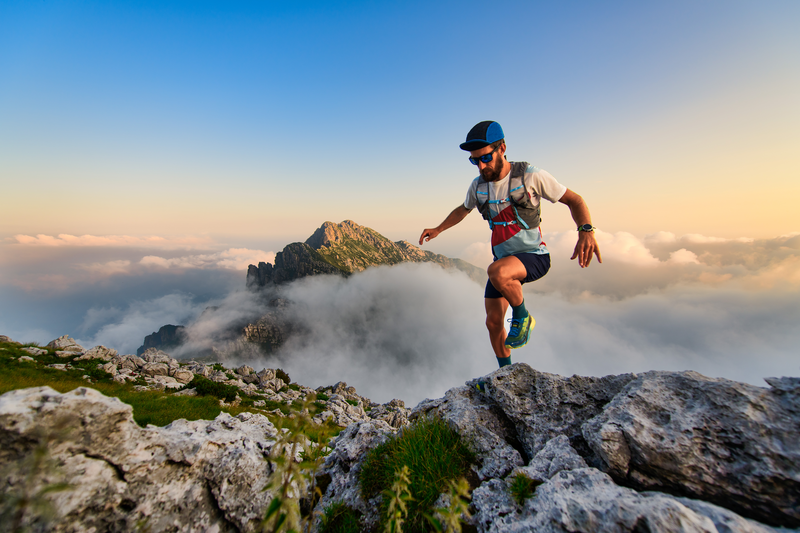
95% of researchers rate our articles as excellent or good
Learn more about the work of our research integrity team to safeguard the quality of each article we publish.
Find out more
ORIGINAL RESEARCH article
Front. Microbiol. , 04 April 2018
Sec. Fungi and Their Interactions
Volume 9 - 2018 | https://doi.org/10.3389/fmicb.2018.00612
Stanhopea tigrina is a Mexican endemic orchid reported as a threatened species. The naturally occurring microorganisms present in S. tigrina are unknown. In this work, we analyzed the diversity of endophytic and epiphytic culturable fungi in S. tigrina according to morphological and molecular identification. Using this combined approach, in this study we retrieved a total of 634 fungal isolates that presented filamentous growth, which were grouped in 134 morphotypes that were associated to 63 genera, showing that S. tigrina harbors a rich diversity of both endophytic and epiphytic fungi. Among these, the majority of the isolates corresponded to Ascomycetes, with Trichoderma and Penicillium as the most frequent genera followed by Fusarium and Aspergillus. Non-ascomycetes isolated were associated only to the genus Mucor (Mucoromycota) and Schizophyllum (Basidiomycota). Identified genera showed a differential distribution considering their epiphytic or endophytic origin, the tissue from which they were isolated, and the ability of the orchid to grow on different substrates. To our knowledge, this work constitutes the first study of the mycobiome of S. tigrina. Interestingly, 21 fungal isolates showed the ability to produce gibberellins. Almost half of the isolates were related to the gibberellin-producer genus Penicillium based on morphological and molecular identification. However, the rest of the isolates were related to the following genera, which have not been reported as gibberellin producers so far: Bionectria, Macrophoma, Nectria, Neopestalotiopsis, Talaromyces, Trichoderma, and Diplodia. Taken together, we found that S. tigrina possess a significant fungal diversity that could be a rich source of fungal metabolites with the potential to develop biotechnological approaches oriented to revert the threatened state of this orchid in the near future.
Fungi play a central role in most ecosystems and they have important functions in soil and plant habitats. It is now clear that the microbiota associated with plants contribute to maintain their biological diversity in terrestrial ecosystems through different biological processes (Coats and Rumpho, 2014; Hardoim et al., 2015). In this regard, the fungal population associated with plants contributes to the adaptation process in response to biotic and abiotic stress, it is involved in the defense against pathogens and it has been related to the production of secondary metabolites with antimicrobial activity or with the synthesis of different phyto-hormones including gibberellins (Tsavkelova et al., 2008; Hajiboland et al., 2010; Contreras-Cornejo et al., 2011; Khan et al., 2012, 2015b). Gibberellins play an essential role in regulation of growth and development of angiospermic plants (Bahalla et al., 2010), they are associated with several plant growth and development processes as seed germination, stem elongation, flowering, and fruit development (Bilkay et al., 2010; Lu et al., 2016).
Fungi can be found as epiphytes (on the surface of the plant) or as endophytes (inside the plant tissue). Both populations are growing apart only by millimeters nevertheless they differ greatly in their composition (Santamaría and Bayman, 2005). Epiphytes grow on the surface with contact to outer environment, whereas endophytes are in contact with the inner microenvironment. It is unclear to what extent plants control which epiphytes are able to enter the leaf, and to what extent endophytes may affect this process and the mycelial growth of fungi complicates the difference between both pupulations, while comparison of endophytic and epiphytic floras may help to determine the basis for selectivity. The coexistence of epiphytic and endophytic microorganisms contributes to maintain the conditions needed for plant health, plant protection (Andrews and Harris, 2000; Santamaría and Bayman, 2005; Rodriguez et al., 2009), and microbial biodiversity (Huang et al., 2008; Kharwar et al., 2010), allowing a global homeostatic state. For this reason, further knowledge of the fungal mycobiota residing in plants is decisive to understand fungal ecology and to generate approaches for plant conservation (Yuan et al., 2009).
Mexico possess a great diversity of orchids with around 1,260 species and 170 genera (Hágsater et al., 2005; Soto et al., 2007) from which 40% correspond to endemic species. Unfortunately, in spite of being one of the largest families of flowering plants of the planet, it is also of the most threatened. Different factors including pollution, climate change, and human activities (Rands et al., 2010) have had a negative impact on orchid diversity. In Mexico, 188 orchid species are categorized as endangered by the government (SEMARNAT, 2010). Stanhopea is a neotropical orchid genus consisting of approximately 40 species out of which 13 occur in Mexico. Although the species belonging to this genus are widespread through Mexico, Central, and South America, some of the species are endemic in Mexico. Stanhopea tigrina is an endemic orchid in Mexico that is considered threatened at the state-level. This orchid shows beautiful massive flowers (18 cm in diameter) with a sweet and penetrating aroma that makes them very attractive for marketing (Soto-Arenas and Solano-Gómez, 2007) and due to its Mexican endemic nature, it was chosen as the symbol of the Mexican Orchid Association. Concerns about the decline of S. tigrina populations in Puebla State and in Mexico require the development of efficient and ecologically viable strategies to preserve the species. Given the importance of the microbiome in the extension of the plants' ability to adapt to many kinds of environmental conditions and changes (Vandenkoornhuyse et al., 2015), it is important to identify the associated microbiome in order to develop strategies that allow its conservation. On the other hand, fungi isolated from orchids may serve as beneficial species that could be used in orchid propagation and reintroduction, improving acclimatization and vigor (Brundrett, 2007; Yuan et al., 2009). However, to our knowledge there are no reports dealing with the diversity of fungi living in the S. tigrina, one of the most spectacular orchids in Mexico.
In this work, we analyzed the fungal diversity of both epiphytic and endophytic fungi in the threatened Mexican endemic orchid S. tigrina (collected from Cuetzalan del Progreso, Puebla, México) according to morphological and molecular identification. Additionally, we examined the ability of the isolates to produce gibberellins and we uncovered several fungal genera as gibberellin producers. Our results indicate that S. tigrina harbors a rich diversity for both epiphytic and endophytic fungi that could be a source of metabolites with a positive effect on the development of this endangered species. With this work, we want to contribute to the knowledge of the filamentous fungal diversity associated to S. tigrina but also to provide some tools that could allow the development of alternative strategies that could help to revert the threatened state of this orchid.
Samples were taken from the Botanical Garden Xoxoctic (20°2′22.63″N, 97°30′32.16″W) located in the municipality of Cuetzalan del Progreso, Puebla, Mexico, which corresponds to a natural area devoted to preserve and investigate the biological richness of the Sierra Norte de Puebla. This location corresponds to a tropical mountain cloud forest ecosystem, to an altitude of 1000 masl, with semi-warm to warm humid climate and rainfall throughout the year (PIGEU, 2009). Considering the endangered status for this species, only six plants and one flower of S. tigrina were collected, from which one was lithophyte, two grew as epiphytes, and three were cultivated on a substrate that contained coconut fiber and soil.
