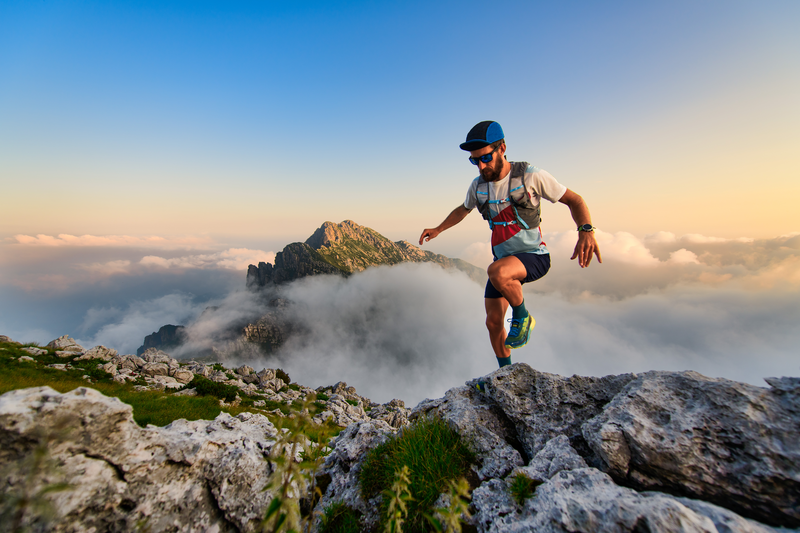
94% of researchers rate our articles as excellent or good
Learn more about the work of our research integrity team to safeguard the quality of each article we publish.
Find out more
ORIGINAL RESEARCH article
Front. Microbiol. , 04 December 2017
Sec. Extreme Microbiology
Volume 8 - 2017 | https://doi.org/10.3389/fmicb.2017.02400
Shallow-water hydrothermal systems represent extreme environments with unique biogeochemistry and high biological productivity, at which autotrophic microorganisms use both light and chemical energy for the production of biomass. Microbial communities of these ecosystems are metabolically diverse and possess the capacity to transform a large range of chemical compounds. Yet, little is known about their diversity or factors shaping their structure or how they compare to coastal sediments not impacted by hydrothermalism. To this end, we have used automated ribosomal intergenic spacer analysis (ARISA) and high-throughput Illumina sequencing combined with porewater geochemical analysis to investigate microbial communities along geochemical gradients in two shallow-water hydrothermal systems off the island of Dominica (Lesser Antilles). At both sites, venting of hydrothermal fluids substantially altered the porewater geochemistry by enriching it with silica, iron and dissolved inorganic carbon, resulting in island-like habitats with distinct biogeochemistry. The magnitude of fluid flow and difference in sediment grain size, which impedes mixing of the fluids with seawater, were correlated with the observed differences in the porewater geochemistry between the two sites. Concomitantly, individual sites harbored microbial communities with a significantly different community structure. These differences could be statistically linked to variations in the porewater geochemistry and the hydrothermal fluids. The two shallow-water hydrothermal systems of Dominica harbored bacterial communities with high taxonomical and metabolic diversity, predominated by heterotrophic microorganisms associated with the Gammaproteobacterial genera Pseudomonas and Pseudoalteromonas, indicating the importance of heterotrophic processes. Overall, this study shows that shallow-water hydrothermal systems contribute substantially to the biogeochemical heterogeneity and bacterial diversity of coastal sediments.
Hydrothermal systems represent extreme environments in the ocean, where reduced and often acidic fluids of high temperature, laden with heavy and toxic metals exit the seafloor. Hydrothermal systems generally exhibit two types of fluid discharge: (1) focused discharge from discrete hydrothermal vents and (2) dispersed or diffuse discharge of fluids (Pichler and Veizer, 1999; Price et al., 2007; Dekov et al., 2010). Diffuse flow hydrothermal systems are characterized by low temperature fluids (<0.2 to ~100°C), composed of mixtures of cold seawater and magmatically heated fluids, slowly discharging through sulfide mounds, fractured lava flows, bare sediments and assemblages of bacterial mats and macrofauna (Bemis et al., 2012). Particularly diffuse venting through sediments in hydrothermal areas creates unique environments with well-developed vertical and lateral (physico)geochemical gradients at scales ranging from a few millimeters to several meters, i.e., with steep and gentle slopes (Price et al., 2007; Karlen et al., 2010). In the uppermost sediment layers, mixing of ascending hydrothermal fluids rich in electron-donors with cold ambient seawater full of electron-acceptors, provides chemical energy for microorganisms (Amend and Shock, 1998). Moreover, due to the mixing of these two water bodies, dissolved compounds precipitate and form energy-rich solid surfaces that can be exploited by microorganisms (e.g., Holden et al., 2012). Microorganisms of hydrothermal ecosystems have the capacity to transform a large range of chemical compounds derived from hydrothermalism through different redox coupling reactions (Fisher et al., 2007; Jørgensen and Boetius, 2007), and to fix carbon via chemosynthesis (Sievert and Vetriani, 2012). These microbial communities possess diverse metabolisms and are capable of oxidizing, e.g., H2, H2S, CH4, Fe2+, NH3 and Mn2+ using variety of electron acceptors, e.g., O2, , , Fe3+ (Fisher et al., 2007; Kato et al., 2012). This promotes the formation of hydrothermal ecosystems with high productivity and biomass, as well as specialized biodiversity (Jørgensen and Boetius, 2007).
Hydrothermal ecosystems have a global distribution from the shallow-water coastal regions to the abyssal plain, occurring on divergent boundaries at mid-ocean ridge systems and convergent boundaries associated with seamounts and island arc volcanoes (Tarasov et al., 2005; Price and Giovannelli, 2017). Shallow-water systems differ from the well-known deep-sea systems in that they lack the emblematic deep-sea vent megafauna and are situated within the photic zone, at water depths shallower than ~200 m, where light still reaches the seafloor (Tarasov et al., 2005). Therefore, in addition to chemosynthetic processes, microbial communities of shallow-water systems may also use light for the production of biomass via photosynthesis, resulting in high biological productivity and likely even more complex microbial communities relative to the deep-sea counterparts (Wang et al., 2015). Despite this, little is known about the ecology of microbial communities of shallow-water hydrothermal systems and they remain largely understudied compared to their deep-sea counterparts (Dawson et al., 2016; Miranda et al., 2016; Gomez-Saez et al., 2017).
Archaea and Bacteria are important members of the communities at shallow-water hydrothermal systems, because they provide the bases of the food webs at these localities and are responsible for the transformation of compounds transported by the venting fluids (Sievert et al., 2000; Lentini et al., 2014). Archaea dominate the hottest areas at hydrothermal vents, while the relative contribution of bacteria is higher in the areas with lower temperatures (Rothschild and Mancinelli, 2001; Price and Giovannelli, 2017). Studies on shallow-water hydrothermal systems using high-throughput sequencing are still few (Price and Giovannelli, 2017 and references therein), and thus the ecology and in specific the extent of microbial diversity in these ecosystems remains poorly understood. Metabolically and taxonomically diverse bacteria dominate individual shallow-water hydrothermal systems, suggesting a high degree of heterogeneity among these ecosystems (Gugliandolo et al., 2012; Zhang et al., 2012; Giovannelli et al., 2013; Lentini et al., 2014; Price and Giovannelli, 2017 and references therein). The factors, however, selecting for the different bacterial communities and thus promoting high heterogeneity remain elusive. The overall aim of this study was to contribute knowledge about the microbial ecology of shallow-water hydrothermal ecosystems, and to assess their diversity and the biogeochemical factors shaping the communities in these ecosystems.
We investigated sediments from two marine shallow-water hydrothermal systems off the island of Dominica (Lesser Antilles). Given the relatively moderate temperatures of the Dominica shallow-water hydrothermal systems (55 and 75°C), the main focus of this study was on the bacterial part of the microbial communities. Our study is the first report on the natural bacterial communities not only of Dominica, but also for the whole Lesser Antilles arc. Here, we combined fingerprinting Automated Ribosomal Intergenic Spacer Analysis (ARISA) and Illumina sequencing techniques with porewater geochemistry analyses to gain understanding of the diversity of the bacterial communities in the context of their biogeochemical surroundings. For comparison, in addition we sampled two areas that were not impacted by fluid venting. The main questions addressed here are: (i) how do the biogeochemistry and associated bacterial communities of shallow-water hydrothermal sediments compare to those not impacted by hydrothermal fluids, (ii) which factors shape the bacterial diversity of hydrothermally-influenced sediments, and (iii) do individual shallow-water hydrothermal systems promote different bacterial diversity.
In April 2013, two shallow-water hydrothermal areas (5 m water depth) in the southwest of Dominica Island, i.e., Champagne Hot Springs (CHSHT; N 15.245846, W 61.373125) and Soufrière Bay (SOUHT; N 15.232771, W 61.362047) were investigated (Figure 1A). Dominica is part of the volcanically active Lesser Antilles arc system and is uniquely characterized by a volcanic complex with potentially nine active centers, both terrestrial and marine (Lindsay et al., 2005; Joseph et al., 2011). Hydrothermally-impacted sampling sites were visually identified via SCUBA diving, through the observation of gas bubbles, discharge of hydrothermal fluid from discrete vents and diffusive venting through the surrounding sediments. Hydrothermal sites were located in the vicinity of local villages and a substantial anthropogenic influence could be visually identified at both sites. The sediments in the vicinity of hydrothermal venting at both sampling sites were covered by a layer of reddish hydrous ferric oxide precipitates (Figures 1B,C; see also McCarthy et al., 2005). Visually, Soufrière appeared to be more active, due to stronger gas bubbling. SCUBA diving surveys revealed that the hydrothermal activity at the Champagne site seemed to have declined compared to previous years (Pichler, unpublished observations). The fluids at the two sites were characterized by elevated temperatures (55–75°C) and Fe2+ concentrations (5–13 mg L−1), as well as decreased pH (6.3) and salinity (11–17‰) compared to seawater (Table 1) (Kleint et al., 2015, 2017).
Figure 1. (A) Map of Dominica Island displaying the two investigated areas (modified after Gomez-Saez et al., 2017). (B,C) In situ photos depicting the sediment conditions at Soufrière and Champagne hydrothermal sites. Orange to red color of the seafloor is due to the Fe-precipitates that overly the sediment at both sites. Photos courtesy of A. Madisetti (April 2013).
Table 1. Geochemical characteristics of hydrothermal fluids and bottom water at the Champagne and Soufrière hydrothermal sites (HT) compared to their respective background samples (BG) and seawater.
For comparison, we investigated background sediments (CHSBG and SOUBG) from both sampling sites that were not impacted by hydrothermalism upon the sampling.
Prior to sampling, temperature measurements were performed at all sampling sites (5 cm resolution, max. 25 cm depth), using a custom-made underwater electric temperature probe. Sediments were retrieved by SCUBA divers using hand operated polycarbonate push cores (∅ 7.5 cm, length 30 cm). Immediately after recovery, the sediments were extruded from the push cores, incrementally sliced in 2-cm resolution using sterile equipment, and subsampled for different types of analyses.
