasets by ecological guild. Fungal Ecol. 20, 241–248. doi: 10.1016/j.funeco.2015.06.006
Öster, M. (2006). Biological Diversity Values in Semi-natural Grasslands - Indicators, Landscape Context and Restoration. Doctoral dissertation, Stockholm University, Stockholm.
Pal, A., Ghosh, S., and Paul, A. K. (2006). Biosorption of cobalt by fungi from serpentine soil of Andaman. Bioresour. Technol. 97, 1253–1258.
Phosri, C., Polme, S., Taylor, A. F. S., Koljalg, U., Suwannasai, N., and Tedersoo, L. (2012). Diversity and community composition of ectomycorrhizal fungi in a dry deciduous dipterocarp forest in Thailand. Biodivers. Conserv. 21, 2287–2298. doi: 10.1007/s10531-012-0250-1
Porras-Alfaro, A., Herrera, J., Natvig, D. O., Lipinski, K., and Sinsabaugh, R. L. (2011). Diversity and distribution of soil fungal communities in a semiarid grassland. Mycologia 103, 10–21. doi: 10.3852/09-297
Porras-Alfaro, A., Liu, K. L., Kuske, C. R., and Xie, G. (2014). From genus to phylum: large-subunit and internal transcribed spacer rRNA operon regions show similar classification accuracies influenced by database composition. Appl. Environ. Microbiol. 80, 829–840. doi: 10.1128/AEM.02894-13
Prosser, J. I. (2010). Replicate or lie. Environ. Microbiol. 12, 1806–1810. doi: 10.1111/j.1462-2920.2010.02201.x
Prosser, J. I. (2015). Dispersing misconceptions and identifying opportunities for the use of ‘omics’ in soil microbial ecology. Nat. Rev. Microbiol. 13, 439–446. doi: 10.1038/nrmicro3468
Rillig, M. C., and Mummey, D. L. (2006). Mycorrhizas and soil structure. New Phytol. 171, 41–53. doi: 10.1111/j.1469-8137.2006.01750.x
Rouphael, Y., Franken, P., Schneider, C., Schwarz, D., Giovannetti, M., Agnolucci, M., et al. (2015). Arbuscular mycorrhizal fungi act as biostimulants in horticultural crops. Sci. Hortic. 196, 91–108. doi: 10.1016/j.scienta.2015.09.002
Salter, S. J., Cox, M. J., Turek, E. M., Calus, S. T., Cookson, W. O., Moffatt, M. F., et al. (2014). Reagent and laboratory contamination can critically impact sequence-based microbiome analyses. BMC Biol. 12:87. doi: 10.1186/s12915-014-0087-z
Santos-González, J. C. (2007). Diversity of Arbuscular Mycorrhizal Fungi in Grasslands and Arable Fields. Doctor’s dissertation, Swedish University of Agricultural Sciences, Uppsala.
Schoch, C. L., Seifert, K. A., Huhndorf, S., Robert, V., Spouge, J. L., Levesque, C. A., et al. (2012). Nuclear ribosomal internal transcribed spacer (ITS) region as a universal DNA barcode marker for fungi. Proc. Natl. Acad. Sci. U.S.A. 109, 6241–6246. doi: 10.1073/pnas.1117018109
Schoenholtz, S. H., Van Miegroet, H., and Burger, J. A. (2000). A review of chemical and physical properties as indicators of forest soil quality: challenges and opportunities. For. Ecol. Manag. 138, 335–356. doi: 10.1016/S0378-1127(00)00423-0
Schöler, A., Jacquiod, S., Vestergaard, G., Schulz, S., and Schloter, M. (2017). Analysis of soil microbial communities based on amplicon sequencing of marker genes. Biol. Fertil. Soil 53, 485–489. doi: 10.1007/s00374-017-1205-1
Smith, D. P., and Peay, K. G. (2014). Sequence depth, not PCR replication, improves ecological inference from next generation DNA sequencing. PLoS One 9:e90234. doi: 10.1371/journal.pone.0090234
Sun, J. M., Irzykowski, W., Jędryczka, M., and Han, F. X. (2005). Analysis of the genetic structure of Sclerotinia sclerotiorum (Lib.) de Bary populations from different regions and host plants by Random Amplified Polymorphic DNA markers. J. Integr. Plant Biol. 47, 385–395. doi: 10.1111/j.1744-7909.2005.00077.x
Swift, M. J. (2005). “Human impacts on biodiversity and ecosystem services: an overview,” in The Fungal Community its Organization and Role in Ecosystems, eds J. Dighton, J. F. White, and P. Oudemans (Boca Raton, FL: CRC Press), 627–641.
