- 1Key Laboratory of Food Safety Risk Assessment, Ministry of Health, China National Center for Food Safety Risk Assessment, Beijing, China
- 2College of Veterinary Medicine, South China Agricultural University, Guangzhou, China
- 3Heilongjiang Provincial Center for Disease Control and Prevention, Harbin, China
- 4Institute of Microbiology, University of Agriculture Faisalabad, Faisalabad, Pakistan
- 5UCD-Centre for Food Safety, School of Public Health, Physiotherapy and Sports Science, University College Dublin, Dublin, Ireland
- 6Institute for Global Food Security, School of Biological Sciences, Queen’s University Belfast, Belfast, Ireland
One mcr-1-carrying ST34-type Salmonella Typhimurium WW012 was cultured from 3,200 ready-to-eat (RTE) pork samples in 2014 in China. Broth dilution method was applied to obtain the antimicrobial susceptibility of Salmonella Typhimurium WW012. Broth matting assays were carried out to detect transferability of this phenotype and whole-genome sequencing was performed to analyze its genomic characteristic. Thirty out of 3,200 RTE samples were positive for Salmonella and the three most frequent serotypes were identified as S. Derby (n = 8), S. Typhimurium (n = 6), and S. Enteritidis (n = 6). One S. Typhimurium isolate (S. Typhimurium WW012) cultured from RTE prepared pork was found to contain the mcr-1 gene. S. Typhimurium WW012 expressed a level of high resistance to seven different antimicrobial compounds in addition to colistin (MIC = 8 mg/L). A single plasmid, pWW012 (151,609-bp) was identified and found to be of an IncHI2/HI2A type that encoded a mcr-1 gene along with six additional antimicrobial resistance genes. Plasmid pWW012 contained an IS30-mcr-1-orf-orf-IS30 composite transposon that can be successfully transferred to Escherichia coli J53. When assessed further, the latter demonstrated considerable similarity to three plasmids pHYEC7-mcr-1, pSCC4, and pHNSHP45-2, respectively. Furthermore, plasmid pWW012 also contained a multidrug resistance (MDR) genetic structure IS26-aadA2-cmlA2-aadA1-IS406-sul3-IS26-dfrA12-aadA2-IS26, which showed high similarity to two plasmids, pHNLDF400 and pHNSHP45-2, respectively. Moreover, genes mapping to the chromosome (4,991,167-bp) were found to carry 28 mutations, related to two component regulatory systems (pmrAB, phoPQ) leading to modifications of lipid A component of the lipopolysaccharide structure. Additionally, one mutation (D87N) in the quinolone resistance determining region (QRDR) gene of gyrA was identified in this mcr-1 harboring S. Typhimurium. In addition, various virulence factors and heavy metal resistance-encoding genes were also identified on the genome of S. Typhimurium WW012. This is the first report of the complete nucleotide sequence of mcr-1-carrying MDR S. Typhimurium strain from RTE pork in China.
Introduction
Salmonella is often acquired from contaminated food, and is an important zoonotic bacterium linked to cases of gastroenteritis and bacteremia. This bacterium is now the leading cause of global bacterial food poisoning out breaks and is associated with increased morbidity and mortality (Kirk et al., 2015). There are estimated to be 94 million cases of gastroenteritis globally per year, with 155,000 deaths attributed to this bacterium (Majowicz et al., 2010; Gallati et al., 2013). It was reported that between 1994 and 2005, approximately 22% of food borne infections in China were caused by Salmonella (Wang et al., 2007). Of note, Salmonella Typhimurium is one of the most prevalent serovars identified among these food borne illnesses in China (Zhang et al., 2014). Salmonella Typhimurium strains are most commonly isolated from retail meat particularly pork in China, and both sporadic and outbreak-associated cases of human salmonellosis caused by this bacterium often need clinical therapy (Zhang et al., 2016). Moreover, this bacterium has the potential to act as a reservoir for different antimicrobial resistance-encoding genes (Zhang et al., 2016).
The relevance of S. Typhimurium is also marked by its capability to acquire resistance determinants, to various drug classes, especially those that are third-generation cephalosporins, tetracyclines, (fluoro) quinolones, folate pathway inhibitors, phenicols, penicillines, aminoglycosides and macrolides (Wong et al., 2014; Yi et al., 2017). Resistance to polymyxin in bacteria including Salmonella is commonly due to chromosomal mutations in two component regulatory systems (e.g., pmrAB and phoPQ) resulting in structural modification of lipid A. Therefore, bacterial resistant to polymyxin was historically thought to be rare (Lee et al., 2014). However, recently, a new plasmid-encoding polymyxin resistance gene, mcr-1, was discovered from bacterial isolated from the environment, animals, and humans in China (Liu et al., 2016). Subsequently, occurrence of the mcr-1 gene has been reported worldwide, indicating a newly recognized global distribution. Furthermore, the mcr-1-bearing plasmids were found to possess the ability to disseminate between food-producing animal and human bacteria and were also highly stable in these backgrounds, even in the absence of polymyxin selection (Anjum et al., 2016; Kempf et al., 2016; Liu et al., 2016; El Garch et al., 2017).
The mcr-1 gene in Salmonella was originally detected from human and food in England and Wales, including 8 S. Typhimurium, 1 S. Paratyphi B var Java and 1 S. Virchow strains (Doumith et al., 2016). Since then, this gene was reported from Salmonella isolates in Europe, the United States, and China, from humans, food-producing animals, and their surrounding environment, and retail meat (Anjum et al., 2016; Campos et al., 2016; Figueiredo et al., 2016; Quesada et al., 2016; Vinueza-Burgos et al., 2016; Yang et al., 2016). Nonetheless, to date, few reports of the detection of mcr-1 have been cited in Salmonella from ready-to-eat (RTE) pork meat in China. In this study, we report for the first time, the prevalence of Salmonella species among RTE pork in China, and while one S. Typhimurium isolate was then found to be positive for the mcr-1 gene, which was located on a large transferable plasmid, along with multiple antimicrobial resistance genes. The complete genome sequence of both the plasmid and chromosome was determined to shed insight into the diversity and complexity of this interesting strain.
Materials and Methods
Strains Isolation
A total of 3,200 RTE pork samples were collected from 32 retail outlets and 32 commercial hypermarkets, in 32 provincial capitals of China in 2014 (Figure 1). Fifty RTE pork samples were collected at each sampling site and all were stored inside tightly sealed aseptic bags, surrounded by a biological ice bag, and then placed in a box maintained at a temperature lower than 4°C. Samples were immediately transported to the laboratory and subjected to microbiological analysis within 2 h. All samples were subjected to qualitative analysis for Salmonella using an enrichment method described by the National Food Safety Standard of China-Food microbiological examination, Salmonella (GB 4789.4-2010). Finally, presumptive Salmonella were selected for biochemical confirmation using API 20E test identification test strips (bioMérieux, Marcy l′ Etoile, France), as well as for molecular identification using PCR assay targeting the invA gene (Malorny et al., 2003). For all of the confirmed Salmonella isolates, serotypes were determined by the slide agglutination test, using Salmonella antisera (Statens Serum Institute, Denmark) according to the Kauffmann–White scheme. All confirmed Salmonella isolates were stored in brain heart infusion broth with 40% [v/v] glycerol (Land Bridge, Beijing, China) at -80°C. Each sample retained was represented by at least one bacterial isolate.
