- 1School of Environmental and Biological Engineering, Nanjing University of Science and Technology, Nanjing, China
- 2State Key Laboratory of Marine Resource Utilization in South China Sea, Key Laboratory of Tropical Biological Resources of Ministry of Education, Hainan University, Haikou, China
- 3Inspection and Pattern Evaluation Department, Suzhou Institute of Measurement and Testing, Suzhou, China
- 4State Key Laboratory of Pharmaceutical Biotechnology, Nanjing University, Nanjing, China
Camellia nitidissima Chi, known as a medicinal and edible plant in China, exhibits multiple bioactivities, especially antibacterial activity. In this study, we investigated the inhibitory effects of the dichloromethane fraction (DF) of C. nitidissima Chi flowers on the pyocyanin production, swarming motility, and swimming motility of Pseudomonas aeruginosa PAO1, at sub-minimum inhibitory concentrations. Results showed that the DF had a remarkable inhibitory effect on pyocyanin production without influencing P. aeruginosa PAO1 growth, and concentration-dependent inhibitory effects on swarming and swimming motility. The half maximal inhibitory concentrations (IC50) were 0.158 ± 0.009, 0.139 ± 0.004, and 0.334 ± 0.049 mg/mL for pyocyanin production, swarming motility, and swimming motility, respectively. Real-time RT-PCR showed that the DF significantly down-regulated the expressions of lasR (p < 0.05) and rhlR (p < 0.01). In addition, gallic acid, catechin, ellagic acid, chlorogenic acid, quercetin, and kaempferol were identified in the DF by HPLC Triple TOF MS/MS analysis. All six identified compounds showed inhibitory effects on pyocyanin production, swarming motility, and swimming motility, though ellagic acid showed the strongest effects, with IC50 values of 0.067 ± 0.002, 0.024 ± 0.008, and 0.020 ± 0.003 mg/mL, respectively. Thus, the inhibitory effects on P. aeruginosa PAO1 virulence factors might be attributable to these six and/or other compounds in the DF of C. nitidissima Chi flowers. Consequently, the C. nitidissima Chi flower, especially the DF, might be a potential quorum sensing inhibitor of P. aeruginosa PAO1.
Introduction
Pseudomonas aeruginosa, which thrives in soil and water, is an opportunistic pathogen of plants, animals, and humans (Parsek and Greenberg, 2000). It is one of the main factors responsible for nosocomial infections in humans, with a high incidence of infection occurring in immunocompromised patients, such as those who are intubated, have prosthetic devices, bear severe burns, or suffer from cystic fibrosis (Castillo-Juárez et al., 2015; Castillo-Juarez et al., 2017). Unfortunately, P. aeruginosa can cause infections that are difficult to treat due to its increasing resistance to antibiotics and the formation of biofilms on abiotic and biotic surfaces (Costerton, 2001; Drenkard, 2003; Schuster and Greenberg, 2006). The virulence factors of P. aeruginosa, such as pyocyanin, rhamnolipid, protease, elastase, and alginate, are complex multifactorial phenomena, which are influenced by the environment and quorum sensing (QS) (Castillo-Juarez et al., 2017). QS is a form of cell-to-cell communication, which monitors bacterial population density and several physiological processes (Schauder and Bassler, 2001; Sheng et al., 2015). Small signals called autoinducers are released and received in the QS system, and are most frequently N-acyl-homoserine lactones in Gram-negative bacteria or peptides in Gram-positive bacteria (Kalia, 2013). QS systems regulate multiple bacterial functions, such as virulence gene expression, swarming motility, and swimming motility (Kalia, 2013; Wang et al., 2013). P. aeruginosa has three QS systems, las, rhl, and pqs (Zhou et al., 2017). In the las system, the lasI gene encodes the signal synthase LasI, which produces the autoinducer N-3-oxo-dodecanoyl-homoserine lactone (3-oxo-C12-HSL), and the lasR gene encodes the signal receptor LasR, with the binding of LasI and LasR then activating other genes, including alkaline protease and elastase B (Gambello and Iglewski, 1991; Castillo-Juarez et al., 2017). In the rhl system, the rhlI gene encodes the enzyme RhlI, and the rhlR gene encodes the signal receptor RhlR, with the binding of RhlI and RhlR then producing and sensing the autoinducer N-butanoyl-homoserine lactone (C4-HSL) to regulate the expression of virulence genes (Ochsner et al., 1994; Castillo-Juarez et al., 2017). In the pqs system, the autoinducer 2-heptyl-3-hydroxy-4(1H)-quinolone is known as the Pseudomonas quinolone signal (PQS). The three systems are intertwined in the QS hierarchy. The LasR initiates the QS regulatory systems and partially activates the transcription of RhlR and other regulators of the Pseudomonas quinolone signal and integrated QS systems (Maisuria et al., 2016).
Recently, inhibition of the QS system has been considered as a novel strategy for the development of antipathogenic agents, especially for combating bacterial infections caused by antibiotic-resistant strains (Rasko and Sperandio, 2010). Quorum sensing inhibitors (QSIs) can reduce virulence factors but do not kill bacteria, thus avoiding the development of the resistance observed for antibiotics (Hentzer and Givskov, 2003). These inhibitors include non-functional A-HSL analogs, such as brominated furanones, which can bind to receptors of A-HSLs competitively (Hentzer et al., 2003), and synthetic non-functional homoserine lactones, which can interfere with binding between autoinducers and their receptors (Reverchon et al., 2002). In addition, the inactivation of A-HSL receptors via covalent modification can also inhibit QS (Castillo-Juarez et al., 2017). Recently, the development of QSIs from natural biological material, especially medicinal and edible plants such as garlic, pea seedlings, pomegranate extract, and R. rugosa tea extract, has gained increasing attention (Puupponen-Pimia et al., 2005; Choo et al., 2006; Truchado et al., 2012; Zhang et al., 2014). As an important and widely distributed constituent of plants, phenolic compounds possess many important physiological functions and have recently been reported to reduce QS-controlled phenotypes in bacteria (Zhang et al., 2014; Ouyang et al., 2016; Skogman et al., 2016). In addition, bacterial motility plays an important role in the pathogenidty. P. aeruginosa has at least three types of motilities, including swarming, swimming, and twitching (Wang et al., 2014). So it is also a strategy for the development of antipathogenic agents to investigate the motility inhibitors. And it is reported that many compounds and extracts, such as zingerone (Kumar et al., 2015), 2,5-piperazinedione (Musthafa et al., 2012), macrolides (Kawamurasato et al., 2000), atorvastatin, rosuvastatin (Dhaliwal, 2015), and Agaricus Blazei hot water extract (Sokovic et al., 2014), are regarded as motility inhibitors to inhibit the bacterial motility.
Camellia nitidissima Chi, a popular medicinal and edible plant in China, is distributed in a narrow region of Southern China and Northern Vietnam. Its flowers, leaves, and seed oils show multiple bioactivities; for example, C. nitidissima flowers have been shown to inhibit the growth of the Eca109 human esophageal squamous cell carcinoma cell line (Dai et al., 2016), whereas its leaves reportedly inhibit the formation of advanced glycation end-products (Wang et al., 2016), and its seeds have been found to exhibit cytotoxicity against human lymphoma cells, as well as cervical and prostate cancer cells (Han et al., 2009). In addition, C. nitidissima Chi has shown antimicrobial effects against Staphylococcus albus, Beta streptococcus, Corynebacterium diphtheriae, and P. aeruginosa (Chen et al., 2009). However, the effects of C. nitidissima Chi flowers on QS-controlled phenotypes in bacteria and whether the flowers are a potential QSI remain unknown. Accordingly, we investigated the inhibitory effects of C. nitidissima Chi flower fractions on P. aeruginosa PAO1 virulence factors.
Materials and Methods
Bacterial Strains and Materials
P. aeruginosa PAO1 was kindly donated by Prof. Q.H. Gong from the Ocean University of China in Qingdao, and was incubated in nutrient broth (NB) at 37°C unless otherwise specified. The C. nitidissima Chi flowers were collected in July 2016 from a cultivated farm in Fangchenggang, Guangxi, China, and stored at 4°C. A C. nitidissima Chi flower voucher specimen (JHCH-001) was deposited in our lab. Gallic acid, catechin, ellagic acid, chlorogenic acid, quercetin, and kaempferol were purchased from Sangon Biotech Co., Ltd. (Shanghai, China) and dissolved in pure dimethyl sulfoxide (DMSO), the concentrations were 30, 30, 10, 30, 20, and 10 mg/mL, respectively. All other reagents in this study were of analytical grade.