At both sampling locations, Soufrière and Champagne, we sampled hydrothermal fluid from discrete vents, bottom seawater and porewater from hydrothermally-impacted sediments. Hydrothermal areas were identified by the elevated sediment temperature compared to the background, emergence of gas bubbles and reddish precipitates of ferric oxide. To serve as background data for comparison, we also collected porewater and seawater away from the area of hydrothermal activity. The bottom water samples were a mixture of hydrothermal fluid and seawater collected just above the sediment-seawater interface. The bottom water and hydrothermal fluids were collected into 60 mL syringes. Temperature of fluids was measured in situ with a custom-made temperature probe, while pH, salinity and dissolved oxygen were measured on shore in sample aliquots using a WTW® multimeter (Weilheim, Germany). Porewater was collected from sediment cores and extracted in 2-cm steps (max. 20 cm sediment depth), using Rhizon moisture samplers with a pore size 0.1 μm (Rhizosphere Research Products, Wageningen, Netherlands) (Seeberg-Elverfeldt et al., 2005), which were inserted into holes of the push core liners. Samples were immediately separated into aliquots for the determination of total sulfide (totS2−), dissolved organic carbon (DOC), dissolved inorganic carbon (DIC), anion and cation concentrations. Total sulfide was determined spectrophotometrically (Pharo 100 Spectroquant, Merck KGaA, Darmstadt, Germany) on subsamples that were fixed with 5% zinc acetate (Cline, 1969). DOC subsamples were pre-filtered with 0.2 μm PES filters, acidified with suprapure HCl to pH = 2 and stored in sealed precombusted glass vials in the field. In the laboratory, DOC concentration was determined on a TOC analyzer (multi N/C® 2100S, Analytik Jena, Germany). Subsamples for total iron (FeT), potassium (K+), magnesium (Mg2+), total manganese (MnT), boron (H3), calcium (Ca2+), sodium (Na+), strontium (Sr2+) and silica (H4) were acidified to 1% with ultrapure HNO3 in the field, and stored at 4°C until measured by inductively coupled-optical emission spectrometry (Optima 7300 ICP-OES; Perkin-Elmer, Rodgau, Germany) following established procedures (Pichler et al., 2004). Measurements of anion concentrations, i.e., chloride (Cl−) and sulfate () were performed by ion chromatography (Metrohm 883 IC Basic Plus; Metrohm AG, Herisau, Switzerland) on previously frozen (−20°C) subsamples. All geochemical data is archived in PANGAEA, Data Publisher for Earth & Environmental Science and is publically available (Pop Ristova et al., 2017b).
Samples used for determination of sediment grain sizes were frozen in the field (−20°C). In the laboratory, sediment grain sizes were determined by sieving of dried sediments with different mesh sizes i.e., 0.5, 0.25, 0.125, and 0.063 mm.
Analysis of the bacterial community was performed on the same sediment cores used for geochemical analysis. Sediments were sliced in 2-cm resolution down to a maximum depth of 20 cm. Sediment samples were preserved in a DNAse- and RNAse-free LifeGuard® Soil Preservation Solution (MoBio Laboratories, Inc., Carlsbad, CA, USA) and stored at −20°C till further processing in the home laboratory.
DNA was extracted from 0.5 g of sediment using the FastDNA®SPIN Kit for Soil (MP Biomedicals, Irvine, USA), following manufacture's protocol with small modifications. Modifications of the protocol included two additional steps: (i) incubation of samples in a water bath at 65°C for 10 min, after protocol's step four of homogenization, and (ii) incubation of samples in water bath at 37°C for 15 min, during protocol's step 16 of final DNA elution. DNA was finally eluted in 50 μl 1 × Tris-EDTA buffer (Promega, Madison, WI, USA).
Changes in the bacterial community were assessed with two molecular techniques, i.e., Illumina sequencing and Automated Ribosomal Intergenic Spacer Analysis (ARISA). Standardized amounts of DNA (10 ng) from each sample were amplified in triplicates using the ARISA-specific ITSReub and ITSF (Hex-labeled) primers (Fisher and Triplett, 1999; Cardinale et al., 2004). The PCR procedure, cleaning of PCR products and separation of amplified fragments via capillary electrophoresis were done as described previously (Ramette, 2009), with the exception that here we used PeqLab dNTPs and peqGOLD Taq DNA Polymerase (PeqLab Biotechnology, Erlangen, Germany). Fragments between 100 and 1,000 bp length were binned into ARISA operational taxonomic units (OTUs; 2 bp window frame) using customized scripts, publically available at https://www.mpi-bremen.de/en/Software-4.html#section1549. PCR triplicates were merged by retaining all fragments present in at least two of the three replicates.
A selection of samples from each site, encompassing the following depth intervals 0–2 cm, 2–4 cm, 8–10 cm, 16–18 cm, were analyzed by Illumina MiSeq sequencing technology. The V3–V4 hypervariable region of the 16S rRNA gene was sequenced using the S-D-Bact-0341-b-S-17 (5′-CCTACGGGNGGCWGCAG-3′) and S-D-Bact-0785-a-A-21 (5′-GACTACHVGGGTATCTAATCC-3′) bacterial primers (Klindworth et al., 2013). Sequencing was performed with the Illumina MiSeq (pair-end technology) at MR DNA laboratory (Molecular Research LP, Shallowater, TX, US). Multifasta files were parsed, checked for quality, and trimmed with the split_libraries.py command as implemented in QIIME v1.9.1 (Caporaso et al., 2010). Processing of raw sequences, including alignment, quality control, dereplication, clustering and classification, was done with the SILVAngs analysis pipeline 1.2 (SILVA SSU Ref dataset 119.1; Quast et al., 2013). EzBioCloud was used to assign pairwise similarity to the most abundant OTUs against their closest (un)cultured relatives (Yoon et al., 2017). Operational taxonomic units (OTU0.03) were formed by clustering the sequences at 97% sequence's identity level. Sequence fastq files were deposited at the GenBank Sequence Read Archives (http://www.ncbi.nlm.nih.gov), and are publically available under the BioProject ID: PRJNA386676.
All analyses, except alpha diversity calculations, were done on the dataset with removed singleton sequences, i.e., OTU0.03 represented by only one sequence within the whole dataset. OTUs that were classified only to the taxonomical level of family, as well as those matching chloroplast and mitochondrial sequences were excluded from the analysis.
Differences between sampling sites, based on their geochemistry or bacterial community structure, were determined and visualized with Principal Component Analysis (PCA; based on Euclidean distance) and nonmetric multidimensional scaling analysis (NMDS; based on Bray-Curtis distance), respectively. Separation of groups identified on the NMDS was tested for significance using the non-parametric Analysis of Similarity (ANOSIM) test. Individual and combined effects of the measured geochemical variables on the structure of microbial communities were assessed by redundancy analysis (RDA) and canonical variation partitioning. Priorly individual geochemistry variables were checked for co-linearity (Supplementary Table 1). Most of the geochemical parameters were significantly correlated with H4. Silica was thus selected and kept in the RDA, as this parameter represents a good proxy for the influence of hydrothermalism. Prior to the RDA the geochemical data were normalized (logarithmic transformation) and standardized (z-score transformation), while the bacterial dataset was Hellinger transformed, as recommended for these types of datasets (Ramette, 2007). All analyses of the beta-diversity, i.e., differences in the microbial community structure between sites, were done on the ARISA-based dataset.
Alpha diversity (i.e., OTU0.03 richness) of each of the samples was determined by calculating the Chao1, ACE and Shannon diversity indices, based on the Illumina sequences dataset. The sequence dataset was previously normalized to the sample with the smallest number of sequences (CHSHT_8–10 cm, 33479; Table 2). Difference in alpha diversity between hydrothermal and background samples was tested for significance using the Kruskal Wallis and Mann Whitney U test for unmatched samples. All statistical analyses were performed with the R software, using vegan (Oksanen et al., 2015) and ggplot2 (Wickham, 2009) packages, as well as custom-based scripts.
The bottom waters at the background sites were similar to the seawater with a temperature of 29.5°C, a salinity of 33‰ and a pH between 7.6 and 7.9 (Table 1). In contrast, bottom water at the hydrothermal sites showed a clear hydrothermal impact, with decreased pH (6.4) and salinity (13‰ and 14‰) as well as elevated temperatures (46°C and 55°C). The FeT concentrations were elevated at the venting sites (6–11 mg L−1), but hardly detectable in the background samples.
Principal component analysis showed that the porewater in the area of venting had a substantially different chemical composition compared to the background sites (Supplementary Figure 1). This difference was slightly greater at the SOUHT than at the CHSHT site. Sediment porewaters from the area of venting had increased concentrations of H3, FeT, H4 and DIC, as well as decreased levels of , Cl−, Na+, K+, and Mg2+ compared to their respective background sites (Figure 2, Supplementary Figure 2). Sulfide was not detected in the porewater at any site, although small amounts of dissolved totS2− were detected in the hydrothermal fluids (0.44 mg L−1).
Figure 2. Depth profiles of (A) calcium, (B) chloride, (C) dissolved inorganic matter, (D) dissolved organic matter, (E) silica, (F) sulfate, (G) temperature, and (H) iron.
Most of the investigated geochemical parameters at both sites were linearly related with seawater and the hydrothermal fluid from the on-land hot springs, i.e., Sulfur Springs (McCarthy et al., 2005) (Supplementary Figure 3). Similar to McCarthy et al. (2005) we used seawater and the terrestrial Sulfur Spring fluids as respective end-members. The intermediate position of the SOUHT and CHSHT porewater and hydrothermal fluids between the two end-members (Supplementary Figure 3) indicates that mixing is the main control for their chemical composition. The only exceptions were Ca2+, and H3 at CHSHT and and H3 at SOUHT (Supplementary Figure 3).
Based on all measured parameters the two hydrothermal sites had a substantially different hydrothermal fluid and porewater geochemistry (Figure 2, Supplementary Figure 1). Temperature rapidly increased with sediment depth at both hydrothermal sites, and reached maximum values in the deepest investigated layer (56.2 ± 0.4°C at SOUHT and 48.3 ± 0.5°C at CHSHT at 25 cm; Figure 2). On average the temperature at SOUHT was 9°C higher than at CHSHT. Showing highly similar depth patterns, Cl− and concentrations were low over the entire investigated depth at CHSHT (Cl− = 7,322 ± 946 mg L−1 and = 1,053 ± 115 mg L−1), while at SOUHT they dropped from 18,422 mg L−1 and 2,413 mg L−1 in the surface to 10,975 mg L−1 and 1,441 mg L−1 at 8–10 cm depth, respectively. Silica was on average two times more concentrated in the porewater of SOUHT (60 ± 3 mg L−1) than at CHSHT (35 ± 12 mg L−1). SOUHT had also higher DIC concentration (max. 805 mg L−1) compared to CHSHT (max. 593 mg L−1), nevertheless both sites showed similar pattern of rapid DIC increase in the top 8 cm of the sediment.