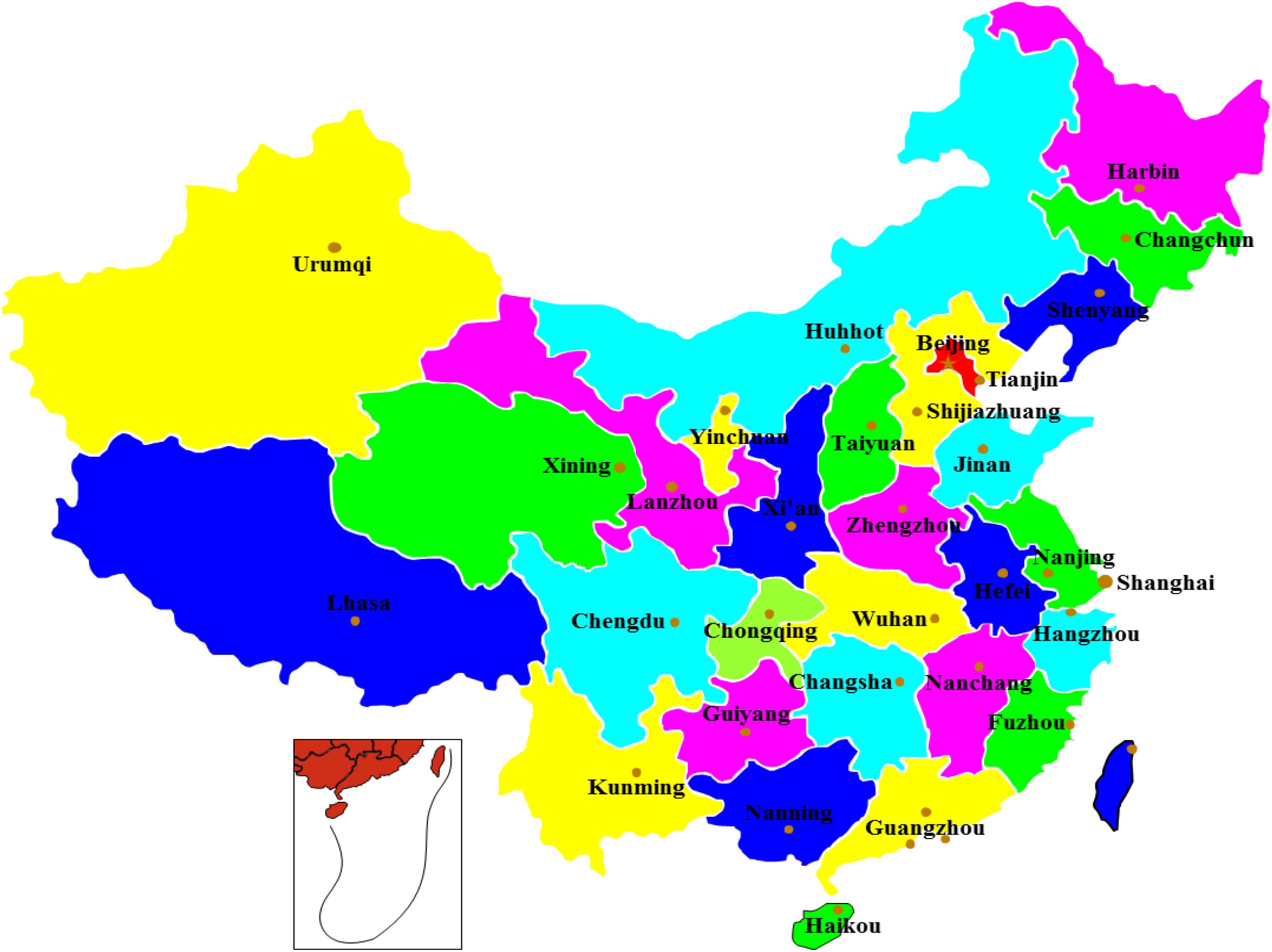
FIGURE 1. Map of China showing the locations of the 32 metropolitan cities from where the Salmonella isolates were collected.
Detection of mcr-1 Gene
The frozen strains were cultured overnight at 37°C in brain heart infusion broth. A TIANamp Bacterial DNA extraction kit (TianGen DNA Kit DP302, Beijing, China) was used to extract the genomic DNA from the culture according to the manufacturer’s instructions. A NanoDrop-2000 spectrophotometer (Thermo Fisher Scientific, NH, United States) was then used to evaluate the quality of DNA. PCR was performed to confirm the presence of the mcr-1 gene among the studied strains using primers targeting mcr-1 as reported previously (Liu et al., 2016). The genetic identity of all amplicons was subsequently confirmed by nucleotide sequencing (Liu et al., 2016). Finally, one S. Typhimurium isolate designated as S. Typhimurium WW012 was determined to harbor the mcr-1 gene and this isolate was selected to perform the follow-up experiments.
Antimicrobial Susceptibility Tests (AST)
Antimicrobial susceptibility testing (AST) of the S. Typhimurium WW012 isolate was performed using broth dilution method, using the Biofosun® Gram-negative panel (Fosun Diagnostics, Shanghai, China). Data obtained was interpreted according to standards and guidelines described by Clinical and Laboratory Standards Institute [CLSI] (2016). Additionally, we also used the National Antimicrobial Resistance Monitoring System (NARMS) protocol when CLSI standards were not available. The panel of 21 antimicrobial compounds as shown in Table 1 was tested Reference Escherichia coli strain ATCCTM25922 was used as the quality control.