Phytochemical Extraction Preparation
Phytochemical extraction procedures followed those of previous research (Wang et al., 2016), with some modifications. The C. nitidissima Chi flowers (500 g) were sun dried, then refluxed with 95% ethanol and evaporated in a rotary evaporator at 45°C to obtain the ethanolic extract (EE, 165 g). The EE was then suspended in water and extracted with dichloromethane, ethyl acetate, and n-butanol to yield the dichloromethane fraction (DF, 5.72 g), ethyl acetate fraction (EAF, 29.3 g), and n-butanol fraction (BF, 61.7 g), with the residue evaporated at 45°C to obtain the water fraction (WF, 33 g).
Minimum Inhibitory Concentrations (MICs)
The MIC values of the five fractions and the six identified compounds were determined as per previously published methods (Zhou et al., 2017), with some modifications. Briefly, overnight culture of P. aeruginosa PAO1 (1%, v/v) were added to Mueller-Hinton Broth supplemented with the samples at concentration gradients (two-fold dilution, 0.05–5.0 mg/mL) in 96-well microtiter plates, then incubated at 37°C and 150 rpm for 15 h. The MIC was the lowest concentration of the samples with visible inhibition of cell growth. The MICs of the five fractions and six identified compounds against P. aeruginosa PAO1 are recorded in Table 1. All further experiments in this study were conducted at sub-MICs.
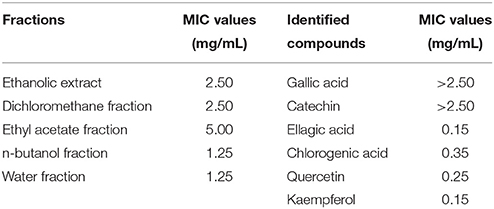
Table 1. Minimum inhibitory concentrations (MICs) of the five fractions and six compounds identified from Camellia nitidissima Chi flowers against Pseudomonas aeruginosa PAO1.
Determination of Pyocyanin Production
Pyocyanin production was determined as per previously published methods (O'Loughlin et al., 2013), with some modifications. The P. aeruginosa PAO1 culture was incubated at 37°C overnight, with 20 μL of the overnight culture then added to 2 mL of fresh medium (2% peptone, 0.14% MgCl2, 1% K2SO4, and 1% glycerinum, pH 7.4) supplemented with the five fractions and six identified compounds with shaking at 37°C and 150 rpm for 17 h. The concentrations of the five fractions were 0.0625, 0.125, 0.25, 0.5, and 0.75 mg/mL, the concentrations of gallic acid, catechin, and chlorogenic acid were 0.0375, 0.075, 0.15, and 0.3 mg/mL, the concentrations of quercetin were 0.025, 0.05, 0.1, and 0.2 mg/mL, and the concentrations of ellagic acid and kaempferol were 0.0125, 0.025, 0.05, and 0.1 mg/mL. DMSO was used as the control (0.75%, v/v). Cells were separated from culture fluids via centrifugation at 12,000 rpm for 15 min at 4°C. The cell-free culture fluids were then analyzed for pyocyanin production at 695 nm using a spectrophotometer (BioTek, Vermont, USA).
Bacterial Growth Measurement
The effect of the DF and six identified compounds on the growth of P. aeruginosa PAO1 were measured following previous methods (Sheng et al., 2015), with some modifications. In brief, overnight culture of P. aeruginosa PAO1 (1%, v/v) were added to NB supplemented with the DF at concentration gradients (0, 0.0625, 0.125, 0.25, 0.5, and 0.75 mg/mL) in Erlenmeyer flasks, then incubated at 37°C and 150 rpm. And the concentrations of gallic acid, catechin, ellagic acid, chlorogenic acid, quercetin, and kaempferol were 300, 300, 100, 300, 200, and 100 μg/mL, respectively. DMSO was used as the control (1%, v/v). The OD620 values of the culture were measured every 2 h for 24 h by a microplate reader (Biotek Elx800, USA). The growth of P. aeruginosa PAO1 was evaluated by plotting the values of OD620 against time.
Swarming Assay
The swarming assay was conducted as per prior published methods (Sheng et al., 2015), with some modifications. Briefly, the DF and six identified compounds were added to molten swarming agar (pH 7.2), which consisted of NB (0.8%), glucose (0.5%), and bacto-agar (0.5%). The concentrations of the five fractions were 0.0625, 0.125, 0.25, 0.5, and 0.75 mg/mL, the concentrations of gallic acid, catechin, and chlorogenic acid were 0.0375, 0.075, 0.15, and 0.3 mg/mL, the concentrations of quercetin were 0.025, 0.05, 0.1, and 0.2 mg/mL, and the concentrations of ellagic acid and kaempferol were 0.0125, 0.025, 0.05, and 0.1 mg/mL. The culture was then dispensed onto Petri dishes after gentle mixing. Once the culture was solidified, 2 μL of overnight P. aeruginosa PAO1 culture was inoculated in the center of the agar and then incubated at 37°C for 24 h. We used DMSO as the control (1%, v/v). Anti-QS properties were identified by the reduction in swarming motility.
Swimming Assay
The swimming assay was conducted according to previous research (Luo et al., 2016), with some modifications. The procedures were the same as those of the swarming assay, except for the swimming agar (pH 7.2), which consisted of peptone (1.0%), sodium chloride (0.5%), and bacto-agar (0.3%).
Real-Time RT-PCR
The real-time RT-PCR procedures were conducted following previous studies (Yang et al., 2012; Sheng et al., 2015), with some modifications. Overnight P. aeruginosa PAO1 culture was diluted (1:1,000) into fresh NB, with the DF added to a final concentration of 0.75 mg/mL. Cells were collected after incubation at 37°C for 16 h with agitation. Total RNA was extracted using an RNeasy Mini Kit (Qiagen, Germany) according to the manufacturer's protocols. The RNA was then reverse transcribed into complementary DNA (cDNA) using a HiScript® Q RT SuperMix for qPCR (+gDNA wiper) (Vazyme, China) according to the manufacturer's instructions. The real-time RT-PCR was performed in a 20 μL volume using an AceQTM qPCR SYBR® Green Master Mix (Vazyme, China) as recommended by the manufacturer. Primers, used to amplify the QS circuit genes lasI, lasR, rhlI, and rhlR and reference gene rpsL, are shown in Table 2. The reaction was performed using the Applied Biosystems 7300 RT-PCR System (USA) and involved incubation at 95°C for 5 min, 40 cycles at 95°C for 15 s, 58°C for 30 s, and 72°C for 30 s. The expressions of the target genes were normalized to the expression of the reference gene rpsL.
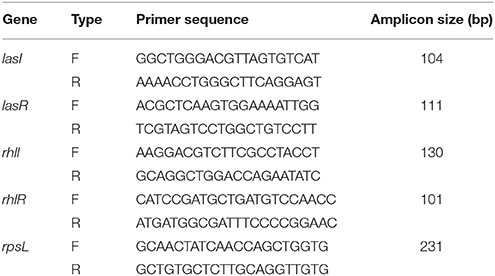
Table 2. Primers used for quorum sensing circuit genes lasI, lasR, rhlI, and rhlR, and reference gene, rpsL.
Dichloromethane Fraction Assay by HPLC Triple TOF MS/MS
We used HPLC Triple TOF MS/MS for the DF assay, as per previously published methods (Wang et al., 2016), with some modifications. The DF was analyzed on a Shimadzu HPLC equipped with a diode array detector, and a Welch Ultimate XB-C18 column (100 × 2.1 mm i.d., 3 μm; Welch Materials, Inc., Shanghai, China). Mobile phase A was 0.1% formic acid of water and mobile phase B was 0.1% formic acid of methanol, and the linear gradient was 0–1 min, 5–5% B; 1–30 min, 5–70% B; 30–35 min, 70–90% B; 35–40 min, 90–90% B; 40–40.1 min, 90–5% B; 40.1–45 min, 5–5% B. The flow rate was 0.4 mL/min and the injection volume was 10 μL. The Triple TOF 4600 system (AB SCIEX, CA) with electrospray ionization was operated at negative mode. The following parameter settings were used: ion spray voltage, 4.5 kV; ion source heater, 550°C; curtain gas, 25 psi; ion source gas 1, 55 psi; and ion source gas 2, 55 psi. Mass spectra were scanned from m/z 100 to 1,500. The collision energy was swept from 30 to 60 eV for MS/MS analysis.