Iron could be detected in only a few samples at the CHSHT, where it remained below 3 mg L−1 (Figure 2), while almost five times higher concentrations were measured throughout the whole investigated depth at the SOUHT (max. 16 mg L−1). Calcium concentrations decreased in the surface layers at the SOUHT (337 to 238 mg L−1) and they were lower over the entire investigated depth compared to CHSHT (407 ± 18 mg L−1), which in turn had similar values as the background samples. Dissolved organic carbon (3–13 mg L−1) showed no clear pattern with sediment depth and it did not differ between the investigated sites.
The grain size of the sediments at SOUHT was smallest (0.07 mm), and about half the size compared to the other sites (Supplementary Figure 4).
Differences in the alpha diversity (estimated OTU0.03 richness) between individual samples, based on all calculated species estimated indices were large. Lowest Chao1 of 18,182 was detected for CHSHT_8–10 cm and almost three times higher for the SOUBG_8–10 cm sample (Chao1 = 49,965; Table 2). At both hydrothermal sites alpha diversity did not vary with sediment depth, and all hydrothermal samples had in general lower estimated species diversity compared to the respective background sediments (Chao1 = 36,786 ± 12,486 at background sites and Chao1 = 27,772 ± 10,743 at the hydrothermal sites). Differences in the alpha diversity between hydrothermal and background sites were statistically significant (Wilcox test W = 64, p < 0.001).
The bacterial community structure of SOUHT was statistically and significantly different compared to the near-by background site (SOUBG), as revealed by the NMDS analysis and the ANOSIM test (Figure 3, Table 3). In contrast, no such pattern was revealed at the Champagne area, where the bacterial communities of the hydrothermal and the background sites were highly similar and had overlapping structures. Furthermore, the structure of the bacterial communities at SOUHT was statistically different compared to the other hydrothermal CHSHT site.
Figure 3. Nonmetric multidimensional scaling (NMDS) analysis, based on Bray-Curtis dissimilarity, revealing differences in the bacterial community structure between SOUHT and all other investigated sites. Samples are color coded according to the sampling sites. Stress level = 19%.
Beta-diversity, i.e., differences in the microbial community structure between sites (including hydrothermal and background samples), was significantly correlated to differences in most of the investigated geochemical parameters, i.e., , DIC, H4, Cl−, K+, Mg2+ (Figures 4A–D, Supplementary Table 2) between the investigated sites. No significant correlation between beta-diversity and temperature could be found. The significant effect of the individual environmental factors on the bacterial community structure was confirmed by redundancy (RDA) and variation partitioning analysis (Figure 4E). The overall RDA model significantly explained 12% (p-value = 0.001) of the total variation in the bacterial communities at Dominica, of which 9% were due to overall differences in the sediment geochemistry between the sites (Figure 4E). Silica was the only important geochemical variable, which explained 8% (p = 0.001) of the variation in the bacterial community structures at Dominica. In contrast, DIC as well as the sediment depth did not significantly contribute to the overall model, and thus did not play any role in structuring the bacterial communities.
Figure 4. Correlation plots depicting positive relation between beta-diversity and differences in (A) DIC, (C) silica and (D) sulfate concentrations, but not (B) DOC (see also Supplementary Table 2 for associated Mantel test results). Solid line represents a LOWESS curve (locally weighted scatterplot smoothing). (E) Variation partitioning analysis. ***Denotes significant p-value.
Overall 58 phyla, 120 classes, and 732 genera were identified in this study. In general, slightly lower numbers of taxa were retrieved at the hydrothermal compared to their respective background sites at the taxonomical levels of class, family and genus (Supplementary Table 3).
All samples, including hydrothermal and background sites, were dominated by Proteobacteria (47–72%) and to lesser extent by Chloroflexi (7–21%) (Figure 5). Gammaproteobacteria was the most abundant class at all investigated sites in this study, comprising 59 % of the total number of sequences, (Figure 5). Pseudomonas (28%) and Pseudoalteromonas (26%) of Gammaproteobacteria were the most sequence abundant genera in the whole dataset. A large number of sequences belonging to these genera were related to Pseudomonas zhaodongensis (99.6% similarity) and Pseudoalteromonas undina (98.4% similarity; previously Alteromonas undina) (Table 4, Supplementary Table 4).
Figure 5. Comparison of the most sequence abundant phyla and classes (top five), as well as genera (top ten) at the hydrothermal and background sites.
Table 4. Twenty most abundant sequences in the whole dataset, including information on their closest relatives and pairwise similarity.
Differences between the hydrothermal and the background sites were larger at the Soufrière compared to Champagne at all taxonomical levels (Figure 5). SOUHT had substantially higher abundance of Bacilli and Deferribacteres, and low proportion of Actinobacteria, Bacteroidetes, and Epsilonproteobacteria compared to all other sites. In contrast, Deltaproteobacteria, Acidimicrobiia, and Alphaproteobacteria classes had relatively higher abundance in background sediments, but also at the Champagne hydrothermal site.
Few of the most abundant sequences belonged to Bacilli, and were closely related to Planococcus maritimus (99.5% similarity), Exiguobacterium aurantiacum (99.7% similarity) and Marinilactibacillus piezotolerans (98.7% similarity) (Supplementary Table 4). Sequences related to Planococcus maritimus were substantially more abundant at SOUHT, while those related to Exiguobacterium aurantiacum and Marinilactibacillus piezotolerans dominated the CHSHT. Other abundant genera at SOUHT included Thermoflexus (Chloroflexi), Caldithrix (Calditrichaeota), Desulfatiglans and Dissulfurirhabdus (Deltaproteobacteria).
Differences in the taxonomical composition between the two hydrothermal sites were larger compared to the differences between the background sites, as only 26% of the total genera were found at both hydrothermal sites, while more than double (61%) were shared by the two background sites. Of the most sequence abundant genera, Caldithrix and Exiguobacterium, along with Pseudomonas and Pseudoalteromonas, were found at both hydrothermal sites but with substantially different sequence abundances. Highly abundant genera at the background sediments included two genera of the Desulfobacteraceae (Sva0081_sediment group and SEEP-SRB1), Cobetia (Gammaproteobacteria), Spirochaeta and Sulfurovum (Epsilonproteobacteria).
In this study we investigated seawater, hydrothermal fluid and sediment porewater at two sites with hydrothermal activity, the Champagne Hot Springs (CHSHT) and Soufrière Bay (SOUHT). The two sites were located at approximately 5 m water depth, and compared to other sites of hydrothermal activity, had intermediate temperatures between 46°C and 75°C (e.g., Pichler and Veizer, 1999). Their pH was slightly acidic and their chemical composition was intermediate between their respective end-members (Supplementary Figure 3). This position indicates that the hydrothermal fluids are a mixture of meteoric water and seawater (e.g., McCarthy et al., 2005; Gomez-Saez et al., 2015). As shown for other shallow-water hydrothermal systems (e.g., Wenzhöfer et al., 2000; Price et al., 2007; Yücel et al., 2013), mixing with hydrothermal fluids substantially altered the porewater geochemistry, which created distinct island-like habitats characterized by elevated temperatures (42°C to 56°C), higher amounts of H4 and H3 and lower concentrations of , Cl−, K+, Mg2+, and Na+ in comparison to the surrounding sediments (Figure 2, Supplementary Figure 2). The porewater was also enriched with electron donors, i.e., FeT and to a certain extent totS2− (this study and McCarthy et al., 2005), as well as DIC, which can in turn promote microbial processes such as chemotrophy, as well as overall benthic primary productivity. In line with this, Kleint et al. (2017) found that the fluids of Dominica were highly enriched in stabilized and bioavailable Fe2+, due to same mechanisms detected at deep-sea hydrothermal systems (Bennett et al., 2008; Hawkes et al., 2013), i.e., strong complexation of Fe2+ with dissolved organic carbon. Upon contact with oxygenated seawater, iron precipitated out of the fluids, and formed extensive hydrous ferric oxide reddish layers that covered the venting areas (Figure 1), similar to the ones found at deep-sea (Fleming et al., 2013) and other shallow-water hydrothermal systems (e.g., Pichler and Veizer, 1999; Handley et al., 2010). These hydrothermal precipitates represent energy-rich solid surfaces that provide micro-niches and energy sources to microorganisms, otherwise not present at the background sediments of Dominica or, generally, in coastal sediments.
Differences in the overall porewater geochemistry between the two hydrothermal sites were higher than between the two background sites (Supplementary Figure 1), indicating that activity of shallow-water hydrothermal systems can contribute substantially to the physicochemical and biogeochemical heterogeneity of coastal sediments.
End-member analysis revealed that fluids at both hydrothermal sites were the product of mixing between meteorically derived hydrothermal fluids (i.e., Sulfur Springs) and seawater, and that the amount of mixing between hydrothermal fluid and seawater controlled the geochemistry of the porewater (see also McCarthy et al., 2005; Supplementary Figure 3). Despite the geographic proximity and the same origin of the hydrothermal fluids, Champagne and Soufrière hydrothermal systems had substantially different porewater geochemistry (Figure 2, Supplementary Figure 1). Silica and Mg2+ concentrations are generally excellent indicators for the amount of hydrothermal component in a mixture between seawater and hydrothermal fluid (e.g., Pichler and Veizer, 1999). The hydrothermal fluid at SOUHT had a higher temperature, higher H4 and lower Mg2+ concentrations, which indicated a larger hydrothermal component at this system compared to CHSHT. This observation was also true for the hydrothermally-influenced porewater at both sites. The hydrothermal component in the sediment porewater was larger at SOUHT than at CHSHT. These differences are likely due to greater hydrothermal activity (as evidenced by more gas bubbling) or decreased rates of mixing of fluids with seawater due to finer sediments at SOUHT (Supplementary Figure 4). The pronounced difference in Ca2+ between the two sites, i.e., CHSHT had higher concentrations than can be explained by the mixing model (Supplementary Figure 3A), is likely a result of the proximity of CHSHT to a coral reef and thus abundance of CaCO3 minerals in the sediment. At a pH of 6.3 aragonite and calcite are readily dissolved (Pichler and Veizer, 2004). The same behavior could also be seen for the variation of Sr2+, which is also supplied to the porewater and hydrothermal fluid through the dissolution of CaCO3 minerals (Supplemental Figure 3A). The deviation of values for Sulfur Springs from the respective CHSHT and SOUHT mixing lines (Supplemental Figure 3) is caused by oxidation of H2S and SO2 to by atmospheric oxygen. Sulfur Springs discharges inland and thus its hydrothermal fluid has extensive contact with the atmosphere prior to sampling. At the SOUHT site precipitation of gypsum (CaSO4) and thus removal of could have an additional effect on the concentration. This, however, was not directly observed, due to the lack of a detailed mineralogical examination. Nevertheless, it is common occurrence because, above 70°C fluid temperature gypsum starts to precipitate in shallow-water hydrothermal systems (Pichler and Humphrey, 2001).
These results are in line with previous studies, which also showed large physicochemical and biogeochemical variations among individual hydrothermal sites (e.g., Zhang et al., 2012; Price et al., 2013b; Lentini et al., 2014). Overall, these findings reveal large variability, not only among shallow-water hydrothermal systems of different regions, but also at small spatial scales within a single volcanic region.