For fungal isolation, we took 2 g of fresh and healthy tissue for each part of the plant (leaf, pseudobulb, root, or flower). The procedure was performed for the six plants independently. To isolate fungal epiphytes, weighed and washed in 100 ml of isotonic saline solution with constant stirring for 3 min. Then, tissues were removed and with the washing suspension we performed serial dilutions (10−1, 10−2, and 10−3) and 100 μl of each dilution were plated in triplicates in different media: Potato Dextrose Agar (PDA, MCD LAB), Malt Extract Agar medium (MEA, Difco), and V8 juice agar. The composition of these media is the following; PDA: potato infusion 20%, dextrose 2%, agar 1.5%; MEA: malt extract 1.2%, dextrin 0.2%, glycerol 02%, peptone 0.07%, agar 1.5%; V8 juice agar: V8 juice 200 ml/l, 3 g/l CaCO3, and 20 g/l agar. To recover fungal endophytes, tissues were rinsed with water and surface-sterilized in a sequence of 70% ethanol for 2 min followed by 1% NaClO2 during 2 min, and finally rinsed twice in sterile distilled water. Disinfected plant tissues were cut and macerated. The extract obtained was recovered and serial dilutions of 10−1, 10−2, and 10−3 were performed. 100 μl of each dilution was plated on PDA, MEA, and V8 juice agar; the plating was performed in triplicate. Rhizospheric fungi were also isolated by taking 1 g of sample which was placed in 100 ml of isotonic saline and the resulting suspension was used to perform serial dilutions of 10−1, 10−2, and 10−3; from each dilution, 100 μl were taken and plated on PDA, MEA, and V8 juice agar. All plates were incubated at 28°C for 1 month. Plates were checked daily and each emerging fungal colony was transferred onto a new PDA plate until axenic cultures were obtained.
Individual strains with apparent distinct morphology were sub-cultured until obtaining a pure isolate. In order to identify distinct and representative morphologies, isolates were plated on PDA and incubated for 1 week at 28°C. To induce sporulation or formation of distinctive structures that could be used to identify fungal genus, isolates were grown under microculture conditions. Briefly, a sterile PDA agar cube (1 cm) was cut and placed on a slide where the fungal isolate was inoculated and then a coverslip was placed on top. This system was deposited in a petri dish with humid chamber environment and incubated for 1 week at 28°C. After the incubation, the coverslip was removed and the fungal growth was stained with lactophenol cotton blue. Morphological characteristics of the isolates were challenged by the identification keys. These isolates were then classified into morphotypes according to the growth rate, color, texture, and morphology of their colonies. Non-sporulating fungal morphotypes were grown in different media such as malt extract agar (MEA), Czapek agar (CZ), oat agar (OA) among others, combined with different light periods to stimulate sporulation.
To further analyze the identity of the fungal isolates recovered, different samples of each morphotype were considered according to the number of members in each group. When the morphotype included 1–3 elements, we used only one isolate to perform the molecular identification. In the same fashion, we used 3 isolates for groups with 7–10 elements and 5 isolates for groups with more than 10 elements. For these fungi, DNA was extracted as previously reported (Liu et al., 2000). Fresh mycelium was scraped from the surface of the agar plate and transferred into a clean tube with 500 μl of lysis buffer (400 mM Tris-HCl [pH 8.0], 60 mM EDTA [pH 8.0], 150 mM NaCl, 1% sodium dodecyl sulfate) and the mixture was vortexed in the presence of glass beads. After 30 min, 150 μl of precipitation solution (3 M potassium acetate, 5 M acetate, pH 4.8) were added to the tube, vortexed briefly and centrifuged at 10,000 rpm for 5 min. The supernatant was recovered and centrifuged again. Precipitation was performed with an equal volume of isopropyl alcohol at −80°C for 20 min with centrifugation at 10,000 rpm for 5 min. DNA pellet was washed with 300 μl of 70% ethanol. After centrifugation at 10,000 rpm for 5 min, the supernatant was discarded and the DNA pellet was dissolved in 40 μl of water.
Fungal ITS-region and partial LSU were amplified using primers ITS1F (Gardes and Bruns, 1993) and LR5 (Hopple and Vilgalys, 1994; Moncalvo et al., 2000), which are specific for fungi. The resulting PCR products ranged from 1.3 to 1.7 kb in size. The LSU region is useful to identify fungi in public databases. PCR reactions were performed with 2 μl of genomic DNA in a total volume of 25 μl, using Taq DNA polymerase with ThermoPol buffer, according to the manufacturer's instructions (New England BioLabs) and further purified with the High Pure PCR purification kit (Roche). The following thermocycling program was used: 95°C for 5 min (1 cycle); 94°C for 35 s, 55°C 35 s, 72°C for 2 min (35 cycles); and 72°C for 10 min (1 cycle). Amplification products were sequenced using primers ITS1F and LR5 using the services provided by Macrogen (Korea). Two sequencing rounds were performed in order to confirm the genetic features. Sequences obtained in this study were annotated in the GenBank (Supplementary Material).
Forward and reverse sequence reads were analyzed using the CLC genomics workbench (QIAGEN). BLAST searches (www.ncbi.nlm.nih.gov/BLAST) were conducted to determine the close known relative for each case. Isolates were assigned as closest to a certain genus when identity was ≥95% (Sánchez et al., 2008; Chen et al., 2012; Tan et al., 2012). The evolutionary history was inferred by using the Maximum Likelihood Phylogeny 1.2 method with the Jukes Cantor nucleotide substitution model. The analysis involved 45 nucleotide sequences with a total of 100 replicates in the bootstrap analysis. Evolutionary analyses were conducted in CLC Main Workbench 7.9.1.
Pure fungal isolates were incubated in 5 ml of ICI medium, a medium with a low concentration of nitrogen that allows the production of gibberellins, for 10 days at 30°C under dark conditions and agitation (200 rpm). After incubation, 0.2 ml of culture media were recovered and combined with 0.2 ml of ethanol (96%, v/v) and 2 ml of a cold mixture of sulfuric acid/ethanol (1:1 v/v). This mixture was incubated at 48°C for 30 min (Candau et al., 1992) and then exposed to an UV lamp. Samples showing green fluorescence were considered positive for gibberellin production.
Seven-day old mycelium of each strain was inoculated in a flask with 100 ml of Czapek broth (1% glucose, 1% peptone) and incubated at 30°C in the presence of light (as described by Bahalla et al., 2010). After 10 days, the culture was filtered and 50 ml of the filtrate was recovered and the pH adjusted to 3 by adding HCl (1N). The extraction was performed three times using ethyl acetate (1:1). The organic phase was separated and passed through anhydrous sodium sulfate. The solvent was eliminated in a rotary evaporator at 40°C and 10 rpm. The residue was dissolved in 50 μl of HPLC grade methanol and used for reverse phase HPLC analysis. The analysis was done in triplicate in a Hewlett Packard HPLC instrument (series 1100) with operation and data analysis using Chemstation software running on a HP Vectra 486 computer (Hewlett Packard Co.). Gibberellins were chromatographed using LiChrospher on a RP-18 column (250 × 4 mm i.d.). Acetonitrile and acidic water (0.01% H3PO4) in a 70:30 ratio was used as mobile phase with a flow rate of 0.6 ml/min for 15 min. One microliter of each sample was injected and gibberellins were identified at 206 nm (Barendse et al., 1980; Bahalla et al., 2010). Gibberellin quantification was performed as previously reported (Bahalla et al., 2010). Full spectra were obtained for commercial GA3 and GA4 (1 μg/μl, Sigma) that were used as standard.
In this work, a total of 891 isolates were recovered from six plants of S. tigrina collected from the botanical garden Xoxoctic in Puebla, Mexico (Figure 1). Out of the 891 fungal isolates recovered from the six S. tigrina plants, 257 isolates did not show filamentous characteristics, but were confirmed as yeast according to Gram and Lactophenol Cotton Blue staining. Moreover, their ability to produce gibberellins was also tested but any of the yeast showed the ability to produce this metabolite under the conditions assayed (data not shown).
Figure 1. Location map of the study site. Sampling was performed in the Botanical Garden Xoxoctic (Cuetzalan del Progreso, Puebla, México), a natural area located to the Sierra Norte (Puebla) devoted to preserve species characteristic of this region.
For the 634 fungal isolates that presented filamentous growth, morphological identification was carried out according to colony and hyphal morphology of the macroscopic culture and characteristics of the spores under microculture conditions. According to these characteristics, the 634 fungal isolates were classified into 139 distinct morphotypes, according to the morphological characteristics observed (Supplementary Table 1). Insights into the distribution and diversity of the fungal isolates recovered from S. tigrina is further presented.
In addition to the morphological characterization, molecular analyses were carried out to confirm the identity of the different morphogroups (Wang et al., 2005; Jin et al., 2013). Using this combinatory approach, we identified 134 morphotypes that comprised 63 genera, while 10 isolates grouped in 5 morphotypes could not be identified.
From the total culturable population recovered, 80% of the isolates were epiphytes, while 20% of them were endophytes. Considering tissue distribution, the greatest number of isolates were recovered from pseudobulb (270 isolates), followed by the leaf and root with 226 and 197 isolates, respectively. A total of 153 isolates were found in the rhizosphere and 45 in the flower (Figure 2). In general, the proportion of epiphytes than endophytes isolated was similar for the different tissues studied. Surprisingly, the flower resulted a very rich tissue if we keep in mind that we only recovered one flower during the sampling process. Considering that the flowers of this orchids only last a few days, the appearance of the flower at the sampling time was timely.