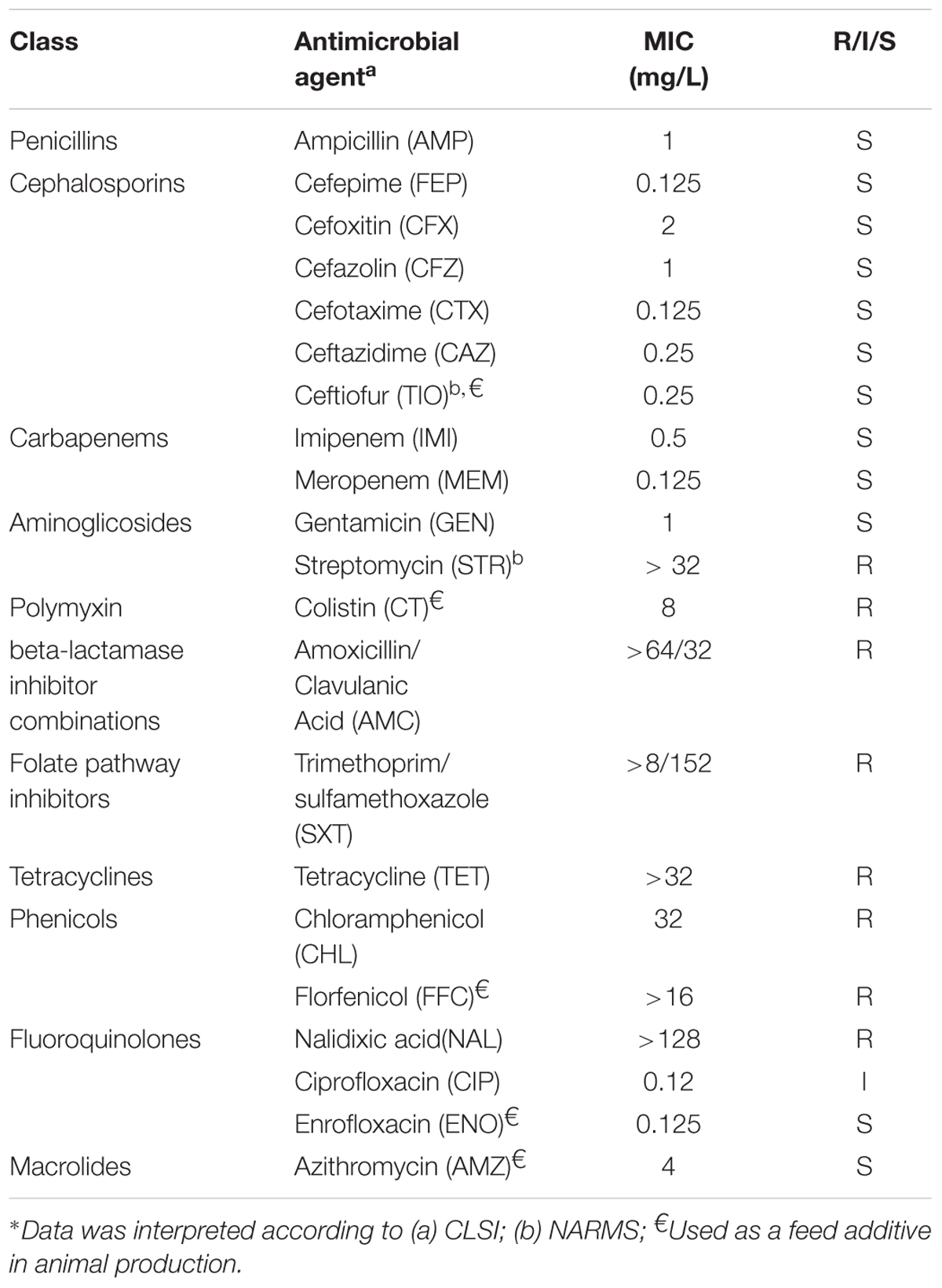
TABLE 1. Antimicrobial resistance test of S. Typhimurium WW012 to a panel of 21 antimicrobial agents∗.
Conjugal Plasmid Transfer and Characterization
The mcr-1 harboring S. Typhimurium WW012 isolate was analyzed for its ability to transfer the colistin resistance phenotype using broth matting to the plasmid-free recipients, E. coli J53. Transfer of mcr-1 to transconjugants was confirmed by PCR (Cui et al., 2017). Plasmid DNA profiles of both the donor and transconjugants were carried out using the S1-nuclease digestion pulsed-field gel electrophoresis (S1-PFGE) molecular sub-typing method (Liu et al., 2016).
Whole Genome Sequencing and Annotation
To assess the genomic background of S. Typhimurium WW012, whole-genome sequencing (WGS) was performed using the SMRT® Pacific Biosciences RS II platform (Pacific Biosciences, Menlo Park, CA, United States). Annotation of the genomes was performed using RAST1, BLASTn and BLASTp2 programs. The open reading frame (ORF) Finder program3 was used to identify features of public health interest. PlasmidFinder-1.34 was used to identify plasmid replicon types. The comprehensive antibiotic resistance database (CARD)5 was used to identify antimicrobial resistance genes. Virulence factor database (VFDB)6 was queried to predict the presence of virulence factors.
Three complete genomes, Salmonella Typhimurium LT2 (Accession number AE006468.1), Salmonella Typhi CT18 (AL513382.1), and Salmonella Indiana C629 (Accession number CP015724) were available from GenBank and used to compare the Salmonella pathogenicity islands (SPIs) with S. Typhimurium WW012 in this study by SPIFinder7. Finally, the antibacterial biocide and metal resistance genes database (BacMet)8 was used to predict the presence of any metal-resistance genes.
Nucleotide Accession Numbers
The complete genome of S. Typhimurium WW012 was deposited at GenBank under the Accession number CP022168 (chromosome) and CP022169 (plasmid p WW012).
Results
Prevalence of mcr-1-Positive Salmonella
In this study, we collected and tested a total of 3,200 RTE pork samples from 32 provincial capitals in China in 2014. Thirty (30/3,200, 0.94%) samples were positive for Salmonella; one isolate from each sample was selected for subsequent analyses. In total, thirty isolates were recovered (comprising 10 serotypes), and the three most frequent serotypes among RTE pork samples covering 66.7% of the total, were identified as S. Derby (n = 8), S. Typhimurium (n = 6), and S. Enteritidis (n = 6). One (1/30, 3.3%) S. Typhimurium isolate (designated as S. Typhimurium WW012) was cultured from RTE prepared pork collected from a convenience market in Nanning city, Guangxi Province, and found to contain the mcr-1 gene. The sampling details of isolates are shown in Supplementary Table S1.
Susceptibility to Antimicrobial Compounds
In this study, S. Typhimurium WW012 expressed a level of high resistance to seven different antimicrobial compounds in addition to colistin (MIC = 8 mg/L) (Table 1). The isolate was susceptible to all tested penicillins, cephalosporins, carbapenems, and macrolides (Table 1). Moreover, S. Typhimurium WW012 was also found to be susceptible to enrofloxacin, whilst expressing an intermediate resistant phenotype to ciprofloxacin (Table 1).
Horizontal Transfer of mcr-1 Genes and Associated Determinants
One plasmid was identified from S. Typhimurium WW012 by S1-PFGE and the conjugation assay showed that it could be transferred to a plasmid-free recipient E. coli J53 with a conjugation frequency of 1.2 × 10-6 as determined under laboratory conditions (Table 2 and Supplementary Figure S1). MIC value of the transconjugant were recorded at 8mg/L using broth dilution test, which showed 64-fold increase in when compared with the recipient E. coli J53 (0.125 mg/L). This transconjugant expressed a MDR phenotype and was found to be resistance to five antimicrobial compounds including of trimethoprim, sulfamethoxazole, streptomycin, tetracycline, chloramphenicol, and florfenicol, in addition to colistin.