Statistical Analyses
All experiments were conducted independently with at least three replicates, and results were expressed as means ± standard deviation or average. Interpolation from linear regression analysis was used to obtain the half maximal inhibitory concentrations (IC50). Unpaired or two-tailed paired t-tests were used to evaluate the significance of differences between two groups. One-way analysis of variance (ANOVA) and Duncan's multiple range tests were performed using SPSS version 17.0 (SPSS Inc., Chicago, IL, USA) software. Statistical significance was determined at p < 0.05.
Results and Discussion
Camellia nitidissima Chi Flowers Inhibit Pyocyanin Production
Pyocyanin is a vital QS-regulated virulence factor of P. aeruginosa PAO1. The inhibitory effects of the C. nitidissima Chi flower fractions on pyocyanin production are shown in Figure 1. The WF showed no inhibition activity, whereas the other four fractions showed remarkable concentration-dependent inhibitory effects on pyocyanin production. Of note, the DF had the highest inhibiting effect on pyocyanin production. At a concentration of 0.75 mg/mL, the percentage of inhibition was 67.511 ± 2.035 for the DF, and 51.265 ± 0.949, 56.962 ± 0.837, and 51.582 ± 1.096, respectively, for the EE, EAF, and BF. In addition, the IC50 value of the DF on pyocyanin production (0.158 ± 0.009 mg/mL) was significantly (p < 0.05) lower than that of the other three fractions (Table 3). As seen in Figure 2A, the DF had no influence on the growth of P. aeruginosa PAO1 at the serial concentrations of 0.0625–0.75 mg/mL. We selected the DF for all further experiments in this study due to its highest activity. As a famous tea, the C. nitidissima Chi flowers are known to have a high content of tea phenolic compounds (Peng et al., 2012). Phenolic compounds, such as ellagic acid, quercetin, and catechin, are able to inhibit pyocyanin production (Vandeputte et al., 2010; Sarabhai et al., 2013; Ouyang et al., 2016). Therefore, the inhibitory effects of C. nitidissima Chi flowers on pyocyanin production observed here might be via their phenolic compounds. In addition, pyocyanin is encoded by virulence genes, which are regulated by the RhlRI QS system in P. aeruginosa PAO1 (Castillo-Juarez et al., 2017). Thus, some compounds in the C. nitidissima Chi flowers, especially in the DF, might influence the expressions of rhlI and/or rhlR to inhibit the production of pyocyanin in P. aeruginosa PAO1.
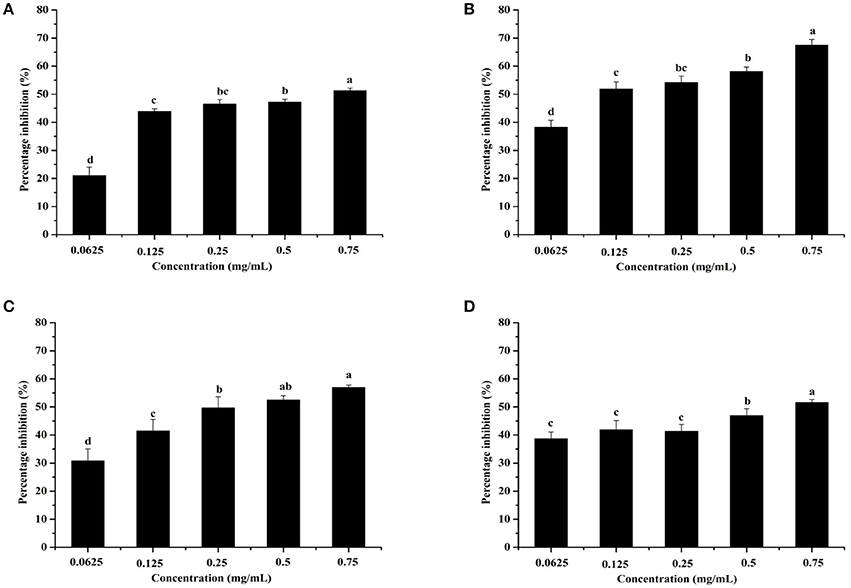
Figure 1. Effects of the Camellia nitidissima Chi flower fractions on pyocyanin production. Ethanolic extract (A), Dichloromethane fraction (B), Ethyl acetate fraction (C), and N-butanol fraction (D). Means with different small letters (a–d) are significantly different (p < 0.05).
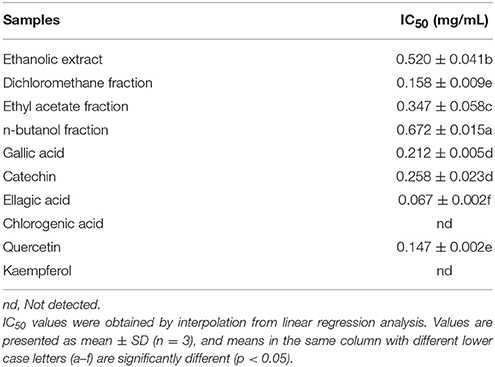
Table 3. Half maximal inhibitory concentrations (IC50) of four fractions and six compounds identified from Camellia nitidissima Chi flowers on pyocyanin production of Pseudomonas aeruginosa PAO1.
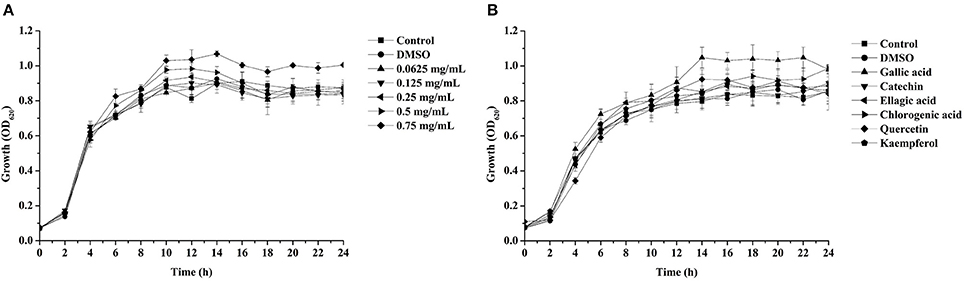
Figure 2. Effects of the dichloromethane fraction from the Camellia nitidissima Chi flower (A) and gallic acid (300 μg/mL), catechin (300 μg/mL), ellagic acid (100 μg/mL), chlorogenic acid (300 μg/mL), quercetin (200 μg/mL), and kaempferol (100 μg/mL) (B) on Pseudomonas aeruginosa PAO1 growth.
Dichloromethane Fraction Inhibits P. aeruginosa PAO1 Swarming Motility
Swarming motility is a type of virulence factor in P. aeruginosa PAO1, and is defined by rapid and coordinated translocation of a bacterial population across a semi-solid surface (Hayouni et al., 2008). As seen in Figure 3, the DF significantly inhibited the swarming motility of P. aeruginosa PAO1 in a concentration-dependent manner. The tendrils of the P. aeruginosa PAO1 bacterial colony decreased with increasing DF concentration, and the IC50 value of the DF on swarming motility was 0.139 ± 0.004 mg/mL (Table 4). At 0.75 mg/mL, there were no defined tendrils observed, and the average swarming diameter was 9.333 ± 0.577 mm. The significant inhibition effect on swarming motility was also observed at the relatively low concentration of 0.0625 mg/mL, with an average swarming diameter of 30.333 ± 1.155 mm, which was significantly lower (p < 0.05) than that of the control (42.333 ± 2.517 mm). Swarming motility is a phenotype controlled by the QS system (Kohler et al., 2000). Therefore, our results strongly indicate that the DF had the ability to interfere with the QS system of P. aeruginosa PAO1.
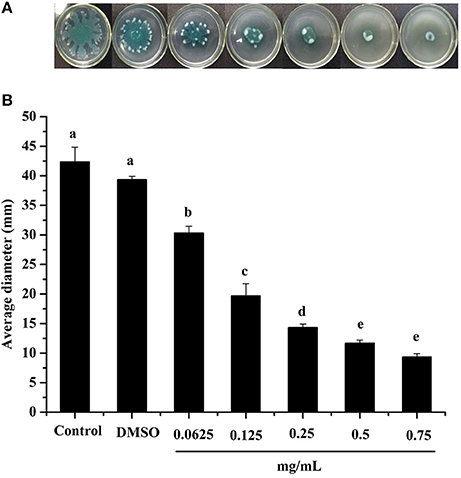
Figure 3. Swarming inhibition assay. Swarming agar inoculated with the dichloromethane fraction of the Camellia nitidissima Chi flowers (A), and average diameter of the bacterial colony tendrils (B). Means with different small letters (a–e) are significantly different (p < 0.05).