Figure 2. Abundance of fungal isolates in different S. tigrina tissues. The number of endophytic (orange), epiphytic (green), and rhizospheric (gray) fungal isolates recovered from different tissues is shown.
Concerning the genera identified, a total of 63 genera was established, being Trichoderma the most recurrent group identified showing 124 isolates, while the second most frequently recovered was Penicillium with 103 isolates (Figure 3). These were the dominant genera retrieved from S. tigrina, followed by Fusarium and Aspergillus with 40 and 33 isolates, respectively. The infrequent groups that presented only one isolate included Alternaria, Cladophialophora, Cochliobolus, Colletotrichum, Fusicolla, Gibberella, Hypocrea, Phialemonium, Phoma, Pseudobotrytis, Schizophyllum, and Talaromyces. Considering the provenance of the isolate, we found that the epiphytic population comprised 57 genera, while 32 genera constituted the endophytic population. On the other hand, we identified 33 genera in the rhizospheric community (Figure 3). Even when a total of 25 genera were found both in the endophytic and epiphytic populations, we also noticed differences in the composition of the two groups. In this regard, 32 genera were found only in the epiphytic population, while the following fungal taxa were recovered only as endophytes: Alternaria, Annulohypoxylon, Anthostomella, Colletotrichum, Letendraea, and Phaeosphaeriopsis (Figure 3). Interestingly, Trichoderma was frequently isolated both as epiphyte and from the rhizosphere.
Figure 3. Tissue distribution of the fungal genera isolated from S. tigrina. Epiphytic (A) and endophytic (B) isolates were recovered from the leaf, pseudobulb, root, and flower of S. tigrina and the relative frequency for the different genera isolated is shown. Genera recovered from the rhizosphere are also shown (C).
We next analyzed the genera distribution for the different plants (Figure 4) and we found that plant 4, which was grown on substratum showed the highest number of associated epiphytic genera, while plant three that grew as litophyte presented the lowest number of fungal genera isolated (Figure 4A). Concerning the endophytic community, plant 1 showed the greatest diversity of genera (Figure 4B).
Figure 4. Distribution of culturable fungi in different S. tigrina plants. The relative isolation frequency was estimated for the epiphytic (A) and endophytic (B) isolates that were recovered from each of the six different plants. Plants 1 and 2 grew as epiphytes, plant 3 was growing as litophyte, while plants 4, 5, and 6 were growing on substratum.
In this study, we recovered fungal isolates from the following tissues in S. tigrina: leaf, pseudobulb, root, and flower. In the roots, the presence of mycorrhizal fungi was sought, however, the presence of pelotons was not observed and no isolates belonging to this type of fungi was obtained. The composition and abundance of the isolated genera varied according to the host tissues investigated. The tissue with the greatest number of associated genera was the leaf with 40, followed by pseudobulb with 32 and 27 from the root, while only 10 isolates were recovered from the flower (Figure 2). For all the tissues studied, epiphytes were retrieved from the surface, while endophytes were obtained from macerated disinfected tissue. Fungal isolates were also recovered from the associated rhizosphere. Out of the 45 genera recovered from the leaf, 12 were found in both the endophytic and epiphytic populations, while 30 genera were recovered only as epiphytes. In the case of the pseudobulb, we found 11 genera in both populations, whereas 19 genera were associated to the epiphyte population and five genera were exclusively endophytic. In the root, 12 genera were ubiquitous while 10 and 8 genera were found only as epiphytic or endophytic, respectively. Finally, in the flower we identified 9 epiphytic genera and only Paecilomyces was encountered as endophyte (Table 2).
Regarding tissue distribution of epiphytes, the leaf was the tissue with the greatest diversity with 38 genera associated, followed 29 in pseudobulb, 21 in root and only 9 in flower, while 33 genera were recovered from the rhizosphere (Figure 3). The most frequent fungal genera were Trichoderma, Penicillium, Fusarium, Mucor, and Aspergillus, which were found in all tissues (Figure 3A). However, we found tissue specificity for some genera. This was the case for Cochliobolus, Diplodia, Elaphocordyceps, Eutypa, Fusicolla, Gibberella, Hypocrea, Nigrograna, Phoma, Thyridaria that were recovered only from the leaf. On the other hand, Phialemonium, Pseudobotrytis, Roussoella, and Umbelopsis were found only in pseudobulb while Bionectria, Cladophialophora, Microsporum, Phialemoniopsis, Pyrenochaeta, and Talaromyces seemed exclusive from rhizosphere.
On the other hand, for the endophytic population we found a more balanced abundance of fungal isolates. In this case, out of the 32 genera identified, 20 were found in the root, 16 in pseudobulb, 15 in leaves and only one in flower (Table 1). Regarding the identity of the isolated genera, only Paecilomyces was identified in all tissues. Consistent with our observations of the epiphytic population, we discovered the following tissue-specificity: Chaetomium, Eutypella, Geotrichum, Hypoxylon, Mucor, Pestalotiopsis, Phaeosphaeriopsis were identified only in the leaf, Alternaria, Bionectria, Daldinia, Macrophoma, Phialemonium, and Pyrenochaeta were found only in the root, while Cladosporium, Colletotrichum, Letendraea, Nectria, and Phialemoniopsis were retrieved only from the pseudobulb (Figure 3B).
Considering the ability of S. tigrina to grow in different substrates, in this study we recovered fungal isolates from one lithophyte plant, two epiphyte specimens and three orchids that grew on substrate. When we compared the abundance of genera according to these growth conditions, we realized that there were 65 genera recovered from plants that grew as epiphytes and 58 from orchids grown on substrate. The similar abundance of genera observed in orchids grown as epiphytes or on the substrate was noticed for both the epiphytic and endophytic fungal populations, albeit their composition was different. On the other hand, we recovered only 28 genera from the lithophyte orchid. Insights into the special composition of the epiphytic and endophytic fungal populations discovered are further presented (Figure 5).
Figure 5. Fungal community composition according to the different growth habits of S. tigrina. Isolation frequency of genera growing as epiphytes, as litophytes, or in substratum are presented for the epiphytic (A) and endophytic (B) fungal communities. The number of genera shared by plants with different growth habits is shown for the epiphytic (C) and endophytic (D) fungal communities.
Considering the endophytic fungal population, all shared a core of four genera: Acremonium, Arthrinium, Penicillium, and Trichoderma. The genera shared between the epiphyte orchids and those grown on substrate were Aspergillus, Daldina, Diplodia, Fonsecae, Fusarium, Paecilomyces, and Scedosporium. Orchids grown on substrate shared the genera Annulohypoxylon, Anthostomella, and Chaunopycnis with the lithophyte plant, while the epiphyte and lithophyte specimens did not show any common genus. In addition, some genera seemed to be exclusive from a particular environment (Table 2). This was the case for Macrophoma and Pyrenochaeta present only in the litophyte plant. Regarding the epiphytic fungal population, all orchids shared a core of 13 genera: Acremonium, Arthrinium, Aspergillus, Cladosporium, Fonsecae, Fusarium, Geotrichum, Mucor, Paecilomyces, Penicillium, Scedosporium, Trichoderma, and Verticillium. It was also observed that the substrate and epiphyte plants shared 12 genera: Anthostomella, Arthopyrenia, Chaunopycnis Curvularia, Daldinia, Diaporthe, Macrophoma, Microdiplodia, Ochlorocladosporium, Paraconiothyrium Pestalotiopsis, and Roussoella. Epiphytic and litophyte plants shared the genus Bipolaris, Exophiala, Trichophyton, and Pyrenochaetopsis. Besides, in the substrate plants were found Aplosporella, Bionectria, Chaetomium, Diplodia, Elaphocordyceps, Epichloe, Hypocrea, Nectria, Neopestalotiopsis, Nigrograna, Phialemoniopsis, Phoma, Pseudobotrytis, Pyrenochaeta, and Umbelopsis as non-shared genera, we found nine genera associated only with epiphytic plants, and Giberella in lithophytes (Table 2). On the other hand, Eutypella was found only in epiphyte habit plants, both in the endophytic and epiphytic populations whereas Nectria, Phialemoniopsis, and Phialemonium were found only in plants grown on substrate.