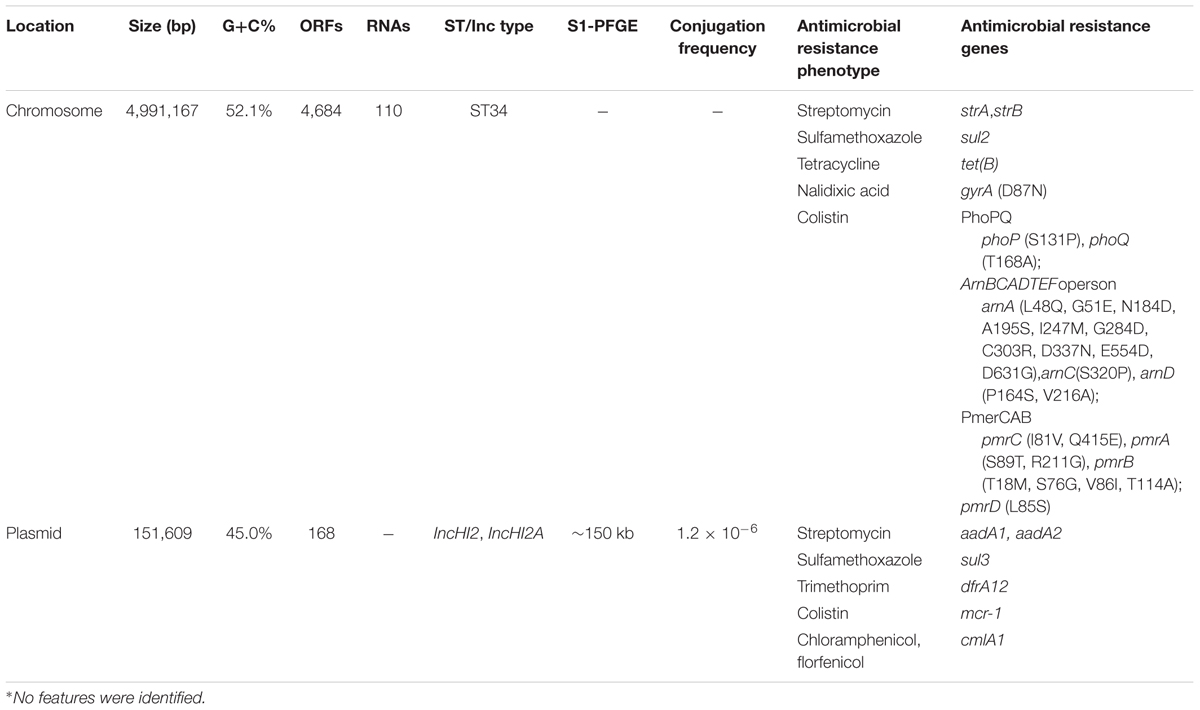
TABLE 2. The summary of the features associated with the genome and plasmid identified in S. Typhimurium WW012∗.
Genome Sequence Information
The S. Typhimurium WW012 genome consisted of a single circular chromosome and a circular plasmid (Table 2 and Supplementary Figure S2). The chromosome consisted of 4,991,167 bp with 4,684 predicted ORFs along with 110 RNAs. Meanwhile, a single circular extra-chromosomal element was identified and denoted as plasmid pWW012 of IncHI2 and IncHI2A replicon types. The total size of plasmid pWW012 was 151,609 bp including 168 predicted ORFs with the average GC content of 45.0% (Table 2). Moreover, the multilocus sequence type (MLST) of S. Typhimurium WW012 was identified as ST34 (Table 2).
Acquired Antimicrobial Resistance-Encoding Genes Identified in the Bacterial Genome
The CARD database was queried to identify resistance related genotypes on the genome of S. Typhimurium WW012. A total of 21 antimicrobial resistance genes (two genes were identified as aadA2) were identified, which encoded resistance to 11 antimicrobial agents of 10 different classes, including 14 genes on the chromosome and 7 on the plasmid (Table 2 and Supplementary Figure S2). In detail, when compared with S. Typhimurium LT2, one missense mutation in gyrA (D87N) and a total of 24 missense mutations in the PmrAB and PhoPQ systems were found within the chromosome of S. Typhimurium WW012. Moreover, four and five additional antimicrobial resistance genes of strA/B, sul2, tet(B) were also found within the chromosome and plasmid, respectively, (Table 2 and Supplementary Figure S2).
Virulence Factors Annotated in the Bacterial Genome
To identify potential virulence-encoding genes in S. Typhimurium WW012, the genome was used to query those factors listed in the VFDB. These were aligned to the ORF protein sequences using BLASTP and filtered with 90% identity and match length. Using this approach, 201 virulence factors were identified on the chromosome, among which 66 genes belonged to type-three secretion system (T3SS) encoding genes (Supplementary Figure S2 and Supplementary Table S2). The genomes of S. Typhimurium WW012 were also compared with those of S. Typhimurium LT2, S. Typhi CT18, and S. Indiana C629 in NCBI to identify the presence of SPIs. It was found that S. Typhimurium WW012 possessed SPI-3, SPI-4, SPI-5, SPI-13, SPI-14, and C63PI (Table 3) in addition to SPI-1 and SPI-2. Moreover, 63 fimbrial adherence-encoding genes and other virulence-encoding genes were identified on the chromosome of S. Typhimurium WW012 (Supplementary Figure S2 and Supplementary Table S2).
Metal Resistance Genes Annotated in the Bacterial Genome
The antibacterial biocide and metal resistance-encoding gene database (BacMet) was used to predict the presence of metal-resistance genes encoded on the genome of S. Typhimurium WW012. Twenty-eight resistance genes related to arsenic, copper, iron, zinc, magnesium, cobalt, mercury, molybdenum, nickel, and silver, were located on the chromosome, whilst 7 tellurium resistance encoding genes were identified as terA/B/C/D/E/W/Z (Supplementary Figure S2 and Supplementary Table S3) on plasmid pWW012.