Tedersoo, L., Bahram, M., Põlme, S., Kõljalg, U., Yorou, N. S., Wijesundera, R., et al. (2014). Global diversity and geography of soil fungi. Science 346:1256688. doi: 10.1126/science.1256688
Thilagar, G., and Bagyaraj, D. J. (2015). Influence of different arbuscular mycorrhizal fungi on growth and yield of chilly. Proc. Natl. Acad. Sci. India B Biol. Sci. 85, 71–75. doi: 10.1007/s40011-013-0262-y
Thomson, B. C., Tisserant, E., Plassart, P., Uroz, S., Griffiths, R. I., Hannula, E. S., et al. (2015). Soil conditions and land use intensification effects on soil microbial communities across a range of European field sites. Soil Biol. Biochem. 88, 403–413. doi: 10.1016/j.soilbio.2015.06.012
Tian, D. L., Peng, Y. Y., Yan, W. D., Fang, X., Kang, W. X., Wang, G. J., et al. (2010). Effects of thinning and litter fall removal on fine root production and soil organic carbon content in Masson pine plantations. Pedosphere 20, 486–493. doi: 10.1016/S1002-0160(10)60038-0
Treseder, K. K., and Lennon, J. T. (2015). Fungal traits that drive ecosystem dynamics on land. Microbiol. Mol. Biol. Rev. 79, 243–262. doi: 10.1128/MMBR.00001-15
Turner, T. R., Ramakrishna, K., Walshaw, J., Heavens, D., Alston, M., Swarbreck, D., et al. (2013). Comparative metatranscriptomics reveals kingdom level changes in the rhizosphere microbiome of plants. ISME J. 7, 2248–2258. doi: 10.1038/ismej.2013.119
Uroz, S., Ioannidis, P., Lengelle, J., Cébron, A., Morin, E., Buée, M., et al. (2013). Functional assays and metagenomic analyses reveals differences between the microbial communities inhabiting the soil horizons of a Norway spruce plantation. PLoS One 8:e55929. doi: 10.1371/journal.pone.0055929
van der Heijden, M. G. A., Klironomos, J. N., Ursic, M., Moutoglis, P., Streitwolf-Engel, R., Boller, T., et al. (1998). Mycorrhizal fungal diversity determines plant biodiversity, ecosystem variability and productivity. Nature 396, 69–72. doi: 10.1038/23932
van West, P., Appiah, A. A., and Gow, N. A. R. (2003). Advances in research on oomycete root pathogens. Physiol. Mol. Plant Pathol. 62, 99–113. doi: 10.1016/S0885-5765(03)00044-4
Wagg, C., Bender, S. F., Widmer, F., and Van der Heijden, M. G. A. (2014). Soil biodiversity and soil community composition determine ecosystem multifunctionality. Proc. Natl. Acad. Sci. U.S.A. 111, 5266–5270. doi: 10.1073/pnas.1320054111
Wakelin, S. A., Macdonald, L. M., O’Callaghan, M., Forrester, S. T., and Condron, L. M. (2014). Soil functional resistance and stability are linked to different ecosystem properties. Austral. Ecol. 39, 522–531. doi: 10.1111/aec.12112
Wardle, D. A. (2002). Communities and Ecosystems: Linking Aboveground and Belowground Components. Princeton, NJ: Princeton University Press.
Watanabe, M., Yonezawa, T., Lee, K., Kumagai, S., Sugita-Konishi, Y., Goto, K., et al. (2011). Evaluation of genetic markers for identifying isolates of the species of the genus Fusarium. J. Sci. Food Agric. 91, 2500–2504. doi: 10.1002/jsfa.4507
Weiss, S. J., Xu, Z., Amir, A., Peddada, S., Bittinger, K., Gonzalez, A., et al. (2015). Effects of library size variance, sparsity, and compositionality on the analysis of microbiome data. PeerJ 3:e1157v1. doi: 10.7287/peerj.preprints.1157v1
Winder, R. S., and Shamoun, S. F. (2006). Forest pathogens: friends or foe to biodiversity? Can. J. Plant Pathol. 28, 221–227. doi: 10.1080/07060660609507378
Wolińska, A., Frąc, M., Oszust, K., Szafranek-Nakonieczna, A., Zielenkiewicz, U., and Stêpniewska, Z. (2017). Microbial biodiversity of meadows under different modes of land use: catabolic and genetic fingerprinting. World J. Microbiol. Biotechnol. 33:154. doi: 10.1007/s11274-017-2318-2
Yang, T., Adams, J. M., Shi, Y., He, J., Jing, X., Chen, L., et al. (2017). Soil fungal diversity in natural grasslands of the Tibetan Plateau: associations with plant diversity and productivity. New Phytol. 215, 756–765. doi: 10.1111/nph.14606
Zhou, J., Jiang, X., Zhou, B., Zhao, B., Ma, M., Guan, D., et al. (2016). Thirty four years of nitrogen fertilization decreases fungal diversity and alters fungal community composition in black soil in northeast China. Soil Biol. Biochem. 95, 135–143. doi: 10.1016/j.soilbio.2015.12.012
Keywords: soil health, soil ecosystem, microbial communities, fungal diversity, fungal functions, fungal plant pathogens, soil biology, soil mycobiome
Citation: Frąc M, Hannula SE, Bełka M and Jędryczka M (2018) Fungal Biodiversity and Their Role in Soil Health. Front. Microbiol. 9:707. doi: 10.3389/fmicb.2018.00707
Received: 30 September 2017; Accepted: 27 March 2018;
Published: 13 April 2018.
Edited by:
Mohamed Hijri, Université de Montréal, CanadaReviewed by:
Mariusz Cycoń, Medical University of Silesia, PolandCopyright © 2018 Frąc, Hannula, Bełka and Jędryczka. This is an open-access article distributed under the terms of the Creative Commons Attribution License (CC BY). The use, distribution or reproduction in other forums is permitted, provided the original author(s) and the copyright owner are credited and that the original publication in this journal is cited, in accordance with accepted academic practice. No use, distribution or reproduction is permitted which does not comply with these terms.
*Correspondence: Małgorzata Jędryczka, bWplZEBpZ3IucG96bmFuLnBs
Disclaimer: All claims expressed in this article are solely those of the authors and do not necessarily represent those of their affiliated organizations, or those of the publisher, the editors and the reviewers. Any product that may be evaluated in this article or claim that may be made by its manufacturer is not guaranteed or endorsed by the publisher.
Research integrity at Frontiers
Learn more about the work of our research integrity team to safeguard the quality of each article we publish.