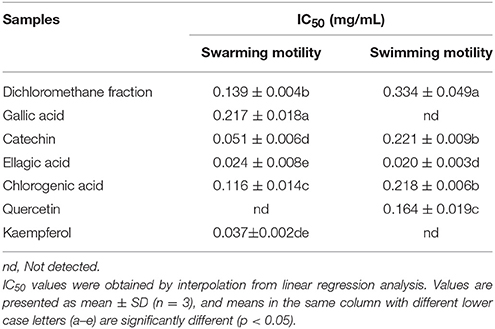
Table 4. Half maximal inhibitory concentrations (IC50) of the dichloromethane fraction and six compounds identified from Camellia nitidissima Chi flowers on the swarming motility and swimming motility of Pseudomonas aeruginosa PAO1.
Dichloromethane Fraction Inhibits P. aeruginosa PAO1 Swimming Motility
Swimming is another major form of P. aeruginosa PAO1 motility, in which bacteria swim in aqueous environments via the flagellum (Rashid and Kornberg, 2000). As shown in Figure 4, the DF inhibited P. aeruginosa PAO1 swimming motility in a concentration-dependent manner. The average diameters of the bacterial colony significantly (p < 0.05) decreased with increasing DF concentration, and were 37.33 and 12.67 mm at concentrations of 0.0625 and 0.75 mg/mL, respectively, compared with 42.33 mm for the control. The IC50 value of the DF on swimming motility was 0.334 ± 0.049 mg/mL (Table 4). Similar to swarming motility, swimming motility is regulated by the QS system in P. aeruginosa PAO1 (Williams and Camara, 2009; Kumar et al., 2015) and is crucial for its pathogenesis, playing an important role in the expression of full virulence and colonization. Thus, the DF might inhibit swimming motility by interfering with QS, thereby contributing to the reduced expression of P. aeruginosa PAO1 virulence factors.
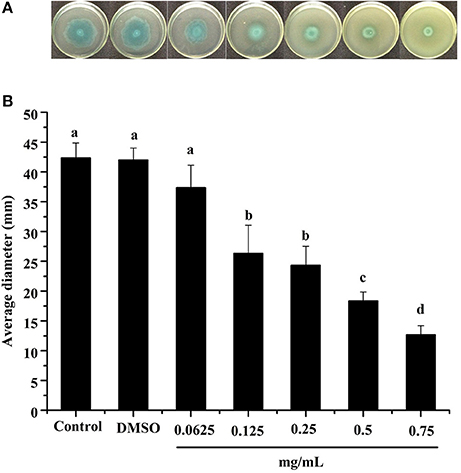
Figure 4. Swimming inhibition assay. Swimming agar inoculated with the dichloromethane fraction of the Camellia nitidissima Chi flowers (A), and average diameter of the bacterial colony (B). Means with different small letters (a–e) are significantly different (p < 0.05).
Dichloromethane Fraction Effects the Expressions of lasI, lasR, rhlI, and rhlR
In the las and rhl systems, the virulence factors of P. aeruginosa PAO1 are mainly encoded by QS-regulated genes lasI, lasR, rhlI, and rhlR (Castillo-Juarez et al., 2017). We investigated whether the DF could influence the expressions of QS-regulated genes to reduce P. aeruginosa PAO1 virulence factors. As shown in Figure 5, the DF (at 0.75 mg/mL) down-regulated the expression of all tested genes. Average relative amounts of the tested genes were normalized to the average relative amount of the rpsL reference gene, with lasR (p < 0.05) and rhlR (p < 0.01) found to be significantly decreased. In the las system, the lasR gene encodes the signal receptor LasR, and binds 3-oxo-C12-HSL to activate certain target gene transcriptions (Pearson et al., 1994; Schuster and Greenberg, 2006). In the rhl system, the rhlR gene encodes the signal receptor RhlR, and induces gene expression when complexed with C4-HSL (Pearson et al., 1995; Schuster and Greenberg, 2006). It has been reported that RhlR antagonists can strongly inhibit pyocyanin production (O'Loughlin et al., 2013), and that LasR and RhlR interacting with and activated by 3-oxo-C12-HSL and C4-HSL, respectively, can trigger the production of pyocyanin and other virulence factors (Vandeputte et al., 2011). Thus, in this study, the significantly decreased expressions of lasR (p < 0.05) and rhlR (p < 0.01) resulted in the decrease in pyocyanin production and swarming and swimming motility. Our results indicate that the DF could reduce P. aeruginosa PAO1 virulence factors via regulation of the QS system.
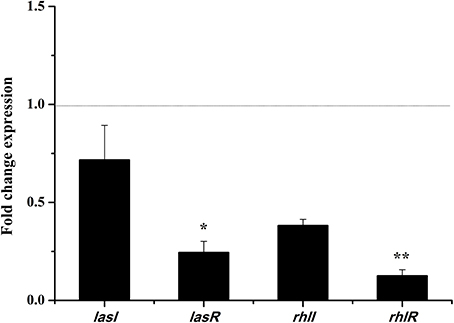
Figure 5. Effects of the dichloromethane fraction (0.75 mg/mL) of the Camellia nitidissima Chi flowers on gene expressions of QS regulatory circuits in Pseudomonas aeruginosa PAO1. Average relative amounts of tested genes were normalized to the average relative amount of the rpsL reference gene. *p < 0.05; **p < 0.01.
Dichloromethane Fraction Assay by HPLC Triple TOF MS/MS
In total, six compounds in the DF of C. nitidissima Chi flowers were identified (Table 5) by HPLC Triple TOF MS/MS analysis. The extract ion chromatogram at m/z 169.0146 showed a peak at Rt 2.09 min (Figure 6). The peak displayed a fragment at m/z 125 (Figure 7A) corresponding to the loss of one CO2 fragment, and was identified as gallic acid (Dou et al., 2007). At m/z 289.0726, the chromatogram showed a peak at Rt 6.14 min (Figure 6). The peak displayed fragments at m/z 245, 205, 203, and 137 (Figure 7B) corresponding to the loss of CO2, C4H4O2, C4H6O2, and C8H8O3 fragments, respectively, and was identified as catechin (Gottumukkala et al., 2014). At m/z 300.99931, the chromatogram showed a peak at Rt 9.76 min (Figure 6). The peak displayed fragments at m/z 257 and 229 (Figure 7C), and was identified as ellagic acid based on the mass spectra (Mullen et al., 2003). At m/z 353.08916, the chromatogram showed a peak at Rt 12.34 min (Figure 6). The peak displayed a fragment at m/z 191 (Figure 7D), and was identified as chlorogenic acid (Fang et al., 2002). At m/z 301.0366, the chromatogram showed a peak at Rt 13.86 min (Figure 6), with fragments at m/z 273, 255, 179, and 151 (Figure 7E) corresponding to the loss of CO, CH2O2, C7H6O2, and C8H6O3 fragments, respectively. The compound was identified as quercetin (McNab et al., 2009). At m/z 285.0405, the chromatogram showed a peak at Rt 15.86 min (Figure 6), with fragments at m/z 239, 229, 211, and 187 (Figure 7F), and was identified as kaempferol (McNab et al., 2009).

Table 5. Mass spectrometric data of the six compounds identified in the dichloromethane fraction of Camellia nitidissima Chi flowers by HPLC Triple TOF MS/MS.
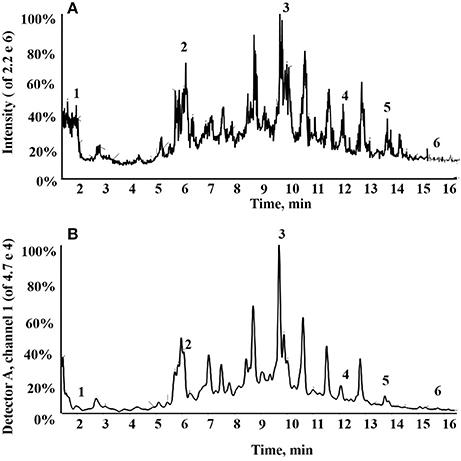
Figure 6. Total ion chromatogram of the dichloromethane fraction of the Camellia nitidissima Chi flowers (A); HPLC chromatogram of the dichloromethane fraction by 360 nm detection (B).