As part of our ongoing efforts toward finding novel alternatives to revert the threatened state of S. tigrina, we investigated the ability of these fungal isolates to produce secondary metabolites. It is also important to identify these potentially bioactive fungi for a better understanding of the characteristics of the relevant fungal community associated to the orchid. In the search of fungal isolates from S. tigrina that could have a positive impact on plant development, we performed a general screening to identify gibberellin-producing isolates using a qualitative method described previously (Candau et al., 1992). Using this approach, we tested all the 891 isolates and found that 21 of them were positive for gibberellin production using the qualitative test. For these 21 isolates, the production of gibberellins was quantitatively determined by HPLC, using commercial GA3 (Sigma G7645) and GA4 (Sigma G7276) as standards (Supplementary Material). This analysis showed the presence of GA3 in the 21 isolates, being STF062 the isolate with the highest production of GA3 (4.1 μg), followed by STF624 that produced 2.0 μg of GA3. Other gibberellin producers were isolates STF282, STF524, STF526, STF538, STF599, STF637, STF657, STF756, and STF757, all with gibberellin production around 1 μg (Table 3). Even when the assay was designed to identify both GA3 and GA4, in most isolates only the production of GA3 was detected and the production of both gibberellins was observed only for isolate STF723, with a predominant production of GA4 (2.1 μg) over GA3 (0.1 μg).
In addition to the morphological characterization, molecular analyses were carried out to confirm the identity of the 21 isolates recovered from S. tigrina that were determined as gibberellin producers and the results are included in Table 4. The ITS1-5.8S-ITS2 sequences of these isolates were compared to the sequences annotated in the GenBank and using this approach, we found that isolates STF62, STF147, STF149, STF538, STF591, STF599, STF654, STF723, and STF859 were associated to various reported sequences for the genus Penicillium. Isolates STF664, STF673, and STF860 showed the highest similarity with the Xylariales order. The ITS sequence for the isolate STF526 did not show significant homology to any known cultured fungal species. The other isolates were associated to the following fungal species: Arthrinium marii (STF757), Bionectria cf Ochroleuca (STF624), Macrophoma (STF282), Nectria pseudotrichia (STF753), Neopestalotiopsis sp. (STF524), Talaromyces funiculosus (STF343), Trichoderma sp. (STF864), and Diplodia quercivora (STF756).
The sequences used for the molecular identification were handled in order to construct the phylogenetic tree shown in Figure 6. The phylogenetic analysis of the sequences divided the strains into two main clades. The first clade comprising 12 isolates includes two classes: Eurotiales and Botryosphaeriales. The Eurotiales clade contains several well-supported branches and represents basically two genera: Penicillium and Talaromyces. The largest group shows a bootstrap support of 96% and includes different known strains for the genus Penicillium. Isolates STF062, STF654, STF599, STF859, and STF599 were all grouped with Penicillium oxalicum and in a different branch STF723 was also associated to this group. Isolate STF149 and Penicillium charlesii formed a group with a bootstrap of 86%. In a different clade, isolate STF538 was grouped with Penicillium pinophilum, while isolate STF343 clustered together with Talaromyces funiculosus with a bootstrap support of 100%. Phylogenetic analysis suggests that isolates STF062, STF149, STF538, STF591, STF599, STF654, STF723, and STF859 belong to the genus Fusarium, while STF343 belong to the genus Talaromyces. The Botryosphaeriales clade was supported for a bootstrap of 100% and groups Macrophoma theicola with the isolate STF282 in one clade and isolate STF756 with Diplodia quercivora in a separate clade (Figure 6), associating the isolates with the corresponding genus.
Figure 6. Phylogenetic relationship among the 21 fungal isolates that showed the ability to produce gibberellin. The tree with the highest log likelihood (−8844.44) is shown. The tree is drawn to scale, with branch lengths measured in the number of substitutions per site. The analysis involved 45 nucleotide sequences with a total of 100 replicates in the bootstrap analysis.
Within the Xylariales clade, STF864 formed a group with Trichoderma asperellum and STF624 clustered together with Bionectria cf. ochroleuca, both with a bootstrap support of 100%. STF664 and STF673 were grouped with Xylaria cf. heliscus with a bootstrap value of 93%, while STF860 was grouped within the Xylariaceae family. Therefore, phylogenetic analysis suggests that STF864 belong to the genus Trichoderma, STF624 relates to the genus Bionectria, and STF664, STF673 match the genus Xylaria, while STF860 associates with the Xylariaceae family.
The final clade groups two isolates and two genera corresponding to Hypocreales. On one hand, we have isolate STF524 grouped with Neopestalotiopsis sp. with a strong bootstrap of 100% and in separate clades STF757 releted to Arthrinium sp. with a bootstrap value of 87%. Given that all the isolates identified as gibberellin producers, four sequences for reported basidiomycetes were used as outgroup.
In this study we identified 63 genera, 30 of which have already been reported as orchid endophytes (McCormick et al., 2004; Albores et al., 2005; Chen et al., 2011; Jiang et al., 2011; Sudheep and Sridhar, 2012; Sawmya et al., 2013; Tao et al., 2013). Some of these endophytes have shown beneficial effects, like the antibacterial activity of Alternaria sp. and Fusarium oxysporum recovered from Brazilian orchids (Vaz et al., 2009) or the ability of Aspergillus flavus, A. ochraceus, and Paecilomyces sp. to protect the orchids Bulbophyllum neilgherrense and Vanda testacea from herbivores (Sudheep and Sridhar, 2012).
Interestingly, we report here for the first time several genera as fungal partners for orchids, including the following: Aplosporella, Microsporum, Mucor, Trichophyton, Diplodia, Fonsecae, and Scedosporium, indicating that the composition of the fungal communities associated to different orchid species should be further analyzed. Even when these genera correspond to endophytes, pathogens, or latent pathogens in other hosts (Meletiadis et al., 2002; Becker, 2003; Burgess et al., 2004; Prusky et al., 2004; Cortez et al., 2008; Lazzizera et al., 2008; Leelasuphakul et al., 2008; Slippers et al., 2009; Taylor et al., 2009; Twizeyimana et al., 2013; Dreaden et al., 2014; Dou et al., 2017), their biotechnological potential as secondary metabolite producers (Malonek et al., 2005; Bi et al., 2013) should also be considered when designing future applications to rescue this endangered orchid. In this regard, fungal isolates reported here could have a strong impact on the health of S. tigrina by maintaining a balance in the composition of the associated microbiome (Omacini et al., 2001), serving as a defense against different pathogens or helping to deal with biotic stress (Brundrett, 2006). Thus, a better knowledge of the fungal community associated with S. tigrina could help to preserve endangered species like this orchid from further destruction.
In this work, a total of 891 fungal isolates was recovered using a culture-dependent approach to identify both epiphytes and endophytes, revealing the richness of the fungal community present in S. tigrina plants. This is the first report about culturable fungi isolated from this endangered orchid. Endophytic fungi have been reported as mutualists that could show entomopathogenic activity or produce biologically active secondary metabolites (Stone et al., 2000). In orchids, endophytic fungi could exhibit strong antibacterial or antifungal activities as well as plant-growth promoting effect (Chen et al., 2010; Xing et al., 2011). These findings support the notion that endophytic fungi isolated from S. tigrina could have potentially diverse applications. On the other hand, it has been shown that epiphytic fungi are determinant for plant health, plant protection (Andrews and Harris, 2000; Santamaría and Bayman, 2005; Rodriguez et al., 2009), and microbial biodiversity maintenance (Huang et al., 2008; Kharwar et al., 2010). However, the relationship between fungal epiphytes and orchids is practically non-existent. In this study, we obtained a higher number of epiphytic isolates than endophytes in all tissues investigated. This behavior has been reported for other plants including Coffea arabica leaves and Eucaluptus citriodora (Santamaría and Bayman, 2005; Osono, 2008; Kharwar et al., 2010). It would be relevant to examine both the endophytic and epiphytic fungal populations associated with orchids to fully uncover the ecological potential associated to these plants as a valuable source of biotechnological applications.
Current studies of orchid-associated fungi are mainly focused on mycorrhizae (Kristiansen et al., 2001; Yamato et al., 2005; Huang et al., 2014; Oliveira et al., 2014) and endophytic fungi associated with roots and leaves (Chen et al., 2010; Chutima et al., 2011; Tan et al., 2012), leaving aside the study of fungi present in other tissues. In this work, we examined both the endophytic and epiphytic fungal populations in the orchid to fully consider the great microbiological richness associated to this plant. We found that the leaf was the tissue with the greatest diversity of epiphytes, while the root exhibited the highest diversity of endophytic genera, in accordance with the significant microbial interactions that occur in these plant tissues and with previous reports (Vendramin et al., 2010; Sudheep and Sridhar, 2012; Bunch et al., 2013; Chen et al., 2013). However, we also found that other tissues like the pseudobulb showed a considerable diversity for both epiphytic and endophytic isolates, supporting the notion that other tissues should also be considered in this type of studies. Moreover, this capability of S. tigrina to relate with many fungal partners could represent a biological and survival advantage for the orchid (Otero et al., 2004).