Comparative Analysis of Plasmid pWW012
The mcr-1 gene located on plasmid pWW012 was bracketed by two IS30 elements that were located in the same orientation. Similar structures were found in plasmids pHYEC7-mcr-1 (KX518745), pHNSHP45-2 (KU341381), and pSCC4 (CP021078) recovered previously from E. coli and Citrobacter braakii, respectively, (Supplementary Figure S3A). The insertion element IS30 showed 100% (95% query coverage) of homologous sequence with ISApl1 in plasmid pHNSHP45-2, where in 17 amino acids were missing at the 5′-end comparing with the latter (Supplementary Figure S3A). In addition to the mcr-1 gene locus, plasmid pWW012 also contained an additional MDR gene cassette embedded in a complex class 1 integron of 15.2-kbp (Supplementary Figure S3B) bracketed by two IS26 elements in but found in inverted orientations directions, at a distance from the mcr-1 gene locus (Supplementary Figure S2). The class 1 integron was preceded by a copy of insertion element IS26, and two transposase-encoding genes tnpR and tnpM, followed by the aadA2, cmlA1, and aadA1 genes. Downstream of these resistance genes, an interesting resistance gene locus consisting of IS406–sul3-orf-orf-orf-IS26 was identified. This locus was followed by one integrase, two resistance genes of dfrA12 and aadA2, and the IS26 element. This structure has also been identified in two E. coli originated plasmids designated as pHNLDF400 (KV019258.1) and pHNSHP45-2 (KU341381) (Supplementary Figure S3B).
Discussion
In the past, Salmonella has attracted much attention owing to its important role as a food-borne pathogen. Recent studies reported on the prevalence of Salmonella and plasmid-encoded polymyxin resistance genes, containing mcr-1, among humans, food-producing animals and food samples in China and elsewhere (Anjum et al., 2016; Campos et al., 2016; Doumith et al., 2016; El Garch et al., 2016; Figueiredo et al., 2016; Kempf et al., 2016; Liu et al., 2016; Quesada et al., 2016; Yang et al., 2016). However, limited comprehensive epidemiological data are available describing the prevalence of Salmonella and mcr-1 gene among RTE pork samples in China, although pork and pork-related products are the main source of animal protein for Chinese consumers. To the best of our knowledge, the current study was the first report on the epidemiological prevalence and detection of Salmonella and mcr-1 gene among RTE pork samples in China. Generally, the prevalence rate of Salmonella and the mcr-1 gene among RTE pork samples was relatively low in this study, while similar results were observed from clinical, pigs and retail pork in previous studies (Figueiredo et al., 2016; Quesada et al., 2016; Cui et al., 2017). However, considering the fact that these RTE pork samples are positive for Salmonella and therefore would not be heated or cooked the infectious risk would increase. In China, only prepackaged RTE foodstuffs have a pathogen limiting standard applied at National level. In this study all RTE pork samples were collected from marketing sites and nearly 87% of the samples that were positive for Salmonella were unpackaged. Although these pork samples were permitted for sale by the food hygiene bureau at the beginning, unpacked treatment may later increase the probability of the contamination of Salmonella during their shelf-life. Therefore, limited standard and effective legislation in RTE pork to prevent and control the contamination of Salmonella should now be considered.
In this study, we successfully recovered and characterized one Salmonella isolate (S. Typhimurium WW012) that harbored a mcr-1-bearing plasmid from a RTE prepared pork sample in China. The genus Salmonella consists of a number of serovars, where in it is reported that the most often detected serovar harboring the mcr-1-was Typhimurium (Doumith et al., 2016; Cui et al., 2017), suggesting that the acquisition and prevalence of mcr-1-bearing mobile elements may require a specific genetic background. The relationship between S. Typhimurium and mcr-1-bearing plasmids warrants further investigation (Doumith et al., 2016). This RTE pork isolate expressed a MDR phenotype in addition to colistin. Notably, all of the resistant antimicrobial agents tested in this study are widely used at human and veterinary clinics. Carnevali et al. (2016) screened for the mcr-1 gene in 4,473 Salmonella isolates from human, food-producing animal, food and environment samples and identified 25 positive isolates of human and veterinary origin, all of which were susceptible to broad-spectrum cephalosporins. The S. Typhimurium WW012 isolate in this study was also susceptible to all tested cephalosporin antimicrobials. Similar results were reported from human, food-producing animals in China (Li et al., 2016; Yi et al., 2017).
A laboratory-based conjugation assay revealed that the mcr-1 gene was transferrable to the plasmid-free recipient E. coli J53, with a frequency of 1.2 × 10-6. In contrast, the transfer frequency of the IncI2-type plasmid carrying the mcr-1 gene reported in the original study was surprisingly higher, ranging from 10-1 to 10-3 between E. coli strains (Liu et al., 2016). In this study these data showed that the transfer of the mcr-1 gene from S. Typhimurium WW012 to E. coli had a frequency that was lower. Nonetheless, it was confirmed that the mcr-1-carrying IncHI2-type plasmid in this study can be transferred between different genera of Enterobacteriaceae. Of note, according to previous reports, the IncHI2 replicon was one of the most common found in Salmonella (Campos et al., 2016; Doumith et al., 2016). Moreover, several reports confirmed mcr-1-positive Salmonella that were expressing a MDR phenotype (Doumith et al., 2016; Figueiredo et al., 2016; Yang et al., 2016). Notably, the current data showed that this transconjugant showed resistance to five antimicrobial agents in addition to colistin, indicating that S. Typhimurium WW012 could not only transfer the mcr-1 gene but also other MDR-encoding genes into other Enterobacteriaceae strains. Therefore, once this clone carrying mcr-1 gene disseminates from RTE pork to humans via the food chain, it could be expected to promote the forward dissemination of the latter marker and other members of the Enterobacteriaceae. In this study S. Typhimurium WW012 belonged to ST34, which is the predominant sequence type in southern China (Sun et al., 2014). Recently, the mcr-1 gene was also detected in MDR S. Typhimurium (ST34) in China and Europe (Doumith et al., 2016; Li et al., 2016).
In this study, the plasmid mediated colistin resistance gene mcr-1 was found to be located in a composite transposon with the structure IS30-mcr-1-orf-orf-IS30, which was similar to those found previously on two E. coli plasmids pHYEC7-mcr-1 (KX518745) and pHNSHP45-2 (KU341381), and one C. braakii plasmid pSCC4 (CP021078, unpublished) (Liu et al., 2016; Wang et al., 2018). Unlike plasmid pHNSHP45-2, the original IncHI2-type mcr-1-carrying episome, the mcr-1 gene located on plasmids pWW012, pHYEC7-mcr-1 and pSCC4 was bracketed by IS30 elements. According to a latest report, ISApl1 is a member of the IS30 family of insertion sequences, which utilize a ‘copy-out, paste-in’ mechanism with a targeted transposition pathway requiring the formation of a synaptic complex between an inverted repeat (IR) in the transposon circle and an IR-like sequence in the target (Wang et al., 2018). IS30 is related to site-specific recombination, which could mediate the transmission of mcr-1 among different bacterial species. Moreover, it was found that several resistance genes were also found to be present and located between two IS26 elements on plasmid pWW012, in which a class 1 integron similar to that found in plasmids pHNSHP45-2 and pHNLDF400 (KY019258.1) also existed (Liu et al., 2016; Wang et al., 2017). The recovery of plasmid pWW012 that harbored structurally similar mobile elements to these contained in these reported plasmids implied that genetic exchange between such plasmids was common, and that such plasmids could readily be transferred to other bacteria, constituting one major evolution and resistance development route for bacterial pathogens.