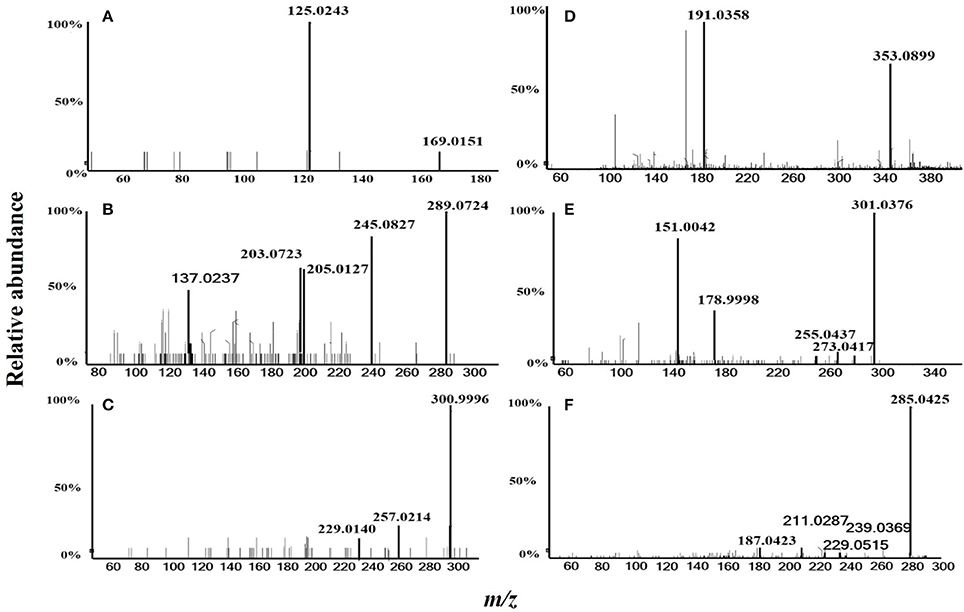
Figure 7. MS/MS spectrometric analysis of the six compounds identified in the dichloromethane fraction of the Camellia nitidissima Chi flowers by HPLC Triple TOF MS/MS. Gallic acid (A), Catechin (B), Ellagic acid (C), Chlorogenic acid (D), Quercetin (E), and Kaempferol (F).
Effects of the Six Identified Compounds on Reducing P. aeruginosa PAO1 Pyocyanin Production and Motility
The effects of the six identified compounds on reducing P. aeruginosa PAO1 pyocyanin production are shown in Figure 8. Obviously, all six identified compounds had the ability to reduce pyocyanin production without the effects on the growth (Figure 2B), and except for kaempferol, the effects of the compounds were in a concentration-dependent manner. Among the six identified compounds, ellagic acid showed the strongest effect on reducing pyocyanin production, with a percentage inhibition of 52.3% at 0.10 mg/mL. The IC50 of ellagic acid on pyocyanin production was 0.067 ± 0.002 mg/mL (Table 3), which was significantly (p < 0.05) lower than that of the other five compounds. The IC50 of quercetin on pyocyanin production was 0.147 ± 0.002 mg/mL, which was significantly (p < 0.05) lower than that of gallic acid and catechin (0.212 ± 0.005 and 0.258 ± 0.023 mg/mL, respectively). The IC50 values of chlorogenic acid and kaempferol on pyocyanin production were not detected. These results suggest that the four compounds, especially ellagic acid, in the DF played important roles in the inhibition of P. aeruginosa PAO1 pyocyanin production.
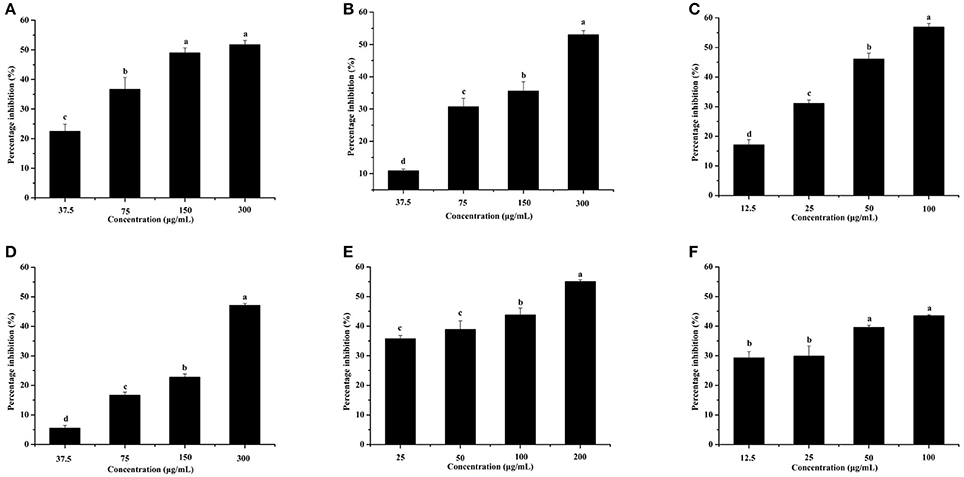
Figure 8. Effects of the six identified compounds on pyocyanin production. Gallic acid (A), Catechin (B), Ellagic acid (C), Chlorogenic acid (D), Quercetin (E), and Kaempferol (F). Means with different small letters (a–d) are significantly different (p < 0.05).
Figure 9 shows that all six identified compounds could inhibit the swarming motility of P. aeruginosa PAO1. Among the six identified compounds, ellagic acid had the most remarkable inhibitory effect on swarming motility; at 0.1 mg/mL, the average swarming diameter of ellagic acid was 10.857 ± 1.309 mm, with no bacterial colony tendrils observed, which was significantly (p < 0.05) lower than that of the control. As shown in Table 4, the IC50 value of ellagic acid on swarming motility was 0.024 ± 0.008 mg/mL, whereas the IC50 values were 0.217 ± 0.018, 0.051 ± 0.006, 0.116 ± 0.014, and 0.037 ± 0.002 mg/mL for gallic acid, catechin, chlorogenic acid, and kaempferol, respectively, which were all higher than that of ellagic acid. In addition, the IC50 of quercetin on swarming motility was not detected because at the four tested concentrations, the inhibiting values all were higher than 50%, but lower than that of ellagic acid. Similarly, all six identified compounds showed inhibitory effects on P. aeruginosa PAO1 swimming motility (Figure 10). Interestingly, the inhibitory effect of ellagic acid on swimming motility was the strongest among the identified six compounds, with average bacterial colony diameters significantly (p < 0.05) decreased compared with the control. At 0.1 mg/mL, the average bacterial colony diameter of ellagic acid was 12.754 ± 1.004 mm, which was significantly (p < 0.05) lower that of the control (42.333 ± 2.517 mm). In addition, the IC50 value of ellagic acid on swimming motility (0.020 ± 0.003 mg/mL) was the lowest among the six compounds, (Table 4). These results indicate that ellagic acid is a remarkable inhibitor for swarming and swimming motility of P. aeruginosa PAO1, and might be the main active constituent of the DF to inhibit swarming and swimming motility of P. aeruginosa PAO1.