Concerning genera distribution that conform the epiphytic or endophytic communities isolated from this orchid, we found Trichoderma and Fusarium as the most prevalent genera for the epiphytic and endophytic communities, respectively. It has been reported that Trichoderma produces enzymes involved in cell plant degradation and could be applied for biocontrol (Naher et al., 2014; Schmoll et al., 2016), while Fusarium produces secondary metabolites such as gibberellins (O'Donnell et al., 1998; Malonek et al., 2005) and protect plants from pathogenic isolates (Minerdi et al., 2011). Besides these prevalent fungi, we identified 25 genera as part of both the epiphytic and endophytic communities in S. tigrina, where the most widely distributed genera were Paecilomyces, Penicillium, and Scedosporium. These fungal isolates could be applied to benefit the orchid by inducing growth promotion through hormone secretion, by generating plant resistance due to the activation of multiple defense signals or they could also show antagonistic activity against pathogens, as reported for other hosts (Kiewnick and Sikora, 2006; Hossain et al., 2007; Khan et al., 2011a; Radhakrishnan et al., 2013).
Even when several genera showed ubiquitous distribution, some appeared to have a preference. This was the case for Alternaria, Annulohypoxylon, Colletotrichum, and Phaeosphaeriopsis which were recovered only as part of the endophytic community and their natural ability to inhabit the orchid could be advantageous for future biotechnological applications. For example, Annulohypoxylon (previously referred as Hypoxylon) presents an endophytic stage as part of its life cycle and produces several secondary metabolites with antibiotic and antiparasitic activities (Piettre et al., 2002; Bills et al., 2012). On the other hand, we found that 31 genera were exclusively epiphytic, which could be related mainly to the general richness observed for this community.
It has been reported that GA3 has the ability to alter orchid seedling morphology by elongating seedlings (Hadley and Harvais, 1968; Ávila-Díaz et al., 2009) and GAs are known to promote cell elongation and division in various plant tissues (Potter et al., 1993; Bianco et al., 1996; Ozga et al., 2002; Kazmierczak, 2003; Little and MacDonald, 2003; Bilkay et al., 2010). Orchid embryos typically form globular structures, expanding outward in all directions and germination could conceivably be enhanced by the action of phytohormones like gibberellins. Here, we identified several gibberellin-producing isolates in suitable yields according to concentrations that have shown biological activity in different plants (Hamayun et al., 2009, 2010b; Khan et al., 2011c; Waqas et al., 2012), suggesting that these isolates could be useful to improve propagation, adaptation, and conservation of S. tigrina, particularly at early stageS. When identified, these isolates were associated to 11 genera that comprise Penicillium and Arthrinium, which have already been reported as gibberellin-producers (Mitter et al., 2002; Kawaide, 2006; Khan et al., 2009; Bahalla et al., 2010; Leitão and Enguita, 2016) with the ability to enhance plant growth and to help the plants to resist abiotic stresses (Hamayun et al., 2010a; Khan et al., 2011b, 2015a).
Gibberellins play a relevant role in plant growth and development. However, many fungi that associate with plants or soil have not been tested for their ability to produce GAs. Here, we found that isolates with the ability to produce gibberellins displayed a high degree of similarity with the following genera, according to the fungal ITS regions: Bionectria, Diplodia, Macrophoma, Nectria, Neopestalotiopsis, Talaromyces, and Trichoderma. Even when we found a high similarity when identifying the isolates, further investigations will help to fully characterize the identity of these isolates and their ability to produce gibberellins and other useful metabolites. Finally, the physiological effects of this metabolite in orchids, particularly in S. tigrina remains to be elucidated.
Overall, this study shows novel information about the composition of the mycobiome present in S. tigrina, an endangered species of the rich endemic Mexican ecosystem. As found for other orchids, S. tigrina shows a complex fungal community that includes diverse groups, suggesting the probable relevance of its biodiversity in maintaining equilibrium with its environment. Moreover, interesting insights related to the composition of the epiphytic and endophytic communities and their distribution in the plant showed a great fungal richness for this orchid. Finally, we believe that the description of the natural mycobiome associated to S. tigrina and the capability of some of the isolates to produce secondary metabolites could be applied in different biotechnological strategies oriented to increase the population of S. tigrina in Mexico. This integrated approach could benefit delicate members of ecosystems, particularly for endangered species. Similar studies could be conducted to gain insights into the fungal microbiome associated to other plants, in order to identify microbial symbionts that may play a role in providing stability for the host in their particular ecosystem.
Overall approach of this study was designed by NM-M and RM-C. SS-C conducted the experiments. MC-L designed and supervised HPLC experiments. All authors were involved in experiment design, data analysis, and manuscript preparation.
The authors declare that the research was conducted in the absence of any commercial or financial relationships that could be construed as a potential conflict of interest.
S. tigrina plants were identified by the Biologist Raúl Álvarez Mora (Xoxoctic Botanical Garden). PCR reactions were partially performed in the Functional Genomics Laboratory, at the University of Sherbrooke (Sherbrooke, Quebec, Canada) with the support of Dr. Benoit Chabot. SS-C received a PhD fellowship from CONACYT.
The Supplementary Material for this article can be found online at: https://www.frontiersin.org/articles/10.3389/fmicb.2018.00612/full#supplementary-material
Albores, V., Adriano, L., and Salvador, M. (2005). Isolation of endophytic fungi and their mycorrhizal potential for the tropical epiphytic orchids Cattleya skinneri, C. aurantiaca and Brassa vola nodosa. Asian J. Plant Sci. 4, 309–315. doi: 10.3923/ajps.2005.309.315
Andrews, J. H., and Harris, R. F. (2000). The ecology and biogeography of microorganisms on plant surfaces. Annu. Rev. Phytopathol. 38, 145–180. doi: 10.1146/annurev.phyto.38.1.145
Ávila-Díaz, I., Oyama, K., Gómez-Alonso, C., and Salgado-Garciglia, R. (2009). In vitro propagation of the endangered orchid Laelia speciosa. Plant Cell Tissue Organ Cult. 99, 335. doi: 10.1007/s11240-009-9609-8
Bahalla, K., Singh, S. B., and Agarwal, R. (2010). Quantitative determination of gibberellins by high performance liquid chromatography from various gibberellins producing Fusarium strains. Environ. Monit. Assess. 167, 515–520. doi: 10.1007/s10661-009-1068-5
Barendse, G. W. M., Van de Werken, P. H., and Takahashi, N. (1980). High-performance liquid chromatography of gibberellins. J. Chromatogr. A 198, 449–455. doi: 10.1016/S0021-9673(00)80514-2
Becker, W. F. (2003). Nectria pseudotrichia, como agente causal de cancro de ramos, ocorrendo em pereira japonesa no Brasil. Fitopatol. Bras. 28, 107. doi: 10.1590/S0100-41582003000100018
Bi, J., Ji, Y., Pan, J., Yu, Y., Chen, H., and Zhu, X. (2013). A new taxol-producing fungus (Pestalotiopsis malicola) and evidence for taxol as a transient product in the culture. Afr. J. Biotechnol. 10, 6647–6654. doi: 10.5897/AJB11.170
Bianco, J., Daymond, J., and LePage-Degivry, M. T. (1996). Regulation of germination and seedling root growth by manipulations of embryo GA levels in sunflower. Acta Physiol. Plan 18, 59–66.
Bilkay, I. S., Karakoç, S., and Aksöz, N. (2010). Indole-3-acetic acid and gibberellic acid production in aspergillus niger. Turkish J. Biol. 34, 313–318. doi: 10.3906/biy-0812-15
Bills, G. F., González-Menéndez, V., Martín, J., Platas, G., Fournier, J., Peršoh, D., et al. (2012). Hypoxylon pulicicidum sp. nov.(Ascomycota, Xylariales), a pantropical insecticide-producing endophyte. PLoS ONE 7:e46687. doi: 10.1371/journal.pone.0046687
Brundrett, M. C. (2006). “Understanding the roles of multifunctional mycorrhizal and endophytic fungi,” in Microbial Root Endophytes, eds B. J. E. Schulz, C. J. C. Boyle, and T. N. Sieber (Berlin; Heidelberg: Springer), 281–298.