Most of the geochemical parameters measured at the two Dominica hydrothermal sites fall within the lower range compared to values reported from other shallow-water hydrothermal systems, i.e., off Panarea Island (Italy) (Maugeri et al., 2010), Milos Island (Greece) (Wenzhöfer et al., 2000; Price et al., 2013a; Yücel et al., 2013) and Kueishan Island (Taiwan) (Zhang et al., 2012; Wang et al., 2015). In line, although SOUHT is regarded as iron-rich (Gomez-Saez et al., 2017), the measured iron concentrations were a magnitude lower compared to similar sites elsewhere (Handley et al., 2010; Hoshino et al., 2016). Despite this, the measured DIC concentrations at CHSHT and SOUHT were up to five times higher than in other shallow-water hydrothermal systems (e.g., Robinson et al., 1997; Wenzhöfer et al., 2000; Zhang et al., 2012). These settings can thus potentially provide conditions for a comparatively enhanced photo- and chemolithotrophic production of organic matter, and consequently base for heterotrophic processes in the sediments of the Dominica shallow-water hydrothermal system.
Hydrothermal systems are regarded as extreme chemosynthetic environments because of the high levels of toxic metals in the emerging fluids, elevated temperatures, increased sulfide concentrations and often low pH (Amend and Shock, 1998; Tarasov et al., 2005; Price et al., 2013b). These extreme environmental conditions pose stress to many organisms and are believed to depress their ability to function (Rothschild and Mancinelli, 2001), resulting in a reduced number of highly adapted and specialized organisms which are able to inhabit these extreme environments. Thus, low diversity and high biomass of deep-sea hydrothermal systems compared to their surroundings is thought to be a universal phenomenon, applicable to all organismal groups from meio- and macro- to megafauna, found at extreme environments (Grassle and Maciolek, 1992; Van Dover, 2000; Ramirez-Llodra et al., 2007).
Here, we show that microorganisms follow the same pattern, in that both Champagne and Soufrière hydrothermal systems had significantly lower alpha diversity, as well as a lower number of taxa for most of the investigated taxonomical levels compared to the respective background sediments (Table 2). This is in agreement with previous studies, which has revealed lower diversity at the shallow-water vent outlet compared to the transition zones in the Aegean Sea (Sievert et al., 2000; Giovannelli et al., 2013). A contrasting trend was shown for the Espalamaca shallow-water hydrothermal system (Rajasabapathy et al., 2014), however this study used cultivation techniques, which are known to bias the diversity of microbial communities.
At both hydrothermal sites of Dominica, alpha diversity did not change with sediment depth, while at the background sediments a tentative increase with sediment depth was observed (Table 2). This result indicates that, unlike at coastal sediments (Böer et al., 2009 and references therein), at extreme chemosynthetic environments in general, i.e., at hydrothermal systems (this study) and cold seeps (Pop Ristova et al., 2012, 2015) the sediment depth likely does not influence the richness of the microbial communities. This is likely due to the flushing of sediments with fluids and the highly variable geochemistry of these ecosystems.
Only the Soufrière hydrothermal site, which was strongly influenced by hydrothermal fluids, bore distinct communities compared to the background sediments (Figure 3). At the less hydrothermally-impacted Champagne site, the community was more similar to the background sediments, likely due to the small differences in geochemistry between these two habitats. An alternative explanation for such high overlap in the bacterial community structure between the different habitats at Champagne can be the fact that the background site was located within a larger area of hydrothermally-impacted sediments, thus potentially representing a past or a temporally inactive hydrothermal site.
Despite differences in geochemistry and temperatures (29°C at the background and 56°C at the hydrothermal site at 25 cm), all hydrothermal and background samples were dominated by Gammaproteobacteria sequences related to Pseudomonas zhaodongensis and Pseudoalteromonas undina (Figure 5, Table 4). Similarly, Pseudoalteromonas undina has been has been previously isolated from a hydrothermal vent in the deep-sea (Rougeaux et al., 1996). Pseudomonas zhaodongensis is known from saline and alkaline soils (Zhang et al., 2015c), and has not been so far reported from shallow-water hydrothermal systems. However, several other species of the Pseudomonas genus have been isolated from deep-sea hydrothermal vents and hot springs (Wang et al., 2002; Liu et al., 2013; Peña et al., 2013). Due to their high genetic and metabolic potential for adaptation to different environmental conditions, e.g., variable temperature and pH regimes, these two genera can inhabit many diverse habitats ranging from soil to marine ecosystems, including also extreme environments such as hydrothermal vents (Mihailov et al., 2006; Mohandass et al., 2012). This can explain their high abundances in both hydrothermal and background sediments of Dominica.
Variation in the abundances of bacterial taxa between habitats, rather than the sheer presence or absences of taxonomical types, was found to be mainly responsible for the observed differences between hydrothermal and background sites (Figure 5). In comparison to background sediments, hydrothermal sites had relatively lower abundance of Deltaproteobacteria, Acidimicrobiia, and Alphaproteobacteria, but higher numbers of Bacilli and Deferribacteres. The latter two classes are common members of bacterial communities of deep and shallow-water hydrothermal systems, and similarly to this study have been found in larger numbers directly at the vents or at sites with higher temperatures (Takai et al., 2003; Vetriani et al., 2005; Handley et al., 2010; Takaki et al., 2010; Gugliandolo et al., 2012; Mohandass et al., 2012; Lentini et al., 2014; Rajasabapathy et al., 2014; Zhang et al., 2015b). Overall, the majority of bacteria detected in this study could inhabit wide ranges of temperatures, likely as an adaptation to the temporarily and spatially variable geochemical conditions at the Dominica hydrothermal systems.
Epsilonproteobacteria and sulfur-oxidizers of Gammaproteobacteria have been identified as the most widespread and abundant bacterial groups at shallow-water hydrothermal systems worldwide (Price and Giovannelli, 2017 and references therein). This is in striking contrast to our findings at the Dominica shallow-water systems, where Epsilonproteobacteria as well as other chemolithotrophs were only present in low numbers, and thus likely played a relatively smaller role at this ecosystem (Figure 5). In contrast to all other known shallow-water hydrothermal systems, Dominica hydrothermal sites were dominated by heterotrophic Gammaproteobacteria.
Despite differences in the sediment geochemistry, both investigated Dominica hydrothermal systems showed similar levels of estimated species richness (Table 2), with only slightly lower alpha diversity values detected at the cooler Champagne hydrothermal site (Shannon diversity CHSHT = 7 ± 1, SOUHT = 7.3 ± 0.5). Comparable estimated species richness have been reported from other shallow-water hydrothermal systems in the Mediterranean and off Taiwan (Shannon diversity 6–9.2) (Lentini et al., 2014; Wang et al., 2015). Based on Chao1 index for estimated species richness, Dominica shallow-water systems harbored higher alpha-diversity compared to their deep-sea counterparts (Ruff et al., 2015).
Reflecting on the heterogeneity in the porewater geochemistry, Champagne and Soufrière hydrothermal sites harbored bacterial communities with significantly different structures (Figure 3). Differences in the bacterial community structure were significantly correlated to variations in the porewater geochemistry and the magnitude of hydrothermal fluid influence (Figure 4). This expands the findings of previous studies that could show an indirect link between variations in the microbial communities and changes in sediment temperature at shallow-water hydrothermal systems (Sievert et al., 1999; Giovannelli et al., 2013). More specifically, the results of this study indicate that likely not only the rate of fluid flow, but more specifically the rate of mixing of hydrothermal fluids with seawater, which in itself depends on the grain size of the sediment (Supplementary Figure 4), can profoundly influence the structure of shallow-water hydrothermal microbial communities. Sediments at the SOUHT were more compact due to the smaller grain size, which impedes the advection of fluids and protrusion of seawater (Bear, 2013). Porewater geochemical parameters measured in this study explained overall a relatively small portion of the total variation in the bacterial community structure (12%; Figure 4E), indicating that other, yet unidentified factors, have an important influence on the microbial community structure of the shallow-water hydrothermal systems of Dominica.
Many of the bacterial taxa detected here were found over the entire range of measured temperatures (38–56°C), but had substantially different relative abundances at the individual hydrothermal sites and thus likely preferred niches with different temperature and specific geochemical conditions (Figure 5). Actinobacteria (Acidimicrobiia) and Alphaproteobacteria, which have been found at hydrothermal systems with higher temperatures (Lentini et al., 2014; Qin et al., 2015), here dominated the cooler Champagne site and favored sediments with relatively lower impact by hydrothermal fluids. In contrast, Chloroflexi (Anaerolinea and Thermoflexia), Aminicenantes, Nitrospira, Caldithrix, and Desulfatiglans were more numerous at the Soufrière site characterized by higher temperatures (47–56°C) and larger hydrothermal impact. These taxa are known from hot springs, deep and shallow-water hydrothermal systems with variable temperatures (Miroshnichenko et al., 2003, 2010; Davis and Moyer, 2008; Lebedeva et al., 2011; Nunoura et al., 2013; Farag et al., 2014; Lentini et al., 2014; Teske et al., 2014; Thiel et al., 2016; Cerqueira et al., 2017). Similar to other shallow-water hydrothermal systems, Bacilli were highly numerous and comprised the second most abundant class at both hydrothermal sites of Dominica (Dando et al., 1998; Maugeri et al., 2010; Cihan et al., 2012; Gugliandolo et al., 2012; Price et al., 2013a; Lentini et al., 2014). They were represented by sequences mainly related to Exiguobacterium aurantiacum, Marinilactibacillus piezotolerans, and Planococcus maritimus. This presents a first report of their occurrence in hydrothermal environments, however, their genera i.e., Exiguobacterium and Planococcus encompass numerous meso- and thermophilic members that have been identified or isolated from shallow-water and deep-sea hydrothermal vents (Crapart et al., 2007; Vishnivetskaya et al., 2009; Maugeri et al., 2010; Zhang et al., 2015a; Chen and Sun, 2017). At Dominica, different hydrothermal sites were dominated by different Bacilli genera: sequences related to Exiguobacterium and Marinilactibacillus dominated the cooler Champagne site, while Planococcus was among the 10 most abundant genera at the hotter Soufriére site. This result suggests an ecological species replacement of microbial members of the Bacilli at shallow-water hydrothermal systems, over a range of 38–56°C temperatures.
With only 26% of the genera common to both hydrothermal sites, while more than 61% to both background sites, each individual shallow-water hydrothermal site of Dominica hosted more unique diversity compared to background sediments. This study demonstrates that each individual shallow-water hydrothermal site within the larger hydrothermal area contributes to the overall biodiversity of coastal sediments.
Existence of steep geochemical and physical gradients, availability of different substrates in the hydrothermal fluids, as well as light and oxygen provide a variety of microniches to metabolically diverse microorganisms at shallow-water hydrothermal systems (e.g., Giovannelli et al., 2013). At the Dominica shallow-water systems we found high taxonomical diversity fueled by a heterogeneous underlying geochemistry, suggesting a versatile metabolic potential of the microbial communities at these sites.