In addition to the plasmid-mediated colistin resistance gene mcr-1, genes found on chromosome containing mutations, encoding two component regulatory systems (pmrAB, phoPQ), were also found in this study. These chromosomal mutations mediated mechanisms were thought to lead to the modification of lipid A, which anchors the lipopolysaccharide molecule to the outer membrane, resulting in reduction in the affinity to colistin (Lee et al., 2014). Moreover, a target gene mutation in the quinolone resistance determining region (QRDR) on the chromosome were identified in gyrA mutation (giving rise to D87N amino acid substitution) in this mcr-1 harboring S. Typhimurium. The latter is frequently reported in other MDR Salmonella species in China (Wong et al., 2014). In addition, strA/B encoding streptomycin resistance and sul2 encoding sulfamethoxazole resistances were also detected to locate on the chromosome of S. Typhimurium WW012.
In this study, 201 virulence genes were found on the chromosome of S. Typhimurium WW012 when queried through VFDB. Type-three secretion systems (T3SS) encoding genes related to SPI-1 and SPI-2 were commonly detected. Both SPI-1 and SPI-2 contained two independent type three secretion systems (denoted as TTSS SPI-1 and TTSS SPI-2, respectively) that can inject effect or proteins into host cells, which are crucial for various stages of infection (Weening et al., 2005). Comparison of the genomes S. Typhimurium WW012 with the reference or complete sequences in NCBI for the presence of SPIs showed that SPIs in S. Typhimurium WW012 were closely related to their equivalents in S. Typhimurium LT2 (McClelland et al., 2001), and shared some of those in S. Typhi CT18 and S. Indiana C629 (Parkhill et al., 2001; Wang et al., 2016). Additionally, 63 fimbrial adherence encoding genes on the chromosome of S. Typhimurium WW012 were also identified in this study. Several in vitro studies have suggested that fimbrial-encoding genes found in Salmonella could mediate the attachment of this bacterium to epithelial cells in the host (Leclerc et al., 2016; Peters et al., 2017). The latter phenotype may support long-term intestinal carriage of this bacterium.
Heavy metal compounds as well as antimicrobial agents are widely used as feed additives in food-producing animals for therapy, and growth purposes in China (Hu et al., 2017). Because of their stable and persistent characteristics, when heavy metals accumulate to specific concentrations, they potentially result in resistance among bacteria cultured from food-producing animals. Heavy metal resistance-encoding genes have also been identified in different environments (Deng et al., 2017). Recent research data showed that a relationship between the acquisition of heavy metal resistance genes and antimicrobial resistance genes, and antimicrobial resistance may arise through co-resistance or cross-resistance to metals or co-regulation of resistance pathways (Argudín et al., 2016; Deng et al., 2017). In this study, 28 and 7 heavy metal resistance genes were found on the chromosome and plasmid, respectively. These heavy metal resistance genes might increase antimicrobial resistance capacity within this bacterium, through co-selection of determinants.
Conclusion
This study firstly reported the epidemiological prevalence and detection of Salmonella and mcr-1 gene among RTE pork samples in China. These data highlight the importance of role played by S. Typhimurium in the dissemination of MDR genes. Although the mechanisms remain to be further described, the emergence of MDR genes particularly mcr-1, along with various virulence factors and heavy metal resistance genes, on the chromosome and plasmid from S. Typhimurium, will challenge therapeutic options for clinicians and others. Moreover, successful transmission of the mcr-1 gene poses a challenge to those with an interest in the protection of public health.
Availability of Data and Materials
The aggregate data supporting findings contained within this manuscript will be shared upon request submitted to the corresponding author.
Author Contributions
WW, ZB, SF, and FL designed the experiments and wrote the manuscript. MZ, YD, YH, ZP, and JX carried out the experiments. ZB, WW, and NY analyzed the experimental results.
Funding
This study was funded by the National Key R&D Program of China (2016YFD0401102) and China Food Safety Talent Competency Development Initiative: CFSA 523 Program.
Conflict of Interest Statement
The authors declare that the research was conducted in the absence of any commercial or financial relationships that could be construed as a potential conflict of interest.
The reviewer MS and handling Editor declared their shared affiliation.
Acknowledgments
We sincerely thank all the participants who took part in this study.
Supplementary Material
The Supplementary Material for this article can be found online at: https://www.frontiersin.org/articles/10.3389/fmicb.2018.00616/full#supplementary-material
FIGURE S1 | S1-PFGE indicating the transfer of single plasmid harboring mcr-1. Lane 1, Escherichia coli J53 (recipient); Lane 2, S. Typhimurium WW012 (donor); Lane 3, CT-E. coli J53(transconjugant); Lane 4, H-9812(PFGE marker strain, S.BraenderupH9812).
FIGURE S2 | A schematic representation of the complete chromosome (A) and plasmid (B) of S. Typhimurium WW012. Each ring, beginning from the inside to out represents the following features: GC content (black); GC skew (green and purple); CDS (blue); Antimicrobial resistance genes (shown in blue colored font), metal resistance genes (shown in green colored font), and virulence factor genes (shown in black colored font).
FIGURE S3 | Major structural features of plasmid pWW012 compared with several other plasmids identified from NCBI. (A) Major structural features of plasmid pWW012 compared with plasmids pHYEC7-mcr-1, pSCC4, and pHNSHP45-2 identified in E. coli and Citrobacter braakii, respectively. (B) Major structural features of plasmid pWW012 compared with plasmids pHNLDF400 and pHNSHP45-2 both identified in E. coli. ORFs are shown with arrows (blue arrows, transposase; red arrows, antibiotic resistance genes; green arrows, integrase; gray arrows, member protein; white arrows, hypothetical protein–see the key to the right hand side of the panel). The missing amino acids in IS30 comparing with ISApl1 were showed in red box.
TABLE S1 | Sampling information of Salmonella isolates in this study.
TABLE S2 | Virulence resistance-encoding genes identified in S. Typhimurium WW012 when comparing the genome of the latter against the current version of the VFDB database.
TABLE S3 | Metal resistance genes of S. Typhimurium WW012 with the current version of the BacMet database.