Brundrett, M. C. (2007). Scientific approaches to Australian temperate terrestrial orchid conservation. Aust. J. Bot. 55, 293–307. doi: 10.1071/BT06131
Bunch, W. D., Cowden, C. C., Wurzburger, N., and Shefferson, R. P. (2013). Geography and soil chemistry drive the distribution of fungal associations in lady's slipper orchid, Cypripedium acaule. Botany 91, 850–856. doi: 10.1139/cjb-2013-0079
Burgess, T. I., Gordon, T. R., Wingfield, M. J., and Wingfield, B. D. (2004). Geographic isolation of Diplodia scrobiculata and its association with native Pinus radiata. Mycol. Res. 108, 1399–1406. doi: 10.1017/S0953756204001443
Candau, R., Avalos, J., and Cerda-Olmedo, E. (1992). Regulation of gibberellin biosynthesis in Gibberella fujikuroi. Plant Physiol. 100, 1184–1188. doi: 10.1104/pp.100.3.1184
Chen, J., Hu, K. X., Hou, X. Q., and Guo, S. X. (2011). Endophytic fungi assemblages from 10 Dendrobium medicinal plants (Orchidaceae). World J. Microbiol. Biotechnol. 27, 1009–1016. doi: 10.1007/s11274-010-0544-y
Chen, J., Wang, H., and Guo, S. X. (2012). Isolation and identification of endophytic and mycorrhizal fungi from seeds and roots of Dendrobium (Orchidaceae). Mycorrhiza 22, 297–307. doi: 10.1007/s00572-011-0404-0
Chen, J., Zhang, L. C., Xing, Y. M., Wang, Y. Q., Xing, X. K., Zhang, D. W., et al. (2013). Diversity and taxonomy of endophytic xylariaceous fungi from medicinal plants of Dendrobium (Orchidaceae). PLoS ONE 8:e58268. doi: 10.1371/journal.pone.0058268
Chen, X. M., Dong, H. L., Hu, K. X., Sun, Z. R., Chen, J., and Guo, S. X. (2010). Diversity and antimicrobial and plant-growth-promoting activities of endophytic fungi in Dendrobium loddigesii Rolfe. J. Plant Growth Regul. 29, 328–337. doi: 10.1007/s00344-010-9139-y
Chutima, R., Dell, B., Vessabutr, S., Bussaban, B., and Lumyong, S. (2011). Endophytic fungi from Pecteilis susannae (L.) Rafin (Orchidaceae), a threatened terrestrial orchid in Thailand. Mycorrhiza 21, 221–229. doi: 10.1007/s00572-010-0327-1
Coats, V. C., and Rumpho, M. E. (2014). The rhizosphere microbiota of plant invaders: an overview of recent advances in the microbiomics of invasive plants. Front. Microbiol. 5:368. doi: 10.3389/fmicb.2014.00368
Contreras-Cornejo, H. A., Macías-Rodríguez, L., Beltrán-Pe-a, E., Herrera-Estrella, A., and López-Bucio, J. (2011). Trichoderma-induced plant immunity likely involves both hormonal-and camalexin-dependent mechanisms in Arabidopsis thaliana and confers resistance against necrotrophic fungi Botrytis cinerea. Plant Signal. Behav. 6, 1554–1563. doi: 10.4161/psb.6.10.17443
Cortez, K. J., Roilides, E., Quiroz-Telles, F., Meletiadis, J., Antachopoulos, C., Knudsen, T., et al. (2008). Infections caused by Scedosporium spp. Clin. Microbiol. Rev. 21, 157–197. doi: 10.1128/CMR.00039-07
Dou, Z. P., Lu, M., Wu, J. R., He, W., and Zhang, Y. (2017). A new species and interesting records of Aplosporella from China. Sydowia 69, 1–7. doi: 10.12905/0380.sydowia69-2017-0001
Dreaden, T. J., Black, A. W., Mullerin, S., and Smith, J. A. (2014). First report of Diplodia quercivora causing shoot dieback and branch cankers on live oak (Quercus virginiana) in the United States. J. Nat. Prod. 77, 2352–2360. doi: 10.1094/PDIS-07-13-0736-PDN
Gardes, M., and Bruns, T. D. (1993). ITS primers with enhanced specificity for basidiomycetes-application to the identification of mycorrhizae and rusts. Mol. Ecol. 2, 113–118. doi: 10.1111/j.1365-294X.1993.tb00005.x
Hadley, G., and Harvais, G. (1968). The effect of certain growth substances on asymbiotic germination and development of Orchis purpurella. New Phytol. 67, 441–445. doi: 10.1111/j.1469-8137.1968.tb06393.x
Hágsater, E., Soto-Arenas, M. A., Salazar, C. G. A., Jiménez, M. R., López, R. M. A., and Dressler, R. L. (2005). Las Orquídeas de México: Orquídeas y gente del Instituto Chinoín. México: Productos Farmaceuticos, 38–71.
Hajiboland, R., Aliasgharzadeh, N., Laiegh, S. F., and Poschenrieder, C. (2010). Colonization with arbuscular mycorrhizal fungi improves salinity tolerance of tomato (Solanum lycopersicum L.) plants. Plant Soil 331, 313–327. doi: 10.1007/s11104-009-0255-z
Hamayun, M., Khan, S. A., Iqbal, I., Ahmad, B., and Lee, I. J. (2010a). Isolation of a gibberellin-producing fungus (Penicillium sp. MH7) and growth promotion of Crown daisy (Chrysanthemum coronarium). J. Microbiol. Biotechnol. 20, 202–207. doi: 10.4014/jmb.0905.05040
Hamayun, M., Khan, S. A., Khan, A. L., Rehman, G., Kim, Y. H., Iqbal, I., et al. (2010b). Gibberellin production and plant growth promotion from pure cultures of Cladosporium sp. MH-6 isolated from cucumber (Cucumis sativus L.). Mycologia 102, 989–995. doi: 10.3852/09-261
Hamayun, M., Khan, S. A., Khan, A. L., Rehman, G., Sohn, E. Y., Shah, A. A., et al. (2009). Phoma herbarum as a new gibberellin-producing and plant growth-promoting fungus. J. Microbiol. Biotechnol. 19, 1244–1249. doi: 10.4014/jmb.0901.0030
Hardoim, P. R., Van Overbeek, L. S., Berg, G., Pirttilä, A. M., Compant, S., Campisano, A., et al. (2015). The hidden world within plants: ecological and evolutionary considerations for defining functioning of microbial endophytes. Microbiol. Mol. Biol. Rev. 79, 293–320. doi: 10.1128/MMBR.00050-14
Hopple, J. S. Jr., and Vilgalys, R. (1994). Phylogenetic relationships among coprinoid taxa and allies based on data from restriction site mapping of nuclear rDNA. Mycologia 86, 96–107.
Hossain, M. M., Sultana, F., Kubota, M., Koyama, H., and Hyakumachi, M. (2007). The plant growth-promoting fungus Penicillium simplicissimum GP17-2 induces resistance in Arabidopsis thaliana by activation of multiple defense signals. Plant Cell Physiol. 48, 1724–1736. doi: 10.1093/pcp/pcm144
Huang, C. L., Jian, F. Y., Huang, H. J., Chang, W. C., Wu, W. L., Hwang, C. C., et al. (2014). Deciphering mycorrhizal fungi in cultivated Phalaenopsis microbiome with next-generation sequencing of multiple barcodes. Fungal Divers.66, 77–88. doi: 10.1007/s13225-014-0281-x
Huang, W. Y., Cai, Y. Z., Hyde, K. D., Corke, H., and Sun, M. (2008). Biodiversity of endophytic fungi associated with 29 traditional Chinese medicinal plants. Fungal Divers. 33, 61–75.