Sulfide-oxidizing Epsilonproteobacteria represent the most dominant and ubiquitous group at shallow-water and deep-sea hydrothermal systems (Teske and Reysenbach, 2015 and references therein). At Dominica hydrothermal systems, we found Epsilonproteobacteria related to sequences from a sulphidic cold-seep site in Japan (95.9% similarity) as well as the sulfide-oxidizing Sulfurimonas autotrophica (98.9% similarity) (Inagaki et al., 2003; Arakawa et al., 2005) (Supplementary Table 4). However, in Dominica hydrothermal sediments, Epsilonproteobacteria comprised overall only a minor component of the hydrothermal communities (<1%). Although some sulfide was present in the venting fluids of Dominica (0.44 mg L−1; Gomez-Saez et al., 2015), likely it was not entirely available to the microbial communities. Sulfide could have escaped as a gas from the system (McCarthy et al., 2005) or it could have precipitate with Fe2+ to form iron sulfide and pyrite, which could in turn explain the low abundances of chemolithotrophic sulfide-oxidizers detected in this study.
Other chemolithotrophs present at the Dominica hydrothermal sites included microorganisms distantly related to Mariprofundus ferrooxydans (95.1% similarity; Zetaproteobacteria), which use reduced iron as their source of energy (Emerson et al., 2007, 2010) (Supplementary Table 4). The surface sediment layers of Dominica hydrothermal sites, where both Fe2+ from the fluids and oxygen from the seawater are available (see Figure 2), provided suitable environments for these microorganisms. Mariprofundus ferrooxydans has a global distribution and it has been reported from various habitats, ranging from deep-sea and shallow-water hydrothermal systems to coastal and brackish sediments (Handley et al., 2010; McAllister et al., 2011; McBeth et al., 2011; Ionescu et al., 2015; Hoshino et al., 2016). The number of sequences related to Mariprofundus ferooxydans detected here were rather low (<1%), however they showed increased abundances and were among the 10 most abundant genera in experiments using sediments from the same sites at Dominica and incubated with 13C-bicarbonate (Gomez-Saez et al., 2017). The same study detected even higher diversity of bacteria potentially involved in the cycling of iron, suggesting that microbial iron cycling plays an important role in the biogeochemistry of the Dominica shallow-water hydrothermal system. In line with this, at the level of the whole bacterial community, which was dominated by Chloroflexi (Anaerolinea), Deltaproteobacteria, Actinobacteria, Aminicenantes, and Caldithrix, the Dominica hydrothermal systems mostly resembled an iron-rich shallow-water hydrothermal sites off Japan (Hoshino et al., 2016) and to a certain extent off Santorini (Handley et al., 2010). Further chemolithotrophic taxa detected in this study belonged to Dissulfurirhabdus, which represented one of the 10 most abundant genera at the hydrothermal sites of Dominica. Sequences of this genus were distantly related to Dissulfurirhabdus thermomarina (88.5% similarity), a thermophilic microorganism that grows chemolithotrophically using molecular hydrogen and has been previously isolated from a shallow-water hydrothermal vent off Kuril Island in Russia (Slobodkina et al., 2016).
Although fluids of hydrothermal ecosystems are often depleted in sulfate (Tivey, 2007), due to mixing with seawater, the surface sediments of Dominica were not limited by sulfate and contained sufficient concentrations (Figure 2) to support microorganisms with sulfur-based metabolisms. Concomitantly, here we identified sequences distantly affiliated to sulfate-reducers Desulfatiglans aniline (91% similarity; Suzuki et al., 2014) and SEEP-SRB1 (98% similarity) of the Deltaproteobacteria (Supplementary Table 4). They were among the ten most abundant genera at the hydrothermal sites, but had highly variable abundances in the different hydrothermal samples (<1–10%; Figure 5). Desulfatiglans aniline and SEEP-SRB1 are widespread sulfate reducers that inhabit various chemosynthetic ecosystems, including hydrothermal vents, but also coastal and pelagic sediments, where they are involved in parts of the cycling of sulfur (Handley et al., 2010; Yanagawa et al., 2012; Frank et al., 2013; Pop Ristova et al., 2015, 2017a; Hoshino et al., 2016).
In comparison to other known shallow-water hydrothermal sites, a unique feature of the Dominica hydrothermal sites was the clear dominance of Gammaproteobacteria (encompassing 59 % of the sequences in the whole dataset), represented mainly by the two heterotrophic genera related to Pseudoalteromonas undina (26%) and Pseudomonas zhaodongensis (28%). In laboratory conditions both Pseudoalteromonas undina and Pseudomonas zhaodongensis display chemo-organotrophic characteristics (Chan et al., 1978; Zhang et al., 2015c). This is in line with field studies, which have shown that these genera occupy heterotrophic niches at hydrothermal ecosystems and are responsible for the reduction of various metals (Wang et al., 2002; Mihailov et al., 2006; Rathgeber et al., 2006; Peña et al., 2013). In addition, we have detected high abundance of heterotrophic Anaerolinea mainly related to sequences from Fe-rich hydrothermal sediments (Sekiguchi et al., 2003; Forget et al., 2010; Nunoura et al., 2013; Podosokorskaya et al., 2013), as well as sequences related to the heterotrophic Paracoccus sediminis (Pan et al., 2014) and the thermophilic chemo-organotrophic bacterium Caldithrix paleochoryensis isolated from a shallow-water hydrothermal site in Milos, Greece (97.3% similarity; Miroshnichenko et al., 2010) (Supplementary Table 4). Overall, these heterotrophic taxa, including Exiguobacterium, Planococcus, and Marinilactibacillus bacilli (Wei et al., 2017), comprised 78% of the sequences in the whole dataset. Clear predominance of heterotrophs indicates that heterotrophy is likely the most dominant metabolism at the hydrothermal systems of Dominica. This may be explained by the fact that both sites were anthropogenically influenced and were located in the vicinity of the coast (5–10 m away), as well as near a river outlet in the case of the Soufrière site. Such conditions are known to enhance heterotrophic processes at shallow-water hydrothermal systems, due to the extra allochthonous input of organic matter (Sievert et al., 2000). Therefore, Dominica shallow-water hydrothermal systems represent an ideal natural laboratory for the study of heterotrophy and its associated microorganisms under high temperatures, which remain understudied compared to autotrophic processes and specialist thermophilic bacteria (Mohandass et al., 2012).
Although heterotrophic microorganisms represented the largest and most important fraction of the microbial communities in this study, we could not detect any correlation between the concentration of dissolved organic carbon and the overall structure of microbial communities at the Dominica hydrothermal sites (Figure 4). Potentially, other aspects of the complex dissolved organic matter, e.g., the quality, which gets altered during hydrothermal circulation (Hawkes et al., 2014), and/or the composition or concentration of the individual organic components, might be more relevant factors for the structuring of the microbial communities. These should be the focus of future studies aiming to better understand heterotrophic processes and its corresponding microorganisms at hydrothermal ecosystems.
PP and SIB designed the research. PP, SIB, and TP carried out field sampling. PP and TP performed laboratory work. PP analyzed data and wrote the manuscript with help and input from SIB, TP, and MWF.
This study has been financially supported by the DFG Emmy-Noether Program (BU 2606/1-1) awarded to SIB, and by the University of Bremen (MWF).
The authors declare that the research was conducted in the absence of any commercial or financial relationships that could be construed as a potential conflict of interest.
We thank all the members of Dominica Scientific Expedition in April 2013 (J. Amend, C. Kleint, A. Koschinsky, M. Sollich, and S. Sztejrenszus). We are especially grateful to C. Kleint for providing help with DOC and DIC analyses. The Dominican Department of Fisheries and especially A. Magloire are gratefully acknowledged for granting sample permission. We thank A. Madisetti and O. Lugay for providing support with underwater photography and logistics, respectively. Special thanks to Christian Quast and the SILVA team for the help with sequence processing. We thank the editor and two reviewers for helpful comments on this manuscript.
The Supplementary Material for this article can be found online at: https://www.frontiersin.org/articles/10.3389/fmicb.2017.02400/full#supplementary-material
Amend, J. P., and Shock, E. L. (1998). Energetics of amino acid synthesis in hydrothermal ecosystems. Science 281, 1659–1662. doi: 10.1126/science.281.5383.1659
Arakawa, S., Mori, M., Li, L., Nogi, Y., Sato, T., Yoshida, Y., et al. (2005). Cold-seep microbial communities are more abundant at deeper depths in the Japan Trench land slope. J. Japanese Soc. Extrem. 4, 50–55. doi: 10.3118/jjse.4.50
Bemis, K., Lowell, R. P., and Farough, A. (2012). Diffuse flow on and around hydrothermal vents at mid-ocean ridges. Oceanography 25, 182–191. doi: 10.5670/oceanog.2012.16
Bennett, S. A., Achterberg, E. P., Connelly, D. P., Statham, P. J., Fones, G. R., and German, C. R. (2008). The distribution and stabilisation of dissolved Fe in deep-sea hydrothermal plumes. Earth Planet. Sci. Lett. 270, 157–167. doi: 10.1016/j.epsl.2008.01.048
Böer, S. I., Hedtkamp, S. I. C., Beusekom, J. E. E., van Fuhrman, J. A., Boetius, A., and Ramette, A. (2009). Time- and sediment depth-related variations in bacterial diversity and community structure in subtidal sands. ISME J. 3, 780–791. doi: 10.1038/ismej.2009.29
Caporaso, J. G., Kuczynski, J., Stombaugh, J., Bittinger, K., Bushman, F. D., Costello, E. K., et al. (2010). QIIME allows analysis of high-throughput community sequencing data. Nat. Methods 7, 335–336. doi: 10.1038/nmeth.f.303
Cardinale, M., Brusetti, L., Quatrini, P., Borin, S., Puglia, A. M., Rizzi, A., et al. (2004). Comparison of different primer sets for use in automated ribosomal intergenic spacer analysis of complex bacterial communities. Appl. Environ. Microbiol. 70, 6147–6156. doi: 10.1128/AEM.70.10.6147-6156.2004
Cerqueira, T., Pinho, D., Froufe, H., Santos, R. S., Bettencourt, R., and Egas, C. (2017). Sediment microbial diversity of three deep-sea hydrothermal vents southwest of the Azores. Microb. Ecol. 74, 332–349. doi: 10.1007/s00248-017-0943-9
Chan, K. Y., Baumann, L., Garza, M. M., and Baumann, P. (1978). Two new species of Alteromonas: Alteromonas espejiana and Alteromonas undina. Int. J. Syst. Bacteriol. 28, 217–222. doi: 10.1099/00207713-28-2-217
Chen, C., and Sun, L. (2017). Draft genome sequence of Exiguobacterium sp. HVEsp1, a thermophilic bacterium isolated from a deep-sea hydrothermal vent in the okinawa trough. Genome Announc. 5:e00253-17. doi: 10.1128/genomeA.00253-17
Cihan, A. C., Tekin, N., Ozcan, B., and Cokmus, C. (2012). The genetic diversity of genus Bacillus and the related genera revealed by 16s rRNA gene sequences and ardra analyses isolated from geothermal regions of Turkey. Braz. J. Microbiol. 43, 309–324. doi: 10.1590/S1517-838220120001000037
Cline, J. D. (1969). Spectrophotometric determination of hydrogen sulfide in natural waters. Limnol. Oceanogr. 14, 454–458. doi: 10.4319/lo.1969.14.3.0454
Crapart, S., Fardeau, M.-L., Cayol, J.-L., Thomas, P., Sery, C., Ollivier, B., et al. (2007). Exiguobacterium profundum sp. nov., a moderately thermophilic, lactic acid-producing bacterium isolated from a deep-sea hydrothermal vent. Int. J. Syst. Evol. Microbiol. 57, 287–292. doi: 10.1099/ijs.0.64639-0
Dando, P. R., Thomm, M., Arab, H., Brehmer, M., Hooper, L. E., Jochimsen, B., et al. (1998). Microbiology of shallow hydrothermal sites off Palaeochori Bay, Milos (Hellenic Volcanic Arc). Cah. Biol. Mar. 39, 369–372.