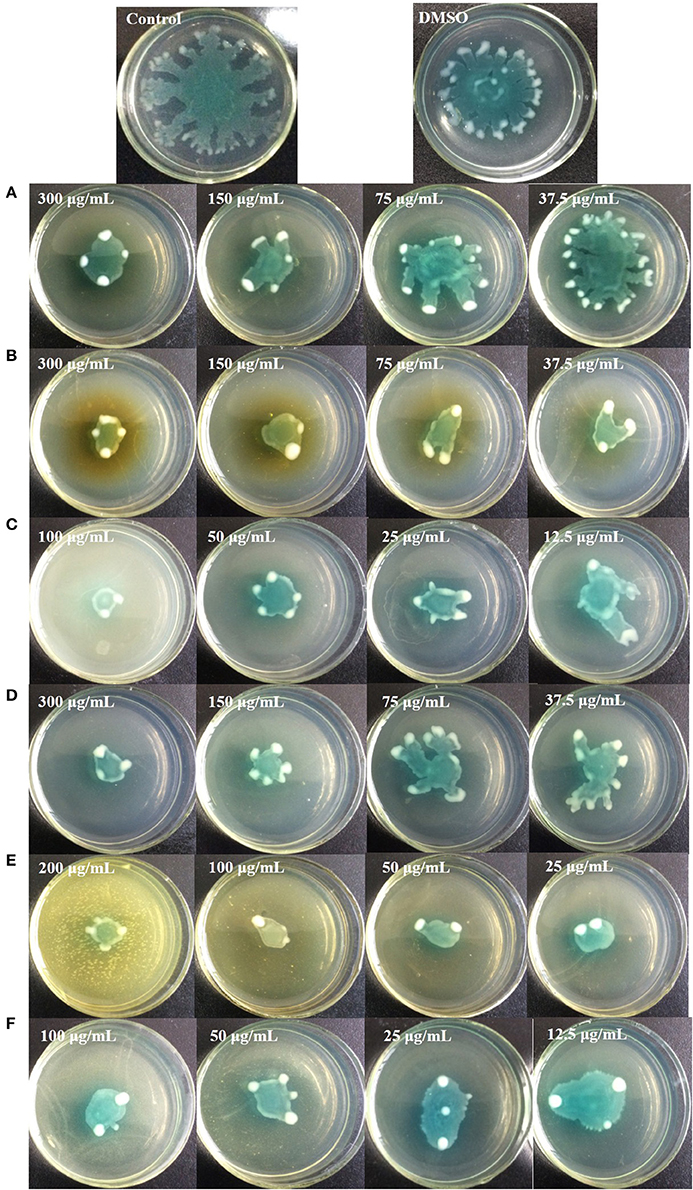
Figure 9. Swarming inhibition assays of the six identified compounds. Gallic acid (A), Catechin (B), Ellagic acid (C), Chlorogenic acid (D), Quercetin (E), and Kaempferol (F).
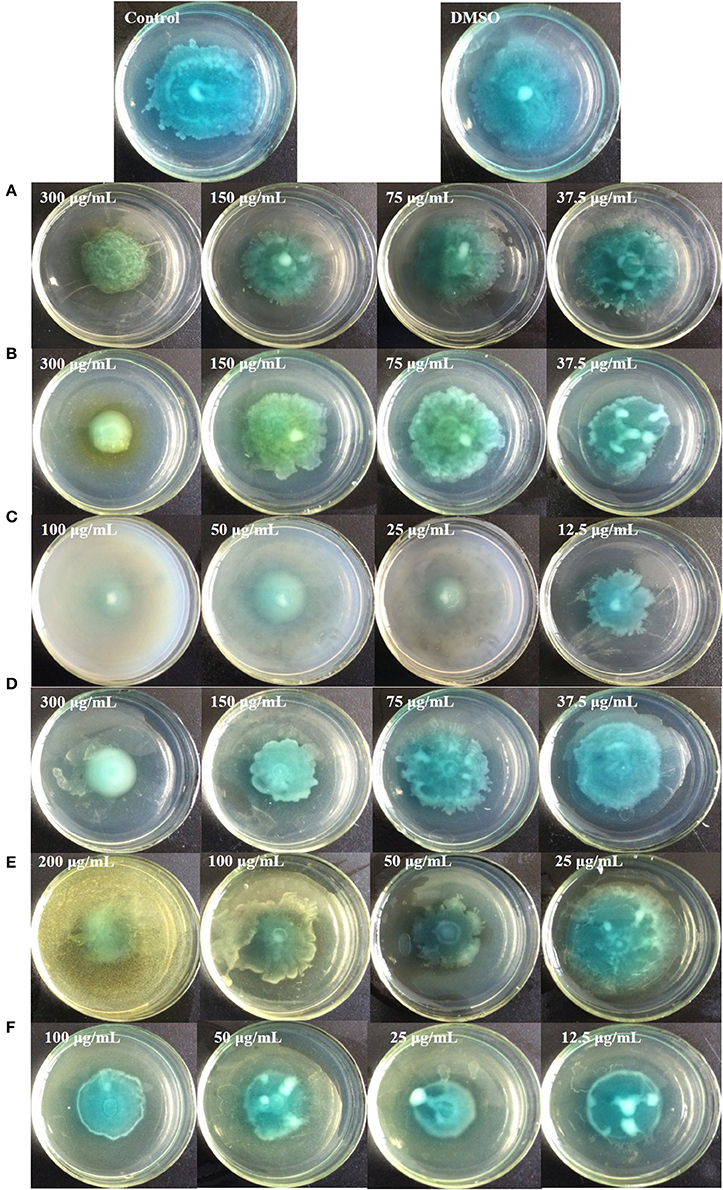
Figure 10. Swimming inhibition assays of the six identified compounds. Gallic acid (A), Catechin (B), Ellagic acid (C), Chlorogenic acid (D), Quercetin (E), and Kaempferol (F).
Our findings are supported by previous studies. Among the six identified compounds, catechin, ellagic acid, quercetin, and kaempferol have been reported to reduce the virulence factors of P. aeruginosa PAO1 (Singh et al., 2009; Vandeputte et al., 2010; Sarabhai et al., 2013; Ouyang et al., 2016), and chlorogenic acid and gallic acid in Rosa rugosa and Moringa oleifera have also shown inhibitory effects on QS-controlled phenotypes, indicating that all identified compounds show anti-quorum sensing potential (Singh et al., 2009; Zhang et al., 2014). Thus, these six compounds contributed to the inhibitory effects on pyocyanin production, swarming motility, and swimming motility of P. aeruginosa PAO1 in the DF of C. nitidissima Chi flowers.
In conclusion, to the best of our knowledge, this is the first study to report on the inhibitory effects of C. nitidissima Chi flower fractions on pyocyanin production, swarming motility, and swimming motility of P. aeruginosa PAO1 at sub-MICs. The C. nitidissima Chi fractions, especially the DF, showed inhibitory effects on pyocyanin production without influencing the growth of P. aeruginosa PAO1. The DF also inhibited swarming and swimming motility of P. aeruginosa PAO1 in a concentration-dependent manner. In addition, the DF significantly down-regulated the expressions of lasR (p < 0.05) and rhlR (p < 0.01) in P. aeruginosa PAO1 to cause the inhibitory effects on pyocyanin production, swarming motility, and swimming motility. We identified six compounds from the DF. All six identified compounds, especially ellagic acid, reduced the pyocyanin production, swarming motility, and swimming motility of P. aeruginosa PAO1. Thus, the inhibitory effects on the QS-controlled phenotypes of P. aeruginosa PAO1 might be attributable to these six and/or other compounds in the DF of C. nitidissima Chi flowers. Thus, the C. nitidissima Chi flower, especially the DF, might be a potential quorum sensing inhibitor of P. aeruginosa PAO1.
Author Contributions
RY and AJ conceived and designed the experiments. RY, YG, JZ, and ZH performed the experiments. RY, BS, ZW, and HC analyzed the data. RY and AJ wrote the paper.
Funding
This work was supported by the grants from the National Key Research and Development Program of China (2017YFD0201401), Natural Science Foundation of Jiangsu Province, China (BK20170859), Science and Technology Development Program of Modern Agriculture, Nanjing (201608052), Six Talent Peaks Project in Jiangsu Province, Fundamental Research Funds for the Central Universities (30916011307).
Conflict of Interest Statement
The authors declare that the research was conducted in the absence of any commercial or financial relationships that could be construed as a potential conflict of interest.
Acknowledgments
The authors express their sincere thanks to Dr. Yuan Xu for providing important suggestions.