Jiang, W., Yang, G., Zhang, C., and Fu, C. (2011). Species composition and molecular analysis of symbiotic fungi in roots of Changnienia amoena (Orchidaceae). Afr. J. Microbiol. Res. 5, 222–228. doi: 10.5897/AJMR10.479
Jin, H., Yan, Z., Liu, Q., Yang, X., Chen, J., and Qin, B. (2013). Diversity and dynamics of fungal endophytes in leaves, stems and roots of Stellera chamaejasme L. in northwestern China. Antonie van Leeuwenhoek 104, 949–963. doi: 10.1007/s10482-013-0014-2
Kawaide, H. (2006). Biochemical and molecular analyses of gibberellin biosynthesis in fungi. Biosci. Biotechnol. Biochem. 70, 583–590. doi: 10.1271/bbb.70.583
Kazmierczak, A. (2003). Ethylene is a positive regulator for GA 3-induced male sex in Anemia phyllitidis gametophytes. Plant Cell Rep. 22, 295–302. doi: 10.1007/s00299-003-0680-z
Khan, A. L., Hamayun, M., Ahmad, N., Hussain, J., Kang, S. M., Kim, Y. H., et al. (2011a). Salinity stress resistance offered by endophytic fungal interaction between Penicillium minioluteum LHL09 and Glycine max. L. J. Microbiol. Biotechnol. 21, 893–902. doi: 10.4014/jmb.1103.03012
Khan, A. L., Hamayun, M., Kang, S. M., Kim, Y. H., Jung, H. Y., Lee, J. H., et al. (2012). Endophytic fungal association via gibberellins and indole acetic acid can improve plant growth under abiotic stress: an example of Paecilomyces formosus LHL10. BMC Microbiol. 12:3. doi: 10.1186/1471-2180-12-3
Khan, A. L., Hamayun, M., Kim, Y. H., Kang, S. M., and Lee, I. J. (2011b). Ameliorative symbiosis of endophyte (Penicillium funiculosum LHL06) under salt stress elevated plant growth of Glycine max L. Plant Physiol. Biochem. 49, 852–861. doi: 10.1016/j.plaphy.2011.03.005
Khan, A. L., Hamayun, M., Kim, Y. H., Kang, S. M., Lee, J. H., and Lee, I. J. (2011c). Gibberellins producing endophytic Aspergillus fumigatus sp. LH02 influenced endogenous phytohormonal levels, isoflavonoids production and plant growth in salinity stress. Process. Biochem. 46, 440–447. doi: 10.1016/j.procbio.2010.09.013
Khan, A. L., Hussain, J., Al-Harrasi, A., Al-Rawahi, A., and Lee, I. J. (2015a). Endophytic fungi: resource for gibberellins and crop abiotic stress resistance. Crit. Rev. Biotechnol. 35, 62–74. doi: 10.3109/07388551.2013.800018
Khan, A. L., Waqas, M., and Lee, I. J. (2015b). Resilience of Penicillium resedanum LK6 and exogenous gibberellin in improving Capsicum annuum growth under abiotic stresses. J. Plant Res. 28, 259–268. doi: 10.1007/s10265-014-0688-1
Khan, S. A., Hamayun, M., Kim, H. Y., Yoon, H. J., Seo, J. C., Choo, Y. S., et al. (2009). A new strain of Arthrinium phaeospermum isolated from Carex kobomugi Ohwi is capable of gibberellin production. Biotechnol. Lett. 31, 283–287. doi: 10.1007/s10529-008-9862-7
Kharwar, R. N., Gond, S. K., Kumar, A., and Mishra, A. (2010). A comparative study of endophytic and epiphytic fungal association with leaf of Eucalyptus citriodora Hook., and their antimicrobial activity. World J. Microbiol. Biotechnol. 26, 1941–1948. doi: 10.1007/s11274-010-0374-y
Kiewnick, S., and Sikora, R. A. (2006). Biological control of the root-knot nematode Meloidogyne incognita by Paecilomyces lilacinus strain 251. Biol. Control 38, 179–187. doi: 10.1016/j.biocontrol.2005.12.006
Kristiansen, K. A., Taylor, D. L., Kjoller, R., Rasmussen, H. N., and Rosendahl, S. (2001). Identification of mycorrhizal fungi from single pelotons of Dactylorhiza majalis (Orchidaceae) using single-strand conformation polymorphism and mitochondrial ribosomal large subunit DNA sequences. Mol. Ecol. 10, 2089–2093. doi: 10.1046/j.0962-1083.2001.01324.x
Lazzizera, C., Frisullo, S., Alves, A., Lopes, J., and Phillips, A. J. L. (2008). Phylogeny and morphology of Diplodia species on olives in southern Italy and description of Diplodia olivarum sp. nov. Fungal Divers. 31, 63–71. doi: 10.1111/j.1365-3059.2008.01842.x
Leelasuphakul, W., Hemmanee, P., and Chuenchitt, S. (2008). Growth inhibitory properties of Bacillus subtilis strains and their metabolites against the green mold pathogen (Penicillium digitatum Sacc.) of citrus fruit. Postharvest Biol. Technol. 48, 113–121. doi: 10.1016/j.postharvbio.2007.09.024
Leitão, A. L., and Enguita, F. J. (2016). Gibberellins in Penicillium strains: challenges for endophyte-plant host interactions under salinity stress. Microbiol. Res. 183, 8–18. doi: 10.1016/j.micres.2015.11.004
Little, C. H. A., and MacDonald, J. E. (2003). Effects of exogenous gibberellin and auxin on shoot elongation and vegetative bud development in seedlings of Pinus sylvestris and Picea glauca. Tree Physiol. 23, 73–83. doi: 10.1093/treephys/23.2.73
Liu, D., Coloe, S., Baird, R., and Pedersen, J. (2000). Rapid mini-preparation of fungal DNA for PCR. J. Clin. Microbiol. 38, 471–471.
Lu, Y. H., Cao, Y. M., Guo, X. F., Wang, H., and Zhang, H. S. (2016). Determination of gibberellins using HPLC coupled with fluorescence detection. Anal. Methods 8, 1520–1526. doi: 10.1039/C5AY03133H
Malonek, S., Bömke, C., Bornberg-Bauer, E., Rojas, M. C., Hedden, P., Hopkins, P., et al. (2005). Distribution of gibberellin biosynthetic genes and gibberellin production in the Gibberella fujikuroi species complex. Phytochemistry 66, 1296–1311. doi: 10.1016/j.phytochem.2005.04.012
McCormick, M. K., Whigham, D. F., and O'Neill, J. (2004). Mycorrhizal diversity in photosynthetic terrestrial orchids. New Phytol. 163, 425–438. doi: 10.1111/j.1469-8137.2004.01114.x
Meletiadis, J., Meis, J. F., Mouton, J. W., Rodriquez-Tudela, J. L., Donnelly, J. P., Verweij, P. E., et al. (2002). In vitro activities of new and conventional antifungal agents against clinical Scedosporium isolates. Antimicrobial Agents Chemother. 46, 62–68. doi: 10.1128/AAC.46.1.62-68.2002
Minerdi, D., Bossi, S., Maffei, M. E., Gullino, M. L., and Garibaldi, A. (2011). Fusarium oxysporum and its bacterial consortium promote lettuce growth and expansin A5 gene expression through microbial volatile organic compound (MVOC) emission. FEMS Microbiol. Ecol. 76, 342–351. doi: 10.1111/j.1574-6941.2011.01051.x
Mitter, N., Srivastava, A. C., Ahamad, S., Sarbhoy, A. K., and Agarwal, D. K. (2002). Characterization of gibberellin producing strains of Fusarium moniliforme based on DNA polymorphism. Mycopathologia 153, 187–193. doi: 10.1023/A:1014946217539
Moncalvo, J. M., Lutzoni, F. M., Rehner, S. A., Johnson, J., and Vilgalys, R. (2000). Phylogenetic relationships of agaric fungi based on nuclear large subunit ribosomal DNA sequences. Syst. Biol. 49, 278–305. doi: 10.1093/sysbio/49.2.278
Naher, L., Yusuf, U. K., Ismail, A., and Hossain, K. (2014). Trichoderma spp.: a biocontrol agent for sustainable management of plant diseases. Pak. J. Bot. 46, 1489–1493.
O'Donnell, K., Cigelnik, E., and Nirenberg, H. I. (1998). Molecular systematics and phylogeography of the Gibberella fujikuroi species complex. Mycologia 90, 465–493.
Oliveira, S. F., Bocayuva, M. F., Veloso, T. G. R., Bazzolli, D. M. S., da Silva, C. C., Pereira, O. L., et al. (2014). Endophytic and mycorrhizal fungi associated with roots of endangered native orchids from the Atlantic Forest, Brazil. Mycorrhiza 24, 55–64. doi: 10.1007/s00572-013-0512-0
Omacini, M., Chaneton, E. J., Ghersa, C. M., and Müller, C. B. (2001). Symbiotic fungal endophytes control insect host–parasite interaction webs. Nature 409, 78–81. doi: 10.1038/35051070
Osono, T. (2008). Endophytic and epiphytic phyllosphere fungi of Camellia japonica: seasonal and leaf age-dependent variations. Mycologia 100, 387–391. doi: 10.3852/07-110R1
Otero, J. T., Ackerman, J. D., and Bayman, P. (2004). Differences in mycorrhizal preferences between two tropical orchids. Mol. Ecol. 13, 2393–2404. doi: 10.1111/j.1365-294X.2004.02223.x
Ozga, J. A., van Huizen, R., and Reinecke, D. M. (2002). Hormone and seed-specific regulation of pea fruit growth. Plant Physiol. 128, 1379–1389. doi: 10.1104/pp.010800
Piettre, A., Chevenier, E., Massardier, C., Gimbert, Y., and Greene, A. E. (2002). Synthetic approach to hypoxyxylerone, novel inhibitor of topoisomerase I. Org. Lett. 4, 3139–3142. doi: 10.1021/ol026454d
PIGEU (2009) Prontuario de Información Geográfica de los Estados Unidos Mexicanos. Cuetzalan del Progreso, Puebla. Clave Geoestadística 21043. Instituto Nacional de Estadística y Geografía, México
Potter, T. I., Zanewich, K. P., and Rood, S. B. (1993). Gibberellin physiology of safflower: endogenous gibberellins and response to gibberellic acid. Plant Growth Regul. 12, 133–140. doi: 10.1007/BF00144594
Prusky, D., McEvoy, J. L., Saftner, R., Conway, W. S., and Jones, R. (2004). Relationship between host acidification and virulence of Penicillium spp. on apple and citrus fruit. Phytopathology 94, 44–51. doi: 10.1094/PHYTO.2004.94.1.44
Radhakrishnan, R., Shim, K. B., Lee, B. W., Hwang, C. D., Pae, S. B., Park, C. H., et al. (2013). IAA-producing Penicillium sp. NICS01 triggers plant growth and suppresses Fusarium sp.-induced oxidative stress in sesame (Sesamum indicum L.). J. Microbiol. Biotechnol. 23, 856–863. doi: 10.4014/jmb.1209.09045
Rands, M. R., Adams, W. M., Bennun, L., Butchart, S. H., Clements, A., Coomes, D., et al. (2010). Biodiversity conservation: challenges beyond 2010. Science 329, 1298–1303. doi: 10.1126/science.1189138
Rodriguez, R. J., White, J. F. Jr., Arnold, A. E., and Redman, R. S. (2009). Fungal endophytes: diversity and functional roles. New Phytol. 182, 314–330. doi: 10.1111/j.1469-8137.2009.02773.x
Sánchez, M. S., Bills, G. F., and Zabalgogeazcoa, I. (2008). Diversity and structure of the fungal endophytic assemblages from two sympatric coastal grasses. Fungal Divers. 33, 87–100.