Footnotes
- ^ http://rast.nmpdr.org
- ^ http://blast.ncbi.nlm.nih.gov/Blast.cgi
- ^ http://www.ncbi.nlm.nih.gov/orffinder
- ^ https://cge.cbs.dtu.dk/services/PlasmidFinder/
- ^ https://card.mcmaster.ca/analyze
- ^ http://www.mgc.ac.cn/VFs/main.htm
- ^ http://cge.cbs.dtu.dk/services/SPIFinder
- ^ http://bacmet.biomedicine.gu.se/
References
Anjum, M. F., Duggett, N. A., AbuOun, M., Randall, L., Nunez-Garcia, J., Ellis, R. J., et al. (2016). Colistin resistance in Salmonella and Escherichia coli isolates from a pig farm in Great Britain. J. Antimicrob. Chemother. 71, 2306–2313. doi: 10.1093/jac/dkw149
Argudín, M. A., Lauzat, B., Kraushaar, B., Alba, P., Agerso, Y., Cavaco, L., et al. (2016). Heavy metal and biocidal agent resistance genes among livestock-associated methicillin-resistant Staphylococcus aureus isolates. Vet. Microbiol. 191, 88–95. doi: 10.1016/j.vetmic.2016.06.004
Campos, J., Cristino, L., Peixe, L., and Antunes, P. (2016). MCR-1 in multidrug-resistant and copper-tolerant clinically relevant Salmonella 1,4,[5],12:i:- and S. Rissen clones in Portugal, 2011 to 2015. Euro Surveill. 21. doi: 10.2807/1560-7917.ES.2016.21.26.30270
Carnevali, C., Morganti, M., Scaltriti, E., Bolzoni, L., Pongolini, S., and Casadei, G. (2016). Occurrence of mcr-1 in colistin-resistant Salmonella enterica isolates recovered from humans and animals in Italy, 2012 to 2015. Antimicrob. Agents Chemother. 60, 7532–7534. doi: 10.1128/AAC.01803-16
Clinical and Laboratory Standards Institute [CLSI] (2016). Performance Standards for Antimicrobial Susceptibility Testing; Twenty-Sixth Informational Supplement. CLSI Document M100-S26. Wayne, PA: Clinical and Laboratory Standards Institute.
Cui, M., Zhang, J., Gu, Z., Li, R., Chan, E. W., Yan, M., et al. (2017). Prevalence and molecular characterization of mcr-1-positive Salmonella strains recovered from clinical specimens in China. Antimicrob. Agents Chemother. 61, e02471–16. doi: 10.1128/AAC.02471-16
Deng, W., Quan, Y., Yang, S., Guo, L., Zhang, X., Liu, S., et al. (2017). Antibiotic resistance in Salmonella from retail foods of animal origin and its association with disinfectant and heavy metal resistance. Microb. Drug Resist. doi: 10.1089/mdr.2017.0127 [Epub ahead of print].
Doumith, M., Godbole, G., Ashton, P., Larkin, L., Dallman, T., Day, M., et al. (2016). Detection of the plasmid-mediated mcr-1 gene conferring colistin resistance in human and food isolates of Salmonella enterica and Escherichia coli in England and Wales. J. Antimicrob. Chemother. 71, 2300–2305. doi: 10.1093/jac/dkw093
El Garch, F., de Jong, A., Simjee, S., Moyaert, H., Klein, U., Ludwig, C., et al. (2016). Monitoring of antimicrobial susceptibility of respiratory tract pathogens isolated from diseased cattle and pigs across Europe, 2009–2012: VetPath results. Vet Microbiol. 194, 11–22. doi: 10.1016/j.vetmic.2016.04.009
El Garch, F., Sauget, M., Hocquet, D., LeChaudee, D., Woehrle, F., and Bertrand, X. (2017). mcr-1 is borne by highly diverse Escherichia coli isolates since 2004 in food-producing animals in Europe. Clin. Microbiol. Infect. 23, 51.e1–51.e4. doi: 10.1016/j.cmi.2016.08.033
Figueiredo, R., Card, R. M., Nunez, J., Pomba, C., Mendonca, N., Anjum, M. F., et al. (2016). Detection of an mcr-1-encoding plasmid mediating colistin resistance in Salmonella enterica from retail meat in Portugal. J. Antimicrob. Chemother. 71, 2338–2340. doi: 10.1093/jac/dkw240
Gallati, C., Stephan, R., Hächler, H., Malorny, B., Schroeter, A., and Nüesch-Inderbinen, M. (2013). Characterization of Salmonella enterica subsp. Enterica serovar 4,[5],12:i:- clones isolated from human and other sources in Switzerland between 2007 and 2011. Foodborne Pathog. Dis. 10, 549–554. doi: 10.1089/fpd.2012.1407
Hu, Y., Cheng, H., and Tao, S. (2017). Environmental and human health challenges of industrial livestock and poultry farming in China and their mitigation. Environ. Int. 107, 111–130. doi: 10.1016/j.envint.2017.s07.003
Kempf, I., Jouy, E., and Chauvin, C. (2016). Colistin use and colistin resistance in bacteria from animals. Int. J. Antimicrob. Agents 48, 598–606. doi: 10.1016/j.ijantimicag.2016.09.016
Kirk, M. D., Pires, S. M., Black, R. E., Caipo, M., Crump, J. A., Devleesschauwer, B., et al. (2015). World Health Organization estimates of the global and regional disease burden of 22 a foodborne bacterial, protozoal, and viral Diseases, 2010: a data synthesis. PLoS Med. 12:e1001921. doi: 10.1371/journal.pmed.1001921
Leclerc, J. M., Quevillon, E. L., Houde, Y., Paranjape, K., Dozois, C. M., and Daigle, F. (2016). Regulation and production of Tcf, a cable-like fimbriae from Salmonella enterica serovar Typhi. Microbiology 162, 777–788. doi: 10.1099/mic.0.000270
Lee, J. Y., Chung, E. S., Na, I. Y., Kim, H., Shin, D., and Ko, K. S. (2014). Development of colistin resistance in pmrA-, phoP-, parR- and cprR-inactivated mutants of Pseudomonas aeruginosa. J. Antimicrob. Chemother. 69, 2966–2971. doi: 10.1093/jac/dku238
Li, X. P., Fang, L. X., Song, J. Q., Xia, J., Huo, W., Fang, J. T., et al. (2016). Clonal spread of mcr-1 in PMQR-carrying ST34 Salmonella isolates from animals in China. Sci. Rep. 6:38511. doi: 10.1038/srep38511
Liu, Y. Y., Wang, Y., Walsh, T. R., Yi, L. X., Zhang, R., Spencer, J., et al. (2016). Emergence of plasmid-mediated colistin resistance mechanism MCR-1 in animals and human beings in China: a microbiological and molecular biological study. Lancet Infect. Dis. 16, 161–168. doi: 10.1016/S1473-3099(15)00424-7
Majowicz, S. E., Musto, J., Scallan, E., Angulo, F. J., Kirk, M., O’Brien, S. J., et al. (2010). The global burden of nontyphoidal Salmonella gastroenteritis. Clin. Infect. Dis. 50, 882–889. doi: 10.1086/650733