References
Castillo-Juarez, I., Lopez-Jacome, L. E., Soberon-Chavez, G., Tomas, M., Lee, J., Castaneda-Tamez, P., et al. (2017). Exploiting quorum sensing inhibition for the control of Pseudomonas Aeruginosa and Acinetobacter Baumannii biofilms. Curr. Top. Med. Chem. 17, 1915–1927. doi: 10.2174/1568026617666170105144104
Castillo-Juárez, I., Maeda, T., Mandujano-Tinoco, E. A., Tomás, M., Pérez-Eretza, B., García-Contreras, S. J., et al. (2015). Role of quorum sensing in bacterial infections. World J. Clin. Cases 3, 575-598. doi: 10.12998/wjcc.v3.i7.575
Chen, Y. Y., Huang, Y. L., and Wen, Y. X. (2009). Advance in study on chemical constituents and pharmacological action of Camellia chrysantha. Guangxi Trop. Agric. 1, 14–16. doi: 10.3969/j.issn.2095-0764.2009.01.005
Choo, J. H., Rukayadi, Y., and Hwang, J. K. (2006). Inhibition of bacterial quorum sensing by vanilla extract. Lett. Appl. Microbiol. 42, 637–641. doi: 10.1111/j.1472-765X.2006.01928.x
Costerton, J. W. (2001). Cystic fibrosis pathogenesis and the role of biofilms in persistent infection. Trends Microbiol. 9, 50–52. doi: 10.1016/S0966-842X(00)01918-1
Dai, L., Li, J. L., Liang, X. Q., Li, L., Feng, Y., Liu, H. Z., et al. (2016). Flowers of Camellia nitidissima cause growth inhibition, cell-cycle dysregulation and apoptosis in a human esophageal squamous cell carcinoma cell line. Mol. Med. Rep. 14, 1117–1122. doi: 10.3892/mmr.2016.5385
Dhaliwal, L. K. (2015). Effect of atorvastatin and rosuvastatin on quorum sensing, biofilm formation and bacterial motilities of Pseudomonas Aeruginosa. Int. J. Pharm. Biol. Sci. 6, 1–8.
Dou, J., Lee, V. S., Tzen, J. T., and Lee, M. R. (2007). Identification and comparison of phenolic compounds in the preparation of oolong tea manufactured by semifermentation and drying processes. J. Agric. Food Chem. 55, 7462–7468. doi: 10.1021/jf0718603
Drenkard, E. (2003). Antimicrobial resistance of Pseudomonas aeruginosa biofilms. Microbes Infect. 5, 1213–1219. doi: 10.1016/j.micinf.2003.08.009
Fang, N. B., Yu, S. G., and Prior, R. L. (2002). LC/MS/MS characterization of phenolic constituents in dried plums. J. Agric. Food Chem. 50, 3579–3585. doi: 10.1021/jf0201327
Gambello, M. J., and Iglewski, B. H. (1991). Cloning and characterization of the Pseudomonas aeruginosa lasR gene, a transcriptional activator of elastase expression. J. Bacteriol. 173, 3000–3009. doi: 10.1128/jb.173.9.3000-3009.1991
Gottumukkala, R. V. S. S., Nadimpalli, N., Sukala, K., and Subbaraju, G. V. (2014). Determination of Catechin and Epicatechin content in chocolates by high-performance liquid chromatography. Int. Sch. Res. Notices 2014:628196. doi: 10.1155/2014/628196
Han, L. C., Shi, L. Y., Yu, D. Y., Tang, Q., Tang, L., Feng, B. M., et al. (2009). Inhibitive effect of seeds of Camellia chrysantha (Hu) Tuyama on gonadal hormones dependent tumour in vitro. Lishizhen Med. Mat. Med. Res. 12:120. doi: 10.3969/j.issn.1008-0805.2009.12.120
Hayouni, E. A., Bouix, M., Abedrabba, M., Leveau, J. Y., and Hamdi, M. (2008). Mechanism of action of Melaleuca armillaris (Sol. Ex Gaertu) Sm. essential oil on six LAB strains as assessed by multiparametric flow cytometry and automated microtiter-based assay. Food Chem. 111, 707–718. doi: 10.1016/j.foodchem.2008.04.044
Hentzer, M., and Givskov, M. (2003). Pharmacological inhibition of quorum sensing for the treatment of chronic bacterial infections. J. Clin. Invest. 112, 1300–1307. doi: 10.1172/JCI20074
Hentzer, M., Wu, H., Andersen, J. B., Riedel, K., Rasmussen, T. B., Bagge, N., et al. (2003). Attenuation of Pseudomonas aeruginosa virulence by quorum sensing inhibitors. EMBO J. 22, 3803–3815. doi: 10.1093/emboj/cdg366
Kalia, V. C. (2013). Quorum sensing inhibitors: an overview. Biotechnol. Adv. 31, 224–245. doi: 10.1016/j.biotechadv.2012.10.004
Kawamurasato, K., Iinuma, Y., Hasegawa, T., Horii, T., Yamashino, T., and Ohta, M. (2000). Effect of subinhibitory concentrations of macrolides on expression of Flagellin in Pseudomonas aeruginosa and Proteus mirabilis. Antimicrob. Agents Chemother. 44, 2869–2872. doi: 10.1128/AAC.44.10.2869-2872.2000
Kohler, T., Curty, L. K., Barja, F., Van Delden, C., and Pechere, J. C. (2000). Swarming of Pseudomonas aeruginosa is dependent on cell-to-cell signaling and requires flagella and pili. J. Bacteriol. 182, 5990–5996. doi: 10.1128/JB.182.21.5990-5996.2000
Kumar, L., Chhibber, S., Kumar, R., Kumar, M., and Harjai, K. (2015). Zingerone silences quorum sensing and attenuates virulence of Pseudomonas aeruginosa. Fitoterapia 102, 84–95. doi: 10.1016/j.fitote.2015.02.002
Luo, J., Kong, J. L., Dong, B. Y., Huang, H., Wang, K., Wu, L. H., et al. (2016). Baicalein attenuates the quorum sensing-controlled virulence factors of Pseudomonas aeruginosa and relieves the inflammatory response in P. aeruginosa-infected macrophages by downregulating the MAPK and NF?B signal-transduction pathways. Drug. Des. Dev. Ther. 10, 183–203. doi: 10.2147/DDDT.S97221
Maisuria, V. B., Los Santos, Y. L., Tufenkji, N., and Deziel, E. (2016). Cranberry-derived proanthocyanidins impair virulence and inhibit quorum sensing of Pseudomonas aeruginosa. Sci. Rep. 6:30169. doi: 10.1038/srep30169
McNab, H., Ferreira, E. S. B., Hulme, A. N., and Quye, A. (2009). Negative ion ESI–MS analysis of natural yellow dye flavonoids-An isotopic labelling study. Int. J. Mass Spectrom. 284, 57–65. doi: 10.1016/j.ijms.2008.05.039
Mullen, W., Yokota, T., Lean, M. E. J., and Crozier, A. (2003). Analysis of ellagitannins and conjugates of ellagic acid and quercetin in raspberry fruits by LC–MSn. Phytochemistry 64, 617–624. doi: 10.1016/S0031-9422(03)00281-4
Musthafa, K. S., Balamurugan, K., Pandian, S. K., and Ravi, A. V. (2012). 2,5-Piperazinedione inhibits quorum sensing-dependent factor production in Pseudomonas aeruginosa PAO1. J. Basic Microbiol. 52, 679–686. doi: 10.1002/jobm.201100292
Ochsner, U. A., Koch, A. K., Fiechter, A., and Reiser, J. (1994). Isolation and characterization of a regulatory gene affecting rhamnolipid biosurfactant synthesis in Pseudomonas aeruginosa. J. Bacteriol. 176, 2044–2054. doi: 10.1128/jb.176.7.2044-2054.1994
O'Loughlin, C. T., Miller, L. C., Siryaporn, A., Drescher, K., Semmelhack, M. F., and Bassler, B. L. (2013). A quorum-sensing inhibitor blocks Pseudomonas aeruginosa virulence and biofilm formation. Proc. Natl. Acad. Sci. U.S.A. 110, 17981–17986. doi: 10.1073/pnas.1316981110
Ouyang, J., Sun, F., Feng, W., Sun, Y., Qiu, X., Xiong, L., et al. (2016). Quercetin is an effective inhibitor of quorum sensing, biofilm formation and virulence factors in Pseudomonas aeruginosa. J. Appl. Microbiol. 120, 966–974. doi: 10.1111/jam.13073
Parsek, M. R., and Greenberg, E. P. (2000). Acyl-homoserine lactone quorum sensing in gram-negative bacteria: a signaling mechanism involved in associations with higher organisms. Proc. Natl. Acad. Sci. U.S.A. 97, 8789–8793. doi: 10.1073/pnas.97.16.8789
Pearson, J. P., Gray, K. M., Passador, L., Tucker, K. D., Eberhard, A., Iglewski, B. H., et al. (1994). Structure of the autoinducer required for expression of Pseudomonas aeruginosa virulence genes. Proc. Natl. Acad. Sci. U.S.A. 91, 197–201. doi: 10.1073/pnas.91.1.197