Santamaría, J., and Bayman, P. (2005). Fungal epiphytes and endophytes of coffee leaves (Coffea arabica). Microb. Ecol. 50, 1–8. doi: 10.1007/s00248-004-0002-1
Sawmya, K., Vasudevan, T. G., and Murali, T. S. (2013). Fungal endophytes from two orchid species pointer towards organ specificity. Czech Mycol. 65, 89–101.
Schmoll, M., Dattenböck, C., Carreras-Villase-or, N., Mendoza-Mendoza, A., Tisch, D., Alemán, M. I., et al. (2016). The genomes of three uneven siblings: footprints of the lifestyles of three Trichoderma species. Microbiol. Mol. Biol. Rev. 80, 205–327. doi: 10.1128/MMBR.00040-15
SEMARNAT (2010). NOM-059-SEMARNAT-2010: Protección Ambiental – Especies Nativas de México de Flora y Fauna Silvestres – Categorías de Riesgo y Especificaciones Para su Inclusión, Exclusión o Cambio – Lista de Especies en Riesgo. México: Secretaría de Medio Ambiente y Recursos Naturales.
Slippers, B., Burgess, T., Pavlic, D., Ahumada, R., Maleme, H., Mohali, S., et al. (2009). A diverse assemblage of Botryosphaeriaceae infect Eucalyptus in native and non-native environments. South. For. 71, 101–110. doi: 10.2989/SF.2009.71.2.3.818
Soto, M. A., Hágsater, E., Jiménez, R., Salazar, G. A., Solano, R., Flores, R., et al. (2007). Las Orquídeas de México: Catálogo digital. México: Instituto Chinoin, A.C.
Soto-Arenas, M. A., and Solano-Gómez, A. R. (2007). “Ficha técnica de Stanhopea tigrina,” in Información Actualizada Sobre las Especies de Orquídeas del PROY-NOM-059-ECOL-2000, ed M. A. Soto-Arenas (compilador) (México: Instituto Chinoin A.C., Herbario de la Asociación Mexicana de Orquideología A.C. Bases de datos SNIB-CONABIO), 1–9.
Stone, J. K., Bacon, C. W., and White, J. F. (2000). An overview of endophytic microbes: endophytism defined. Microb. Endophytes 3, 29–33.
Sudheep, N. M., and Sridhar, K. R. (2012). Non-mycorrhizal fungal endophytes in two orchids of Kaiga forest (Western Ghats), India. J. For. Res. 23, 453–460. doi: 10.1007/s11676-012-0284-y
Tan, X. M., Chen, X. M., Wang, C. L., Jin, X. H., Cui, J. L., Chen, J., et al. (2012). Isolation and identification of endophytic fungi in roots of nine Holcoglossum plants (Orchidaceae) collected from Yunnan, Guangxi, and Hainan provinces of China. Curr. Microbiol. 64, 140–147. doi: 10.1007/s00284-011-0045-8
Tao, G., Liu, Z. Y., Liu, F., Gao, Y. H., and Cai, L. (2013). Endophytic Colletotrichum species from Bletilla ochracea (Orchidaceae), with descriptions of seven new speices. Fungal Divers. 61, 139–164. doi: 10.1007/s13225-013-0254-5
Taylor, K., Barber, P. A., Hardy, G. E. S. J., and Burgess, T. I. (2009). Botryosphaeriaceae from tuart (Eucalyptus gomphocephala) woodland, including descriptions of four new specieS. Mycol. Res. 113, 337–353. doi: 10.1016/j.mycres.2008.11.010
Tsavkelova, E. A., Bömke, C., Netrusov, A. I., Weiner, J., and Tudzynski, B. (2008). Production of gibberellic acids by an orchid-associated Fusarium proliferatum strain. Fungal Genet. Biol. 45, 1393–1403. doi: 10.1016/j.fgb.2008.07.011
Twizeyimana, M., Förster, H., McDonald, V., Wang, D. H., Adaskaveg, J. E., and Eskalen, A. (2013). Identification and pathogenicity of fungal pathogens associated with stem-end rot of avocado in California. Plant Dis. 97, 1580–1584 doi: 10.1094/PDIS-03-13-0230-RE
Vandenkoornhuyse, P., Quaiser, A., Duhamel, M., Le Van, A., and Dufresne, A. (2015). The importance of the microbiome of the plant holobiont. New Phytol. 206, 1196–1206. doi: 10.1111/nph.13312
Vaz, A. B., Mota, R. C., Bomfim, M. R. Q., Vieira, M. L., Zani, C. L., Rosa, C. A., et al. (2009). Antimicrobial activity of endophytic fungi associated with Orchidaceae in Brazil. Can. J. Microbiol. 55, 1381–1391. doi: 10.1139/W09-101
Vendramin, E., Gastaldo, A., Tondello, A., Baldan, B., Villani, M., and Squartini, A. (2010). Identification of two fungal endophytes associated with the endangered orchid Orchis militaris L. J. Microbiol. Biotechnol. 20, 630–636. doi: 10.4014/jmb.0909.09023
Wang, Y. U., Guo, L. D., and Hyde, K. D. (2005). Taxonomic placement of sterile morphotypes of endophytic fungi from Pinus tabulaeformis (Pinaceae) in northeast China based on rDNA sequences. Fungal Divers. 20, 235–260.
Waqas, M., Khan, A. L., Kamran, M., Hamayun, M., Kang, S. M., Kim, Y. H., et al. (2012). Endophytic fungi produce gibberellins and indoleacetic acid and promotes host-plant growth during stress. Molecules 17, 10754–10773. doi: 10.3390/molecules170910754
Xing, Y. M., Chen, J., Cui, J. L., Chen, X. M., and Guo, S. X. (2011). Antimicrobial activity and biodiversity of endophytic fungi in Dendrobiumdevonianum and Dendrobium thyrsiflorum from Vietman. Curr. Microbiol. 62, 1218–1224. doi: 10.1007/s00284-010-9848-2
Yamato, M., Iwase, K., Yagame, T., and Suzuki, A. (2005). Isolation and identification of mycorrhizal fungi associating with an achlorophyllous plant, Epipogium roseum (Orchidaceae). Mycoscience 46, 73–77. doi: 10.1007/S10267-004-0218-4
Keywords: fungal diversity, orchid, Stanhopea tigrina, endemic, endophytes, epiphytes, gibberellins
Citation: Salazar-Cerezo S, Martinez-Montiel N, Cruz-Lopez MC and Martinez-Contreras RD (2018) Fungal Diversity and Community Composition of Culturable Fungi in Stanhopea trigrina Cast Gibberellin Producers. Front. Microbiol. 9:612. doi: 10.3389/fmicb.2018.00612
Received: 13 December 2017; Accepted: 16 March 2018;
Published: 04 April 2018.
Edited by:
Mohamed Hijri, Université de Montréal, CanadaReviewed by:
Giovanna Cristina Varese, Università degli Studi di Torino, ItalyCopyright © 2018 Salazar-Cerezo, Martinez-Montiel, Cruz-Lopez and Martinez-Contreras. This is an open-access article distributed under the terms of the Creative Commons Attribution License (CC BY). The use, distribution or reproduction in other forums is permitted, provided the original author(s) and the copyright owner are credited and that the original publication in this journal is cited, in accordance with accepted academic practice. No use, distribution or reproduction is permitted which does not comply with these terms.
*Correspondence: Rebeca D. Martinez-Contreras, cmViZWNhLm1hcnRpbmV6QGNvcnJlby5idWFwLm14
†Present Address: Nancy Martínez-Montiel, Département de Microbiologie et d'Infectiologie, Faculté de Médecine et des Sciences de la Santé, Université de Sherbrooke, Sherbrooke, QC, Canada
Disclaimer: All claims expressed in this article are solely those of the authors and do not necessarily represent those of their affiliated organizations, or those of the publisher, the editors and the reviewers. Any product that may be evaluated in this article or claim that may be made by its manufacturer is not guaranteed or endorsed by the publisher.
Research integrity at Frontiers
Learn more about the work of our research integrity team to safeguard the quality of each article we publish.