Malorny, B., Hoorfar, J., Hugas, M., Heuvelink, A., Fach, P., Ellerbroek, L., et al. (2003). Interlaboratory diagnostic accuracy of a Salmonella specific PCR-based method. Int. J. Food Microbiol. 89, 241–249. doi: 10.1016/S0168-1605(03)00154-5
McClelland, M., Sanderson, K. E., Spieth, J., Clifton, S. W., Latreille, P., Courtney, L., et al. (2001). Complete genome sequence of Salmonella enterica serovar Typhimurium LT2. Nature 413, 852–856. doi: 10.1038/35101614
Parkhill, J., Dougan, G., James, K. D., Thomson, N. R., Pickard, D., Wain, J., et al. (2001). Complete genome sequence of a multiple drug resistant Salmonella enterica serovar Typhi CT18. Nature 413, 848–852. doi: 10.1038/35101607
Peters, B., Stein, J., Klingl, S., Sander, N., Sandmann, A., Taccardi, N., et al. (2017). Structural and functional dissection reveals distinct roles of Ca2+-binding sites in the giant adhesin SiiE of Salmonella enterica. PLoS Pathog. 13:e1006418. doi: 10.1371/journal.ppat.1006418
Quesada, A., Ugarte-Ruiz, M., Iglesias, M. R., Porrero, M. C., Martinez, R., Florez-Cuadrado, D., et al. (2016). Detection of plasmid mediated colistin resistance (MCR-1) in Escherichia coli and Salmonella enterica isolated from poultry and swine in Spain. Res. Vet. Sci. 105, 134–135. doi: 10.1016/j.rvsc.2016.02.003
Sun, J., Ke, B., Huang, Y., He, D., Li, X., Liang, Z., et al. (2014). The molecular epidemiological characteristics and genetic diversity of Salmonella Typhimurium in Guangdong, China, 2007–2011. PLoS One 9:e113145. doi: 10.1371/journal.pone.0113145
Vinueza-Burgos, C., Cevallos, M., Ron-Garrido, L., Bertrand, S., and De Zutter, L. (2016). Prevalence and diversity of Salmonella serotypes in ecuadorian broilers at slaughter age. PLoS One 11:e0159567. doi: 10.1371/journal.pone.0159567
Wang, J., Zhi, C. P., Chen, X. J., Guo, Z. W., Liu, W. L., Luo, J., et al. (2017). Characterization of oqxAB in Escherichia coli isolates from animals, retail meat, and human patients in Guangzhou, China. Front. Microbiol. 8:1982. doi: 10.3389/fmicb.2017.01982
Wang, R. B., van Dorp, L., Shaw, L. P., Bradley, P., Wang, Q., Wang, X. J., et al. (2018). The global distribution and spread of the mobilized colistin resistance gene mcr-1. Nat. Commun. 9:1179. doi: 10.1038/s41467-018-03205-z
Wang, S., Duan, H., Zhang, W., and Li, J. W. (2007). Analysis of bacterial foodborne disease outbreaks in China between 1994 and 2005. FEMS Immunol. Med. Microbiol. 51, 8–13. doi: 10.1111/j.1574-695X.2007.00305.x
Wang, W., Liu, F., Peng, Z., Li, F., and Ma, A. (2016). Complete genome sequence of Salmonella enterica subsp. Enterica serovar Indiana C629, a carbapenem-resistant bacterium isolated from chicken carcass in China. Genome Announc. 4, e00662–16. doi: 10.1128/genomeA.00662-1
Weening, E. H., Barker, J. D., Laarakker, M. C., Humphries, A. D., Tsolis, R. M., and Bäumler, A. J. (2005). The Salmonella enterica serotype Typhimurium lpf, bcf, stb, stc, std, and sth fimbrial operons are required for intestinal persistence in mice. Infect. Immun. 73, 3358–3366. doi: 10.1128/IAI.73.6.3358-3366.2005
Wong, M. H., Yan, M., Chan, E. W., Biao, K., and Chen, S. (2014). Emergence of clinical Salmonella enterica serovar Typhimurium isolates with concurrent resistance to ciprofloxacin, ceftriaxone, and azithromycin. Antimicrob. Agents Chemother. 58, 3752–3756. doi: 10.1128/AAC.02770-13
Yang, Y. Q., Zhang, A. Y., Ma, S. Z., Kong, L. H., Li, Y. X., Liu, J. X., et al. (2016). Co-occurrence of mcr-1 and ESBL on a single plasmid in Salmonella enterica. J. Antimicrob. Chemother. 71, 2336–2338. doi: 10.1093/jac/dkw243
Yi, L., Wang, J., Gao, Y., Liu, Y., Doi, Y., Wu, R., et al. (2017). mcr-1–harboring Salmonella enterica serovar Typhimurium sequence type 34 in pigs, China. Emerg. Infect. Dis. 23, 291–295. doi: 10.3201/eid2302.161543
Zhang, J., Jin, H., Hu, J., Yuan, Z., Shi, W., Ran, L., et al. (2014). Serovars and antimicrobial resistance of non-typhoidal Salmonella from human patients in Shanghai, China, 2006-2010. Epidemiol. Infect. 142, 826–832. doi: 10.1017/S0950268813001659
Keywords: MDR Salmonella enteric serovar Typhimurium, conjugation, mcr-1, phoP/Q, pmrA/B, plasmids, ready-to-eat pork
Citation: Wang W, Baloch Z, Zou M, Dong Y, Peng Z, Hu Y, Xu J, Yasmeen N, Li F and Fanning S (2018) Complete Genomic Analysis of a Salmonella enterica Serovar Typhimurium Isolate Cultured From Ready-to-Eat Pork in China Carrying One Large Plasmid Containing mcr-1. Front. Microbiol. 9:616. doi: 10.3389/fmicb.2018.00616
Received: 12 December 2017; Accepted: 16 March 2018;
Published: 27 April 2018.
Edited by:
Giovanna Suzzi, Università di Teramo, ItalyReviewed by:
Haijian Zhou, Chinese Center for Disease Control and Prevention, ChinaRong Zhang, Second Affiliated Hospital of Zhejiang University School of Medicine, China
Maria Schirone, Università di Teramo, Italy
Copyright © 2018 Wang, Baloch, Zou, Dong, Peng, Hu, Xu, Yasmeen, Li and Fanning. This is an open-access article distributed under the terms of the Creative Commons Attribution License (CC BY). The use, distribution or reproduction in other forums is permitted, provided the original author(s) and the copyright owner are credited and that the original publication in this journal is cited, in accordance with accepted academic practice. No use, distribution or reproduction is permitted which does not comply with these terms.
*Correspondence: Fengqin Li, lifengqin@cfsa.net.cn Séamus Fanning, sfanning@ucd.ie
†These authors have contributed equally to this work as co-first authors.