Pearson, J. P., Passador, L., Iglewski, B. H., and Greenberg, E. P. (1995). A second N-acylhomoserine lactone signal produced by Pseudomonas aeruginosa. Proc. Natl. Acad. Sci. U.S.A. 92, 1490–1494. doi: 10.1073/pnas.92.5.1490
Peng, X., Yu, D. Y., Feng, B. M., Wang, Y. Q., and Shi, L. Y. (2012). A new acylated flavonoid glycoside from the flowers of Camellia nitidissima and its effect on the induction of apoptosis in human lymphoma U937 cells. J. Asian Nat. Prod. Res. 14, 799–804. doi: 10.1080/10286020.2012.691475
Puupponen-Pimia, R., Nohynek, L., Hartmann-Schmidlin, S., Kahkonen, M., Heinonen, M., Maatta-Riihinen, K., et al. (2005). Berry phenolics selectively inhibit the growth of intestinal pathogens. J. Appl. Microbiol. 98, 991–1000. doi: 10.1111/j.1365-2672.2005.02547.x
Rashid, M. H., and Kornberg, A. (2000). Inorganic polyphosphate is needed for swimming, swarming, and twitching motilities of Pseudomonas aeruginosa. Proc. Natl. Acad. Sci. U.S.A. 97, 4885–4890. doi: 10.1073/pnas.060030097
Rasko, D. A., and Sperandio, V. (2010). Anti-virulence strategies to combat bacteria-mediated disease. Nat. Rev. Drug Discov. 9, 117-128. doi: 10.1038/nrd3013
Reverchon, S., Chantegrel, B., Deshayes, C., Doutheau, A., and Cotte-Pattat, N. (2002). New synthetic analogues of N-acyl homoserine lactones as agonists or antagonists of transcriptional regulators involved in bacterial quorum sensing. Bioorg. Med. Chem. Lett. 12, 1153–1157. doi: 10.1016/S0960-894X(02)00124-5
Sarabhai, S., Sharma, P., and Capalash, N. (2013). Ellagic acid derivatives from Terminalia chebula Retz. downregulate the expression of quorum sensing genes to attenuate Pseudomonas aeruginosa PAO1 virulence. PLoS ONE 8:e53441. doi: 10.1371/journal.pone.0053441
Schauder, S., and Bassler, B. L. (2001). The languages of bacteria. Genes Dev. 15, 1468–1480. doi: 10.1101/gad.899601
Schuster, M., and Greenberg, E. P. (2006). A network of networks: quorum-sensing gene regulation in Pseudomonas aeruginosa. Int. J. Med. Microbiol. 296, 73–81. doi: 10.1016/j.ijmm.2006.01.036
Sheng, J. Y., Chen, T. T., Tan, X. J., Chen, T., and Jia, A. Q. (2015). The quorum-sensing inhibiting effects of stilbenoids and their potential structure-activity relationship. Bioorg. Med. Chem. Lett. 25, 5217–5220. doi: 10.1016/j.bmcl.2015.09.064
Singh, B. N., Singh, B. R., Singh, R. L., Prakash, D., Dhakarey, R., Upadhyay, G., et al. (2009). Oxidative DNA damage protective activity, antioxidant and anti-quorum sensing potentials of Moringa oleifera. Food Chem. Toxicol. 47, 1109–1116. doi: 10.1016/j.fct.2009.01.034
Skogman, M. E., Kanerva, S., Manner, S., Vuorela, P. M., and Fallarero, A. (2016). Flavones as quorum sensing inhibitors identified by a newly optimized screening platform using chromobacterium violaceum as reporter bacteria. Molecules 21:E1211. doi: 10.3390/molecules21091211
Sokovic, M., Ciric, A., Glamoclija, J., Nikolic, M., and Van Griensven, L. J. (2014). Agaricus blazei hot water extract shows anti quorum sensing activity in the nosocomial human pathogen Pseudomonas aeruginosa. Molecules 19, 4189–4199. doi: 10.3390/molecules19044189
Truchado, P., Tomás-Barberán, F. A., Larrosa, M., and Allende, A. (2012). Food phytochemicals act as quorum sensing inhibitors reducing production and/or degrading autoinducers of Yersinia enterocolitica and Erwinia carotovora. Food Control 24, 78–85. doi: 10.1016/j.foodcont.2011.09.006
Vandeputte, O. M., Kiendrebeogo, M., Rajaonson, S., Diallo, B., Mol, A., El Jaziri, M., et al. (2010). Identification of catechin as one of the flavonoids from Combretum albiflorum bark extract that reduces the production of quorum-sensing-controlled virulence factors in Pseudomonas aeruginosa PAO1. Appl. Environ. Microbiol. 76, 243–253. doi: 10.1128/AEM.01059-09
Vandeputte, O. M., Kiendrebeogo, M., Rasamiravaka, T., Stevigny, C., Duez, P., Rajaonson, S., et al. (2011). The flavanone naringenin reduces the production of quorum sensing-controlled virulence factors in Pseudomonas aeruginosa PAO1. Microbiology 157, 2120–2132. doi: 10.1099/mic.0.049338-0
Wang, H. H., Ye, K. P., Zhang, Q. Q., Dong, Y., Xu, X. L., and Zhou, G. H. (2013). Biofilm formation of meat-borne Salmonella enterica and inhibition by the cell-free supernatant from Pseudomonas aeruginosa. Food Control 32, 650–658. doi: 10.1016/j.foodcont.2013.01.047
Wang, S. W., Yu, S., Zhang, Z. Y., Wei, Q., Yan, L., Ai, G. M., et al. (2014). Coordination of swarming motility, biosurfactant synthesis, and biofilm matrix exopolysaccharide production in Pseudomonas aeruginosa. Appl. Environ. Microbiol. 80, 6724–6732. doi: 10.1128/AEM.01237-14
Wang, W. X., Liu, H. Y., Wang, Z. N., Qi, J., Yuan, S. T., Zhang, W. J., et al. (2016). Phytochemicals from Camellia nitidissima Chi inhibited the formation of advanced glycation end-products by scavenging methylglyoxal. Food Chem. 205, 204–211. doi: 10.1016/j.foodchem.2016.03.019
Williams, P., and Camara, M. (2009). Quorum sensing and environmental adaptation in Pseudomonas aeruginosa: a tale of regulatory networks and multifunctional signal molecules. Curr. Opin. Microbiol. 12, 182–191. doi: 10.1016/j.mib.2009.01.005
Yang, Y. X., Xu, Z. H., Zhang, Y. Q., Tian, J., Weng, L. X., and Wang, L. H. (2012). A new quorum-sensing inhibitor attenuates virulence and decreases antibiotic resistance in Pseudomonas aeruginosa. J. Microbiol. 50, 987–993. doi: 10.1007/s12275-012-2149-7
Zhang, J. M., Rui, X., Wang, L. X., Guan, Y., Sun, X. M., and Dong, M. S. (2014). Polyphenolic extract from Rosa rugosa tea inhibits bacterial quorum sensing and biofilm formation. Food Control 42, 125–131. doi: 10.1016/j.foodcont.2014.02.001
Keywords: Camellia nitidissima Chi flowers, phytochemicals, Pseudomonas aeruginosa PAO1, virulence factors, real-time RT-PCR, HPLC triple TOF MS/MS
Citation: Yang R, Guan Y, Zhou J, Sun B, Wang Z, Chen H, He Z and Jia A (2018) Phytochemicals from Camellia nitidissima Chi Flowers Reduce the Pyocyanin Production and Motility of Pseudomonas aeruginosa PAO1. Front. Microbiol. 8:2640. doi: 10.3389/fmicb.2017.02640
Received: 14 August 2017; Accepted: 18 December 2017;
Published: 09 January 2018.
Edited by:
Aldo Corsetti, Università di Teramo, ItalyReviewed by:
Akanksha Singh, Central Institute of Medicinal and Aromatic Plants (CIMAP), IndiaYosuke Tashiro, Shizuoka University, Japan
Copyright © 2018 Yang, Guan, Zhou, Sun, Wang, Chen, He and Jia. This is an open-access article distributed under the terms of the Creative Commons Attribution License (CC BY). The use, distribution or reproduction in other forums is permitted, provided the original author(s) or licensor are credited and that the original publication in this journal is cited, in accordance with accepted academic practice. No use, distribution or reproduction is permitted which does not comply with these terms.
*Correspondence: Aiqun Jia, aiqunj302@njust.edu.cn
†These authors have contributed equally to this work.