Davis, R. E., and Moyer, C. L. (2008). Extreme spatial and temporal variability of hydrothermal microbial mat communities along the Mariana island arc and southern Mariana back-arc system. J. Geophys. Res. Solid Earth 113, 1–17. doi: 10.1029/2007JB005413
Dawson, K. S., Scheller, S., Dillon, J. G., and Orphan, V. J. (2016). Stable isotope phenotyping via cluster analysis of NanoSIMS data as a method for characterizing distinct microbial ecophysiologies and sulfur-cycling in the environment. Front. Microbiol. 7:774. doi: 10.3389/fmicb.2016.00774
Dekov, V. M., Petersen, S., Garbe-Schönberg, C.-D., Kamenov, G. D., Perner, M., Kuzmann, E., et al. (2010). Fe–Si-oxyhydroxide deposits at a slow-spreading centre with thickened oceanic crust: the Lilliput hydrothermal field (9°33′S, Mid-Atlantic Ridge). Chem. Geol. 278, 186–200. doi: 10.1016/j.chemgeo.2010.09.012
Emerson, D., Fleming, E. J., and McBeth, J. M. (2010). Iron-oxidizing bacteria: an environmental and genomic perspective. Annu. Rev. Microbiol. 64, 561–583. doi: 10.1146/annurev.micro.112408.134208
Emerson, D., Rentz, J. A., Lilburn, T. G., Davis, R. E., Aldrich, H., Chan, C., et al. (2007). A novel lineage of proteobacteria involved in formation of marine Fe-oxidizing microbial mat communities. PLoS ONE 2:e667. doi: 10.1371/journal.pone.0000667
Farag, I. F., Davis, J. P., Youssef, N. H., and Elshahed, M. S. (2014). Global patterns of abundance, diversity and community structure of the Aminicenantes (Candidate Phylum OP8). PLoS ONE 9:e92139. doi: 10.1371/journal.pone.0092139
Fisher, C., Takai, K., Bris, N., and Le (2007). Hydrothermal vent ecosystems. Oceanography 20, 14–23. doi: 10.5670/oceanog.2007.75
Fisher, M. M., and Triplett, E. W. (1999). Automated approach for ribosomal intergenic spacer analysis of microbial diversity and its application to freshwater bacterial communities. Appl. Environ. Microbiol. 65, 4630–4636.
Fleming, E. J., Davis, R. E., Mcallister, S. M., Chan, C. S., Moyer, C. L., Tebo, B. M., et al. (2013). Hidden in plain sight: discovery of sheath-forming, iron-oxidizing Zetaproteobacteria at Loihi Seamount, Hawaii, USA. FEMS Microbiol. Ecol. 85, 116–127. doi: 10.1111/1574-6941.12104
Forget, N. L., Murdock, S. A., and Juniper, S. K. (2010). Bacterial diversity in Fe-rich hydrothermal sediments at two South Tonga Arc submarine volcanoes. Geobiology 8, 417–432. doi: 10.1111/j.1472-4669.2010.00247.x
Frank, K. L., Rogers, D. R., Olins, H. C., Vidoudez, C., and Girguis, P. R. (2013). Characterizing the distribution and rates of microbial sulfate reduction at Middle Valley hydrothermal vents. ISME J. 7, 1391–1401. doi: 10.1038/ismej.2013.17
Giovannelli, D., D'Errico, G., Manini, E., Yakimov, M., and Vetriani, C. (2013). Diversity and phylogenetic analyses of bacteria from a shallow-water hydrothermal vent in Milos island (Greece). Front. Microbiol. 4:184. doi: 10.3389/fmicb.2013.00184
Gomez-Saez, G. V., Pop Ristova, P., Sievert, S. M., Elvert, M., Hinrichs, K.-U., and Bühring, S. I. (2017). Relative importance of chemoautotrophy for primary production in a light exposed marine shallow hydrothermal system. Front. Microbiol. 8:702. doi: 10.3389/fmicb.2017.00702
Gomez-Saez, G. V., Riedel, T., Niggemann, J., Pichler, T., Dittmar, T., and Bühring, S. I. (2015). Interaction between iron and dissolved organic matter in a marine shallow hydrothermal system off Dominica Island (Lesser Antilles). Mar. Chem. 177, 677–686. doi: 10.1016/j.marchem.2015.10.003
Grassle, F. J., and Maciolek, N. J. (1992). Deep-sea species richness: regional and local diversity estimates from quantitative bottom samples. Am. Nat. 139, 313–341. doi: 10.1086/285329
Gugliandolo, C., Lentini, V., Spanò, A., and Maugeri, T. L. (2012). New bacilli from shallow hydrothermal vents of Panarea Island (Italy) and their biotechnological potential. J. Appl. Microbiol. 112, 1102–1112. doi: 10.1111/j.1365-2672.2012.05272.x
Handley, K. M., Boothman, C., Mills, R. A., Pancost, R. D., and Lloyd, J. R. (2010). Functional diversity of bacteria in a ferruginous hydrothermal sediment. ISME J. 4, 1193–1205. doi: 10.1038/ismej.2010.38
Hawkes, J. A., Connelly, D. P., Gledhill, M., and Achterberg, E. P. (2013). The stabilisation and transportation of dissolved iron from high temperature hydrothermal vent systems. Earth Planet. Sci. Lett. 375, 280–290. doi: 10.1016/j.epsl.2013.05.047
Hawkes, J. A., Connelly, D. P., Rijkenberg, M. J. A., and Achterberg, E. P. (2014). The importance of shallow hydrothermal island arc systems in ocean biogeochemistry. Geophys. Res. Lett. 41, 942–947. doi: 10.1002/2013GL058817
Holden, J., Breier, J., Rogers, K., Schulte, M., and Toner, B. (2012). Biogeochemical processes at hydrothermal vents: microbes and minerals, bioenergetics, and carbon fluxes. Oceanography 25, 196–208. doi: 10.5670/oceanog.2012.18
Hoshino, T., Kuratomi, T., Morono, Y., Hori, T., Oiwane, H., Kiyokawa, S., et al. (2016). Ecophysiology of Zetaproteobacteria associated with shallow hydrothermal iron-oxyhydroxide deposits in Nagahama Bay of Satsuma Iwo-Jima, Japan. Front. Microbiol. 6:1554. doi: 10.3389/fmicb.2015.01554
Inagaki, F., Takai, K., Kobayashi, H., Nealson, K. H., and Horikoshi, K. (2003). Sulfurimonas autrotrophica gen. nov., sp. nov., a novel sulfur-oxidizing ε-proteobacterium isolated from hydrothermal sediments in the Mid-Okinawa Trough. Int. J. Syst. Evol. Microbiol. 53, 1801–1805. doi: 10.1099/ijs.0.02682-0
Ionescu, D., Heim, C., Polerecky, L., Ramette, A., Haeusler, S., Bizic-Ionescu, M., et al. (2015). Diversity of iron oxidizing and reducing bacteria in flow reactors in the Aspo Hard Rock Laboratory. Geomicrobiol. J. 32, 207–220. doi: 10.1080/01490451.2014.884196
Jørgensen, B. B., and Boetius, A. (2007). Feast and famine — microbial life in the deep-sea bed. Nat. Rev. Microbiol. 5, 770–781. doi: 10.1038/nrmicro1745
Joseph, E. P., Fournier, N., Lindsay, J. M., and Fischer, T. P. (2011). Gas and water geochemistry of geothermal systems in Dominica, Lesser Antilles island arc. J. Volcanol. Geotherm. Res. 206, 1–14. doi: 10.1016/j.jvolgeores.2011.06.007
Karlen, D. J., Price, R. E., Pichler, T., and Garey, J. R. (2010). Changes in benthic macrofauna associated with a shallow-water hydrothermal vent gradient in Papua New Guinea. Pacific Sci. 64, 391–404. doi: 10.2984/64.3.391
Kato, S., Nakamura, K., Toki, T., Ishibashi, J., Tsunogai, U., and Moyer, C. L. (2012). Iron-based microbial ecosystem on and below the seafloor: a case study of hydrothermal fields of the Southern Mariana Trough. Front. Microbiol. 3:89. doi: 10.3389/fmicb.2012.00089
Kleint, C., Kuzmanovski, S., Powell, Z., Bühring, S. I., Sander, S. G., and Koschinsky, A. (2015). Organic Cu-complexation at the shallow marine hydrothermal vent fields off the coast of Milos (Greece), Dominica (Lesser Antilles) and the Bay of Plenty (New Zealand). Mar. Chem. 173, 244–252. doi: 10.1016/j.marchem.2014.10.012
Kleint, C., Pichler, T., and Koschinsky, A. (2017). Geochemical characteristics, speciation and size-fractionation of iron (Fe) in two marine shallow-water hydrothermal systems, Dominica, Lesser Antilles. Chem. Geol. 454, 44–53. doi: 10.1016/j.chemgeo.2017.02.021
Klindworth, A., Pruesse, E., Schweer, T., Peplies, J., Quast, C., Horn, M., et al. (2013). Evaluation of general 16S ribosomal RNA gene PCR primers for classical and next-generation sequencing-based diversity studies. Nucleic Acids Res. 41, 1–11. doi: 10.1093/nar/gks808
Lebedeva, E. V., Off, S., Zumbrägel, S., Kruse, M., Shagzhina, A., Lücker, S., et al. (2011). Isolation and characterization of a moderately thermophilic nitrite-oxidizing bacterium from a geothermal spring. FEMS Microbiol. Ecol. 75, 195–204. doi: 10.1111/j.1574-6941.2010.01006.x
Lentini, V., Gugliandolo, C., Bunk, B., Overmann, J., and Maugeri, T. L. (2014). Diversity of prokaryotic community at a shallow marine hydrothermal site elucidated by Illumina sequencing technology. Curr. Microbiol. 69, 457–466. doi: 10.1007/s00284-014-0609-5
Lindsay, J. M., Trumbull, R. B., and Siebel, W. (2005). Geochemistry and petrogenesis of late pleistocene to recent volcanism in Southern Dominica, Lesser Antilles. J. Volcanol. Geotherm. Res. 148, 253–294. doi: 10.1016/j.jvolgeores.2005.04.018
Liu, Y. C., Young, L. S., Lin, S. Y., Hameed, A., Hsu, Y. H., Lai, W. A., et al. (2013). Pseudomonas guguanensis sp. nov., a gammaproteobacterium isolated from a hot spring. Int. J. Syst. Evol. Microbiol. 63(Pt. 12), 4591–4598. doi: 10.1099/ijs.0.047712-0.
Maugeri, T. L., Lentini, V., Gugliandolo, C., Cousin, S., and Stackebrandt, E. (2010). Microbial diversity at a hot, shallow-sea hydrothermal vent in the southern Tyrrhenian Sea (Italy). Geomicrobiol. J. 27, 380–390. doi: 10.1080/01490450903451518
McAllister, S. M., Davis, R. E., McBeth, J. M., Tebo, B. M., Emerson, D., and Moyer, C. L. (2011). Biodiversity and emerging biogeography of the neutrophilic iron-oxidizing Zetaproteobacteria. Appl. Environ. Microbiol. 77, 5445–5457. doi: 10.1128/AEM.00533-11
McBeth, J. M., Little, B. J., Ray, R. I., Farrar, K. M., and Emerson, D. (2011). Neutrophilic iron-oxidizing “Zetaproteobacteria” and mild steel corrosion in nearshore marine environments. Appl. Environ. Microbiol. 77, 1405–1412. doi: 10.1128/AEM.02095-10
McCarthy, K. T., Pichler, T., and Price, R. E. (2005). Geochemistry of Champagne Hot Springs shallow hydrothermal vent field and associated sediments, Dominica, Lesser Antilles. Chem. Geol. 224, 55–68. doi: 10.1016/j.chemgeo.2005.07.014
Mihailov, V. V., Romanenko, L. A., and Ivanova, E. P. (2006). “The genus Alteromonas and related Proteobacteria,” in The Prokaryotes A Handbook on the Biology of Bacteria, 3rd Edn., eds M. Dworkin, S. Falkow, E. Rosenberg, K.-H. Schleifer, and E. Stackebrandt (New York, NY: Springer), 597–645.
Miranda, P. J., McLain, N. K., Hatzenpichler, R., Orphan, V. J., and Dillon, J. G. (2016). Characterization of chemosynthetic microbial mats associated with intertidal hydrothermal sulfur vents in white point, San Pedro, CA, USA. Front. Microbiol. 7:1163. doi: 10.3389/fmicb.2016.01163
Miroshnichenko, M. L., Kolganova, T. V., Spring, S., Chernyh, N., and Bonch-Osmolovskaya, E. A. (2010). Caldithrix palaeochoryensis sp. nov., a thermophilic, anaerobic, chemo-organotrophic bacterium from a geothermally heated sediment, and emended description of the genus Caldithrix. Int. J. Syst. Evol. Microbiol. 60, 2120–2123. doi: 10.1099/ijs.0.016667-0
Miroshnichenko, M. L., Kostrikina, N. A., Chernyh, N. A., Pimenov, N. V., Tourova, T. P., Antipov, A. N., et al. (2003). Caldithrix abyssi gen. nov., sp. nov., a nitrate-reducing, thermophilic, anaerobic bacterium isolated from a Mid-Atlantic Ridge hydrothermal vent, represents a novel bacterial lineage. Int. J. Syst. Evol. Microbiol. 53, 323–329. doi: 10.1099/ijs.0.02390-0
Mohandass, C., Rajasabapathy, R., Ravindran, C., Colaco, A., Santos, R. S., and Meena, R. M. (2012). Bacterial diversity and their adaptations in the shallow water hydrothermal vent at D. Joao de Castro Seamount (DJCS), Azores, Portugal. Cah. Biol. Mar. 53, 65–76. doi: 10.1371/journal.pone.0032859
Nunoura, T., Hirai, M., Miyazaki, M., Kazama, H., Makita, H., Hirayama, H., et al. (2013). Isolation and characterization of a thermophilic, obligately anaerobic and heterotrophic marine Chloroflexi bacterium from a Chloroflexi-dominated microbial community associated with a japanese shallow hydrothermal system, and proposal for Thermomarinilin. Microb. Environ. 28, 228–235. doi: 10.1264/jsme2.ME12193
Oksanen, J., Blanchet, F. G., Roeland, K., Legendre, P., Minchin, P. R., O'Hara, R. B., et al. (2015). Vegan: Community Ecology Package. R package version 2.3-0. Available online at: http://CRAN.R-project.org/package=vegan
Pan, J., Sun, C., Zhang, X. Q., Huo, Y. Y., Zhu, X. F., and Wu, M. (2014). Paracoccus sediminis sp. nov., isolated from Pacific Ocean marine sediment. Int. J. Syst. Evol. Microbiol. 64, 2512–2516. doi: 10.1099/ijs.0.051318-0
Peña, A., Busquets, A., Gomila, M., Mayol, J., Bosch, R., Nogales, B., et al. (2013). Draft genome of Pseudomonas stutzeri strain NF13, a nitrogen fixer isolated from the Galapagos rift hydrothermal vent. Genome Announc. 1, 2–3. doi: 10.1128/genomeA.00113-13
Pichler, T., and Humphrey, J. D. (2001). Formation of dolomite in recent island-arc sediments due to gas-seawater-sediment interaction. J. Sediment. Res. 71, 395–399. doi: 10.1306/110101711067
Pichler, T., and Veizer, J. (1999). Precipitation of Fe(III) oxyhydroxide deposits from shallow-water hydrothermal fluids in Tutum Bay, Ambitle Island, Papua New Guinea. Chem. Geol. 162, 15–31. doi: 10.1016/S0009-2541(99)00068-6
Pichler, T., and Veizer, J. (2004). The precipitation of aragonite from shallow-water hydrothermal fluids in a coral reef, Tutum Bay, Ambitle Island, Papua New Guinea. Chem. Geol. 207, 31–45. doi: 10.1016/j.chemgeo.2004.02.002
Pichler, T., Veizer, J., and Hall, G. E. M. (2004). The chemical composition of shallow-water hydrothermal fluids in Tutum Bay, Ambitle Island, Papua New Guinea and their effect on ambient seawater. Mar. Chem. 64, 229–252. doi: 10.1016/S0304-4203(98)00076-0
Podosokorskaya, O. A., Bonch-Osmolovskaya, E. A., Novikov, A. A., Kolganova, T. V., and Kublanov, I. V. (2013). Ornatilinea apprima gen. nov., sp. nov., a cellulolytic representative of the class Anaerolineae. Int. J. Syst. Evol. Microbiol. 63, 86–92. doi: 10.1099/ijs.0.041012-0
Pop Ristova, P., Bienhold, C., Rossel, P. E., Wenzhöfer, F., and Boetius, A. (2017a). Temporal and spatial variations of bacterial and faunal communities associated with deep-sea wood falls. PLoS ONE 12:e0169906. doi: 10.1371/journal.pone.0169906
Pop Ristova, P., Pichler, T., and Bühring, S. I. (2017b). Porewater Geochemistry of Two Shallow-Water Hydrothermal Vents off Dominica Island (Lesser Antilles). PANGAEA. Available online at: https://doi.pangaea.de/10.1594/PANGAEA.882614
Pop Ristova, P., Wenzhöfer, F., Ramette, A., Felden, J., and Boetius, A. (2015). Spatial scales of bacterial community diversity at cold seeps (Eastern Mediterranean Sea). ISME J. 9, 1306–1318. doi: 10.1038/ismej.2014.217
Pop Ristova, P., Wenzhöfer, F., Ramette, A., Zabel, M., Fischer, D., Kasten, S., et al. (2012). Bacterial diversity and biogeochemistry of different chemosynthetic habitats of the REGAB cold seep (West African margin, 3160 m water depth). Biogeosciences 9, 5031–5048. doi: 10.5194/bg-9-5031-2012
Price, R. E., Amend, J. P., and Pichler, T. (2007). Enhanced geochemical gradients in a marine shallow-water hydrothermal system: unusual arsenic speciation in horizontal and vertical pore water profiles. Appl. Geochem. 22, 2595–2605. doi: 10.1016/j.apgeochem.2007.06.010
Price, R. E., and Giovannelli, D. (2017). “A review of the geochemistry and microbiology of marine shallow-water hydrothermal vents,” in Reference Module in Earth Systems and Environmental Sciences (Elsevier), 1–29. doi: 10.1016/B978-0-12-409548-9.09523-3
Price, R. E., Lesniewski, R., Nitzsche, K. S., Meyerdierks, A., Saltikov, C., Pichler, T., et al. (2013a). Archaeal and bacterial diversity in an arsenic-rich shallow-sea hydrothermal system undergoing phase separation. Front. Microbiol. 4:158. doi: 10.3389/fmicb.2013.00158
Price, R. E., Savov, I., Planer-Friedrich, B., Bühring, S. I., Amend, J., and Pichler, T. (2013b). Processes influencing extreme arsenic enrichment in shallow-sea hydrothermal fluids of milos island, greece. Chem. Geol. 348, 15–26. doi: 10.1016/j.chemgeo.2012.06.007
Qin, S., Li, W.-J., Dastager, S. G., and Hozzein, W. N. (2015). Actinobacteria in special and extreme habitats: diversity, function roles and environmental adaptations. Front. Microbiol. 7:1415. doi: 10.3389/fmicb.2016.01415
Quast, C., Pruesse, E., Yilmaz, P., Gerken, J., Schweer, T., Yarza, P., et al. (2013). The SILVA ribosomal RNA gene database project: improved data processing and web-based tools. Nucleic Acids Res. 41, 590–596. doi: 10.1093/nar/gks1219
Rajasabapathy, R., Mohandass, C., Colaco, A., Dastager, S. G., Santos, R. S., and Meena, R. M. (2014). Culturable bacterial phylogeny from a shallow water hydrothermal vent of Espalamaca (Faial, Azores) reveals a variety of novel taxa. Curr. Sci. 106, 58–69.
Ramette, A. (2007). Multivariate analyses in microbial ecology. FEMS Microbiol. Ecol. 62, 142–160. doi: 10.1111/j.1574-6941.2007.00375.x
Ramette, A. (2009). Quantitative community fingerprinting methods for estimating the abundance of operational taxonomic units in natural microbial communities. Appl. Environ. Microbiol. 75, 2495–2505. doi: 10.1128/AEM.02409-08
Ramirez-Llodra, E., Shank, T., and German, C. (2007). Biodiversity and biogeography of hydrothermal vent species: thirty years of discovery and investigations. Oceanography 20, 30–41. doi: 10.5670/oceanog.2007.78
Rathgeber, C., Yurkov, N., Stackebrandt, E., Schumann, P., Humphrey, E. J., Beatty, T., et al. (2006). Metalloid reducing bacteria isolated from deep ocean hydrothermal vents of the Juan de Fuca Ridge, Pseudoalteromonas telluritireducens sp. nov. and Pseudoalteromonas spiralis sp. nov. Curr. Microbiol. 53, 449–456. doi: 10.1007/s00284-006-0320-2
Robinson, C., Ziebis, W., Muller, S., Eichstaedt, K., Dando, P., Linke, P., et al. (1997). “In situ investigations of shallow water hydrothermal vent systems, Palaeochori Bay, Milos, Aegean Sea,” in Fourth Underwater Science Symposium (Newcastle upon Tyne), 85–100.
Rothschild, L. J., and Mancinelli, R. L. (2001). Life in extreme environments. Nature 409, 1092–1101. doi: 10.1038/35059215
Rougeaux, H., Pichon, R., Kervarec, N., Raguenes, H. C., and Guezennec, J. G. (1996). Novel bacterial exopolysaccharides from deep-sea hydrothermal vents. Carb Polym. 31, 237–242.
Ruff, E. S., Biddle, J. F., Teske, A. P., Knittel, K., Boetius, A., and Ramette, A. (2015). Global dispersion and local diversification of the methane seep microbiome. Proc. Natl. Acad. Sci. U.S.A. 112, 4015–4020. doi: 10.1073/pnas.1421865112
Seeberg-Elverfeldt, J., Schlüter, M., Feseker, T., and Koelling, M. (2005). Rhizon sampling of porewaters near the sediment-water interface of aquatic systems. Limnol. Oceanogr. 3, 361–371. doi: 10.4319/lom.2005.3.361
Sekiguchi, Y., Yamada, T., Hanada, S., Ohashi, A., Harada, H., and Kamagata, Y. (2003). Anaerolinea thermophila gen. nov., sp. nov. and Caldilinea aerophila gen. nov., sp. nov., novel filamentous thermophiles that represent a previously uncultured lineage of the domain bacteria at the subphylum level. Int. J. Syst. Evol. Microbiol. 53, 1843–1851. doi: 10.1099/ijs.0.02699-0
Sievert, S. M., Brinkhoff, T., Muyzer, G., Ziebis, W., and Kuever, J. (1999). Spatial heterogeneity of bacterial populations along an environmental gradient at a shallow submarine hydrothermal vent near Milos Island (Greece). Appl. Environ. Microbiol. 65, 3834–3842.
Sievert, S. M., Kuever, J., and Muyzer, G. (2000). Identification of 16S Ribosomal DNA-defined bacterial populations at a shallow submarine hydrothermal vent near Milos Island (Greece). Appl. Environ. Microbiol. 66, 3102–3109. doi: 10.1128/AEM.66.7.3102-3109.2000
Sievert, S. M., and Vetriani, C. (2012). Chemoautotrophy at deep-sea vents. Oceanography 25, 218–233. doi: 10.5670/oceanog.2012.21
Slobodkina, G. B., Kolganova, T. V., Kopitsyn, D. S., Viryasov, M. B., Bonch-Osmolovskaya, E. A., and Slobodkin, A. I. (2016). Dissulfurirhabdus thermomarina gen. nov., sp. nov., a thermophilic, autotrophic, sulfite-reducing and disproportionating deltaproteobacterium isolated from a shallow-sea hydrothermal vent. Int. J. Syst. Evol. Microbiol. 66, 2515–2519. doi: 10.1099/ijsem.0.001083
Suzuki, D., Li, Z., Cui, X., Zhang, C. h., and Katayama, A. (2014). Reclassification of Desulfobacterium anilini as Desulfatiglans anilini comb. nov. within Desulfatiglans gen. nov., and description of a 4-chlorophenol-degrading sulfate-reducing bacterium, Desulfatiglans parachlorophenolica sp. nov. Int. J. Syst. Evol. Microbiol. 64, 3081–3086. doi: 10.1099/ijs.0.064360-0
Takai, K., Kobayashi, H., Nealson, K. H., and Horikoshi, K. (2003). Deferribacter desulfuricans sp. nov., a novel sulfur-, nitrate- and arsenate-reducing thermophile isolated from a deep-sea hydrothermal vent. Int. J. Syst. Evol. Microbiol. 53, 839–846. doi: 10.1099/ijs.0.02479-0
Takaki, Y., Shimamura, S., Nakagawa, S., Fukuhara, Y., Horikawa, H., Ankai, A., et al. (2010). Bacterial lifestyle in a deep-sea hydrothermal vent chimney revealed by the genome sequence of the thermophilic bacterium Deferribacter desulfuricans SSM1. DNA Res. 17, 123–137. doi: 10.1093/dnares/dsq005
Tarasov, V. G., Gebruk, A. V., Mironov, A. N., and Moskalev, L. I. (2005). Deep-sea and shallow-water hydrothermal vent communities: two different phenomena? Chem. Geol. 224, 5–39. doi: 10.1016/j.chemgeo.2005.07.021
Teske, A., Callaghan, A. V., and LaRowe, D. E. (2014). Biosphere frontiers of subsurface life in the sedimented hydrothermal system of Guaymas Basin. Front. Microbiol. 5:362. doi: 10.3389/fmicb.2014.00362
Teske, A., and Reysenbach, A. L. (2015). Editorial: hydrothermal microbial ecosystems. Front. Microbiol. 6:884. doi: 10.3389/fmicb.2015.00884
Thiel, V., Wood, J. M., Olsen, M. T., Tank, M., Klatt, C. G., Ward, D. M., et al. (2016). The dark side of the mushroom spring microbial mat: life in the shadow of chlorophototrophs. I. Microbial diversity based on 16S rRNA gene amplicons and metagenomic sequencing. Front. Microbiol. 7:919. doi: 10.3389/fmicb.2016.00919
Tivey, M. (2007). Generation of seafloor hydrothermal vent fluids and associated mineral deposits. Oceanography 20, 50–65. doi: 10.5670/oceanog.2007.80
Van Dover, C. L. (2000). The Ecology of Deep-Sea Hydrothermal Vents. Princeton, NJ: Princeton University Press.
Vetriani, C., Chew, Y. S., Miller, S. M., Coombs, J., Lutz, R. A., and Barkay, T. (2005). Mercury adaptation among bacteria from a deep-sea hydrothermal vent. Appl. Environ. Microbiol. 71, 220–226. doi: 10.1128/AEM.71.1.220-226.2005
Vishnivetskaya, T., Kathariou, S., and Tiedje, J. M. (2009). The Exiguobacterium genus: biodiversity and biogeography. Extremophiles 13, 541–555. doi: 10.1007/s00792-009-0243-5
Wang, C. L., Ozuana, S. C., Clark, D. S., and Keasling, J. D. (2002). A deep-sea hydrothermal vent isolate, Pseudomonas aeruginosa CW961, requires thiosulfate for Cd2+ tolerance and precipitation. Biotechology Lett. 24, 637–641. doi: 10.1023/A:1015043324584
Wang, L., Cheung, M. K., Kwan, H. S., Hwang, J. S., and Wong, C. K. (2015). Microbial diversity in shallow-water hydrothermal sediments of Kueishan Island, Taiwan as revealed by pyrosequencing. J. Basic Microbiol. 55, 1–11. doi: 10.1002/jobm.201400811
Wei, Y., Cao, J., Fang, J., Kato, C., and Cui, W. (2017). First complete genome sequence of Marinilactibacillus piezotolerans strain 15R, Marine Lactobacillus isolated from coal-bearing sediment 2.0 kilometers below the seafloor, determined by PacBio single-molecule real-time technology. Genome Announc. 5:e01625-16. doi: 10.1128/genomeA.01625-16
Wenzhöfer, F., Holby, O., Glud, R. N., Nielsen, H. K., and Gundersen, J. K. (2000). In situ microsensor studies of a shallow water hydrothermal vent at Milos, Greece. Mar. Chem. 69, 43–52. doi: 10.1016/S0304-4203(99)00091-2
Yanagawa, K., Morono, Y., De Beer, D., Haeckel, M., Sunamura, M., Futagami, T., et al. (2012). Metabolically active microbial communities in marine sediment under high-CO2 and low-pH extremes. ISME J. 7, 555–567. doi: 10.1038/ismej.2012.124
Yoon, S. H., Ha, S. M., Kwon, S., Lim, J., Kim, Y., Seo, H., et al. (2017). Introducing EzBioCloud: A taxonomically united database of 16S rRNA and whole genome assemblies. Int. J. Syst. Evol. Microbiol. 67, 1613–1617. doi: 10.1099/ijsem.0.001755
Yücel, M., Sievert, S. M., Vetriani, C., Foustoukos, D. I., Giovannelli, D., and Le Bris, N. (2013). Eco-geochemical dynamics of a shallow-water hydrothermal vent system at Milos Island, Aegean Sea (Eastern Mediterranean). Chem. Geol. 356, 11–20. doi: 10.1016/j.chemgeo.2013.07.020
Zhang, D.-C., Liu, Y.-X., Huo, Y.-Y., Xu, X.-W., and Li, X.-Z. (2015a). Draft genome sequence of thermophilic Exiguobacterium sp. Strain JLM-2, isolated from deep-sea ferromanganese nodules. Genome Announc. 3:e00794-15. doi: 10.1016/j.ajodo.2005.02.022
Zhang, J., Sun, Q. L., Zeng, Z. G., Chen, S., and Sun, L. (2015b). Microbial diversity in the deep-sea sediments of Iheya North and Iheya Ridge, Okinawa Trough. Microbiol. Res. 177, 43–52. doi: 10.1016/j.micres.2015.05.006
Zhang, L., Pan, Y., Wang, K., Zhang, X., Zhang, C., Zhang, S., et al. (2015c). Pseudomonas zhaodongensis sp. nov., isolated from saline and alkaline soils. Int. J. Syst. Evol. Microbiol. 65, 1022–1030. doi: 10.1099/ijs.0.000057
Keywords: shallow-water, hydrothermal systems, bacterial diversity, geochemistry, Dominica
Citation: Pop Ristova P, Pichler T, Friedrich MW and Bühring SI (2017) Bacterial Diversity and Biogeochemistry of Two Marine Shallow-Water Hydrothermal Systems off Dominica (Lesser Antilles). Front. Microbiol. 8:2400. doi: 10.3389/fmicb.2017.02400
Received: 04 June 2017; Accepted: 20 November 2017;
Published: 04 December 2017.
Edited by:
Jesse G. Dillon, California State University, Long Beach, United StatesReviewed by:
Donato Giovannelli, Earth-Life Science Institute, JapanCopyright © 2017 Pop Ristova, Pichler, Friedrich and Bühring. This is an open-access article distributed under the terms of the Creative Commons Attribution License (CC BY). The use, distribution or reproduction in other forums is permitted, provided the original author(s) or licensor are credited and that the original publication in this journal is cited, in accordance with accepted academic practice. No use, distribution or reproduction is permitted which does not comply with these terms.
*Correspondence: Petra Pop Ristova, cHJpc3RvdmFAbWFydW0uZGU=
Disclaimer: All claims expressed in this article are solely those of the authors and do not necessarily represent those of their affiliated organizations, or those of the publisher, the editors and the reviewers. Any product that may be evaluated in this article or claim that may be made by its manufacturer is not guaranteed or endorsed by the publisher.
Research integrity at Frontiers
Learn more about the work of our research integrity team to safeguard the quality of each article we publish.